An Update on the Molecular Mechanism of the Vertebrate Isthmic Organizer Development in the Context of the Neuromeric Model
- 1Departamento de Biología Celular, Facultad de Ciencias, Universidad de Extremadura, Badajoz, Spain
- 2Instituto de Neurociencias de Alicante, Universidad Miguel Hernández-CSIC, Alicante, Spain
A crucial event during the development of the central nervous system (CNS) is the early subdivision of the neural tube along its anterior-to-posterior axis to form neuromeres, morphogenetic units separated by transversal constrictions and programed for particular genetic cascades. The narrower portions observed in the developing neural tube are responsible for relevant cellular and molecular processes, such as clonal restrictions, expression of specific regulatory genes, and differential fate specification, as well as inductive activities. In this developmental context, the gradual formation of the midbrain-hindbrain (MH) constriction has been an excellent model to study the specification of two major subdivisions of the CNS containing the mesencephalic and isthmo-cerebellar primordia. This MH boundary is coincident with the common Otx2-(midbrain)/Gbx2-(hindbrain) expressing border. The early interactions between these two pre-specified areas confer positional identities and induce the generation of specific diffusible morphogenes at this interface, in particular FGF8 and WNT1. These signaling pathways are responsible for the gradual histogenetic specifications and cellular identity acquisitions with in the MH domain. This review is focused on the cellular and molecular mechanisms involved in the specification of the midbrain/hindbrain territory and the formation of the isthmic organizer. Emphasis will be placed on the chick/quail chimeric experiments leading to the acquisition of the first fate mapping and experimental data to, in this way, better understand pioneering morphological studies and innovative gain/loss-of-function analysis.
Early Development of the Central Nervous System: The Neuromeric Theory
The complex central nervous system (CNS) of a vertebrate is a result of both ontogenic and evolutionary events. The first morphological evidence of the organization of the developing neural tube was reported by Orr (1887) introducing the term neuromeres as morphogenetic units arranged along its anterior-to-posterior axis and separated by transversal constrictions. In the chick embryos, classical anatomical studies showed that the incipient neuromeric units grouped in three primordial vesicles at the 10–12 somites stage (Vaage, 1969), representing the anlagen of the forebrain (secondary prosencephalon and diencephalon), midbrain (M; mesencephalon, mes), and hindbrain (H; classically divided in metencephalon, met, and myelencephalon, although nowadays these terms are considered obsolete). These main divisions will be further subdivided in the aforementioned neuromeres to produce a common complexity in all analyzed vertebrates according to the neuromeric model proposed by Luis Puelles’ group (Figure 1; Puelles, 2018). It is worth mentioning that the secondary prosencephalon contains the hypothalamo-telencephalic prosomeres (hp1 and hp2), whereas the diencephalon presents three diencephalic prosomeres (dp1, dp2, and dp3). In the same manner, the midbrain is divided into two mesomeres (mp1 and mp2) and the hindbrain is alienated into 13 rhombomeres clustered in: prepontine cryptorhombomeres (istmus, r0, and rostral and caudal parts of r1, r1r and r1c), pontine overt rhombomeres (r2-r4), retropontine overt rhombomeres (r5-r6), and medullary cryptorhombomeres (r7-r11; Figure 1; Puelles, 2018).
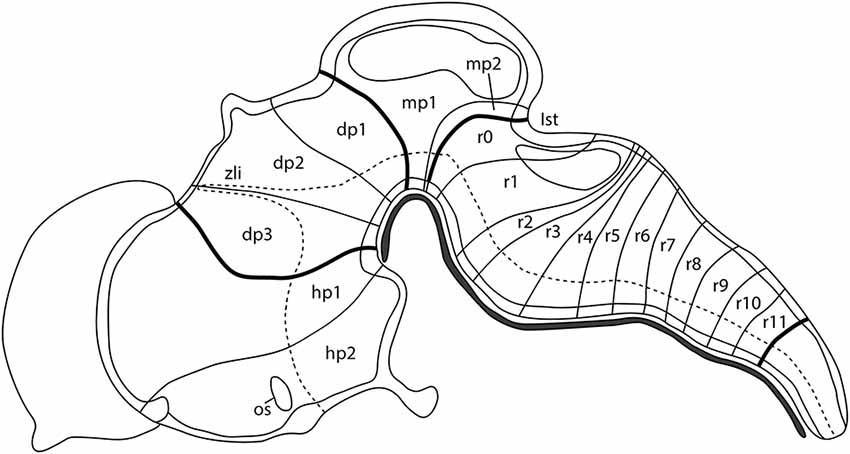
Figure 1. Schematic representation showing the neuromeric units of the developing mouse brain along its anterior-to-posterior axis: two hypothalamo-telencephalic prosomeres (hp1, hp2), three diencephalic prosomeres (dp1, dp2, dp3), two midbrain prosomeres (mp1, mp2), and 13 hindbrain rhombomeres (r0-r11). The dotted line defines the limit between the alar and basal plate of the developing neural tube. Ist, isthmus; os, optic stalk; zli, zona limitans intrathalamica. Adapted from Puelles (2018).
To obtain this conserved arrangement along the longitudinal axis of the brain, the development of the vertebrate neural tube is conducted by multi-step mechanisms, involving inductive and morphogenetic events. Long- and short-range diffusible molecules, morphogens, govern cell survival and fate specification through the control of dynamic spatial-temporal expression patterns of key transcription factors, which provides positional identities. Innovative genoarchitectural studies and fate mapping studies established correlations between how this intricate genetic network modulates the specification of neuroepithelial territories in vertebrate CNS (Puelles and Rubenstein, 2003; Puelles and Ferran, 2012; Puelles, 2018). According to the expression patterns of significant regulatory genes, such as Otx2, Shh, Nk2.2, and Pax7, and the distribution of dopaminergic and serotonergic neurons, among other molecular and cellular characteristics, the above-mentioned neuromeres could be grouped into three broad areas or tagmatic regions: forebrain (sum of the secondary prosencephalon, diencephalon, and midbrain), hindbrain, and spinal cord (Puelles, 2018). Our intention in this review is to focus on summarizing and discussing evidence concerning classical morphological and genetic views in the formation of the interface between the rostralmost tagma (forebrain) and the intermediate one (hindbrain), i.e., the interface between the caudal midbrain and the rostral hindbrain, the so-named midbrain-hindbrain (MH) boundary [This border is also referred to in classical studies as mesencephalic-metencephalic (mes-met) constriction]. The MH boundary, defined by the juxtaposition of the Otx2-expressing (rostral) and the Gbx2-expressing (caudal) domains in which the double decreasing gradients of Engrailed and Pax2 expressions are centered, is associated with complex regulatory activities of a secondary organizer center, the isthmic organizer (IsO), mediated mainly by FGF8 and WNT1, two relevant morphogenes involved in the specification of the MH domain, including the meso-isthmo-cerebellar territory.
The Mesencephalic/Metencephalic Constriction
One of the main goals in traditional embryology was to establish a model system to fate map the anterior-to-posterior arranged regions of the developing neural tube according to the proposed neuromeric model. The chick/quail chimeric system, introduced by Le Douarin (1969, 1973) has been a very useful approach to identifying cell derivatives from a small grafted portion of tissue. Using immuno-histochemical staining, the grafted cells from a donor quail embryo were easily identified when they were integrated with a host chick embryo. Alvarado-Mallart and Sotelo (1984) carried out innovative work to study the mesencephalic/metencephalic development using this well-designed experimental approach.
Furthermore, early anatomical observations in birds suggested that, at the 10-somite stage (HH10), the cerebellar primordium could be raised from the pro-rhombomere A1 (RhA1), the rostral most neuromere of the developing hind brain at this developmental stage, located therefore just caudal to the so-called mesencephalic/metencephalic (mes/met) constriction. This clearly defined vesicle of the developing neural tube would contain the presumptive territories of r1 and r2 (Vaage, 1969). Alvarado-Mallart’s group provided clear evidence of the location of the cerebellar primordium at this developmental stage (Figure 2A). Surprisingly, chick/quail homotopic grafts of the dorsal half of the mesencephalic vesicle at the stage HH10 showed that, in addition to the optic tectum, the isthmic nuclei and the rostromedial portion of the cerebellum raised from the grafted territory (Martinez and Alvarado-Mallart, 1989). Therefore, the “mesencephalic” vesicle observed at stage HH10 is the apparent midbrain vesicle that also includes the anterior portion of the hindbrain in chick embryos. The contribution of the “mesencephalic” vesicle to the cerebellum was also confirmed by Le Douarin’s group (Hallonet et al., 1990). At stage HH10, the isthmocerebellar primordium is, therefore, located more rostral than previously supposed, on both sides of the “mesencephalic/metencephalic” (“mes/met”) constriction (Figure 2A). Therefore, the final position of the cerebellum in the rostralmost portion of the hindbrain must be determined by important morphogenetic movements as development proceeds. At least in chick embryos, the cerebellum originates from several morphogenetic units (Figure 2; Martinez and Alvarado-Mallart, 1989; Hallonet et al., 1990; Alvarez-Otero et al., 1993; Marín and Puelles, 1995; Millet et al., 1996; reviewed in Alvarado-Mallart, 2000; Hidalgo-Sánchez et al., 2005a; Puelles, 2018).
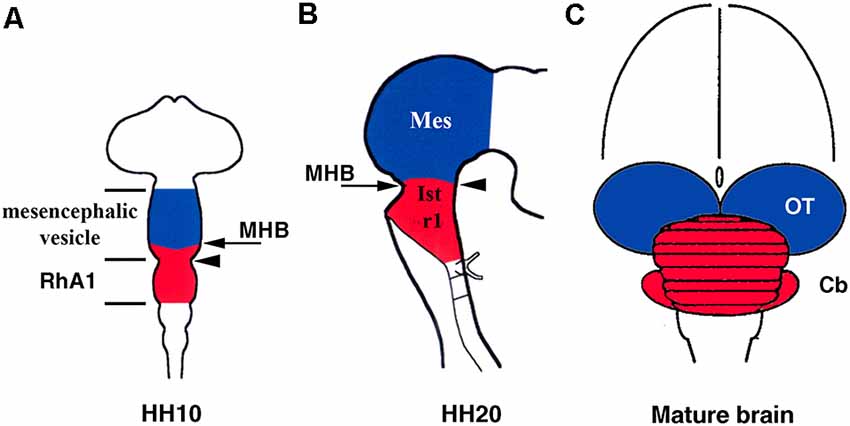
Figure 2. Location of the isthmocerebellar (red) and mesencephalic (blue) anlages in the chick neural tube at stage HH10 (A) and HH20 (B) with respect to the midbrain/hindbrain boundary (MHB; arrows in A,B). The arrowheads in (A) and (B) point to the mesencephalic/metencephalic (mes/met) constriction. Note that at stage HH20, the MH boundary and the mes/met constriction are coincident (B), but not at stage HH10 (A). The cerebellum (red) and mesencephalon (blue) are also shown in the mature brain (C). Cb, cerebellum; Ist, isthmus (r0); Mes, mesencephalon; OT, optic tectum; RhA1, pro-rhombomere A1; r1, rhombomere 1. From Hidalgo-Sánchez et al. (2005a).
Otx2 Confers Anterior Positional Identity in the Developing CNS
Over the last decades, numerous efforts have been made to identify genes controlling programs of fate determination in the progressive subdivision of the neural tube along its anterior-to-posterior axis. The Drosophila orthodenticle (otd) gene and its vertebrate homolog (Otx) are clearly involved in the correct early specification of the anterior part of the developing head (Simeone et al., 1993; Finkelstein and Boncinelli, 1994). In mice, the expression of the Otx2 gene, a homeobox-containing gene, is detected in the epiblast and the embryonic visceral endoderm before the onset of gastrulation and in the anterior neuroectoderm at the end of gastrulation stages (Acampora et al., 2001; Simeone et al., 2002; Kurokawa et al., 2004). A similar Otx2 expression pattern was described in other vertebrate embryos (chick: Bally-Cuif et al., 1995; Figure 3A; Xenopus: Pannese et al., 1995; zebrafish: Mori et al., 1994; Mercier et al., 1995; Kesavan et al., 2017). Otx2−/− mutant mice die early in development and show defects in anterior neuroectoderm specification. As a consequence, all analyzed mutant mice displayed an absence of the rostralmost tagmatic domain: secondary prosencephalon, diencephalon, and midbrain. In some phenotypes, an atypical hindbrain morphology, resembling the spinal cord, or even a deletion rostral to r3 were also observed, probably due to the mixed genetic background of progenitors (Acampora et al., 1995; Matsuo et al., 1995; Ang et al., 1996; Suda et al., 1997; Rhinn et al., 1998). In addition, studies of Otx1/Otx2 mutant mice strongly suggest that Otx share functional similarities, being the dosage of Otx gene responsible for the correct anterior patterning of the developing brain (Acampora et al., 1997, 1998, 1999; Simeone et al., 2002; Kurokawa et al., 2004). In zebrafish, Otx loss-of-function embryos using morpholinos against two of the three zebrafish Otx genes (Mercier et al., 1995) gastrulate normally displaying a reduction of midbrain and an anterior shift of the isthmic tissue, together with an enlarged cerebellum, confirming that Otx is a repressor of cerebellar fate (Foucher et al., 2006). Therefore, Otx genes would govern the development of the secondary prosencephalon, diencephalon, and midbrain through the region-specific expression patterns of homeobox and cell adhesion genes (Rhinn et al., 1999).
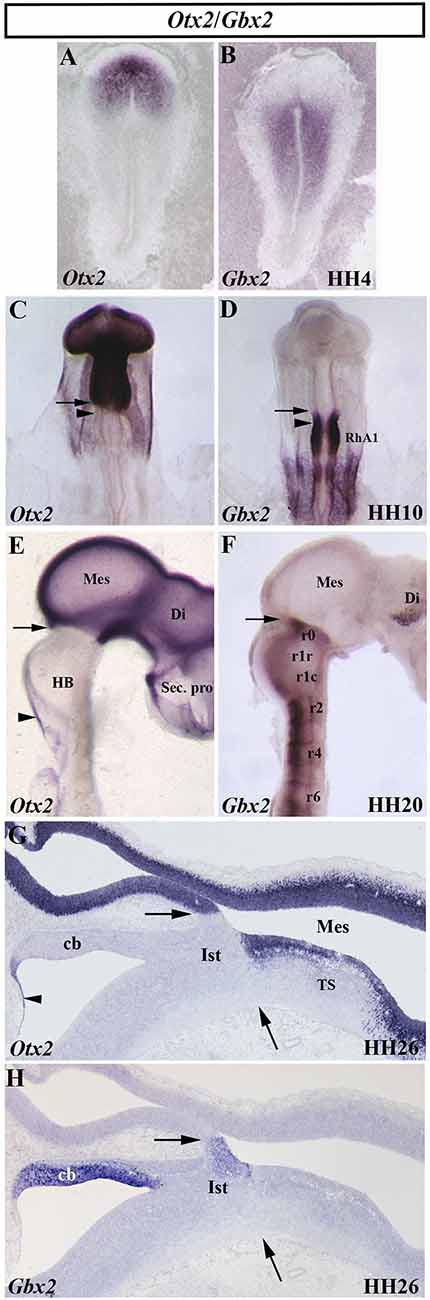
Figure 3. Spatial and temporal distribution of Otx2 and Gbx2 transcripts in the chick developing midbrain-hindbrain (MH) domain at different developmental stages. Single in situ hybridization performed in whole-mount (A–F) and in sagittal sections (G,H). Note that, at all analyzed stages, the Otx2- and Gbx2-expressing domains are contiguous and complementary: stage HH4 (A,B), HH10 (C,D), HH20 (E,F), and HH26 (G,H). The arrows point to the MH boundary (C–H), whereas the arrowheads in (C) and (D) point to the mes/met constriction. Otx2 expression is observed in the mesencephalon (Mes), diencephalon (Di), and secondary presencephalon (Sec. pro; E and G). Otx2 staining is also detected in the choroidal tissue (arrowheads in E,G). Different levels of Gbx2 expression are detected in the rhombomeres (r) of the developing hindbrain (HB) at HH10 and HH20 stages (D,F). Note the presence of Gbx2 expression in the isthmus (Ist) and cerebellum (Cb) at stage HH26 (H). RhA1, pro-rhombomere A1; TS, torus semicircularis. From Hidalgo-Sánchez et al. (1999a, 2005a,b).
In birds, analysis of whole-mount in situ hybridization with the Otx2 probe (Figures 3C,E,G), anti-β-tubulin immunostaining as a marker of the first postmitotic neurons of the caudal midbrain, and chick/quail chimeras with various types of homotopic grafts analyzed after shorter and longer survival times showed that: (1) the caudal limit of Otx2 expression is curved, forming a caudalwards “beak”, which coincides perfectly with the caudal border of the “mesencephalic” territory; (2) a transient Otx2-negative domain was observed in the caudalmost portion of the “mesencephalic” vesicle between stages HH10 and HH17/18, which gradually reduces its size until it disappears completely from stages HH17/18 (Figure 3C); (3) so that, the caudal limit of the Otx2 expression coincides with the constriction observed at stage HH20, but not before (Figure 3E); (4) the Otx2-negative territory detected at stage HH10 in the caudal “mesencephalic” vesicle will give rise to isthmic nuclei and the mediorostral cerebellum (Figure 3G) as shown in long-survival chimeras; and (5) similar embryonic events were also observed in mouse embryos. Rostrocaudal morphogenetic movements take place in the meso-isthmo-cerebellar domain between stages HH10 and HH17/18. Therefore, the constriction observed in the meso-isthmo-cerebellar domain at stages 10 and 20 is not the same entity (Figures 2A,B; Millet et al., 1996; Alvarado-Mallart, 2000; Garda et al., 2001; Hidalgo-Sánchez et al., 2005a).
Gbx2 Is Involved in the Anterior Hindbrain Specification
The gastrulation brain homoebox (Gbx) genes, vertebrate homologs of the unplugged (unp) gene of Drosophila, are also directly involved in the specification of the MH domain (Wassarman et al., 1997; Broccoli et al., 1999; Hidalgo-Sánchez et al., 1999a, b; Li et al., 2002; Kikuta et al., 2003; Rhinn et al., 2003, 2004, 2009; Sunmonu et al., 2009, 2011; Burroughs-Garcia et al., 2011; Su et al., 2014; Tsuda et al., 2019). In the chick, a detailed analysis showed a dynamic expression of the Gbx2 gene, together with the Otx2 gene, at early developmental stages (Figure 3). At stage HH4, Otx2 and Gbx2 expressions are observed in the rostral and caudal portion of the chick embryo, respectively (Figures 3A,B). While the expressing domains of both genes are not contiguous at stage HH8, with a small gap of expression between them, a slight overlap is observed at stage HH9 (Garda et al., 2001). At stage HH10, the Otx2-expressing domain and the Gbx2-expressing domain are contiguous and exclusive (Figures 3C,D). As expected, the Otx2/Gbx2 common limit is far from the “mest/met” constriction a stage HH10. However, it is coincident with the constriction observed at stage HH20 (Figure 2; Millet et al., 1996; Hidalgo-Sánchez et al., 1999a, 2005a; Garda et al., 2001). Although some differences could be observed (Bulfone et al., 1993; Su and Meng, 2002; Rhinn et al., 2003), the posterior border of Otx2 expression and the anterior border of Gbx2 expression are coincident and labeled the MH boundary in all analyzed vertebrates (Figures 3C–H; Bouillet et al., 1995; von Bubnoff et al., 1996; Niss and Leutz, 1998; Hidalgo-Sánchez et al., 1999a; Garda et al., 2001; Tour et al., 2001; Pose-Méndez et al., 2016). Therefore, the role of the Gbx2 gene in the anterior hindbrain specification could be conserved across evolution (Castro et al., 2006). It is worth remarking that Otx2- and Gbx2- expressing domains are firstly established independently of each other, with antagonistic interactions between them as development proceeds to create the Otx2/Gbx2 MH interface (Figure 3; Li and Joyner, 2001).
In mice, Gbx2 is essential for the precise location of the MH boundary (Millet et al., 1999; Sunmonu et al., 2009, 2011). A histological study of Gbx2 mutant embryos showed the absence of a cerebellum, which is formed in normal conditions by distinct vermal, hemispheric, and floccular portions that coincide with the three prepontine units from which it originates (r0, r1r, and r1c; Puelles, 2018). The isthmic nuclei and IV motor nucleus (derived from isthmus; r0), locus coeruleus (r1), and V motor nucleus (r2/3) were absent in mutant homozygotes. Derivatives from the hindbrain caudal to r3 were normal under histological analysis. Thus, the VII motor nucleus (r4/5) displayed normal development in the correct places. Interestingly, Hoxb1 expression, a marker of r4, was observed close to the midbrain caudal end, whereas Krox20, a marker of r3 and r5, was exclusively present in its caudal domain (r5). Therefore, the Gbx2 mutant shows a relevant alteration of derivatives of r1–3, giving rise to a shortened area with abnormal histological characteristics. In the caudal midbrain, the III motor nucleus (midbrain) developed in the correct position. However, the midbrain displays abnormalities in its anterior/posterior patterning, suggesting that the caudal midbrain, inferior colliculi, could suffer a caudal extension to the r3/r4 border according to a caudal shift of the Otx2 domain (Wassarman et al., 1997).
The analysis of a conditional mouse mutant using the Cre/loxP system and lacking Gbx2 expression in r1 after E8.5 showed that these Gbx2-CKO embryos displayed no apparent alterations in motor coordination and developed cerebellum with variable defects only in the vermis, this medial region showing a disrupted foliation pattern (Li et al., 2002). Interestingly, a similar defect of the cerebellar vermis was found in mice that express Otx2 in r1 from the En1 locus (En1+/Otx2; Broccoli et al., 1999). The cerebellar hemispheres of Gbx2-CKO embryos displayed a normal development, the cytoarchitecture of the entire cerebellum being completely normal. Also, the inferior colliculi seemed slightly enlarged, suggesting a posterior shift of the MH boundary location. Of interest, strong ectopic Otx2 expression was found at E14,5 in abnormal cell aggregates near the ventricular layer of the Gbx2-CKO cerebellum, which likely is involved in altering vermis development (Li et al., 2002).
In addition, Gbx2 seems to have a permissive role in r3 specification (Li and Joyner, 2001), ectopic Gbx2 expression in Hoxb1-Gbx2 transgenic mice not being enough to induce r1–3 development in r4 (Li et al., 2005). It seems to be possible that a Gbx dosage (Gbx1 and Gbx2) requirement could be necessary for the correct development of specific rhombomeres in the rostral hindbrain (Waters and Lewandoski, 2006). In this sense, some Otx2 deficient mice also failed to form a normal brain rostral to r3 (Acampora et al., 1995; Matsuo et al., 1995; Ang et al., 1996). Besides, Gbx2 activity could confine Otx2 expression by binding to its FM enhancer (Inoue et al., 2012). These findings strongly suggest mutual Otx2/Gbx2 interactions for correct MH domain development by means of the establishment of the precise Otx2/Gbx2 common border and its consequence inductive events (see below).
In zebrafish, gbx genes play a redundant role in morphogenesis and differentiation of the cerebellum (Kikuta et al., 2003; Rhinn et al., 2003, 2004, 2009; Su et al., 2014). gbx2 is very relevant in MH specification in zebrafish embryogenesis in a dose-dependent manner. Injections of a low dose of gbx2 mRNA cause exclusively a less evident MH constriction, with a normal anterior brain, while injections of high doses provoked repression of regional markers of the secondary prosencephalon, diencephalon, and midbrain and a strong disruption of these brain areas (Nakayama et al., 2013). Also, ectopic gbx2 expression by mRNA injection in other works confirmed the loss of these three areas of the zebrafish brain and caused a severely altered rostral hindbrain, isthmus, and cerebellum (Kikuta et al., 2003), with an increased cell death in r2/3 and r5 and disorganized nerve V neurons (r2/3; Burroughs-Garcia et al., 2011). Similar results were obtained in Xenopus with overexpression of Xgbx2a or Xgbx2b genes (Tour et al., 2002a). A shortening of the anterior hindbrain was also detected in zebrafish gbx1-; gbx2- double mutants, produced by a progressive loss of the genetic program leading to MH specification (Su et al., 2014), with a phenotype similar to or less severe than that observed in mutant mice (Wassarman et al., 1997). In summary, the establishment of the Otx2/Gbx common boundary is mediated by mutual inhibitory interactions between these two genes at early developmental stages in all analyzed vertebrates. Concerning the molecular evolution of this regulatory mechanism, the duplication-degeneration complementation (DDC) model, based on complementary degenerative mutations in a pair of duplicated genes, was used to elucidate how the mechanism diverged between tetrapod and teleost for vertebrate Gbx genes (Islam et al., 2006).
The Meso-Isthmo-Cerebellar Region Expresses High Levels of En Genes
The Drosophila engrailed gene is essential for accurate embryonic segmentation and neurogenesis in the developing insects, its conserved sequence and biochemical function being identified in a high number of organisms throughout the animal kingdom. The members of the homeobox-containing gene En family (En1 and En2), homologs to the Drosophila engrailed gene (Joyner et al., 1985; Joyner and Martin, 1987), is the first gene whose expression pattern was described in the developing MH domain of all analyzed vertebrate embryos, both genes showing a similar expression pattern (Davidson et al., 1988; Davis and Joyner, 1988; Gardner et al., 1988; Patel et al., 1989; Davis et al., 1991; Hemmati-Brivanlou et al., 1991; Ekker et al., 1992; Logan et al., 1992). Focused on chick embryos, En2 expression starts to be observed at stage HH9 (Figure 4A), its high expression levels being coincident with the prospective cerebellar anlagen (Martinez and Alvarado-Mallart, 1989). At stage HH19–20, the En2-staining domain forms a double decreasing gradient (Figure 4B) in a broad area around the Otx2/Gbx2 MH boundary, this En2-positive area including the presumptive territory of the cerebellar, isthmic, and mesencephalic anlagen (Figure 4C; Gardner et al., 1988; Davis et al., 1991; Logan et al., 1992; Millet and Alvarado-Mallart, 1995).
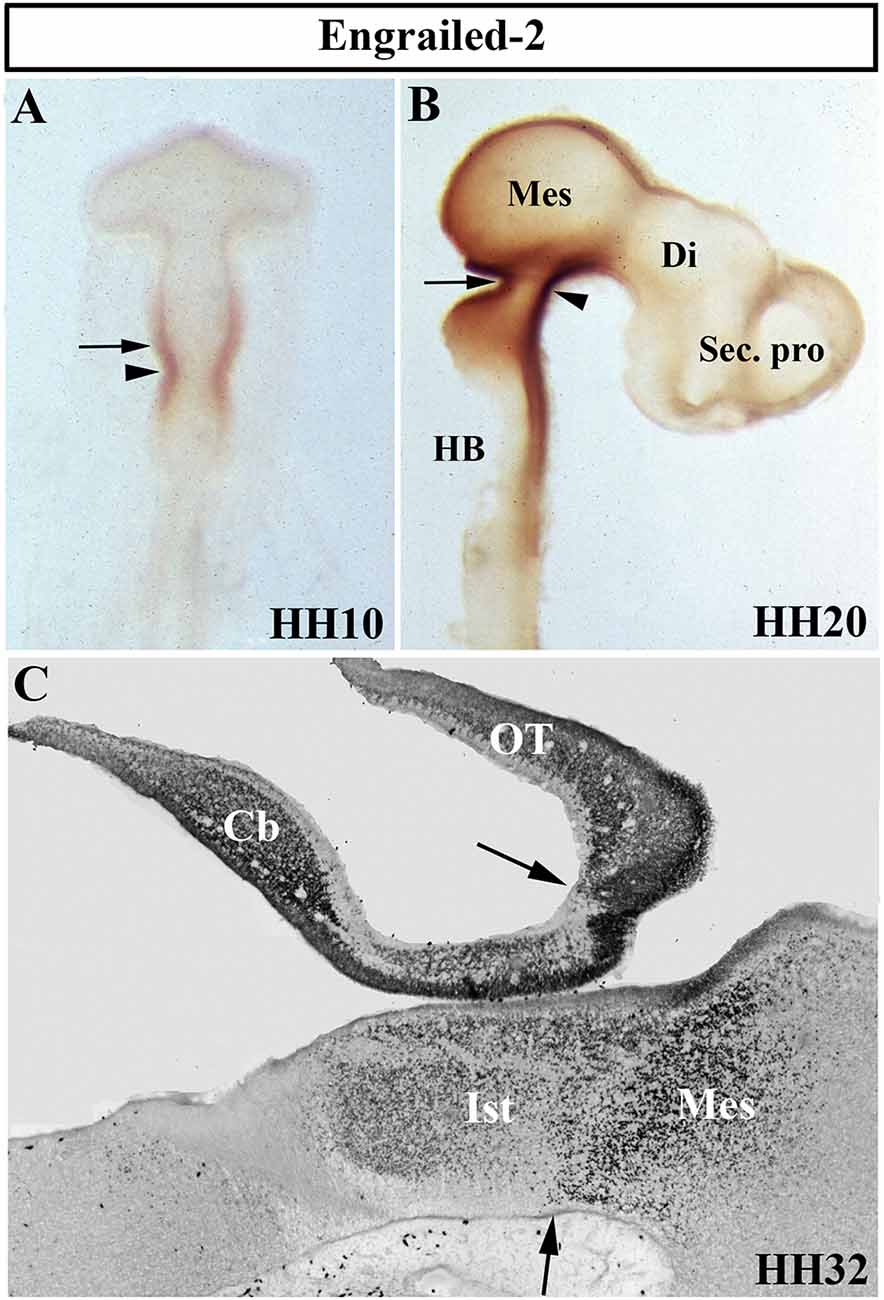
Figure 4. Distribution of Engrailed-2 (En2) at stages HH10 (A), HH20 (B), and HH32 (C) using immunoreaction with mAb 4D9 antibody in whole mount embryos (A,B) and in a sagittal section (C). The arrowheads in (A) and (B) point to the mes/met constriction. En2 staining is observed on both sides of the MH boundary (arrows in A–C), in a territory which includes the prospective territories of the cerebellum (Cb), isthmus (Ist), and mesencephalon (Mes), the latter developing in part the optic tectum (OT). Di, diencephalon; HB, hindbrain; Sec. pro (secondary prosencephalon). From Hidalgo-Sánchez et al. (2005a).
En1 null mutant mice died soon after birth with a great number of developmental alterations, in particular a relevant loss of both cerebellar and mesencephalic structures. The mesencephalic III (oculomotor) and rhombencephalic IV (trochlear) cranial nerves were lacking in homozygous mutant embryos. This phenotype may be caused by regional control of cell precursor proliferation instead of by cell fate determination; there is a loss or lack of proliferation of mid-hindbrain cells in En1 mutants (Wurst et al., 1994). However, ectopic cell death was also detected during the development of the prospective midbrain and cerebellum in En1 null homozygotes (Chi et al., 2003). En2 mutant mice are viable and display a relatively mild phenotype with some motor control problems as a consequence of the slight reduction in cerebellar size, a disturbed cerebellar foliation pattern in the caudal cerebellum, and a delay in the fusion of the cerebellar rudiments at the midline, together with smaller colliculi (Joyner et al., 1989, 1991; Millen et al., 1994). Therefore, the analysis of both En1 and En2 homozygous mutant mice suggests a partially redundant function of these genes in MH domain specification (Hanks et al., 1995). However, the comparison of the phenotypes of a varied combination (conditional, knock-in, and null) of En1/2 double-mutant mice clearly showed a differential requirement for En1 and En2 proteins in the specification of subdomains in the developing cerebellum and tectum (Sgaier et al., 2007). In a very significant way, the analysis of double En1/En2 CKO mutants and single CKOs showed that both En1 and En2 genes act together and are essential to guarantee the foliation patterning of the developing vermis and hemispheres by regulating the spatial restriction of key gene expressions, as well as to ensure the correct position and formation over time of fissures in the vermis, these genes acting late in embryogenesis (Cheng et al., 2010). In any case, En2 has a greater potential to control foliation than En1 (Sgaier et al., 2007). In summary, an “engrailed code” could be necessary for a correct dose-dependent subdivision of the tectal and cerebellar primordiain antero-to-posterior arranged functional subdomains (Sgaier et al., 2007; see also Simon et al., 2005 and Sgado et al., 2006 for other derivatives from MH domain).
In the tectal chick anlagen, En2 expression shows a decreasing gradient from caudal (stronger) to rostral (weaker). The works of Nakamura’s group have shown that En2 determines the exact position of the rostral mesencephalic boundary by repressing diencephalic markers, such as Pax6, and activating mesencephalic genes (Araki and Nakamura, 1999). Also, En2 confers posterior positional information in the developing tectum for tectal polarity formation and retinotopic projections. Using the chick/quail transplantation experimental model at the 10-somite stage, heterotopic grafts of the mesencephalic alar plate into the diencephalon showed a reverse En2 expression pattern, i.e., a rostrocaudal decreasing gradient from the mes-diencephalon border (Itasaki et al., 1991), and the topographic order of retinal fiber projections in these chimeric embryos changes in concordance with the inverted gradient of En2 expression (Itasaki and Nakamura, 1992; see Itasaki and Nakamura, 1996 for retroviral gene experiments). In addition, the prosencephalon differentiates into an optic tectum when transplanted into the mesencephalon, showing a typical laminar pattern and optic nerve fibers from the eyes extending to it (Nakamura et al., 1991). Similar results were obtained when the optic tectum was rotated in quail-chick chimeric embryos (Matsuno et al., 1991). Furthermore, En2 is involved in the tectal laminar formation, the rostral part of the optic tectum showing more advanced lamination than the caudal one, as a potential regulator of neuronal cell migration (Omi et al., 2014). In summary, these findings strongly suggest that En2 expression is controlled by environmental influences, governing the rostro-caudal polarity of the optic tectum (Friedman and O’Leary, 1996; Logan et al., 1996; Shigetani et al., 1997; Bilovocky et al., 2003; reviewed by Omi and Nakamura, 2015).
Pax2/5/8 Genes in the Midbrain-Hindbrain Specification
The vertebrate paired-box-containing Pax gene family, ortholog of the Drosophila Prd gene, encodes transcription factors which were highly conserved during evolution. This gene family is involved directly in animal body plan, regulating morphogenesis, organogenesis, and cell differentiation. These genes are also clearly implicated in the neuromeric organization of the brain because of their restricted expression patterns along the anterior-to-posterior axis of the developing neural tube. In particular, the Pax2/5/8 subfamily is expressed in the MH domain during ontogenesis with possible functional redundancy and equivalency between them (Krauss et al., 1991; Asano and Gruss, 1992; Stoykova and Gruss, 1994; Urbánek et al., 1994; Rowitch and McMahon, 1995; Heller and Brändli, 1997; Schwarz et al., 1997; Pfeffer et al., 1998; Rowitch et al., 1999; Goode and Elgar, 2009; Namm et al., 2014; Kesavan et al., 2017 among others). In chick embryos (Figure 5), Pax2 transcripts are detected in a portion of the developing neural tube on both sides of the MH junction at stages HH10 and HH20 (Figures 5C,D; Okafuji et al., 1999; Hidalgo-Sánchez et al., 1999a, 2005b). Pax2 expression is previous to Pax5 expression, labeling a smaller area included in the broader Pax5-positive domain (Funahashi et al., 1999). Thus, both Pax2- and Pax5-expressing domains form double rostral and caudal decreasing gradients, centered on the common Otx2/Gbx2MH boundary, similar to En2 expression (Figures 3–5). The area of overlap of Otx2 and Pax2 expressions in the caudal midbrain defines the preisthmic domain, contiguous to the MH boundary (Figures 3G; 5E). In both birds and mammalians, this histogenetic domain contains a caudal part of the midbrain reticular formation, the interfascicular nucleus, and the magnocellular (pre) isthmic nucleus, as well as the matching part of the periaqueductal gray (Hidalgo-Sánchez et al., 2005b; Puelles et al., 2013). These studies settle the four distinct midbrain histogenetic domains present in the midbrain alar plate: griseum tectale (rostrally), the optic tectum, the torus semicircularis, and the preisthmic domain (caudally; Hidalgo-Sánchez et al., 2005b).
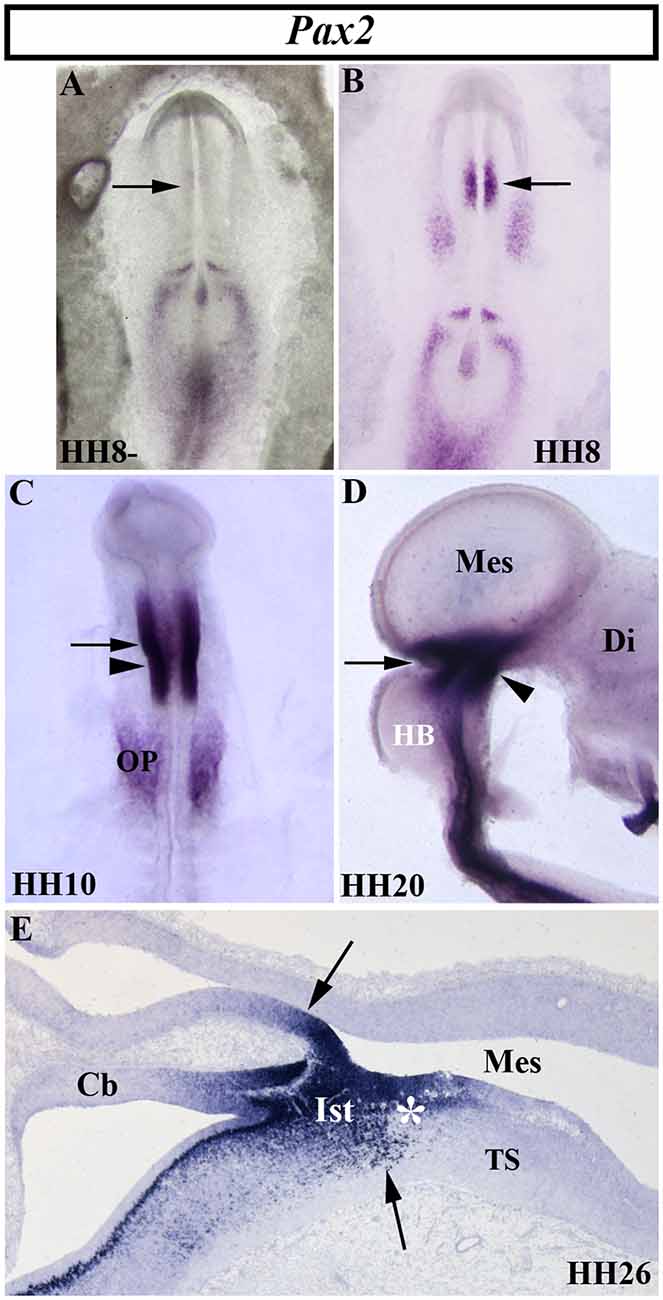
Figure 5. Spatial and temporal distribution of Pax2 transcripts in the chick developing MH domain at different developmental stages. Single in situ hybridization performed in whole-mount (A–D) and in sagittal sections (E). In the presumptive MH domain, Pax2 expression starts to be detected at stage HH8, but not before (arrows in A,B). At stages HH10, HH20, and HH26, Pax2 transcripts are detected on both sides of the MH boundary (arrows in C,E). The arrowheads in (C) and (D) point to the mes/met constriction. At stage HH26 (E), Pax2 expression is observed in the isthmic (Its) and pre-isthmic (asterisk in E) domains. Cb, cerebellum; Di, diencephalon; HB, hindbrain; Mes, mesencephalon; OP, otic placode; TS, torus semicircularis. From Hidalgo-Sánchez et al. (1999a, 2005b).
In mice, Pax2 expression is observed at E7.5, also previous to Pax5 expression, the latter occurring at E8.25 (Asano and Gruss, 1992; Urbánek et al., 1994; Rowitch and McMahon, 1995). The homozygous Krd mutation, a deletion in chromosome 19 resulting in the whole deletion of Pax2 locus, is lethal at the preimplantation stage. Deletion of one Pax2 allele in Krd(+/–) mutant mice exhibited a normal development of the MH domain (Urbánek et al., 1997). On the other hand, Pax5 homozygous mutants display a weak brain phenotype with slight morphological alterations in the posterior midbrain (inferior colliculus) and a perturbation of the cerebellum foliation pattern (Urbánek et al., 1994). Interestingly, Pax5 (+/–) Krd(+/–) mouse embryos show a significant absence of the caudal midbrain and alteration of the cerebellar vermis, whereas Pax5(−/−) Krd(+/–) embryos display a complete loss of the midbrain and the rostral hindbrain (Urbánek et al., 1997). Similar morphological alterations were reported for the spontaneous Pax21Neu mouse mutation (Favor et al., 1996). Other works showed that one allele of Pax2, but not Pax5, is necessary and sufficient for MH development in the absence of Pax5, these genes not being subjected to cross-regulation by each other (Schwarz et al., 1997). The possible discrepancies could be caused by the different genetic backgrounds used. In the zebrafish, alterations of Paz[b] gene function confirm the evidence from mouse embryos. Injection of neutralizing antibodies raised against the Paz[b] protein (Krauss et al., 1992), the noi (noistmus) mutation (Brand et al., 1996; Lun and Brand, 1998; Pfeffer et al., 1998), or the homozygous Tg [pax2a-hs:eGFP] embryos (Ota et al., 2016) also result in the loss of the MH domain. All these findings strongly suggest the regulatory interaction between Pax2 and Pax5 genes in the accurate development of the MH domain by the activation of common target genes according to a critical dose-dependent effect of Pax proteins (Brand et al., 1996; Schwarz et al., 1997, 1999; Urbánek et al., 1997; Bouchard et al., 2005). Interestingly, Pax5 (−/−) Krd (+/–) mutant mice display an absence of En1/2 expressions (Urbánek et al., 1997) and their phenotypes are similar to that reported in En1 mutant mice (Wurst et al., 1994). Thus, Pax genes could cooperate with other early markers of the MH domain to assure MH fate (Tallafuß and Bally-Cuif, 2003).
Using in ovo electroporation for gain-of-function experiments, it was reported that Pax2 plays a relevant role in the first step of midbrain specification, whereas Pax5 could sustain the state of midbrain differentiation once it is committed (Funahashi et al., 1999; Okafuji et al., 1999). Pax2 ectopic expression in the diencephalon transformed it into a mesencephalon after En2 induction and Pax6 repression, the latter being a diencephalic marker (Okafuji et al., 1999; Matsunaga et al., 2000). Although, Pax2 and Pax5 genes could be higher hierarchical elements in the genetic cascades devoted to the specification and determination of the midbrain primordium (Lun and Brand, 1998; Funahashi et al., 1999; Bouchard et al., 2005; Goode and Elgar, 2009), a mutual regulation between En and Pax2 functions has also been suggested (Picker et al., 2002). Different enhancers with functional Pax2/5/8-biding sites in mouse Pax genes could govern the activation and maintenance of their expressions in the MH domain at different developmental stages (Pfeffer et al., 2000, 2002). The existence of redundant regulatory elements in members of the Pax gene family could explain their mutual cooperation in MHB specification (Song et al., 1996).
An Organizer Center Is Present in the Central Portion of the Mid-Hindbrain Domain
The central portion of the mid-hindbrain domain expressing high levels of En1/2 and Pax2/5 could be considered the first organizer center described in the developing neural tube. Using the chick/quail chimeric experimental model, the alar plate of the quail isthmocerebellar primordium was transplanted ectopically to the caudal chick diencephalon (dp1 and dp2) at the 10-somite stage (Figures 6A,B; HH10), i.e., restricted in an area just caudal to the zona limitans intratalamica (Martinez and Alvarado-Mallart, 1990; Martinez et al., 1991). The graft maintained the original En2 expression and kept its cerebellar and isthmic nuclei fate. Interestingly, the quail-grafted En2-positive territory was able to induce a caudal decreasing gradient of En2 expression in the contiguous chick diencephalon (Figure 6C), which never expresses En2 under normal conditions (Figure 4B). The host En2-positive induced tissue developed an ectopic supernumerary midbrain (Figure 6C), showing the laminar cytoarchitectonic structure of an optic tectum (Martinez et al., 1991). Both supernumerary generated structures in the host, the grafted isthmocerebellum and the induced mesencephalon, displayed an inverted rostral-to-caudal order and orientation with respect to the meso-isthmo-cerebellar structures observed in the normal MH domain (Martinez and Alvarado-Mallart, 1990; Martinez et al., 1991; reviewed by Alvarado-Mallart, 1993, 1997, 2000). A conserved regulatory mechanism was also observed between mammals and birds (Martinez et al., 1991). These results agree with those from En2 ectopic-induced experiments in the caudal diencephalon concomitant with a Pax6 repression (Bloch-Gallego et al., 1996; see also Okafuji et al., 1999; Matsunaga et al., 2000).
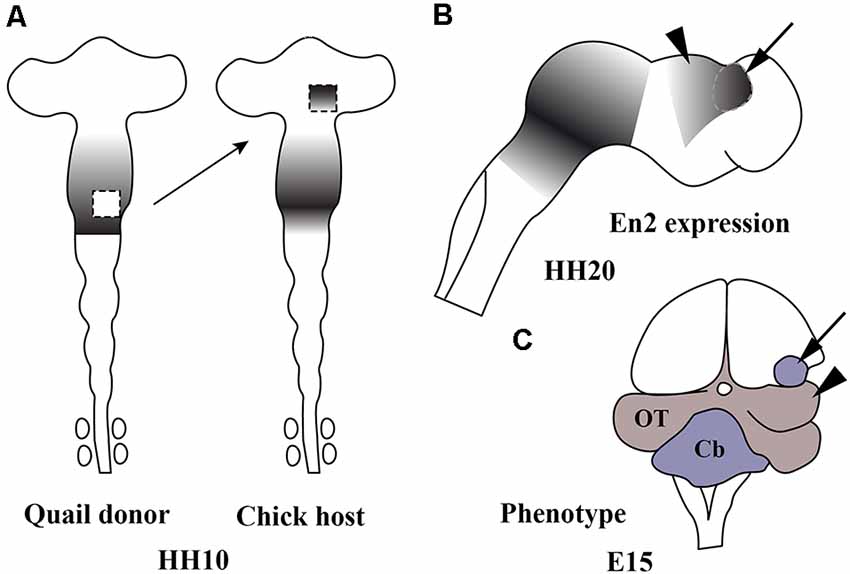
Figure 6. Schematic representation of chick/quail chimeric experiments showing the ectopic induction of En2 in the alar plate of the caudal diencephalon of chick (host; A) when the isthmocerebellar primordium of quail (donor; A), expressing high levels of En2, was grafted into it at stage HH10 (Martinez et al., 1991). The En2-expressing area is represented by gray areas. At stage HH20 (B), the quail graft (arrow) maintains its original En2 expression and induces an inverted gradient of En2 expression within the caudal prosencephalon (arrowhead), typical of the mesencephalic vesicle. A long-survival analysis performed at El5 (C) shows that the graft (arrow) developed a small cerebellum and the En2-induced host primordium changed its fate for an ectopic mesencephalon (arrowhead). Cb, cerebellum; OT, optic tectum. From Alvarado-Mallart (2000).
In addition, the caudal diencephalon (dp1 and dp2), but not the dp3 and the secondary prosencephalon, was competent to express En2 and changed its phenotype to be adapted to the integration side when it was transplanted in close contact with the Otx2/Gbx2 MH boundary, from where the mentioned double Pax2/En2 decreasing gradients originated (Martinez et al., 1991; Bloch-Gallego et al., 1996). Thus, the caudal-grafted diencephalon is able to develop isthmic and cerebellar structures, as well as an optic tectum. These phenotypic changes never take place when the rostral mesencephalic vesicle is considered in the experimental model, which displays low levels of En2 expression (Gardner and Barald, 1991). It is worth noting that the rostral mesencephalic vesicle transplanted into the prosencephalon at the 10-somite stage lost its original En2 expression, while the caudal mesesenphalic vesicle maintained En2 expression (Gardner and Barald, 1991). Therefore, different regulatory/inductive properties could be considered between the rostral and caudal halves of the mesencephalic vesicle observed at stage HH10 (Alvarado-Mallart et al., 1990).
These inductive properties were studied in detail analyzing all MH neuronal structures generated after the inversion of a portion of the neural tube containing the presumptive midbrain plus its caudally adjacent territory, the isthmocerebellar primordium (Figure 7A; Marín and Puelles, 1994). The analysis of short-survival chick/quail chimeric embryos showed that the En2 expression pattern of the inverted portion was abnormal, displaying two mirror-duplicated areas of high En2 expression on both its rostral and caudal halves. As expected, the caudal diencephalon was induced to express the En2 gene. Cytoarchitecture analysis of long-survival resulting chimeras showed that the inverted graft developed an inverted isthmocerebellar structure in its rostral aspect plus an altered midbrain whose rostral and caudal halves show a caudal alar mesencepahilic grisea phenotype with symmetrical morphology and polarity, the rostral alar mesenphalic area (the griseum tectale) being absent (Figure 7B). The contiguous caudal diencephalon changed its fate drastically to an isthmic and caudal mesencephalic phenotype with a normal polarity with respect to the entire brain axis (for more anatomical details concerning the alar and basal plates, see Marín and Puelles, 1994). Experiments in which the inversion of the portion of the neural tube containing exclusively the prospective mesencephalon, without any portion of the isthmocerebellar prospective domain, were also performed. In these chimeric cases, the grafted neuroepithelium showed a normal pattern of En2 expression in short survival analysis, while the torus semicircularis, optic tectum, and griseum tectalis formation presented a normal caudal-to-rostral order in long survival studies. Thus, these findings fit well with the existence of a source of polarizing signal placed in the prospective isthmocerebellar domain, located just in the caudal most portion of the “mesencephalic” vesicle observed at stage HH10 (Martinez and Alvarado-Mallart, 1989; Crespo-Enriquez et al., 2012).
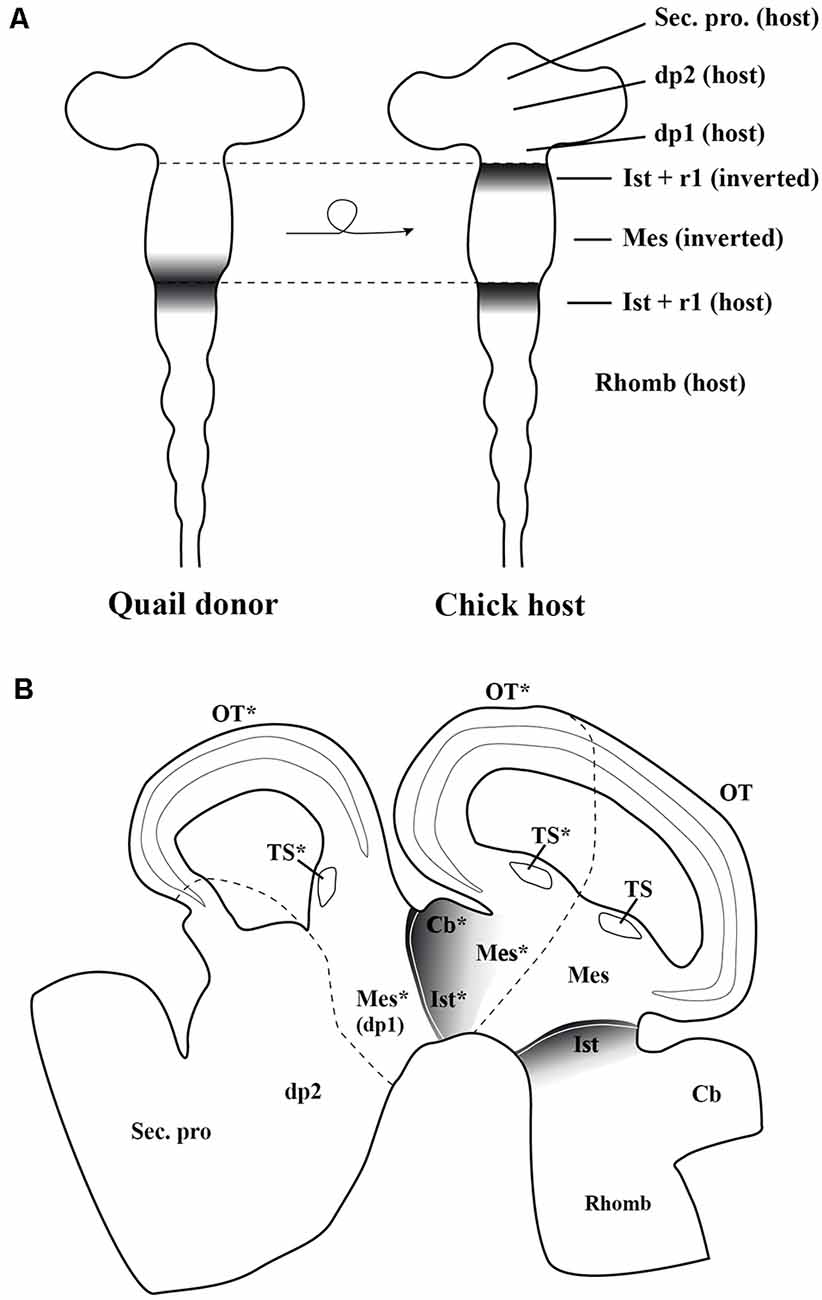
Figure 7. (A) Schematic representation of the chick/quail chimeric experiments in which the quail (donor) “mesencephalic” vesicle at stage HH10, containing both the mesencephalic primordium and the rostral part of the isthmocerebellum, was grafted into a chick (host) embryo after inversion of its rostrocaudal axis (Marín and Puelles, 1995). The En2-expressing area is represented by gray areas. In the resulting chimeric embryo, the grafted isthmocerebellum was positioned just in contact with the host pd1 (diencephalon), whereas the rostra1 “mesencephalic” vesicle was in direct interaction with the staying host pro-rhombomere 1, RhA1. (B) Sagittal section of the resulting chimeric embryo in a long-survival analysis. The grafted-rotated portion of the neural tube is located between both dotted lines. The rostral and caudal portions of isthmocerebellum kept its original phenotype giving rise to the cerebellum (Cb) and isthmic nuclei (Ist). The grafted mesencephalic primordium formed a bicaudal phenotype, as showed by the symmetry of its cytoarchitecture organization of the optic tectum (OT) and a duplication of the torus semicirculais (TS). The host dp1 changed its fate for a complex caudal mesencephalic structure (OT plus TS), the phenotype of the contiguous dp2 not being perturbed. Asterisks, ectopic structures observed in experimental studies. Mes, mesencephalon; r1, rhombomere 1; Rhomb, rhombecephalon; Sec. pros, secondary prosencephalon. Adapted from Marín and Puelles (1995).
The possible plasticity of the entire rhombencephalon with respect to the En2-expressing MH boundary was also studied. When transplanted into the rhombencephalon, the grafted “mesencephalic” vesicle differentiates into an optic tectum, without any structure resembling a cerebellar cytoarchitecture (Nakamura, 1990). However, the presumptive territory of the isthmocerebellum maintained always its En2 expression and cerebellar phenotype, and also induced a fate change in the hindbrain; it induced ectopically En2 expression in the competent rhombomeres, but caused the development of a cerebellum with a typical cytoarchitectural profile in the host contiguous neural tissue instead of an optic tectum (Martínez et al., 1995). It is interesting to remark that, in this kind of experiment, the observed inductive consequences did not cross the boundaries between adjacent rhombomeres (clonal restriction; Martínez et al., 1995). However, the prospective isthmic area, grafted in the posterior hindbrain, caudal to the rhombomere 8, or in the spinal cord was not able to induce En2 expression and to develop a cerebellar-like structure in host tissue, the grafted area changing its phenotype to adapt to its new environment (Grapin-Botton et al., 1999).
The competence of developing a neural tube to express the En2 gene has been studied. In the chick, a great portion of the developing neural tube at stage HH10, extending from the dp2 to r7, is able to express En2 and to change its prospective fate. The induced phenotype appears to depend on its position in relation to the “mes/met” constriction. When a neuromere is situated rostral to the “mes/met” constriction, the En2-induced tissue develops a mesencephalic phenotype, whereas caudal to it, it develops a cerebellar structure (Alvarado-Mallart, 2000; Hidalgo-Sánchez et al., 2005a).
FGF8 Is the Molecular Effector of the Isthmic Organizer Activity
The gene family of the fibroblastic growth factors (Fgf) is required in embryonic development leading to growth and patterning events (Delgado and Torres, 2017; Thawani and Groves, 2020). The Fgf8 gene, firstly identified by encoding a secreted, androgen-induced signaling molecule (Tanaka et al., 1992), is one of the most relevant signaling molecules with long-range diffusible activity. The well-defined spatial and temporal expression pattern of the Fgf8 gene in the prospective isthmic domain clearly proposes the FGF8 as the main inductive molecule emanating from it and controlling all the previously mentioned developmental events taking place in the MH domain of all analyzed vertebrates (mouse: Heikinheimo et al., 1994; Ohuchi et al., 1994, 2000; Crossley and Martin, 1995; Bueno et al., 1996; chick: Figures 8A–C,E,G,I; Hidalgo-Sánchez et al., 1999a; Ohuchi et al., 2000; Garda et al., 2001; Xenopus: Christen and Slack, 1997; zebrafish: Reifers et al., 1998; reviewed by Echevarria et al., 2005; Vieira et al., 2010). In the chick, Fgf8 expression is first detected at stage HH9 (Figures 8A,B), much later than Otx2 and Gbx2 expressions (Figures 3A,B). At stage HH10, the Fgf8-expressing domain is observed in a portion of the neural tube containing the caudal most portion of the “mesencephalic” vesicle and the rostral half of the RhA1 (Figure 8C), that is on both sides of the so-named “mes/met” constriction (Figure 2A; Hidalgo-Sánchez et al., 1999a; Garda et al., 2001). Remarkably, this Fgf8-expressing area is located just in a narrow transversal band where Otx2-positive and Gbx2-positive domains overlap (Garda et al., 2001). At stage HH14–15, when the Otx2 and Gbx2 co-expression disappears and both Otx2-expressing and Gbx2-expressing domains are mutually exclusive and complementary, i.e., when the Otx2/Gbx2 midbrain/hindbrain boundary is rightly established, the Fgf8-positive area overlaps the rostralmost portion of the Gbx2-positive domain, where the prospective isthmus develops, abutting the Otx2-positive domain (see Figures 8E,G,I for stages HH20 and HH26, respectively; Hidalgo-Sánchez et al., 1999a, 2005b; Garda et al., 2001).
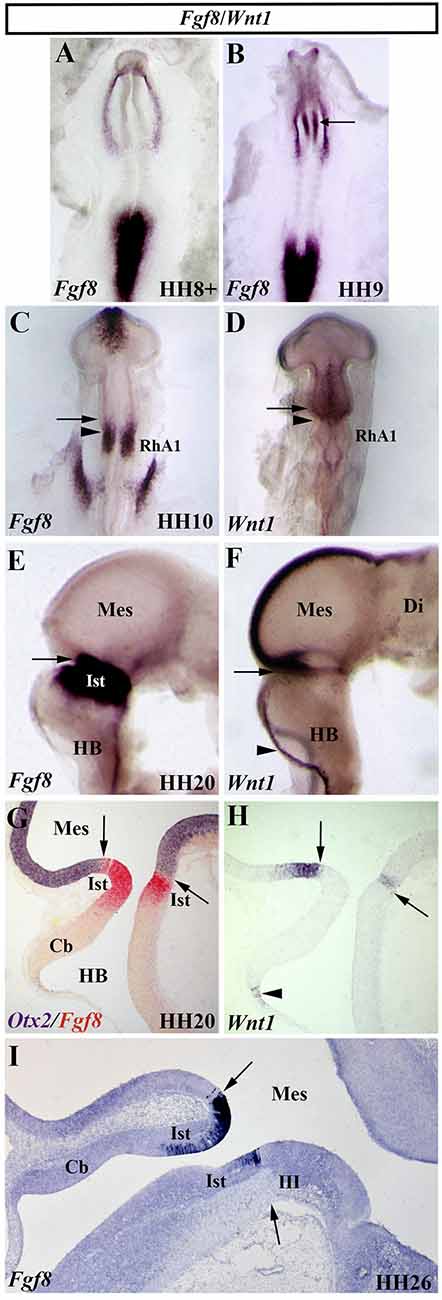
Figure 8. Spatial and temporal expression of Fgf8 and Wnt1 genes in the chick developing MH domain at different developmental stages. Single (A–F,H,I) and double (G) in situ hybridization performed in whole-mount (A–F) and in sagittal sections (G–I). The arrows point to the Otx2/Gbx2 MH boundary. In the developing MH domain, Fgf8 transcripts are first detected at stage HH9 (A; arrow in B). At stage HH10, Fgf8 expression is observed in a portion of the neural tube just caudal to the Otx2/Gbx2 MH boundary (arrow in C) and rostral to the mes/met constriction (arrowhead in C). Wnt1 expression is detected in the entire “mesencephalic” vesicle, rostral to the mes/met constriction (arrowhead in D), with a stronger expression in its dorsal midline (D). At stage HH20, Fgf8 and Wnt1 expressions are present on both sides of the Otx2/Gbx2 MH boundary (arrows in E–H), as confirmed by double Otx2 and Fgf8 staining (arrow in G). Wnt1 transcripts are also detected in the choroidal tissue (arrowheads in F,H). Note that the isthmic region (Ist, r0) is clearly Fgf8 positive at stages HH20 (E,G) and HH26 (I). Cb, cerebellum; Di, diencephalon; HB, hindbrain; III, trochlear nucleus; Mes, mesencephalon; RhA1, pro-rhombomere 1. From Hidalgo-Sánchez et al. (1999a); Hidalgo-Sánchez et al. (2005a, 2005b).
The isthmus, also named r0, is the rostralmost segmental unit of the hindbrain (Aroca and Puelles, 2005; Puelles, 2013). Using Fgf8-Cre-LacZ mice to determine the cell derivatives from the Fgf8 expressing domain and anatomical characteristics, the Puelles’s group defined the isthmus as a distinct transverse segment of the mammalian brain delimited rostrally by the Otx2/Gbx2 boundary and the MH constriction, whereas caudally the r0/r1 boundary is defined exclusively by the borders of regulatory gene expression patterns such as Irx2 (Watson et al., 2017). Therefore, the r0/r1 could be considered a cryptic (molecular) boundary. In mice, the isthmus contains the same derivatives which have been previously described in birds (Puelles et al., 2007). In addition to contributing to the formation of the cerebellar vermis (the rostralmost portion of the cerebellum), the developing isthmic domain in birds contains the avian parvicellular and semilunar isthmic nuclei (homologous to the parabigeminal and microcellular tegmental nuclei in mammals), the trochlear nucleus (whose cranial motor root emerges from the dorsal isthmic surface), the dorsal nucleus of the lateral lemniscus, the caudal linear nucleus, a rostral part of the interpeduncular nucleus, and the caudal isthmic part of the substantia nigra, among other alar and basal derivatives (for more anatomical details, see Alvarez-Otero et al., 1993; Aroca et al., 2006; Puelles et al., 2007; Lorente-Cánovas et al., 2012; Alonso et al., 2013; Watson et al., 2017). Although some isthmic neurons migrate into the contiguous neuromeres, the caudal midbrain and r1, these findings do not question the existence of lineage restriction in r0 (Watson et al., 2017).
FGF8 is the molecule responsible for the isthmic organizer properties of the isthmus or r0. Beads soaked with FGF8 recombinant protein, inserted in the caudal diencephalon or in the rostral mesencephalon, are able to induce ectopic En1/2 expression and to change the fate of the insertion tissue into two ectopic, mirror-image mesencephalon and isthmic nuclei, as well as cerebellar structures with cells expressing CaBP, a Purkinje cell marker (Crossley et al., 1996; Martinez et al., 1999; Garda et al., 2001), mimicking the inductive effects obtaining through grafting experiments (Martinez and Alvarado-Mallart, 1990; Martinez et al., 1991). FGF8 induced Fgf8 expression and repressed Otx2 expression in the neuroepithelium around the implanted beads. Besides, FGF8-soaked beads also induced Gbx2 expression in mouse explant (Liu et al., 1999). On the other hand, Fgf8-expressing isthmic grafts integrated with the caudal hindbrain or the spinal cord show a downregulation of its Fgf8 expression together with a progressive loss of its inductive ability, the fate being adapted to the host environment (Grapin-Botton et al., 1999). In summary, FGF8-soaked beads and the resulting effects of ectopic-grafted isthmic organizer tissue strongly suggest that FGF8 is responsible for inductive events mediated by the prospective isthmic domain. In this genetic context, it is relevant to highlight that Pax2 is crucial for the induction of Fgf8 expression at the MH boundary (Ye et al., 2001). Loss-of-function studies in mice confirm the involvement of an FGF8 signaling pathway during gastrulation and in the induction and specification of the MH domain (Meyers et al., 1998; Sun et al., 1999; Chi et al., 2003). Fgf8−/− mutant mouse development fails easily at gastrulation, these mice show a prospective neuroectoderm with crucial alterations (Sun et al., 1999).
According to the described Fgf8−/− phenotypes in mice, the spatiotemporal action of a dose-dependent FGF8 activity could also regulate tissue specification of the tectal-isthmo-cerebellar structures in several periods of the embryonic periods (Basson et al., 2008; Sato and Joyner, 2009). A sustained and strong FGF8 action may control the formation of the structures next to the isthmus (part of the medial inferior colliculi, the isthmus, and the medial-anterior cerebellum), whereas low levels and brief FGF8 activity could be sufficient for the correct formation of structures far from it (the superior colliculi and the lateral cerebellum). It could involve a Fgf8-mediated Otx2 repression and the maintenance of an accurate relationship among expression patterns of key regulatory genes in the proximity of the MH boundary. Other Fgf genes could also be involved. The reduced Fgf17 expression observed in Fgf8 temporal CKOs could explain the observed survival of most midbrain/r1 cells near the isthmus (Sato and Joyner, 2009).
In zebrafish, the acerebellar (ace) mutant embryos show an absence of isthmus and cerebellum, whereas an enlarged tectum is formed (Brand et al., 1996; Reifers et al., 1998; Picker et al., 1999; Jászai et al., 2003; Tallafuß and Bally-Cuif, 2003; see also Araki and Brand, 2001 for morpholino-induced knockdown of Fgf8), together with a loss of eng and pax-b expressions (Brand et al., 1996; Reifers et al., 1998). The observed tectal expansion was caused by caudal-to-rostral transformation, with a noticeable cell fate change and without perturbation of cell proliferation and apoptosis patterns. FGF8-soaked beads rescue MH development with normal isthmocerebellar structures, confirming the key role of FGF8 in patterning and polarizing the MH domain (Jászai et al., 2003). Also, these mutant embryos show a partial disruption of the retinotectal map compared to the wild type, these results suggesting that FGF8 from the isthmic area could mediate the establishment of midbrain polarity (Picker et al., 1999). However, early Fgf8 expression does not require pax2a (Reifers et al., 1998), supporting the hypothesis of an early MH domain specification in at least two phases (Reifers et al., 1998; Tallafuß and Bally-Cuif, 2003). In the initial establishment phase in late gastrulation, midbrain and rostral hindbrain specification could be established in an independent way within an MH-pro-domain, probably depending on Otx2 and Gbx2 instructive regulatory activities. In a second maintenance phase, MH development could be governed by a complex regulatory network of signaling molecules generated all around the MH boundary, fgf8 being mainly involved in the reinforcement of an r1 vs. r2 identity. Besides, zebrafish embryos with reduced Otx activity and without fgf8 function (fgf8−/−; OtxH mutants) display an enlarged r1 but with some cerebellar cells, suggesting that neither the fgf8 gene nor other members of the Fgf family could be required for r1 specification and cerebellar development, as well as for cerebellar cell differentiation. However, fgf8 seems to be relevant to maintaining r1 devoid of Otx2 expression defining the MH boundary, in addition to being necessary for cell proliferation and cerebellar primordium morphogenesis (Foucher et al., 2006). Interestingly, the locus coeruleus, originated in the alar plate of r1 (Aroca et al., 2006), was absent in these fgf8−/−; OtxH mutant embryos (Foucher et al., 2006).
The FGF signaling pathway is mediated via four tyrosine kinase-type transmembrane receptor (FGFR) proteins, which trigger several intracellular signaling cascades, including Ras-ERK pathways as a subclass of mitogen-activated protein kinase pathway (Echevarria et al., 2005). The expressions of the Fgfrs genes have been described in the developing nervous system of vertebrates, particularly in the midbrain and anterior hindbrain (Friesel and Brown, 1992; Yamaguchi et al., 1992; Walshe and Mason, 2000; Liu et al., 2003; Trokovic et al., 2003, 2005; Scholpp and Brand, 2004; Jukkola et al., 2006; Blak et al., 2007b; Saarimäki-Vire et al., 2007; Ota et al., 2010; Rohs et al., 2013; Leerberg et al., 2019). Fgfr1 expression was present in the dorsal aspect of the MH domain (Friesel and Brown, 1992; Walshe and Mason, 2000; Trokovic et al., 2003, 2005; Scholpp and Brand, 2004). FGFR1 seems to be the main FGF receptor receiving FGF signals from the isthmic organizer (Trokovic et al., 2003, 2005; Jukkola et al., 2006), Fgfr2 and Fgfr3 being dispensable for normal MH development (Blak et al., 2007a). Thus, tissue-specific inactivation of Fgfr1 in En1-Cre/+; Fgfr1flox/flox mice caused a failure of the MH constriction and a deletion of the posterior hindbrain (the posterior colliculi) and the anterior portion of the cerebellum (the vermis), the cerebellar hemispheres being present only with an altered foliation. Large deletions were also detected in the midbrain, mainly affecting the inferior colliculi. Similar effects were observed in mice homozygous for hypomorphic Fgfr1 alleles. In contrast, the basal plate of the MH domain (the oculomotor and trochlear nuclei, as well as the locus coeruleus and the substance nigra) did not display any deletions or alterations in En1-Cre/+; Fgfr1flox/flox mice (Trokovic et al., 2003, 2005). Once the isthmic organizing center has been established, a sustained Fgfr1 expression could be necessary for maintaining the response to the isthmic signals and for correct specific cell-adhesive characteristics to take place at the MH border (Trokovic et al., 2003).
Using a conditional gene inactivation method without any perturbed Fgf8 expression during gastrulation, it has been shown that part of the FGF8 regulatory activity is due to its involvement in cell survival, associated with En and Gbx2 activities, among other genes, the prospective midbrain being affected earlier than the cerebellum (Chi et al., 2003). Thus, cell death in the presumptive MH domain increases when the level of Fgf8 expression decreases (Chi et al., 2003). This failure of cell survival was also confirmed at least in part in works with loss-of-function analysis of the Fgf signaling pathway (Basson et al., 2008; Sato and Joyner, 2009). In this sense, the Lmx1b.1 and Lmx1b.2 genes could participate in this cellular event by the regulation of Fgf8 and Wnt1 (O’Hara et al., 2005).
Surprisingly, Fgfr1 inactivation does not affect cellular survival (Trokovic et al., 2003). However, the study of mice embryos with different combinations of Fgfr1, Fgfr2, and Fgfr3 mutations showed a redundant participation of FGFRs in supporting cell survival, governing the anterior-to-posterior specification of the MH domain (Saarimäki-Vire et al., 2007).
Wnt1 Activity During MH Domain Development
The vertebrate Wnt1 gene, homolog of the segment polarity wingless (wg) gene in Drosophila (Rijsewijk et al., 1987), encodes a short-range diffusible molecule involved in complex cell-cell signaling processes, governing several embryonic events (tissue patterning, cell fate determination, apoptosis, and proliferation, among others). In the developing neural tube of vertebrates, Wnt1 expression is detected in the MH domain (mouse: Wilkinson et al., 1987; Bally-Cuif et al., 1992, 1995; Parr et al., 1993; chick: Bally-Cuif and Wassef, 1994; Hollyday et al., 1995; Sugiyama et al., 1998; Hidalgo-Sánchez et al., 1999a; zebrafish: Molven et al., 1991; Kelly and Moon, 1995; Lekven et al., 2003; Buckles et al., 2004; Xenopus: Wolda et al., 1993). In chick embryos, the Wnt1 gene displays a dynamic expression pattern. At stage HH7, Wnt1 transcripts are present in the presumptive mesencephalic area of the neural plate prior to the neural tube closure. At stage HH10, Wnt1 expression is observed in the entire “mesencephalic” vesicle with a higher expression in its caudal half (Figure 8D). At this developmental stage, the caudal border of Wnt1 expression extends slightly more caudal than the Otx2-positive domain, thus overlapping somewhat with the Gbx2-expressing domain (Figures 3C,D, Figure 8D; Hidalgo-Sánchez et al., 1999a). In stage-HH20 chick embryos, Wnt1 expression is confined to a ring encircling the neural tube in the most caudal portion of the mesencephalic vesicle and on its dorsal midline, the latter extending rostral into the caudal prosencephalon until the prospective epiphysis (Figure 8F). Wnt1 expression is also observed in the rhombic lip of the developing hindbrain (Figures 8F,H; Bally-Cuif and Wassef, 1994; Hollyday et al., 1995). At this developmental stage, double in situ hybridization showed that the encircling ring of Wnt1 expression is located in the caudal most portion of the Otx2-positive mesencephalic domain, abutting dorsally with the Fgf8- and Gbx2-expressing domain (Figures 8G,H; Hidalgo-Sánchez et al., 1999a).
Using the chimeric experimental model, Bally-Cuif and co-workers showed that, when the caudal mesencephalic vesicle was transplanted into the prosencephalon, a chick En2 induction was observed in the surrounding tissue together with Wnt1 expression in the graft (Bally-Cuif et al., 1992; Bally-Cuif and Wassef, 1994). Of course, the grafted tissue included an Fgf8-expressing neuropithelium (Hidalgo-Sánchez et al., 1999a), therefore it is not possible to assign this inductive effect exclusively to WNT1 diffusible molecules. In this sense, in vitro assays showed that a selective antagonist of WNT signals or an inhibitor of FGFR activation can block the expression of MH markers in MH explants at stage 4, suggesting that WNT and FGF signaling pathways are required and sufficient for the initial induction of the isthmic activity at the gastrula stage in chick embryos (Olander et al., 2006). However, similar neural explant assays with tissues isolated from HH12 embryos showed that WNT signaling post-gastrulation is involved in maintaining the early pattern of Fgf8 expression in the MH domain and, consequently, the isthmic identity (Canning et al., 2007). Therefore, it is not possible to confirm if WNT1 and FGF8 instructive actions are already present at the gastrula stage (Olander et al., 2006) or shortly thereafter (Canning et al., 2007).
Mouse embryos lacking Wnt1 function fail to form an MH boundary with a severe reduction of the midbrain and no obvious cerebellum, the rostral midbrain juxtaposing directly with the caudal hindbrain (McMahon and Bradley, 1990). Although En1 expression was apparently normal in 4-somite littermate homozygous for mutated Wnt1 allele, the En1-expressing domain was completely lost in the MH domain at the 21–30-somite stage (McMahon et al., 1992). These anatomical abnormalities in the development of the midbrain and cerebellum were also confirmed by the Capechi’ group analyzing other less-severe Wnt1 (swaying/sw) disruptions in mice (Thomas and Capecchi, 1990; Thomas et al., 1991). In Wnt1sw/sw embryos with a severe phenotype, the transverse Wnt1sw-expressing ring of the MH boundary was completely missing and ectopic patches of Otx2-expressing cells were detected in r1, without crossing the r1/2 border. These Wnt1sw/sw embryos showed an MH domain that was severely shortened. In Wnt1sw/sw embryos with mild phenotype, the Wnt1sw ring was interrupted and scattered patches of ectopic Wnt1sw-expressing cells were observed in the rostral r1, just in the rostralmost aspect of some ectopic Otx2-expressing patches of cells. In all analyzed Wnt1sw/sw embryos, both cranial nerve III (caudal most midbrain) and IV motoneurons (rostralmost hindbrain) were absent, which normally develop on both sides of the transverse Wnt1-positive ring. Therefore, the straight common border of Otx2/Wnt1sw expressions at the MH boundary failed to form correctly. In summary, Wnt1 could be needed firstly for the specification of the MH domain, probably by the maintenance of En expression territory (McMahon et al., 1992; Wurst et al., 1994; Danielian and McMahon, 1996; Sugiyama et al., 1998) and later for the segregation of mesencephalic (Otx2-positive) and isthmocerebellar (Otx2-negative, and so Gbx2-positive) phenotypes. The latter effect could be mediated by correct adhesive properties of cell-cell interactions at the Otx2/Wnt1 MH boundary (Bally-Cuif et al., 1995).
Some differences have been observed among chick, mouse, and zebrafish development (Lekven et al., 2003; Buckles et al., 2004). In zebrafish, the analysis of homozygous wnt1/wnt10b deficient embryos and morpholino antisense (MO)-mediated oligonucleotide knockdown showed that only a portion of the pax2a and en2a-expressing areas in the MH domain is dependent on the wnt1 and wnt10b activities. In these experimental embryos, the resulting phenotype was almost normal with only faint defects (Lekven et al., 2003). Both wnt1 and wn10b genes are not necessary for the standard maintenance of fgf8 and en2b expressions, as well as those of other wnt genes (wnt8b and wnt3a). Therefore, the wnt1 and wn10b genes seem not to be needed, surprisingly, for the correct development of the midbrain and cerebellum in zebrafish development (Lekven et al., 2003). Morpholinos knockdown in zebrafish also showed that wnt3a is required for the correct MH domain development in absence of wnt1 and wnt10b activities, the expression of en2, pax2a, and fgf8 not being maintained after mid-somitogenesis (Buckles et al., 2004). wnt3/wnt1/wnt10b deficient embryos suffer wide-ranging apoptosis in the prospective MH domain resulting in a major loss of the midbrain and cerebellum (Buckles et al., 2004). Because of the fact that wnt genes have self-governing and coinciding functions during zebrafish development, the possible function of other wnt genes, such as wnt8b, in the accurate development of the MH domain cannot be ruled out (Buckles et al., 2004; Rhinn et al., 2005).
The Genetic Cascade at the Mid-Hindbrain Boundary
The juxtaposition of differently pre-specified areas could generate interfaces and specific diffusible morphogenes. The action of these long- or short-ranging molecules with possible antagonist effects could trigger specific genetic programs in a dose-dependent manner devoted to governing the specification of large domains by means of key regulatory genes (Meinhardt, 2009). In the developing MH domain, the specification of Otx2- and Gbx2-expressing confronting areas at earlier developmental stages could determine the precise location of the MH boundary. The co-regulation of two relevant signaling pathways, FGF and WNT, together with the action of transcription factors, such as PAX and EN, among others, could be responsible for the inductive events mediated by the isthmic organizer center (Broccoli et al., 1999; Hidalgo-Sánchez et al., 1999b; Irving and Mason, 1999; Martinez et al., 1999; Millet et al., 1999; Katahira et al., 2000; Garda et al., 2001; Li and Joyner, 2001; Martinez-Barbera et al., 2001; Matsunaga et al., 2002; Hidalgo-Sánchez and Alvarado-Mallart, 2002; Tour et al., 2002a, b; Panhuysen et al., 2004; Li et al., 2005).
Pivotal studies using chick/quail chimeric systems carried out by the Alvarado-Mallart’s group are devoted to unveiling the role of the Otx2/Gbx2 mutual interaction in the MH domain (Figure 9; Alvarado-Mallart, 2000; Hidalgo-Sánchez et al., 2005a). When the quail alar portion of the Otx2-expressing caudal diencephalon, competent to express En2, was transplanted at the 10-somite stage (HH10) into the chick MH territory, always in direct contact with Gbx2-expressing RhA1 (Figure 9A), the expression of Gbx2 and Fgf8 was induced in a small portion of the graft just contiguous to the host Gbx2-positive domain (Figure 9B). Interestingly, the original Otx2 expression was repressed in the Gbx2/Fgf8 induced domain within the transplant (Figures 9C,D). Indeed, a new Otx2/Gbx2 interface was created within the grafted territory. The other genes involved in the specification of the MH domain (Fgf8, Wnt1, En2, and Pax2) showed expression patterns similar to those described in normal development: Fgf8 and Wnt1 were expressed in two narrow bands, while Pax2 and En2 formed double decreasing gradients centered on the new intra-graft Otx2/Gbx2 boundary (Figures 9C–H; Hidalgo-Sánchez et al., 1999b). In the induced genetic event, En2 and Pax2 were the first genes to be expressed, while Otx2 repression and Gbx2 induction occurred later with an initial gap of Otx2/Gbx2 expression as observed in normal development (Garda et al., 2001). Fgf8 was the last gene to be expressed in the Gbx2-induced portion of the graft (Hidalgo-Sánchez and Alvarado-Mallart, 2002). A long-term survival analysis showed that the grafts developed a supernumerary optic tectum at the level of the host cerebellum from the Otx2/En2/Pax2/Wnt1-expressing area. This area also contributed to both in situ isthmus and cerebellum, which develops from the Gbx2/En1–2/Pax2/Fgf8-expressing one (Figures 9I,J; Hidalgo-Sánchez et al., 1999b, 2005a). It is also worth noting that the induction of Fgf8 expression was also evident in experiments in which the prospective territories of the midbrain (Otx2-positive) and r1 (Gbx2-positive) were juxtaposed (Irving and Mason, 1999). The mutual repression of Otx2 and Gbx2 genes was also confirmed in gain-of-function experiments in chicks using in ovo electroporation. When Otx2 was ectopically expressed in the rostral hindbrain, the alar plate of r1 differentiated into the optic tectum instead of an isthmocerebellar structure. In addition, when Gbx2 was ectopically expressed in the mesencephalon, the Otx2 caudal mesencephalic limit shifted rostrally. Thus, ectopic activations of Otx2 and Gbx2 induced Fgf8 expression at the new Otx2/Gbx2 interface (Katahira et al., 2000).
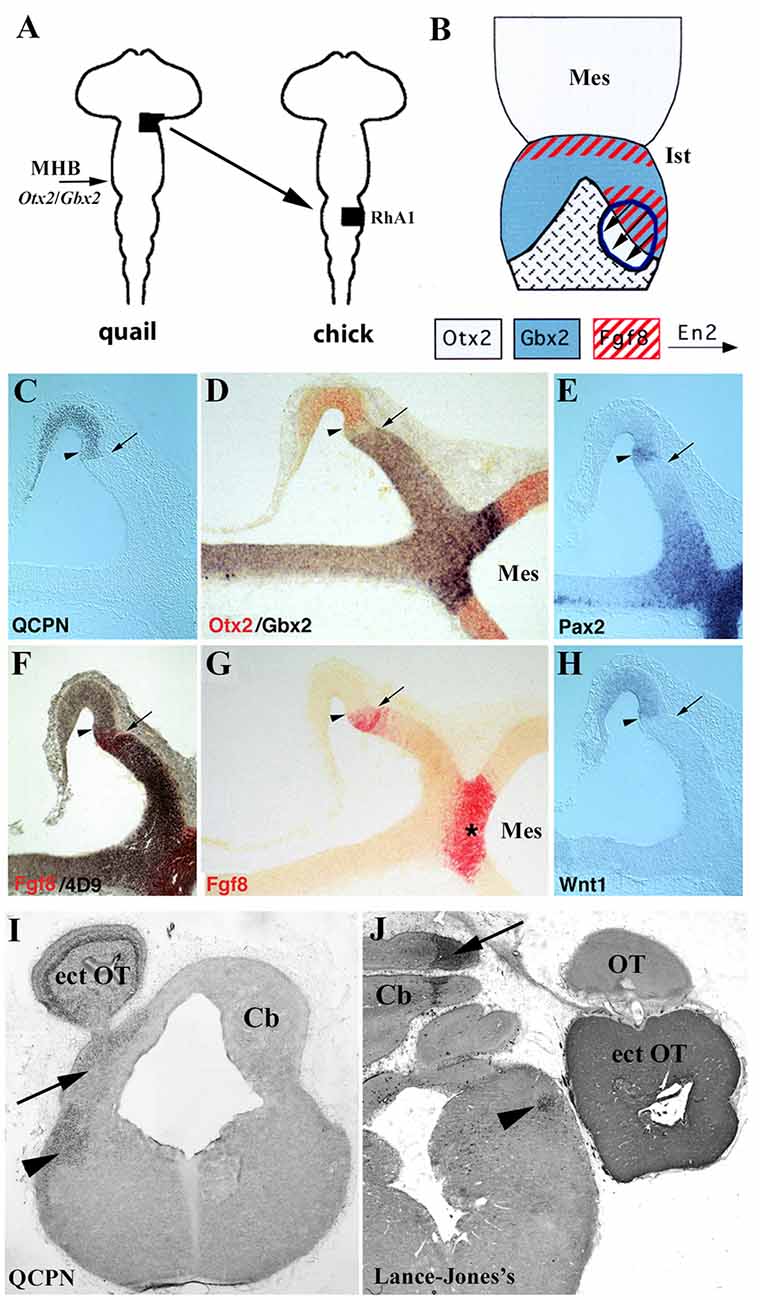
Figure 9. (A) Schematic representation of heterotopic transplant, black areas, of the quail (donor) dp1/dp2 neuroepithelium to the chick (host) pro-rhombomere A1 (RhA1), caudal to the mes/met constriction, performed at stage HH10. (B) Schematic representations of a dorsal view of short survival chimeric embryos. In light gray, Otx2-positive territories in the midbrain, in the choroid tissue, and in the graft. In blue, Gbx2 positive domains. The red, Fgf8-positive territories. The arrows indicate the En2 induction. The graft is outlined by blue lines. A new Otx2/Gbx2 boundary is formed within the graft. (C–H) Serial sagittal sections of a resulting chimeric embryo at stage HH20. The arrows point to the QCPN-positive graft/host interface and the arrowheads to the newly created intragraft Otx2/Gbx2 boundary. Pax2, En2, Fgf8, and Wntexpressions display a pattern on both sides of the intragraft Otx2/Gbx2 boundary similar to that observed in the host MH domain: the Fgf8 and Wnt1 genes are expressed within the Gbx2 and Otx2 domains, respectively, and Pax2 and En2 (4D9 immunoreaction) form double decreasing gradients centered on this intragraft Otx2/Gbx2 boundary. The Fgf8-induced area is undoubtedly separated from the host Fgf8-positive isthmic ring (asterisk in G). (I,J) Frontal sections of two long-survival chimeric embryos treated with QCPN (I) and Lance-Jones’s (J) antibodies, which labeled quail cells from the grafts. The transplants form an ectopic optic tectum (ect OT), also contributing to both in situ isthmus (Ist; arrowheads) and cerebellum (Cb; arrows). Mes, mesencephalon; MHB, midbrain/hindbrain boundary; OT, host optic tectum.
Knock-in Otx2 in En1 domain mice showed an Otx2 overexpression in the En1-positive territory, which includes the prospective anterior hindbrain. In these experimental embryos, a posterior expansion of Otx2 expression into rh1 was observed, whereas Gbx2 expression was downregulated in the ectopic Otx2-expressing territory (Broccoli et al., 1999). Therefore, theOtx2/Gbx2 border changed caudally towards a new position, together with the expression of associated genes (Pax2, Fgf8, and Wnt1). The histological analysis of these En1+/Otx2lacZ transgenic mice showed that the inferior colliculi were enlarged, prolonging caudally, whereas the cerebellum was smaller than in normal conditions with a loss of the anterior vermis and a deficiency of cerebellar midline fusion (Broccoli et al., 1999). In other kinds of experiments combining Gbx2-GOF and EnCre knock-in allele in mice, an ectopic Gbx2 expression was also induced in the midbrain and in the rostralmost portion of the hindbrain (Sunmonu et al., 2009). Unexpectedly, the midbrain and cerebellum were truncated in the most severe phenotype of these EnCre/+; Gbx2-GOF mutant mice (Sunmonu et al., 2009), instead of a predictable enlarged cerebellum and a reduction of the midbrain (Millet et al., 1999; Katahira et al., 2000). Fgf8 expression was significantly reduced to a few clusters of cells in the altered MH domain, which could explain the observed phenotypes (Sunmonu et al., 2009). Therefore, the correct spatiotemporal juxtaposition of Otx2- and Gbx2-expressing territories might be essential for the maintenance of Fgf8 expression and the anterior-to-posterior patterning of the developing MH domain (Sunmonu et al., 2009).
In this line of evidence, ectopic expression of Gbx2 in the caudal midbrain under the control of the Wnt1 enhancer caused the creation of a new shared Otx2/Gbx2 interface positioned more rostral than in wild conditions. Fgf8 and Wnt1 expressions also shifted and adapted to the newly created Otx2/Gbx2 border (Millet et al., 1999). As a consequence, the midbrain was clearly reduced in these Wnt1-Gbx2 transgenic mice at early developmental stages. Remarkably, these genetic changes were transient and the Gbx2 upregulation disappeared at later stages, together with the reestablishment of the Otx2/Gbx2 boundary in its normal position (Millet et al., 1999; see also Li et al., 2005). The changes observed in later stages could be one of the possible reasons by which the adult brains of these mutant mice do not show morphological alterations in the MH domain. In summary, all these words clearly show that both Otx2 and Gbx2 genes cooperate in the establishment of the isthmic organizer position, triggering the inductive events mediated by Fgf8 and Wnt1 signaling pathways (Broccoli et al., 1999; Millet et al., 1999; Li et al., 2005), confirming and extending previously reported results. Gain/loss of function experiments in Xenopus (Tour et al., 2002a, b) and zebrafish (Rhinn et al., 2003, 2009) have added substantial information to the demonstration that most probably Otx2 is sufficient to activate the MH genetic cascade, while Gbx2 restricts and defines the sharp Otx2-expressing domain, positioning the MH boundary in a dosage-dependent manner.
Lmx1b Genes in the Midbrain-Hindbrain Specification
The Lmx1b gene encodes an LIM homeodomain protein with a dynamic expression pattern in the developing central nervous system, including the MH domain (Adams et al., 2000; Liu and Joyner, 2001; Matsunaga et al., 2002; O’Hara et al., 2005; Guo et al., 2007; Kim et al., 2016; Pose-Méndez et al., 2016). In chicks, Lmx1b starts to be expressed immediately after Fgf8 initiation (Adams et al., 2000). At stage HH15–20, Lmxb1 expression is observed in a band located just rostral to the MH boundary, as defined by the caudal Otx2 expression, and in the dorsal and ventral midlines of the developing midbrain. The caudal borders of the Wnt1- and Lmx1b-expressing areas are coincident and abut the Fgf8-positive area (Adams et al., 2000; Matsunaga et al., 2002). Using Fgf8-soaked beads and retroviral-mediated Lmx1b expression (Lmx1b/RCAS), it has been reported that Lmx1b seems to act as an effector of Fgf8 in the regulation of Wnt1 expression (Adams et al., 2000). Thus, Lmxb1 could be both necessary and sufficient to maintain Wnt1 expression in the MH domain, regulating the midbrain morphogenesis (Adams et al., 2000). In another line of evidence, Lmx1b misexpression by in ovo electroporation induced Wnt1 and Otx2 expressions, while Fgf8 expression was repressed (Matsunaga et al., 2002). Ectopic Fgf8 expression was induced around Lmx1b-misexpressing cells. Besides, Otx2 was able to induce Lmx1b expression, whereas Gbx2 repressed it (Matsunaga et al., 2002). In zebrafish, two Lmx1b orthologs, lmx1b.1 and lmx1b.2, have been described, with expression patterns similar to those described in chick embryos (O’Hara et al., 2005). Single and double knockdown of lmx1b.1 and lmx1b.2 have shown that both genes have a redundant function in the specification of the MH domain in fish, these genes being necessary and sufficient for the maintenance of wnt1 and fgf8 expressions. Interestingly, Pax2.1 is required for the correct preservation of lmx1b.1 and lmx1b.2 expression patterns in the developing MH domain in a manner independent of Wnt1 and Fgf8, whereas both lmx1b genes are necessary for pax8 maintenance (O’Hara et al., 2005). All these results clearly suggest that Lmx1b may be involved in the positioning of the Otx2-Wnt1/Gbx2-Fgf8 MH boundary in the Pax2-expressing competent domain.
In mice, the Lmxb1 expression patterns displayed some differences with respect to chicks and fish. In mammals, Lmxb1 expression encompassed the MH boundary, its expressing domain extending to most of the Fgf8-expressing area at the 4-somite stage (Guo et al., 2007). The study of Lmx1b−/− mutant embryos showed that Fgf8 expression in the MH domain was fully absent. Wnt1, En1, and Pax2 expressions were downregulated prior to the 4-somite stage, while Gbx2 expression was downregulated at this developmental stage (Guo et al., 2007). Despite the co-expression of Lmx1b and Otx2 genes in the MH domain, Otx2 expression was not disrupted. This finding suggests that Lmx1b is not involved in the positioning of the MH boundary. As a consequence of this changed genetic network, the tectum and cerebellum of Lmx1b−/− embryos showed strong alterations, with an appreciable reduction in size. The inferior colliculus was completely lost and the reduced cerebellum contacted with the smaller superior colliculus (Guo et al., 2007). In addition, FGF8 can also induce Lmx1b expression in the midbrain explant (Liu and Joyner, 2001). Therefore, Lmx1b could be considered a key factor in the specification of the MH domain in mammals, with a relevant position in the hierarchical genetic cascade governing inductive isthmic activities (Figure 10).
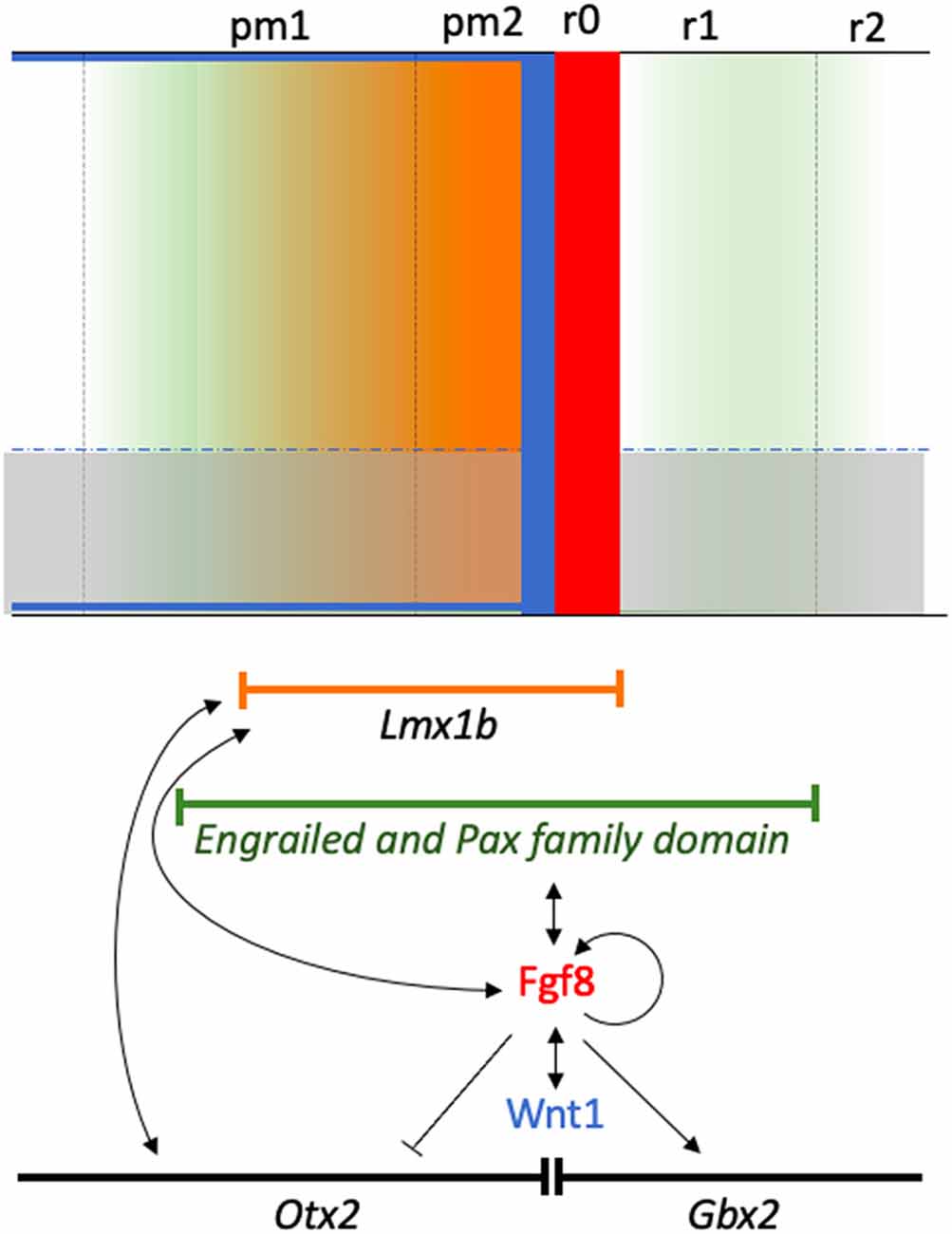
Figure 10. A schematic diagram showing the isthmic neural territory influenced by the morphogenetic activity. The molecular pathways regulating organizer specification are also shown, together with specific local activity associated with the gradient of the signaling molecules (color gradients-color codes). Genetic patterns are represented by their respective symbols inside a lineal sector. Gene interactions are represented by arrows showing the direction of the interaction and inductive effects either positively (arrow) or negatively (no arrow).
The Zebrafish Her5 Gene and Its Vertebrate Orthologous in Patterning and Neurogenesis
The her5 gene encodes a basic helix-loop-helix (bHLH) protein, homolog of the Drosophila hairy-E(spl) family. In zebrafish, her5 expression is observed in the entire presumptive MH domain at gastrulation (Müller et al., 1996; Tallafuß and Bally-Cuif, 2003). After brain morphogenesis, her5 transcriptsare detected in the caudal portion of the developing midbrain, its caudal limit being coincident with the MH boundary (Müller et al., 1996). Studies using transgenic embryos carrying the recombined her5PAC:egfp construct have shown that the spatiotemporal dynamic expression of zebrafish her5 governs neurogenesis progression in a converging manner towards the MH constriction (Tallafuß and Bally-Cuif, 2003). During the growth and regionalization of the developing MH domain in zebrafish embryos, the bHLH Hairy/E(spl)-like factor Her5 accurately defines the “intervening zone” (IZ) as a neuron-free transverse stripe of delayed differentiation (Geling et al., 2003, 2004; Tallafuß and Bally-Cuif, 2003), translating early positional information into accurate neurogenesis and cell proliferation in the developing MH domain in collaboration with other E(spl) factors in a dose-dependent manner (Sieger et al., 2004; Ninkovic et al., 2005, 2008; Chapouton et al., 2006; Webb et al., 2011; Galant et al., 2016).
It is worth noting that Her5 does not affect other aspects of the MH patterning such as the expression patterns of MH markers (pax2.1, eng2, eng3, wnt1, and fgf8), her5 being necessary for the maintenance of the integrity of the MH domain but not for the establishment and early maintenance of the MH domain (Geling et al., 2003). In zebrafish noi mutant, affecting the pax2.1 gene, her5 expression occurs normally (Lun and Brand, 1998). In acerebellar mutant embryos, a zebrafish Fgf8 mutation, her5 decreases by the 5-somite stage, being absent at the 18-somite stage, suggesting that, similar to wnt1 and pax2.1, Her5 requires Fgf8 activity during the maintenance phase of MH development (Reifers et al., 1998). When FGF action decreases along the anterior-to-posterior axis of the developing midbrain as a consequence of the growth of the MH region and an increased distance from the isthmic source of FGF, a posterior retraction of her5 expression towards the isthmus is detected, together with a loss of Her5-mediated repression of neuronal differentiation (Dyer et al., 2014).
Concerning the WNT signaling pathway, the zebrafish Dfw5 mutant embryos display deletions in wnt1 and wnt10b genes (Lekven et al., 2003). In Dfw5 embryos, zebrafish her5 expression started to be reduced at the 12-somite stage, its expression being undetectable in the ventral portion of the MH domain by 24 hpf (Lekven et al., 2003). Interestingly, pax2.1 and en2 expressions were also lost where her5 was absent in Dfw5 embryos, whereas fgf8 and en3 expressions, as well as those of wnt8b and wnt3a genes, were not disturbed. Thus, either wnt1 or wnt10b seems to be necessary and sufficient for pax2, en3, and her5 maintenance, but not for fgf8 and en3 conservation (Lekven et al., 2003; Green et al., 2020). However, pax2.1 may also play a relevant role in the her5 regulation (Lun and Brand, 1998).
It is interesting to remark that, in the MH domain, the her5-expressing domain forms a sharp border with the gbx2-expressing domain, both areas being closely complementary (Nakayama et al., 2017). In gbx1/gbx2 double homozygotes, her5 expression expands caudally, which suggests that her5 could be repressed by gbx1 and gbx2 in the rostral-most hindbrain. Also, her5 expression was downregulated when gbx2 was overexpressed using mRNA microinjections. Thus, her5 could participate in positioning of the otx2/gbx2 MH boundary (Nakayama et al., 2017). Because her5 negatively regulates neural differentiation in the caudal midbrain, gbx2 could also promote neurogenesis in the anterior hindbrain by excluding her5 expression (Nakayama et al., 2017).
Studies of mouse Hes genes, orthologous of zebrafish her genes, have also reported an evident role of these genes in early midbrain and hindbrain patterning (Lobe, 1997). In E12.5 mice, Hes3 expression is detected in the caudal midbrain, its expressing domain overlapping the Wn1-positive area and abutting the Fgf8-positive domain (Hirata et al., 2001). In Hes1-Hes3 double-mutant embryos, which showed a tendency of growth retardation, the midbrain and the rostral-most portion of the hindbrain are absent. The locus ceruleus (r1) and cranial nerve III and IV were also missing in the double mutant, whereas more caudal cranial nerves (V, VII, IX, and X) were present (Hirata et al., 2001). In these mutant mice, the isthmic organizer cells prematurely differentiate into neurons, together with the progressive loss of expression of MH markers, such as Fgf8, Wnt1, and Pax2/5 (Hirata et al., 2001). Besides, loss of FGF activity in the ventral midbrain caused a loss of Hes1 (Lahti et al., 2011). This finding clearly confirms that Hes1 and Hes3 could cooperate to avoid too early differentiation and preserve the isthmic regulatory activities over time (Hirata et al., 2001), probably participating, directly or indirectly, with additional genes in the intricate network of the isthmic organizer. Since Hes1 expression is activated in Wnt1/PC12 cells, which display a block of differentiation and an increase in proliferation, Hes1 could be a possible target of the long-term Wnt1 signaling pathway (Issack and Ziff, 1998).
In chick embryos, Hes5 transcripts were firstly detected in the rostral midbrain at stage 14, where En2 expression is not strong (Kimura et al., 2004). When development proceeds, Hes5 expression expanded to the caudal mesencephalon at stage HH20, the isthmic region being clearly Hes5 negative. As expected, misexpression of En2 downregulated Hes5 expression in the mesencephalon. In addition, Hes5 expression was induced in the isthmus when the siRNA expression vector was used to silence En2. Thus, the chick Hes5 gene could be regulated by En proteins to determine the anterior-to-posterior polarity of the midbrain (Kimura et al., 2004).
The XenopusHES-related 1 (XHR1) gene encodes a protein homologous to members of the HES family, very close to zebrafish Her5 (Shinga et al., 2001). XHR1 expression was detected in the presumptive MH domain in Xenopus early gastrula stage (Shinga et al., 2001; Takada et al., 2005; Takahashi et al., 2005), much earlier than XPax-2, En-2, Xotx2, and Xgbx2 (Shinga et al., 2001). At tailbud stage, XFGF-8 expression was included in the XHR1-expressing domain. Interestingly, ectopic expression of XHR1 in the MH domain enhanced En-2 expression, whereas overexpression of XHR1 dominant-negative forms caused an evident reduction of XPax-2 and En-2 expression (Shinga et al., 2001).
POU-Domain Transcription Factor Pou2 in the MH Specification
The Pou2 gene encodes a POU-domain transcription factor. spiel ohne grenzen (spg) zebrafish mutants, which carry loss-of-function mutations in the pou2 gene and display alterations in hindbrain segmentation (Hauptmann et al., 2002). In zebrafish spg/pou2 mutants, the MH boundary is not correctly established (Schier et al., 1996), with an absence of isthmus and cerebellum, the midbrain being reduced in size (Belting et al., 2001). In these spg embryos, the trochlear nerve is absent (Reim and Brand, 2002). Regarding the MH genetic cascade, the complementary expression of otx2 and gbx1 is normal in spg mutant embryos, suggesting that the pou2 gene is not crucial in positioning the MH boundary. However, it was reported that gbx2 could be dependent on spg function (Rhinn et al., 2003). The initial expression of pax2.1, wnt1, her5, and eng1/2/3 are severely reduced in spg mutants. In an interesting way, Fgf8 and Wnt1 signaling activities are activated normally but become dependent onspg at the end of gastrulation (Belting et al., 2001; Reim and Brand, 2002). A more severe mutant (spgm793) displays a complete absence of fgf8 expression in the isthmus at 24 hpf (Belting et al., 2001). These results suggest thatspg/pou2 could intervene in generating regional competence to respond to Fgf8 activity (Belting et al., 2001; Reim and Brand, 2002). However, pou2 gene is expressed correctly in noi (pax2.1) and ace (fgf8) zebrafish mutants, suggesting that pou2 does not depend on fgf8 and pax2.1 expression (Belting et al., 2001). Nevertheless, the pou2 could be necessary for the establishment of the pax2.1-expressing area in the developing MH domain in a permissive manner (Belting et al., 2001; Burgess et al., 2002). Interestingly, the pou5f3/pou2 gene, closely related to Oct4/pou5f1, is repressed by gbx2 around the MH domain (Nakayama et al., 2017), being involved in neurogenesis (Inomata et al., 2020).
In mice, the Oct3/4 (Pou5f1) gene, ortholog of the zebrafish pou2 gene (Burgess et al., 2002), encodes a protein which is able to bind to a functional POU recognition sequence present in the Pax2 enhancer, suggesting a direct control of Pax2 expression by one or more members of the POU protein family (Pfeffer et al., 2002). Overexpression of Oct4 in the neuroectoderm causes a strong upregulation of Pax2, suggesting that Oct4 can regulate Pax2 during MH development (Ramos-Mejía et al., 2005). A higher dosage of Oct4 also results in a transient alteration of MH patterning. Thus, En2 was downregulated at E8,5, its levels of expression being recovered at E9,5. This En2 alteration could be due to Pax proteins, partly disagreeing with the fact that Pax proteins are involved in the positive regulation of En2 expression in the developing MH domain (Song et al., 1996). Other regulatory factors should be considered to explain this finding (Parvin et al., 2008). None of the key regulatory factors of the isthmic organizer (Otx2, Gbx2, Fgf8, and Wnt1) displayed an altered expression pattern in the dorsal portion of the MH domain. As a consequence, the gross MH morphology was not altered (Ramos-Mejía et al., 2005).
bTHS1 and Grainy Head-Like Genes as Additional Regulatory Factors
During zebrafish gastrulation, the bts1 gene, a btd/Sp1-related gene, is expressed in the presumptive MH domain before her5, pax2.1, wnt1, and eng2, overlapping the her5-expressing area and slightly crossing the otx2 border (Tallafuß et al., 2001). Gain- and loss-of-function experiments have clearly reported that Bts1 is necessary and sufficient for the inductions of pax2.1, not being involved in regulating the expression of the other molecular elements of the isthmic genetic cascade, including fgf8. Although bts1 expression was never completely eliminated in the lack of Fgf activity, it probably requires in part the Fgf8 action. Thus, Bts1 seems to be an early upstream component of the inductive pathway leading to the specific induction of the pax2.1 gene from the mid-gastrulation stages (Tallafuß et al., 2001).
The grainy head-like (Grhl) family of transcription factors are key regulatory elements in several developmental events (Rifat et al., 2010). The zebrafish grhl2b and grhl3 genes are directly involved in the specification and morphogenesis of the MH domain (Dworkin et al., 2012; Miles et al., 2017), probably by means of dissociable events through diverse transcriptional targets (Dworkin et al., 2012). Loss of grhl2b expression causes no alteration of otx2 and gbx1 expression, suggesting that grhl2b is not involved in positioning the MH boundary. However, the expression of MH markers such as eng2a, wnt1, pax2a, and her5 was lost by 26 hpf, but not before. These results strongly suggest that grhl2b participates in the maintenance of the MH domain at both structural and molecular levels. Interestingly, the normal expression patterns of these genes were recovered by re-expression of eng2a in grhl2b morphants (Dworkin et al., 2012), eng2a being a target of the Grh family (Wilanowski et al., 2002). In this sense, grhl2b and fgf8 seem to cooperate to govern the specification and morphogenesis of the MH domain in zebrafish, grhl2b probably acting downstream of fgf8, through eng2a (Dworkin et al., 2012).
Lineage Restriction at the MH Domain
Descriptive and experimental studies suggested the existence of lineage segregation at the mid- hindbrain junction in birds. Using Otx2 riboprobe and tissue grafting experiments concerning the formation of the sharp MH boundary, Millet and colleagues showed that the MH constriction prevents the intermingling of midbrain and isthmic cells (Millet et al., 1996). However, more recent experiments in chicks conducted by cell labeling experiments in ovo at stages HH11 and HH13 showed that the midbrain and anterior hindbrain could not be separated by cell lineage restriction, with stained cells moving between both tagmas (Jungbluth et al., 2001). A restriction of DiI-crystal labeled cells was observed exclusively in the roof plate of the MH domain (Alexandre and Wassef, 2003; Louvi et al., 2003). In clear contrast, the posterior border of the midbrain Lrrn1 expression was proposed again to present cell restriction properties; disturbing this compartment-restricted border by ectopical Lrrn1 expression in the isthmus provoked an alteration of MH organizer genes and a mix of cells from two separated compartments (Tossell et al., 2011a). This Lrrn1 activity would be mediated by Notch signaling pathways through regulation of the posterior border of LFng and Ser1 expressions (Tossell et al., 2011b).
In mice, the nature of the caudal midbrain and isthmus, i.e., the MH (Otx2/Gbx2) interface, as entities separated by a lineage restriction boundary was demonstrated using a Wnt1-CreERT approach as long-term fate mapping, the Wnt1-CreERT-stained cells being registered with Otx2 expression (Zervas et al., 2004). These findings were confirmed later when a transgenic mouse line MHB-Cre was analyzed, in which a small group of cells was stained at E10.5 next to the caudal border of Otx2 expression (Kala et al., 2008). Other studies using a Gbx2CreER knock-in line in mice as a genetic inducible fate mapping showed without any doubt that the Otx2/Gbx2 MH boundary separates proliferating progenitors of the considered contiguous neuromeres, theGbx2-labeled progenies not migrating through it at E7.5 (Sunmonu et al., 2011). In the formation of this lineage boundary, Fgf8 plays an essential role too; its partial deletion disordered the local organization of cell populations, promoting movements across the MH boundary (Sunmonu et al., 2011). In this sense, Wnt1-Cre/+; Fgfr1flox/Δflox embryos showed that the posterior border of the Otx2-expressing domain and the anterior border of the Gbx2- and Fgf8-expressing domain were significantly altered with a mixture of midbrain and hindbrain cells at this interface (Trokovic et al., 2003). In the analyzed Fgfr1 mutants, Wnt1-positive cells also appear also to mix with Wnt1-negative cells, a cellular event in which PB-cadherin seems to be involved (Kitajima et al., 1999; Trokovic et al., 2003).
Similar evidence of accurate neuroepithelial patterning and cell differentiation in the MH domain were also reported in the zebrafish MH domain. By staining live embryos with vital dyes and matching the positions of hundreds of stained nuclei within the Otx2-labeled domain, it was plainly demonstrated that midbrain and hindbrain (isthmic) cells generate from two separate cell populations during late gastrulation stages (Langenberg and Brand, 2005). Using innovative methodological approaches with several transgenic reporter fish involving MH markers and live imaging, among others, also showed that the lineage restriction taking place along the MH junction is controlled by differential cell-cell adhesion properties in which N-cadherin and Eph-ephrin signaling or even Cx43 based Gap junction might clearly be involved (Bosone et al., 2016; Kesavan et al., 2017, 2018, 2020). Considering all these works, the existence of lineage segregation at the mid- hindbrain junction is confirmed.
Future Perspectives
In the present review, we provide tons of evidence in which different levels of transcription factors together with signaling molecules are orchestrated and are required to properly develop the specific regions within the embryonic midbrain and cerebellum. It has been also demonstrated that such differences in level and created gradients may underlie the anatomical variations between species. Future studies may reveal and relate the proper levels of these signaling molecules to build different brain regions in lower vertebrate brains. Moreover, we are far from understanding the precise spread mechanisms of these signaling molecules and the exact mechanism of cell-cell communication to acquire specific positional information and proper polarity in order to be a particular brain nucleus and how this communication between embryonic cells in a particular region is propagated.
Author Contributions
MH-S and DE conceived the research. All authors contributed to the article and approved the submitted version.
Funding
This work was supported by Junta de Extremadura, Grant/Award numbers: GR18114 (MH-S and SV-C); Junta de Extremadura, Fondo Europeo de Desarrollo Regional, “Una manera de hacer Europa”, Grant/Award Number: IB18046 (MH-S and SV-C). MINECO/AEI/FEDER (SAF2017-83702-R and PID2020-118171RB-I00), GVA (PROMETEO/2018/041), TerCel ISCIII (RD16/001/0010; DE and AA-C). Co-funded by the ERDF/ESF, “Investing in your future,” and FTPGB (FTPGB18/SMP and The Institute of Neurosciences “Centre of Excellence Severo Ochoa”; SEV-2017-0723; DE and AA-C).
Conflict of Interest
The authors declare that the research was conducted in the absence of any commercial or financial relationships that could be construed as a potential conflict of interest.
Publisher’s Note
All claims expressed in this article are solely those of the authors and do not necessarily represent those of their affiliated organizations, or those of the publisher, the editors and the reviewers. Any product that may be evaluated in this article, or claim that may be made by its manufacturer, is not guaranteed or endorsed by the publisher.
Acknowledgments
We thank the members of our scientific groups for helpful discussions.
References
Acampora, D., Avantaggiato, V., Tuorto, F., Barone, P., Perera, M., Choo, D., et al. (1999). Differential transcriptional control as the major molecular event in generating Otx1−/− and Otx2−/− divergent phenotypes. Development 126, 1417–1426. doi: 10.1242/dev.126.7.1417
Acampora, D., Avantaggiato, V., Tuorto, F., Briata, P., Corte, G., and Simeone, A. (1998). Visceral endoderm-restricted translation of Otx1 mediates recovery of Otx2 requirements for specification of anterior neuralplate and normal gastrulation. Development 125, 5091–5104. doi: 10.1242/dev.125.24.5091
Acampora, D., Avantaggiato, V., Tuorto, F., and Simeone, A. (1997). Genetic control of brain morphogenesis through Otx gene dosage requirement. Development 124, 3639–3650. doi: 10.1242/dev.124.18.3639
Acampora, D., Gulisano, M., Broccoli, V., and Simeone, A. (2001). Otx genes in brain morphogenesis. Progr. Neurobiol. 64, 69–95. doi: 10.1016/s0301-0082(00)00042-3
Acampora, D., Mazan, S., Lallemand, Y., Avantaggiato, V., Maury, M., Simeone, A., et al. (1995). Forebrain and midbrain regions are deleted in Otx2−/− mutants due to a defective anterior neuroectoderm specification during gastrulation. Development 121, 3279–3290. doi: 10.1242/dev.121.10.3279
Adams, K. A., Maida, J. M., Golden, J. A., and Riddle, R. D. (2000). The transcription factor Lmx1b maintains Wnt1 expression within the isthmic organizer. Development 127, 1857–1867. doi: 10.1242/dev.127.9.1857
Alexandre, P., and Wassef, M. (2003). The isthmic organizer links anteroposterior and dorsoventral patterning in the mid/hindbrain by generating roof plate structures. Development 130, 5331–5338. doi: 10.1242/dev.00756
Alonso, A., Merchán, P., Sandoval, J. E., Sánchez-Arrones, L., Garcia-Cazorla, A., Artuch, R., et al. (2013). Development of the serotonergic cells in murine raphe nuclei and their relations with rhombomeric domains. Brain Struct. Funct. 218, 1229–1277. doi: 10.1007/s00429-012-0456-8
Alvarado-Mallart, R.-M. (1993). Fate and potentialities of the avian mesencephalic/metencephalic neuroepithelium. J. Neurobiol. 24, 1341–1355. doi: 10.1002/neu.480241007
Alvarado-Mallart, R.-M. (1997). The chick/quail chimeric system: a model for early cerebellar development. Perspect. Dev. Neurobiol. 5, 17–31.
Alvarado-Mallart, R.-M. (2000). “The chick/quail transplantation model to study central nervous system development,” in Functional Neural Transplantation II. Novel Cell Therapies For CNS Disorders. Progress in Brain Research, Vol. 127, eds S. B. Dunnett, and A. Bjorklund (Elsevier B.V.), 67–98, doi: 10.1016/S0079-6123(00)27006-4
Alvarado-Mallart, R.-M., and Sotelo, C. (1984). Homotopic and heterotopic transplantations of quail tectal primordia in chick embryos: organization of the retinotectal projections in the chimeric embryos. Dev. Biol. 103, 378–398. doi: 10.1016/0012-1606(84)90326-9
Alvarado-Mallart, R.-M., Martinez, S., and Lance-Jones, C. C. (1990). Pluripotentiality of the 2-day-old avian germinative neuroepithelium. Dev. Biol. 139, 75–88. doi: 10.1016/0012-1606(90)90280-v
Alvarez-Otero, R., Sotelo, C., and Alvarado-Mallart, R.-M. (1993). Chick/quail chimeras with partial cerebellar grafts: an analysis of the origin and migration of cerebellar cells. J. Comp. Neurol. 333, 597–615. doi: 10.1002/cne.903330411
Ang, S. L., Jin, O., Rhinn, M., Daigle, N., Stevenson, L., and Rossant, J. (1996). A targeted mouse Otx2 mutation leads to severe defects in gastrulation and formation of axial mesoderm and to deletion of rostral brain. Development 122, 243–252. doi: 10.1242/dev.122.1.243
Araki, I., and Brand, M. (2001). Morpholino-induced knockdown of Fgf8 efficiently phenocopies the acerebellar (ACE) phenotype. Genesis 30, 157–159. doi: 10.1002/gene.1054
Araki, I., and Nakamura, H. (1999). Engrailed defines the position of dorsal di-mesencephalic boundary by repressing diencephalic fate. Development 126, 5127–5135. doi: 10.1242/dev.126.22.5127
Aroca, P., Lorente-Cánovas, B., Mateos, F. R., and Puelles, L. (2006). Locus coeruleus neurons originate in alar rhombomere 1 and migrate into the basal plate: studies in chick and mouse embryos. J. Comp. Neurol. 496, 802–818. doi: 10.1002/cne.20957
Aroca, P., and Puelles, L. (2005). Postulated boundaries and differential fate in the developing rostral hindbrain. Brain Res. Rev. Res. Rev. 49, 179–190. doi: 10.1016/j.brainresrev.2004.12.031
Asano, M., and Gruss, P. (1992). Pax-5 is expressed at the midbrain-hindbrain boundary during mouse development. Mech. Dev. 39, 29–39. doi: 10.1016/0925-4773(92)90023-d
Bally-Cuif, L., Alvarado-Mallart, R.-M., Darnell, D. K., and Wassef, M. (1992). Relationship between Wnt-1 and En-2 expression domains during early development of normal and ectopic met-mesencephalon. Development 115, 999–1009. doi: 10.1242/dev.115.4.999
Bally-Cuif, L., Gulisano, M., Broccoli, V., and Boncinelli, E. (1995). c-otx2 is expressed in two different phases of gastrulation and is sensitive to retinoic acid treatment in chick embryo. Mech. Dev. 49, 49–63. doi: 10.1016/0925-4773(94)00301-3
Bally-Cuif, L., and Wassef, M. (1994). Ectopic induction and reorganization of Wnt-1 expression in quail/chick chimeras. Development 120, 3379–3394. doi: 10.1242/dev.120.12.3379
Basson, M. A., Echevarria, D., Petersen Ahn, C., Sudarov, A., Joyner, A. L., Mason, I. J., et al. (2008). Specific regions within the embryonic midbrain and cerebellum require different levels of FGF signaling during development. Development 135, 889–898. doi: 10.1242/dev.011569
Belting, H. G., Hauptmann, G., Meyer, D., Abdelilah-Seyfried, S., Chitnis, A., Eschbach, C., et al. (2001). spiel ohne grenzen/pou2 is required during establishment of the zebrafish midbrain-hindbrain boundary organizer. Development 128, 4165–4176. doi: 10.1242/dev.128.21.4165
Bilovocky, N. A., Romito-DiGiacomo, R. R., Murcia, C. L., Maricich, S. M., and Herrup, K. (2003). Factors in the genetic background suppress the Engrailed-1 cerebellar phenotype. J. Neurosci. 23, 5105–5112. doi: 10.1523/JNEUROSCI.23-12-05105.2003
Blak, A. A., Naserken, T., Saarimäki-Vire, J., Peltopuro, P., Giraldo-Velasquez, M., Weisenhorn, D. M. V., et al. (2007a). Fgfr2 and Fgfr3 are not required for patterning and maintenance of the midbrain and anterior hindbrain. Dev. Biol 303, 231–243. doi: 10.1016/j.ydbio.2006.11.008
Blak, A. A., Naserken, T., Weisenhorn, D. M. V., Prakash, N., Partanen, J., and Wurst, W. (2007b). Expression of Fgf receptors 1, 2 and 3 in the developing Mid- and hindbrain of the mouse. Dev. Dyn. 233, 1023–1030. doi: 10.1002/dvdy.20386
Bloch-Gallego, E., Millet, S., and Alvarado-Mallart, R.-M. (1996). Further observations on the susceptibility of diencephalic prosomeres to En-2 induction and on the resulting histogenetic capabilities. Mech. Dev. 58, 51–63. doi: 10.1016/s0925-4773(96)00557-6
Bosone, C., Andreu, A., and Echevarria, D. (2016). GAP junctional communication in brain secondary organizers. Dev. Growth Differ. 58, 446–455. doi: 10.1111/dgd.12297
Bouchard, M., Grote, D., Craven, S. E., Sun, Q., Steinlein, P., and Busslinger, M. (2005). Identification of Pax2-regulated genes by expression profiling of the mid-hindbrain organizer region. Development 132, 2633–2643. doi: 10.1242/dev.01833
Bouillet, P., Chazaud, C., Oulad-Abdelghani, M., Dollé, P., and Chambon, P. (1995). Sequence and expression pattern of the Stra7 (Gbx-2) homeobox-containing gene induced by retinoic acid in P19 embryonal carcinoma cells. Dev. Dyn. 204, 372–382. doi: 10.1002/aja.1002040404
Brand, M., Heisenberg, C. P., Jiang, Y. J., Beuchle, D., Lun, K., Furutani-Seiki, M., et al. (1996). Mutations in zebrafish genes affecting the formation of the boundary between midbrain and hindbrain. Development 123, 179–190. doi: 10.1242/dev.123.1.179
Broccoli, V., Boncinelli, E., and Wurst, W. (1999). The caudal limit of Otx2 expression positions the isthmic organizer. Nature 401, 164–168. doi: 10.1038/43670
Buckles, G. R., Thorpe, C. J., Ramel, M.-C., and Lekven, A. C. (2004). Combinatorial Wnt control of zebrafish midbrain-hindbrain boundary formation. Mech. Dev. 121, 437–447. doi: 10.1016/j.mod.2004.03.026
Bueno, D., Skinner, J., Abud, H., and Heath, J. K. (1996). Spatial and temporal relationships between Shh, Fgf4 and Fgf8 gene expression at diverse signalling centers during mouse development. Dev. Dyn. 207, 291–299. doi: 10.1002/(SICI)1097-0177(199611)207:3<291::AID-AJA6>3.0.CO;2-C
Bulfone, A., Puelles, L., Porteus, M., Frohman, M., Martin, G., and Rubenstein, J. (1993). Spatially restricted expression of Dlx-1, Dlx-2 (Tes-1), Gbx-2 and Wnt- 3 in the embryonic day 12.5 mouse forebrain defines potential transverse and longitudinal segmental boundaries. J. Neurosci. 13, 3155–3172. doi: 10.1523/JNEUROSCI.13-07-03155.1993
Burgess, S., Reim, G., Chen, W., Hopkins, N., and Brand, M. (2002). The zebrafish spiel-ohne-grenzen (spg) gene encodes the POU domain protein Pou2 related to mammalian Oct4 and is essential for formation of the midbrain and hindbrain and for pre-gastrula morphogenesis. Development 129, 905–916. doi: 10.1242/dev.129.4.905
Burroughs-Garcia, J., Sittaramane, V., Chandrasekhar, A., and Waters, S. T. (2011). Evolutionarily conserved function of Gbx2 in anterior hindbrain development. Dev. Dyn. 240, 828–838. doi: 10.1002/dvdy.22589
Canning, C. A., Lee, L., Irving, C., Mason, I., and Jones, C. M. (2007). Sustained interactive Wnt and FGF signaling is required to maintain isthmic identity. Dev. Biol. 305, 276–286. doi: 10.1016/j.ydbio.2007.02.009
Castro, L. F. C., Rasmussen, S. L. K., Holland, P. W. H., Holland, N. D., and Holland, L. Z. (2006). A Gbx homeobox gene in amphioxus: insights into ancestry of the ANTP class and evolution of the midbrain/hindbrain boundary. Dev. Biol. 295, 40–51. doi: 10.1016/j.ydbio.2006.03.003
Chapouton, P., Adolf, B., Leucht, C., Tannhäuser, B., Ryu, S., Driever, W., et al. (2006). her5 expression reveals a pool of neural stem cells in the adult zebrafish midbrain. Development 133, 4293–4303. doi: 10.1242/dev.02573
Cheng, Y., Sudarov, A., Szulc, K. U., Sgaier, S. K., Stephen, D., Turnbull, D. H., et al. (2010). The Engrailed homeobox genes determine the different foliation patterns in the vermis and hemispheres of the mammalian cerebellum. Development 137, 519–529. doi: 10.1242/dev.027045
Chi, C. L., Martinez, S., Wurst, W., and Martin, G. R. (2003). The isthmic organizer signal FGF8 is required for cell survival in the prospective midbrain and cerebellum. Development 130, 2633–2644. doi: 10.1242/dev.00487
Christen, B., and Slack, J. M. W. (1997). FGF-8 is associated with anteroposterior patterning and limb regeneration in Xenopus. Dev. Biol. 192, 455–466. doi: 10.1006/dbio.1997.8732
Crespo-Enriquez, I., Partanen, J., Martinez, S., and Echevarria, D. (2012). Fgf8-related secondary organizers exert different polarizing planar instructions along the mouse anterior neural tube. PLoS One 7:e39977. doi: 10.1371/journal.pone.0039977
Crossley, P. H., and Martin, G. R. (1995). The mouse Fgf8 gene encodes a family of polypeptides and is expressed in regions that direct outgrowth and patterning in the developing embryo. Development 121, 439–451. doi: 10.1242/dev.121.2.439
Crossley, P. H., Martinez, S., and Martin, G. R. (1996). Midbrain development induced by FGF8 in the chick embryo. Nature 380, 66–68. doi: 10.1038/380066a0
Danielian, P. S., and McMahon, A. P. (1996). Engrailed-1 as a target of the Wnt-1 signalling pathway in vertebrate midbrain development. Nature 383, 332–334. doi: 10.1038/383332a0
Davidson, D., Graham, E., Sime, C., and Hill, R. (1988). A gene with sequence similarity to Drosophila engrailed is expressed during the development of the neural tube and vertebrae in the mouse. Development 104, 305–316. doi: 10.1242/dev.104.2.305
Davis, C. A., Holmyard, D. P., Millen, K. J., and Joyner, A. L. (1991). Examining pattern formation in mouse, chicken and frog embryos with an En-specific antiserum. Development 111, 287–298. doi: 10.1242/dev.111.2.287
Davis, C. A., and Joyner, A. L. (1988). Expression patterns of the homeo box-containing genes En-1 and En-2 and the proto-oncogene int-1 diverge during mouse development. Genes Dev. 2, 1736–1744. doi: 10.1101/gad.2.12b.1736
Delgado, I., and Torres, M. (2017). Coordination of limb development by crosstalk among axial patterning pathways. Dev. Biol. 429, 382–386. doi: 10.1016/j.ydbio.2017.03.006
Dworkin, S., Darido, C., Georgy, S. R., Wilanowski, T., Srivastava, S., Ellett, F., et al. (2012). Midbrain-hindbrain boundary patterning and morphogenesis are regulated by diverse grainy head-like 2-dependent pathways. Development 139, 525–536. doi: 10.1242/dev.066522
Dyer, C., Blanc, E., Hanisch, A., Roehl, H., Otto, G. W., Yu, T., et al. (2014). A bi-modal function of Wnt signalling directs an FGF activity gradient to spatially regulate neuronal differentiation in the midbrain. Development 141, 63–72. doi: 10.1242/dev.099507
Echevarria, D., Belo, J. A., and Martinez, S. (2005). Modulation of Fgf8 activity during vertebrate brain development. Brain Res. Rev. 49, 150–157. doi: 10.1016/j.brainresrev.2004.12.035
Ekker, M., Wegner, J., Akimenko, M. A., and Westerfield, M. (1992). Coordinate embryonic expression of three zebrafish engrailed genes. Development 116, 1001–1010. doi: 10.1242/dev.116.4.1001
Favor, J., Sandulache, R., Neuhauser-Klaus, A., Pretsch, W., Chatterjee, B., Senft, E., et al. (1996). The mouse Pax21Neu mutation is identical to a human PAX2 mutation in a family with renal-coloboma syndrome and results in developmental defects of the brain, ear, eye and kidney. Proc. Natl. Acad. Sci. U S A 93, 13870–13875. doi: 10.1073/pnas.93.24.13870
Finkelstein, R., and Boncinelli, E. (1994). From fly head to mammalian forebrain: the story of otd and Otx. Trends Genet. 10, 310–315. doi: 10.1016/0168-9525(94)90033-7
Foucher, I., Mione, M., Simeone, A., Acampora, D., Bally-Cuif, L., and Houart, C. (2006). Differentiation of cerebellar cell identities in absence of Fgf signalling in zebrafish Otx morphants. Development 133, 1891–1900. doi: 10.1242/dev.02352
Friedman, G. C., and O’Leary, D. D. M. (1996). Retroviral misexpression of engrailed genes in the chick optic tectum perturbs the topographic targeting of retinal axons. J. Neurosci. 16, 5498–5509. doi: 10.1523/JNEUROSCI.16-17-05498.1996
Friesel, R., and Brown, S. A. (1992). Spatially restricted expression of fibroblast growth factor receptor-2 during Xenopus development. Development 116, 1051–1058. doi: 10.1242/dev.116.4.1051
Funahashi, J., Okafuji, T., Ohuchi, H., Noji, S., Tanaka, H., and Nakamura, H. (1999). Role of Pax-5 in the regulation of a mid-hindbrain organizer’s activity. Dev. Growth Differ. 41, 59–72. doi: 10.1046/j.1440-169x.1999.00401.x
Galant, S., Furlan, G., Coolen, M., Dirian, L., Foucher, I., and Bally-Cuif, L. (2016). Embryonic origin and lineage hierarchies of the neural progenitor subtypes building the zebrafish adult midbrain. Dev. Biol. 420, 120–135. doi: 10.1016/j.ydbio.2016.09.022
Garda, A. L., Echevarría, D., and Martínez, S. (2001). Neuroepithelial co-expression of Gbx2 and Otx2 precedes Fgf8 expression in the isthmic organizer. Mech. Dev. 101, 111–118. doi: 10.1016/s0925-4773(00)00567-0
Gardner, C. A., and Barald, K. F. (1991). The cellular environment controls the expression of engrailed-like protein in the cranial neuroepithelium of quail-chick chimeric embryos. Development 113, 1037–1048. doi: 10.1242/dev.113.3.1037
Gardner, C. A., Darnell, D. K., Poole, S. J., Ordahl, C. P., and Barald, K. F. (1988). Expression of anengrailed-like gene during development of the early embryonic chick nervous system. J. Neurosci. Res. 21, 426–437. doi: 10.1002/jnr.490210234
Geling, A., Itoh, M., Tallafuβ, A., Chapouton, P., Tannhäuser, B., Kuwada, J. Y., et al. (2003). bHLH transcription factor Her5 links patterning to regional inhibition of neurogenesis at the midbrain-hindbrain boundary. Development 130, 1591–1604. doi: 10.1242/dev.00375
Geling, A., Plessy, C., Rastegar, S., Strähle, U., and Bally-Cuif, L. (2004). Her5 acts as a prepattern factor that blocks neurogenin1 and coe2 expression upstream of Notch to inhibit neurogenesis at the midbrain-hindbrain boundary. Development 131, 1993–2006. doi: 10.1242/dev.01093
Goode, D. K., and Elgar, G. (2009). The PAX258 gene subfamily: a comparative perspective. Dev. Dyn. 238, 2951–2974. doi: 10.1002/dvdy.22146
Grapin-Botton, A., Cambronero, F., Weiner, H. L., Bonnin, M.-A., Puelles, L., and Le Douarin, N. M. (1999). Patterning signals acting in the spinal cord override the organizing activity of the isthmus. Mech. Dev. 84, 41–53. doi: 10.1016/s0925-4773(99)00069-6
Green, D. G., Whitener, A. E., Mohanty, S., Mistretta, B., Gunaratne, P., Yeh, A. T., et al. (2020). Wnt signaling regulates neural plate patterning in distinct temporal phases with dynamic transcriptional outputs. Dev. Biol. 462, 152–164. doi: 10.1016/j.ydbio.2020.03.016
Guo, C., Qiu, H. Y., Huang, Y. H., Chen, H., Yang, R. Q., Chen, S. D., et al. (2007). Lmx1b is essential for Fgf8 and Wnt1 expression in the isthmic organizer during tectum and cerebellum development in mice. Development 134, 317–325. doi: 10.1242/dev.02745
Hallonet, M. E., Teillet, M. A., and Le Douarin, N. M. (1990). A new approach to the development of the cerebellum provided by the quail-chick marker system. Development 108, 18–31. doi: 10.1242/dev.108.1.19
Hanks, M., Wurst, W., Anson-Cartwright, L., Auerbach, A. B., and Joyner, A. L. (1995). Rescue of the En-1 mutant phenotype by replacement of En-1 with En-2. Science 269, 679–682. doi: 10.1126/science.7624797
Hauptmann, G., Belting, H. G., Wolke, U., Lunde, K., Söll, I., Abdelilah-Seyfried, S., et al. (2002). spiel ohne grenzen/pou2 is required for zebrafish hindbrain segmentation. Development 129, 1645–1655. doi: 10.1242/dev.129.7.1645
Heikinheimo, M., Lawshé, A., Shackleford, G. M., Wilson, D. B., and MacArthur, C. A. (1994). Fgf-8 expression in the post-gastrulation mouse suggests roles in the development of the face, limbs and central nervous system. Mech. Dev. 48, 129–138. doi: 10.1016/0925-4773(94)90022-1
Heller, N., and Brändli, A. W. (1997). Xenopus Pax-2 displays multiple splice forms during embryogenesis and pronephric kidney development. Mech. Dev. 69, 83–104. doi: 10.1016/s0925-4773(97)00158-5
Hemmati-Brivanlou, A., de-la-Torre, J. R., Holt, C., and Harland, R. M. (1991). Cephalic expression and molecular characterization of Xenopus En-2. Development 111, 715–724. doi: 10.1242/dev.111.3.715
Hidalgo-Sánchez, M., and Alvarado-Mallart, R.-M. (2002). Temporal sequence of gene expression leading caudal prosencephalon to develop a midbrain/hindbrain phenotype. Dev. Dyn. 223, 141–147. doi: 10.1002/dvdy.1224
Hidalgo-Sánchez, M., Millet, S., Bloch-Gallego, E., and Alvarado-Mallart, R.-M. (2005a). Specification of the meso-isthmo-cerebellar region: the Otx2/Gbx2 boundary. Brain Res. Rev. 49, 134–149. doi: 10.1016/j.brainresrev.2005.01.010
Hidalgo-Sánchez, M., Martínez-de-la-Torre, M., Alvarado-Mallart, R.-M., and Puelles, L. (2005b). A distinct preisthmic histogenetic domain is defined by overlap of Otx2 and Pax2 gene expression in the avian caudal midbrain. J. Comp. Neurol. 483, 17–29. doi: 10.3390/s22031080
Hidalgo-Sánchez, M., Millet, S., Simeone, A., and Alvarado-Mallart, R.-M. (1999a). Comparative analysis of Otx2, Gbx2, Pax2, Fgf8 and Wnt1 gene expressions during the formation of the chick midbrain/hindbrain domain. Mech. Dev. 81, 175–178. doi: 10.1016/s0925-4773(98)00224-x
Hidalgo-Sánchez, M., Simeone, A., and Alvarado-Mallart, R.-M. (1999b). Fgf8 and Gbx2 induction concomitant with Otx2 repression is correlated with midbrain-hindbrain fate of caudal prosencephalon. Development 126, 3191–3203. doi: 10.1242/dev.126.14.3191
Hirata, H., Tomita, K., Bessho, Y., and Kageyama, R. (2001). Hes1 and Hes3 regulate maintenance of the isthmic organizer and development of the mid/hindbrain. EMBO J. 20, 4454–4466. doi: 10.1093/emboj/20.16.4454
Hollyday, M., McMahon, J. A., and McMahon, A. P. (1995). Wnt expression patterns in chick embryo nervous system. Mech. Dev. 52, 9–25. doi: 10.1016/0925-4773(95)00385-e
Inomata, C., Yuikawa, T., Nakayama-Sadakiyo, Y., Kobayashi, K., Ikeda, M., Chiba, M., et al. (2020). Involvement of an Oct4-related PouV gene, pou5f3/pou2, in neurogenesis in the early neural plate of zebrafish embryos. Dev. Biol. 457, 30–42. doi: 10.1016/j.ydbio.2019.09.002
Inoue, F., Kurokawa, D., Takahashi, M., and Aizawa, S. (2012). Gbx2 Directly Restrictssource. Otx2 expression to forebrain and midbrain, competing with Class III POU Factors. Mol. Cel. Biol. 32, 2618–2627. doi: 10.1128/MCB.00083-12
Irving, C., and Mason, I. (1999). Regeneration of isthmic tissue is the result of a specific and direct interaction between rhombomere 1 and midbrain. Development 126, 3981–3989. doi: 10.1242/dev.126.18.3981
Islam, M. E., Kikuta, H., Inoue, F., Kanai, M., Kawakami, A., Parvin, M. S., et al. (2006). Three enhancer regions regulate gbx2 gene expression in the isthmic region during zebrafish development. Mech. Dev. 123, 907–924. doi: 10.1016/j.mod.2006.08.007
Issack, P. S., and Ziff, E. B. (1998). Altered expression of helix-loop-helix transcriptional regulators and cyclin D1 in Wnt-1-transformed PC12 cells. Cell Growth Differ. 9, 837–845.
Itasaki, N., and Nakamura, H. (1992). Rostrocaudal polarity of the tectum in birds: correlation of en gradient and topographic order in retinotectal projection. Neuron 8, 787–798. doi: 10.1016/0896-6273(92)90099-y
Itasaki, N., and Nakamura, H. (1996). A role for gradient en expression in positional specification on the optic tectum. Neuron 16, 55–62. doi: 10.1016/s0896-6273(00)80023-9
Itasaki, N., Ichijo, H., Hama, C., Matsuno, T., and Nakamura, H. (1991). Establishment of rostrocaudal polarity in tectal primordium: engrailed expression and subsequent tectal polarity. Development 113, 1133–1144. doi: 10.1242/dev.113.4.1133
Jászai, J., Reifers, F., Picker, A., Langenberg, T., and Brand, M. (2003). Isthmus-to-midbrain transformation in the absence of midbrain-hindbrain organizer activity. Development 130, 6611–6623. doi: 10.1242/dev.00899
Joyner, A. L., Herrup, K., Auerbach, B. A., Davis, C. A., and Rossant, J. (1991). Subtle cerebellar phenotype in mice homozygous for a targeted deletion of the En-2 homeobox. Science 251, 1239–1243. doi: 10.1126/science.1672471
Joyner, A. L., Kornberg, T., Coleman, K. G., Cox, D. R., and Martin, G. R. (1985). Expression during embryogenesis of a mouse gene with sequence homology to the Drosophila engrailed gene. Cell 43, 29–37. doi: 10.1016/0092-8674(85)90009-1
Joyner, A. L., and Martin, G. R. (1987). En-1 and En-2, two mouse genes with sequence homology to the Drosophila engrailed gene: expression during embryogenesis. Genes Dev. 1, 29–38. doi: 10.1101/gad.1.1.29
Joyner, A. L., Skarnes, W. C., and Rossant, J. (1989). Production of a mutation in mouse En-2 gene by homologous recombination in embryonic stem cells. Nature 338, 153–156. doi: 10.1038/338153a0
Jukkola, T., Lahti, L., Naserke, T., Wurst, W., and Partanen, J. (2006). FGF regulated gene-expression and neuronal differentiation in the developing midbrain-hindbrain region. Dev. Biol. 297, 141–157. doi: 10.1016/j.ydbio.2006.05.002
Jungbluth, S., Larsen, C., Wizenmann, A., and Lumsden, A. (2001). Cell mixing between the embryonic midbrain and hindbrain. Curr. Biol. 11, 204–207. doi: 10.1016/s0960-9822(01)00049-5
Kala, K., Jukkola, T., Pata, I., and Partanen, J. (2008). Analysis of the midbrain-hindbrain boundary cell fate using a boundary cell-specific Cre-mouse strain. Genesis 46, 29–36. doi: 10.1002/dvg.20344
Katahira, T., Sato, T., Sugiyama, S., Okafuji, T., Araki, I., Funahashi, J., et al. (2000). Interaction between Otx2 and Gbx2 defines the organizing center for the optic tectum. Mech. Dev. 91, 43–52. doi: 10.1016/s0925-4773(99)00262-2
Kelly, G. M., and Moon, R. T. (1995). Involvement of Wnt1 and Pax2 in the formation of the midbrain-hindbrain boundary in the zebrafish gastrula. Dev. Genet. 17, 129–140. doi: 10.1016/s0925-4773(99)00262-2
Kesavan, G., Chekuru, A., Machate, A., and Brand, M. (2017). CRISPR/Cas9-mediated zebrafish knock-in as a novel strategy to study midbrain-hindbrain boundary development. Front. Neuroanat. 11:52. doi: 10.3389/fnana.2017.00052
Kesavan, G., Hammer, J., Hans, S., and Brand, M. (2018). Targeted knock-in of CreERT2 in zebrafish using CRISPR/Cas9. Cell Tissue Res. 372, 41–50. doi: 10.1007/s00441-018-2798-x
Kesavan, G., Machate, A., Hans, S., and Brand, M. (2020). Cell-fate plasticity, adhesion and cell sorting complementarily establish a sharp midbrain-hindbrain boundary. Development 147:dev186882. doi: 10.1242/dev.186882
Kikuta, H., Kanai, M., Ito, Y., and Yamasu, K. (2003). gbx2 Homeobox gene is required for the maintenance of the isthmic region in the zebrafish embryonic brain. Dev. Dyn. 228, 433–450. doi: 10.1002/dvdy.10409
Kim, S., Zhao, Y., Lee, J. M., Kim, W. R., Gorivodsky, M., Westphal, H., et al. (2016). Ldb1 is essential for the development of isthmic organizer and midbrain dopaminergic neurons. Stem Cells Dev. 25, 986–994. doi: 10.1089/scd.2015.0307
Kimura, J., Katahira, T., Araki, I., and Nakamura, H. (2004). Possible role of Hes5 for the rostrocaudal polarity formation of the tectum. Dev. Growth Differ. 46, 219–227. doi: 10.1111/j.1440-169X.2004.00739.x
Kitajima, K., Koshimizu, U., and Nakamura, T. (1999). Expression of a novel type of classic cadherin, PB-cadherin in developing brain and limb buds. Dev. Dyn. 215, 206–214. doi: 10.1002/(SICI)1097-0177(199907)215:3<206::AID-AJA3>3.0.CO;2-X
Krauss, S., Johansen, T., Korzh, V., and Fjose, A. (1991). Expression of the zebrafish paired box gene pax[zf-b] during early neurogenesis. Development 113, 1193–1206. doi: 10.1242/dev.113.4.1193
Krauss, S., Maden, M., Holder, N., and Wilson, S. W. (1992). Zebrafish pax[b] is involved in the formation of the midbrain-hindbrain boundary. Nature 360, 87–89. doi: 10.1038/360087a0
Kurokawa, D., Kiyonari, H., Nakayama, R., Kimura-Yoshida, C., Matsuo, I., and Aizawa, S. (2004). Regulation of Otx2 expression and its functions in mouse forebrain and midbrain. Development 131, 3319–3331. doi: 10.1242/dev.01220
Lahti, L., Saarimäki-Vire, J., Rita, H., and Partanen, J. (2011). FGF signaling gradient maintains symmetrical proliferative divisions of midbrain neuronal progenitors. Dev. Biol. 349, 270–282. doi: 10.1016/j.ydbio.2010.11.008
Langenberg, T., and Brand, M. (2005). Lineage restriction maintains a stable organizer cell population at the zebrafish midbrain-hindbrain boundary. Development 132, 3209–3216. doi: 10.1242/dev.01862
Le Douarin, N. M. (1969). Particularites du noyau interphasique chez la caille japonaise (Coturnix coturnix japonica). Bull. Biol. Fr. Belg. 103, 435–452.
Le Douarin, N. M. (1973). A feulgen-positive nucleolus. Exp. Cell Res. 77, 459–468. doi: 10.1016/0014-4827(73)90600-9
Leerberg, D. M., Hopton, R. E., and Draper, B. W. (2019). Fibroblast growth factor receptors function redundantly during zebrafish embryonic development. Genetics 212, 1301–1319. doi: 10.1534/genetics.119.302345
Lekven, A. C., Buckles, G. R., Kostakis, N., and Moon, R. T. (2003). wnt1 and wnt10b function redundantly at the zebrafish midbrain-hindbrain boundary. Dev. Biol. 254, 172–187. doi: 10.1016/s0012-1606(02)00044-1
Li, J. Y., and Joyner, A. L. (2001). Otx2 and Gbx2 are required for refinement and not induction of mid-hindbrain gene expression. Development 128, 4979–4991. doi: 10.1242/dev.128.24.4979
Li, J. Y. H., Lao, Z., and Joyner, A. L. (2002). Changing requirements for Gbx2 in development of the cerebellum and maintenance of the mid/hindbrain organizer. Neuron 36, 31–43. doi: 10.1016/s0896-6273(02)00935-2
Li, J. Y. H., Lao, Z., and Joyner, A. L. (2005). New regulatory interactions and cellular responses in the isthmic organizer region revealed by altering Gbx2 expression. Development 132, 1971–1981. doi: 10.1242/dev.01727
Liu, A., and Joyner, A. L. (2001). EN and GBX2 play essential roles downstream of FGF8 in patterning the mouse mid/hindbrain region. Development 128, 181–191. doi: 10.1242/dev.128.2.181
Liu, A., Li, J. H. L., Bromleigh, C., Lao, Z., Niswander, L. A., and Joyner, A. L. (2003). FGF17b and FGF18 have different midbrain regulatory properties from FGF8b or activated FGF receptors. Development 130, 6175–6185. doi: 10.1242/dev.00845
Liu, A., Losos, K., and Joyner, A. L. (1999). FGF8 can activate Gbx2 and transform regions of the rostral mouse brain into a hindbrain fate. Development 126, 4827–4838. doi: 10.1242/dev.126.21.4827
Lobe, C. G. (1997). Expression of the helix-loop-helix factor, Hes3, during embryo development suggests a role in early midbrain-hindbrain patterning. Mech. Dev. 62, 227–237. doi: 10.1016/s0925-4773(97)00665-5
Logan, C., Hanks, M. C., Noble-Topham, S., Nallainathan, D., Provart, N. J., and Joyner, A. L. (1992). Cloning and sequence comparison of the mouse, human and chicken engrailed genes reveal potential functional domains and regulatory regions. Dev. Genet. 13, 345–358. doi: 10.1002/dvg.1020130505
Logan, C., Wizenmann, A., Drescher, U., Monschau, B., Bonhoeffer, F., and Lumsden, A. (1996). Rostral optic tectum acquires caudal characteristics following ectopic engrailed expression. Curr. Biol. 6, 1006–1014. doi: 10.1016/s0960-9822(02)00645-0
Lorente-Cánovas, B., Marín, F., Corral-San-Miguel, R., Hidalgo-Sánchez, M., Ferran, J. L., Puelles, L., et al. (2012). Multiple origins, migratory paths and molecular profiles of cells populating the avian interpeduncular nucleus. Dev. Biol. 361, 12–26. doi: 10.1016/j.ydbio.2011.09.032
Louvi, A., Alexandre, P., Me?tin, C., Wurst, W., and Wassef, M. (2003). The isthmic neuroepithelium is essential for cerebellar midline fusion. Development 130, 5319–5330. doi: 10.1242/dev.00736
Lun, K., and Brand, M. (1998). A series of no isthmus (noi) alleles of the zebrafish pax2.1 gene reveals multiple signaling events in development of the midbrain-hindbrain boundary. Development 125, 3049–3062. doi: 10.1242/dev.125.16.3049
Müller, M., von Weizsäcker, E., and Campos-Ortega, J. A. (1996). Transcription of a zebrafish gene of the hairy-enhancer of split family delineates the midbrain anlage in the neural plate. Dev. Genes Evol. 206, 153–160. doi: 10.1007/s004270050041
Marín, F., and Puelles, L. (1994). Patterning of the embryonic avian midbrain after experimental inversions: a polarizing activity from the isthmus. Dev. Biol. 163, 19–37. doi: 10.1006/dbio.1994.1120
Marín, F., and Puelles, L. (1995). Morphological fate of rhombomeres in quail/chick chimeras: a segmental analysis of hindbrain nuclei. Eur. J. Neurosci. 7, 1714–1738. doi: 10.1111/j.1460-9568.1995.tb00693.x
Martinez, S., and Alvarado-Mallart, R.-M. (1989). Rostral cerebellum originates from the caudal portion of the so-called “mesencephalic” vesicle: a study using Chick/Quail chimeras. Eur. J. Neurosci. 1, 549–560. doi: 10.1111/j.1460-9568.1989.tb00362.x
Martinez, S., and Alvarado-Mallart, R.-M. (1990). Expression of the homeobox Chick-en gene in chick/quail chimeras with inverted mes-metencephalic grafts. Dev. Biol. 139, 432–436. doi: 10.1016/0012-1606(90)90312-7
Martinez, S., Crossley, P. H., Cobos, I., Rubenstein, J. L., and Martin, G. R. (1999). FGF8 induces formation of an ectopic isthmic organizer and isthmocerebellar development via a repressive effect on Otx2 expression. Development 126, 1189–1200. doi: 10.1242/dev.126.6.1189
Martínez, S., Marín, F., Nieto, M. A., and Puelles, L. (1995). Induction of ectopic engrailed expression and fate change in avian rhombomeres: intersegmental boundaries as barriers. Mech. Dev. 51, 289–303. doi: 10.1016/0925-4773(95)00376-2
Martinez, S., Wassef, M., and Alvarado-Mallart, R.-M. (1991). Induction of a mesencephalic phenotype in the 2-day-old chick prosencephalon is preceded by the early expression of the homeobox gene en. Neuron 6, 971–981. doi: 10.1016/0896-6273(91)90237-t
Martinez-Barbera, J. P., Signore, M., Boyl, P. P., Puelles, E., Acampora, D., Gogoi, R., et al. (2001). Regionalisation of anterior neuroectoderm and its competence in responding to forebrain and midbrain inducing activities depend on mutual antagonism between OTX2 and GBX2. Development 128, 4789–4800. doi: 10.1242/dev.128.23.4789
Matsunaga, E., Araki, I., and Nakamura, H. (2000). Pax6 defines the di-mesencephalic boundary by repressing En1 and Pax2. Development 127, 2357–2365. doi: 10.1242/dev.127.11.2357
Matsunaga, E., Katahira, T., and Nakamura, H. (2002). Role of Lmx1b and Wnt1 in mesencephalon and metencephalon development. Development 129, 5269–5277. doi: 10.1242/dev.129.22.5269
Matsuno, T., Ichijo, H., and Nakamura, H. (1991). Regulation of the rostrocaudal axis of the optic tectum: histological study after rostrocaudal rotation in quail-chick chimeras. Dev. Brain Res. 58, 265–270. doi: 10.1016/0165-3806(91)90014-a
Matsuo, I., Kuratani, S., Kimura, C., Takeda, N., and Aizawa, S. (1995). Mouse Otx2 functions in the formation and patterning of rostral head. Genes Dev. 9, 2646–2658. doi: 10.1101/gad.9.21.2646
McMahon, A. P., and Bradley, A. (1990). The Wnt-1 (int-1) proto-oncogene is required for development of a large region of the mouse brain. Cell 62, 1073–1085. doi: 10.1016/0092-8674(90)90385-r
McMahon, A. P., Joyner, A. L., Bradley, A., and McMahon, J. A. (1992). The midbrain-hindbrain phenotype of Wnt-1−Wnt-1− mice results from stepwise deletion of engrailed-expressing cells by 9.5 days postcoitum. Cell 69, 581–595. doi: 10.1016/0092-8674(92)90222-x
Meinhardt, H. (2009). Models for the generation and interpretation of gradients. Cold Spring Harb. Perspect. Biol. 1:a001362. doi: 10.1101/cshperspect.a001362
Mercier, P., Simeone, A., Cotelli, F., and Boncinelli, E. (1995). Expression pattern of two otx genes suggests a role in specifying anterior body structures in zebrafish. Int. J. Dev. Biol 39, 559–573.
Meyers, E. N., Lewandoski, M., and Martin, G. R. (1998). An Fgf8 mutant allelic series generated by Cre- and Flp-mediated recombination. Nat. Genet. 18, 136–141. doi: 10.1038/ng0298-136
Miles, L. B., Darido, C., Kaslin, J., Heath, J. K., Jane, S. M., and Dworkin, S. (2017). Mis-expression of grainyhead-like transcription factors in zebrafish leads to defects in enveloping layer (EVL) integrity, cellular morphogenesis and axial extension. Sci. Rep. 7:17607. doi: 10.1038/s41598-017-17898-7
Millen, K. J., Wurst, W., Herrup, K., and Joyner, A. L. (1994). Abnormal embryonic cerebellar development and patterning of postnatal foliation in two mouse Engrailed-2 mutants. Development 120, 695–706. doi: 10.1242/dev.120.3.695
Millet, S., and Alvarado-Mallart, R.-M. (1995). Expression of the homeobox-containing gene En-2 during the development of the chick central nervous system. Eur. J. Neurosci. 7, 777–791. doi: 10.1111/j.1460-9568.1995.tb00681.x
Millet, S., Bloch-Gallego, E., Simeone, A., and Alvarado-Mallart, R.-M. (1996). The caudal limit of Otx2 gene expression as a marker of the midbrain/hindbrain boundary: a study using in situ hybridisation and chick/quail homotopic grafts. Development 122, 3785–3797. doi: 10.1242/dev.122.12.3785
Millet, S., Campbell, K., Epstein, D. J., Losos, K., Harris, E., and Joyner, A. L. (1999). A role for Gbx2 in repression of Otx2 and positioning the mid/hindbrain organizer. Nature 401, 161–164. doi: 10.1038/43664
Molven, A., Njølstad, P. R., and Fjose, A. (1991). Genomic structure and restricted neural expression of the zebrafish wnt-1 (int-1) gene. EMBO J. 10, 799–807.
Mori, H., Miyazaki, Y., Morita, T., Nitta, H., and Mishina, M. (1994). Different spatio-temporal expressions of three otx homeoprotein transcripts during zebrafish embryogenesis. Brain Res. Mol. Brain Res. 27, 221–231. doi: 10.1016/0169-328x(94)90004-3
Nakamura, H. (1990). Do CNS anlagen have plasticity in differentiation? Analysis in quail-chick chimera. Brain Res. 511, 122–128. doi: 10.1016/0006-8993(90)90231-y
Nakamura, H., Matsui, K. A., Takagi, S., and Fujisawa, H. (1991). Projection of the retinal ganglion cells to the tectum differentiated from the prosencephalon. Neurosci. Res. 11, 189–197. doi: 10.1016/0168-0102(91)90042-w
Nakayama, Y., Inomata, C., Yuikawa, T., Tsuda, S., and Yamasu, K. (2017). Comprehensive analysis of target genes in zebrafish embryos reveals gbx2 involvement in neurogenesis. Dev. Biol. 430, 237–248. doi: 10.1016/j.ydbio.2017.07.015
Nakayama, Y., Kikuta, H., Kanai, M., Yoshikawa, K., Kawamura, A., Kobayashi, K., et al. (2013). Gbx2 functions as a transcriptional repressor to regulate the specification and morphogenesis of the mid-hindbrain junction in a dosage- and stage-dependent manner. Mech. Dev. 130, 532–552. doi: 10.1016/j.mod.2013.07.004
Namm, A., Arend, A., and Aunapuu, M. (2014). Expression of Pax2 protein during the formation of the central nervous system in human embryos. Folia Morphol. (Warsz) 73, 272–278. doi: 10.5603/FM.2014.0043
Ninkovic, J., Stigloher, C., Lillesaar, C., and Bally-Cuif, L. (2008). Gsk3ß/PKA and Gli1 regulate the maintenance of neural progenitors at the midbrain-hindbrain boundary in concert with E(Spl) factor activity. Development 135, 3137–3148. doi: 10.1242/dev.020479
Ninkovic, J., Tallafuß, A., Leucht, C., Topczewski, J., Tannhäuser, B., Solnica-Krezel, L., et al. (2005). Inhibition of neurogenesis at the zebrafish midbrain-hindbrain boundary by the combined and dose-dependent activity of a new hairy/E(spl) gene pair. Development 132, 75–88. doi: 10.1242/dev.01525
Niss, K., and Leutz, A. (1998). Expression of the homeobox gene GBX2 during chicken development. Mech. Dev. 76, 151–155. doi: 10.1016/s0925-4773(98)00103-8
O’Hara, F. P., Beck, E., Barr, L. K., Wong, L. L., Kessier, D. S., and Riddle, R. D. (2005). Zebrafish Lmx1b.1 and Lmb1b.2 are required for maintenance of the isthmic organizer. Development 132, 3163–3173. doi: 10.1242/dev.01898
Ohuchi, H., Kimura, S., Watamoto, M., and Itoh, N. (2000). Involvement of fibroblast growth factor (FGF)18-FGF8 signaling in specification of left-right asymmetry and brain and limb development of the chick embryo. Mech. Dev. 95, 55–66. doi: 10.1016/s0925-4773(00)00331-2
Ohuchi, H., Yoshioka, H., Tanaka, A., Kawakami, Y., Nohno, T., and Noji, S. (1994). Involvement of androgen-induced growth factor (FGF-8) gene in mouse embryogenesis and morphogenesis. Biochem. Biophys. Res. Commun. 204, 882–888. doi: 10.1006/bbrc.1994.2542
Okafuji, T., Funahashi, J., and Nakamura, H. (1999). Roles of Pax-2 in initiation of the chick tectal development. Dev. Brain Res. 116, 41–49. doi: 10.1016/s0165-3806(99)00073-5
Olander, S., Nordström, U., Patthey, C., and Edlund, T. (2006). Convergent Wnt and FGF signaling at the gastrula stage induce the formation of the isthmic organizer. Mech. Dev. 123, 166–176. doi: 10.1016/j.mod.2005.11.001
Omi, M., Harada, H., Watanabe, Y., Funahashi, J., and Nakamura, H. (2014). Role of En2 in the tectal laminar formation of chick embryos. Development 141, 2131–2138. doi: 10.1242/dev.102905
Omi, M., and Nakamura, H. (2015). Engrailed and tectum development. Dev. Growth Differ. 57, 135–145. doi: 10.1111/dgd.12197
Orr, H. (1887). Contribution to the embryology of the lizard; with especial reference to the central nervous system and some organs of the head; together with observations on the origin of the vertebrates. J. Morphol. 1, 311–372.
Ota, S., Tonou-Fujimori, N., Nakayama, Y., Ito, Y., Kawamura, A., and Yamasu, K. (2010). FGF receptor gene expression and its regulation by FGF signaling during early zebrafish development. Genesis 48, 707–716. doi: 10.1002/dvg.20682
Ota, S., Taimatsu, K., Yanagi, K., Namiki, T., Ohga, R., Higashijima, S., et al. (2016). Functional visualization and disruption of targeted genes using CRISPR/Cas9-mediated eGFP reporter integration in zebrafish. Sci. Rep. 6:34991. doi: 10.1038/srep34991
Panhuysen, M., Vogt Weisenhorn, D. M., Blanquet, V., Brodski, C., Heinzmann, U., Beisker, W., et al. (2004). Effects of Wnt1 signaling on proliferation in the developing mid-/hindbrain region. Mol. Cell. Neurosci. 26, 101–111. doi: 10.1016/j.mcn.2004.01.011
Pannese, M., Polo, C., Andreazzoli, M., Vignali, R., Kablar, B., Barsacchi, G., et al. (1995). The Xenopus homologue of Otx2 is a maternal homeobox gene that demarcates and specifies anterior body regions. Development 121, 707–720. doi: 10.1242/dev.121.3.707
Parr, B. A., Shea, M. J., Vassileva, G., and McMahon, A. P. (1993). Mouse Wnt genes exhibit discrete domains of expression in the early embryonic CNS and limb buds. Development 119, 247–261. doi: 10.1242/dev.119.1.247
Parvin, M. S., Okuyama, N., Inoue, F., Islam, M. E., Kawakami, A., Takeda, H., et al. (2008). Autoregulatory loop and retinoic acid repression regulate pou2/pou5f1 gene expression in the zebrafish embryonic brain. Dev. Dyn. 237, 1373–1388. doi: 10.1002/dvdy.21539
Patel, N. H., Martin-Blanco, E., Coleman, K. G., Poole, S. J., Ellis, M. C., Kornberg, T. B., et al. (1989). Expression of engrailed proteins in arthropods, annelids and chordates. Cell 58, 955–968. doi: 10.1016/0092-8674(89)90947-1
Pfeffer, P. L., Bouchard, M., and Busslinger, M. (2000). Pax2 and homeodomain proteins cooperatively regulate a 435 bp enhancer of the mouse Pax5 gene at the midbrain-hindbrain boundary. Development 127, 1017–1028. doi: 10.1242/dev.127.5.1017
Pfeffer, P. L., Gerster, T., Lun, K., Brand, M., and Busslinger, M. (1998). Characterization of three novel members of the zebrafish Pax2/5/8 family: dependency of Pax5 and Pax8 expression on the Pax2.1 (noi) function. Development 125, 3063–3074. doi: 10.1242/dev.125.16.3063
Pfeffer, P. L., Payer, B., Reim, G., di Magliano, M. P., and Busslinger, M. (2002). The activation and maintenance of Pax2 expression at the mid-hindbrain boundary is controlled by separate enhancers. Development 129, 307–318. doi: 10.1242/dev.129.2.307
Picker, A., Brennan, C., Reifers, F., Clarke, J. D., Holder, N., and Brand, M. (1999). Requirement for the zebrafish mid-hindbrain boundary in midbrain polarisation, mapping and confinement of the retinotectal projection. Development 126, 2967–2978. doi: 10.1242/dev.126.13.2967
Picker, A., Scholpp, S., Böhli, H., Takeda, H., and Brand, M. (2002). A novel positive transcriptional feedback loop in midbrain-hindbrain boundary development is revealed through analysis of the zebrafish pax2.1 promoter in transgenic lines. Development 129, 3227–3239. doi: 10.1242/dev.129.13.3227
Pose-Méndez, S., Candal, E., Mazan, S., and Rodríguez-Moldes, I. (2016). Genoarchitecture of the rostral hindbrain of a shark: basis for understanding the emergence of the cerebellum at the agnathan-gnathostome transition. Brain Struct. Funct. 221, 1321–1335. doi: 10.1007/s00429-014-0973-8
Puelles, L. (2013). “Plan of the developing vertebrate nervous system relating embryology to the adult nervous system (prosomere model, overview of brain organization),” in Comprehensive Developmental Neuroscience: Patterning and Cell Type Specification in the Developing CNS and PNS, eds J. L. R. Rubenstein and P. Rakic (Amsterdam: Academic Press), 187–209. doi: 10.1016/B978-0-12-397265-1.00118-0
Puelles, L. (2018). Developmental studies of avian brain organization. Int. J. Dev. Biol. 62, 207–224. doi: 10.1387/ijdb.170279LP
Puelles, L., and Ferran, J. L. (2012). Concept of neural genoarchitecture and its genomic fundament. Front. Neuroanat. 6:47. doi: 10.3389/fnana.2012.00047
Puelles, L., Harrison, M., Paxinos, G., and Watson, C. (2013). A developmental ontology for the mammalian brain based on the prosomeric model. Trends Neurosci. 36, 570–578. doi: 10.1016/j.tins.2013.06.004
Puelles, L., Martínez-de-la-Torre, M., Paxinos, G., Watson, C., and Martínez, S. (2007). The Chick Brain in Stereotaxic Coordinates: An Atlas Featuring Neuromeric Subdivisions and Mammalian Homologies. San Diego, CA: Elsevier/Academic Press.
Puelles, L., and Rubenstein, J. L. R. (2003). Forebrain gene expression domains and the evolving prosomeric model. Trends Neurosci. 26, 469–476. doi: 10.1016/S0166-2236(03)00234-0
Ramos-Mejía, V., Escalante-Alcalde, D., Kunath, T., Ramírez, L., Gertsenstein, M., Nagy, A., et al. (2005). Phenotypic analyses of mouse embryos with ubiquitous expression of Oct4: effects on mid-hindbrain patterning and gene expression. Dev. Dyn. 232, 180–190. doi: 10.1002/dvdy.20211
Reifers, F., Böhli, H., Walsh, E. C., Crossley, P. H., Stainier, D. Y., and Brand, M. (1998). Fgf8 is mutated in zebrafish acerebellar (ace) mutants and is required for maintenance of midbrain-hindbrain boundary development and somitogenesis. Development 125, 2381–2395. doi: 10.1242/dev.125.13.2381
Reim, G., and Brand, M. (2002). Spiel-ohne-grenzen/pou2 mediates regional competence to respond to Fgf8 during zebrafish early neural development. Development 129, 917–933. doi: 10.1242/dev.129.4.917
Rhinn, M., Dierich, A., le Meur, M., and Ang, S. (1999). Cell autonomous and non-cell autonomous functions of Otx2 in patterning the rostral brain. Development 126, 4295–4304. doi: 10.1242/dev.126.19.4295
Rhinn, M., Dierich, A., Shawlot, W., Behringer, R. R., le Meur, M., and Ang, S. L. (1998). Sequential roles for Otx2 in visceral endoderm and neuroectoderm for forebrain and midbrain induction and specification. Development 125, 845–856. doi: 10.1242/dev.125.5.845
Rhinn, M., Lun, K., Ahrendt, R., Geffarth, M., and Brand, M. (2009). Zebrafish gbx1 refines the midbrain-hindbrain boundary border and mediates the Wnt8 posteriorization signal. Neural Dev. 4:12. doi: 10.1186/1749-8104-4-12
Rhinn, M., Lun, K., Amores, A., Yan, Y.-L., Postlethwait, J. H., and Brand, M. (2003). Cloning, expression and relationship of zebrafish gbx1 and gbx2 genes to Fgf signaling. Mech. Dev. 120, 919–936. doi: 10.1016/s0925-4773(03)00135-7
Rhinn, M., Lun, K., Luz, M., Werner, M., and Brand, M. (2005). Positioning of the midbrain-hindbrain boundary organizer through global posteriorization of the neuroectoderm mediated by Wnt8 signaling. Development 132, 1261–1272. doi: 10.1242/dev.01685
Rhinn, M., Lun, K., Werner, M., Simeone, A., and Brand, M. (2004). Isolation and expression of the homeobox gene Gbx1 during mouse development. Dev. Dyn. 229, 334–339. doi: 10.1002/dvdy.10435
Rifat, Y., Parekh, V., Wilanowski, T., Hislop, N. R., Auden, A., Ting, S. B., et al. (2010). Regional neural tube closure defined by the Grainy head-like transcription factors. Dev. Biol. 345, 237–245. doi: 10.1016/j.ydbio.2010.07.017
Rijsewijk, F., Schuermann, M., Wagenaar, E., Parren, P., Weigel, D., and Nusse, R. (1987). The Drosophila homology of the mouse mammary oncogene int-1 is identical to the segment polarity gene wingless. Cell 50, 649–657. doi: 10.1016/0092-8674(87)90038-9
Rohs, P., Ebert, A. M., Zuba, A., and McFarlane, S. (2013). Neuronal expression of fibroblast growth factor receptors in zebrafish. Gene Expr. Patterns 13, 354–361. doi: 10.1016/j.gep.2013.06.006
Rowitch, D. H., Kispert, A., and McMahon, A. P. (1999). Pax-2 regulatory sequences that direct transgene expression in the developing neural plate and external granule cell layer of the cerebellum. Dev. Brain Res. 117, 99–108. doi: 10.1016/s0165-3806(99)00104-2
Rowitch, D. H., and McMahon, A. P. (1995). Pax-2 expression in the murine neural plate precedes and encompasses the expression domains of Wnt-1 and En-1. Mech. Dev. 52, 3–8. doi: 10.1016/0925-4773(95)00380-j
Saarimäki-Vire, J., Peltopuro, P., Lahti, L., Naserke, T., Blak, A. A., Weisenhorn, D. M. V., et al. (2007). Fibrobast growth factor receptors cooperate to regulate neural progenitor properties in the developing midbrain and hindbrain. J. Neurosci. 27, 8581–8592. doi: 10.1523/JNEUROSCI.0192-07.2007
Sato, T., and Joyner, A. L. (2009). The duration of. Fgf8isthmic organizer expression is key to patterning different tectal-isthmo-cerebellum structures. Development 136, 3617–3626. doi: 10.1242/dev.041210
Schier, A. F., Neuhauss, S. C. F., Harvey, M., Malicki, J., Solnica-Krezel, L., Stainier, D. Y. R., et al. (1996). Mutations affecting the development of the embryonic zebrafish brain. Development 123, 165–178. doi: 10.1242/dev.123.1.165
Scholpp, S., and Brand, M. (2004). Zebrafish fgfr1 is a member of the fgf8 synexpression group and is required for fgf8 signalling at the midbrain-hindbrain boundary. Dev. Genes Evol. 214, 285–295. doi: 10.1007/s00427-004-0409-1
Schwarz, M., Alvarez-Bolado, G., Dressler, G., Urbánek, P., Busslinger, M., and Gruss, P. (1999). Pax2/5 and Pax6 subdivide the early neural tube into three domains. Mech. Dev. 82, 29–39. doi: 10.1016/s0925-4773(99)00005-2
Schwarz, M., Alvarez-Bolado, G., Urbánek, P., Busslinger, M., and Gruss, P. (1997). Conserved biological function between Pax-2 and Pax-5 in midbrain and cerebellum development: evidence from targeted mutations. Proc. Natl. Acad. Sci. U S A 94, 14518–14523. doi: 10.1073/pnas.94.26.14518
Sgado, P., Alberi, L., Gherbassi, D., Galasso, S. L., Ramakers, G. M. J., Alavian, K. N., et al. (2006). Slow progressive degeneration of nigral dopaminergic neurons in postnatal Engrailed mutant mice. Proc. Nat. Acad. Sci. U S A 103, 15242–15247. doi: 10.1073/pnas.0602116103
Sgaier, S. K., Lao, Z., Villanueva, M. P., Berenshteyn, F., Stephen, D., Turnbull, R. K., et al. (2007). Genetic subdivision of the tectum and cerebellum into functionally related regions based on differential sensitivity to engrailed proteins. Development 134, 2325–2335. doi: 10.1242/dev.000620
Shigetani, Y., Funahashi, J., and Nakamura, H. (1997). En-2 regulates the expression of the ligands for Eph type tyrosine kinases in chick embryonic tectum. Neurosci. Res. 27, 211–217. doi: 10.1016/s0168-0102(96)01151-0
Shinga, J., Itoh, M., Shiokawa, K., Taira, S., and Taira, M. (2001). Early patterning of the prospective midbrain-hindbrain boundary by the HES-related gene XHR1 in Xenopus embryos. Mech. Dev 109, 225–239. doi: 10.1016/s0925-4773(01)00528-7
Sieger, D., Tautz, D., and Gajewski, M. (2004). her11 is involved in the somitogenesis clock in zebrafish. Dev. Genes Evol. 214, 393–406. doi: 10.1007/s00427-004-0427-z
Simeone, A., Acampora, D., Mallamaci, A., Stornaiuolo, A., D’Apice, M. R., Nigro, V., et al. (1993). A vertebrate gene related to orthodenticle contains a homeodomain of the bicoid class and demarcates anterior neuroectoderm in the gastrulating mouse embryo. EMBO J. 12, 2735–2747.
Simeone, A., Puelles, E., and Acampora, D. (2002). The Otx family. Curr. Opin. Genet. Dev. 12, 409–415. doi: 10.1016/s0959-437x(02)00318-0
Simon, H. H., Scholz, C., and O’Leary, D. D. M. (2005). Engrailed genes control developmental fate of serotonergic and noradrenergic neurons in mid- and hindbrain in a gene dose-dependent manner. Mol. Cell Neurosci. 28, 96–105. doi: 10.1016/j.mcn.2004.08.016
Song, D. L., Chalepakis, G., Gruss, P., and Joyner, A. L. (1996). Two Pax-binding sites are required for early embryonic brain expression of an Engrailed-2 transgene. Development 122, 627–635. doi: 10.1242/dev.122.2.627
Stoykova, A., and Gruss, P. (1994). Roles of Pax-genes in developing and adult brain as suggested by expression patterns. J. Neurosci. 14, 1395–1412. doi: 10.1523/JNEUROSCI.14-03-01395.1994
Su, C.-Y., Kemp, H. A., and Moens, C. B. (2014). Cerebellar development in the absence of Gbx function in zebrafish. Dev. Biol. 386, 181–190. doi: 10.1016/j.ydbio.2013.10.026
Su, Y., and Meng, A. (2002). The expression of gbx-2 during zebrafish embryogenesis. Mech. Dev. 113, 107–110. doi: 10.1016/s0925-4773(02)00011-4
Suda, Y., Matsuo, I., and Aizawa, S. (1997). Cooperation between Otx1 and Otx2 genes in developmental patterning of rostral brain. Mech. Dev. 69, 125–141. doi: 10.1016/s0925-4773(97)00161-5
Sugiyama, S., Funahashi, J., Kitajewski, J., and Nakamura, H. (1998). Crossregulation between En-2 and Wnt-1 in chick tectal development. Dev. Growth Differ. 40, 157–166. doi: 10.1046/j.1440-169x.1998.00005.x
Sun, X., Meyers, E. N., Lewandoski, M., and Martin, G. R. (1999). Targeted disruption of Fgf8 causes failure of cell migration in the gastrulating mouse embryo. Genes Dev. 13, 1834–1846. doi: 10.1101/gad.13.14.1834
Sunmonu, N. A., Chen, L., and Li, J. Y. H. (2009). Misexpression of Gbx2 throughout the mesencephalon by a conditional gain-of-function transgene leads to deletion of the midbrain and cerebellum in mice. Genesis 47, 667–673. doi: 10.1002/dvg.20546
Sunmonu, N. A., Li, K., Guo, Q., and Li, J. Y. H. (2011). Gbx2 and Fgf8 are sequentially required for formation of the midbrain-hindbrain compartment boundary. Development 138, 725–734. doi: 10.1242/dev.055665
Takada, H., Hattori, D., Kitayama, A., Ueno, N., and Taira, M. (2005). Identification of target genes for the Xenopus Hes-related protein XHR1, a prepattern factor specifying the midbrain-hindbrain boundary. Dev. Biol. 283, 253–267. doi: 10.1016/j.ydbio.2005.04.020
Takahashi, N., Tochimoto, N., Ohmori, S. Y., Mamada, H., Itoh, M., Inamori, M., et al. (2005). Systematic screening for genes specifically expressed in the anterior neuroectoderm during early Xenopus development. Int. J. Dev. Biol. 49, 939–951. doi: 10.1387/ijdb.052083nt
Tallafuß, A., and Bally-Cuif, L. (2003). Tracing of her5 progeny in zebrafish transgenics reveals the dynamics of midbrain-hindbrain neurogenesis and maintenance. Development 130, 4307–4323. doi: 10.1242/dev.00662
Tallafuß, A., Wilm, T. P., Crozatier, M., Pfeffer, P., Wassef, M., and Bally-Cuif, L. (2001). The zebrafish buttonhead-like factor Bts1 is an early regulator of pax2.1 expression during mid-hindbrain development. Development 128, 4021–4034. doi: 10.1242/dev.128.20.4021
Tanaka, A., Miyamoto, K., Minamino, N., Takeda, M., Sato, B., Matsuo, H., et al. (1992). Cloning and characterization of an androgen-induced growth factor essential for the androgen-dependent growth of mouse mammary carcinoma cells. Proc. Natl. Acad. Sci. U S A 89, 8928–8932. doi: 10.1073/pnas.89.19.8928
Thawani, A., and Groves, A. K. (2020). Building the border: development of the chordate neural plate border region and its derivatives. Front. Physiol. 11:608880. doi: 10.3389/fphys.2020.608880
Thomas, K. R., and Capecchi, M. R. (1990). Targeted disruption of the murine int-1 proto-oncogene resulting in severe abnormalities in midbrain and cerebellar development. Nature 346, 847–850. doi: 10.1038/346847a0
Thomas, K. R., Musci, T. S., Neumann, P. E., and Capecchi, M. R. (1991). Swaying is a mutant allele of the proto-oncogene Wnt-1. Cell 67, 969–976. doi: 10.1016/0092-8674(91)90369-a
Tossell, K., Andreae, L. C., Cudmore, C., Lang, E., Muthukrishnan, U., Lumsden, A., et al. (2011a). Lrrn1 is required for formation of the midbrain-hindbrain boundary and organiser through regulation of affinity differences between midbrain and hindbrain cells in chick. Dev. Biol. 352, 341–352. doi: 10.1016/j.ydbio.2011.02.002
Tossell, K., Kiecker, C., Wizenmann, A., Lang, E., and Irving, C. (2011b). Notch signalling stabilises boundary formation at the midbrain-hindbrain organiser. Development 138, 3745–3757. doi: 10.1242/dev.070318
Tour, E., Pillemer, G., Gruenbaum, Y., and Fainsod, A. (2001). The two Xenopus Gbx2 genes exhibit similar, but not identical expression patterns and can affect head formation. FEBS Lett. 507, 205–209. doi: 10.1016/s0014-5793(01)02963-5
Tour, E., Pillemer, G., Gruenbaum, Y., and Fainsod, A. (2002a). Gbx2 interacts with Otx2 and patterns the anterior-posterior axis during gastrulation in Xenopus. Mech. Dev. 112, 141–151. doi: 10.1016/s0925-4773(01)00653-0
Tour, E., Pillemer, G., Gruenbaum, Y., and Fainsod, A. (2002b). Otx2 can activate the isthmic organizer genetic network in the Xenopus embryo. Mech. Dev. 110, 3–13. doi: 10.1016/s0925-4773(01)00591-3
Trokovic, R., Jukkola, T., Saarimäki, J., Peltopuro, P., Naserke, T., Weisenhorn, D. M. V., et al. (2005). Fgfr1-dependent boundary cells between developing mid- and hindbrain. Dev. Biol. 278, 428–439. doi: 10.1016/j.ydbio.2004.11.024
Trokovic, R., Trokovic, N., Hernesniemi, S., Pirvola, U., Weisenhorn, D. M. V., Rossant, J., et al. (2003). FGFR1 in independently required in both developing mid- and hindbrain for sustained response to isthmic signals. EMBO J. 22, 1822–1823. doi: 10.1093/emboj/cdg169
Tsuda, S., Hiyoshi, K., Miyazawa, H., Kinno, R., and Yamasu, K. (2019). 4D imaging identifies dynamic migration and the fate of gbx2-expressing cells in the brain primordium of zebrafish. Neurosci. Lett. 690, 112–119. doi: 10.1016/j.neulet.2018.09.027
Urbánek, P., Fetka, I., Meisler, M. H., and Busslinger, M. (1997). Cooperation of Pax2 and Pax5 in midbrain and cerebellum development. Proc. Natl. Acad. Sci. U S A 94, 5703–5708. doi: 10.1073/pnas.94.11.5703
Urbánek, P., Wang, Z. Q., Fetka, I., Wagner, E. F., and Busslinger, M. (1994). Complete block of early B cell differentiation and altered patterning of the posterior midbrain in mice lacking Pax5/BSAP. Cell 79, 901–912. doi: 10.1016/0092-8674(94)90079-5
Vaage, S. (1969). The segmentation of the primitive neural tube in chick embryos (Gallus domesticus). A morphological, histochemical and autoradiographical investigation. Ergeb. Anat. Entwicklungsgesch. 41, 3–87.
Vieira, C., Pombero, A., Garcia-Lopez, R., Gimeno, L., Echevarria, D., and Martinez, S. (2010). Molecular mechanisms controlling brain development: an overview of neuroepithelial secondary organizers. Int. J. Dev. Biol. 54, 7–20. doi: 10.1387/ijdb.092853cv
von Bubnoff, A., Schmidt, J. E., and Kimelman, D. (1996). The Xenopus laevis homeobox gene Xgbx-2 is an early marker of anteroposterior patterning in the ectoderm. Mech. Dev. 54, 149–160. doi: 10.1016/0925-4773(95)00468-8
Walshe, J., and Mason, I. (2000). Expression of FGFR1, FGFR2 and FGFR3 during early neural development in the chick embryo. Mech. Dev. 90, 103–110. doi: 10.1016/s0925-4773(99)00225-7
Wassarman, K. M., Lewandoski, M., Campbell, K., Joyner, A. L., Rubenstein, J. L., Martinez, S., et al. (1997). Specification of the anterior hindbrain and establishment of a normal mid/hindbrain organizer is dependent on Gbx2 gene function. Development 124, 2923–2934. doi: 10.1242/dev.124.15.2923
Waters, S. T., and Lewandoski, M. (2006). A threshold requirement for Gbx2 levels in hindbrain development. Development 133, 1991–2000. doi: 10.1242/dev.02364
Watson, C., Shimogori, T., and Puelles, L. (2017). Mouse Fgf8-Cre-LacZ lineage analysis defines the territory of the postnatal mammalian isthmus. J. Comp. Neurol. 525, 2782–2799. doi: 10.1002/cne.24242
Webb, K. J., Coolen, M., Gloeckner, C. J., Stigloher, C., Bahn, B., Topp, S., et al. (2011). The Enhancer of split transcription factor Her8a is a novel dimerisation partner for Her3 that controls anterior hindbrain neurogenesis in zebrafish. BMC Dev. Biol. 11:27. doi: 10.1186/1471-213X-11-27
Wilanowski, T., Tuckfield, A., Cerruti, L., O’Connell, S., Saint, R., Parekh, V., et al. (2002). A highly conserved novel family of mammalian developmental transcription factors related to Drosophila grainyhead. Mech. Dev. 114, 37–50. doi: 10.1016/s0925-4773(02)00046-1
Wilkinson, D. G., Bailes, J. A., and McMahon, A. P. (1987). Expression of the proto-oncogene int-1 is restricted to specific neural cells in the developing mouse embryo. Cell 50, 79–88. doi: 10.1016/0092-8674(87)90664-7
Wolda, S. L., Moody, C. J., and Moon, R. T. (1993). Overlapping expression of Xwnt-3A and Xwnt-1 in neural tissue of Xenopus laevis embryos. Dev. Biol. 155, 46–57. doi: 10.1006/dbio.1993.1005
Wurst, W., Auerbach, A. B., and Joyner, A. L. (1994). Multiple developmental defects in Engrailed-1 mutant mice: an early mid-hindbrain deletion and patterning defects in forelimbs and sternum. Development 120, 2065–2075. doi: 10.1242/dev.120.7.2065
Yamaguchi, T. P., Conlon, R. A., and Rossant, J. (1992). Expression of the fibroblast growth factor receptor FGFR-1/flg during gastrulation and segmentation in the mouse embryo. Dev. Biol 152, 75–88. doi: 10.1016/0012-1606(92)90157-c
Ye, W., Bouchard, M., Stone, D., Liu, X., Vella, F., Lee, J., et al. (2001). Distinct regulators control the expression of the mid-hindbrain organizer signal FGF8. Nat. Neurosci. 4, 1175–1181. doi: 10.1038/nn761
Keywords: midbrain, hindbrain, Otx2, Gbx2, engrailed, PAX, Fgf8, Wnt1
Citation: Hidalgo-Sánchez M, Andreu-Cervera A, Villa-Carballar S and Echevarria D (2022) An Update on the Molecular Mechanism of the Vertebrate Isthmic Organizer Development in the Context of the Neuromeric Model. Front. Neuroanat. 16:826976. doi: 10.3389/fnana.2022.826976
Received: 01 December 2021; Accepted: 28 February 2022;
Published: 24 March 2022.
Edited by:
Fernando de Castro, Cajal Institute (CSIC), SpainReviewed by:
Kyo Yamasu, Saitama University, JapanJames Y. H. Li, University of Connecticut Health Center, United States
Copyright © 2022 Hidalgo-Sánchez, Andreu-Cervera, Villa-Carballar and Echevarria. This is an open-access article distributed under the terms of the Creative Commons Attribution License (CC BY). The use, distribution or reproduction in other forums is permitted, provided the original author(s) and the copyright owner(s) are credited and that the original publication in this journal is cited, in accordance with accepted academic practice. No use, distribution or reproduction is permitted which does not comply with these terms.
*Correspondence: Matías Hidalgo-Sánchez, mhidalgo@unex.es; Diego Echevarria, diegoaza@umh.es