Silver Chitosan Nanocomposites are Effective to Combat Sporotrichosis
- 1Laboratório de Glicobiología de Eucariotos (LaGE), Departamento de Microbiologia Geral, Instituto de Microbiologia Paulo de Góes (IMPG), Universidade Federal do Rio de Janeiro, Rio de Janeiro, Brazil
- 2Laboratório de Bioquímica e Imunologia das Micoses, Departamento de Microbiologia e Parasitologia, Universidade Federal Fluminense, Niterói, Brazil
- 3Laboratório de Ultraestrutura Celular Hertha Meyer, Instituto de Biofísica Carlos Chagas Filho, Centro Nacional de Biologia Estrutural e Bioimagem, Universidade Federal do Rio de Janeiro, Rio de Janeiro, Brazil
The use of silver nanoparticles (AgNPs) embedded in polymeric matrix has acquired special attention as a strategy to reduce metal toxicity without losing its antimicrobial effect. In this work, the green synthesis of AgNPs and their functionalization with chitosan (AgNPs@Chi) was performed, and their antifungal activity investigated against the foremost species responsible for causing sporotrichosis, Sporothrix brasiliensis and Sporothrix schenckii. In vitro studies revealed inhibitory concentrations ranging from 0.12–1 μg/ml for both nanocomposites (NCs). Silver release in suspension displayed chitosan as a potential vehicle for continuous silver discharge, with a complete release after 52 days. No synergistic effects were observed in vitro when the NCs were combined with itraconazole or amphotericin B. Treatment of S. brasiliensis with NCs caused morphological deformities, cell membrane discontinuity and an intense cytoplasmic degeneration. Remarkably, both NCs induced the growth and migration of keratinocytes and fibroblasts when compared to control conditions. In addition, functionalization of AgNPs with chitosan significantly reduced its hemolytic activity, suggesting their potential use in vivo. Finally, silver nanocomposites were used as a daily topical treatment in a murine model of subcutaneous sporotrichosis, showing the ability to reduce the Sporothrix infection and stimulate tissue repair. In combination, our results demonstrate that AgNPs@Chi can be a non-toxic and efficient alternative for sporotrichosis.
Introduction
Sporotrichosis is an infection caused by Sporothrix spp., stablished after implantation of the fungus in the skin tissue. The fungus is usually found in decaying matter, plants, soil, and is often known as the etiological agent of the “rose gardener’s disease” (Mora-Montes and Héctor, 2018). However, transmission between animals and from animals to humans have been frequently described through contact with exudate from skin lesions, bite and scratch of an infected animal (Gremião et al., 2017; Valeriano et al., 2020). Whereas S. schenckii, S. globosa, S. luriei and S. brasiliensis can infect mammals (Monteiro et al., 2014; Orofino-Costa et al., 2017; Ramírez-Soto et al., 2018), S. brasiliensis has been reported particularly as etiological agent in several cases of feline sporotrichosis (Montenegro et al., 2014; Sanchotene et al., 2015; Orofino-Costa et al., 2017), as well as in cat-human transmission (Gremião et al., 2017).
In the last 20 years Brazil has suffered from an outbreak of sporotrichosis affecting mostly humans and cats (Chakrabarti et al., 2015; Gremião et al., 2020a; Rodrigues et al., 2020). Sporotrichosis outbreaks has also been informed in countries in the Americas and Asia such as Argentina, Malaysia, Panama, India, Thailand, the United States, and Mexico (Chakrabarti et al., 2015; Kano et al., 2015; Gold et al., 2016; Duangkaew et al., 2019; Etchecopaz et al., 2019; Kamal Azam et al., 2019). This disease is characterized by cutaneous and extracutaneous clinical manifestations, of variable severity consistent with the host immune status and also the infecting species (Gold et al., 2016; Wolff et al., 2018; Queiroz-Telles et al., 2019). In this context, S. brasiliensis is considered one of the most virulent species probably because of factors such as thermotolerance, the high adherence ability, and the presence of melanin produced during host-pathogen interaction, promoting both invasion of tissue and evasion of the host’s immune response (Arrillaga-Moncrieff et al., 2009; Almeida-Paes et al., 2017; Della Terra et al., 2017).
The most common therapies for human and animal sporotrichosis includes itraconazole (ITC), terbinafine, potassium iodide (KI) and amphotericin B (AMB), drugs with significative undesirable reactions, limited distribution in certain tissues and drug interaction (Moriyama et al., 2012; Hamill 2013; Kyriakidis et al., 2017; Seibel et al., 2017). In refractory cases, especially in cats with respiratory signs and mucosal injuries, ITC-KI association has been successfully used (Reis et al., 2016; Gremião et al., 2020b). Another treatment possibility is the use of intralesional or subcutaneous AMB associated with oral ITC (Lloret et al., 2013; Gilaberte et al., 2014). Despite the diversity of therapeutic options, cases of treatment failure and recrudescence are frequent (Almeida-Paes et al., 2016; da Rocha et al., 2018).
Because no vaccines are available, the development of alternative compounds to treat sporotrichosis are imperative. Nanotechnology, a science that involves the control of matter in the size scale between 1–100 nm, has been exploited as a tool to combat infectious diseases, including mycosis (Goyal et al., 2016; Niemirowicz et al., 2017; Adler-Moore et al., 2019). Among the nanomaterials with antimicrobial activity, silver nanoparticles stand out (AgNPs) (Zhang et al., 2016). The nanometric size of silver particles increases their surface area and improves interaction with microbial cells (Nadworny et al., 2010). In addition, the biocidal activity is more effective due to the high reactivity of silver with functional chemical groups such as amines, hydroxyl, carboxyl and thiols inhibiting different biological processes of microorganism (Durán et al., 2016; Radhakrishnan et al., 2018). AgNPs were efficient to combat Gram negative and Gram positive bacteria, counting multidrug resistant strains (Baptista et al., 2018; Choi et al., 2018). Likewise, AgNPs have been described as effective against fungi and viruses (Artunduaga Bonilla et al., 2015; Xia et al., 2016; Morris et al., 2019; Sharifi-Rad and Pohl 2020). At least three possible mechanisms have been described for AgNPs: 1) a direct attachment to the cell membrane, followed by damage and permeability increase, 2) the induction of reactive oxygen species (ROS), and 3) the interruption of ATP production and DNA replication, an effect caused after cellular internalization of AgNPs/Ag+ (Dakal et al., 2016; Durán et al., 2016; Riaz Ahmed et al., 2017). Although these mechanisms of action have also been described as causes of silver toxicity in mammalian cells (Hsiao et al., 2015; Radhakrishnan et al., 2018), incorporation into polymeric matrices has proven to be a viable strategy to reduce silver toxicity without significantly losing biocidal activity against pathogens (Danhier et al., 2012; Cinteza et al., 2018; Gaballah et al., 2019).
One of the foremost promising polymers is chitosan, a biodegradable and biocompatible polymer produced by chemical deacetylation of chitin (Bellich et al., 2016). Functionalization of AgNPs with chitosan (AgNPs@Chi) enhances the surface charge of the nanocomposites (NCs) and influences both colloidal stability and biological interaction (Bellich et al., 2016). The polymeric matrix reduces cytotoxicity generally caused by a massive delivery of AgNPs, since it promotes a gradual release (Kumari, Yadav, and Yadav 2010; Baranwal et al., 2018; Cinteza et al., 2018). Furthermore, antibacterial and antifungal properties of chitosan have been described (Raafat et al., 2008; Shih et al., 2019).
We recently demonstrated NCs obtained by chemical reduction with cysteine and functionalized with chitosan (AgNPs@Chi) displayed low toxicity in mammalian cells and high tolerance in insects and mice. Remarkably, no silver bioaccumulation was detected when healthy mice were exposed to these nanoparticles (Artunduaga Bonilla et al., 2021a). Furthermore, these NCs showed antifungal efficiency against distinct pathogenic species of Candida, in both planktonic and sessile cells (Artunduaga Bonilla et al., 2021a). The topical therapy using silver nanocomposites in a murine model of subcutaneous candidiasis decreased the fungal load in the skin injures and favored wound repair. In this work, we investigated whether these NCs could be used in a chronic fungal infection that affects the subcutaneous tissue, such as sporotrichosis. Both inhibitory and synergistic effect of AgNPs and AgNPs@Chi against S. schenckii and S. brasiliensis strains were tested in vitro. The influence of silver nanocomposite on cell migration was also evaluated to determine their ability to stimulate tissue recovery. Finally, these NCs were used to treat a murine model of cutaneous sporotrichosis.
Materials and Methods
Reagents
Amphotericin B, Sabouraud 2% Dextrose Agar (SDA) and silver nitrate (AgNO3, 99.98%), were purchased from Merck. Chitosan (MW 50–190 kDa, deacetylation degree: ≥85%), Polyvinyl Alcohol (PVA, 99%), RPMI 1640 [supplemented with L-glutamine, 0.2% (w/v) of glucose and without sodium bicarbonate], Fetal Bovine Serum (FBS), L-cysteine (C3H7NO2S, >99%), Cetyl trimethyl ammonium bromide (CTAB, 99%), Bovine Serum Albumin (BSA) and itraconazole (ITC, ≥98% Thin-Layer Chromatography) were purchased from Sigma-Aldrich, United States. Brain Heart Infusion (BHI) was acquired from Oxoid, Brazil. All chemicals used in this research were used without further purification.
Fungal Strains and Culture Conditions
Sporothrix brasiliensis ATCC 5110/MYA4823 and Sporothrix schenckii ATCC 1099-18/MYA4821 strains were obtained from the American Type Culture Collection (Manassas, VA 20108 United States) and stored on BHI plates at 4°C or cryopreserved at −20°C in BHI broth with 10% glycerol. For the inoculum of the in vitro tests, Sporothrix cells were cultured in BHI broth at 37°C to favor the development of their yeast phase. For the in vivo experiments, the inoculum was prepared as previously described (Artunduaga Bonilla et al., 2021b). Briefly, S. brasiliensis was cultivated on BHI plates for 7 days at 25°C prior to use. Cells (2 × 106) were transferred to flasks containing 150 ml of BHI and then incubated at 37°C/150 rpm for 4 days. Yeasts were collected by centrifugation at 3.500 rpm for 10 min (4°C) and then washed three times in 0.1 M phosphate-buffered saline pH 7.4 (PBS). Yeast suspension (2 × 108 cells/ml) were assessed for viability by trypan blue staining. Suspensions with cell viability greater than 90% were used for the tests (Della Terra et al., 2017; Artunduaga Bonilla et al., 2021b).
Silver Nanocomposites Synthesis
The silver chitosan nanocomposites tested in the present work were prepared as previously described by our group (Artunduaga Bonilla et al., 2021a). Briefly, a solution containing 4 ml of 0.01 M AgNO3, 800 µL of 0.01 M cysteine and 1.2 ml of 0.01 M CTAB were mixed and stirred using a magnetic bar. Deionized water was added to a final volume of 20 ml and PVA added at a final concentration of 0.02% (w/v). Then, the suspension was sonicated at 37 KHz during 2 h. The appearance of a red-orange suspension indicates that AgNPs was formed (Artunduaga Bonilla et al., 2015; Almeida-Paes et al., 2017). Synthesis was performed at room temperature and in the presence of light. Once the AgNPs were characterized, additional steps were taken to incorporate them into chitosan. Initially, 10 ml of AgNPs (298 μg/ml) was added to 10 ml of chitosan (1%, w/v) in 1% acetic acid and the pH was adjusted to 12 with NaOH. The mixture was subjected to five cycles of 5 s pulses alternating with pauses of 5 s, using a sonicator probe (Sonopuls HD3200) at 150 W. The suspension was then stirred for 1 h at 300 rpm and room temperature (Artunduaga Bonilla et al., 2021a). Finally, the suspension pH was adjusted to 7.5 with NaOH. The nanocomposites were stored in amber flask at room temperature.
Nanocomposites Characterization
Physicochemical properties of the nanocomposites were determined according to our previous publication (Artunduaga Bonilla et al., 2021a). Succinctly, the AgNPs spectrum in the UV-visible region was observed using a spectrophotometer from wavelength of 300–600 nm. To determine the average hydrodynamic size and surface charge of the nanomaterial, Dynamic Light Scattering (DLS) and Zeta potential analysis were performed using a Brookhaven instrument (United States) with suspension at 100 μg/ml concentration. Quantification of silver using a ContrAA 700—High-Resolution Continuum Source Graphite Furnace Atomic Absorption Spectrometer (HR-CS GF AAS), Analytik jena AG, was performed. For all tests, the AgNPs@Chi concentration corresponds to the silver concentration. The size, morphology and crystalline nature of the AgNPs, were determined by transmission electron microscopy (TEM) and X-ray diffraction (XRD). For these analyses, a drop of AgNPs was dispensed on a Formvar coated copper grids (300 mesh) (polyvinyl resin, Ted Pella, Inc.) and dried in a vacuum desiccator. Images were acquired using a JEOL 1200 EX and FEI Tecnai G2 FEG transmission electron microscopes operating at 80 and 200 kV, respectively. For the AgNPs@Chi, the characterization was performed by Zeta potential analysis and TEM respectively, to compare the surface charge and observe the distribution of the AgNPs within the polymer.
Silver Release From Chitosan Matrix
In vitro silver release from chitosan matrix was carried out as previously demonstrated in our recent work (Artunduaga Bonilla et al., 2021a). Briefly, 50 μg/ml aliquots of AgNPs@Chi were added to a small dialysis bag and placed in a beaker containing 20 ml of 85 mM phosphate buffer, pH 7.4 and incubated at 37°C/100 rpm. Aliquots of the buffer (2 ml) were collected sporadically until day 52 and the concentration of silver diffused through the dialysis membrane was measured by atomic absorption spectroscopy.
In vitro Antifungal Activity
Anti-Sporothrix tests were performed following the Clinical and Laboratory Standards Institute (CLSI) M27-A3 protocol with cell suspension adjustments. Yeasts were cultivated in BHI broth at 37°C with orbital agitation (150 rpm) for 3 days. Cells were then washed in PBS and adjusted to 5 × 105 yeasts/ml in RPMI 1640. NCs were diluted in RPMI, and 100 µl volumes were added to 96-well plates in serial dilutions from 16 to 0.12 μg/ml. A volume of 100 µl of the yeast suspension was added to each well. Microplates were incubated at 37°C for 3 days. RPMI 1640 and itraconazole (ITC) were used as controls. The fungal growth was measured spectrophotometrically (λ: 530 nm) using a BioTeK ELx808 microplate reader (Netherlands). Minimal Inhibitory Concentration (MIC50 and MIC90) were considered as the lower concentrations of NCs able to reduce microbial growth in 50 and 90%, respectively, comparing to control conditions. Subsequently, 100 µl aliquots from each of the MIC90 wells were suspended in 900 µl of RPMI and then incubated at 37°C for 48 h. Aliquots of 20 μl were then seeded in BHI plates and incubated for 48 h to check absence of fungal growth. In order to confirm the non-antifungal effect of the chemical reagents used to synthesize the silver nanocomposites, 100 µl aliquots of each working solution (CTAB, PVA, Chitosan) were incubated with Sporothrix yeasts following the broth microdilution protocol in 96-well plates, under the same conditions used to test NCs. All the experiments were carried out in triplicates.
Drug Interactions
The antifungal combinatory effect of NCs with ITC or amphotericin B (AMB) was investigated using the checkerboard test (Zhao et al., 2019). Tests using 96-well plates were performed according to methods of previous studies (Zhao et al., 2019; Artunduaga Bonilla et al., 2021a). Serial two-fold dilutions of each drug (AgNPs, AgNPs@Chi, ITC and AMB) were prepared in RPMI. The 96-well microplates were inoculated with 100 µl of 5 × 105 cells/ml and incubated at 37°C for 3 days. The optical density (OD) was measured at 530 nm using a microplate reader (Biotek ELx808). The fractional inhibitory concentration index (FICi) was defined as (MIC combined/MIC drug A alone) + (MIC combined/MIC drug B alone). The drug interaction was considered synergistic when the FICi is ˂0.5, indifferent when the FICi is between 0.5 and 4, and antagonistic when the FICi is >4 (Zhao et al., 2019; Artunduaga Bonilla et al., 2021a).
Ultrastructural Analysis of S. brasiliensis
Yeasts were treated with MIC50 of the NCs (0.06 and 0.25 μg/ml of AgNPs and AgNPs@Chi, respectively) in RPMI 1640 at 37°C for 48 h. Then, cells were washed twice in PBS and fixed overnight with 2.5% glutaraldehyde, 4% formaldehyde and 10 mM calcium chloride in 0.1 M cacodylate buffer, pH 7.2 at 4°C. Post-fixation in 1% osmium tetroxide in cacodylate buffer containing 0.08% potassium ferrocyanide was performed for 2 h at room temperature. Subsequent, the fungal cells were dehydrated in acetone and embedded in spurr’s resin (Ted Pella Inc.). Ultrathin sections (70 nm) were acquired using a ultramicrotome (LEICA EM UC6). Sections placed onto copper grids (300 mesh) were stained with lead citrate and uranyl acetate and visualized in a transmission electron microscope (JEOL 1200 EX) operated at 80 Kv.
Hemolysis Assay
The hemolytic effect of silver nanocomposites was determined according to Shiny, Mukherjee, and Chandrasekaran 2014, with some modifications. Briefly, red cells present in defibrinated sheep blood (Ebafarma) were washed three times with PBS (1,000 g, 3 min). Then, 100 μl of 2 × 108 cells/ml were added to 96-well plates with 100 μl of NCs in serial dilution from 16 to 0.25 μg/ml. The combinations were incubated at 37°C for 1 h. Relative hemoglobin concentration in supernatants after centrifugation at 1700 g for 5 min was monitored by measuring optical absorbance (Abs) at 540 nm using a microplate reader (Biotek ELx808). Red blood cells incubated with PBS were considered as a negative control, and those incubated with water were used as positive controls for hemolysis. Percent hemolysis was calculated using the formula: Hemolysis (%) = 100 * (Abs treated cells−Abs negative control)/(Abs positive control−Abs negative control). The experiment was carried out in triplicate and three independent tests were performed.
Scratch Assay
The scratch assay was performed according to You et al. (2017). Briefly, a total of 106 cells of spontaneously immortalized human skin keratinocyte line (HaCaT), immortalized human fibroblasts (HFb) and NIH/3T3 murine fibroblasts were plated separately in six-well plates to obtain a confluent culture. The monolayer was then scraped straight with a 200 µl micropipette tip. Thereafter, cell debris was removed by washing the cells twice with PBS or culture medium. Silver nanocomposites suspended in RPMI 1640 and supplemented with 0.2% FBS (2 ml) were added carefully in the wells at concentration of 1 μg/ml of silver and immediately incubated at 37°C and 5% CO2 for up to 72 h. To monitor the cell migration or scratch closure, the plates were placed under an inverted Olympus CKX41 microscope coupled to an AmScope MU500 camera and images were acquired every 24 h. The test was performed in three independent assays by duplicates.
Murine Model Test
The efficacy of the silver nanocomposites was investigated using a murine model for subcutaneous sporotrichosis (Della Terra et al., 2017). Groups of five female C57BL/6 mice weighing 16–18 g (7–8 weeks old) were obtained from the Núcleo de Criação de Animais de Laboratório of the Universidade Federal do Rio de Janeiro (UFRJ, RJ, Brazil). To develop the infections mice were inoculated by subcutaneous injection of 25 µl (5 × 106 yeasts) into the left hind footpad, previously anesthetized with ketamine (100 mg/kg, ip) and xylazine (10 mg/kg, ip). Daily treatment with silver nanocomposites was started after lesion development (∼20 days). The application of AgNPs or AgNPs@Chi was by spray (three applications of ∼100 μl/at a time, from a suspension with 5 μg/ml of silver). The negative controls (ITC or PBS) were administered intravenously (i.v). Two independent tests with treatment and monitoring of injures were performed. The body weight, the paw diameter and the survival pattern were monitored in the second test. Digital Vernier caliper was used to measure the diameter of the paw.
Statistical Analysis
The experimental data were analyzed by one-way ANOVA and multiple comparisons tests (Sidak’s, and Dunnett’s for in vitro tests and Tukey’s for the in vivo experiments data) using Graph Pad Prism seven software. Statistical significance was considered at p value ˂ 0.05.
Results
Nanocomposites Characterization
Synthesis and characterization of the silver nanocomposites were carried out following previously published protocols (Artunduaga Bonilla et al., 2021a). The NCs used here displayed the same physical-chemical characteristics as observed previously by our group (Supplementary Figures S1, S2 for the physicochemical details).
Anti-Sporothrix Activity of NCs
Treatment with AgNPs inhibited ≥90% of S. brasiliensis and S. schenckii growth at concentrations of 0.12 and 0.25 μg/ml, respectively (Table 1). AgNPs functionalized with chitosan (AgNPs@Chi) displayed the same inhibitory activity at higher concentrations, ranging from 0.5 to 1 μg/ml. Thus, a 4-fold reduction in activity was observed. Remarkably, the effect of NCs was comparable and even more efficient than the activity exhibited by itraconazole (1 μg/ml for both species), a first-line drug to combat sporotrichosis. The reduced antifungal activity of AgNPs embedded in the chitosan occurred most probably due to the slower release of silver from the polymeric matrix. Similar concentrations of chemical reagents used as working solutions for the NCs synthesis did not impact fungal growth. The optical densities (OD) of the wells corresponding to the cells exposed to the chemical reagents ranged from 0.296 to 0.324, values similar to the fungal growth under control conditions (RPMI) (0.330 ± 0.08 OD).
Combinatory Effect of NCs With Conventional Antifungals Drugs
To explore the possibility of using silver nanocomposites combined with ITC or AMB as an unconventional therapy against Sporothrix, the combinatory effect was studied by the checkerboard method. As seen in Table 2, none of the combinations showed synergism with either ITC or AMB against the S. brasiliensis and S. schenckii strains tested. We observed an indifferent or non-interactive effect (FICi ˃0.5–4) for all experiments.
Nanocomposites Promote Ultrastructural Changes in S. brasiliensis
Under control conditions, yeasts of S. brasiliensis showed a uniform cell wall and a continuous plasma membrane. Intracellularly, the yeasts displayed regular nucleus and mitochondria with a homogeneous electron-dense cytoplasm (Figures 1A,B). Treatment with AgNPs promoted deformities in yeast morphology (Figures 1C,D). The exposition of the fungus to AgNPs promoted plasma membrane discontinuity, rupture of organelles and a heterogeneous density at the cytoplasm (Figure 1D, white arrows). In addition, the cell wall displayed a decreased electron-density. The detachment of the cytoplasmic membrane was also observed with the disintegration of the surface of the cells treated with AgNPs@Chi (Figures 1E,F). The images are representative of the effects observed in 20–30 cells viewed at high magnification and for each treatment. The ultrastructural characteristics described for the cells treated with the experimental nanocomposites correspond to the alterations observed in more than 80% of the cells visualized by each group.
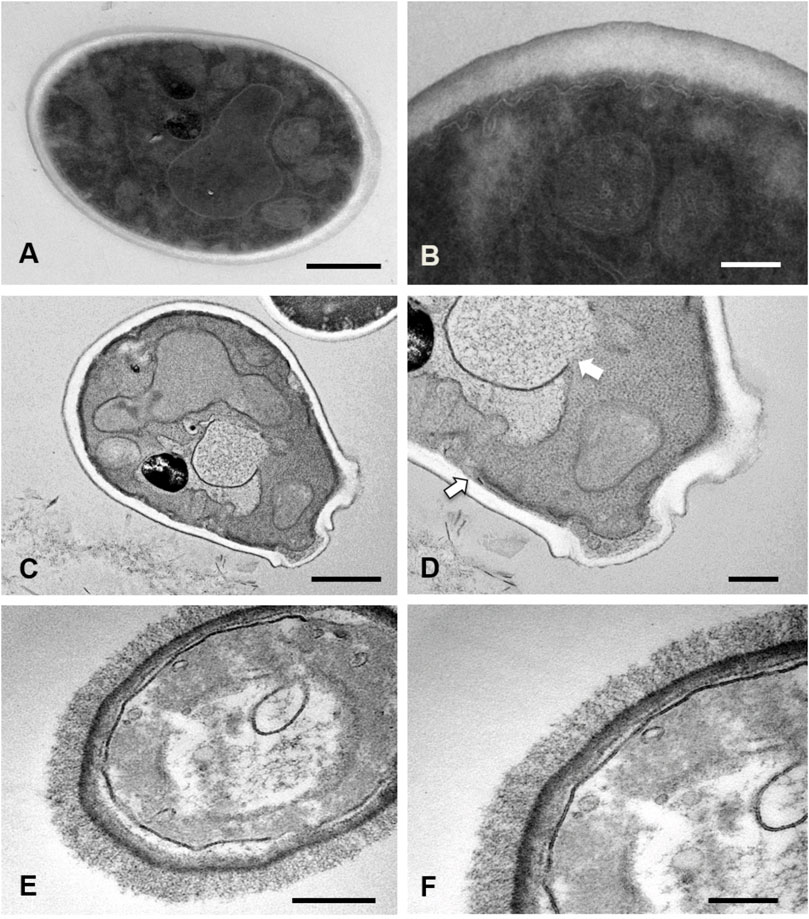
FIGURE 1. Ultrastructural analysis of Sporothrix brasiliensis treated with silver nanocomposites. S. brasiliensis ATCC 5110 treated for 48 h with MIC50 of nanocomposites were analyzed by TEM. Untreated yeasts exhibited an intact structure: compact cell wall, continuous cytoplasmic membrane and electrodense (A,B). While yeasts exposed to AgNPs showed damage to the integrity of the membrane of cytoplasmic organelles and the cell membrane (white arrows) (C,D). Cells treated with AgNPs@Chi exhibited membrane detachment and cell surface disaggregation (E,F). Bars scale = 500 nm (A,C,E) and 200 nm (B,D,F).
Silver Nanocomposites Have Low Red Blood Cell Toxicity
The hemolytic concentration of NCs capable of lysing 50% (HC50) of the red blood cells was approximately 2 and 8 μg/ml for AgNPs and the functionalized compound, respectively (Figure 2). For concentrations below 1 μg/ml, a modest variability in toxicity was observed, ranging between 20 and 30% when using AgNPs, and 15–18% for the AgNPs@Chi. The cytotoxic effect was directly relative to the concentration of the NCs, with a reduced activity observed for the functionalized compound. Red blood cells in presence of PBS showed no signs of hemolysis.
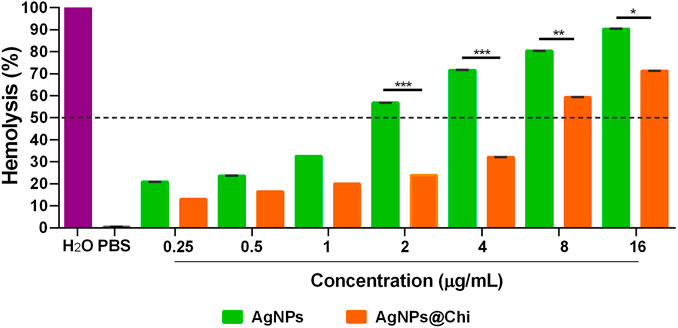
FIGURE 2. Hemolytic activity of silver nanocomposites. Sheep red blood cells (1 × 108 cells/ml) were exposed to NCs for 1 h. Hemoglobin, proportional to hemolysis, was measured at 540 nm. The data represents means with standard deviations of three independent assays performed by triplicate. The statistics analysis was performed using Sidak’s multiple comparison test, *p < 0.05; **p < 0.01; ***p < 0.001.
Nanocomposites Stimulate in vitro Keratinocytes and Fibroblasts Migration
Keratinocytes and fibroblast proliferation and/or migration was individually visualized by the scratch assay. The effect on cellular migration/tissue repair was investigated at 1 μg/ml of NCs, an efficient concentration to inhibit Sporothrix spp. growth. Figure 3 demonstrates that restoration of cell density for all cell lines was faster in the group stimulated with AgNPs@Chi. For HaCaT, cellular restoration in the presence of nanoparticles was considerably higher than control conditions (Figure 3A). For these cells the migration was quantified by counting their number in the scratch area, delimited by red lines, at each time point, confirming a better wound closure (p ˂ 0.05) after 48–72 h of exposure to the functionalized compound (Figure 3B). The cell density of human (Figure 3C) and murine (Figure 3D) fibroblasts, after 24 h of exposure to nanocomposites was considerably higher, making the cell number determination inaccessible. Qualitatively, a higher cell convergence with AgNPs@Chi was observed, reaching the total closure of the scratch in the first 48 h.
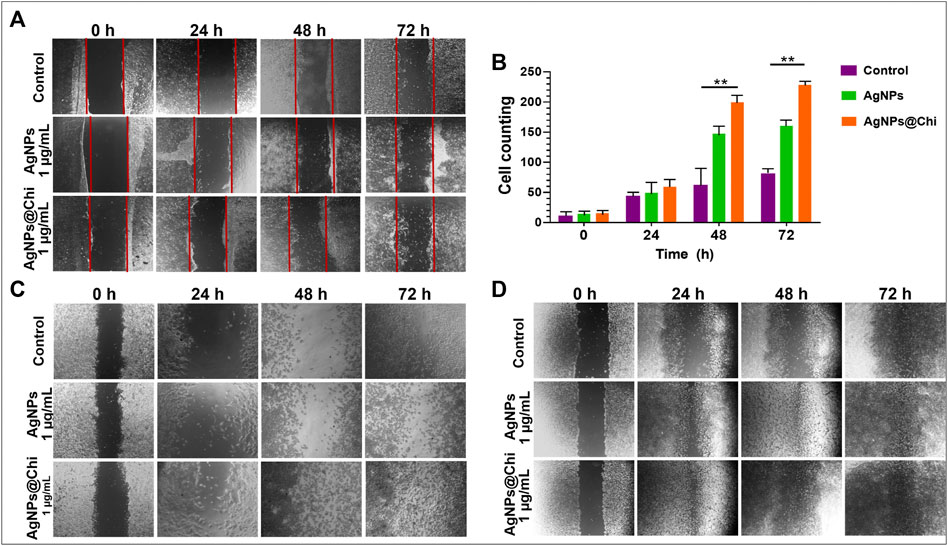
FIGURE 3. In vitro migration of mammalian cells. Cells (106) were plated in 6-well plates and stimulated for up to 72 h to nanocomposites previously diluted in RPMI 1640/0.2% FBS. The cell monolayer was scratched with a tip and monitored until cell convergence. Images representative both (A) Human keratinocytes and (C) fibroblast, and (D) NIH/3T3 fibroblast cells were acquired using a 4× objective lens [except for those corresponding to 24 h in (C) (10×)]. (B) represents the keratinocytes counting [cells within the area bounded by the red lines in (A)] of an experiment performed in duplicate. Experimental data was analyzed using Dunnett’s multiple comparison test, **p ˂ 0.01.
Silver Nanocomposites are Effective Against Murine Sporotrichosis
Considering the anti-Sporothrix efficacy of NCs in vitro along with a potential activity to stimulate keratinocytes and fibroblasts migration, we investigated their effect in a murine sporotrichosis model. The timeline followed for in vivo testing is shown in Figure 4. For the first test (Figure 4A), treatment was started on day 25 post-infection, once swelling and skin injure were evident in the paw of the mice. (Figure 5, day 1). AgNPs, AgNPs@Chi and ITC were administered separately. Treatment was performed daily for 35 days (Figure 5). Under these conditions the paw swelling of the control mice continued even at day 35 although the lesion has diminished. Treatment with AgNPs displayed paw recovery with swelling reduction at day 25. Finally, treatment with AgNPs@Chi generated the fastest recovery, reducing inflammation and skin lesion within 15 days of treatment (Figure 5). At the end of the experiment (day 35), animals treated with NCs showed complete recovery from the infection. Monitoring of the mice was performed for 3 weeks after the last day of treatment. Silver nanocomposites-treated mice had no development of new injures. Mice treated with ITC displayed inflammation reduction at endpoint test when the control group showed chronic lesions with mild variations in size and swelling. Remarkably, about 20% of the mice under control conditions died (data not shown).
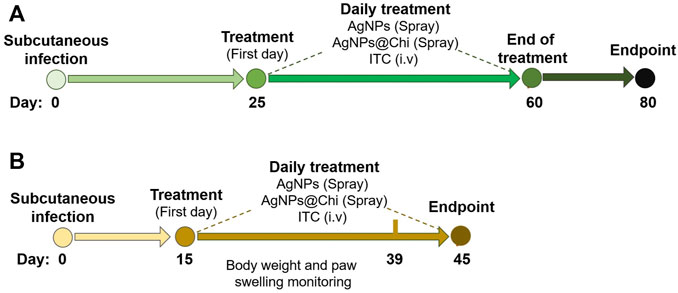
FIGURE 4. Experimental scheme for in vivo assays. The strategy used to develop the murine subcutaneous infection and antifungal treatment was represented as a timeline. Yeasts of S. brasiliensis (5 × 106 cells) were inoculated by subcutaneously injection of in the left hind paw. Daily Treatment was started once the lesions were evident in all mice. In the first test (A), treatments for 35 days were performed. Then, the mice were monitored for 20 days. For the second experiment (B), a higher virulent strain of S. brasiliensis ATCC 5110 was used, and topical treatments with NCs were administered for 30 days.
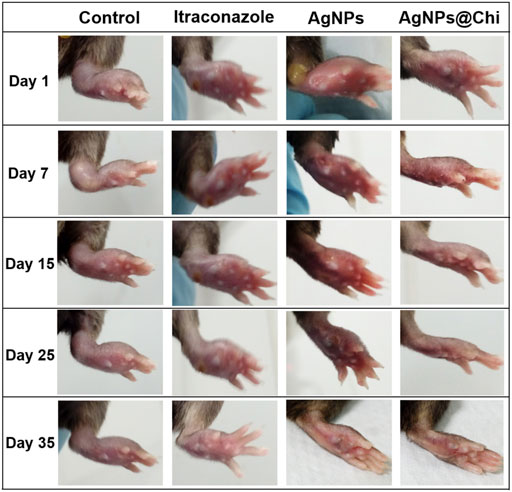
FIGURE 5. Protective effect of nanoparticles in a murine model of mild sporotrichosis. Cells (5 × 106 cells/25 μl) of S. brasiliensis was inoculated into the paw C57BL/6 mice. Treatments with nanocomposites were performed by spray (three applications from a 5 μg/ml silver suspension). Itraconazole was administered intravenously (0.5 mg/kg/day/per tail). For intravenous treatment, mice were previously anesthetized with ketamine and xylazine. The images correspond to days 1 to day 35 of the treatment started 25 days’ post-infection. Mice treated with AgNPs@Chi exhibited better recovery and during the 3 weeks of monitoring after the end of the treatment, they showed no lesions or signs of disease.
Considering that the strain exhibited low virulence according to the minor lesions observed in mice, we used a recent pick from the same strain (S. brasiliensis 5110) with two passages in culture medium from frozen stock to ensure the protecting effect of the NCs. In this case, a severe infection was since the skin lesions were more serious (Figure 6A) and both swelling and injures were developed in less time, at 15 days’ post-infection (Figure 6, day 1). Treatment was started on day 15 after yeast’ inoculation, as indicated in the timeline scheme (Figure 4B). The NCs were administered in the same way as in the first test. The course of the infection was monitoring by the measure of the paw swelling and body weight.
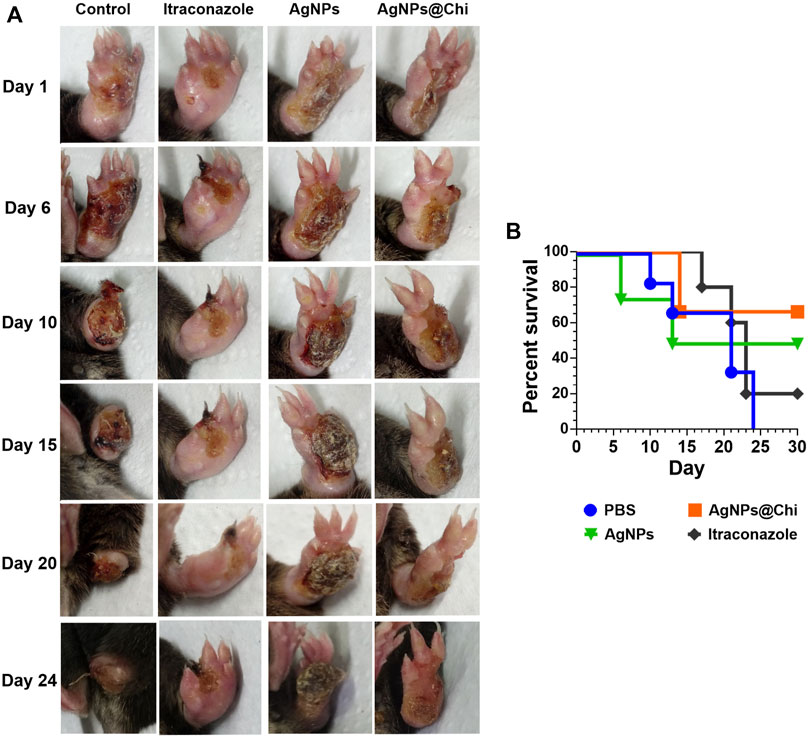
FIGURE 6. Protective effect of nanoparticles in a murine model of severe sporotrichosis. (A) Mice subcutaneously infected with S. brasiliensis were treated with silver nanocomposites and itraconazole. For AgNPs and AgNPs@Chi, the treatment was carried out by spray (three applications from a 5 μg/ml silver suspension). Itraconazole was administered intravenously (0.5 mg/kg/day/per tail) as a control treatment. (B) Survival of mice infected with S. brasiliensis. Daily treatment was started 15 days after infection, when the injuries were evident. Once the last mouse in the control group died, mice survivors from other groups were treated for a further 6 days.
The silver nanocomposites treatment reduced the severity of the infection resulting in a better prognosis since there was no loss of the paw, as observed at day 10 in the control group. Although all mice from the control group displayed a severe tissue loss, swelling measurements were continued at the base of the paw to monitor the infection progress, moving to the surrounding tissues. In this sense, from day 10 the swelling data correspond to the diameter of the paw’s base. Minimal variations were observed in the diameter of the base of the paw with no signs of fungal dissemination, suggesting that the infection was localized. Weight loss was lower in mice from the group treated with AgNPs@Chi comparing to control (p = 0.001) and ITC (p ˂ 0.05) groups (Figure 7A). In addition, AgNPs@Chi-treated mice showed paw swelling reduction, being significantly different from the ITC-treated mice (p ˂ 0.001) suggesting a decreased inflammatory response (Figure 7B). Until day 24 of treatment, the mice treated with NCs no showed significant difference in weight variation (Figure 7A). From day 14, a greater paw swelling of AgNPs-treated mice was observed when contrasted to those treated with ITC (p ˂ 0.05) and with the functionalized compound (p ˂ 0.001) (Figure 7B). AgNPs@Chi treated mice showed 70% survival, while 50% of those treated with AgNPs survived at the end of the experiment (Figure 6B).
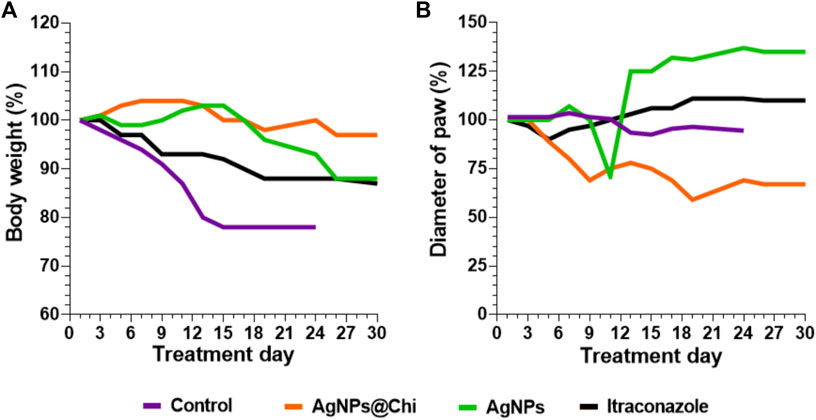
FIGURE 7. Paw diameter and body weight evaluation in mice infected with S. brasiliensis. C57BL/6 mice were subcutaneously infected with yeasts (5 × 106 cells/25 µl). The injuries were evident 15 days’ post-infection, then topical (silver nanocomposites) or intravenous (ITC) antifungal treatment was started. Mice from the nanocomposites and ITC groups were treated and followed up to day 30. The mice weight (A) and the inflamed paw diameter (B) from each tested group were monitored.
Discussion
In previous studies we have exploited the use of AgNPs as antifungal formulations with a reduced toxicity to the host (Artunduaga Bonilla et al., 2015; Artunduaga Bonilla et al., 2021a). In this work we investigate the application of silver nanocomposites to combat sporotrichosis. Our study compared the anti-Sporothrix efficiency of AgNPs when incorporated or not into the polymeric matrix chitosan, strategy that significantly increased the compound biocompatibility without compromising the antifungal effect (Cinteza et al., 2018; Artunduaga Bonilla et al., 2021a). In addition, we observed a potential anti-inflammatory effect and confirmed tissue repair properties already suggested for silver and chitosan in skin infections (You et al., 2017; Sandri et al., 2019; Ye, Cheng, and Yu 2019).
The NCs used in this work exhibited the same physicochemical properties previously observed by our group (Artunduaga Bonilla et al., 2021a), suggesting a highly reproducible strategy for NCs synthesis. It is worth mentioning that although AgNPs display an absorption peak between 400–420 nm produced by the AgNPs-light beam interaction, the absorption shoulder at around 410 nm observed in the synthesized AgNPs has already been reported when these are obtained by chemical reduction with cysteine (Khan et al., 2009). Khan and colleagues have attributed the “shoulder” of absorption to the possible formation of an insoluble cysteine-Ag complex. The XRD analysis displayed the crystalline phase of silver within the NCs. Other peaks were observed corresponding to impurities that would consequence from electrostatic interactions between Ag+ (not reduced) and bromide (Br−) ion from CTAB (Khan et al., 2009). Conventional TEM analysis strongly indicates that AgNPs are spherical, but additional experiments are required to confirm their exact morphology. Small homogeneous particles were displayed, a physical characteristic commonly related to a higher antimicrobial effect (Ivask et al., 2014; Dasgupta et al., 2015; Lee and June 2019).
Several groups have demonstrated the effectiveness of chitosan as a vehicle for drug administration and to increase the biocompatibility of metal nanomaterials (Cinteza et al., 2018; El-Sherbiny et al., 2019). For instance, Cinteza et al. (2018) used chitosan as a capping agent, stabilizing and enhancing biocompatibility of AgNPs with antibacterial effects. In our experiments, functionalization of AgNPs with chitosan was performed using a modified cross-linking method, trapping AgNPs into the polymeric matrix by chitosan precipitation at the particles surface. Chitosan increased the positive surface charge of AgNPs due to the free amino groups (+25 mV for AgNPs@Chi, compared with +4.5 mV for AgNPs), which could benefit the electrostatic interaction with the negative surface of the fungal cells. In addition, chitosan incorporation resulted in a gradual silver release. These data, together with the fact that chitosan promotes an interface barrier between the metal and mammalian cells, could potentially decrease the toxic effect of the AgNPs (Cinteza et al., 2018; Hernández-Rangel et al., 2019).
AgNPs were able to attach to the fungal cell wall where they could stimulate changes in strength and permeability. These changes, ultimately, can cause cell lysis with extrusion of intracellular material, leading to fungal death (Dakal et al., 2016; Durán et al., 2016). Nevertheless, there are considerable evidences showing that ionic silver (Ag+) is associated with antimicrobial activities, particularly since the silver cation contains a high affinity for negatively charged molecules such as DNA, as well as an extensive reactivity with hydroxyl, sulfate and phosphate chemical groups (Durán et al., 2016; Choi et al., 2018; Kędziora et al., 2018). Thus, once Ag+ are transported to their biological targets and interact with biomolecules and regulatory enzymes, they inhibit vital processes such as replication, respiration and protein synthesis. In this sense, the role of AgNPs also consists in generating a continuous flow of Ag+ ions through the oxidation of Ag0. According to our results, both AgNPs and AgNPs@Chi were able to inhibit the in vitro growth of Sporohtrix species at concentrations lower than 1 μg/ml. To our knowledge, only one study has tested silver compounds against Sporothrix. Mathias et al., 2020 investigated the efficacy of silver salts of Keggin-type heteropolyacid (Ag-HPA, non-nanoparticulate compound) against S. schenckii ATCC 32285 and isolates from feline sporotrichosis. They observed MIC values between 8 and 128 μg/ml, in addition to a synergistic interaction of Ag-HPA salts with ITC and AMB. These concentrations were up to 128 times higher than that used in our work. In addition, no synergism between NCs and those drugs were found in our experimental conditions. Such disparities could be explained by the chemical differences between Ag-HPA salts and AgNPs, the strains tested and also by the methodology used to determine synergism. However, the yeast ultrastructural effects observed in both studies were similar. Cells treated with silver composites exhibited detachment and discontinuity of the cell membrane, disruption of cytoplasmic organelles and cell wall disaggregation. Increased permeability of fungal cells due to damage to the fungal wall and membrane were observed in cells treated with both chitosan-functionalized AgNPs and unmodified AgNPs. It is possible that the antifungal activity of NCs is caused primarily by the alteration of the fungal cell structure allowing the penetration of AgNPs/Ag+ and causing biochemical alterations already described by Dakal and colleagues, such as oxidative stress derivative by the higher production of ROS and free radicals (Dakal et al., 2016). However, additional experiments are necessary to confirm that the NCs are localized within the cells. Remarkably, the incorporation of chitosan also reduced the hemolytic activity of the NCs. Thus, chitosan incorporation to AgNPs reduces NCs toxicity and does not compromise the antifungal effect against Sporothrix spp. in vitro.
The process of wound healing is particularly relevant during cutaneous and subcutaneous infections. Some studies have suggested that metallic nanoparticles have inherent features to promote wound repair (Tian et al., 2007; Liu et al., 2010; Sandri et al., 2019). The AgNPs generated in our work considerably stimulated proliferation and migration of keratinocytes and fibroblasts, primary criteria for successful wound healing (Liu et al., 2010; You et al., 2017). Remarkably, the presence of chitosan improved the stimulatory effect. These results are in accordance with the data published by You and colleagues (You et al. (2017) showing that antibacterial AgNPs loaded in collagen/chitosan scaffolds induced the transformation of fibroblasts into myofibroblasts, intensifying dermal regeneration and injury healing. The use of chitosan to stimulate proliferation and migration of keratinocytes and fibroblasts was also showed by Sharma and colleagues (Sharma et al. 2021). In their studies, the association of chitosan with chondroitin sulfate (CH-CS) resulted in proliferation and migration of keratinocytes and fibroblasts. In addition, CH-CS displayed a potent effect when tested in a rat skin-excisional wound model.
The potent antifungal activity of AgNPs and AgNPs@Chi against S. brasiliensis and S. schenckii associated with their ability to stimulate keratinocytes and fibroblasts suggested that these NCs could be tested in vivo to control sporotrichosis. In addition, we recently demonstrated that mice exposed to AgNPs at doses of 0.16 mg/kg/day for 30 days did not show silver bioaccumulation in organs such as spleen, liver, kidneys, lungs, and brain (Artunduaga Bonilla et al., 2021a). The antifungal efficacy of the NCs was then investigated in a subcutaneous model of murine sporotrichosis. The impact of the virulence strain was also evaluated. For our first infection model, we prepared the inoculum of S. brasiliensis from a pick with serial passages in the laboratory, a condition that decreases the strain virulence. Mild infections were previously reported under these conditions (Lima, Schäffer, and Borba 2003; Converse et al., 2010; Artunduaga Bonilla et al., 2021b). Treatment with AgNPs@Chi reduced the paw swelling, indicating a decrease of the local inflammatory response by the end of the first and third week, respectively. In fact, previous studies showed that AgNPs and AgNPs-chitosan have the ability to suppress pro-inflammatory cytokine release in tissue specimens (You et al., 2017; Ye, Cheng, and Yu 2019). However, additional experiments are necessary to confirm the NCs anti-inflammatory effect in our model. AgNPs@Chi and AgNPs showed a better outcome when compared to ITC and clinical cure was clearly reached on day 35 for both NCs. The efficacy of NCs was then confirmed using a strain displaying a higher virulence profile (Artunduaga Bonilla et al., 2021b). A severe paw loss in mice treated with PBS confirmed the virulence phenotype. Remarkably, AgNPs@Chi was more efficient than AgNPs and ITC in both in vivo experiments, leading to a faster and better tissue recover. Corroborating with that, a slight variation in body weight and less progression of injury were observed in mice treated with AgNPs@Chi. Accordingly, the progressive release of silver incorporated in chitosan could favor both the antifungal action on the infection causal agent and the recovery of the affected tissue, since the compound was released continuously.
In conclusion, silver nanoparticles obtained by chemical reduction with cysteine were effective in inhibiting the in vitro growth of Sporothrix yeasts in concentrations comparable to the treatment of first choice, itraconazole. Furthermore, NCs functionalization with chitosan increased the biocompatibility of silver and promoted the gradual release of AgNPs/Ag+, stimulating the prolonged antifungal effect and a better tissue recovery. Together, the results here suggest that AgNPs@Chi can be a good and effective choice to be exploited against chronic subcutaneous infection that requires prolonged treatment such as cutaneous sporotrichosis.
Data Availability Statement
The original contributions presented in the study are included in the article/Supplementary Material, further inquiries can be directed to the corresponding author.
Ethics Statement
The animal study was reviewed and approved by the Commission on Ethics in the Use of Animals (CEUA, Federal University of Rio de Janeiro).
Author Contributions
JA, LH, and LN contributed to conception and design of the study. JA performed the synthesis and characterization of the nanocomposites. JA and LH performed the in vitro tests. JA, LH, and AG performed the in vivo tests and statistical analysis. JA wrote the first draft of the manuscript. All authors contributed to manuscript revision and approved the submitted version.
Funding
This work was supported by grants from the Brazilian agency Conselho Nacional de Desenvolvimento Científico e Tecnológico (CNPq, grants 311179/2017-7 and 408711/2017-7 to LN), FAPERJ (E-26/202.809/2018 to LN) and Coordenação de Aperfeiçoamento de Pessoal de Nível Superior (CAPES, Finance Code 001). JA was supported by Organization of American States-Coimbra Group of Brazilian Universities (OAS-CGBU, 2015). LH was supported by CAPES (2019).
Conflict of Interest
The authors declare that the research was conducted in the absence of any commercial or financial relationships that could be construed as a potential conflict of interest.
Publisher’s Note
All claims expressed in this article are solely those of the authors and do not necessarily represent those of their affiliated organizations, or those of the publisher, the editors and the reviewers. Any product that may be evaluated in this article, or claim that may be made by its manufacturer, is not guaranteed or endorsed by the publisher.
Acknowledgments
The authors thank Rodrigo Araújo Gonçalves and Douglas Cordeiro de Oliveira, from the Departamento de Química, Pontifícia Universidade Católica do Rio de Janeiro for technical support in the quantification of silver within the nanocomposites.
Supplementary Material
The Supplementary Material for this article can be found online at: https://www.frontiersin.org/articles/10.3389/fnano.2022.857681/full#supplementary-material
References
Adler-Moore, J. R. E. L., Lewis, R. E., Brüggemann, R. J. M., RijndersGroll, B. J. A., Groll, A. H., and Walsh, T. J. (2019). Preclinical Safety, Tolerability, Pharmacokinetics, Pharmacodynamics, and Antifungal Activity of Liposomal Amphotericin B. Clin. Infect. Dis. : Official Publ. Infect. Dis. Soc. America 68 (Suppl. 4), S244–S259. doi:10.1093/cid/ciz064
Almeida-Paes, R., Figueiredo-Carvalho, M. H. G. F., Almeida-, S., Brito-Santos, F., Almeida-Silva, F., Oliveira, M. M. E., et al. (2016). Melanins Protect Sporothrix Brasiliensis and Sporothrix Schenckii from the Antifungal Effects of Terbinafine. PLoS One 11 (3), e0152796. doi:10.1371/journal.pone.0152796
Almeida-Paes, R., Oliveira, M. M. E., Freitas, D. F. S., Valle, A. C. F. d., Gutierrez-Galhardo, M. C., and Zancopé-Oliveira, R. M. 2017. Refractory Sporotrichosis Due toSporothrix Brasiliensisin Humans Appears to Be Unrelated Toin Vivoresistance. Med. Myco. 55 (5): myw103–17. doi:10.1093/mmy/myw103
Arrillaga-Moncrieff, I., Capilla, J., Mayayo, E., Marimon, R., Marine, M., Genis, J., et al. (2009). Different Virulence Levels of the Species of Sporothrix in a Murine Model. Clin. Microbiol. Infect. 15 (7), 651–655. doi:10.1111/j.1469-0691.2009.02824.x
Artunduaga Bonilla, J. J., Honorato, L., Cordeiro de Oliveira, D. F., Araújo Gonçalvesde Oliveira, R., GuimarãesGonçalves, A., Miranda, K., et al. (2021a). Silver Chitosan Nanocomposites as a Potential Treatment for Superficial Candidiasis. Med. Mycol. 59, 993–1005. doi:10.1093/mmy/myab028
Artunduaga Bonilla, J. J., Honorato, L., Haranahalli, K., Dib Ferreira Gremião, I., Pereira, S. A., Guimarães, A., et al. (2021b). Antifungal Activity of Acylhydrazone Derivatives against Sporothrix Spp. Antimicrob. Agents Chemother. 65. doi:10.1128/AAC.02593-20
Artunduaga Bonilla, J. J., Paredes Guerrero, D. J., Sánchez Suárez, C. I., Ortiz López, C. C., Torres Sáez, R. G., Claudia, C., et al. (2015). In Vitro Antifungal Activity of Silver Nanoparticles against Fluconazole-Resistant Candida Species. World J. Microbiol. Biotechnol. 31 (11), 1801–1809. doi:10.1007/s11274-015-1933-z
Baptista, P. V., McCusker, M. P., Carvalho, A., Ferreira, D. A., Mohan, N. M., Martins, M., et al. (2018). Nano-Strategies to Fight Multidrug Resistant Bacteria-"A Battle of the Titans". Front. Microbiol. 9 (JUL), 1–26. doi:10.3389/fmicb.2018.01441
Baranwal, A., Srivastava, A., Kumar, P., Bajpai, V. K., Maurya, P. K., and Chandra., P. (2018). Prospects of Nanostructure Materials and Their Composites as Antimicrobial Agents. Front. Microbiol. 9 (MAR). doi:10.3389/fmicb.2018.00422
Bellich, B., D’Agostino, I., Semeraro, S., Gamini, A., and Cesàro, A. (2016). "The Good, the Bad and the Ugly" of Chitosans. Mar. Drugs 14, 99. doi:10.3390/md14050099
Bonilla, J. J. A., Guerrero, D. J. P., Sáez, R. G. T., Ishida, K., Fonseca, B. B., Rozental, S., et al. (2017). Green Synthesis of Silver Nanoparticles Using Maltose and Cysteine and Their Effect on Cell Wall Envelope Shapes and Microbial Growth of Candida Spp. j nanosci nanotechnol 17 (3), 1729–1739. doi:10.1166/jnn.2017.12822
Chakrabarti, A., Bonifaz, A., Gutierrez-Galhardo, M. C., Mochizuki, T., and Li, S. (2015). Global Epidemiology of Sporotrichosis. Med. Mycol. 53 (1), 3–14. doi:10.1093/mmy/myu062
Choi, Y., Kim, H.-A., Kim, K.-W., and Lee, B.-T. (2018). Comparative Toxicity of Silver Nanoparticles and Silver Ions to Escherichia Coli. J. Environ. Sci. 66 (April), 50–60. doi:10.1016/j.jes.2017.04.028
Cinteza, L., Scomoroscenco, C., Voicu, S., Nistor, C., Nitu, S., Trica, B., et al. (2018). Chitosan-Stabilized Ag Nanoparticles with Superior Biocompatibility and Their Synergistic Antibacterial Effect in Mixtures with Essential Oils. Nanomaterials 8 (10), 826. doi:10.3390/nano8100826
Converse, P. J., EisenachEisenach, K. D., Theus, S. A., Nuermberger, E. L., Tyagi, S., Ly, L. H., et al. (2010). The Impact of Mouse Passaging of Mycobacterium Tuberculosis Strains Prior to Virulence Testing in the Mouse and Guinea Pig Aerosol Models. PLoS One 5 (4), e10289. doi:10.1371/journal.pone.0010289
da Rocha, R. F. D. B., Schubach, T. M. P., Pereira, S. A., dos Reis, É. G., Carvalho, B. W., and Gremião, I. D. F. (2018). Refractory Feline Sporotrichosis Treated with Itraconazole Combined with Potassium Iodide. J. Small Anim. Pract. 59 (11), 720–721. doi:10.1111/jsap.12852
Dakal, T. C., Kumar, A., Majumdar, R. S., and Yadav, V. (2016). Mechanistic Basis of Antimicrobial Actions of Silver Nanoparticles. Front. Microbiol. 7 (NOV), 1–17. doi:10.3389/fmicb.2016.01831
Danhier, F., Ansorena, E., Silva, J. M., Coco, R., Le Breton, A., and Préat, V. (2012). Régis Coco, Aude Le Breton, and Véronique PréatPLGA-Based Nanoparticles: An Overview of Biomedical Applications. J. Controlled Release 161 (2), 505–522. doi:10.1016/j.jconrel.2012.01.043
Dasgupta, N., Ranjan, S., Rajendran, B., Manickam, V., Ramalingam, C., Avadhani, G. S., et al. (2015). Thermal Co-reduction Approach to Vary Size of Silver Nanoparticle: Its Microbial and Cellular Toxicology. Environ. Sci. Pollut. Res. 23 (5), 4149–4163. doi:10.1007/s11356-015-4570-z
de Lima, R. F., SchäfferSchäffer, G. V., and Borba, C. d. M. (2003). Variants of Sporothrix Schenckii with Attenuated Virulence for Mice. Microbes Infect. 5 (11), 933–938. doi:10.1016/S1286-4579(03)00181-3
Della Terra, P. P., RodriguesRodrigues, A. M., Fernandes, G. F., Nishikaku, A. S., Burger, E., and de Camargo, Z. P. (2017). Exploring Virulence and Immunogenicity in the Emerging Pathogen Sporothrix Brasiliensis. Plos Negl. Trop. Dis. 11 (8), e0005903–23. doi:10.1371/journal.pntd.0005903
Duangkaew, L., Yurayart, C., Limsivilai, O., Chen, C., and Kasorndorkbua, C. (2019). Cutaneous Sporotrichosis in a Stray Cat from Thailand. Med. Mycol. Case Rep. 23 (March), 46–49. doi:10.1016/j.mmcr.2018.12.003
Durán, N., Durán, M., de Jesus, M. B., Seabra, A. B., Fávaro, W. J., and Nakazato, G. (2016). Silver Nanoparticles: A New View on Mechanistic Aspects on Antimicrobial Activity. Nanomedicine: Nanotechnology, Biol. Med. 12 (3), 789–799. doi:10.1016/j.nano.2015.11.016
El-Sherbiny, I. M., Sedki, M., and Sedki, M. (2019). Green Synthesis of Chitosan-Silver/Gold Hybrid Nanoparticles for Biomedical Applications. Methods Mol. Biol. (Clifton, N.J.) 2000, 79–84. 84. doi:10.1007/978-1-4939-9516-5_7
Etchecopaz, A. N., Lanza, N., Toscanini, M. A., Devoto, T. B., Pola, S. J., Daneri, G. L., et al. (2020). Sporotrichosis Caused by Sporothrix Brasiliensis in Argentina: Case Report, Molecular Identification and In Vitro Susceptibility Pattern to Antifungal Drugs. J. de Mycologie Médicale 30, 100908. October, 100908. doi:10.1016/j.mycmed.2019.100908
Gaballah, S. T., El-Nazer, H. A., Abdel-MonemAbdel-MonemEl-Liethy, R. A. M. A., El-Liethy, M. A., Hemdan, B. A., and Rabie, S. T. (2019). Synthesis of Novel Chitosan-PVC Conjugates Encompassing Ag Nanoparticles as Antibacterial Polymers for Biomedical Applications. Int. J. Biol. Macromolecules 121, 707–717. doi:10.1016/j.ijbiomac.2018.10.085
Genç, A., Patarroyo, J., Sancho-Parramon, J., Bastús, N. G., Puntes, V., and Arbiol, J. (2017). Hollow Metal Nanostructures for Enhanced Plasmonics: Synthesis, Local Plasmonic Properties and Applications. Nanophotonics 6 (1), 193–213. doi:10.1515/nanoph-2016-0124
Gilaberte, Y., Aspiroz, C., Alejandre, M. C., Andres-Ciriano, E., Fortuño, B., Charlez, L., et al. (2014). Cutaneous Sporotrichosis Treated with Photodynamic Therapy: An In Vitro and In Vivo Study. Photomed. Laser Surg. 32 (1), 54–57. doi:10.1089/pho.2013.3590
Gold, J. A. W., Derado, G., Mody, R. K., and Benedict., K. (2016). Sporotrichosis-Associated Hospitalizations, United States, 2000-2013. Emerg. Infect. Dis. 22 (10), 1817–1820. doi:10.3201/eid2210.16067110.3201/eid2210/160671
Goyal, R., Macri, L. K., Kaplan, H. M., and Kohn., J. (2016). Nanoparticles and Nanofibers for Topical Drug Delivery. J. Controlled Release 240, 77–92. doi:10.1016/j.jconrel.2015.10.049
Gremião, I. D. F., Martins da Silva da Rocha, E., Montenegro, H., Carneiro, A. J. B., Xavier, M. O., de Farias, M. R., et al. (2020b). Guideline for the Management of Feline Sporotrichosis Caused by Sporothrix Brasiliensis and Literature Revision. Braz. J. Microbiol. 52, 107–124. [Publication of the Brazilian Society for Microbiology], September. doi:10.1007/s42770-020-00365-3
Gremião, I. D. F., MirandaRodrigues, L. H. M., Reis, E. G., Rodrigues, A. M., and Pereira, S. A. (2017). Zoonotic Epidemic of Sporotrichosis: Cat to Human Transmission. Plos Pathog. 13 (1), e1006077–8. doi:10.1371/journal.ppat.1006077
Gremião, I. D. F., Oliveira, M. M. E., Monteiro de Miranda, L. H., Saraiva Freitas, D. F., and Pereira, S. A. (2020a). Geographic Expansion of Sporotrichosis, Brazil. Emerg. Infect. Dis. 26 (3), 621–624. doi:10.3201/eid2603.190803
Hamill, R. J. (2013). Amphotericin B Formulations: A Comparative Review of Efficacy and Toxicity. Drugs 73 (9), 919–934. doi:10.1007/s40265-013-0069-4
Hernández-Rangel, A., Silva-Bermudez, P., España-Sánchez, B. L., Luna-Hernández, E., Almaguer-Flores, A., Ibarra, C., et al. (2019). Fabrication and In Vitro Behavior of Dual-Function Chitosan/Silver Nanocomposites for Potential Wound Dressing Applications. Mater. Sci. Eng. C 94 (November 2017), 750–765. doi:10.1016/j.msec.2018.10.012
Hsiao, I.-L., Hsieh, Y.-K., Wang, C.-F., Chen, I.-C., and Huang, Y.-J. (2015). Trojan-Horse Mechanism in the Cellular Uptake of Silver Nanoparticles Verified by Direct Intra- and Extracellular Silver Speciation Analysis. Environ. Sci. Technol. 49 (6), 3813–3821. doi:10.1021/es504705p
Ivask, A., Kurvet, I., Kasemets, K., Blinova, I., Aruoja, V., Suppi, S., et al. (2014). Size-Dependent Toxicity of Silver Nanoparticles to Bacteria, Yeast, Algae, Crustaceans and Mammalian Cells In Vitro. PLoS ONE 9 (7), e102108. doi:10.1371/journal.pone.0102108
Kamal Azam, N. K., Selvarajah, G. T., Santhanam, J., Abdul Razak, M. F., Ginsapu, S. J., James, J. E., et al. (2019). Molecular Epidemiology of Sporothrix Schenkii Isolates in Malaysia. Med. Mycol. 58, 617–625. doi:10.1093/mmy/myz106
Kano, R., Okubo, M., SiewSiew, H. H., Kamata, H., and Hasegawa, A. (2015). Molecular Typing ofSporothrix Schenckiiisolates from Cats in Malaysia. Mycoses 58 (4), 220–224. doi:10.1111/myc.12302
Kędziora, A., Speruda, M., Krzyżewska, E., Rybka, J., Łukowiak, A., and Bugla-Płoskońska, G. (2018). Similarities and Differences between Silver Ions and Silver in Nanoforms as Antibacterial Agents. Ijms 19 (2), 444. doi:10.3390/ijms19020444
Khan, Z., Al-Tnabaiti, S. A., El-Mossalamy, E. H., and Obaid, A. Y. (2009). Effect of Macromolecule Poly(Vinyl Alcohol) on the Growth of Cetyltrimethylammonium Bromide Stabilized Ag-Nanoparticles. Colloids Surf. A: Physicochemical Eng. Aspects 352 (1–3), 31–37. doi:10.1016/j.colsurfa.2009.09.045
Kumari, A., Yadav, S. K., and Yadav, S. C. (2010). Biodegradable Polymeric Nanoparticles Based Drug Delivery Systems. Colloids Surf. B: Biointerfaces 75 (1), 1–18. doi:10.1016/j.colsurfb.2009.09.001
Kyriakidis, I., Tragiannidis, A., Munchen, S., and GrollGroll, A. H. (2017). Clinical Hepatotoxicity Associated with Antifungal Agents. Expert Opin. Drug Saf. 16 (2), 1–17. doi:10.1080/14740338.2017.1270264
Lee, S., and Jun, B.-H. (2019). Silver Nanoparticles: Synthesis and Application for Nanomedicine. Ijms 20 (4), 865. doi:10.3390/ijms20040865
Liu, X., Lee, P.-y., Ho, C.-m., Lui, V. C. H., Chen, Y., Che, C.-m., et al. (2010). Silver Nanoparticles Mediate Differential Responses in Keratinocytes and Fibroblasts during Skin Wound Healing. ChemMedChem 5 (3), 468–475. doi:10.1002/cmdc.200900502
Lloret, A., Hartmann, K., Pennisi, M. G., Ferrer, L., Addie, D., Belák, S., et al. (2013). Sporotrichosis in Cats. J. Feline Med. Surg. 15 (7), 619–623. doi:10.1177/1098612X13489225
Marková, Z., Šišková, K., Filip, J., Šafářová, K., Prucek, R., Panáček, A., et al. (2012). Chitosan-Based Synthesis of Magnetically-Driven Nanocomposites with Biogenic Magnetite Core, Controlled Silver Size, and High Antimicrobial Activity. Green. Chem. 14 (9), 2550–2558. doi:10.1039/c2gc35545k
Mathias, L., Almeida, J., Passoni, L., Gossani, C., Taveira, G., Gomes, V., et al. (2020). Antifungal Activity of Silver Salts of Keggin-type Heteropolyacids against Sporothrix Spp. J. Microbiol. Biotechnol. 30 (4), 540–551. doi:10.4014/jmb.1907.07064
Monteiro, D. R., Negri, M., Silva, S., Gorup, L. F., Camargo, E. R. d., Oliveira, R., et al. (2014). Adhesion of Candida Biofilm Cells to Human Epithelial Cells and Polystyrene after Treatment with Silver Nanoparticles. Colloids Surf. B: Biointerfaces 114, 410–412. doi:10.1016/j.colsurfb.2013.10.027
Montenegro, H., RodriguesRodrigues, A. M., Dias, M. A. G., da Silva, E. A., Bernardi, F., and de Camargo, Z. P. (2014). Feline Sporotrichosis Due to Sporothrix Brasiliensis: an Emerging Animal Infection in São Paulo, Brazil. BMC Vet. Res. 10 (November), 269. doi:10.1186/s12917-014-0269-5
Mora-Montes, H. (2018). Special Issue “Sporothrix and Sporotrichosis”. Journal of Fungi 4 (4), 116. doi:10.3390/jof4040116
Moriyama, B., Henning, J., Falade-Nwulia, O., Jarosinski, P., PenzakPenzak, S. R., and Walsh, T. J. (2012). Adverse Interactions between Antifungal Azoles and Vincristine: Review and Analysis of Cases. Mycoses 55 (4), 290–297. doi:10.1111/j.1439-0507.2011.02158.x
Morris, D., Ansar, M., Speshock, J., Ivanciuc, T., Qu, Y., Casola, A., et al. (2019). Antiviral and Immunomodulatory Activity of Silver Nanoparticles in Experimental RSV Infection. Viruses 11 (8), 732. doi:10.3390/v11080732
Nadworny, P. L., Landry, B. K., Wang, J., Tredget, E. E., and Burrell, R. E. (2010). Does Nanocrystalline Silver Have a Transferable Effect? Wound Repair Regen. 18 (2), 254–265. doi:10.1111/j.1524-475X.2010.00579.x
Niemirowicz, K., Durnaś, B., Piktel, E., and Bucki, R. (2017). Development of Antifungal Therapies Using Nanomaterials. Nanomedicine 12 (15), 1891–1905. doi:10.2217/nnm-2017-0052
Orofino-Costa, R., Macedo, P. M. d., Rodrigues, A. M., and Bernardes-Engemann, A. R. (2017). Sporotrichosis: An Update on Epidemiology, Etiopathogenesis, Laboratory and Clinical Therapeutics. Bras. Dermatol. 92 (5), 606–620. doi:10.1590/abd1806-4841.2017279
Queiroz-Telles, F., Buccheri, R., and Benard, G. (2019). Sporotrichosis in Immunocompromised Hosts. JoF 5 (1), 8–23. doi:10.3390/jof5010008
Raafat, D., von Bargen, K., Haas, A., and Sahl, H.-G. (2008). Insights into the Mode of Action of Chitosan as an Antibacterial Compound. Appl. Environ. Microbiol. 74 (12), 3764–3773. doi:10.1128/AEM.00453-08
Radhakrishnan, V. S., Reddy Mudiam, M. K., Kumar, M., Dwivedi, S. P., Singh, S. P., and Prasad, T. (2018). Silver Nanoparticles Induced Alterations in Multiple Cellular Targets, Which Are Critical for Drug Susceptibilities and Pathogenicity in Fungal Pathogen (Candida Albicans). Ijn Vol. 13, 2647–2663. doi:10.2147/IJN.S150648
Ramírez-Soto, M., Aguilar-Ancori, E., Tirado-Sánchez, A., Bonifaz, A., and Bonifaz, A. (2018). Ecological Determinants of Sporotrichosis Etiological Agents. JoF 4 (3), 95–11. doi:10.3390/jof4030095
Reis, É. G., SchubachSchubach, T. M. P. S. A., Silva, J. N., Carvalho, B. W., Quintana, M. S. B., and Gremião, I. D. F. (2016). Association of Itraconazole and Potassium Iodide in the Treatment of Feline Sporotrichosis: A Prospective Study. Med. Myco. 54 (7), 684–690. doi:10.1093/mmy/myw027
Riaz Ahmed, K. B., Nagy, A. M., BrownNagyBrown, R. P. R. P., Zhang, Q., Malghan, S. G., and Goering, P. L. (2017). Silver Nanoparticles: Significance of Physicochemical Properties and Assay Interference on the Interpretation of In Vitro Cytotoxicity Studies. Toxicol. Vitro 38, 179–192. doi:10.1016/j.tiv.2016.10.012
Rodrigues, A. M., Della Terra, P. P., Gremião, I. D., Pereira, S. A., Orofino-Costa, R., and de Camargo, Z. P. (2020). The Threat of Emerging and Re-emerging Pathogenic Sporothrix Species. Mycopathologia 185, 813–842. doi:10.1007/s11046-020-00425-0
Sanchotene, K. O., Madrid, I. M., Klafke, G. B., Bergamashi, M., TerraRodrigues, P. P. D., Rodrigues, A. M., et al. (2015). Sporothrix Brasiliensisoutbreaks and the Rapid Emergence of Feline Sporotrichosis. Mycoses 58 (11), 652–658. doi:10.1111/myc.12414
Sandri, G., Miele, D., Faccendini, A., Bonferoni, M. C., Rossi, S., Grisoli, P., et al. (2019). Chitosan/Glycosaminoglycan Scaffolds: The Role of Silver Nanoparticles to Control Microbial Infections in Wound Healing. Polymers 11 (7), 1207–1216. doi:10.3390/polym11071207
Seibel, N. L., Shad, A. T., Bekersky, I., Groll, A. H., Gonzalez, C., Wood, L. V., et al. (2017). Safety, Tolerability, and Pharmacokinetics of Liposomal Amphotericin B in Immunocompromised Pediatric Patients. Antimicrob. Agents Chemother. 61 (2). doi:10.1128/AAC.01477-16
Sharifi-Rad, M., and Pohl, P. (2020). Synthesis of Biogenic Silver Nanoparticles (AgCl-NPs) Using a Pulicaria Vulgaris Gaertn. Aerial Part Extract and Their Application as Antibacterial, Antifungal and Antioxidant Agents. Nanomaterials 10 (4), 638. doi:10.3390/nano10040638
Sharma, S., Madhyastha, H., Laxmi Swetha, K., Maravajjala, K. S., Singh, A., Madhyastha, R., et al. (2021). Development of an In-Situ Forming, Self-Healing Scaffold for Dermal Wound Healing: In-Vitro and Iin-Vivo Studies. Mater. Sci. Eng. C 128 (September), 112263. doi:10.1016/j.msec.2021.112263
Shih, P.-Y., Liao, Y.-T., Tseng, Y.-K., Deng, F.-S., and Lin, C.-H. (2019). A Potential Antifungal Effect of Chitosan against Candida Albicans Is Mediated via the Inhibition of SAGA Complex Component Expression and the Subsequent Alteration of Cell Surface Integrity. Front. Microbiol. 10, 602. doi:10.3389/fmicb.2019.00602
Shiny, P. J., Mukherjee, A., and Chandrasekaran, N. (2014). Haemocompatibility Assessment of Synthesised Platinum Nanoparticles and its Implication in Biology. Bioproc. Biosyst Eng 37 (6), 991–997. doi:10.1007/s00449-013-1069-1
Tian, J., Wong, K. K. Y., Ho, C.-M., Lok, C.-N., Yu, W.-Y., Che, C.-M., et al. (2007). Topical Delivery of Silver Nanoparticles Promotes Wound Healing. ChemMedChem 2 (1), 129–136. doi:10.1002/cmdc.200600171
Valeriano, C. A. T., Ferraz, C. E., Oliveira, M. M. E., Inácio, C. P., de Oliveira, E. P., Lacerda, A. M., et al. (2020). Cat-Transmitted Disseminated Cutaneous Sporotrichosis Caused by Sporothrix Brasiliensis in a New Endemic Area: Case Series in the Northeast of Brazil. JAAD Case Rep. 6 (10), 988–992. doi:10.1016/j.jdcr.2020.07.047
Wei, L., Lu, J., Xu, H., Patel, A., Chen, Z.-S., and Chen, G. (2015). Silver Nanoparticles: Synthesis, Properties, and Therapeutic Applications. Drug Discov. Today 20 (5), 595–601. doi:10.1016/j.drudis.2014.11.014.Silver
Wolff, D., Feldt, T., Reifenberger, J., Sebald, H., and Bogdan, C. (2018). The Brief Case: Cutaneous Sporotrichosis in an Immunocompetent Patient after Travel to Peru. J. Clin. Microbiol. 56 (11), 1–6. doi:10.1128/JCM.01958-17
Xia, Z.-K., MaMa, Q.-H., Li, S.-Y., ZhangZhang, D.-Q., Cong, L., Tian, Y.-L., et al. (2016). The Antifungal Effect of Silver Nanoparticles on Trichosporon Asahii. J. Microbiol. Immunol. Infect. 49 (2), 182–188. doi:10.1016/j.jmii.2014.04.013
Ye, H., Cheng, J., and Yu, K. (2019). In Situ Reduction of Silver Nanoparticles by Gelatin to Obtain Porous Silver Nanoparticle/Chitosan Composites with Enhanced Antimicrobial and Wound-Healing Activity. Int. J. Biol. Macromolecules 121, 633–642. doi:10.1016/j.ijbiomac.2018.10.056
You, C., Li, Q., Wang, X., Wu, P., Ho, J. K., Jin, R., et al. (2017). Silver Nanoparticle Loaded Collagen/Chitosan Scaffolds Promote Wound Healing via Regulating Fibroblast Migration and Macrophage Activation. Sci. Rep. 7 (1), 1–11. doi:10.1038/s41598-017-10481-0
Yuan, Q., Hein, S., and Misra, R. D. K. (2010). New Generation of Chitosan-Encapsulated ZnO Quantum Dots Loaded with Drug: Synthesis, Characterization and In Vitro Drug Delivery Response. Acta Biomater. 6 (7), 2732–2739. doi:10.1016/j.actbio.2010.01.025
Zhang, X.-F., Liu, Z.-G., Shen, W., and Gurunathan, S. (2016). Silver Nanoparticles: Synthesis, Characterization, Properties, Applications, and Therapeutic Approaches. Ijms 17 (9), 1534. doi:10.3390/ijms17091534
Keywords: silver nanoparticles, chitosan, Sporothrix spp., murine model, subcutaneous sporotrichosis
Citation: Artunduaga Bonilla JJ, Honorato L, Guimarães AJ, Miranda K and Nimrichter L (2022) Silver Chitosan Nanocomposites are Effective to Combat Sporotrichosis. Front. Nanotechnol. 4:857681. doi: 10.3389/fnano.2022.857681
Received: 18 January 2022; Accepted: 07 March 2022;
Published: 08 April 2022.
Edited by:
Felipe Alves de Almeida, Juiz de Fora Federal University, BrazilReviewed by:
Roberto Vazquez Munoz, UConn Health, United StatesPatrícia Albuquerque, University of Brasilia, Brazil
Silvana Alborés, Universidad de la República, Uruguay
Copyright © 2022 Artunduaga Bonilla, Honorato, Guimarães, Miranda and Nimrichter. This is an open-access article distributed under the terms of the Creative Commons Attribution License (CC BY). The use, distribution or reproduction in other forums is permitted, provided the original author(s) and the copyright owner(s) are credited and that the original publication in this journal is cited, in accordance with accepted academic practice. No use, distribution or reproduction is permitted which does not comply with these terms.
*Correspondence: Leonardo Nimrichter, nimrichter@micro.ufrj.br