Improving the stability and transdermal permeability of phycocyanin loaded cubosomes
- 1School of Chinese Materia Medica, Guangdong Pharmaceutical University, Guangzhou, China
- 2Department of Nephrology, Guangdong Provincial People’s Hospital, Guangdong Academy of Medical Sciences, Guangzhou, China
- 3School of Pharmaceutical Sciences, Sun Yat-sen University, Guangzhou, China
- 4School of Pharmaceutical Sciences, Jinan University, Guangzhou, China
Instability and low transdermal permeability of protein antioxidants are major obstacles to resist oxidative stress in transdermal drug delivery system. To overcome these shortcomings, cubosomes were developed as an advanced transdermal delivery system to improve stability and transdermal absorption of the model antioxidant phycocyanin in this study. Glyceryl monooleate and poloxamer 407 (P407) were used to prepare cubosomes as carrier matrix and stabilizer, respectively. Phycocyanin loaded cubosomes (PC-cubosomes) were prepared by the emulsification and homogenization method. A 33 full factorial design was used to optimize the cubosome formulations. The final optimal PC-cubosomes possessed an average particle size of 183.2 ± 0.5 nm and a negative surface charge as well as achieved a high encapsulation efficiency of 87.2% ± 2.7%. PC-cubosomes appeared as nano-sized and well-shaped spheres with highly ordered cubical structures. The residual amount of phycocyanin in PC-cubosomes was 3-fold higher than that in the free drug solution after 10 days ultraviolet radiation exposure. In vitro release kinetics of phycocyanin from PC-cubosomes fitted to the Higuchi kinetic model, indicating that phycocyanin released from cubosomes mainly attributed to drug diffusion and dissolution. PC-cubosomes also exhibited higher permeability (39.79 μg⋅cm−2⋅hour−1) across the rat skin than phycocyanin solution (16.33 μg⋅cm−2⋅hour−1). Furthermore, PC-cubosomes were easily taken up by keratinocytes, thereby achieving a prolonged anti-oxidative stress effect. These results therefore suggested that cubosomes could be a promising transdermal delivery system to improve the stability and transdermal permeability of phycocyanin.
1 Introduction
Skin is the largest organ and external barrier in human body. However, it subjects to wounds either by pathology or physical trauma, especially when free radicals are involved. For example, long-term exposure to ultraviolet (UV) radiation could induce the imbalance between pro-oxidants and antioxidants, which may lead to oxidative stress, photo aging, or cancer (Feng et al., 2022; Dai et al., 2023).
In order to prevent these damages, topical antioxidant supplementation has been used as a strategy in pharmaceutical and cosmetic industries to quench free radicals with many phytochemicals or bioactive compounds showing antioxidant activity through the radical scavenging mechanism (Castangia et al., 2016a). However, most of the bioactive substances are unstable if exposed to light during storage, transport, and usage. Once the photo-degradation occurs, the antioxidant’s oxidation resistance would dramatically reduce and lose their protective function to the skin (Morsi et al., 2014). Another challenge is the low transdermal permeability due to the presence of stratum corneum (SC), which limits the transport of active ingredient to the epidermis (E) and dermis (D) (Hardiningtyas et al., 2018; Despotopoulou et al., 2022). Therefore, it is urgent to explore new carriers to deliver antioxidants via the transdermal route.
Phycocyanin is an abundant pigment present in Spirulina platensis that has attracted attention because of its nutritional value besides its wide and medicinal application (Sayed et al., 2022). This natural pigment could scavenge reactive oxygen species (ROS) and reactive nitrogen species (RNS), thereby protecting skin from inflammation and cancer as well as promoting wound healing (Castangia et al., 2016b; Ashaolu et al., 2021). However, phycocyanin is highly sensitive to light and can be easily inactivated during storage (Azari et al., 2023). The properties of phycocyanin, such as high-molecular weight and hydrophilicity, also hinder its penetration across the skin (Hardiningtyas et al., 2018). Therefore, it is necessary to develop an advanced drug delivery system to protect the bioactivity of phycocyanin and to enhance its transdermal permeability.
Nowadays, many approaches have been used to enhance transdermal penetration such as iontophoresis, electroporation, microneedles, cubosomes, in situ gel and cosolvents (Li et al., 2023; Ranade et al., 2023). Among these techniques, the use of cubosomes as delivery vehicles for hydrophobic, amphiphilic, and hydrophilic agents like peptides and proteins has attracted considerable attention due to their excellent biocompatibility and versatility (Boge et al., 2017; Mehanna et al., 2022). Cubosomes consist of a curved continuous lipid bilayer extending in three dimensions and separating two congruent networks of water channels (Carducci et al., 2019) (The cubosomes nanostructure is shown in Figure 1). Drug molecules with different properties, including hydrophilic, amphiphilic, and hydrophobic molecules, can be incorporated within its complex structure. In addition, cubosomes are also believed to enhance the biological adhesion of skin, increase the drug retention in the epidermis and dermis, and minimize the adverse reactions of the system (Strachan et al., 2021; Jiang et al., 2022). Glyceryl monooleate (GMO) is commonly used matrix to fabricate cubosomes due to its non-toxic, biodegradable, and biocompatible characteristics (Al-mahallawi et al., 2021). Once contacting with water, GMO can form either reversed micellar phase (L2) or liquid crystalline phases (lamellar, reversed hexagonal, and cubic phase), which is controlled by the preparation temperature and concentration (Elgindy et al., 2016; Gorantla et al., 2022; Atlibatur et al., 2023).
In this study, phycocyanin-encapsulated cubosomes (PC-cubosomes) were fabricated via emulsification and homogenization to improve the stability, permeability, and skin retention of phycocyanin. GMO and a triblock copolymer Poloxamer 407 (P407) were used as carrier matrix and stabilizer, respectively (Sherif et al., 2014). The impact of formulation variables and their interactions on cubosomes properties was investigated via a 33 full factorial design. The stability of phycocyanin in PC-cubosomes was evaluated and compared with free drugs. Furthermore, ex vivo skin permeation of the optimal PC-cubosomes was investigated using Sprague Dawley (SD) rat abdominal skin. The antioxidant efficacy of PC-cubosomes was evaluated via a cell viability study using HaCaT cells (a human keratinocyte cell line), which was treated with H2O2 beforehand.
2 Materials and methods
2.1 Materials
Phycocyanin was kindly donated by Danzi Company (China). GMO and P407 were purchased from Wako Pure Chemical Industries, Ltd., (Japan) and BASF, Ltd., (Germany), respectively. Immortalized human keratinocytes (HaCaT) were obtained from China Center for Type Culture Collection (China). Cell medium, fetal bovine serum, penicillin, and streptomycin were purchased from Gibco America, Ltd., (United States). All other chemicals were analytical grade and used as received.
2.2 Preparation of PC-cubosomes
PC-cubosomes were prepared by melt dispersion emulsification technique with some modifications (Makhlouf and Elnawawy, 2023). As showed in Figure 1, the dispersed phase consisting of GMO and P407 was heated at 50 °C in a water bath to form oil phase. Phycocyanin was dissolved in MilliQ water by a vortex mixer for 5 s to form phycocyanin solution at a concentration of 0.59 μg/mL. Afterwards, the oil phase was gently injected into the preheated aqueous phase (phycocyanin solution) through using a syringe at 50 C and emulsified using a rotor-stator homogenizer (Avestin Em-C3, Ottawa, Canada) at 10,000 rpm for 5 min. The final dispersion was cooled and maintained at room temperature for further investigation.
2.3 Experimental design via (33) full factorial
A three-factor, three-level (33) full factorial design was applied to identify the impact of mass fractions of GMO, P407, and phycocyanin as independent variables on the PC-cubosomes physicochemical properties (Table 1). Five dependent variables include particle size (Y1), polydispersity index (PDI, Y2), zeta potential (ZP) (Y3), encapsulation efficiency (EE%) (Y4), and cumulative drug release through an artificial membrane within 24 h (Y5) were evaluated. The experimental design matrix generated by Design Expert® (version 8.0.3, State-Ease Inc., Minneapolis, Minnesota) and the composition of PC-cubosomes formulations were showed in Table 1 and Table 2.
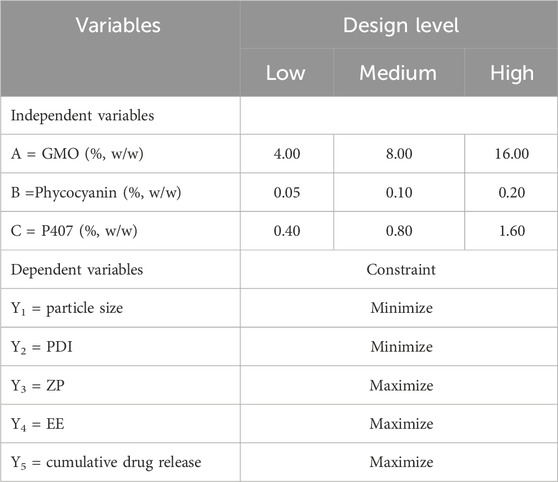
Table 1. Variables and their respective levels in the applied 33 full factorial experimental design.
2.4 Particle size, PDI and ZP of PC-cubosomes
Particle size, PDI and ZP of PC-cubosomes were determined by dynamic light scattering (DLS) (Zetasizer, Malvern Instruments, United Kingdom). Samples were diluted with deionized water, adjusted to a suitable light scattering intensity (300 Hz), and measured at 25°C in triplicate.
2.5 EE%
The non-encapsulated phycocyanin was separated from cubosomes by dialysis as follows (Castangia et al., 2016b). 5 mg PC-cubosomes were loaded into dialysis bag (300 kDa Float-A-Lyzer G2, Spectrum Laboratories Inc., DG Breda, Netherlands) and dialyzed for 2 h at 25 C, which allows dissolution and consequent removal of non-encapsulated phycocyanin. Samples were analyzed for phycocyanin concentration using a fluorescence spectrophotometer (LS55, PerkinElmer, United States) at 615 nm. EE% was calculated according to the following equation:
2.6 In vitro release of phycocyanin from PC-cubosomes
PC-cubosomes (n = 3) containing 2 mg of phycocyanin were sealed in a cellulosic dialysis membrane (Spectra-Por1 dialysis tubing, cut-off 30 kDa, Spectrum laboratories Inc., United States) and immersed in a beaker containing 200 mL of phosphate buffer saline (PBS, pH 7.4) at 32°C ± 0.5 C with gentle agitation at 100 rpm. A volume of 2 mL of release medium was withdrawn at pre-determined time points and replaced with an equal volume of fresh pre-warmed release medium to maintain sink conditions. The concentration of phycocyanin was analysed by fluorescence spectrophotometry and kinetic models (zero-order, first order, Higuchi, and Korsmeyer-Peppas models) on drug release from PC-cubosomes was also performed. All measurements were carried out in triplicate and the values were expressed as mean ± S.D.
2.7 Morphology of PC-cubosomes
The morphology of the optimal PC-cubosomes was examined using a transmission electron microscope (TEM, JEM−100CX, JEOL, Japan) at an accelerating voltage of 120 kV. Briefly, PC-cubosomes were diluted with distilled water and a drop of suspension was placed on a copper-coated grid to form a thin film. Subsequently, samples were stained by phosphotungstic acid solution (2% w/v, pH 6.8) for 1 min, air dried at room temperature, and observed by TEM.
2.8 Stability
The stability of phycocyanin encapsulated in the optimal PC-cubosomes and solution was determined according to the method of Zhong with some modifications (Yejun Zhong et al., 2024). PC-cubosomes and PC solution were exposure to UV radiation at 25°C for 10 days. At predetermined time points, 2 mL of each sample were withdrawn and analysed by UV-VIS spectrophotometry (TU−1901, General Instruments Co., Ltd, China) (Zhuang et al., 2023). Encapsulated phycocyanin was extracted from PC-cubosomes using ethanol and free phycocyanin in solution was used as a control.
2.9 Ex-vivo transdermal permeability
Ex-vivo transdermal permeability of phycocyanin solution and PC-cubosomes was evaluated using modified Franz diffusion cells with a contact area of 3.14 cm2 and a receptor volume of 8.5 mL (Chen et al., 2023). After removal of hairs and subcutaneous fat, the abdominal skin isolated from SD rats was placed in between the donor and receptor compartments with the stratum corneum (SC) side facing the donor compartment. The receptor compartment was filled with 8.5 mL of PBS (pH 7.4) to maintain sink conditions (El-Enin and Al-Shanbari, 2018). The diffusion cells were kept in a water bath at 32°C ± 0.5 C with shaking at 100 rpm in order to mimic the in vivo environment (Tu et al., 2014). After equilibration of 15 min, formulations containing 6.0 mg of phycocyanin were added to the donor compartment. The medium in the receptor compartment were withdrawn and replaced by equal volume of fresh pre-warmed PBS. Each sample was filtered through a 0.22 μm syringe filter before quantified by fluorescence spectrophotometry. Cumulative penetration amounts (Q) were plotted against time (t) by Equation (2).
2.10 Skin retention of phycocyanin
At the end of the ex-vivo transdermal permeation study, the skin was carefully removed from Franz diffusion cells and cleaned by gauze soaked with fresh PBS (El-Enin and Al-Shanbari, 2018). To separate the SC from the remaining epidermis (E) and dermis (D), the skin sections were stripped with 15 pieces of adhesive tape. All tapes, except the first one, were immersed in 5 mL of water and sonicated for 10 min after vortex stirring. The remaining E and D were cut into small pieces and immersed in 10 mL of water and sonicated for 10 min. All samples were centrifuged for 10 min at 15,000 rpm, the supernatant was collected and analysed by fluorescence spectrophotometry.
2.11 In vitro cell studies
2.11.1 Cell culture
HaCaT cells were cultured with Dulbecco’s Modified Eagle Medium (DMEM), which was supplemented with 100 IU/mL penicillin, 100 mg/mL streptomycin, and 10% FBS at 37 C in a humidity atmosphere of 5% CO2.
2.11.2 Cytotoxicity studies
HaCaT cells (7.5
2.12 Antioxidant effect of PC-cubosomes
The protective activity of PC-cubosomes and phycocyanin solution against hydrogen peroxide (H2O2) induced oxidative damage was evaluated on HaCaT cells (Rachitha et al., 2023). The cells were co-treated with H2O2 and PC-cubosomes or phycocyanin solution (final drug concentration of 100 μg/mL) for 3 or 6 h, followed by repeated washing with PBS. Then the absorbance of all groups were determined by a standard MTT assay (Murgia et al., 2015). All measurements were performed in sextuplicate. Untreated cells and the cells treated with H2O2 only (1:30,000 dilution) were used as negative and positive controls, respectively.
3 Results and discussion
3.1 Experimental design via (33) full factorial
Full factorial design (33) is a commonly used method to optimize experiments with multiple experimental designs involved to generate polynomial mathematical relationships and to map the response over the experimental domain. In this work, full factorial design was applied to optimize PC-cubosome formulations.
Twenty-seven formulations were prepared according to the optimization parameters in full factorial design (33) (Table 2). All the formulations were valued by the factors mentioned above (Y1, Y2, Y3, Y4, and Y5). F2, F11, and F20 were set to evaluate the influence of GMO. The influence of P407 mass fraction was investigated through F1, F2, and F3. Furthermore, F2, F5 and F8 were used to explore the impacts of phycocyanin mass fraction. Thirteen formulations were successfully prepared to form cubosomes, while viscous bulk cubic gels were observed in the remaining formulations after cooling down. Only the formulations with cubosome properties were further analysed. Responses of PC-cubosome parameters generated by (33) full factorial experimental design were showed in Table 3.
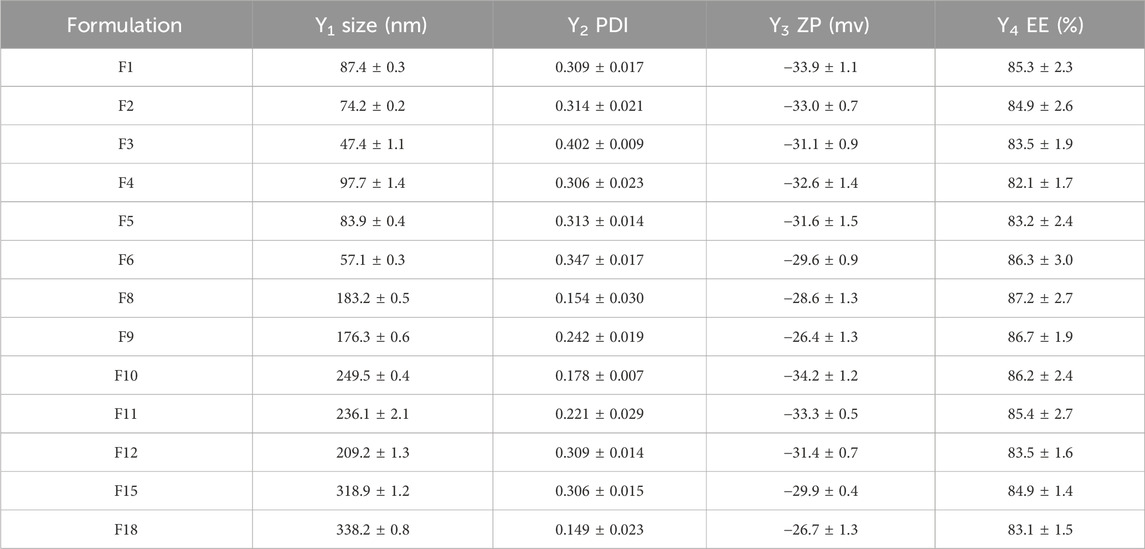
Table 3. Responses of the evaluated PC-cubosomes parameters fabricated via (33) full factorial experimental design (n = 3).
For formulation optimization, particle size and PDI of different formulations were firstly compared. Nanoformulations have many advantages for transdermal drug delivery system such as high skin penetration ability, greater skin deposition and improved physicochemical stability of the loaded drug (Ghasemiyeh and Mohammadi-Samani, 2020). The desirability constrains were minimizing particle size, polydispersity index and maximizing zeta potential as absolute value. According to the results, F1-F6, F12, and F15 were excluded due to their broad particle size distribution with PDI values of higher than 0.3. Formulation 18 was also excluded from analysis due to its large particle size of 338.2 ± 0.8 nm. In vitro release kinetics of F8-F11 was subsequently evaluated in order to choose the optimal formulation, as we discuss later. F8 was eventually identified as the optimal cubosome formulation with further characterize and study.
3.2 Particle size and PDI
Small particle size of nanoparticles generally facilitates transdermal permeation of drugs. The regression equations showed the influence of mass fractions of GMO (A), phycocyanin (B), and P407 (C) on particle size (Y1) and PDI (Y2) in terms of coded values according to equation 3 and 4, respectively. According to the results, the particle size and PDI values of PC-cubosomes were significantly affected by the mass fractions of GMO, P407, and phycocyanin (p < 0.05). The mass fraction of P407 (C) showed a noticeable negative effect on particle size (Y1), while both mass fractions of GMO (A) and phycocyanin (B) showed positive effects. In contrast, mass fractions of GMO (A) and phycocyanin (B) displayed negative effects on PDI (Y2), whereas P407 (C) exhibited a positive effect on it.
It was found that the particle size of cubosomes increased with increasing the amount of GMO, which could be attributed to its high viscosity. Also, higher mass fraction of phycocyanin could decrease the surface tension and lead to the formation of cubosomes with uniform particle sizes (Chen et al., 2024). The positive effect of amphiphilic polymer P407 on PDI was mainly due to its ability to overcome intrinsic adhesion of GMO and to promote the formation of well-dispersed cubosomes (Shamma and Aburahma, 2014).
3.3 ZP
ZP is an important parameter to evaluate the stability and bio-distribution of colloidal dispersions (Zhang and Wu, 2021). Generally, high surface charges lead to electrostatic repulsion between particles, thereby preventing them from aggregation (Makhlouf and Elnawawy, 2023). In this study, the prepared PC-cubosomes showed a high absolute ZP values ranged from −26.4 ± 1.3 mV (F9) to −33.9 ± 1.1 mV (F1) (Table 3), which could effectively avoid particle aggregation.
The ANOVA analysis indicated that the mass fractions of GMO (A), phycocyanin (B), and P407 (C) significantly affected the ZP parameter (p < 0.05) according to Equation 5.
The absolute value of ZP increased with increasing the mass fraction of GMO, which consistent with previous reports (Al-Shoubki et al., 2023). This may be due to the small amount of free oleic acid, which could absorb on the surface of cubosomes and exhibit a negative surface charge based on the presence of carboxyl groups at the terminal of oleic acid chains. Both mass fractions of phycocyanin and P407 showed negative impacts on the ZP value, which was probably due to their adsorption on the outer surface of PC-cubosomes (Sherif et al., 2014). Overall, the high ZP value could allow enhanced carrier penetration due to the electric potential differences across the skin.
3.4 EE%
The EE% values of preliminary screening 13 formulations were measured with data shown in Table 3. All the EE% values of PC-cubosomes ranged from 82.1% ± 1.7% (F4) to 87.2% ± 2.7% (F8), which might be attributed to the strong attraction between phycocyanin and the hydrophilic domain of cubic phase bilayer (Driever et al., 2013). P407 is a commonly used moisturizer in transdermal drug delivery systems. Since both hydrophilic (ethylene oxide blocks) and hydrophobic (propylene oxide blocks) segments exist, P407 could facilitate phycocyanin to be incorporated into cubosomes through intermolecular hydrogen binding or molecular interactions between the copolymers and drugs (Dumortier et al., 2006).
3.5 In Vitro release of phycocyanin from PC-cubosomes
In vitro drug release kinetics of the formulations with a particle size smaller than 300 nm and uniform particle size distribution (PDI <0.3) (i.e., F8, F9, F10 and F11) were investigated (Figure 2). It was observed that the viscosity of formulations increased with increasing the mass fraction of GMO (F10 and F11), which in turn restricted drug diffusion from the outer monoglyceride bilayer to the aqueous release medium (Atlibatur et al., 2023). Meanwhile, according to the literature, GMO as a basic cubosomes component might lead to decreasing the drug partitioning rate from the oily medium to the aqueous one when compared to the free drug which could diffuse easily to the dissolution media (Nguyen et al., 2017; El-Enin and Al-Shanbari, 2018). Compared with the phycocyanin solution, PC-cubosomes showed decreased drug release rates, particularly for the formulations of F10 and F11, which could be attributed to the neutral stabilizers of P407 (Driever et al., 2013). Moreover, the cubosomes prepared with a higher mass fraction of P407 (F9) exhibited delayed drug release compared to the formulation with a low mass fraction of P407 (F8). The possible explanation is the surface of F9 was covered more by P407, which restricted release of encapsulated phycocyanin (Moura et al., 2020). Therefore, F8 was considered the optimal formulation and its release kinetics were further analysed.
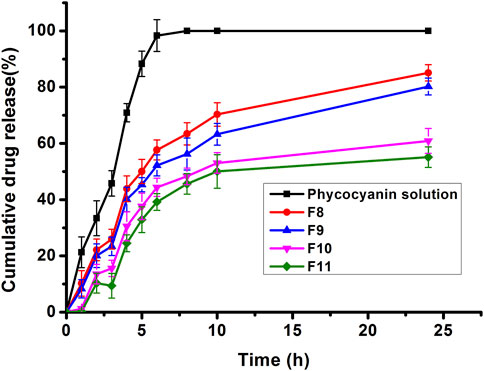
Figure 2. Release profiles of phycocyanin from PC-cubosomes (F8, F9, F10, F11) and phycocyanin solution in PBS (pH 7.4) at 32°C ± 0.5 C (n = 3)
The correlation coefficients (R2) were obtained after fitting the in vitro release data to various release kinetic models. Results indicate that best fit was obtained with Higuchi kinetic model (R2 = 0.9901), which was consistent with previously reported findings (Guo et al., 2010). The calculated value of 0.70059 (0.43 < n < 0.85) obtained by fitting the release data to Korsmeyer Peppas model indicated that the drug release mechanism was anomalous (non-Fickian) and coupled with the swelling of glyceryl monooleate (Hu et al., 2023).
3.6 Morphology of PC-cubosomes
The morphology of F8 was investigated by TEM (Figure 3) with showing a spherical shape. The particle size was around 200 nm (Figure 3A), which was consistent with the result obtained by dynamic light scattering. Figure 3B shows the cubical structure of PC-cubosomes with light lines and black dots representing water channels and lipid bilayers, respectively. These results therefore indicated that the PC-cubosomes were successfully formed in a highly ordered cubic internal structure (Kwon et al., 2012).
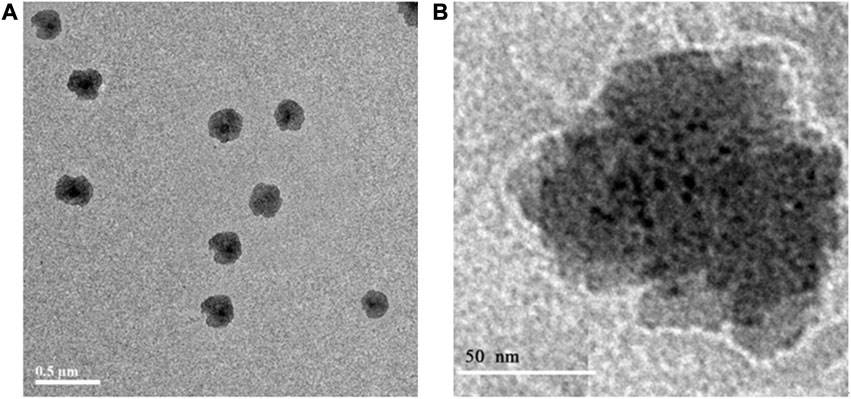
Figure 3. Transmission electron microscopy images of PC-cubosomes (F8), (A) is the overall image and (B) is the partial enlarged image.
3.7 Stability
The stability of PC-cubosomes and phycocyanin in solution was evaluated by exposure the formulations to UV radiation for 10 days (25 C). As shown in Figure 4, over 70% of free phycocyanin was degraded, whereas only 3% of the encapsulated phycocyanin in cubosomes was degraded on the day 10, indicating cubosomes protected the encapsulated phycocyanin from degradation. P407 in cubosomes also could contribute to the protective effect by increasing the hydrophilicity of proteins, demonstrating that cubosomes could efficiently protect phycocyanin from rapid degradation due to their high encapsulation capacity of phycocyanin (Guo et al., 2010; Chong et al., 2011).
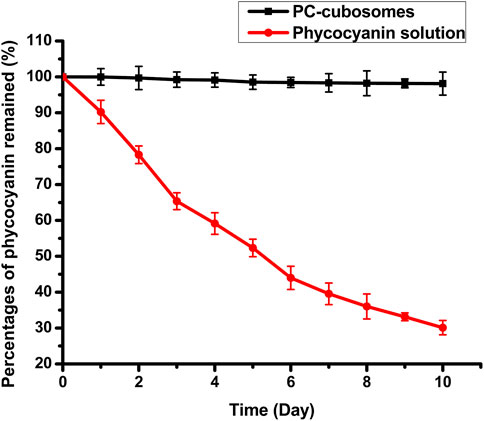
Figure 4. Drug residue of phycocyanin solution and PC-cubosomes with UV radiation exposure for 10 days at 25 C (n = 3)
3.8 Ex-vivo transdermal permeation
Figure 5A showed the cumulative drug penetration per unit area across the excised SD rat abdominal skin. The drug permeation rates of F8 and free drug at steady state (Js) were obtained from the slope of the linear portion. PC-cubosomes exhibited a higher permeation rate (39.79 μg⋅cm−2⋅hour−1) than the phycocyanin solution (16.33 μg⋅cm−2⋅hour−1), suggesting that cubosomes improved the permeability of phycocyanin across the skin tissue. This was possibly due to the presence of penetration enhancer GMO, which modifies the intercellular structure of lipid layer and changes the fluidity of cell membrane. Moreover, the repulsive force between cubosomes and lipids in the skin also could contribute to the improved drug penetration (Jia et al., 2014).
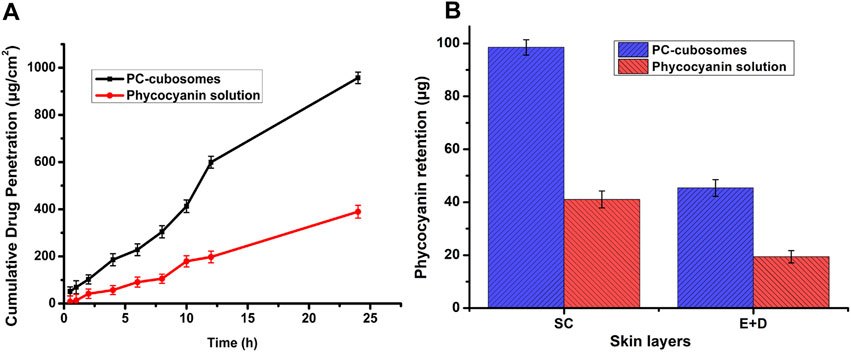
Figure 5. (A) Cumulative amount of phycocyanin penetrated through rat skin from PC-cubosomes or phycocyanin solution within 24 h; (B) Ex-vivo skin retention of phycocyanin for PC-cubosomes and phycocyanin solution (n = 3)
3.9 Skin retention
As shown in Figure 5B, the amounts of phycocyanin released from PC-cubosomes retained in SC and (E + D) were 98.47 ± 3.34 μg and 45.38 ± 2.37 μg, respectively. For the free drug group, only 41.03 ± 3.21 μg (SC) and 19.38 ± 2.34 μg (E + D) of phycocyanin were retained in the skin tissue after release studies. This may be attributed to the fact that cubosomes with molecular weight copolymers could restrict drug transport from the skin to the whole body, delay drug clearance by the systemic circulation, and prolong drug retention in the target tissue (Chong et al., 2011). Cubosomes therefore can be considered as a sustained release depot for transdermal drug delivery (Zakaria et al., 2022).
3.10 Cell studies
The cytotoxicity of PC-cubosomes (F8) and phycocyanin in solution was evaluated using HaCaT cells. The cells were treated with PC-cubosomes and drug solutions with different phycocyanin concentrations ranged from 20 to 400 μg/mL for 48 h. In the phycocyanin solution group, the rates of cell viability were 85.13% ± 2.94% and 69.87% ± 2.73% at the lowest (20 μg/mL) and highest concentrations (400 μg/mL) (Figure 6A). The cell viability reduced with increasing the drug concentration, because high concentration of phycocyanin may impair the mitochondrial respiratory chain, reduce energy supply, and induce cell death (Chong et al., 2011). In contrast, the relative viability of cells treated with PC-cubosomes at all the concentrations was higher than 95.98% ± 4.54%, as phycocyanin was slowly released from cubosomes, which allows the cells to compensate for the change of phycocyanin concentration in a certain degree with their metabolism. Cell viability results indicated that PC-cubosomes were a safe carrier for transdermal drug delivery.
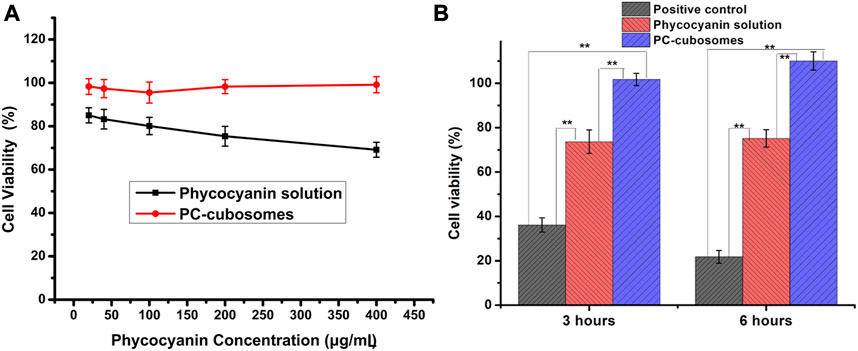
Figure 6. (A) Viability of HaCaT cells incubated for 48 h with different concentrations of PC-cubosomes and phycocyanin solution; (B) Cell viability of HaCaT cells after H2O2-induced oxidative stress tests (n = 6)
3.11 Protective effect against cell oxidative damage
As Figure 6B showed, the cells treated with H2O2 only were used as a positive control with cell viability decreasing to 36.13% ± 3.18% and 21.79% ± 2.87% after 3 h and 6 h of incubation, respectively. In contrast, higher cell viability was achieved in the phycocyanin solution group with 73.69% ± 5.26% and 75.14% ± 3.92% observed after the same treatments. These results indicated that the oxidative stress damage induced by H2O2 was rescued by the treatment with phycocyanin due to its antioxidant potentials. Interesting, the PC-cubosomes group showed a further improvement on the cell viability with 101.73% ± 2.74% and 110.04% ± 4.01% (p < 0.05 versus all others) survived after 3 h and 6 h of treatment, respectively. This was possibly due to the lipid structures of GMO and P407 that facilitated the exchange between proteins and intracellular lipids, which are crucial for maintaining cell function (Zeng et al., 2023). Therefore, this study demonstrated that phycocyanin could counteract the ROS oxidative activity towards keratinocyte damages and loading phycocyanin into cubosomes further improved its aptitude due to the enhanced cell permeability (Chen et al., 2024).
4 Conclusion
Phycocyanin loaded cubosomes (PC-cubosomes) were successfully prepared using GMO and P407 by the emulsification method. The formulations were optimized via (33) full factorial method with the final optimal PC-cubosomes possessing a particle size of 200 nm with a narrow size distribution, and a high drug encapsulation efficiency (≥80%). The optimal PC-cubosomes showed highly ordered cubic internal structure, good capacity to re-suspend and excellent stability under the exposure to UV. The in vitro release and ex-vivo transdermal permeation studies showed PC-cubosomes could prolong drug release and improve drug permeation across the skin tissue when compared with the free drug. Furthermore, PC-cubosomes protected HaCaT cells from oxidative damage induced by H2O2 and exhibited good biocompatibility with no significant cytotoxicity. Overall, present study provides a novel insight into phycocyanin skin penetration via the cubosomes for transdermal delivery. It may also be applied to the percutaneous penetration study of other protein drugs. Despite great potentials of PC-cubosomes shown as a transdermal drug delivery system, in vivo studies should be performed to further investigate their safety and efficacy.
Data availability statement
The original contributions presented in the study are included in the article/Supplementary materials, further inquiries can be directed to the corresponding authors.
Ethics statement
The animal study was approved by Laboratory Animal Center of Sun Yat-sen University. The study was conducted in accordance with the local legislation and institutional requirements.
Author contributions
CZ: Writing–original draft, Writing–review and editing, Supervision. WD: Writing–review and editing. HJ: Writing–original draft. JL: Data curation, Writing–review and editing. YH: Supervision, Writing–review and editing. DH: Investigation, Writing–review and editing. CW: Writing–review and editing.
Funding
The author(s) declare financial support was received for the research, authorship, and/or publication of this article. This work was financially supported by the National Natural Science Foundation of China (Grant No. 82003665) and Guangdong Graduate Education Innovation Program (Grant No. 2023JGXM_087)
Conflict of interest
The authors declare that the research was conducted in the absence of any commercial or financial relationships that could be construed as a potential conflict of interest.
Publisher’s note
All claims expressed in this article are solely those of the authors and do not necessarily represent those of their affiliated organizations, or those of the publisher, the editors and the reviewers. Any product that may be evaluated in this article, or claim that may be made by its manufacturer, is not guaranteed or endorsed by the publisher.
References
Al-mahallawi, A. M., Abdelbary, A. A., and El-Zahaby, S. A. (2021). Norfloxacin loaded nano-cubosomes for enhanced management of otitis externa: in vitro and in vivo evaluation. Int. J. Pharm. 600, 120490. doi:10.1016/j.ijpharm.2021.120490
Al-Shoubki, A. A., Teaima, M. H. M., Abdelmonem, R., ElNabarawi, M. A., and Elhabal, S. F. (2023). Potential application of sucrose acetate isobutyrate, and glyceryl monooleate for nanonization and bioavailability enhancement of rivaroxaban tablets. Pharm. Sci. Adv. 2, 100015. doi:10.1016/j.pscia.2023.100015
Ashaolu, T. J., Samborska, K., Lee, C. C., Tomas, M., Capanoglu, E., Tarhan, Ö., et al. (2021). Phycocyanin, a super functional ingredient from algae; properties, purification characterization, and applications. Int. J. Biol. Macromol. 193, 2320–2331. doi:10.1016/j.ijbiomac.2021.11.064
Atlibatur, R., Bahadori, F., Kizilcay, G. E., Ide, S., and Gürsel, Y. (2023). Preparation and characterization of glyceryl dibehenate and glyceryl monostearate-based lyotropic liquid crystal nanoparticles as carriers for hydrophobic drugs. J. Drug Deliv. Sci. Technol. 87, 104821. doi:10.1016/j.jddst.2023.104821
Azari, A., Ghaboos, S. H. H., Moghadam, V. E., and Jafari, S. M. (2023). Influence of chitosan coating on the physicochemical and antioxidant properties of phycocyanin-loaded nanoliposomes. Algal Research-Biomass Biofuels Bioprod. 72, 103120. doi:10.1016/j.algal.2023.103120
Boge, L., Umerska, A., Matougui, N., Bysell, H., Ringstad, L., Davoudi, M., et al. (2017). Cubosomes post-loaded with antimicrobial peptides: characterization, bactericidal effect and proteolytic stability. Int. J. Pharm. 526 (1), 400–412. doi:10.1016/j.ijpharm.2017.04.082
Carducci, F., Casadei, B. R., Mariani, P., and Barbosa, L. R. S. (2019). X-ray characterization of pharmaceutical and cosmetic lipidic nanoparticles for cutaneous application. Curr. Pharm. Des. 25 (21), 2364–2374. doi:10.2174/1381612825666190709210211
Castangia, I., Manca, M. L., Caddeo, C., Bacchetta, G., Pons, R., Demurtas, D., et al. (2016a). Santosomes as natural and efficient carriers for the improvement of phycocyanin reepithelising ability in vitro and in vivo. Eur. J. Pharm. Biopharm. 103, 149–158. doi:10.1016/j.ejpb.2016.03.033
Castangia, I., Manca, M. L., Catalán-Latorre, A., Maccioni, A. M., Fadda, A. M., and Manconi, M. (2016b). Phycocyanin-encapsulating hyalurosomes as carrier for skin delivery and protection from oxidative stress damage. J. Mater. Sci. Mater. Med. 27 (4), 75. doi:10.1007/s10856-016-5687-4
Chen, H. L., Guo, X., Yu, S., Meng, H., Ai, C., Song, S., et al. (2024). Phycocyanin/tannic acid complex nanoparticles as Pickering stabilizer with synergistic interfacial antioxidant properties. Food Chem. 434, 137353. doi:10.1016/j.foodchem.2023.137353
Chen, Z. Z., Huang, Q., Song, Y., Feng, X., Zeng, L., Liu, Z., et al. (2023). Cubosomes-assisted transdermal delivery of doxorubicin and indocyanine green for chemo-photothermal combination therapy of melanoma. Biomed. Pharmacother. 166, 115316. doi:10.1016/j.biopha.2023.115316
Chong, J. Y. T., Mulet, X., Waddington, L. J., Boyd, B. J., and Drummond, C. J. (2011). Steric stabilisation of self-assembled cubic lyotropic liquid crystalline nanoparticles: high throughput evaluation of triblock polyethylene oxide-polypropylene oxide-polyethylene oxide copolymers. Soft Matter 7 (10), 4768. doi:10.1039/c1sm05181d
Dai, X., Hu, Y., Jiang, L., Lei, L., Fu, C., Wu, S., et al. (2023). Decreased oxidative stress response and oxidant detoxification of skin during aging. Mech. Ageing Dev. 216, 111878. doi:10.1016/j.mad.2023.111878
Despotopoulou, D., Lagopati, N., Pispas, S., Gazouli, M., Demetzos, C., and Pippa, N. (2022). The technology of transdermal delivery nanosystems: from design and development to preclinical studies. Int. J. Pharm. 611, 121290. doi:10.1016/j.ijpharm.2021.121290
Driever, C. D., Mulet, X., Waddington, L. J., Postma, A., Thissen, H., Caruso, F., et al. (2013). Layer-by-Layer polymer coating on discrete particles of cubic lyotropic liquid crystalline dispersions (cubosomes). Langmuir 29 (42), 12891–12900. doi:10.1021/la401660h
Dumortier, G., Grossiord, J. L., Agnely, F., and Chaumeil, J. C. (2006). A review of poloxamer 407 pharmaceutical and pharmacological characteristics. Pharm. Res. 23 (12), 2709–2728. doi:10.1007/s11095-006-9104-4
El-Enin, H. A., and Al-Shanbari, A. H. (2018). Nanostructured liquid crystalline formulation as a remarkable new drug delivery system of anti-epileptic drugs for treating children patients. Saudi Pharm. J. 26 (6), 790–800. doi:10.1016/j.jsps.2018.04.004
Elgindy, N. A., Mehanna, M. M., and Mohyeldin, S. M. (2016). Self-assembled nano-architecture liquid crystalline particles as a promising carrier for progesterone transdermal delivery. Int. J. Pharm. 501 (1), 167–179. doi:10.1016/j.ijpharm.2016.01.049
Feng, Z. A., Qin, Y., Huo, F., Jian, Z., Li, X., Geng, J., et al. (2022). NMN recruits GSH to enhance GPX4-mediated ferroptosis defense in UV irradiation induced skin injury. Biochimica Biophysica Acta-Molecular Basis Dis. 1868 (1), 166287. doi:10.1016/j.bbadis.2021.166287
Ghasemiyeh, P., and Mohammadi-Samani, S. (2020). Potential of nanoparticles as permeation enhancers and targeted delivery options for skin: advantages and disadvantages. Drug Des. Dev. Ther. 14, 3271–3289. doi:10.2147/dddt.s264648
Gorantla, S., Saha, R. N., and Singhvi, G. (2022). Exploring the affluent potential of glyceryl mono oleate - myristol liquid crystal nanoparticles mediated localized topical delivery of Tofacitinib: study of systematic QbD, skin deposition and dermal pharmacokinetics assessment. J. Mol. Liq. 346, 117053. doi:10.1016/j.molliq.2021.117053
Guo, C., Wang, J., Cao, F., Lee, R. J., and Zhai, G. (2010). Lyotropic liquid crystal systems in drug delivery. Drug Discov. Today 15 (23), 1032–1040. doi:10.1016/j.drudis.2010.09.006
Hardiningtyas, S. D., Wakabayashi, R., Kitaoka, M., Tahara, Y., Minamihata, K., Goto, M., et al. (2018). Mechanistic investigation of transcutaneous protein delivery using solid-in-oil nanodispersion: a case study with phycocyanin. Eur. J. Pharm. Biopharm. 127, 44–50. doi:10.1016/j.ejpb.2018.01.020
Hu, Z., Wang, G., Zhao, S., Jiang, S., Hu, J., and Reheman, A. (2023). Sustained release properties of liquid crystal functionalized poly (amino acid)s nanoparticles. J. Mol. Liq. 369, 120891. doi:10.1016/j.molliq.2022.120891
Jia, N., Li, T., Diao, X., and Kong, B. (2014). Protective effects of black currant (Ribes nigrum L.) extract on hydrogen peroxide-induced damage in lung fibroblast MRC-5 cells in relation to the antioxidant activity. J. Funct. Foods 11, 142–151. doi:10.1016/j.jff.2014.09.011
Jiang, T., Xie, Y., Dong, J., Yang, X., Qu, S., Wang, X., et al. (2022). The dexamethasone acetate cubosomes as a potential transdermal delivery system for treating skin inflammation. J. Drug Deliv. Sci. Technol. 75, 103567. doi:10.1016/j.jddst.2022.103567
Kwon, T. K., Hong, S. K., and Kim, J.-C. (2012). In vitro skin permeation of cubosomes containing triclosan. J. Industrial Eng. Chem. 18 (1), 563–567. doi:10.1016/j.jiec.2011.11.031
Li, B., Xiao, T., Guo, S., Wu, Y., Lai, R., Liu, Z., et al. (2023). Oxymatrine-fatty acid deep eutectic solvents as novel penetration enhancers for transdermal drug delivery: formation mechanism and enhancing effect. Int. J. Pharm. 637, 122880. doi:10.1016/j.ijpharm.2023.122880
Makhlouf, A., and Elnawawy, T. (2023). Hair regrowth boosting via minoxidil cubosomes: formulation development, in vivo hair regrowth evaluation, histopathological examination and confocal laser microscopy imaging. Int. J. Pharm. 634, 122665. doi:10.1016/j.ijpharm.2023.122665
Mehanna, M. M., Abla, K. K., Domiati, S., and Elmaradny, H. (2022). Superiority of microemulsion-based hydrogel for non-steroidal anti-inflammatory drug transdermal delivery: a comparative safety and anti-nociceptive efficacy study. Int. J. Pharm. 622, 121830–121915. doi:10.1016/j.ijpharm.2022.121830
Morsi, N. M., Abdelbary, G. A., and Ahmed, M. A. (2014). Silver sulfadiazine based cubosome hydrogels for topical treatment of burns: development and in vitro/in vivo characterization. Eur. J. Pharm. Biopharm. 86 (2), 178–189. doi:10.1016/j.ejpb.2013.04.018
Moura, S., Noro, J., Cerqueira, P., Silva, C., Cavaco-Paulo, A., and Loureiro, A. (2020). Poloxamer 407 based-nanoparticles for controlled release of methotrexate. Int. J. Pharm. 575, 118924. doi:10.1016/j.ijpharm.2019.118924
Murgia, S., Falchi, A. M., Meli, V., Schillén, K., Lippolis, V., Monduzzi, M., et al. (2015). Cubosome formulations stabilized by a dansyl-conjugated block copolymer for possible nanomedicine applications. Colloids Surfaces B-Biointerfaces 129, 87–94. doi:10.1016/j.colsurfb.2015.03.025
Nguyen, H. T. P., Soucé, M., Perse, X., Vial, F., Perrier, T., Yvergnaux, F., et al. (2017). Lipid-based submicron capsules as a strategy to include high concentrations of a hydrophobic lightening agent in a hydrogel. Int. J. Cosmet. Sci. 39 (4), 450–456. doi:10.1111/ics.12397
Rachitha, P., Krupashree, K., Kandikattu, H. K., Nagaraj, G., Alahmadi, T. A., Alharbi, S. A., et al. (2023). Nanofabrication of cobalt-tellurium using Allium sativum extract and its protective efficacy against H2O2-induced oxidative damage in HaCaT cells. Environ. Res. 226, 115659. doi:10.1016/j.envres.2023.115659
Ranade, V., Dalal, Y., Palampalle, H. Y., and Singh, K. (2023). Formulation, development, and comparative study of Azelastine-loaded temperature sensitive in situ gelling micelles for allergic conjunctivitis. J. Pharm. Innovation 18, 1966–1980. doi:10.1007/s12247-023-09760-3
Sayed, A. A., Soliman, A. M., Taha, M. A., and Sadek, S. A. (2022). Spirulina and C-phycocyanin mitigate titanium dioxide nanoparticle-induced hematobiochemical and hepatorenal disorders through antioxidative pathway. Food Chem. Adv. 1, 100035–100115. doi:10.1016/j.focha.2022.100035
Shamma, R. N., and Aburahma, M. H. (2014). Follicular delivery of spironolactone via nanostructured lipid carriers for management of alopecia. Int. J. Nanomedicine 9, 5449–5460. doi:10.2147/ijn.s73010
Sherif, S., Bendas, E. R., and Badawy, S. (2014). The clinical efficacy of cosmeceutical application of liquid crystalline nanostructured dispersions of alpha lipoic acid as anti-wrinkle. Eur. J. Pharm. Biopharm. 86 (2), 251–259. doi:10.1016/j.ejpb.2013.09.008
Strachan, J. B., Dyett, B. P., Jones, N. C., Hoffmann, S. V., Valery, C., and Conn, C. E. (2021). Reduction of enzymatic degradation of insulin via encapsulation in a lipidic bicontinuous cubic phase. J. Colloid Interface Sci. 592, 135–144. doi:10.1016/j.jcis.2021.02.027
Tu, Y. S., Fu, J. W., Sun, D. M., Zhang, J. J., Yao, N., Huang, D. E., et al. (2014). Preparation, characterisation and evaluation of curcumin with piperine-loaded cubosome nanoparticles. J. Microencapsul. 31 (6), 551–559. doi:10.3109/02652048.2014.885607
Yejun Zhong, Y., Sun, S., Dai, T., Zhang, H., Wu, J., and Gong, E. S. (2024). Phycocyanin-chitosan complex stabilized emulsion: preparation, characteristics, digestibility, and stability. Int. J. Biol. Macromol. 260, 129253. doi:10.1016/j.ijbiomac.2024.129253
Zakaria, F., Ashari, S. E., Azmi, I. D. M., and Abdul Rahman, M. B. (2022). Recent advances in encapsulation of drug delivery (active substance) in cubosomes for skin diseases. J. Drug Deliv. Sci. Technol. 68, 103097. doi:10.1016/j.jddst.2022.103097
Zeng, L. J., Ke, Y., Zheng, C., Song, H., Liu, Z., Hu, X., et al. (2023). Remote loading of hydrophilic drug into cubosomes by transmembrane pH-gradient and characterization of drug- loaded cubosomes prepared by different method. J. Pharm. Sci. 112 (4), 1119–1129. doi:10.1016/j.xphs.2022.12.024
Zhang, X. W., and Wu, W. (2021). Liquid crystalline phases for enhancement of oral bioavailability. Aaps Pharmscitech 22 (3), 81. doi:10.1208/s12249-021-01951-w
Keywords: cubosomes, phycocyanin, transdermal delivery system, antioxidants, stability
Citation: Zhu C, Duan W, Jing H, Long J, Huang Y, Huang D and Wu C (2024) Improving the stability and transdermal permeability of phycocyanin loaded cubosomes. Front. Nanotechnol. 6:1359219. doi: 10.3389/fnano.2024.1359219
Received: 21 December 2023; Accepted: 29 March 2024;
Published: 11 April 2024.
Edited by:
Sudip K Das, Butler University, United StatesReviewed by:
Van-An Duong, University of Texas Health Science Center at Houston, United StatesPatricia Targon Campana, University of São Paulo, Brazil
Copyright © 2024 Zhu, Duan, Jing, Long, Huang, Huang and Wu. This is an open-access article distributed under the terms of the Creative Commons Attribution License (CC BY). The use, distribution or reproduction in other forums is permitted, provided the original author(s) and the copyright owner(s) are credited and that the original publication in this journal is cited, in accordance with accepted academic practice. No use, distribution or reproduction is permitted which does not comply with these terms.
*Correspondence: Chune Zhu, zhuchune1108@163.com; Chuanbin Wu, wuchuanb@mail.sysu.edu.cn
†Present address: Di Huang, Massachusetts Eye and Ear Infirmary, Harvard Medical School, Boston, MA, United States
‡These authors contributed equally to this work and share first authorship