Sex-dependent effects of microglial reduction on impaired fear extinction induced by single prolonged stress
- 1Department of Basic Sciences, Ponce Research Institute, Ponce Health Sciences University, Ponce, Puerto Rico
- 2Department of Biomedical Sciences, Pontifical Catholic University of Puerto Rico, Ponce, Puerto Rico
Single prolonged stress (SPS) is a preclinical rodent model for studying post-traumatic stress disorder (PTSD)-like behaviors. Previously we found that increased expression of the microglial marker Iba-1 in the ventral hippocampus after SPS exposure was associated with impaired fear extinction, suggesting that microglial activity contributed to the SPS-induced behavioral changes. To test this, we examined whether reducing microglia with the colony-stimulating factor 1 receptor blocker, PLX3397, in the diet would prevent the SPS-induced extinction impairment. Male rats exposed to SPS showed enhanced fear acquisition and impaired fear extinction memory. Adding PLX3397 to the diet prevented these behavioral changes. In contrast, PLX3397 did not prevent SPS from impairing fear extinction memory in the female rats. Despite the sex-dependent behavioral effects, we found a reduced number and area fraction of Iba-1+ microglia in both male and female rats suggesting that PLX3397 had similar effects on microglia in both sexes. Altogether, these results suggest that microglia contribute to the behavioral changes induced by SPS in male but not female rats.
Introduction
Increasing clinical evidence links inflammation with posttraumatic stress disorder (PTSD) (Gill et al., 2013; Groer et al., 2015; Michopoulos et al., 2017; Wang et al., 2019; Kim et al., 2020; Katrinli et al., 2022). As the major producer of pro-inflammatory cytokines in the CNS (Kim and Joh, 2006), microglia could play a role in PTSD. Although evidence of microglial activation in PTSD is lacking, several rodent models of stress such as exposure to repeated predator stress (Wilson et al., 2013), inescapable electrical foot-shocks (Li et al., 2021), repeated social defeat (Wohleb et al., 2014), and single prolonged stress (SPS) (Lee et al., 2016; Lai et al., 2018; Cotrone et al., 2021) induce microglial activation and production of pro-inflammatory cytokines in the brain. In addition, cytokines that are produced by microglia such as interferon alpha (IFNα; Bi et al., 2016), tumor necrosis factor-alpha (TNFα; Connor et al., 1998), and interleukin 1 beta (IL-1β; Connor et al., 1998; Swiergiel and Dunn, 2007) generate abnormal fear responses or anxiety-like behaviors.
SPS is a pre-clinical model of traumatic stress that induces molecular and behavioral changes similar to those observed in PTSD (Liberzon et al., 1999) including impaired fear extinction (Knox et al., 2012; Kataoka et al., 2019), impaired cognitive flexibility (George et al., 2015, 2018; Chaby et al., 2019), and enhanced fear learning (Liu et al., 2016). In addition to the behavioral changes, exposure to SPS increases Iba-1 expressing microglia in the basolateral amygdala (BLA; Lai et al., 2018) and hippocampus (Sun et al., 2016) of male rats. Furthermore, minocycline prevented the increase in hippocampal microglia and the anxiety-like behavior and allodynia induced by SPS (Sun et al., 2016). SPS also increases microglial expression of proinflammatory P2X7 receptors and blocking P2X7 receptors prevented impaired fear extinction after SPS exposure (Torres-Rodriguez et al., 2022). Overall, the literature suggests that microglial activation contributes to the behavioral changes induced by SPS.
In this study, we further examined the role of microglia underlying PTSD-related behaviors induced by SPS exposure in male and female rats by inhibiting colony-stimulating factor 1 receptors (CSF1R). Since activation of the CSF1R signaling in microglia is required for the maintenance and development of microglia (Erblich et al., 2011; Chitu et al., 2016), the oral administration of the CSF1R inhibitor Pexidartinib (PLX3397) reduces drastically the number of microglia (Chitu et al., 2016; Merry et al., 2020). Depleting microglia with oral PLX3397 suggests that microglia support synaptic connections, neuronal plasticity, and behavioral changes (Linker et al., 2020; Liu et al., 2021; Ferrara et al., 2022). Therefore, we examined whether reducing microglia could prevent SPS-induced PTSD-related behavior. We found that oral administration of PLX3397 reduced Iba-1-expressing microglia in both male and female rats. However, PLX3397 prevented SPS from impairing fear extinction only in males suggesting a sex-dependent activation of microglia by the traumatic stress of SPS.
Materials and Methods
Animal subjects
All animal procedures were approved by the Institutional Animal Care and Use Committee of the Ponce Health Sciences University (PHSU) in compliance with NIH guidelines for the care and use of laboratory animals. A total of 32 adult male and 59 female Sprague-Dawley rats around postnatal day 60 were transported from the PHSU colony to a satellite facility nearby where they were individually housed on a 12/12 h light/dark schedule with free access to food and water. All behavioral experiments were carried out at the same time of the day (afternoon) to avoid the influence of the circadian rhythm. At the end of the behavioral assessments, a Wright’s stained vaginal cytological smear was taken to allow determination of the estrous cycle phase in the female rats.
SPS
Male and female Sprague-Dawley rats approximately post-natal day 60 (P60) were pseudorandomly assigned to the SPS protocol as designed by (Liberzon and Young, 1997; Knox et al., 2012) or a non-stressed (NO-SPS) group. The SPS protocol started with 2 h of restraint stress using a disposable rodent restrainer (DecapiCone®; Cat. No. DC-200), followed by immediate exposure to a 20-min forced swim in a cylindrical container (20 cm × 45 cm) containing tap water at 24°C. After the forced swim, we placed the rats in a recovery cage under direct light (soft white 60 watts light bulb) as a heat source for a 10-min recovery period to allow the animals to recover from the physical stress. In the last step of our SPS protocol, we placed the rats in an anesthetic chamber (16 cm × 16 cm) and exposed them to ethyl ether (Millipore Corporation, Cat. No. EX0185-8) vapor until general anesthesia induction. Each animal received the battery of stressors contained in the SPS protocol individually. Following the SPS protocol, animals were single-housed and left undisturbed for 7 days before behavioral testing. The housing conditions for SPS and NO-SPS groups were identical.
Diet administration of PLX3397
We pseudorandomly divided the rats into three groups (NO-SPS, SPS-ONLY, and SPS-PLX3397). All animals received the control diet (AIN-76A, Research Diets Inc., Cat. No. D15112401) for 2 days. Then, the animals belonging to the SPS-PLX3397 group received a diet switch to 290 mg/kg PLX3397 (Research Diets Inc., Cat. No. D15112401) for 3 days prior to the SPS exposure. The rats belonging to the NO-SPS, and the SPS-ONLY groups continued the control diet AIN-76A throughout the entire experiment.
Auditory fear conditioning (AFC) and extinction (EXT)
All rats were exposed to an Auditory Fear Conditioning (AFC) and extinction (EXT) paradigm designed to test their ability to acquire and extinguish a fear memory associated with an auditory cue. During day 1, animals were exposed to a total of six tones (1 kHz, 80 dB), one unpaired tone, and five tones paired with a 0.44 mA electrical footshock with a 3-min intertrial interval (ITI) in context A. Context A consisted of a clear acrylic box with electrified grid floor (ID#46002; Ugo Basile). During day 2, animals were subjected to fear EXT which consisted of 14 tones (1 kHz, 80 dB), with a 3-min ITI in context B. On day 3, animals were presented with two tones (1 kHz, 80 dB), in context B to test their fear EXT memory. In context B, the visual, tactile, and olfactory cues were changed to avoid contextual association.
Open field test
On day 4, we exposed the animals to a 10-min open field test (OFT) in a 94 cm × 94 cm × 44 cm arena to assess their anxiety-like behavior. No auditory cues were presented during the OFT session.
Molecular assessments
Immunofluorescence staining
We collected brain tissue 24 h after behavioral testing. Brain samples were fixed, dehydrated, and embedded in paraffin. We mounted paraffin-embedded ventral hippocampal coronal slices (4 μm) onto positively charged slides. Tissue was deparaffinized in xylene and rehydrated in a descending CDA19 ethanol series. Antigen retrieval consisted of incubation with 0.01 M Citrate-EDTA solution (pH = 6.2) for 40 min followed by a 20-min incubation at room temperature. The slides were incubated overnight in a humidified chamber at 4°C with the primary antibody, Iba-1 (1:1,000; Wako Chemicals; Cat. No. 019-19741). The primary antibody was labeled with Alexa Fluor 488 Goat Anti-Rabbit (Cat. No. A-21206). A control reaction was performed without primary antibodies for each test. Tissues were covered with ProLongTM Gold antifade reagent (Thermo Fischer Scientific; Cat. No. P36934) and a cover slide. Nuclei were stained with NucBlue Fixed Cell Stain (DAPI, Cat. No. 12333553). Images were taken using a Nikon Confocal Microscope A1 (Ver.4.10). Images were acquired by investigators blinded to treatment groups. Cell counting and fluorescence analyses of all images were performed using the cell-counter plug-in of the ImageJ software (NIH, USA) by investigators blinded to treatment groups. Only cells with clear DAPI-stained nuclei were counted as cells.
MILLIPLEX rat cytokine/chemokine magnetic panel (Cat. No. RECYTMAG-65K)
Upon sacrifice, we pseudorandomly selected either the right or the left hemisphere of the brain and stored it at −80°C until protein extraction processing. The whole hippocampus was dissected and cut into small sections using a petri dish and a scalpel. The tissue was homogenized in a low surfactant molecular grade PBS-based lysis buffer pH 7.5 containing 20 mmol/L Tris-HCl (Cat. No. 1185-53), 150 mmol/L NaCl (Cat. No. S7653), 0.05% Tween-20 (Cat. No. P9416). Protease inhibitor was diluted at 1:100 and phenylmethylsulfonyl fluoride (PMSF) was diluted at 1:1,000 in the lysis buffer. The sample was sonicated at ~3 watts for 15 s and immediately placed on ice. Then, the sample was passed through a Hamilton syringe (700 series 22 gauge) until minimal clumping. Lysates were centrifuged at 10,000 rpm for 5 min at 4°C and the supernatant was collected for protein quantification using the PIERCE BCA Protein Assay Kit (Cat. No. 23225, 23227). All hippocampal protein samples were diluted up to 1,000 μg/ml per well in the assay. Using this customized magnetic panel, we quantified the protein expression of IL-1β, TNFα, IL-6, IL-10, and IFNγ. All procedures were followed as stated by the manufacturer.
Data analysis
All behavioral and molecular data were analyzed with Graphpad Prism (version 9.1.0, San Diego, California). Samples sizes were based on previous studies that observed differences in similar behavior or molecular analysis (Cruz et al., 2015; Criado-Marrero et al., 2017; Castillo-Ocampo et al., 2021). Animals were not excluded from the data analysis. Auditory fear was measured as the percent of time spent freezing during each 30-s tone of training and recall with ANY-maze software (Stoelting Co., Wood Dale, IL). All freezing behavioral data are presented as the mean of two trials ± SEM. Unpaired t-tests were utilized for group comparisons (Graphpad Prism version 9.1.0, San Diego, California). Two-way analysis of variance (ANOVA) for repeated measures was employed for comparisons between treatment groups over time. Sidak’s Multiple Comparisons were used for post hoc comparisons when appropriate. Significance was set at p ≤ 0.05. Details of all statistical analyses are included in a statistical table. During the 10-min OFT session, we quantified the time spent in the center, the number of entries to the center, and the total distance traveled with ANY-maze software. Two-tailed unpaired t-tests were performed for group comparisons of the acquired data (Graphpad Prism version 9.1.0, San Diego, California).
Results
PLX3397 prevents the SPS-induced enhanced fear acquisition in male, but not in female rats
First, we examined the effects of microglial reduction on auditory fear learning in male and female rats (Figure 1A). We found that the male groups did not acquire the same auditory fear on day 1 (Figure 1B; F(2,87) = 8.120, p = 0.0006). The SPS-only male rats froze more than the NO-SPS male rats (p = 0.0195) indicating that exposure to SPS enhanced auditory fear learning. The SPS-PLX3397 male rats froze less than the SPS-only male group (p = 0.0009) suggesting that treatment with PLX3397 prevented the SPS enhanced fear learning in male rats. In addition, the NO-SPS and SPS-PLX3397 groups acquired similar auditory fear (p = 0.9999) suggesting that PLX3397 prevented the effects of the SPS without impairing normal fear learning. In contrast to the male groups, no differences in freezing were found among the female groups across the AFC session (Figure 1D; F(2,96) = 1.105, p = 0.3355), suggesting that SPS did not enhance fear acquisition in the female rats.
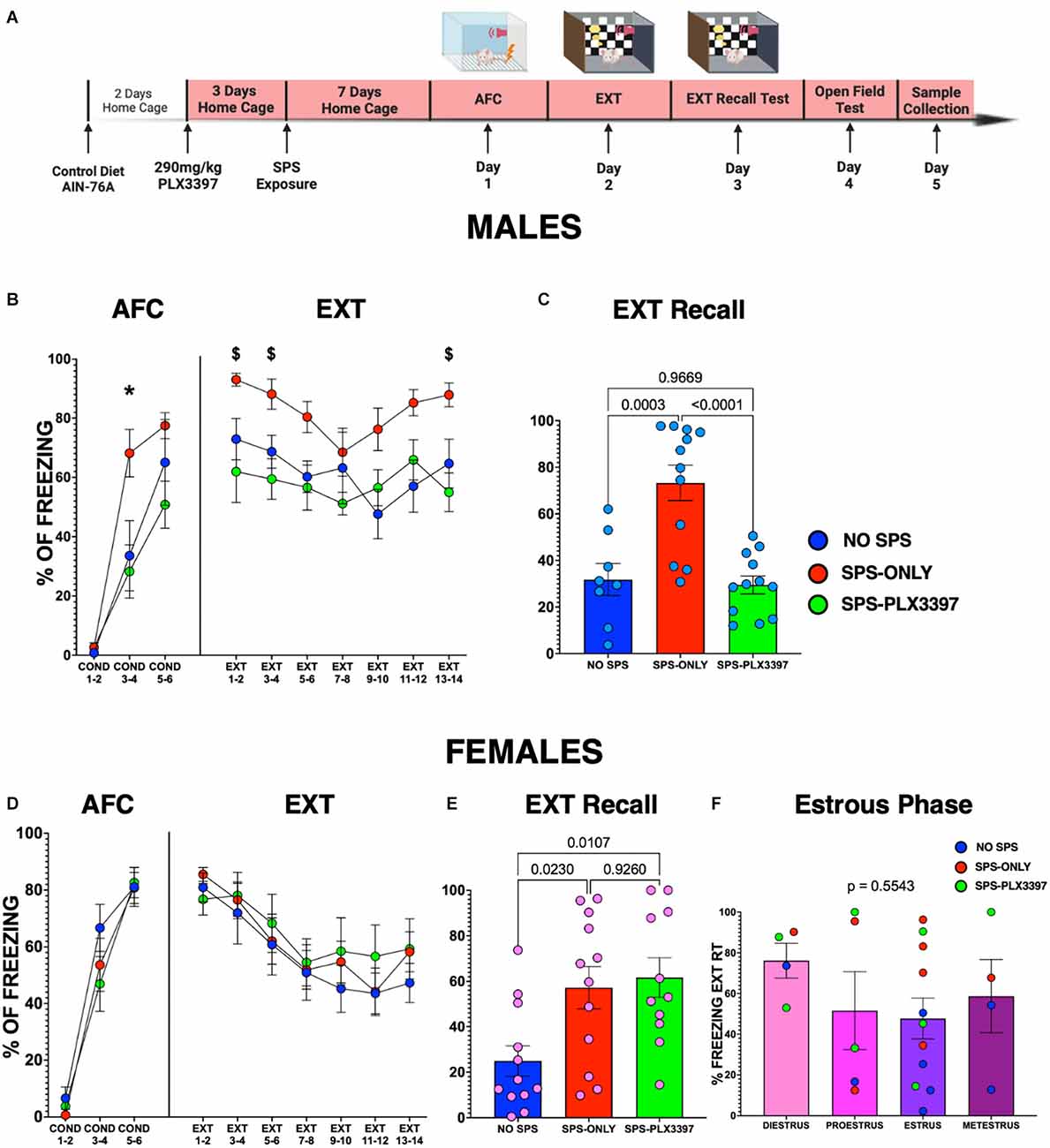
Figure 1. PLX3397 prevents SPS-induced impaired fear extinction in male, but not in female rats. (A) Experimental timeline. (B) Percent freezing of male rats during auditory fear conditioning (AFC), and extinction (EXT). *Denotes SPS-only is different from the other groups, p < 0.05. $Denotes SPS-only is different from SPS-PLX3397 group, p < 0.05. (C) Percent freezing of male rats during the extinction recall test (n = 8 NO SPS, n = 12 SPS-ONLY, n = 12 SPS-PLX3397 males). (D) Percent freezing of female rats during auditory fear conditioning, and extinction training. (E) Percent freezing of female rats during the extinction recall test. (F) Percent freezing during the extinction recall test of female rats grouped by their estrous cycle phase at sacrifice (n = 12 NO SPS, n = 12 SPS-ONLY, n = 11 SPS-PLX3397 female rats; One-way ANOVA, Tukey’s multiple comparisons test).
PLX3397 prevents SPS from enhancing fear memory and impairing extinction learning only in male rats
On day 2, we exposed the animals to 14 tones to test their recall of the fear memory and their ability to extinguish their conditioned fear response and learn that the auditory cue is not aversive. Our results show that the males belonging to the SPS-ONLY group froze more at the beginning (EXT 1–2, EXT 3–4), and the end (EXT 13–14) of the EXT session than the NO-SPS and SPS-PLX3397 male rats (Figure 1B; Day 2). This further suggests that SPS enhances fear learning and impairs extinction learning in male rats, and it could be prevented by PLX3397 oral administration. On the other hand, we found no differences in the freezing response among the female groups across the EXT session (Figure 1D; Day 2), suggesting that neither SPS exposure nor PLX3397 changes fear acquisition or extinction learning in female rats.
PLX3397 prevents SPS from impairing fear extinction memory only in male rats
On day 3, we presented two tones to all animals to test their fear extinction recall. The male groups showed different fear extinction recall (F(2,29) = 16.52, p < 0.0001). We found that SPS-ONLY male rats froze more than NO-SPS (p = 0.0003) and SPS-PLX3397 (p < 0.0001) male rats during extinction recall (Figure 1C). This suggests that SPS impairs fear extinction recall in male rats and that oral PLX3397 prevented the impairment. In addition, the NO-SPS and SPS-PLX3397 groups showed similar extinction recall (p = 0.9669) suggesting that PLX3397 prevented the effects of the SPS without enhancing normal fear extinction. The female groups also showed different extinction recall (F(2,32) = 5.899, p = 0.0066). Female rats in the SPS-only group displayed more freezing than the NO-SPS group (p = 0.0230) indicating that SPS also impaired extinction recall in the female rats (Figure 1E). However, the SPS-PLX3397 group froze as much as the SPS-only group (p = 0.9260) during extinction recall and the SPS-PLX3397 group froze more (p = 0.0107) than the NO-SPS female rats. Therefore, in contrast to the male rats, oral PLX3397 did not prevent SPS from impairing fear extinction recall in the female rats.
Since the estrous cycle can affect fear extinction in female rats (Milad et al., 2009), we compared the estrous phase of the individual rats of each group at sacrifice to their freezing during extinction recall (Figure 1F). All four estrous cycle phases had some animals from each group. There was no difference in freezing during extinction recall among the groups (F(3,20) = 0.7155, p = 0.5543) suggesting that the behavioral differences were not due to differences in the estrous cycle phase of the rats. However, it is important to note that since animals were sacrificed 48 h after the extinction recall test, the distribution of estrous cycle phases may have been different on other days of the behavioral protocol.
Open field test (OFT)
The male groups spent similar amounts of time in the center of the OFT (F(2,29) = 2.830, p = 0.0754, Figures 2A,B) and traveled similar distances during the entire OFT session (F(2,29) = 0.6343, p = 0.5375, Figure 2C) suggesting that SPS and PLX3397 did not alter anxiety-like behavior. Similarly, no difference was found within the female groups in the OFT when comparing the time spent in the center (F(2,32) = 2.432, p = 0.1039, Figures 2D,E). Consistent with this, no differences in the total distance traveled were found within the female groups in the OFT (F(2,32) = 2.242, p = 0.1227, Figure 2F) suggesting that neither SPS nor microglial depletion exerts changes in the anxiety-like behavior of female rats.
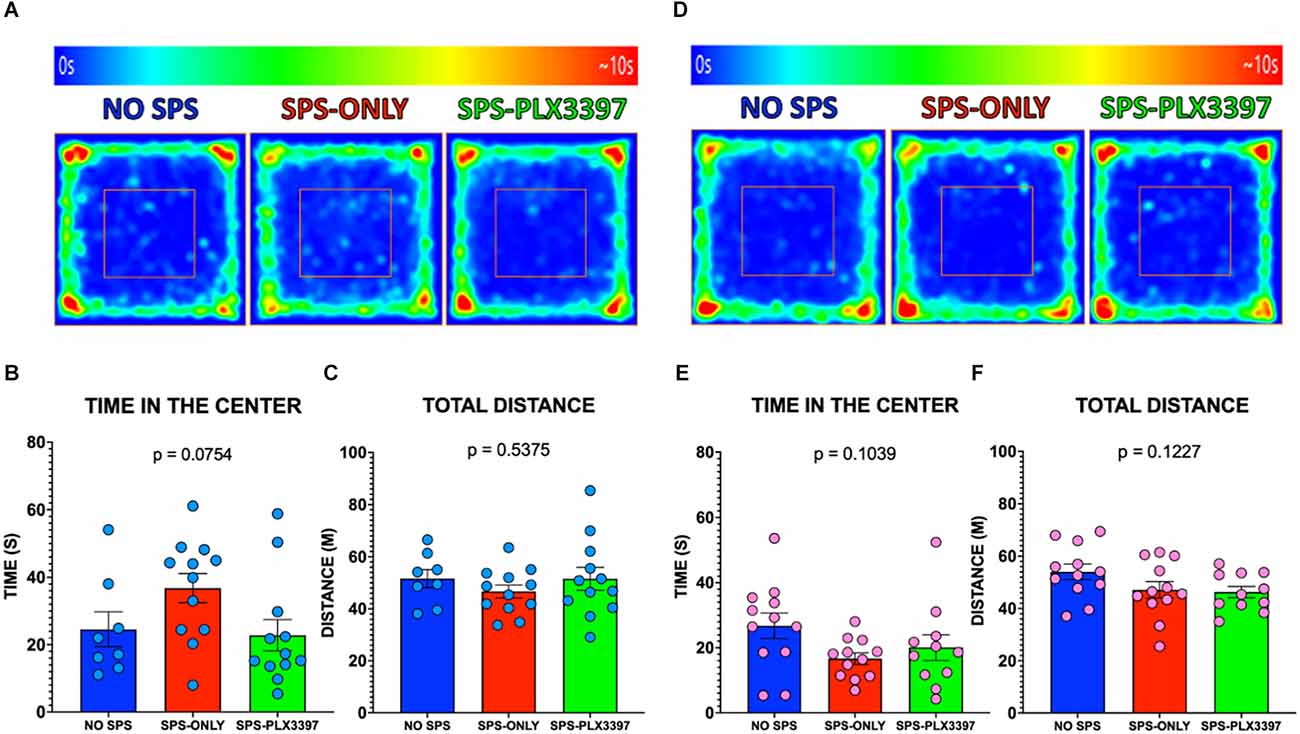
Figure 2. Effects of oral PLX3397 on anxiety-like behaviors in male and female rats. (A) Average heat maps of the center of the body of the male group during the open field testing. (B,C) Graphs of the time in the center and total distance traveled for the male rats. (D) Average heat maps of the center of the body of the female group during the open field testing. (E,F) Graphs of the time spent in the center and total distance traveled in the OFT for female rats. One-way ANOVA, Tukey’s multiple comparisons test.
Oral administration of PLX3397 reduced Iba-1 positive cells in the ventral hippocampus of male and female rats
The inability of PLX3397 to prevent the behavioral effects of SPS could be due to the inability of 290 mg/kg PLX3397 to reduce microglia in the female rats. To test this possibility, we immunostained ventral hippocampal slices for Iba-1 to examine the effects of oral administration of PLX3397 on microglia (Figures 3A–F). We chose to examine the ventral hippocampus because SPS increases microglial expression of Iba-1 in the ventral hippocampus (Torres-Rodriguez et al., 2022) and inhibition of the ventral hippocampus impairs extinction recall (Sierra-Mercado et al., 2011; Park et al., 2020). The male groups showed differences in Iba-1 expression in both cell numbers (F(2,20) = 11.54, p = 0.0005) and area fraction (F(2,20) = 7.082, p = 0.0047). Ventral hippocampal slices from the SPS-PLX3397 male rats showed fewer Iba-1 positive cells (p = 0.0003) and reduced area fraction (p = 0.0043) compared to the SPS-ONLY group suggesting that PLX3397 reduced the microglia. In addition, the SPS-PLX3397 group showed a reduction in area fraction (p = 0.0475) and a trend towards fewer Iba-1 positive cells (p = 0.0799) compared to the NO-SPS male rats (Figures 3G,H). This suggests the oral administration of PLX3397 reduced microglia. In addition, more Iba-1 positive cells were found in the SPS-ONLY male group than in the NO-SPS group (p = 0.0444), suggesting that SPS induces changes in microglial activity in male rats.
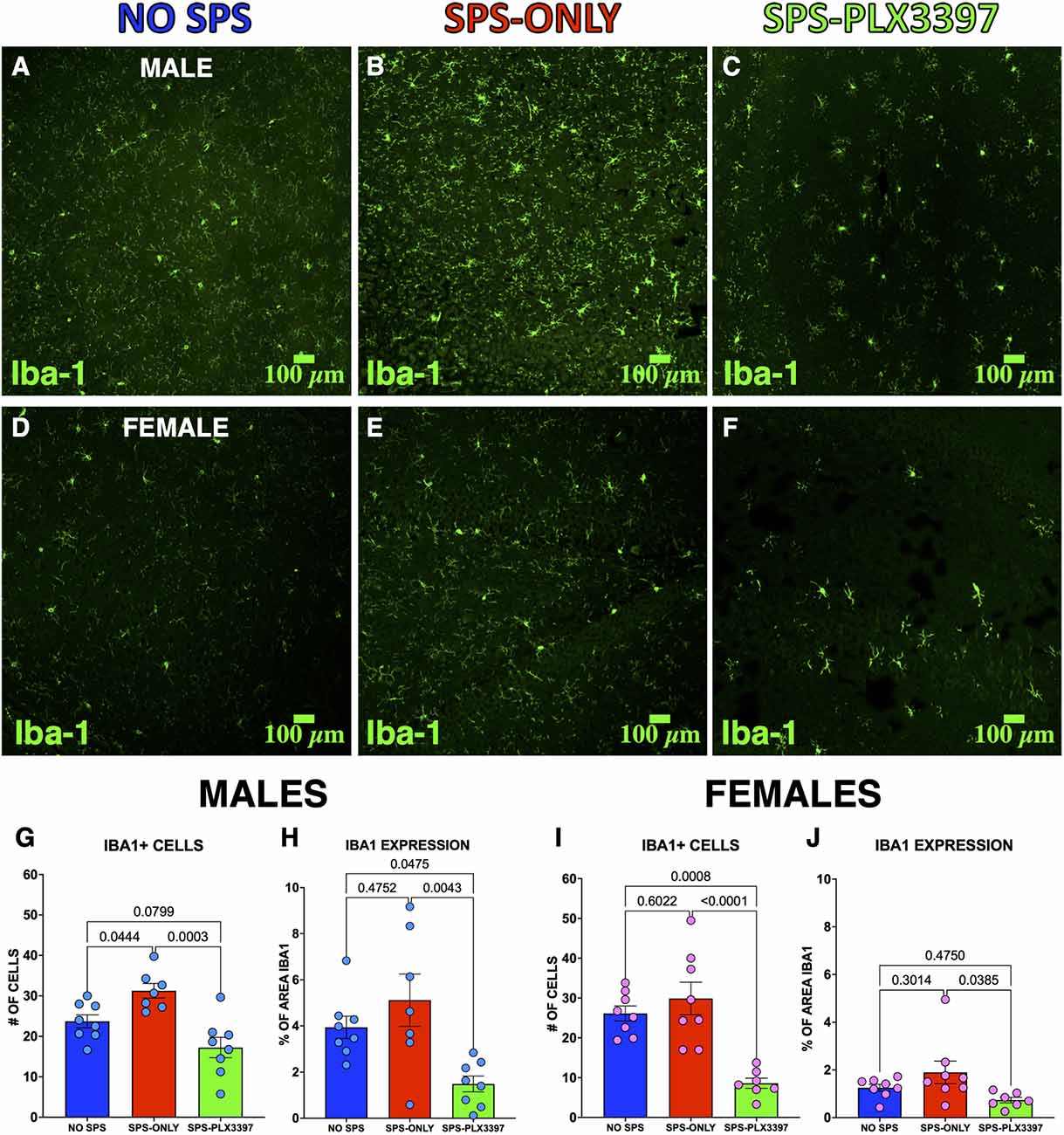
Figure 3. PLX3397 reduced the number of Iba-1+ cells in the ventral hippocampus in both male and female rats. (A–C) Representative images of Iba1-labeled ventral hippocampal slices of (A) NO SPS, (B) SPS-ONLY, and (C) SPS-PLX3397 male rats. (D–F) Representative images of Iba1-labeled ventral hippocampal slices of (D) NO SPS, (E) SPS-ONLY, and (F) SPS-PLX3397 female rats. (G,H) Iba-1+ cells and Iba1 area fraction in the ventral hippocampus of male rats. (I,J) Iba-1+ cells and Iba1 area fraction in the ventral hippocampus of female rats. One-way ANOVA, Tukey’s multiple comparisons test.
The female groups also showed differences in Iba-1 expression in both cell numbers (F(2,20) = 15.73, p < 0.0001) and area fraction (F(2,20) = 3.569, p = 0.0472). In contrast to the males, the female SPS-only group did not show more Iba-1 positive cells (p = 0.6022) than the female NO-SPS group suggesting that SPS did not alter microglial activity in the female rats. However, PLX3397 had similar effects in the female groups since the SPS-PLX3397 female rats displayed fewer Iba-1 positive cells (p < 0.0001) and area fraction (p = 0.0385) than the SPS-only female rats (Figures 3I,J). In addition, we found fewer Iba-1 positive cells in the SPS-PLX3397 group (p = 0.0008) compared to the NO-SPS female rats. These results validate that the oral administration of 290 mg/kg of PLX33937 produced similar reductions in microglia in male and female rats and suggest that the development of stress-induced extinction impairment involves different mechanisms in male and female rats.
Microglial depletion alters hippocampal pro-inflammatory cytokine expression in female rats
All animals were sacrificed the day after the OFT and hippocampal tissue was collected. To determine whether microglial depletion altered hippocampal cytokine expression, we quantified hippocampal TNFα, IL-1β, IL-6, IL-10, and INFγ pro-inflammatory cytokines using a customized MILLIPLEX Rat Cytokine/Chemokine Magnetic Panel (Cat. No. RECYTMAG-65K). Our results showed no changes in hippocampal cytokine expression in the SPS-only male groups compared to both NO-SPS or SPS-PLX3397 male groups (Figure 4) suggesting that neither SPS nor PLX3397 altered these cytokines at this time point. In contrast, we found increased hippocampal IL-1β in PLX3397-treated females when compared to the NO-SPS (p = 0.0080) or the SPS-only (p = 0.0064) female rats (Figure 4F). In addition, increased hippocampal IL-10 was found in PLX3397-treated females when compared to NO-SPS (p = 0.0005) or the SPS-only (p = 0.0009) female rats (Figure 4J).
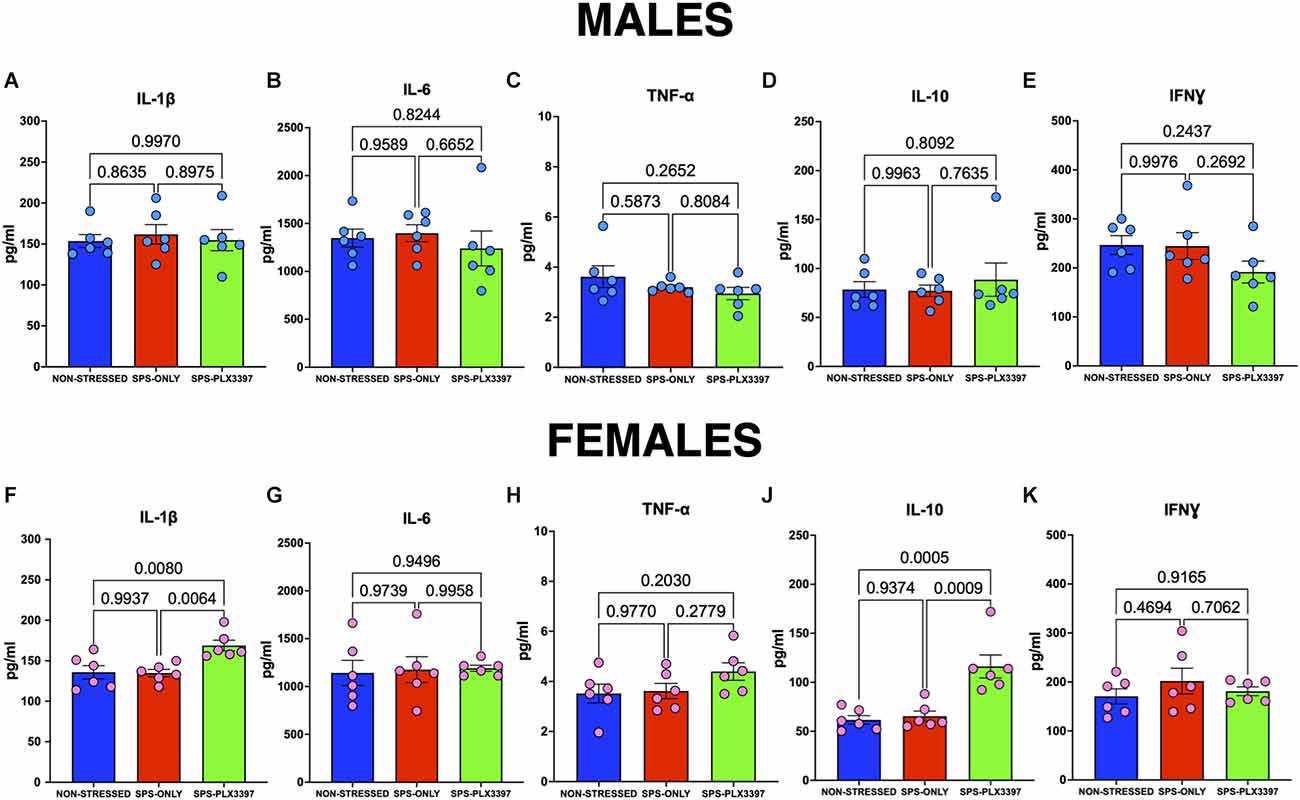
Figure 4. PLX3397 treatment increases hippocampal IL-1β and IL-10 in females, but not in male rats. (A–E) Hippocampal cytokine expression of male rats. (F–K) Hippocampal cytokine expression of female rats. One-way ANOVA, Tukey’s multiple comparisons test.
Oral administration of PLX3397 alone does not disrupt fear expression but produces anxiogenic behavior in female rats
Since the oral PLX3397 did not prevent SPS from impairing fear extinction recall in female rats, it is possible that PLX3397 alone impairs fear extinction memory in female rats. Therefore, we examined the effects of PLX3397 on fear conditioning and extinction in non-stressed female rats (Figure 5A). Our results showed that both PLX3397-treated and control diet-treated female rats displayed similar fear during AFC on Day 1 (Figure 5B) and during extinction on Day 2 (Figure 5B) indicating that PLX3397 did not cause significant changes in either fear acquisition or extinction. In addition, female rats fed the PLX3397 diet exhibited similar freezing during extinction recall as those fed the AIN-76A control diet (p = 0.9410) indicating that PLX3397 did not impair fear extinction recall in female rats (Figure 5C). On the other hand, PLX3397-treated female rats spent less time (Figures 5D,E) and entered fewer times into the center of the OFT (Figure 5F) than the female rats fed the AIN-76A control diet. PLX3397 treatment did not change the total distance traveled during the OFT session suggesting that the less time spent in the center of the OFT was not due to reduced movement (Figure 5G). This suggests that oral PLX3397 may produce anxiogenic behaviors in female rats.
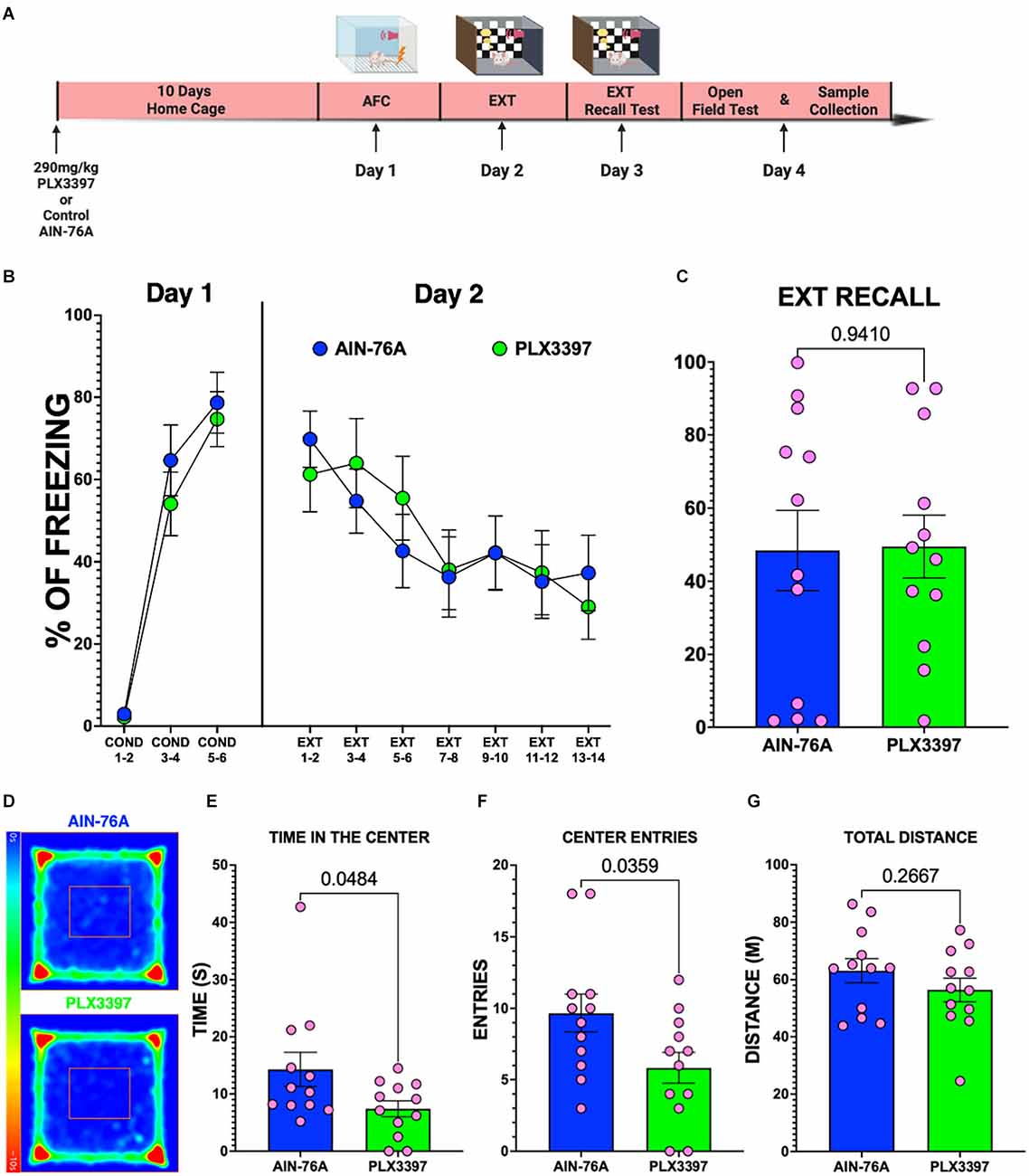
Figure 5. Oral administration of PLX3397 alone does not disrupt fear, but reduces the time spent in the center of the OFT in female rats. (A) Experimental timeline. (B) Percent freezing of female rats across the behavioral paradigm. (C) Percent freezing during EXT-Recall on Day 3 of female rats (unpaired t-test, two-tailed; n = 12 female rats per group). (D) Average heat maps of the center of the body of female groups during the OFT. (E–G) Graphs of the time in the center, number of entries to the center of the arena, and the total distance traveled for the female rats (unpaired t-test, two-tailed; n = 12 female rats per group).
Discussion
In this study, we tested whether microglia contribute to impaired fear extinction after exposure to the traumatic stress of SPS, a well-studied animal model of PTSD (Lisieski et al., 2018; Ferland-Beckham et al., 2021). We found that reducing microglia with oral administration of PLX3397 prevented impaired fear extinction from developing after exposure to SPS in male rats. Although PLX3397 caused a similar reduction in hippocampal microglia in female and male rats, PLX3397 did not prevent SPS from impairing fear extinction in female rats. Consistent with previous reports suggesting that stress affects males and females differently (Keller et al., 2015; Wohleb et al., 2018), this sex-dependent effect of microglial depletion suggests that traumatic stress activates different mechanisms to cause behavioral impairment in male and female rats.
Previous studies suggested that pharmacological activation of microglia could impair fear extinction in male rats. Exposure to lipopolysaccharide (LPS), which activates microglia through their toll-like receptor 4 (Hanke and Kielian, 2011; Pardon, 2015) impaired fear extinction in male rats (Quiñones et al., 2016). Furthermore, blocking angiotensin 1 receptors that are associated with pro-inflammatory microglial activity (Labandeira-Garcia et al., 2017; Jackson et al., 2018), prevented the LPS-induced extinction deficit. Another study found that minocycline, which inhibits LPS activation of microglia (Kobayashi et al., 2013), prevented the infusion of IFNα into the rat amygdala from activating microglia and impairing fear extinction (Bi et al., 2016). Although these studies suggested that the activation of microglia impaired fear extinction, the pharmacological tools used affect cells other than microglia and female rodents were not examined.
Several studies have reported sex-dependent mechanisms associated with microglia-mediated pro-inflammatory responses which could alter their responses to PLX3397. For example, unstressed adult female rats have more microglia (Schwarz et al., 2012) and more primed microglia than male rats (Bollinger et al., 2017), suggesting that females could have more microglia after SPS which would require more PLX3397 to cause the same degree of depletion as in males. We did not find more Iba-1 positive microglia in males than females suggesting that both sexes had similar numbers of microglia prior to PLX3397 treatment. However, SPS increased microglia in the males but not in the females suggesting that exposure to the traumatic stress caused more changes in the microglia in the males.
Alternatively, since acute stress increases primed microglia in male rats and reduces them in females (Bollinger et al., 2017) and chronic stress increases microglial CSF1R expression more in male than in female mice (Wohleb et al., 2018), the acute traumatic stress of SPS could induce more activation of microglia in male rats and make them more sensitive to PLX3397. Some previous studies suggest that male microglia are more sensitive to PLX3397-mediated depletion (Berve et al., 2020; Smith et al., 2020), while others found similar sensitivity to PLX3397 in male and female rodents (Rice et al., 2015; Ma et al., 2020; Delizannis et al., 2021). In the current study, PLX3397 reduced microglia to a similar degree in both sexes suggesting that both sexes exhibited similar sensitivity to PLX3397. However, more Iba-1 positive cells were observed in the ventral hippocampus of the male rats exposed to SPS suggesting that SPS had a larger effect on microglia in the male rats. The larger effect of SPS on microglia in the males could explain why PLX3397 only prevented the impaired fear extinction in the male rats.
We also found evidence that oral PLX3397 treatment exerted sex-dependent effects on hippocampal cytokine expression. Although PLX3397 treatment did not prevent SPS-impaired extinction recall in female rats, increased hippocampal IL-1β and IL-10 cytokine expression were found in PLX3397-treated females, but not in PLX3397-treated male rats. Contrary to our expectations, we did not find alterations in pro-inflammatory cytokines in the hippocampus of SPS-exposed males or female rats. This could be due to the limitations of this study including the time of sacrifice and the lack of cell-type specificity since we examined whole hippocampal cytokine expression 1 day after behavioral procedures. In a related study, we found that isolated hippocampal microglia expressed more IL-1β and TNFα 3 days after SPS exposure (Torres-Rodriguez et al., 2022) suggesting that SPS enhances hippocampal inflammatory cytokines during the first week after exposure.
Previous work found that fear extinction varies with the estrous cycle in female rodents (Milad et al., 2009). Female rodents in the high progesterone/estrogen (proestrus) phase during extinction show better extinction recall than rats in the low progesterone/estrogen (metestrus) phase (Milad et al., 2009). Therefore, the lack of effect of PLX3397 in the females could be due to a higher proportion of animals in the metestrus phase. We did not find any difference in the extinction recall when the females were grouped based on the estrous cycle phase at the time of sacrifice, suggesting that differences in estrous phase among the groups did not obscure an effect of PLX3397 in the female rats. However, it should be noted that the estrous cycle phases were determined 2 days after extinction learning and sample sizes are insufficient to subdivide by estrous cycle phase.
It is important to note several limitations of this study. Although previous studies suggest that PLX3397 causes a global reduction in microglia (Elmore et al., 2015; Green et al., 2020), it is possible that PLX3397 failed to reduce microglia in other key structures involved in fear extinction learning and memory such as the amygdala or infralimbic cortex in the female rats. Furthermore, due to the global effect of oral PLX3397, we could not determine in which brain structure microglia were impairing extinction recall in the males. The effects observed in the ventral hippocampus provide a representation of the effects of SPS and PLX3397 on brain microglia that likely occur in many structures involved in fear regulation, since SPS also increases Iba-1 positive cells in the infralimbic cortex (Torres-Rodriguez et al., 2022) and basolateral amygdala (Lai et al., 2018). In addition, since Iba-1 expression does not distinguish between activated and quiescent microglia, the microglia remaining after depletion with PLX3397 may be more activated or functionally distinct in the female rats. Further experiments are needed to more completely understand how microglia contribute to SPS-induced behavior in male and female rodents.
In conclusion, the lack of effect of PLX3397 on the impaired fear extinction in the female rats exposed to SPS suggests that microglia play a more central role in stress-induced extinction impairment in male rodents due to sex-dependent differences in their transcriptome, proteome profile, and functionality (Guneykaya et al., 2018). Further studies are required to identify sex-dependent microglial mechanisms that explain why PLX3397 treatment only prevented SPS-induced enhanced fear acquisition and impaired fear extinction in male rats.
Data Availability Statement
The raw data supporting the conclusions of this article will be made available by the authors, without undue reservation.
Ethics Statement
The animal study was reviewed and approved by Institutional Animal Care and Use Committee of the Ponce Health Sciences University.
Author Contributions
OT-R and JP designed research and wrote the article. OT-R, EO-N, YR-E, BV, and MC performed research. OT-R, EO-N, YR-E, BV, MC, and JP analyzed data. All authors contributed to the article and approved the submitted version.
Funding
This work was supported by the RCMI BRAIN and MAGIC Cores (National Institute on Minority Health and Health Disparities, NIMHHD U54 MD007579), PR-INBRE Institutional Development Award (IDeA) P20GM103475, and R15 MH116345 from the National Institutes of Health. OT-R and YR-E were supported by the PHSU RISE Program R25-GM082406 and T32 GM144896.
Acknowledgments
A special thanks to Giorgio Perticari, Vanessa Markgraf, and Karina Ruiz for their assistance with this project.
Conflict of Interest
The authors declare that the research was conducted in the absence of any commercial or financial relationships that could be construed as a potential conflict of interest.
Publisher’s Note
All claims expressed in this article are solely those of the authors and do not necessarily represent those of their affiliated organizations, or those of the publisher, the editors and the reviewers. Any product that may be evaluated in this article, or claim that may be made by its manufacturer, is not guaranteed or endorsed by the publisher.
Supplementary Material
The Supplementary Material for this article can be found online at: https://www.frontiersin.org/articles/10.3389/fnbeh.2022.1014767/full#supplementary-material.
References
Berve, K., West, B. L., Martini, R., and Groh, J. (2020). Sex- and region-biased depletion of microglia/macrophages attenuates CLN1 disease in mice. J. Neuroinflammation 17:323. doi: 10.1186/s12974-020-01996-x
Bi, Q., Shi, L., Yang, P., Wang, J., and Qin, L. (2016). Minocycline attenuates interferon-α-induced impairments in rat fear extinction. J. Neuroinflammation 13:172. doi: 10.1186/s12974-016-0638-z
Bollinger, J. L., Collins, K. E., Patel, R., and Wellman, C. L. (2017). Behavioral stress alters corticolimbic microglia in a sex- and brain region-specific manner. PLoS One 12:e0187631. doi: 10.1371/journal.pone.0187631
Castillo-Ocampo, Y., Colón, M., Hernández, A., Lopez, P., Gerena, Y., and Porter, J. T. (2021). Plasticity of GluN1 at ventral hippocampal synapses in the infralimbic cortex. Front. Synaptic Neurosci. 13:695964. doi: 10.3389/FNSYN.2021.695964
Chaby, L. E., Karavidha, K., Lisieski, M. J., Perrine, S. A., and Liberzon, I. (2019). Cognitive flexibility training improves extinction retention memory and enhances cortical dopamine with and without traumatic stress exposure. Front. Behav. Neurosci. 13:24. doi: 10.3389/fnbeh.2019.00024
Chitu, V., Gokhan, Ś., Nandi, S., Mehler, M. F., and Stanley, E. R. (2016). Emerging roles for CSF-1 receptor and its ligands in the nervous system. Trends Neurosci. 39, 378–393. doi: 10.1016/j.tins.2016.03.005
Connor, T. J., Song, C., Leonard, B. E., Merali, Z., and Anisman, H. (1998). An assessment of the effects of central interleukin-1,-2,-6 and tumor necrosis factor-administration on some behavioural, neurochemical, endocrine and immune parameters in the rat. Neuroscience 84, 923–933. doi: 10.1016/s0306-4522(97)00533-2
Cotrone, T. S., Hocog, C. B., Ramsey, J. T., Sanchez, M. A., Sullivan, H. M., and Scrimgeour, A. G. (2021). Phenotypic characterization of frontal cortex microglia in a rat model of post-traumatic stress disorder. Brain Behav. 11:e02011. doi: 10.1002/brb3.2011
Criado-Marrero, M., Morales Silva, R. J., Velazquez, B., Hernández, A., Colon, M., Cruz, E., et al. (2017). Dynamic expression of FKBP5 in the medial prefrontal cortex regulates resiliency to conditioned fear. Learn. Mem. 24, 145–152. doi: 10.1101/lm.043000.116
Cruz, E., Soler-Cedeño, O., Negrón, G., Criado-Marrero, M., Chompré, G., and Porter, J. T. (2015). Infralimbic EphB2 modulates fear extinction in adolescent rats. J. Neurosci. 35, 12394–12403. doi: 10.1523/JNEUROSCI.4254-14.2015
Delizannis, A. T., Nonneman, A., Tsering, W., de Bondt, A., van den Wyngaert, I., Zhang, B., et al. (2021). Effects of microglial depletion and TREM2 deficiency on Aβ plaque burden and neuritic plaque tau pathology in 5XFAD mice. Acta Neuropathol. Commun. 9:9. doi: 10.1186/s40478-021-01251-1
Elmore, M. R. P., Lee, R. J., West, B. L., and Green, K. N. (2015). Characterizing newly repopulated microglia in the adult mouse: impacts on animal behavior, cell morphology and neuroinflammation. PLoS One 10:e0122912. doi: 10.1371/journal.pone.0122912
Erblich, B., Zhu, L., Etgen, A. M., Dobrenis, K., and Pollard, J. W. (2011). Absence of colony stimulation factor-1 receptor results in loss of microglia, disrupted brain development and olfactory deficits. PLoS One 6:e26317. doi: 10.1371/journal.pone.0026317
Ferland-Beckham, C., Chaby, L. E., Daskalakis, N. P., Knox, D., Liberzon, I., Lim, M. M., et al. (2021). Systematic review and methodological considerations for the use of single prolonged stress and fear extinction retention in rodents. Front. Behav. Neurosci. 15:652636. doi: 10.3389/fnbeh.2021.652636
Ferrara, N. C., Trask, S., Yan, L., Padival, M., Helmstetter, F. J., and Rosenkranz, J. A. (2022). Isolation driven changes in Iba1-positive microglial morphology are associated with social recognition memory in adults and adolescents. Neurobiol. Learn. Mem. 192:107626. doi: 10.1016/j.nlm.2022.107626
George, S. A., Rodriguez-Santiago, M., Riley, J., Abelson, J. L., Floresco, S. B., and Liberzon, I. (2015). Alterations in cognitive flexibility in a rat model of post-traumatic stress disorder. Behav. Brain Res. 286, 256–264. doi: 10.1016/j.bbr.2015.02.051
George, S. A., Rodriguez-Santiago, M., Riley, J., Abelson, J. L., Floresco, S. B., and Liberzon, I. (2018). D-cycloserine facilitates reversal in an animal model of post-traumatic stress disorder. Behav. Brain Res. 347, 332–338. doi: 10.1016/j.bbr.2018.03.037
Gill, J. M., Saligan, L., Lee, H., Rotolo, S., and Szanton, S. (2013). Women in recovery from PTSD have similar inflammation and quality of life as non-traumatized controls. J. Psychosom. Res. 74, 301–306. doi: 10.1016/j.jpsychores.2012.10.013
Green, K. N., Crapser, J. D., and Hohsfield, L. A. (2020). To kill a microglia: a case for CSF1R inhibitors. Trends Immunol. 41, 771–784. doi: 10.1016/j.it.2020.07.001
Groer, M. W., Kane, B., Williams, S. N., and Duffy, A. (2015). Relationship of PTSD symptoms with combat exposure, stress and inflammation in american soldiers. Biol. Res. Nurs. 17, 303–310. doi: 10.1177/1099800414544949
Guneykaya, D., Ivanov, A., Hernandez, D. P., Haage, V., Wojtas, B., Meyer, N., et al. (2018). Transcriptional and translational differences of microglia from male and female brains. Cell Rep. 24, 2773–2783.e6. doi: 10.1016/j.celrep.2018.08.001
Hanke, M. L., and Kielian, T. (2011). Toll-like receptors in health and disease in the brain: mechanisms and therapeutic potential. Clin. Sci. 121, 367–387. doi: 10.1042/CS20110164
Jackson, L., Eldahshan, W., Fagan, S. C., and Ergul, A. (2018). Within the brain: the renin angiotensin system. Int. J. Mol. Sci. 19:876. doi: 10.3390/ijms19030876
Kataoka, T., Fuchikami, M., Nojima, S., Nagashima, N., Araki, M., Omura, J., et al. (2019). Combined brain-derived neurotrophic factor with extinction training alleviate impaired fear extinction in an animal model of post-traumatic stress disorder. Genes Brain Behav. 18:e12520. doi: 10.1111/gbb.12520
Katrinli, S., Oliveira, N. C. S., Felger, J. C., Michopoulos, V., and Smith, A. K. (2022). The role of the immune system in posttraumatic stress disorder. Transl. Psychiatry 12:313. doi: 10.1038/s41398-022-02094-7
Keller, S. M., Schreiber, W. B., Staib, J. M., and Knox, D. (2015). Sex differences in the single prolonged stress model. Behav. Brain Res. 286, 29–32. doi: 10.1016/j.bbr.2015.02.034
Kim, Y. S., and Joh, T. H. (2006). Microglia, major player in the brain inflammation: their roles in the pathogenesis of Parkinson’s disease. Exp. Mol. Med. 38, 333–347. doi: 10.1038/emm.2006.40
Kim, T. D., Lee, S., and Yoon, S. (2020). Inflammation in post-traumatic stress disorder (PTSD): a review of potential correlates of PTSD with a neurological perspective. Antioxidants 9:107. doi: 10.3390/antiox9020107
Knox, D., George, S. A., Fitzpatrick, C. J., Rabinak, C. A., Maren, S., and Liberzon, I. (2012). Single prolonged stress disrupts retention of extinguished fear in rats. Learn. Mem. 19, 43–49. doi: 10.1101/lm.024356.111
Kobayashi, K., Imagama, S., Ohgomori, T., Hirano, K., Uchimura, K., Sakamoto, K., et al. (2013). Minocycline selectively inhibits M1 polarization of microglia. Cell Death Dis. 4:e525. doi: 10.1038/cddis.2013.54
Labandeira-Garcia, J. L., Rodríguez-Perez, A. I., Garrido-Gil, P., Rodriguez-Pallares, J., Lanciego, J. L., and Guerra, M. J. (2017). Brain renin-angiotensin system and microglial polarization: implications for aging and neurodegeneration. Front. Aging Neurosci. 9:129. doi: 10.3389/fnagi.2017.00129
Lai, S., Wu, G., and Jiang, Z. (2018). Glycyrrhizin treatment facilitates extinction of conditioned fear responses after a single prolonged stress exposure in rats. Cell. Physiol. Biochem. 45, 2529–2539. doi: 10.1159/000488271
Lee, B., Sur, B., Yeom, M., Shim, I., Lee, H., and Hahm, D. H. (2016). Effects of systemic administration of ibuprofen on stress response in a rat model of post-traumatic stress disorder. Korean J. Physiol. Pharmacol. 20, 357–366. doi: 10.4196/kjpp.2016.20.4.357
Li, S., Liao, Y., Dong, Y., Li, X., Li, J., Cheng, Y., et al. (2021). Microglial deletion and inhibition alleviate behavior of post-traumatic stress disorder in mice. J. Neuroinflammation 18:7. doi: 10.1186/s12974-020-02069-9
Liberzon, I., and Young, E. A. (1997). Effects of stress and glucocorticoids on cns oxytocin receptor binding. Psychoneuroendocrinology 22, 411–422. doi: 10.1016/s0306-4530(97)00045-0
Liberzon, I., López, J. F., Flagel, S. B., Vázquez, D. M., and Young, E. A. (1999). Differential regulation of hippocampal glucocorticoid receptors mRNA and fast feedback: relevance to enhanced cort sensitivity seen in post-traumatic stress disorder. J. Neuroendocrinol. 11, 11–17. doi: 10.1046/j.1365-2826.1999.00288.x
Linker, K. E., Elabd, M. G., Tawadrous, P., Cano, M., Green, K. N., Wood, M. A., et al. (2020). Microglial activation increases cocaine self-administration following adolescent nicotine exposure. Nat. Commun. 11:306. doi: 10.1038/s41467-019-14173-3
Lisieski, M. J., Eagle, A. L., Conti, A. C., Liberzon, I., and Perrine, S. A. (2018). Single-prolonged stress: a review of two decades of progress in a rodent model of post-traumatic stress disorder. Front. Psychiatry 9:196. doi: 10.3389/fpsyt.2018.00196
Liu, Y. J., Spangenberg, E. E., Tang, B., Holmes, T. C., Green, K. N., and Xu, X. (2021). Microglia elimination increases neural circuit connectivity and activity in adult mouse cortex. J. Neurosci. 41, 1274–1287. doi: 10.1523/JNEUROSCI.2140-20.2020
Liu, F.-F., Yang, L.-D., Sun, X.-R., Zhang, H., Pan, W., Wang, X.-M., et al. (2016). NOX2 mediated-parvalbumin interneuron loss might contribute to anxiety-like and enhanced fear learning behavior in a rat model of post-traumatic stress disorder. Mol. Neurobiol. 53, 6680–6689. doi: 10.1007/s12035-015-9571-x
Ma, X., Chen, K., Cui, Y., Huang, G., Nehme, A., Zhang, L., et al. (2020). Depletion of microglia in developing cortical circuits reveals its critical role in glutamatergic synapse development, functional connectivity and critical period plasticity. J. Neurosci. Res. 98, 1968–1986. doi: 10.1002/jnr.24641
Merry, T. L., Brooks, A. E. S., Masson, S. W., Adams, S. E., Jaiswal, J. K., Jamieson, S. M. F., et al. (2020). The CSF1 receptor inhibitor pexidartinib (PLX3397) reduces tissue macrophage levels without affecting glucose homeostasis in mice. Int. J. Obes. (Lond) 44, 245–253. doi: 10.1038/s41366-019-0355-7
Michopoulos, V., Powers, A., Gillespie, C. F., Ressler, K. J., and Jovanovic, T. (2017). Inflammation in fear-and anxiety-based disorders: PTSD, GAD and beyond. Neuropsychopharmacology 42, 254–270. doi: 10.1038/npp.2016.146
Milad, M. R., Igoe, S. A., Lebron-Milad, K., and Novales, J. E. (2009). Estrous cycle phase and gonadal hormones influence conditioned fear extinction. Neuroscience 164, 887–895. doi: 10.1016/j.neuroscience.2009.09.011
Pardon, M.-C. (2015). Lipopolysaccharide hyporesponsiveness: protective or damaging response to the brain? Rom. J. Morphol. Embryol. 56, 903–913. Available online at: http://www.rjme.ro/.
Park, C. H. J., Ganella, D. E., Perry, C. J., and Kim, J. H. (2020). Dissociated roles of dorsal and ventral hippocampus in recall and extinction of conditioned fear in male and female juvenile rats. Exp. Neurol. 329:113306. doi: 10.1016/j.expneurol.2020.113306
Quiñones, M. M., Maldonado, L., Velazquez, B., and Porter, J. T. (2016). Candesartan ameliorates impaired fear extinction induced by innate immune activation. Brain Behav. Immun. 52, 169–177. doi: 10.1016/j.bbi.2015.10.017
Rice, R. A., Spangenberg, E. E., Yamate-Morgan, H., Lee, R. J., Arora, R. P. S., Hernandez, M. X., et al. (2015). Elimination of microglia improves functional outcomes following extensive neuronal loss in the hippocampus. J. Neurosci. 35, 9977–9989. doi: 10.1523/JNEUROSCI.0336-15.2015
Schwarz, J. M., Sholar, P. W., and Bilbo, S. D. (2012). Sex differences in microglial colonization of the developing rat brain. J. Neurochem. 120, 948–963. doi: 10.1111/j.1471-4159.2011.07630.x
Sierra-Mercado, D., Padilla-Coreano, N., and Quirk, G. J. (2011). Dissociable roles of prelimbic and infralimbic cortices, ventral hippocampus and basolateral amygdala in the expression and extinction of conditioned fear. Neuropsychopharmacology 36, 529–538. doi: 10.1038/npp.2010.184
Smith, B. L., Laaker, C. J., Lloyd, K. R., Hiltz, A. R., and Reyes, T. M. (2020). Adolescent microglia play a role in executive function in male mice exposed to perinatal high fat diet. Brain Behav. Immun. 84, 80–89. doi: 10.1016/j.bbi.2019.11.010
Sun, R., Zhang, Z., Lei, Y., Liu, Y., Lu, C., Rong, H., et al. (2016). Hippocampal activation of microglia may underlie the shared neurobiology of comorbid posttraumatic stress disorder and chronic pain. Mol. Pain 12:1744806916679166. doi: 10.1177/1744806916679166
Swiergiel, A. H., and Dunn, A. J. (2007). Effects of interleukin-1β and lipopolysaccharide on behavior of mice in the elevated plus-maze and open field tests. Pharmacol. Biochem. Behav. 86, 651–659. doi: 10.1016/j.pbb.2007.02.010
Torres-Rodriguez, O., Rivera-Escobales, Y., Velazquez, B., Colón, M., and Porter, J. T. (2022). Purinergic P2X7 receptor-mediated inflammation precedes PTSD-related behaviors in rats. bioRxiv [Preprint]. doi: 10.1101/2022.03.10.483788
Wang, W., Wang, L., Xu, H., Cao, C., Liu, P., Luo, S., et al. (2019). Characteristics of pro- and anti-inflammatory cytokines alteration in PTSD patients exposed to a deadly earthquake. J. Affect. Disord. 248, 52–58. doi: 10.1016/j.jad.2019.01.029
Wilson, C. B., McLaughlin, L. D., Nair, A., Ebenezer, P. J., Dange, R., and Francis, J. (2013). Inflammation and oxidative stress are elevated in the brain, blood and adrenal glands during the progression of post-traumatic stress disorder in a predator exposure animal model. PLoS One 8:e76146. doi: 10.1371/journal.pone.0076146
Wohleb, E. S., McKim, D. B., Shea, D. T., Powell, N. D., Tarr, A. J., Sheridan, J. F., et al. (2014). Re-establishment of anxiety in stress-sensitized mice is caused by monocyte trafficking from the spleen to the brain. Biol. Psychiatry 75, 970–981. doi: 10.1016/j.biopsych.2013.11.029
Keywords: ventral hippocampus, fear extinction, microglia, cytokines, PTSD-like, sex-dependent
Citation: Torres-Rodriguez O, Ortiz-Nazario E, Rivera-Escobales Y, Velazquez B, Colón M and Porter JT (2023) Sex-dependent effects of microglial reduction on impaired fear extinction induced by single prolonged stress. Front. Behav. Neurosci. 16:1014767. doi: 10.3389/fnbeh.2022.1014767
Received: 08 August 2022; Accepted: 12 December 2022;
Published: 09 January 2023
Edited by:
Sandeep Sharma, University of Calgary, CanadaReviewed by:
Miriam Beatriz Virgolini, Universidad Nacional de Córdoba, ArgentinaSusan K. Wood, University of South Carolina, United States
Copyright © 2023 Torres-Rodriguez, Ortiz-Nazario, Rivera-Escobales, Velazquez, Colón and Porter. This is an open-access article distributed under the terms of the Creative Commons Attribution License (CC BY). The use, distribution or reproduction in other forums is permitted, provided the original author(s) and the copyright owner(s) are credited and that the original publication in this journal is cited, in accordance with accepted academic practice. No use, distribution or reproduction is permitted which does not comply with these terms.
*Correspondence: James T. Porter, jporter@psm.edu