Delirium from the gliocentric perspective
- 1Psychiatry, Patton State Hospital, CA, USA
- 2Psychiatry, Loma Linda University, CA, USA
- 3Evidence Based Health Care, University of Oxford, Oxford, UK
- 4Pathology, Sapienza University, Rome, Italy
Delirium is an acute state marked by disturbances in cognition, attention, memory, perception, and sleep-wake cycle which is common in elderly. Others have shown an association between delirium and increased mortality, length of hospitalization, cost, and discharge to extended stay facilities. Until recently it was not known that after an episode of delirium in elderly, there is a 63% probability of developing dementia at 48 months compared to 8% in patients without delirium. Currently there are no preventive therapies for delirium, thus elucidation of cellular and molecular underpinnings of this condition may lead to the development of early interventions and thus prevent permanent cognitive damage. In this article we make the case for the role of glia in the pathophysiology of delirium and describe an astrocyte-dependent central and peripheral cholinergic anti-inflammatory shield which may be disabled by astrocytic pathology, leading to neuroinflammation and delirium. We also touch on the role of glia in information processing and neuroimaging.
Is Delirium an Astropathy?
The search for cellular and molecular underpinnings of delirium became important as it was demonstrated that after an episode of delirium in elderly, there is a 63% probability of developing dementia at 48 months compared to 8% in patients without delirium1 (Pandharipande et al., 2013). Furthermore, an association was shown between delirium and increased mortality, length of hospitalization, cost, and discharge to extended stay facilities (Witlox et al., 2010).
Examined exclusively from the neuronal standpoint, delirium is often described as a neurobehavioral syndrome. In this article we take a gliocentric approach and look at the role of astrocytes in the pathophysiology of delirium. Observed from this angle, delirium is as much a neuro as a gliobehavioral disorder marked by a global cholinergic deficit and a malignant inflammation. We believe that both hypo-cholinergia and inflammation are symptoms of astrocytic failure. Furthermore, aside from reconciling neuroinflammation and cholinergic deficit in the pathogenesis of delirium, adopting a glial perspective explains the astrocytic origin of most delirium markers.
Acethylcholine (ACh) signaling was suggested to engender a potent cholinergic anti-inflammatory shield (CAIS), protecting against peripheral and central inflammation (Tracey, 2009). CAIS operates in the extracellular space (ECS) both peripherally and centrally by blocking the release of pro-inflammatory mediators (Shaked et al., 2009; Han et al., 2014). CAIS consists of extracellular ACh biosynthesis, its diffusion through the interstitium and action on alpha-7 nicotinic ACH receptors (nAChR) expressed on the peripheral and central immune cells (macrophages, natural killer cells, lymphocytes, astrocytes and microglia), as well as on neuronal and endothelial cells in the CNS (Perry and Teeling, 2013; Figure 1).
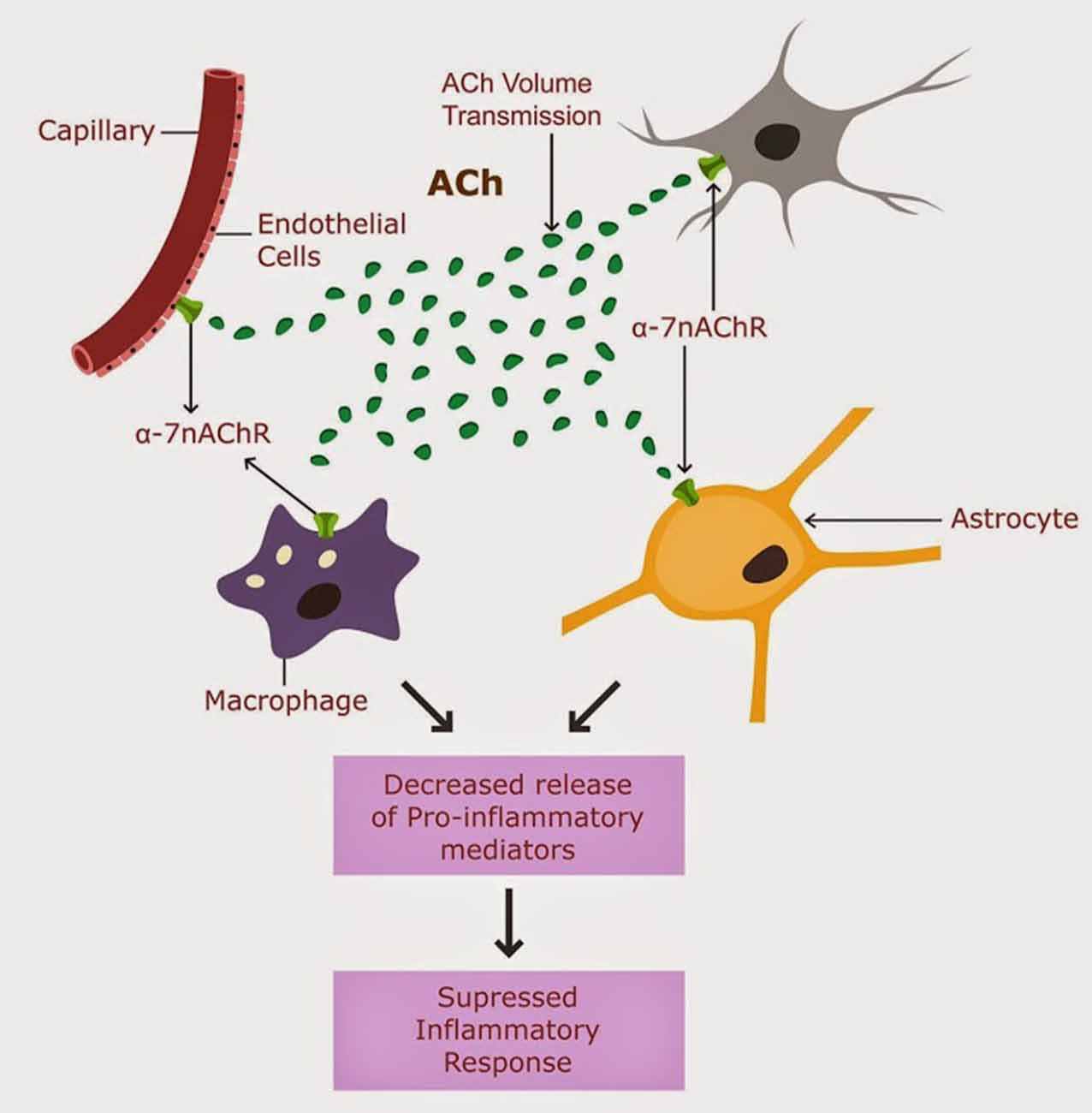
Figure 1. CAIS is operational in the CNS, and involves ACh action of alpha 7 nAChRs expressed on astrocytes, neurons, microglia and brain macrophages (Tyagi et al., 2010).
The brain is not protected from peripheral pro-inflammatory mediators, as it was believed in the past. In fact, recent studies demonstrate that peripheral cytokines cross routinely the intact blood brain barrier and interact with brain cells. Despite their entry into the CNS, the peripheral pro-inflammatory cytokines are inactivated by CAIS, thus preventing the seeding of inflammation into the brain.
We propose that CAIS is engendered by the astrocytes by secretion of choline acetyltransferase (ChAT), the ACh synthesizing enzyme, into the ECS and by regulation of the interstitial fluid (ISF) volume.
Astrocytes maintain brain water homeostasis by regulating the ISF volume. They accomplish this as their membranes are more permeable to water than those of other brain cells (8). This unique property of astrocytes is based on aquaporine-4 (AQP-4) water channels expressed in large numbers on their end feet processes. In fact, astrocytes express the largest number of AQP-4 receptors in the entire CNS (Nagelhus and Ottersen, 2013). This unique property of their membranes confers astrocytes a sponge-like ability to absorb and release water as needed, thus regulating ISF volume (Gundersen, 2013). With the same token, this property renders astrocytes vulnerable to cytotoxic edema with resultant ISF hypovolemia. As a result of swelling, astrocytes become insufficient, thus unable to secrete ChAT into the ECS which disables CAIS, unleashing neuroinflammation. Moreover, ISF hypovolemia disables beta-amyloid clearance, cluttering the ECS, and disabling CAIS further. Indeed, astrocyte swelling and accumulation of molecular waste in the interstitium was described in several neuro-psychiatric conditions including: sepsis-induced delirium (SID), stroke, traumatic brain injury, brain tumors and metastases, meningitis, brain abscesses, water intoxication, altitude sickness, malignant hypertension, hypoglycemia, and metabolic encephalopathies (Thrane et al., 2014).
We hypothesize that delirium is the clinical manifestation of astrocytic failure caused by astrocytic edema. Edema is the result of AQP-4 water channels’ overexpression on astrocytic membranes. We hypothesize further that failed astrocytes are unable to secrete ChAT into the ECS, disabling CAIS, while the resultant ISF hypovolemia disables beta-amyloid clearance, further impairing CAIS. In the absence CAIS protection, uncontrolled neuroinflammation flares up possibly resulting in delirium. To illustrate this hypothesis, we envision astrocytes as the firefighters of the brain. They keep the fire (neuroinflammation) under control, by maintaining enough water reserves into the interstitial space. In the absence of this water, fire flares up, resulting in uncontrolled neuroinflammation and delirium.
The Brain-Immune Interface
The fact that the brain is an immunologically privileged organ is a myth. Recent studies demonstrate a continuous two-way communication between the CNS and the peripheral immune system (Perry and Teeling, 2013). For example, it was demonstrated that a cardiac arrest can induce alterations in the central cholinergic signaling, including reduction of ChAT and vesicular ACh transporter mRNA (Norman et al., 2011; Zhao et al., 2013). Conversely, global cerebral ischemia often induces peripheral inflammation (Zhan et al., 2010). This inflammatory response can be pharmacologically reversed by the administration of selective alpha 7 nAChR agonists such as GTS-21, suggesting a role for selective nicotinic agonists in delirium (de Jonge and Ulloa, 2007; Kox et al., 2011).
Alpha 7 nAChRs agonistic ligands are currently being investigated in the treatment of sepsis, inflammation, dementia and schizophrenia (Han et al., 2014). In addition, CAIS was shown to prevent obesity-induced inflammation and insulin-resistance (Lakhan and Kirchgessne, 2011). It is well known that muscarinic M3 receptors in the pancreas are involved in the metabolic syndrome (Duttaroy et al., 2004). This beneficial effect of ACh can be reversed by the administration of alpha 7 nAChR antagonists (Kox et al., 2011). Furthermore, the action of ACh on selective muscarinic receptors was demonstrated to modulate cellular proliferation and is therefore of interest in various cancers (Spindel, 2012; Ferretti et al., 2013). Interestingly, an aberrant neuronal cell cycle entry was suggested in the pathogenesis of Alzheimer’s disease (AD; Stieler et al., 2001).
Biological Markers: Not too Specific for Delirium, but Specific for Astrocytes
Most biological markers of interest in delirium can be traced to astrocytes. It is well established that Matrix Metaloprotease-9 (MMP-9; Yin et al., 2006), S 100B protein (Tateishi et al., 2006) and glial fibrillary acidic protein (GFAP; Brownell et al., 1991) are secreted by astrocytes. Procalcitonin (McGrane et al., 2011), a newly identified marker of delirium caused by sepsis is expressed on neurons, astrocytes, microglia and oligodendrocytes (Ojeda et al., 2006).
A recent CSF proteomic analysis identified four categories of markers in delirium. Interestingly, they can be traced to the astrocytes as well (Poljak et al., 2014). They include: acute phase proteins, granins, serine protease inhibitors, and apolipoproteins (references in parenthesis demonstrate glial origin).
Acute phase proteins: lipocalins (Jang et al., 2013) (includes alpha-1-acidic glycoprotein), alpha-2 microglobulin (Bauer et al., 1988), fibrinogen (Pichler et al., 1999; Hsiao et al., 2013), alpha-1 antitrypsin (Gollin et al., 1992), alpha-1 antichymotrypsin (Gollin et al., 1992), transferrin (Qian et al., 1999), complement component 3 (Maranto et al., 2008), and haptoglobin (Lee et al., 2002).
Interestingly, alpha 1-acidic glycoprotein was shown to decrease brain cellular edema after experimental stroke, possibly by down-regulating AQP-4 receptors (Pichler et al., 1999).
Granins: secretogranin 3 (Paco et al., 2010).
Serine protease inhibitors: occur both in neurons and glial cells (Buisson et al., 1998).
Apolipoproteins: Apo A1 (Ito et al., 2002), ApoJ (DeMattos et al., 2001), ApoE (DeMattos et al., 2001).
These markers point to glial pathology in delirium and are in line with our hypothesis on astrocytic failure.
Astrocytes and ChAT Secretion
At the cellular level, ACh biosynthesis occurs in two distinct brain compartments: the neuronal bodies of brain cholinergic tracts and the ECS of CNS (Vijayaraghavan et al., 2013). Regardless of their origin, ACh manufacture depends on the availability of its synthesizing enzyme ChAT. Secreted by astrocytes into the ECS, this enzyme ensures a constant presence of ACh in the interstitium (Vijayaraghavan et al., 2013). Figure 1. This ambient ACh is crucial for proper CAIS functioning. Previously it was assumed that ACh could not participate in long distance intercellular signaling because of the presence of its hydrolyzing enzymes, acetylcholinesterase (AChE) and butyrylcholinesterase (BuChE) in the ECS. Recent studies reveal that ACh can “survive” in the ECS and participate in long distance signaling despite physiological levels of AChE and BuChE because it can be synthesized ad hoc with astrocyte-secreted ChAT (Vijayaraghavan et al., 2013).
Diminished ACh signaling in the ECS disables CAIS and this event is marked by an immediate compensatory up-regulation of alpha 7 nAChRs, the first sign of inflammation. We believe that alpha 7 nAChRs up-regulation is the first measurable marker of astrocytic failure. Indeed, a recent study demonstrates that increased astrocytic expression of alpha 7 nAChRs is positively correlated with the extent of neuropathological alterations in AD (Yu et al., 2012). Measuring ECS level of ChAT may provide a biological marker of pending inflammation as it expresses alpha 7 nAChRs up-regulation.
Astrocytes contribute to beta-amyloid clearance either directly, by phagocytosis, or indirectly by the glymphatic system (Nagele et al., 2003; Lasagna-Reeves and Kayed, 2011; Sokolowski and Mandell, 2011; Iliff et al., 2012). We believe that swollen, failed astrocytes may be inefficient in disposing of beta amyloid and other molecular waste, leading to their accumulation and neuroinflammation. Indeed, studies in AD reveal that excess beta-amyloid in the ECS alters cholinergic signaling by activating BuChE, decreasing secretion of astrocytic ChAT and up-regulating alpha 7 nACh receptors on astrocytic membranes (Vijayaraghavan et al., 2013; Malmsten et al., 2014).
Beta amyloid clearance via the glymphatic system is highly dependent on the intra-parenchymal water exchange between the cerebrospinal fluid (CSF) and the ISF, occurring via AQP-4 channels in astrocytic end-feet (Yang et al., 2013) Figure 2.
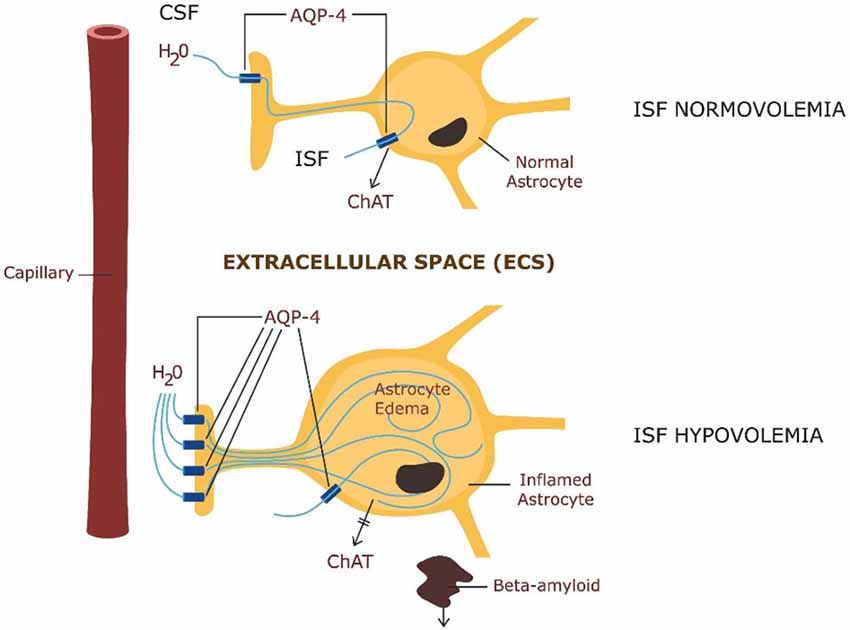
Figure 2. ABOVE: Astrocytic physiology—intra-parenchymal CSF exchange with the interstitial fluid (ISF) via AQP-4 channels and ChAT secretion. BELOW: Pathology—astrocytic edema: up-regulation of AQP-4 in astrocytic end-feet with impairment of ChAT secretion and beta amyloid clearance.
Accumulation of beta-amyloid and low ACh were documented in delirium (Hshieh et al., 2008) and led to the emission of the cholinergic hypothesis in the pathogenesis of this condition. However, since cholinesterase inhibitors showed only mild or no efficacy in delirium, this hypothesis seemed to have been invalidated (van Eijk et al., 2010; Brinker et al., 2014).
Astrocytic failure hypothesis offers an alternative explanation that does not necessarily invalidate the cholinergic hypothesis. On the contrary, since they do not restore astrocytic secretion of ChAT, cholinesterase inhibitors are not expected to beneficial in delirium. In the absence of ACh biosynthesis in the ECS, inhibiting its hydrolyzing enzymes would not resurrect CAIS function. On the other hand, co-administration of cholinesterase inhibitors with drugs capable of restoring the extracellular water volume (such as agonists of alpha 7 nAChRs, direct AQP-4 blockers or indirect ones, such as erytropoietin) may be salutary.
Is there a Water Connectome?
Being more permeable to water, astrocytes serve as brain fluid reservoirs where water can be stored and also retrieved as needed (Gundersen, 2013). The high AQP-4 expression on their membranes enables astrocytes to move water in and out of the neuropile. Studies done three decades ago show that water homeostasis is dependent on the neuropile activity (Dudek and Rogawski, 2005). For example, during the day (when information processing is usually more intense), water is shifted into the astrocytic compartment, while during the night (when there is less information processing), water is shifted back into the interstitium (Xie et al., 2013). This water movement was hypothesized to help beta amyloid clearance during sleep (Brinker et al., 2014).
Water can cross cellular membranes slowly by diffusion or co-transport with other substances, but the quickest modality of water transport occurs via AQP-4 receptors which abound on astrocytic membranes (Gundersen, 2013). The high permeability of astrocytic membranes and the sponge-like properties of these cells render neuroimaging possible. Diffusion tensor imaging (DTI) visualizes water diffusion across membranes which can be either unrestricted (isotropy) or restricted by the myelin sheath (anisotropy). Since water does not diffuse through the myelin, but follows it, this technique is used for tracing white matter tracts (Lazar et al., 2003; Lazar, 2010).
We suggest that DTI could be utilized for assessing glial integrity by adapting its algorithm to the areas of highest isotropy. Since astrocytes are the most water permeable cells in the brain, the highest CNS isotropy must coincide anatomically with astrocytes.
Recent data point to the fact that DTI signal changes, attributed earlier to white matter tracts and axonal myelination, may in fact mirror astrocytic expression of AQP-4 receptors (Meng et al., 2004; Tourdias et al., 2009), astrocytic edema (Harsan et al., 2007; Fukuda and Badaut, 2012), ISF volume (Fukuda and Badaut, 2012), or glial scars (Budde et al., 2011).
In its journey through the brain fluid compartments, water carries along signaling molecules that comprise an extra-cellular communication platform described as volume transmission (VT; Agnati et al., 1995). Does this signaling platform fathom a liquid connectome? Furthermore, since it mirrors the water flow along white matter tracts, is the connectome in general a liquid connectome? It was proposed that point-to-point synaptic transmission engenders a quick and precise mode of information processing, such as the one necessary for playing tennis or piano, while the diffuse and widespread communication platform by water signaling may engender more pervasive functions such as awareness, attention, mood, circadian rhythm or cognition (Gualtieri, 2002; Taber and Hurley, 2014; Vizi et al., 2010). As these functions are known to “wax and wane” in delirium, can they be part of an astrocyte-centered information processing? Recent studies imply that astrocytic networks are crucial for information integration and cholinergic plasticity (Pereira and Furlan, 2010; Takata et al., 2011). A recent study demonstrated that ACh signaling is highly correlated with gamma oscillations on the EEG, which are believed to facilitate the somatosensory cortex plasticity (Rodriguez et al., 2004). This may suggest an astrocytic-based cognition, possibly complementary to the rapid, point-to-point information processing established by neurons.
Conclusions
Memory characterizes both, cellular immunity and human cognition. Both systems are adapted to interact with the surrounding world and to protect from it. Both are capable of distinguishing “self” from the environment (“non-self; Lampron et al., 2013). Since nature rarely wastes ideas, immunologic memory may be a distant phylogenetic ancestor of human cognition. Controlled inflammation and cellular proliferation may benefit hippocampal neuroplasticity and long term potentiation. Out of control, inflammation, on the other hand, may lead to sepsis, while aberrant cellular proliferation to cancer. With the same token, activation of cellular proliferation in non-dividing cells, like neurons, may lead to aberrant cell cycle entry, resulting in apoptosis. This may be the case in AD.
Both astrocytes and microglia are part of the innate immune system which responds to the early stages of infection by triggering non-specific, high collateral damage, inflammatory responses, which are later expected to die down, as the high precision, specific, adaptive immune system is activated. Failure of the innate system to shut down may result in “cytokine storms” encountered in sepsis and sepsis-induced delirium (SID). On the other hand, intact astrocytes with proper CAIS calm down the innate immune system, containing inflammatory responses. Failed astrocytes unleash exaggerated inflammation which may lead to delirium. Engel and Romano (1959) described delirium as “brain failure”; we think of it as a primary astrocytic failure with secondary neuronal involvement. Since astrocytic suffering occurs prior to neuronal damage, identifying it early may offer a window of opportunity for preventive interventions that could salvage the cognitive domain before the occurrence of neuronal apoptosis.
Conflict of Interest Statement
The authors declare that the research was conducted in the absence of any commercial or financial relationships that could be construed as a potential conflict of interest.
Footnotes
- ^ American Delirium Society. https://www.americandeliriumsociety.org/about-delirium/healthcare-professionals, available on line.
References
Agnati, L. F., Zoli, M., Strömberg, I., and Fuxe, K. (1995). Intercellular communication in the brain: wiring versus volume transmission. Neuroscience 69, 711–726. doi: 10.1016/0306-4522(95)00308-6
Bauer, J., Gebicke-Haerter, P. J., Ganter, U., Richter, I., and Gerok, W. (1988). Astrocytes synthesize and secrete alpha 2-macroglobulin: differences between the regulation of alpha 2-macroglobulin synthesis in rat liver and brain. Adv. Exp. Med. Biol. 240, 199–205. doi: 10.1007/978-1-4613-1057-0_24
Brinker, T., Stopa, E., Morrison, J., and Klinge, P. (2014). A new look at cerebrospinal fluid circulation. Fluids Barriers CNS 11:10. doi: 10.1186/2045-8118-11-10
Brownell, E., Lee, A. S., Pekar, S. K., Pravtcheva, D., Ruddle, F. H., and Bayney, R. M. (1991). Glial fibrillary acid protein, an astrocytic-specific marker, maps to human chromosome 17. Genomics 10, 1087–1089. doi: 10.1016/0888-7543(91)90205-s
Budde, M. D., Janes, L., Gold, E., Turtzo, L. C., and Frank, J. A. (2011). The contribution of gliosis to diffusion tensor anisotropy and tractography following traumatic brain injury: validation in the rat using fourier analysis of stained tissue sections. Brain 134, 2248–2260. doi: 10.1093/brain/awr161
Buisson, A., Nicole, O., Docagne, F., Sartelet, H., Mackenzie, E. T., and Vivien, D. (1998). Up-regulation of a serine protease inhibitor in astrocytes mediates the neuroprotective activity of transforming growth factor beta1. FASEB J. 12, 1683–1691.
de Jonge, W. J., and Ulloa, L. (2007). The alpha7 nicotinic acetylcholine receptor as a pharmacological target for inflammation. Br. J. Pharmacol. 151, 915–929. doi: 10.1038/sj.bjp.0707264
DeMattos, R. B., Brendza, R. P., Heuser, J. E., Kierson, M., Cirrito, J. R., Fryer, J., et al. (2001). Purification and characterization of astrocyte-secreted apolipoprotein E and J-containing lipoproteins from wild-type and human apoE transgenic mice. Neurochem. Int. 39, 415–425. doi: 10.1016/s0197-0186(01)00049-3
Dudek, F. E., and Rogawski, M. A. (2005). Regulation of brain water: is there a role for aquaporins in epilepsy? Epilepsy Curr. 5, 104–106. doi: 10.1111/j.1535-7511.2005.05310.x
Duttaroy, A., Zimliki, C. L., Gautam, D., Cui, Y., Mears, D., and Wess, J. (2004). Muscarinic stimulation of pancreatic insulin and glucagon release is abolished in m3 muscarinic acetylcholine receptor-deficient mice. Diabetes 53, 1714–1720. doi: 10.2337/diabetes.53.7.1714
Engel, G. L., and Romano, J. (1959). Delirium, a syndrome of cerebral insufficiency. J. Chronic Dis. 9, 260–277. doi: 10.1016/0021-9681(59)90165-1
Ferretti, M., Fabbiano, C., Di Bari, M., Conte, C., Castigli, E., Sciaccaluga, M., et al. (2013). M2 receptor activation inhibits cell cycle progression and survival in human glioblastoma cells. J. Cell. Mol. Med. 17, 552–566. doi: 10.1111/jcmm.12038
Fukuda, A. M., and Badaut, J. (2012). Aquaporin 4: a player in cerebral edema and neuroinflammation. J. Neuroinflammation 9:279. doi: 10.1186/1742-2094-9-279
Gollin, P. A., Kalaria, R. N., Eikelenboom, P., Rozemuller, A., and Perry, G. (1992). Alpha 1-antitrypsin and alpha 1-antichymotrypsin are in the lesions of Alzheimer’s disease. Neuroreport 3, 201–203. doi: 10.1097/00001756-199202000-00020
Gualtieri, T. C. (2002). Brain Injury and Mental Retardation: Psychopharmacology and Neuropsychiatry. (Philadelphia: Lippincott Williams and Wilkins), 464–465.
Gundersen, G. A. (2013). Roles of Aquaporin-4 in Brain Fluid Dynamics. Thesis for the degree of Philosophiae Doctor. University of Oslo, Norway: Centre for Molecular Medicine Norway University of Oslo. (ISBN 978-82-8264-090-9)
Han, Z., Li, L., Wang, L. L., Degos, V., Maze, M., and Su, H. (2014). Alpha-7 nicotinic acetylcholine receptor agonist treatment reduces neuroinflammation, oxidative stress and brain injury in mice with ischemic stroke and bone fracture. J. Neurochem. 131, 498–508. doi: 10.1111/jnc.12817
Harsan, L. A., Poulet, P., Guignard, B., Parizel, N., Skoff, R. P., and Ghandour, M. S. (2007). Astrocytic hypertrophy in dysmyelination influences the diffusion anisotropy of white matter. J. Neurosci. Res. 85, 935–944. doi: 10.1002/jnr.21201
Hshieh, T. T., Fong, T. G., Marcantonio, E. R., and Inouye, S. K. (2008). Cholinergic deficiency hypothesis in delirium: a synthesis of current evidence. J. Gerontol. A Biol. Sci. Med. Sci. 63, 764–772. doi: 10.1093/gerona/63.7.764
Hsiao, T. W., Swarup, V. P., Kuberan, B., Tresco, P. A., and Hlady, V. (2013). Astrocytes specifically remove surface-adsorbed fibrinogen and locally express chondroitin sulfate proteoglycans. Acta Biomater. 9, 7200–7208. doi: 10.1016/j.actbio.2013.02.047
Iliff, J. J., Wang, M., Liao, Y., Plogg, B. A., Peng, W., Gundersen, G. A., et al. (2012). A paravascular pathway facilitates CSF flow through the brain parenchyma and the clearance of interstitial solutes, including amyloid β. Sci. Transl. Med. 4:147ra111. doi: 10.1126/scitranslmed.3003748
Ito, J., Nagayasu, Y., Kato, K., Sato, R., and Yokoyama, S. (2002). Apolipoprotein A-I induces translocation of cholesterol, phospholipid and caveolin-1 to cytosol in rat astrocytes. J. Biol. Chem. 277, 7929–7935. doi: 10.1074/jbc.m103878200
Jang, E., Kim, J. H., Lee, S., Kim, J. H., Seo, J. W., Jin, M., et al. (2013). Phenotypic polarization of activated astrocytes: the critical role of lipocalin-2 in the classical inflammatory activation of astrocytes. J. Immunol. 15, 5204–5219. doi: 10.4049/jimmunol.1301637
Kox, M., Pompe, J. C., Peters, E., Vaneker, M., van der Laak, J. V., van der Hoeven, J. G., et al. (2011). α7 nicotinic acetylcholine receptor agonist GTS-21 attenuates ventilator-induced tumour necrosis factor-α production and lung injury. Br. J. Anaesth. 107, 559–566. doi: 10.1093/bja/aer202
Lakhan, S. E., and Kirchgessne, A. (2011). Anti-inflammatory effects of nicotine in obesity and ulcerative colitis. J. Transl. Med. 9:129. doi: 10.1186/1479-5876-9-129
Lampron, A., Elali, A., and Rivest, S. (2013). Innate immunity in the CNS: redefining the relationship between the cns and its environment. Neuron 78, 214–232. doi: 10.1016/j.neuron.2013.04.005
Lasagna-Reeves, C. A., and Kayed, R. (2011). Astrocytes contain amyloid-β annular protofibrils in Alzheimer’s disease brains. FEBS Lett. 585, 3052–3057. doi: 10.1016/j.febslet.2011.08.027
Lazar, M. (2010). Mapping brain anatomical connectivity using white matter tractography. NMR Biomed. 23, 821–835. doi: 10.1002/nbm.1579
Lazar, M., Weinstein, D. M., Tsuruda, J. S., Hasan, K. M., Arfanakis, K., Meyerand, M. E., et al. (2003). White matter tractography using diffusion tensor deflection. Hum. Brain Mapp. 18, 306–321. doi: 10.1002/hbm.10102
Lee, M. Y., Kim, S. Y., Choi, J. S., Lee, I. H., Choi, Y. S., Jin, J. Y., et al. (2002). Upregulation of haptoglobin in reactive astrocytes after transient forebrain ischemia in rats. J. Cereb. Blood Flow Metab. 22, 1176–1180. doi: 10.1097/00004647-200210000-00004
Malmsten, L., Vijayaraghavan, S., Hovatta, O., Marutle, A., and Darreh-Shori, T. (2014). Fibrillar β-amyloid 1–42 alters cytokine secretion, cholinergic signalling and neuronal differentiation. J. Cell. Mol. Med. 18, 1874–1888. doi: 10.1111/jcmm.12343
Maranto, J., Rappaport, J., and Datta, P. K. (2008). Regulation of complement component C3 in astrocytes by IL-1beta and morphine. J. Neuroimmune Pharmacol. 3, 43–51. doi: 10.1007/s11481-007-9096-9
McGrane, S., Girard, T. D., Thompson, J. L., Shintani, A. K., Woodworth, A., Ely, E. W., et al. (2011). Procalcitonin and C-reactive protein levels at admission as predictors of duration of acute brain dysfunction in critically ill patients. Crit. Care 15:R78. doi: 10.1186/cc10070
Meng, S., Qiao, M., Lin, L., Del Bigio, M. R., Tomanek, B., and Tuor, U. I. (2004). Correspondence of AQP4 expression and hypoxic-ischaemic brain oedema monitored by magnetic resonance imaging in the immature and juvenile rat. Eur. J. Neurosci. 19, 2261–2269. doi: 10.1111/j.0953-816x.2004.03315.x
Nagele, R. G., D’Andrea, M. R., Lee, H., Venkataraman, V., and Wang, H. Y. (2003). Astrocytes accumulate A beta 42 and give rise to astrocytic amyloid plaques in Alzheimer disease brains. Brain Res. 971, 197–209. doi: 10.1016/s0006-8993(03)02361-8
Nagelhus, E. A., and Ottersen, O. P. (2013). Physiological roles of Aquaporin-4 in brain. Physiol. Rev. 93, 1543–1562. doi: 10.1152/physrev.00011.2013
Norman, G. J., Morris, J. S., Karelina, K., Weil, Z. M., Zhang, N., Al-Abed, Y., et al. (2011). Cardiopulmonary arrest and resuscitation disrupts cholinergic anti-inflammatory processes: a role for cholinergic α7 nicotinic receptors. J. Neurosci. 31, 3446–3452. doi: 10.1523/JNEUROSCI.4558-10.2011
Ojeda, M. L., Ambrosiani, J., Tavares, E., Maldonado, R., and Miñano, F. J. (2006). Identification and localization of procalcitonin-like immunoreactivity in the rat hypothalamus. Neurosci. Lett. 408, 40–45. doi: 10.1016/j.neulet.2006.07.076
Paco, S., Pozas, E., and Aguado, F. (2010). Secretogranin III is an astrocyte granin that is overexpressed in reactive glia. Cereb. Cortex 20, 1386–1397. doi: 10.1093/cercor/bhp202
Pandharipande, P. P., Girard, T. D., Jackson, J. C., Morandi, J. A., Thompson, J. L., Pun, B. T., et al. (2013). Long-term cognitive im-pairment after critical illness. N. Engl. J. Med. 369, 1306–1316. doi: 10.1056/NEJMoa1301372
Pereira, A. Jr., and Furlan, A. (2010). Astrocytes and human cognition: modeling information integration and modulation of neuronal activity. Prog. Neurobiol. 92, 405–420. doi: 10.1016/j.pneurobio.2010.07.001
Perry, V. H., and Teeling, J. (2013). Microglia and macrophages of the central nervous system: the contribution of microglia priming and systemic inflammation to chronic neurodegeneration. Semin. Immunopathol. 35, 601–612. doi: 10.1007/s00281-013-0382-8
Pichler, L., Muchitsch, E. M., and Schwarz, H. P. (1999). Preclinical investigation of alpha 1-acid glycoprotein (orosomucoid). Wien. Klin. Wochenschr. 111, 192–198.
Poljak, A., Hill, M., Hall, R. J., MacLullich, A. M., Raftery, M. J., Tai, J., et al. (2014). Quantitative proteomics of delirium cerebrospinal fluid. Transl. Psychiatry 4:e477. doi: 10.1038/tp.2014.114
Qian, Z. M., To, Y., Tang, P. L., and Feng, Y. M. (1999). Transferrin receptors on the plasma membrane of cultured rat astrocytes. Exp. Brain Res. 129, 473–476. doi: 10.1007/s002210050916
Rodriguez, R., Kallenbach, U., Singer, W., and Munk, M. H. J. (2004). Short- and long-term effects of cholinergic modulation on gamma oscillations and response synchronization in the visual cortex. J. Neurosci. 24, 10369–10378. doi: 10.1523/jneurosci.1839-04.2004
Shaked, I., Meerson, A., Wolf, Y., Avni, R., Greenberg, D., Gilboa-Geffen, A., et al. (2009). MicroRNA-132 potentiates cholinergic anti-inflammatory signaling by targeting acetylcholinesterase. Immunity 31, 965–973. doi: 10.1016/j.immuni.2009.09.019
Sokolowski, J. D., and Mandell, J. W. (2011). Phagocytic clearance in neurodegeneration. Am. J. Pathol. 178, 1416–1428. doi: 10.1016/j.ajpath.2010.12.051
Spindel, E. R. (2012). Muscarinic receptor agonists and antagonists: effects on cancer. Handb. Exp. Pharmacol. 208, 451–468. doi: 10.1007/978-3-642-23274-9_19
Stieler, J. T., Lederer, C., BruÈckner, M. K., Wolf, H., Holzer, M., Gertz, H. J., et al. (2001). Impairment of mitogenic activation of peripheral blood lymphocytes in Alzheimer’s disease. Neuroreport 12, 3969–3972. doi: 10.1097/00001756-200112210-00023
Taber, K. H., and Hurley, R. A. (2014). Volume transmission in the brain: beyond the synapse. J. Neuropsychiatry Clin. Neurosci. 26, 1–4. doi: 10.1176/appi.neuropsych.13110351
Takata, N., Mishima, T., Hisatsune, C., Nagai, T., Ebisui, E., Mikoshiba, K., et al. (2011). Astrocyte calcium signaling transforms cholinergic modulation to cortical plasticity in vivo. J. Neurosci. 31, 18155–18165. doi: 10.1523/JNEUROSCI.5289-11.2011
Tateishi, N., Shimoda, T., Yada, N., Shinagawa, R., and Kagamiishi, Y. (2006). S100B: astrocyte specific protein. Nihon Shinkei Seishin Yakurigaku Zasshi 26, 11–16.
Thrane, A. S., Rangroo Thrane, V., and Nedergaard, M. (2014). Drowning stars: reassessing the role of astrocytes in brain edema. Trends Neurosci. 37, 620–628. doi: 10.1016/j.tins.2014.08.010
Tourdias, T., Dragonu, I., Fushimi, Y., Deloire, M. S., Boiziau, C., Brochet, B., et al. (2009). Aquaporin 4 correlates with apparent diffusion coefficient and hydrocephalus severity in the rat brain: a combined MRI-histological study. Neuroimage 47, 659–666. doi: 10.1016/j.neuroimage.2009.04.070
Tracey, K. J. (2009). Reflex control of immunity. Nat. Rev. Immunol. 9, 418–428. doi: 10.1038/nri2566
Tyagi, E., Agrawal, R., Nath, C., and Shukla, R. (2010). Inhibitory role of cholinergic system mediated via alpha7 nicotinic acetylcholine receptor in LPS-induced neuro-inflammation. Innate Immun. 16, 3–13. doi: 10.1177/1753425909104680
van Eijk, M. M., Roes, K. C., Honing, M. L., Kuiper, M. A., Karakus, A., van der Jagt, M., et al. (2010). Effect of rivastigmine as an adjunct to usual care with haloperidol on duration of delirium and mortality in critically ill patients: a multicentre, double-blind, placebo-controlled randomised trial. Lancet 376, 1829–1837. doi: 10.1016/S0140-6736(10)61855-7
Vijayaraghavan, S., Karami, A., Aeinehband, S., Behbahani, H., Grandien, A., Nilsson, B., et al. (2013). Regulated extracellular choline acetyltransferase activity—the Plausible missing link of the distant action of Acetylcholine in the cholinergic anti-inflammatory pathway. PLOS One 8:e65936. doi: 10.1371/journal.pone.0065936
Vizi, E. S., Fekete, A., Karoly, R., and Mike, A. (2010). Non-synaptic receptors and transporters involved in brain functions and targets of drug treatment. Br. J. Pharmacol. 160, 785–809. doi: 10.1111/j.1476-5381.2009.00624.x
Witlox, J., Eurelings, L. S., de Jonghe, J. F., Kalisvaart, K. J., Eikelenboom, P., and van Gool, W. A. (2010). Delirium in elderly patients and the risk of postdischarge mortality, institutionalization and dementia: a meta-analysis. JAMA 304, 443–451. doi: 10.1001/jama.2010.1013
Xie, L., Kang, H., Xu, Q., Chen, M. J., Liao, Y., Thiyagarajan, M., et al. (2013). Sleep drives metabolite clearance from the adult brain. Science 342, 373–377. doi: 10.1126/science.1241224
Yang, L., Kress, B. T., Weber, H. J., Thiyagarajan, M., Wang, B., Deane, R., et al. (2013). Evaluating glymphatic pathway function utilizing clinically relevant intrathecal infusion of CSF tracer. J. Transl. Med. 11:107. doi: 10.1186/1479-5876-11-107
Yin, K. J., Cirrito, J. R., Yan, P., Hu, X., Xiao, Q., Pan, X., et al. (2006). Matrix metalloproteinases expressed by astrocytes mediate extracellular amyloid-beta peptide catabolism. J. Neurosci. 26, 10939–10948. doi: 10.1523/jneurosci.2085-06.2006
Yu, W., Mechawar, N., Krantic, S., Chabot, J. G., and Quirion, R. (2012). Upregulation of astrocytic α7 nicotinic receptors in Alzheimer’s disease brain- possible relevant to amyloid pathology. Mol. Neurodegener. 7(Suppl. 1):O7. doi: 10.1186/1750-1326-7-s1-o7
Zhan, X., Ander, B. P., Jickling, G., Turner, R., Stamova, B., Xu, H., et al. (2010). Brief focal cerebral ischemia that simulates transient ischemic attacks in humans regulates gene expression in rat peripheral blood. J. Cereb. Blood Flow Metab. 30, 110–118. doi: 10.1038/jcbfm.2009.189
Keywords: immunity, acethylcholine, volume transmission, AQP-4, extracellular space
Citation: Sfera A, Osorio C, Price AI, Gradini R and Cummings M (2015) Delirium from the gliocentric perspective. Front. Cell. Neurosci. 9:171. doi: 10.3389/fncel.2015.00171
Received: 12 February 2015; Accepted: 17 April 2015;
Published online: 11 May 2015.
Edited by:
Gilles J. Guillemin, Macquarie University, AustraliaReviewed by:
Hermona Soreq, The Hebrew University of Jerusalem, IsraelRobert Weissert, University of Regensburg, Germany
Copyright © 2015 Sfera, Osorio, Price, Gradini and Cummings. This is an open-access article distributed under the terms of the Creative Commons Attribution License (CC BY). The use, distribution and reproduction in other forums is permitted, provided the original author(s) or licensor are credited and that the original publication in this journal is cited, in accordance with accepted academic practice. No use, distribution or reproduction is permitted which does not comply with these terms.
*Correspondence: Adonis Sfera, Psychiatry, Patton State Hospital, 3102 E Highland Ave, Patton, 92369, CA, USA, dr.sfera@gmail.com