Pregabalin as a Pain Therapeutic: Beyond Calcium Channels
- 1Michael Smith Laboratories, University of British Columbia, Vancouver, BC, Canada
- 2Djavad Mowafaghian Center for Brain Health, University of British Columbia, Vancouver, BC, Canada
- 3Department of Anesthesiology and Critical Care Medicine, University of New Mexico School of Medicine, Albuquerque, NM, United States
Initially developed to generate new treatments for epilepsy, gabapentin, and pregabalin (“gabapentinoids”) were engineered to mimic the action of GABA and to modulate GABA metabolism. Rather than their intended pharmacological action on GABA neurotransmission, instead, they exhibit a high affinity for the α2δ-1 and α2δ-2 subunits of voltage-activated calcium channels, wherein binding of gabapentinoids inhibits cellular calcium influx and attenuates neurotransmission. Despite a lack of activity on GABA levels, gabapentin and pregabalin are effective at suppressing seizures and subsequently approved as a new class of antiepileptic therapy for partial-onset epilepsy. Through the same hypothesized molecular mechanism and by controlling neuronal hyperexcitability, gabapentinoids demonstrate clear efficacy in pain management, which has arguably been their most extensively prescribed application to date. In this review, we focus on pregabalin as a second-generation gabapentinoid widely employed in the treatment of a variety of pain conditions. We also discuss the wider functional roles of α2δ subunits and the contributions that pregabalin might play in affecting physiological and pathophysiological processes.
Pharmacology and Protein Interactions of Pregabalin
Pregabalin Is a High-Affinity α2δ Subunit Ligand
The alpha-2-delta (α2δ) subunits were first described as auxiliary subunits of the high voltage-activated calcium channels and are encoded by four genes: α2δ1, α2δ-2, α2δ-3 and α2δ-4. α2δ-1-3 are expressed in neurons as well as heart and skeletal muscle tissue (Gong et al., 2001), whereas α2δ–4 is found in non-neuronal cell types such as the retina and endocrine tissues (Dolphin, 2013). Pregabalin selectively binds to the α2δ-1 and α2δ-2 subunits and despite its original design as a GABA mimetic, pregabalin does not affect GABAA or GABAB receptor activity (Li et al., 2011).
Interestingly, α2δ-1 expression appears to co-localize mainly with excitatory neurons whereas α2δ-2 is found largely in inhibitory neurons (Cole et al., 2005). Recently, it has been shown that gabapentin exerts differential effects on putative excitatory and inhibitory spinal dorsal horn neurons (Alles et al., 2017). It is thus possible that pregabalin has different effects on excitatory compared to inhibitory neurons through a mechanism involving α2δ-1 and α2δ-2 subunits. This distinction may represent an important pregabalin-mediated therapeutic action since excitation-inhibition imbalance occurs in the spinal cord and brain in chronic pain states such as diabetic neuropathy and chronic pain accompanying multiple sclerosis (Coull et al., 2005; Petrou et al., 2012; Kuner, 2015; Potter et al., 2016).
Most pain studies addressing the pregabalin mechanism of action have focussed on α2δ-1 binding. α2δ-1 itself has been implicated in neuropathic pain and it is upregulated in the dorsal root ganglia (DRG) and primary afferent nerve terminals following nerve injury in rodent models (Luo et al., 2001). Mice overexpressing α2δ-1 show behavioral hypersensitivity to pain (Nguyen et al., 2009) and further, pregabalin analgesic efficacy is lost in transgenic mice carrying a α2δ-1 R217A mutation in the gabapentinoid binding site (Field et al., 2006). The α2δ-1 R217A mutation results in a significant decrease in calcium current through Cav2.2 (N-type) channels, possibly due to a change in structural interaction with the pore-forming α1 subunit; however, this mutation does not appear to alter α2δ-1 function in vivo (Field et al., 2006).
α2δ-1 expression is enriched in the spinal dorsal horn, periaqueductal gray (PAG), anterior cingulate cortex (ACC), insula and amygdala (Stahl et al., 2013). The ACC and PAG are strongly implicated in nociceptive (dorsal horn) and emotional aspects of pain processing (Peirs and Seal, 2016) and pregabalin efficacy as a pain drug is most likely in part due to its action on α2δ-1 in these areas. In support, pregabalin reduces the release of glutamate in the spinal dorsal horn in rodent pain models (Kumar et al., 2013) and also reduces activation of the insula and amygdala during emotional processing that plays a role in the experience of pain (Aupperle et al., 2011).
Distinct from high voltage-activated calcium channel interactions, it has been demonstrated that astrocyte-secreted factors called thrombospondins (TSPs) are endogenous ligands of α2δ-1. The TSP-α2δ-1 complex is thought to form a “synaptogenic signaling complex” (Eroglu et al., 2009). In terms of neuropathic pain pathogenesis, it has been hypothesized that when α2δ-1 is upregulated, there is increased excitatory synaptogenesis driving increased neuronal network excitability. This mechanism is supported by earlier studies demonstrating that “central sensitization” of pain circuitry, a form of pathological learning, underlies neuropathic pain (Woolf, 1983; Costigan et al., 2009).
Several other recent findings have shed light on novel functions of α2δ-1 in the context of inflammatory and neuropathic pain (see Figure 1). For example, it has been shown that α2δ-1 interacts with the N terminus region of BK potassium channels (Zhang et al., 2018). Co-expression of BK channels with Cav2 channels reduces cell surface expression and whole-cell calcium current density due to competition for α2δ-1 for binding. Reproducing this situation in vivo results in analgesia in both inflammatory and neuropathic pain models. Pregabalin may enhance an interaction between endogenous BK and Cav2 channels through its α2δ-1-binding site. Alternatively, pregabalin might sequester free α2δ-1 and prevent it from associating with Cav2 channels before BK channels. Another study demonstrated that α2δ-1 also associates with NMDA receptors to form complexes and that the physical interaction promotes synaptic expression of α2δ-1-NMDA receptor complexes (Chen et al., 2018). Notably, gabapentin targets these complexes to alleviate neuropathic pain. Although pregabalin was not tested in this study, it would likely operate by a similar mechanism in this context. Given the broad functions and roles of the NMDA receptor, this mechanism might help to explain how the gabapentinoids successfully reduce cellular hyperexcitability in various systems and disease states (Paoletti et al., 2013).
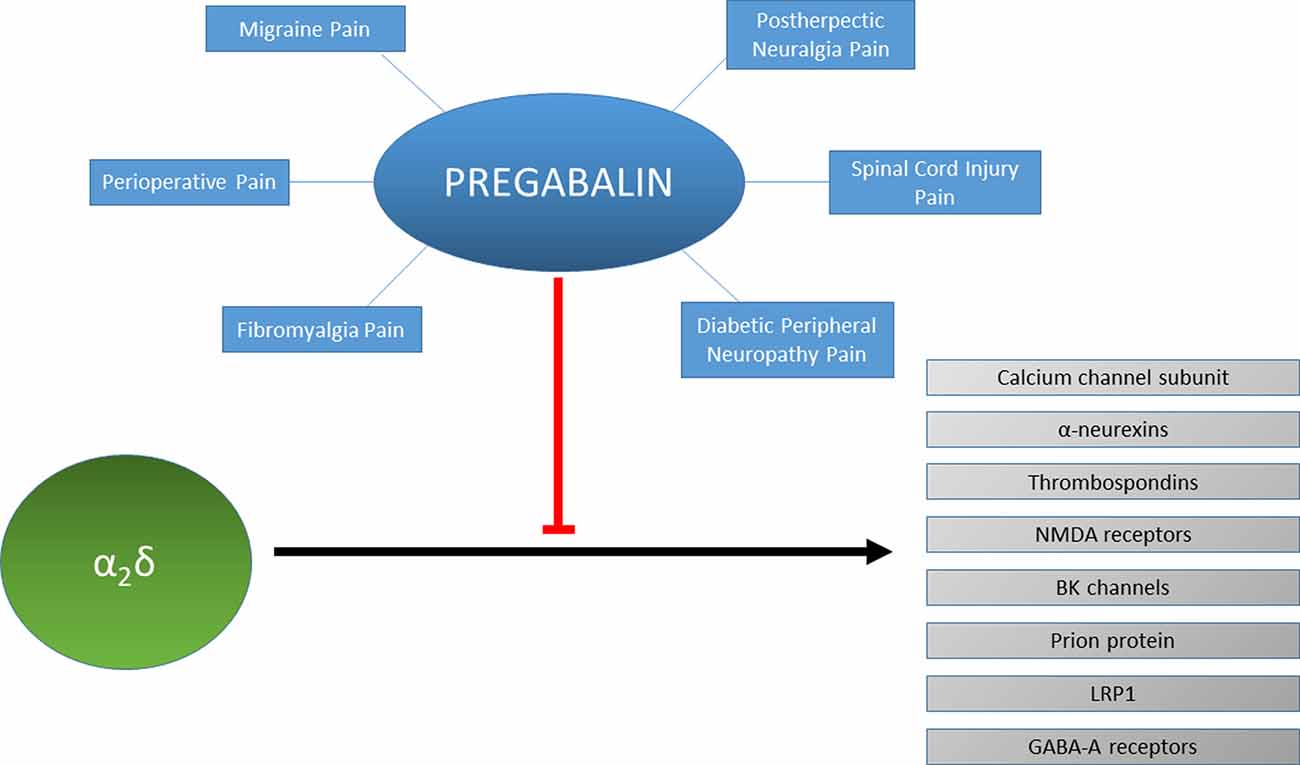
Figure 1. Protein interactions of α2δ and their inhibition by pregabalin. Pregabalin is used to treat different types of chronic pain such as migraine, spinal cord injury pain or fibromyalgia. The effectiveness of pregabalin for treating each type of chronic pain for each individual patient may be reflected by the modulation of the protein interactions of α2δ such as with neurexins, thrombospondins, NMDA receptors, BK channels, prion proteins, LRP1 or GABA-A receptors.
It has been shown that synaptic cell-adhesion molecules α-neurexins regulate Ca2+ influx through Cav2.1 channels with α2δ-1 (Brockhaus et al., 2018). In cultured hippocampal neurons alpha-neurexins with α2δ-1, but not α2δ-3, facilitated calcium currents of Cav2.1. Therefore, in disorders involving perturbation of the Cav2.1 channel function, pregabalin may help correct α2δ-1-α-neurexin interactions.
Associations between α2δ and other proteins have been demonstrated including prion proteins (Alvarez-Laviada et al., 2014), GABA-A receptors (Geisler et al., 2019) and an important N-type calcium channel trafficking-related protein called low-density lipoprotein (LDL) receptor-related protein-1 (LRP1; Kadurin et al., 2017). LRP1 binding to α2δ-1 has been suggested to involve the binding pocket for gabapentin, therefore LRP-1 may occlude the binding site for pregabalin as well. With regards to a potential role of LRP-1 in pain pathophysiology, one study has shown that the perisciatic application of LRP-1 agonists facilitated an increase in nociceptive thresholds by local application of opioids (Yang et al., 2016). However, no studies of LRP-1 in chronic pain patients have been reported to date.
Acute vs. Chronic Actions of Pregabalin: Comparing Clinical and Basic Science Findings
Clinically relevant concentrations of gabapentin have been calculated from plasma levels in neuropathic pain patients at approximately 15–30 mg/L (88–175 μM; Juenke et al., 2011). Generally, pregabalin displays higher potency than gabapentin due to a higher affinity for α2δ-1 (Field et al., 2006). In humans, the maximum recommended single oral dose of pregabalin is 300 mg, which reaches a maximum plasma concentration of roughly 40 μM within 1 h and a maximum CSF concentration of 2.6 μM within 8 h (Buvanendran et al., 2010). Steady-state levels of pregabalin after repeated administration of 600 mg daily vary between 17–51 μM in plasma (Bockbrader et al., 2010a) and are ~1%–30% lower in CSF (Bockbrader et al., 2010b).
In animals, pregabalin exerts acute actions on calcium channel-mediated currents recorded from neuromuscular junctions and Calyx of Held excitatory synapses (Uchitel et al., 2010). However, other studies have suggested that pregabalin actions are mainly chronic through reducing calcium channel trafficking from DRG to primary afferent terminals synapsing in the spinal dorsal horn (Bauer et al., 2009, 2010; Hendrich et al., 2012). It is not clear whether acute or chronic actions may predominate in a clinical setting since some patients only report benefit from pregabalin after several days (consistent with a chronic mode of action; Sharma et al., 2010), whereas some patients derive benefit with pregabalin when provided perioperatively (consistent with an acute mode of action; Schmidt et al., 2013). Further studies that attempt to correlate basic science with clinical findings are required to hone in on a consensus for the mechanism(s) of action of the gabapentinoids.
The general mechanism of pregabalin physiological cellular action involves a decrease in the release of several types of neurotransmitters including glutamate, noradrenaline, and substance P (Fink et al., 2000; Dooley et al., 2007; Brawek et al., 2008). In one study pregabalin inhibited K+-evoked acetylcholine, noradrenaline and serotonin release without affecting dopamine release in human neocortex (Brawek et al., 2008). There is little else to suggest that pregabalin is selective for a specific neurotransmitter type. Experiments on rodent neocortical slices have examined the effects of gabapentin on norepinephrine release evoked by a high K+ challenge in the presence of different voltage-gated calcium channel blockers (Dooley et al., 2002). This study showed that gabapentin appeared to show a preference for action on P/Q-type channels. Several studies other show that the gabapentinoids also reduce neurotransmitter release in the spinal dorsal horn wherein N-type channels are the most predominant high voltage-activated subtype (Coderre et al., 2005; Kumar et al., 2013; Alles et al., 2017). These differences likely speak to the complexity of both gabapentinoid actions and the distinct roles of specific calcium channel subtypes in controlling release, although are generally consistent with the notion that the main therapeutic action of these drugs involves reducing calcium-mediated neurotransmitter release to reduce neuronal excitability.
Conversely, Baba et al. (2016) demonstrated that clinically relevant concentrations of bath applied pregabalin have no acute inhibitory effects on evoked intracellular Ca2+ responses of dorsal horn neurons under naïve or neuropathic conditions. This is in contrast to previous studies showing that pregabalin can reduce the release of glutamate in the dorsal horn following systemic or intrathecal injection in both naïve and neuropathic conditions (Coderre et al., 2005; Kumar et al., 2013). These discrepancies may be due to differences in the method of delivery of pregabalin and/or in the assays employed for determining pregabalin effects. For example, calcium responses are an indirect indicator of neurotransmitter release and the stimulation paradigm for evoking calcium responses may not be physiologically relevant. Also, there are time course and concentration issues to consider when comparing bath applied with systemically injected pregabalin.
Functional MRI studies show that pregabalin decreases activation of the insula and amygdala during emotional processing (Aupperle et al., 2011). Paradoxically, in the same study pregabalin produced an increase in ACC activation, which might be predicted to exacerbate pain sensation. The authors argue that this could be a top-down effect on modulation of emotional processing.
The α2δ subunits in part contribute to setting presynaptic calcium channel abundance and release probability in central synapses (Hoppa et al., 2012). Pregabalin may exert its effects via a mechanism that reduces release probability, which would serve to dampen excitability in neuronal circuits. A recent article has demonstrated that gabapentin can promote regeneration following spinal cord injury via a blockade of α2δ-2, which inhibits axon growth (Sun et al., 2020). It has also been demonstrated that presynaptic α2δ-2 controls postsynaptic clustering of GABAA receptors and that when this process is disrupted may cause excitation-inhibition imbalance as observed in chronic pain states and neuropsychiatric diseases (Geisler et al., 2019). As such, pregabalin therapeutic effects may in part be due to its effect of α2δ-2 subunits, serving to promote repair, aberrant axonal wiring, and shifts in excitation-inhibition imbalance.
There is more work to be done on improving understanding of the role of α2δ subunit subtypes in basic physiology and disease states (for a review on emerging neuronal functions and post-translational processing of α2δ subunits, see Geisler et al., 2015; Dolphin, 2018).
On-Label Uses for Pain
Pregabalin is approved by the FDA for use to treat peripheral neuropathic pain, generalized anxiety disorder, and as an adjunct therapy for epilepsy (Dworkin and Kirkpatrick, 2005; Bagal et al., 2013). Although the gabapentinoids are considered first-line treatment options for neuropathic pain, the number needed to treat (NNT) for 50% pain relief in patients is 7.7 for pregabalin and 7.2 for gabapentin (Finnerup et al., 2015). In part, the high NNT likely reflects the complexity and many distinct types of chronic pain together with the high variability in pain phenotypes and severity across patients.
The most common causes of chronic pain are fibromyalgia, diabetic peripheral neuropathy, postherpetic neuralgia (nerve damage following shingles), and neuropathic pain associated with spinal cord injury (Dworkin and Kirkpatrick, 2005). The term neuropathic pain is applied to any acute or chronic pain syndrome thought to result from aberrant central or peripheral somatosensory pain processing (Portenoy and Cruciani, 2009). Common symptoms of neuropathic pain sensation include a sensation of burning or electric shock, hyperalgesia, hyperpathia, dysesthesia, allodynia, and paresthesia. Pregabalin is an effective treatment for neuropathic pain irrespective of the length of time the patient has displayed symptoms (Pérez et al., 2017). For this review we discuss the use of pregabalin for treating specific types of chronic pain, however we recognize that pain phenotypes exist trans-etiologically and that classifying patients according to disease is not necessarily predictive of drug response.
Pregabalin in Fibromyalgia
Fibromyalgia is a form of idiopathic or “nociplastic” pain that causes widespread musculoskeletal pain for which no alternative cause can be identified, presenting as diffuse hyperalgesia and/or allodynia and is the most common cause of widespread body pain in humans (Derry et al., 2016; Sluka and Clauw, 2016). It is more prevalent in women and prevalence increases with age, with up to approximately 4% of women and 0.01% of men reported in the general population (Heidari et al., 2017). Fibromyalgia in a number of peripheral pain conditions is predicted to occur as a result of enhanced nociceptive signaling and the development of CNS-mediated symptoms related to sleep/fatigue, mood and memory deficits (Sluka and Clauw, 2016). Secondary fibromyalgia can also occur, when comorbid with a disease-causing constant nociceptor activation (Clauw, 2014).
Current therapeutic interventions for fibromyalgia include both non-pharmacological and pharmacological approaches, often integrated for best effect (Clauw, 2014). Drug therapies cover a wide range of pharmacological approaches and include opioids, cannabinoids, tricyclic antidepressants, selective serotonin reuptake inhibitors (SSRIs) and gabapentinoids (Fitzcharles et al., 2013). Pregabalin is recommended for fibromyalgia at a dose starting at 25 mg to 50 mg per day increasing to a maximum dose of 300 mg to 450 mg per day (Boomershine, 2010). A recent review examining clinical data from multiple studies found a significant reduction in moderate to severe pain from fibromyalgia at doses of 300 mg to 600 mg pregabalin compared to placebo (Derry et al., 2016). The authors also noted adverse reactions in approximately 10% of individuals treated with pregabalin compared to placebo.
Mechanistically, pregabalin is predicted to reduce fibromyalgia pain by preventing sensory propagation of nociception due to inhibition of calcium channels and neurotransmitter release in the ascending pain pathway (Biggs et al., 2014). Also, pregabalin has been shown to directly reduce glutamate and glutamine levels in the posterior insula in fibromyalgia patients, also interfering with its functional connectivity to the default mode network (DFN; Harris et al., 2013). This finding is of particular interest given that the DFN, which is a group of brain regions normally involved in self-referential thinking and autobiographical memory, shows increased activity in patients with fibromyalgia (Buckner et al., 2008; Napadow et al., 2010). It is uncertain whether enhanced basal activity in the DFN is a causative factor of neuropathy in fibromyalgia, or simply that increased sensory input increases basal activity in the DFN.
Studies of fibromyalgia patients using occipital nerve stimulation (ONS) show disturbed conditioned pain modulation (Ahmed et al., 2018). Pregabalin has been shown to exert effects on descending pain circuitry in nerve-injured mice (Bee and Dickenson, 2008), therefore these central effects of pregabalin may contribute to its effectiveness for treating fibromyalgia.
Pregabalin in Diabetic Peripheral Neuropathy
Diabetic peripheral neuropathy is the most common complication in Type II diabetes mellitus, resulting from nerve damage caused by chronically high blood sugar levels (Çakici et al., 2016). Nerve damage is predicted to occur via microvascular injury of blood vessels supplying nerves. Symptoms usually appear first in the lower extremities (feet and legs) and can appear later in the upper extremities, manifesting as numbness, tingling, burning sensations, sharp pain and loss of balance (Çakici et al., 2016). Prevalence can occur at up to 29% in populations with Type II diabetes (Davies et al., 2006; Bansal et al., 2014) and is more common in non-Caucasians and women (Marmiroli and Cavaletti, 2016).
Similar to fibromyalgia, tricyclic antidepressants and opioids are commonly used to treat the symptoms of diabetic peripheral neuropathy (Javed et al., 2015; Tajti et al., 2016) and a capsaicin patch has been shown to display similar efficacy to oral drugs, albeit with limits in tolerability (van Nooten et al., 2017). Both gabapentin and pregabalin are also effective treatments, with pregabalin recommended at a dose of 300 mg to 600 mg daily resulting in a significant reduction in pain scores (Juhn et al., 2015).
Pregabalin suppresses the activity of excitatory primary afferent fibers that carry nociceptive information to the spinal dorsal horn (Biggs et al., 2014). However, at clinically relevant concentrations acute pregabalin does not affect calcium entry in dorsal horn A- and C- fibers induced by dorsal root ganglion stimulation in either normal rats or a rat model of streptozotocin-induced painful diabetic peripheral neuropathy (Baba et al., 2016). This is in contrast to gabapentin that has been shown to inhibit synaptic transmission from DRG to the dorsal spinal cord in the same pain model (Patel et al., 2000). However, it takes several days for pregabalin to induce anti-nociceptive effects in patients with diabetic peripheral neuropathy and, therefore it seems likely that a chronic mechanism of action involving the downregulation of pro-nociceptive proteins and/or synaptic plasticity may explain its effects (Freeman et al., 2008; Bauer et al., 2010).
Postherpetic (Herpes Zoster) Neuralgia
The varicellar zoster virus causes chickenpox, which when reactivated from its latent state up to decades later results in herpes zoster, a condition manifesting as a painful rash, also known as shingles (Fashner and Bell, 2011). While the virus can be treated with acyclovir and acyclovir-generic antivirals postherpetic neuralgia is a painful sensation that can persist for months or even years following an attack of herpes zoster without a continuing rash (Mallick-Searle et al., 2016). A high proportion (>90%) of adults show serology indicative of prior herpes zoster infection (Kilgore et al., 2003) and up to 20% of those suffering an initial infection go on to develop postherpetic neuralgia (Klompas et al., 2011). Nerve fibers may be damaged due to the inflammatory response accompanying the reactivation of the varicellar zoster virus (Woolf and Mannion, 1999; Mallick-Searle et al., 2016). The resulting pain can manifest as constant or intermittent without a stimulus, or as hyperalgesia.
Like other neuropathic pain disorders, tricyclic antidepressants are commonly used to address pain transmission, or basic topical analgesics, such as lidocaine can be used to suppress nociception in postherpetic neuralgia. The gabapentinoids are also first choice drugs, with pregabalin displaying similar efficacy when postherpetic neuralgia patients were switched from gabapentin to pregabalin at one-sixth of the dose (Ifuku et al., 2011). Pregabalin is recommended at 150 mg to 600 mg daily and consistently improves pain scores in patients with postherpetic neuralgia (Frampton and Foster, 2005). Of note, pregabalin combined with topical lidocaine (5% patch), resulted in significant pain reduction in patients who previously did not respond to either drug (Nalamachu and Morley-Forster, 2012).
Neuropathic Pain Associated With Spinal Cord Injury
Following an injury to the spinal cord, motor and sensory deficits occur, occasionally leading to paralysis. These often occur concurrently with nociceptive or neuropathic pain reported in approximately two-thirds of patients (Hagen and Rekand, 2015). The source of neuropathic pain can occur at, above or below the spinal cord injury, arising from damage to nerve roots, the site of spinal cord injury itself or pathophysiological alterations to neurons in the pain transduction pathway (Hagen and Rekand, 2015). Pregabalin (150–600 mg/day) is effective at treating patients with neuropathic pain associated with spinal cord injury, also improving sleep, anxiety and general patient status (Siddall et al., 2006; Cardenas et al., 2013). With regards to possible mechanism of action, it has recently been shown that gabapentinoids promote sprouting and regeneration of corticospinal axons in mice following spinal cord injury (Sun et al., 2020). Pregabalin also enhances myelin repair and attenuates glial activation in a demyelination model of rat optic chiasm, which may also contribute to its effectiveness in treating damage caused by spinal cord injury (Daneshdoust et al., 2017).
Off-Label Uses of Pregabalin
Aside from neuropathic pain, pregabalin is frequently prescribed for off-label use at the discretion of the attending physician.
Migraine Headache
Migraine is a debilitating painful condition described as a recurrent headache, often accompanied by visual disturbances and nausea (Burstein et al., 2015). The condition can occur accompanied by a genetic component but also spontaneously and the underlying mechanisms are still controversial (Ferrari et al., 2015). Spreading depolarization (SD) is a pathophysiological neurological phenomenon proposed to underlie migraine aura, yet it is not always observed in patients (Lauritzen et al., 2011). This may suggest that SD does not occur in all migraines, or perhaps that cortical electrodes are insufficient to detect SD-mediated voltage shifts that occur in isolation subcortically. SD is a multifactorial cascade, which can be prevented by blockade of synaptic transmission, but is also critically dependent on diffusion of extracellular neuroactive factors that are released by affected neurons during prolonged depolarization (Pietrobon and Moskowitz, 2014). As a result, the pharmacological prevention of SD is problematic without also severely affecting normal neurotransmission. Still, triptans and ergot derivatives act at serotonin receptors and are commonly used as abortive therapies in migraine via predicted vasoconstriction of blood vessels (Goadsby et al., 2002).
Interestingly, onabotulinumtoxin A is effective as a therapy for the treatment of chronic migraine, which is characterized by a headache frequency of 15 episodes per month or more (Frampton, 2012; Lipton et al., 2016). The mechanism of action of onabotulinumtoxin A is to inhibit excitatory neurotransmitter release by blocking synaptic vesicle fusion to the plasma membrane (Durham and Cady, 2011). Pregabalin also attenuates neurotransmitter release, albeit by a different mechanism than onabotulinumtoxin A, therefore there is a strong argument for its use as a potential chronic migraine treatment.
Although initial studies indicated that gabapentin may be effective in the treatment of migraines, it has since been shown to be only marginally effective (Mathew et al., 2001; Hoffmann et al., 2010; Linde et al., 2013; Zain et al., 2013; Mulleners et al., 2015). Conversely, pregabalin has shown encouraging early, clinical results in the treatment for migraine headaches (Calandre et al., 2010; Pizzolato et al., 2011; Bakhshandeh Bali et al., 2015) and in mouse models effectively suppresses SD velocity and propagation to subcortical brain structures following an acute dose (Cain et al., 2017). While promising findings, further clinical evidence is required to establish a definitive role for pregabalin in migraine therapy.
Pre-operative Administration for Post-operative Pain
Human studies have demonstrated a clear anti-nociceptive effect of pregabalin when administered as an acute dose before surgery. A single dose (75–150 mg) has demonstrated significant efficacy in reducing post-operative pain in for example orthopedic surgery (Akhavanakbari et al., 2013; Dong et al., 2016), lumbar dissectomy (Spreng et al., 2011), septoplasty (Sagit et al., 2013), thyroidectomy (Bindu et al., 2015), and hysterectomy (Rajappa et al., 2016). These clinical findings provide strong support for the acute mechanism of pregabalin efficacy. However, variables such as the degree of surgery invasiveness, length of post-operative follow-ups and low statistical power often make these studies difficult to evaluate by meta-analysis.
Visceral pain is an important clinical problem such as that associated with irritable bowel syndrome (Camilleri and Boeckxstaens, 2017). Pregabalin has been shown to reduce visceral hypersensitivity in a mouse model of opioid-induced hypersensitivity (Bannister et al., 2011), therefore may prove an effective treatment for visceral pain.
Future Perspectives
The gabapentinoids constitute a distinct pharmacological class of pain therapeutic with a well-defined molecular target yet a still poorly understood physiological mechanism of action. There remain many unanswered questions. For example, whether pregabalin acts as an allosteric modulator of TSPs via α2δ-1 or has a direct inhibitory effect on TSPs. Also to be better defined are the exact physiological and pathophysiological contributions of pregabalin binding to α2δ-2 subunits in central and peripheral pain pathways. Controversies also remain regarding the role of pregabalin as an acute vs. chronic therapeutic in terms of distinct or overlapping mechanisms correlating with clinical efficacy. Also, while a large body of data has defined the effects of pregabalin in DRGs and the spinal cord, the actions of pregabalin in the brain are less well understood.
The effectiveness of pregabalin in the clinic varies widely with some chronic pain patients deriving benefits more quickly than others and in response to much lower doses (Sharma et al., 2010). Studies that attempt to correlate these inter-patient differences with individual genetic, epigenetic and/or phenotypic characteristics are urgently required. This may lead to the development of more effective pain therapeutics as well as a deeper understanding of pain neurobiology.
Off-label uses for pregabalin are numerous while FDA approved uses for pregabalin remain limited to a few conditions raising the question of whether FDA approval will be sought for use in emerging disorders or whether off-label use will become increasingly common.
Newer gabapentinoids have been developed with mirogabalin undergoing clinical trials for neuropathic pain (Calandre et al., 2016). Whether the clinical efficacy of this new derivative will differ sufficiently from gabapentin and pregabalin remains to be determined.
Author Contributions
All authors contributed to all aspects of the review including literature review and manuscript writing and revision.
Funding
This work was supported by a grant from the Canadian Institutes of Health Research (#10677) and the Canada Research Chair in Biotechnology and Genomics-Neurobiology (to TS). This work was also supported by funds from the Department of Anesthesiology and Critical Care Medicine, University of New Mexico School of Medicine (to SA).
Conflict of Interest
The authors declare that the research was conducted in the absence of any commercial or financial relationships that could be construed as a potential conflict of interest.
References
Ahmed, S., Plazier, M., Ost, J., Stassijns, G., Deleye, S., Ceyssens, S., et al. (2018). The effect of occipital nerve field stimulation on the descending pain pathway in patients with fibromyalgia: a water PET and EEG imaging study. BMC Neurol. 18:191. doi: 10.1186/s12883-018-1190-5
Akhavanakbari, G., Entezariasl, M., Isazadehfar, K., and Mirzarahimi, T. (2013). The effects of oral pregabalin on post-operative pain of lower limb orthopedic surgery: a double-blind, placebo-controlled trial. Perspect. Clin. Res. 4, 165–168. doi: 10.4103/2229-3485.115376
Alles, S. R. A., Bandet, M. V., Eppler, K., Noh, M.-C., Winship, I. R., Baker, G., et al. (2017). Acute anti-allodynic action of gabapentin in dorsal horn and primary somatosensory cortex: correlation of behavioural and physiological data. Neuropharmacology 113, 576–590. doi: 10.1016/j.neuropharm.2016.11.011
Alvarez-Laviada, A., Kadurin, I., Senatore, A., Chiesa, R., and Dolphin, A. C. (2014). The inhibition of functional expression of calcium channels by prion protein demonstrates competition with α2δ for GPI-anchoring pathways. Biochem. J. 458, 365–374. doi: 10.1042/bj20131405
Aupperle, R. L., Ravindran, L., Tankersley, D., Flagan, T., Stein, N. R., Simmons, A. N., et al. (2011). Pregabalin influences insula and amygdala activation during anticipation of emotional images. Neuropsychopharmacology 36, 1466–1477. doi: 10.1038/npp.2011.32
Baba, H., Petrenko, A. B., and Fujiwara, N. (2016). Clinically relevant concentration of pregabalin has no acute inhibitory effect on excitation of dorsal horn neurons under normal or neuropathic pain conditions: an intracellular calcium-imaging study in spinal cord slices from adult rats. Brain Res. 1648, 445–458. doi: 10.1016/j.brainres.2016.08.018
Bagal, S. K., Brown, A. D., Cox, P. J., Omoto, K., Owen, R. M., Pryde, D. C., et al. (2013). Ion channels as therapeutic targets: a drug discovery perspective. J. Med. Chem. 56, 593–624. doi: 10.1021/jm3011433
Bakhshandeh Bali, M., Rahbarimanesh, A. A., Sadeghi, M., Sedighi, M., Karimzadeh, P., and Ghofrani, M. (2015). Comparison of propranolol and pregabalin for prophylaxis of childhood migraine: a randomised controlled trial. Acta Med. Iran 53, 276–280.
Bannister, K., Sikandar, S., Bauer, C. S., Dolphin, A. C., Porreca, F., and Dickenson, A. H. (2011). Pregabalin suppresses spinal neuronal hyperexcitability and visceral hypersensitivity in the absence of peripheral pathophysiology. Anesthesiology 115, 144–152. doi: 10.1097/aln.0b013e31821f6545
Bansal, D., Gudala, K., Muthyala, H., Esam, H. P., Nayakallu, R., and Bhansali, A. (2014). Prevalence and risk factors of development of peripheral diabetic neuropathy in type 2 diabetes mellitus in a tertiary care setting. J. Diabetes Investig. 5, 714–721. doi: 10.1111/jdi.12223
Bauer, C. S., Nieto-Rostro, M., Rahman, W., Tran-Van-Minh, A., Ferron, L., Douglas, L., et al. (2009). The increased trafficking of the calcium channel subunit α2δ-1 to presynaptic terminals in neuropathic pain is inhibited by the α2δ ligand pregabalin. J. Neurosci. 29, 4076–4088. doi: 10.1523/JNEUROSCI.0356-09.2009
Bauer, C. S., Rahman, W., Tran-van-Minh, A., Lujan, R., Dickenson, A. H., and Dolphin, A. C. (2010). The anti-allodynic α2δ ligand pregabalin inhibits the trafficking of the calcium channel α2δ-1 subunit to presynaptic terminals in vivo. Biochem. Soc. Trans. 38, 525–528. doi: 10.1042/bst0380525
Bee, L. A., and Dickenson, A. H. (2008). Descending facilitation from the brainstem determines behavioural and neuronal hypersensitivity following nerve injury and efficacy of pregabalin. Pain 140, 209–223. doi: 10.1016/j.pain.2008.08.008
Biggs, J. E., Boakye, P. A., Ganesan, N., Stemkowski, P. L., Lantero, A., Ballanyi, K., et al. (2014). Analysis of the long-term actions of gabapentin and pregabalin in dorsal root ganglia and substantia gelatinosa. J. Neurophysiol. 112, 2398–2412. doi: 10.1152/jn.00168.2014
Bindu, M., Kumar, A. A., Kesavan, M., and Suresh, V. (2015). Effect of preoperative pregabalin on postoperative pain relief in thyroidectomy patients: a prospective observational study. Anesth. Essays Res. 9, 161–166. doi: 10.4103/0259-1162.156292
Bockbrader, H. N., Radulovic, L. L., Posvar, E. L., Strand, J. C., Alvey, C. W., Busch, J. A., et al. (2010a). Clinical pharmacokinetics of pregabalin in healthy volunteers. J. Clin. Pharmacol. 50, 941–950. doi: 10.1177/0091270009352087
Bockbrader, H. N., Wesche, D., Miller, R., Chapel, S., Janiczek, N., and Burger, P. (2010b). A comparison of the pharmacokinetics and pharmacodynamics of pregabalin and gabapentin. Clin. Pharmacokinet. 49, 661–669. doi: 10.2165/11536200-000000000-00000
Boomershine, C. S. (2010). Pregabalin for the management of fibromyalgia syndrome. J. Pain Res. 3, 81–88. doi: 10.2147/jpr.s7884
Brawek, B., Löffler, M., Dooley, D. J., Weyerbrock, A., and Feuerstein, T. J. (2008). Differential modulation of K+-evoked 3H-neurotransmitter release from human neocortex by gabapentin and pregabalin. Naunyn Schmiedebergs Arch. Pharmacol. 376, 301–307. doi: 10.1007/s00210-007-0237-8
Brockhaus, J., Schreitmüller, M., Repetto, D., Klatt, O., Reissner, C., Elmslie, K., et al. (2018). α-neurexins together with α2δ-1 auxiliary subunits regulate Ca2+ influx through Cav2.1 channels. J. Neurosci. 38, 8277–8294. doi: 10.1523/JNEUROSCI.0511-18.2018
Buckner, R. L., Andrews-Hanna, J. R., and Schacter, D. L. (2008). The brain’s default network: anatomy, function, and relevance to disease. Ann. N Y Acad. Sci. 1124, 1–38. doi: 10.1196/annals.1440.011
Burstein, R., Noseda, R., and Borsook, D. (2015). Migraine: multiple processes, complex pathophysiology. J. Neurosci. 35, 6619–6629. doi: 10.1523/jneurosci.0373-15.2015
Buvanendran, A., Kroin, J. S., Kari, M., and Tuman, K. J. (2010). Can a single dose of 300 mg of pregabalin reach acute antihyperalgesic levels in the central nervous system? Reg. Anesth. Pain Med. 35, 535–538. doi: 10.1097/aap.0b013e3181fa6b7a
Cain, S. M., Bohnet, B., LeDue, J., Yung, A. C., Garcia, E., Tyson, J. R., et al. (2017). in vivo imaging reveals that pregabalin inhibits cortical spreading depression and propagation to subcortical brain structures. Proc. Natl. Acad. Sci. U S A 114, 2401–2406. doi: 10.1073/pnas.1614447114
Çakici, N., Fakkel, T. M., van Neck, J. W., Verhagen, A. P., and Coert, J. H. (2016). Systematic review of treatments for diabetic peripheral neuropathy. Diabet. Med. 33, 1466–1476. doi: 10.1111/dme.13083
Calandre, E. P., Garcia-Leiva, J. M., Rico-Villademoros, F., Vilchez, J. S., and Rodriguez-Lopez, C. M. (2010). Pregabalin in the treatment of chronic migraine: an open-label study. Clin. Neuropharmacol. 33, 35–39. doi: 10.1097/wnf.0b013e3181bf1dbe
Calandre, E. P., Rico-Villademoros, F., and Slim, M. (2016). Alpha2delta ligands, gabapentin, pregabalin and mirogabalin: a review of their clinical pharmacology and therapeutic use. Expert Rev. Neurother. 16, 1263–1277. doi: 10.1080/14737175.2016.1202764
Camilleri, M., and Boeckxstaens, G. (2017). Dietary and pharmacological treatment of abdominal pain in IBS. Gut 66, 966–974. doi: 10.1136/gutjnl-2016-313425
Cardenas, D. D., Nieshoff, E. C., Suda, K., Goto, S.-I., Sanin, L., Kaneko, T., et al. (2013). A randomized trial of pregabalin in patients with neuropathic pain due to spinal cord injury. Neurology 80, 533–539. doi: 10.1212/WNL.0b013e318281546b
Chen, J., Li, L., Chen, S.-R., Chen, H., Xie, J.-D., Sirrieh, R. E., et al. (2018). The α2δ-1-NMDA receptor complex is critically involved in neuropathic pain development and gabapentin therapeutic actions. Cell Rep. 22, 2307–2321. doi: 10.1016/j.celrep.2018.02.021
Clauw, D. J. (2014). Fibromyalgia: a clinical review. JAMA 311, 1547–1555. doi: 10.1001/jama.2014.3266
Coderre, T. J., Kumar, N., Lefebvre, C. D., and Yu, J. S. (2005). Evidence that gabapentin reduces neuropathic pain by inhibiting the spinal release of glutamate. J. Neurochem. 94, 1131–1139. doi: 10.1111/j.1471-4159.2005.03263.x
Cole, R. L., Lechner, S. M., Williams, M. E., Prodanovich, P., Bleicher, L., Varney, M. A., et al. (2005). Differential distribution of voltage-gated calcium channel alpha-2 delta (α2δ) subunit mRNA-containing cells in the rat central nervous system and the dorsal root ganglia. J. Comp. Neurol. 491, 246–269. doi: 10.1002/cne.20693
Costigan, M., Scholz, J., and Woolf, C. J. (2009). Neuropathic pain: a maladaptive response of the nervous system to damage. Annu. Rev. Neurosci. 32, 1–32. doi: 10.1146/annurev.neuro.051508.135531
Coull, J. A., Beggs, S., Boudreau, D., Boivin, D., Tsuda, M., Inoue, K., et al. (2005). BDNF from microglia causes the shift in neuronal anion gradient underlying neuropathic pain. Nature 438, 1017–1021. doi: 10.1038/nature04223
Daneshdoust, D., Khalili-Fomeshi, M., Ghasemi-Kasman, M., Ghorbanian, D., Hashemian, M., Gholami, M., et al. (2017). Pregabalin enhances myelin repair and attenuates glial activation in lysolecithin-induced demyelination model of rat optic chiasm. Neuroscience 344, 148–156. doi: 10.1016/j.neuroscience.2016.12.037
Davies, M., Brophy, S., Williams, R., and Taylor, A. (2006). The prevalence, severity, and impact of painful diabetic peripheral neuropathy in type 2 diabetes. Diabetes Care 29, 1518–1522. doi: 10.2337/dc05-2228
Derry, S., Cording, M., Wiffen, P. J., Law, S., Phillips, T., and Moore, R. A. (2016). Pregabalin for pain in fibromyalgia in adults. Cochrane Database Syst. Rev. 9:CD011790. doi: 10.1002/14651858.cd011790
Dolphin, A. C. (2013). The α2δ subunits of voltage-gated calcium channels. Biochim. Biophys. Acta 1828, 1541–1549. doi: 10.1016/j.bbamem.2012.11.019
Dolphin, A. C. (2018). Voltage-gated calcium channel α2δ subunits: an assessment of proposed novel roles. F1000Res. 7:1830. doi: 10.12688/f1000research.16104.1
Dong, J., Li, W., and Wang, Y. (2016). The effect of pregabalin on acute postoperative pain in patients undergoing total knee arthroplasty: a meta-analysis. Int. J. Surg. 34, 148–160. doi: 10.1016/j.ijsu.2016.08.521
Dooley, D. J., Donovan, C. M., Meder, W. P., and Whetzel, S. Z. (2002). Preferential action of gabapentin and pregabalin at P/Q-type voltage-sensitive calcium channels: inhibition of K+-evoked [3H]-norepinephrine release from rat neocortical slices. Synapse 45, 171–190. doi: 10.1002/syn.10094
Dooley, D. J., Taylor, C. P., Donevan, S., and Feltner, D. (2007). Ca2+ channel α2δ ligands: novel modulators of neurotransmission. Trends Pharmacol. Sci. 28, 75–82. doi: 10.1016/j.tips.2006.12.006
Durham, P. L., and Cady, R. (2011). Insights into the mechanism of onabotulinumtoxinA in chronic migraine. Headache 51, 1573–1577. doi: 10.1111/j.1526-4610.2011.02022.x
Dworkin, R. H., and Kirkpatrick, P. (2005). Pregabalin. Nat. Rev. Drug Discov. 4, 455–456. doi: 10.1038/nrd1756
Eroglu, C., Allen, N. J., Susman, M. W., O’Rourke, N. A., Park, C. Y., Ozkan, E., et al. (2009). Gabapentin receptor α2δ-1 is a neuronal thrombospondin receptor responsible for excitatory CNS synaptogenesis. Cell 139, 380–392. doi: 10.1016/j.cell.2009.09.025
Fashner, J., and Bell, A. L. (2011). Herpes zoster and postherpetic neuralgia: prevention and management. Am. Fam. Physician 83, 1432–1437.
Ferrari, M. D., Klever, R. R., Terwindt, G. M., Ayata, C., and van den Maagdenberg, A. M. J. M. (2015). Migraine pathophysiology: lessons from mouse models and human genetics. Lancet Neurol. 14, 65–80. doi: 10.1016/S1474-4422(14)70220-0
Field, M. J., Cox, P. J., Stott, E., Melrose, H., Offord, J., Su, T. Z., et al. (2006). Identification of the α2-δ-1 subunit of voltage-dependent calcium channels as a molecular target for pain mediating the analgesic actions of pregabalin. Proc. Natl. Acad. Sci. U S A 103, 17537–17542. doi: 10.1073/pnas.0409066103
Fink, K., Meder, W., Dooley, D. J., and Göthert, M. (2000). Inhibition of neuronal Ca2+ influx by gabapentin and subsequent reduction of neurotransmitter release from rat neocortical slices. Br. J. Pharmacol. 130, 900–906. doi: 10.1038/sj.bjp.0703380
Finnerup, N. B., Attal, N., Haroutounian, S., McNicol, E., Baron, R., Dworkin, R. H., et al. (2015). Pharmacotherapy for neuropathic pain in adults: a systematic review and meta-analysis. Lancet Neurol. 14, 162–173. doi: 10.1016/S1474-4422(14)70251-0
Fitzcharles, M.-A., Ste-Marie, P. A., Goldenberg, D. L., Pereira, J. X., Abbey, S., Choinière, M., et al. (2013). 2012 Canadian Guidelines for the diagnosis and management of fibromyalgia syndrome: executive summary. Pain Res. Manag. 18, 119–126. doi: 10.1155/2013/918216
Frampton, J. E. (2012). OnabotulinumtoxinA (BOTOX®): a review of its use in the prophylaxis of headaches in adults with chronic migraine. Drugs 72, 825–845. doi: 10.2165/11208880-000000000-00000
Frampton, J. E., and Foster, R. H. (2005). Pregabalin: in the treatment of postherpetic neuralgia. Drugs 65, 111–118; discussion 119–120. doi: 10.2165/00003495-200565010-00011
Freeman, R., Durso-Decruz, E., and Emir, B. (2008). Efficacy, safety, and tolerability of pregabalin treatment for painful diabetic peripheral neuropathy: findings from seven randomized, controlled trials across a range of doses. Diabetes Care 31, 1448–1454. doi: 10.2337/dc07-2105
Geisler, S., Schöpf, C. L., and Obermair, G. J. (2015). Emerging evidence for specific neuronal functions of auxiliary calcium channel α2δ subunits. Gen. Physiol. Biophys. 34, 105–118. doi: 10.4149/gpb_2014037
Geisler, S., Schöpf, C. L., Stanika, R., Kalb, M., Campiglio, M., Repetto, D., et al. (2019). Presynaptic α2δ-2 calcium channel subunits regulate postsynaptic GABAA receptor abundance and axonal wiring. J. Neurosci. 39, 2581–2605. doi: 10.1523/JNEUROSCI.2234-18.2019
Goadsby, P. J., Lipton, R. B., and Ferrari, M. D. (2002). Migraine—current understanding and treatment. N. Engl. J. Med. 346, 257–270. doi: 10.1056/NEJMra010917
Gong, H. C., Hang, J., Kohler, W., Li, L., and Su, T.-Z. (2001). Tissue-specific expression and gabapentin-binding properties of calcium channel α2δ subunit subtypes. J. Membr. Biol. 184, 35–43. doi: 10.1007/s00232-001-0072-7
Hagen, E. M., and Rekand, T. (2015). Management of neuropathic pain associated with spinal cord injury. Pain Ther. 4, 51–65. doi: 10.1007/s40122-015-0033-y
Harris, R. E., Napadow, V., Huggins, J. P., Pauer, L., Kim, J., Hampson, J., et al. (2013). Pregabalin rectifies aberrant brain chemistry, connectivity, and functional response in chronic pain patients. Anesthesiol. J. Am. Soc. Anesthesiol. 119, 1453–1464. doi: 10.1097/ALN.0000000000000017
Heidari, F., Afshari, M., and Moosazadeh, M. (2017). Prevalence of fibromyalgia in general population and patients, a systematic review and meta-analysis. Rheumatol. Int. 37, 1527–1539. doi: 10.1007/s00296-017-3725-2
Hendrich, J., Bauer, C. S., and Dolphin, A. C. (2012). Chronic pregabalin inhibits synaptic transmission between rat dorsal root ganglion and dorsal horn neurons in culture. Channels 6, 124–132. doi: 10.4161/chan.19805
Hoffmann, U., Dileköz, E., Kudo, C., and Ayata, C. (2010). Gabapentin suppresses cortical spreading depression susceptibility. J. Cereb. Blood Flow Metab. 30, 1588–1592. doi: 10.1038/jcbfm.2010.92
Hoppa, M. B., Lana, B., Margas, W., Dolphin, A. C., and Ryan, T. A. (2012). α2δ expression sets presynaptic calcium channel abundance and release probability. Nature 486, 122–125. doi: 10.1038/nature11033
Ifuku, M., Iseki, M., Hidaka, I., Morita, Y., Komatus, S., and Inada, E. (2011). Replacement of gabapentin with pregabalin in postherpetic neuralgia therapy. Pain Med. 12, 1112–1116. doi: 10.1111/j.1526-4637.2011.01162.x
Javed, S., Alam, U., and Malik, R. A. (2015). Burning through the pain: treatments for diabetic neuropathy. Diabetes Obes. Metab. 17, 1115–1125. doi: 10.1111/dom.12535
Juenke, J. M., Brown, P. I., Johnson-Davis, K. L., and McMillin, G. A. (2011). Simultaneous quantification of levetiracetam and gabapentin in plasma by ultra-pressure liquid chromatography coupled with tandem mass spectrometry detection. Ther. Drug Monit. 33, 209–213. doi: 10.1097/ftd.0b013e31820b1fce
Juhn, M. S., Parsons, B., Varvara, R., and Sadosky, A. (2015). Pregabalin for painful diabetic peripheral neuropathy: strategies for dosing, monotherapy vs. combination therapy, treatment-refractory patients and adverse events. Curr. Med. Res. Opin. 31, 1017–1026. doi: 10.1185/03007995.2015.1030375
Kadurin, I., Rothwell, S. W., Lana, B., Nieto-Rostro, M., and Dolphin, A. C. (2017). LRP1 influences trafficking of N-type calcium channels via interaction with the auxiliary α2δ-1 subunit. Sci. Rep. 7:43802. doi: 10.1038/srep43802
Kilgore, P. E., Kruszon-Moran, D., Seward, J. F., Jumaan, A., Van Loon, F. P. L., Forghani, B., et al. (2003). Varicella in Americans from NHANES III: implications for control through routine immunization. J. Med. Virol. 70, S111–S118. doi: 10.1002/jmv.10364
Klompas, M., Kulldorff, M., Vilk, Y., Bialek, S. R., and Harpaz, R. (2011). Herpes zoster and postherpetic neuralgia surveillance using structured electronic data. Mayo Clin. Proc. 86, 1146–1153. doi: 10.4065/mcp.2011.0305
Kumar, N., Cherkas, P. S., Varathan, V., Miyamoto, M., Chiang, C. Y., Dostrovsky, J. O., et al. (2013). Systemic pregabalin attenuates facial hypersensitivity and noxious stimulus-evoked release of glutamate in medullary dorsal horn in a rodent model of trigeminal neuropathic pain. Neurochem. Int. 62, 831–835. doi: 10.1016/j.neuint.2013.02.022
Kuner, R. (2015). Spinal excitatory mechanisms of pathological pain. Pain 156, S11–S17. doi: 10.1097/j.pain.0000000000000118
Lauritzen, M., Dreier, J. P., Fabricius, M., Hartings, J. A., Graf, R., and Strong, A. J. (2011). Clinical relevance of cortical spreading depression in neurological disorders: migraine, malignant stroke, subarachnoid and intracranial hemorrhage, and traumatic brain injury. J. Cereb. Blood Flow Metab. 31, 17–35. doi: 10.1038/jcbfm.2010.191
Li, Z., Taylor, C. P., Weber, M., Piechan, J., Prior, F., Bian, F., et al. (2011). Pregabalin is a potent and selective ligand for α2δ-1 and α2δ-2 calcium channel subunits. Eur. J. Pharmacol. 667, 80–90. doi: 10.1016/j.ejphar.2011.05.054
Linde, M., Mulleners, W. M., Chronicle, E. P., and McCrory, D. C. (2013). Gabapentin or pregabalin for the prophylaxis of episodic migraine in adults. Cochrane Database Syst. Rev. 6:CD010609. doi: 10.1002/14651858.cd010609
Lipton, R. B., Rosen, N. L., Ailani, J., DeGryse, R. E., Gillard, P. J., and Varon, S. F. (2016). OnabotulinumtoxinA improves quality of life and reduces impact of chronic migraine over one year of treatment: pooled results from the PREEMPT randomized clinical trial program. Cephalalgia 36, 899–908. doi: 10.1177/0333102416652092
Luo, Z. D., Chaplan, S. R., Higuera, E. S., Sorkin, L. S., Stauderman, K. A., Williams, M. E., et al. (2001). Upregulation of dorsal root ganglion α2δ calcium channel subunit and its correlation with allodynia in spinal nerve-injured rats. J. Neurosci. 21, 1868–1875. doi: 10.1523/jneurosci.21-06-01868.2001
Mallick-Searle, T., Snodgrass, B., and Brant, J. M. (2016). Postherpetic neuralgia: epidemiology, pathophysiology, and pain management pharmacology. J. Multidiscip. Healthc. 9, 447–454. doi: 10.2147/jmdh.s106340
Marmiroli, P., and Cavaletti, G. (2016). Drugs for the treatment of peripheral neuropathies. Expert Opin. Pharmacother. 17, 381–394. doi: 10.1517/14656566.2016.1120719
Mathew, N. T., Rapoport, A., Saper, J., Magnus, L., Klapper, J., Ramadan, N., et al. (2001). Efficacy of gabapentin in migraine prophylaxis. Headache 41, 119–128. doi: 10.1046/j.1526-4610.2001.111006119.x
Mulleners, W. M., McCrory, D. C., and Linde, M. (2015). Antiepileptics in migraine prophylaxis: an updated Cochrane review. Cephalalgia 35, 51–62. doi: 10.1177/0333102414534325
Nalamachu, S., and Morley-Forster, P. (2012). Diagnosing and managing postherpetic neuralgia. Drugs Aging 29, 863–869. doi: 10.1007/s40266-012-0014-3
Napadow, V., LaCount, L., Park, K., As-Sanie, S., Clauw, D. J., and Harris, R. E. (2010). Intrinsic brain connectivity in fibromyalgia is associated with chronic pain intensity. Arthritis Rheum. 62, 2545–2555. doi: 10.1002/art.27497
Nguyen, D., Deng, P., Matthews, E. A., Kim, D. S., Feng, G., Dickenson, A. H., et al. (2009). Enhanced pre-synaptic glutamate release in deep-dorsal horn contributes to calcium channel alpha-2-delta-1 protein-mediated spinal sensitization and behavioral hypersensitivity. Mol. Pain 5:6. doi: 10.1186/1744-8069-5-6
Paoletti, P., Bellone, C., and Zhou, Q. (2013). NMDA receptor subunit diversity: impact on receptor properties, synaptic plasticity and disease. Nat. Rev. Neurosci. 14, 383–400. doi: 10.1038/nrn3504
Patel, M. K., Gonzalez, M. I., Bramwell, S., Pinnock, R. D., and Lee, K. (2000). Gabapentin inhibits excitatory synaptic transmission in the hyperalgesic spinal cord. Br. J. Pharmacol. 130, 1731–1734. doi: 10.1038/sj.bjp.0703530
Peirs, C., and Seal, R. P. (2016). Neural circuits for pain: recent advances and current views. Science 354, 578–584. doi: 10.1126/science.aaf8933
Pérez, C., Latymer, M., Almas, M., Ortiz, M., Clair, A., Parsons, B., et al. (2017). Does duration of neuropathic pain impact the effectiveness of pregabalin? Pain Pract. 17, 470–479. doi: 10.1111/papr.12469
Petrou, M., Pop-Busui, R., Foerster, B. R., Edden, R. A., Callaghan, B. C., Harte, S. E., et al. (2012). Altered excitation-inhibition balance in the brain of patients with diabetic neuropathy. Acad. Radiol. 19, 607–612. doi: 10.1016/j.acra.2012.02.004
Pietrobon, D., and Moskowitz, M. A. (2014). Chaos and commotion in the wake of cortical spreading depression and spreading depolarizations. Nat. Rev. Neurosci. 15, 379–393. doi: 10.1038/nrn3770
Pizzolato, R., Villani, V., Prosperini, L., Ciuffoli, A., and Sette, G. (2011). Efficacy and tolerability of pregabalin as preventive treatment for migraine: a 3-month follow-up study. J. Headache Pain 12, 521–525. doi: 10.1007/s10194-011-0338-0
Portenoy, R. K., and Cruciani, R. (2009). “Chapter 28—Neuropathic pain,” in Pain Management Secrets (Third Edition) [Internet], eds C. E. Argoff and G. McCleane (Philadelphia: Mosby), 184–209.
Potter, L. E., Paylor, J. W., Suh, J. S., Tenorio, G., Caliaperumal, J., Colbourne, F., et al. (2016). Altered excitatory-inhibitory balance within somatosensory cortex is associated with enhanced plasticity and pain sensitivity in a mouse model of multiple sclerosis. J. Neuroinflammation 13:142. doi: 10.1186/s12974-016-0609-4
Rajappa, G. C., Vig, S., Bevanaguddaiah, Y., and Anadaswamy, T. C. (2016). Efficacy of pregabalin as premedication for post-operative analgesia in vaginal hysterectomy. Anesth. Pain Med. 6:e34591. doi: 10.5812/aapm.34591
Sagit, M., Yalcin, S., Polat, H., Korkmaz, F., Cetinkaya, S., and Somdas, M. A. (2013). Efficacy of a single preoperative dose of pregabalin for postoperative pain after septoplasty. J. Craniofac. Surg. 24, 373–375. doi: 10.1097/SCS.0b013e31827fece5
Schmidt, P. C., Ruchelli, G., Mackey, S. C., and Carroll, I. R. (2013). Perioperative gabapentinoids: choice of agent, dose, timing, and effects on chronic postsurgical pain. Anesthesiology 119, 1215–1221. doi: 10.1097/ALN.0b013e3182a9a896
Sharma, U., Griesing, T., Emir, B., and Young, J. P. Jr. (2010). Time to onset of neuropathic pain reduction: a retrospective analysis of data from nine controlled trials of pregabalin for painful diabetic peripheral neuropathy and postherpetic neuralgia. Am. J. Ther. 17, 577–585. doi: 10.1097/mjt.0b013e3181d5e4f3
Siddall, P. J., Cousins, M. J., Otte, A., Griesing, T., Chambers, R., and Murphy, T. K. (2006). Pregabalin in central neuropathic pain associated with spinal cord injury: a placebo-controlled trial. Neurology 67, 1792–1800. doi: 10.1212/01.wnl.0000244422.45278.ff
Sluka, K. A., and Clauw, D. J. (2016). Neurobiology of fibromyalgia and chronic widespread pain. Neuroscience 338, 114–129. doi: 10.1016/j.neuroscience.2016.06.006
Spreng, U. J., Dahl, V., and Raeder, J. (2011). Effect of a single dose of pregabalin on post-operative pain and pre-operative anxiety in patients undergoing discectomy. Acta Anaesthesiol. Scand. 55, 571–576. doi: 10.1111/j.1399-6576.2011.02410.x
Stahl, S. M., Porreca, F., Taylor, C. P., Cheung, R., Thorpe, A. J., and Clair, A. (2013). The diverse therapeutic actions of pregabalin: is a single mechanism responsible for several pharmacological activities? Trends Pharmacol. Sci. 34, 332–339. doi: 10.1016/j.tips.2013.04.001
Sun, W., Larson, M. J. E., Kiyoshi, C. M., Annett, A. J., Stalker, W. A., Peng, J., et al. (2020). Gabapentinoid treatment promotes corticospinal plasticity and regeneration following murine spinal cord injury. J. Clin. Invest. 130, 345–358. doi: 10.1172/jci130391
Tajti, J., Szok, D., Majláth, Z., Csáti, A., Petrovics-Balog, A., and Vécsei, L. (2016). Alleviation of pain in painful diabetic neuropathy. Expert Opin. Drug Metab. Toxicol. 12, 753–764. doi: 10.1080/17425255.2016.1184648
Uchitel, O. D., Di Guilmi, M. N., Urbano, F. J., and Gonzalez-Inchauspe, C. (2010). Acute modulation of calcium currents and synaptic transmission by gabapentinoids. Channels 4, 490–496. doi: 10.4161/chan.4.6.12864
van Nooten, F., Treur, M., Pantiri, K., Stoker, M., and Charokopou, M. (2017). Capsaicin 8% patch versus oral neuropathic pain medications for the treatment of painful diabetic peripheral neuropathy: a systematic literature review and network meta-analysis. Clin. Ther. 39, 787.e18–803.e18. doi: 10.1016/j.clinthera.2017.02.010
Woolf, C. J. (1983). Evidence for a central component of post-injury pain hypersensitivity. Nature 306, 686–688. doi: 10.1038/306686a0
Woolf, C. J., and Mannion, R. J. (1999). Neuropathic pain: aetiology, symptoms, mechanisms, and management. Lancet 353, 1959–1964. doi: 10.1016/s0140-6736(99)01307-0
Yang, S., Krug, S. M., Heitmann, J., Hu, L., Reinhold, A. K., Sauer, S., et al. (2016). Analgesic drug delivery via recombinant tissue plasminogen activator and microRNA-183-triggered opening of the blood-nerve barrier. Biomaterials 82, 20–33. doi: 10.1016/j.biomaterials.2015.11.053
Zain, S., Khan, M., Alam, R., Zafar, I., and Ahmed, S. (2013). Comparison of efficacy and safety of topiramate with gabapentin in migraine prophylaxis: randomized open label control trial. J. Pak. Med. Assoc. 63, 3–7.
Keywords: pregabalin (PGB), chronic pain, therapeutic targets, calcium channel, hyperexcitability
Citation: Alles SRA, Cain SM and Snutch TP (2020) Pregabalin as a Pain Therapeutic: Beyond Calcium Channels. Front. Cell. Neurosci. 14:83. doi: 10.3389/fncel.2020.00083
Received: 17 February 2020; Accepted: 20 March 2020;
Published: 15 April 2020.
Edited by:
Divya P. Kumar, JSS Academy of Higher Education and Research, IndiaReviewed by:
Ryan Patel, University College London, United KingdomLorenzo Di Cesare Mannelli, University of Florence, Italy
Copyright © 2020 Alles, Cain and Snutch. This is an open-access article distributed under the terms of the Creative Commons Attribution License (CC BY). The use, distribution or reproduction in other forums is permitted, provided the original author(s) and the copyright owner(s) are credited and that the original publication in this journal is cited, in accordance with accepted academic practice. No use, distribution or reproduction is permitted which does not comply with these terms.
*Correspondence: Terrance P. Snutch, snutch@msl.ubc.ca
† These authors have contributed equally to this work