A New Functional Magnetic Resonance Imaging Localizer for Preoperative Language Mapping Using a Sentence Completion Task: Validity, Choice of Baseline Condition, and Test–Retest Reliability
- 1Center for Language and Brain, HSE University, Moscow, Russia
- 2Department of Radiology, National Medical and Surgical Center Named After N.I. Pirogov, Moscow, Russia
- 3Institute of Linguistics, Russian Academy of Sciences, Moscow, Russia
To avoid post-neurosurgical language deficits, intraoperative mapping of the language function in the brain can be complemented with preoperative mapping with functional magnetic resonance imaging (fMRI). The validity of an fMRI “language localizer” paradigm crucially depends on the choice of an optimal language task and baseline condition. This study presents a new fMRI “language localizer” in Russian using overt sentence completion, a task that comprehensively engages the language function by involving both production and comprehension at the word and sentence level. The paradigm was validated in 18 neurologically healthy volunteers who participated in two scanning sessions, for estimating test–retest reliability. For the first time, two baseline conditions for the sentence completion task were compared. At the group level, the paradigm significantly activated both anterior and posterior language-related regions. Individual-level analysis showed that activation was elicited most consistently in the inferior frontal regions, followed by posterior temporal regions and the angular gyrus. Test–retest reliability of activation location, as measured by Dice coefficients, was moderate and thus comparable to previous studies. Test–retest reliability was higher in the frontal than temporo-parietal region and with the most liberal statistical thresholding compared to two more conservative thresholding methods. Lateralization indices were expectedly left-hemispheric, with greater lateralization in the frontal than temporo-parietal region, and showed moderate test-retest reliability. Finally, the pseudoword baseline elicited more extensive and more reliable activation, although the syllable baseline appears more feasible for future clinical use. Overall, the study demonstrated the validity and reliability of the sentence completion task for mapping the language function in the brain. The paradigm needs further validation in a clinical sample of neurosurgical patients. Additionally, the study contributes to general evidence on test–retest reliability of fMRI.
Introduction
Language Mapping in Neurosurgical Patients
When patients undergo neurosurgical interventions for brain tumors, refractory epilepsy, arteriovenous malformations, a crucial goal is to remove pathological tissue while sparing eloquent (functionally necessary) areas, so that the respective functions, including cognitive ones (Satoer et al., 2016), are not impaired following neurosurgery (Duffau, 2012). One critical function is language processing: it lies at the core of human communication, and its impairment negatively impacts return to work, social inclusion, and general quality of life (Hilari et al., 2003; Gabel et al., 2019).
Localization of the language network is highly variable across individuals (Ojemann, 1979), and the variability is further enhanced by functional re-organization that happens in case of brain pathology: for example, over the course of brain tumor growth (Almairac et al., 2018; Zhang et al., 2018). Thus, to avoid damage to brain areas critical for the language function and to prevent subsequent language impairment, the neurosurgical team performs mapping of “language-eloquent” brain areas in individual patients. The gold standard for localizing language-eloquent brain areas is intraoperative mapping with direct electrical stimulation (DES) during awake craniotomy (Ojemann, 1979; Rofes et al., 2019). During this procedure, an electric current is applied to exposed brain tissue, causing a temporary disruption of neural activity. Meanwhile, the patient is awake from anesthesia and is performing a language task. If application of DES to an area reliably leads to errors or speech arrest, this means that the area is eloquent and should be spared during neurosurgery, if possible.
While intraoperative DES is the standard procedure for language mapping, there are reasons to complement it with additional preoperative mapping. Firstly, preoperative language mapping allows to plan the surgical procedure in advance (Silva et al., 2018; Weng et al., 2018). Based on preoperative mapping data, the neurosurgeon can decide whether intraoperative DES mapping of the language function is necessary (for example, when operating over a presumably non-language-dominant hemisphere) or plan an optimal access route to bypass language-eloquent areas. Secondly, data from preoperative mapping can be used if DES cannot be completed: for example, if the patient does not cooperate or if there are epileptic seizures during DES (Hervey-Jumper et al., 2015). In such cases, the results of preoperative mapping, even though providing less direct information than DES, can still inform the neurosurgeon and reduce the risks of functional impairment.
Historically, preoperative language mapping was performed using the intracarotid sodium amobarbital procedure, known as the Wada test (Wada and Rasmussen, 1960). The test involves anesthetizing one hemisphere by injecting sodium amobarbital through a catheter while the patient is performing a language task. Failure to perform the task indicates that the anesthetized hemisphere is crucial for language processing. However, the Wada test has major limitations. It is a highly invasive procedure that can cause complications: according to Loddenkemper et al. (2008), they emerge in up to 11% patients and include serious adverse events such as stroke. Another limitation is that effects of sodium amobarbital only last for a few minutes, providing very limited time for testing (Loring et al., 1992). Critically, the Wada test can only assess hemispheric dominance of language processing but cannot address specific localization of language-eloquent areas within a hemisphere.
Therefore, other technologies have been replacing the Wada test for preoperative language mapping: navigated transcranial magnetic stimulation (nTMS; Picht et al., 2013), functional magnetic resonance imaging (fMRI; for review, see Silva et al., 2018; Agarwal et al., 2019), magnetoencephalography (MEG; Van Poppel et al., 2012), or combination thereof (Ille et al., 2015; Sollmann et al., 2016). Unlike the Wada test, these methods are largely safe and non-invasive. On top of that, they have high spatial resolution and allow to identify language-eloquent brain areas with the precision of millimeters. To take full advantage of these methods, it is crucial to choose an optimal functional paradigm that would be sensitive, specific and reliable in identifying both lateralization (hemispheric dominance) and specific localization of brain networks comprehensively enabling the language function. In this paper, we present such paradigm for pre-operative language mapping using fMRI in Russian-speaking individuals and provide methodological evidence on its test-retest reliability and the optimal baseline condition.
Choice of a Language Task for Functional Magnetic Resonance Imaging Mapping
The quality of a language mapping paradigm critically depends on the choice of a language task: that is, whether it is able to comprehensively engage all levels of linguistic processing while remaining feasible. Previous fMRI “language localizer” paradigms have used a variety of tasks (for review, see Bradshaw et al., 2017; Manan et al., 2020). Below, we review most popular language tasks and summarize previous evidence on their success in identifying language networks in the brain.
Most “language localizers” have used single-word tasks, particularly expressive single-word tasks (Manan et al., 2020). A traditional expressive single-word task adapted from early intraoperative batteries (Ojemann, 1993; Ruge et al., 1999) was number counting. However, counting is a highly automated process that engages linguistic processing only superficially and is no longer considered sufficiently sensitive to identify language networks (Brennan et al., 2007; Morrison et al., 2016b). Today, other tasks are used to engage word retrieval, such as picture naming (Pouratian et al., 2002; Rutten et al., 2002; Roux et al., 2003; Brennan et al., 2007) and verbal fluency, where the participant has to name as many words as possible from a given semantic category or starting with a given letter (Ruff et al., 2008; Sanjuan et al., 2010). Expressive single-word tasks are intuitive for the participant and are easily timed relative to fMRI scanning. Still, they only engage the sound and word levels of language production, leaving out any grammatical processing and any language comprehension. Thus, they cannot fully identify brain areas that are crucial for sentence-level communication. Indeed, compared to sentence-level tasks, expressive single-word tasks elicit less activation in both anterior and posterior left-hemispheric “language regions” (Połczyńska et al., 2017; Manan et al., 2020). Additionally, picture naming shows a poor lateralizing ability (Deblaere et al., 2002; Bradshaw et al., 2017).
Similar problems are faced by receptive single-word tasks, such as phonemic judgment, requiring to make a decision about the sound structure of a word (for example, whether two words rhyme; Jones et al., 2011), or semantic judgment, requiring to make a decision about the meaning of a word (for example, whether it refers to an animate object, or whether two words are opposite in meaning; Binder et al., 1996; Szaflarski et al., 2008). Again, such tasks are easily integrated with the timing of fMRI scanning. Moreover, unlike expressive single-word tasks, they do not evoke head motion artifacts due to articulation. However, they are even more limited in engaging linguistic processes and cannot detect brain networks enabling language production or any grammatical processing beyond the word level. Empirically, these tasks have shown limited lateralizing ability (Deblaere et al., 2002; Jansen et al., 2006) and reliability (Jansen et al., 2006; reliability will be discussed in more detail below).
To more fully activate language networks, a number of fMRI protocols used sentence-level tasks requiring to process not only individual words but also their grammatical and semantic relations. Examples of expressive sentence-level tasks are describing a picture with a sentence (Partovi et al., 2012; Mauler et al., 2017) or generating a sentence with given words (Hakyemez et al., 2006). Such tasks appear to successfully activate both anterior and posterior language areas (Hakyemez et al., 2006; Partovi et al., 2012; Mauler et al., 2017). However, they are taxing for the patient and difficult to time relative to fMRI scanning, especially given interindividual variability in task completion speed across patients, so they are not widely used.
Much more popular are receptive sentence-level tasks. These are sentence or passage listening (Pillai and Zaca, 2011; Suarez et al., 2014; Wilson et al., 2017) or reading (Grummich et al., 2006; Fedorenko et al., 2010), which may be passive or accompanied by comprehension questions, such as to judge real-world plausibility of a sentence or match it to a picture (Pillai and Zaca, 2011; Kinno et al., 2014). These tasks are more easily timed than expressive sentence-level tasks but also successfully engage grammatical processing: that is, the participant has both to process individual words and analyze their relations. Passive receptive sentence-level tasks have an additional advantage of feasibility in patients with compromised language production or non-cooperative patients (for example, in the pediatric population, Suarez et al., 2014). However, lack of response requirements simultaneously presents a drawback: it is impossible to control or monitor the participant’s task engagement and compliance. This makes it difficult to estimate individual-level data validity in clinical populations with cognitive difficulties. Another limitation is that receptive sentence-level tasks may place most emphasis on activating posterior language-related areas and engage anterior language-related areas to a lesser extent than expressive tasks (Lehéricy et al., 2000; Nadkarni et al., 2015). Finally, some studies have shown low lateralizing abilities of sentence-level receptive tasks (Pillai and Zaca, 2011; Ocklenburg et al., 2013), although many others have not replicated this result (Lehéricy et al., 2000; Thivard et al., 2005).
Taken together, in order to comprehensively identify brain networks that enable real-life language use, an fMRI paradigm needs to engage both language production and comprehension in a task that goes beyond the word level. One solution is a conjunction analysis of multiple tasks targeting different language processes separately (Pouratian et al., 2002; De Guibert et al., 2010). However, interpretation of the conjunction analysis is not straightforward if tasks elicit largely different activations. Additionally, from the clinical viewpoint, multiple-task paradigms are time-consuming and less feasible in clinical settings. Thus, another solution is an fMRI localizer paradigm that uses a single task engaging both language production and comprehension beyond the word level.
One such comprehensive task is sentence completion, advocated in a recent white paper of the American Society of Functional Neuroradiology (Black et al., 2017) and a metaanalysis by Manan et al. (2020). In this task, the participant has to read aloud a sentence with a missing final word and complete it with a semantically and grammatically appropriate word. This task comprehensively involves many linguistic processes in both comprehension (orthographic processing, word access, grammatical parsing, semantic integration) and production (word search, grammatical inflection in morphologically complex languages such as Russian, phonological encoding and articulation). Empirically, previous works have successfully used the sentence completion task to assess both lateralization and localization of language processing networks (Zacà et al., 2012; Barnett et al., 2014; Połczyńska et al., 2017; Salek et al., 2017; Wilson et al., 2017; Unadkat et al., 2019). For example, Unadkat et al. (2019) found that sentence completion elicited more lateralized language-related activation in posterior regions, compared to antonym generation and auditory naming. Salek et al. (2017) found that sentence completion was better able to localize activity in posterior temporal and angular gyri than category naming or verbal fluency. Based on the analysis of activation location, lateralization and test-retest reliability, Wilson et al. (2017) concluded that sentence completion and narrative comprehension were superior to picture naming and “naturalistic comprehension” in providing the balance of validity and reliability.
Inspired by these sentence completion paradigms in English, the present study presents a similar paradigm in Russian. Russian is the 8th most spoken language in the world, with about 120 million first-language speakers worldwide (Eberhard et al., 2020), so a new clinical tool in Russian would serve the needs of a large Russian-speaking clinical population. So far, Russian-language paradigms for presurgical language mapping have been very few and have never used a sentence completion task (Litvinova et al., 2012; Rumshiskaya et al., 2014).
Choice of a Baseline Task for Functional Magnetic Resonance Imaging Mapping
A crucial concept in classic fMRI analysis is “subtraction logic”: to isolate neural activation related to the process of interest, the analysis should “subtract” the activation in a “lower-level” baseline (control) task from the activation in a “higher-level” experimental task (Huettel et al., 2008). Specifically in case of “language localizer” paradigms, such subtraction allows to isolate language-related neural activity from activity due to sensorimotor processes, general alertness, and non-language-related cognitive processes. Due to the subtraction principle, not only the choice of an experimental language task but also the choice of a lower-level baseline task can vastly impact the findings of fMRI “language localizers” (Bradshaw et al., 2017).
Some previous fMRI “language localizers” used passive rest or viewing of a fixation cross as the baseline condition (Jones et al., 2011; Suarez et al., 2014). However, a passive baseline is problematic for several reasons. Firstly, a passive baseline does not require any sensorimotor or cognitive activity, so subtracting it from the experimental condition does not fully isolate language-related activity from lower-level processes. Secondly, it is not possible to control or monitor the patient’s cognitive activity during passive rest, so mind-wandering or other patient-initiated cognitive activity may confound the results. Indeed, fMRI analyses using passive baselines have elicited less specific and less lateralized activation compared to analyses using active baselines (Hund-Georgiadis et al., 2001; Dodoo-Schittko et al., 2012; although see Newman et al., 2001; Miró et al., 2014).
Therefore, active baselines are typically recommended for more specific isolation of language-related activity from lower-level processes (Bradshaw et al., 2017). Choosing an optimal active baseline presents a challenge: for most language tasks, the respective lower-level processes can be addressed by several theoretically possible baselines. For example, for listening tasks, the baseline condition can involve listening to backwards speech (Lehéricy et al., 2000; Thivard et al., 2005), various types of noise (Rodd et al., 2005), or music (Bleich-Cohen et al., 2009). Although all these baselines involve auditory processing (lower-level sensory processing) and presumably no linguistic processing, an empirical comparison by Stoppelman et al. (2013) showed that different baselines yielded very different results. So far, such direct empirical comparisons in order to choose an optimal baseline have only been made for few language tasks and baseline types (Newman et al., 2001; Binder et al., 2008; Stoppelman et al., 2013).
To the best of our knowledge, our study is the first to make an empirical comparison between two different baselines for the sentence completion task. These are a syllable baseline, where the participant has to read aloud a sequence consisting of the same syllable and repeat the syllable once more, and a pseudoword baseline, where the participant has to read aloud a sequence of pseudowords and repeat any of them once. Both baselines are theoretically plausible. In contrast to the experimental condition, they do not consist of real words or resemble grammatical structures, so they do not elicit any lexical or grammatical processing. At the same time, they involve the same “lower-level” processes as the experimental condition: visual and orthographic processing, motor planning and articulation, and initiation of a response (completion of a sequence). Thus, their subtraction allows to maximally isolate language-related from “lower-level” neural activity. Previous sentence completion paradigms used other baselines that subtracted “lower-level” activity less fully (rest: Połczyńska et al., 2017; passive viewing of nonsense symbols: Zacà et al., 2012; Barnett et al., 2014) or a conjunction analysis approach without an explicit baseline (Wilson et al., 2017). The present study is the first to employ and compare a syllable and pseudoword baseline for the sentence completion task.
Reliability of Functional Magnetic Resonance Imaging Mapping
Another methodological contribution of the present study is estimating test-retest reliability of the fMRI paradigm. Reliability is critical for any clinical usage of fMRI “language localizers”: distribution of brain activity needs to be reproducible at multiple testing sessions in order to consider it clinically meaningful and draw any implications for neurosurgical treatment. Recent studies have raised concerns about test–retest reliability of task-based fMRI in general, due to inherent physiological noise, scanner noise and changes in concurrent non-task-related cognitive activity in participants (Bennett and Miller, 2010; Holiga et al., 2018; Elliott et al., 2020). In light of these general concerns, it is important to quantify and report reliability of any paradigms suggested for clinical use.
Previous studies have started estimating test-retest reliability of fMRI “language localizer” paradigms in healthy control participants (Fesl et al., 2010; Morrison et al., 2016a; Wilson et al., 2017; Nettekoven et al., 2018) and clinical populations (Fernández et al., 2003; Morrison et al., 2016a). For example, Morrison et al. (2016a) showed high individual variability in test-retest reliability, which was on average lower in a phonemic fluency task than in a rhyming task, and in patients with high-grade gliomas than patients with low-grade gliomas and healthy control participants. Using an overt object naming task in healthy participants, Nettekoven et al. (2018) showed high reliability of the activation peak location but low reliability of activation extent, particularly in the right hemisphere.
To the best of our knowledge, only two studies so far have estimated test–retest reliability of sentence completion paradigms. Whalley et al. (2009) used the Hayling sentence completion task in individuals with high genetic risk of schizophrenia and showed good test-retest reliability. Wilson et al. (2017) compared test–retest reliability of four language tasks in healthy participants and found the best reliability in picture naming, followed by naturalistic comprehension, sentence completion, and narrative comprehension. Despite this pattern, the authors concluded that sentence completion was one of two tasks offering the best balance of reliability and validity for an fMRI language localizer. Our study aims to add to these emerging data and provide more evidence on test–retest reliability of a sentence completion fMRI paradigm.
Previous studies used different metrics to quantify test–retest reliability: Dice coefficient (Fesl et al., 2010; Morrison et al., 2016a; Wilson et al., 2017; Nettekoven et al., 2018), Jaccard index (Morrison et al., 2016a), Euclidean distance (Morrison et al., 2016a; Nettekoven et al., 2018), voxelwise intraclass correlation coefficient (Fernández et al., 2003; Whalley et al., 2009; Nettekoven et al., 2018), correlation of lateralization indices (LIs; Fesl et al., 2010; Morrison et al., 2016a). The present study adopted two of them: between-session Dice coefficient and correlation of LIs. Advantages of the Dice coefficient are that it is widely used in the literature, is straightforward to interpret and, unlike intraclass correlation coefficient, can provide a global whole-brain measure and is calculated individually with no reference to group data (Bennett and Miller, 2010; Wilson et al., 2017). Besides, using a Dice coefficient ensures comparability to Wilson et al. (2017), the only previous study that included a sentence completion task and compared its reliability to other tasks. Additionally, we measured the test-retest correlation of LIs because, just as individual activation maps, they also present a clinically relevant measure that can inform a neurosurgeon’s decision on the necessity of awake surgery.
The Present Study
To summarize, this paper presents a new fMRI localizer paradigm for preoperative language mapping in Russian-speaking individuals with brain tumors, refractory epilepsy, and other conditions when neurosurgery is indicated. Following the best practices in other languages, we used a sentence completion task that comprehensively engages language production and comprehension processes at the word and sentence level. We present the data from a control group of neurologically healthy individuals, test whether the paradigm can successfully identify the expected language-related areas in this group, compare two different baseline conditions (syllables versus pseudowords), and quantify the test–retest reliability of the paradigm.
Materials and Methods
Participants
The study included 21 right-handed native speakers of Russian with no history of neurological or psychiatric disorders. Data of three participants were excluded from analysis due to excessive head movement in the scanner (more than 5 mm), resulting in a sample of 18 participants (14 females; age: mean 41.3, SD 6.6, range 30–53 years; years of education: mean 16.2, SD 4.7, range 11–30 years; Edinburgh Handedness Inventory score: mean 52, SD 2.67, range 46–55). All participants had normal hearing and normal or corrected-to-normal vision. All participants gave written informed consent.
Task and Stimuli
During each scanning session, participants performed two identically structured language mapping paradigms. Both mapping paradigms comprised an experimental condition and a baseline condition. The experimental condition was identical in the two paradigms: participants were visually presented with a Russian sentence with a missing final word and instructed to read the sentence aloud and produce an appropriate final word aloud. The two paradigms differed with regard to the baseline condition, including either a syllable (henceforth, SYLL) or a pseudoword (henceforth, PW) baseline. In the SYLL baseline condition, participants read aloud a visually presented string consisting of one syllable repeated three times (e.g., «Φeeeϕeeeeeeϕeeeeee…» – «Feee feeeeee feeeeee…») and repeated the syllable aloud one more time. In the PW baseline condition, participants read aloud a visually presented string of pseudowords (pseudonouns) phonotactically legal in Russian (e.g., «Уптилья пикаш измеха…» – «Uptilja pikaš izmeha.») and repeated any single of the pseudowords. In both the experimental and baseline condition, each stimulus was presented as a whole in one row, in 44 point font size, requiring eye movements for reading. We chose visual rather than auditory stimuli presentation for two reasons. First, thanks to gist reading, it allowed for faster response times as compared to auditory presentation, where full semantic information for completing a sentence would only become available after the uniqueness point of the last auditorily presented word. Second, visual presentation allowed to map brain activation supporting lexical reading processes.
The stimuli in the experimental condition were Russian sentences (60 per paradigm). The full stimuli list is publicly available online: https://www.hse.ru/en/neuroling/research/fmri-mapping. The sentences were three words long and had one of the following syntactic structures:
(1) Adjective + noun (subject) + verb.
Умная соседка прочла ….
A clever neighbor read…
(2) Noun (subject) + verb + adjective.
Скрипачка сдала сложный …
A violinist passed a challenging…
(3) Noun (subject) + adverb + verb.
Грабитель ловко украл …
A thief skillfully stole…
All verbs were used in the present or past tense and required a direct object. In sentences of structure (2), the inflectional form of the adjective unambiguously determined the gender and number of the direct object. Words were no longer than three syllables and at least one word in each sentence was no longer than two syllables, resulting in the mean length of 7.38 syllables per sentence (SD 0.75, range 5–8). In both paradigms, verb tense and subject gender could repeat in no more than two consecutive trials both within and across presentation blocks consisting of three sentences (see section “Procedure”), with one exception of three consecutive past tense forms per paradigm.
The sentences were selected from a set of 160 sentences tested in an online pilot study, where 100 participants (50 females, age: mean 38.3, SD 11.5, range 18–68 years) read the sentences and finished them aloud with a semantically plausible word within 5 s, matching the task timing in the fMRI study. Their responses were recorded and scored for accuracy by a single rater. A response was considered accurate if it was a semantically plausible single word in a grammatically correct form (direct object). Based on the results, 120 sentences were selected and split into two halves with similar accuracy, to be used in the SYLL and PW paradigm [SYLL: mean accuracy 90.8%, SD 5.8%, range 78–100%; PW: mean accuracy 89.9%, SD 5.4%, range 80–100%; t(118) = 0.842, p = 0.401].
The two final lists were matched for the gender of the subject (31 feminine, 29 masculine), sentence structure (type 1: n = 20, type 2: n = 19, type 3: n = 21), number of present and past verb forms (SYLL: 30 present/30 past forms, PW: 29 present/31 past forms), length in syllables [SYLL: mean 7.43, SD 0.76, range 5–8; PW: mean 7.31, SD 0.74, range 5–8; t(118) = 0.843, p = 0.400] and overall word frequency [SYLL: mean 39.37, SD 55.18, range 0.4–424.1; PW: mean 33.75, SD 45.71, range 0.5–277; t(358) = 1.051, p = 0.293].
In the SYLL baseline condition, stimuli were 60 phonologically legal Russian syllables (consonant + vowel), where the vowel was spelled multiple times in order to match the experimental condition for length in letters (for example, for the syllable “ϕe” – “fe”, the stimulus was «Φeeeϕeeeeeeϕeeeeee…» – «Feee feeeeee feeeeee…»). Participants were instructed to ignore the exact number of vowel letters and pronounce the syllable duration approximately. In the PW baseline condition, the stimuli were 60 pseudowords phonotactically legal in Russian, constructed to pairwise match the number of syllables in experimental sentences. All pseudowords were constructed as pseudonouns: that is, none of them had inflection typical for other parts of speech.
MRI Data Acquisition
MRI data were obtained on a Siemens Magnetom Skyra 3T scanner with a 20-channel head coil at the National Medical and Surgical Center named after N.I. Pirogov of the Ministry of Healthcare of the Russian Federation. Participants wore MRI-compatible headphones to reduce scanner noise and head movement. Visual stimuli were presented using head-coil mounted goggles (NordicNeuroLab, Bergen, Norway). Stimuli presentation was controlled with nordicAktiva, version 1.2.1. Oral responses were recorded with an MRI-compatible FOMRI III microphone (Optoacoustics LTD) using OptiMRI recording software, version 3.1.
For anatomical reference, T1-weighted MPRAGE structural images were acquired with the following parameters: voxel size 1.0 × 1.0 × 1.0 mm, 176 axial slices in ascending order, slice thickness 1.00 mm, field of view (FoV) 320 × 320 mm, repetition time (TR) 2200 ms, echo time (TE) 2.43 ms, flip angle 8°. Functional blood-oxygenation-level-dependent (BOLD) data were obtained using the following parameters: voxel size 3.0 × 3.0 × 3.0 mm, 30 oblique slices in interleaved order, slice thickness 3 mm, FoV 205 × 205 mm, TR 7000 ms, TE 30 ms, delay in TR 5000 ms (sparse sampling), flip angle 90°, 128 volumes per paradigm.
Procedure
Each participant was scanned on two separate occasions with an interval of 14 days. The first session began with a short instruction and practice to familiarize participants with the task outside the scanner. Inside the scanner, acquisition of T1 anatomical images was followed by the two functional paradigms (SYLL and PW). Their order was balanced across participants and remained constant in the two sessions. The duration of each functional paradigm was 14 min 56 s in total.
Each functional paradigm started with visually presented instructions followed by one training block per condition (not analyzed). Then, 120 stimuli (60 experimental and 60 baseline) were presented in blocks of three (Figure 1). A sparse sampling procedure was used (Hall et al., 1999). During a 5-s delay in TR, participants gave oral response to the current stimulus (Figure 1). They were instructed to remain silent during the next 2 s, indicated by a «!» sign. During this time, MR images were acquired. The sparse sampling procedure allowed to minimize motion-induced artifacts due to articulation and to monitor participants’ responses with no interference from the scanner acoustic noise. The opportunity to continuously monitor performance and engagement without any interference from the scanner noise is particularly relevant for future routine use in clinical populations, where task compliance may be compromised due to cognitive difficulties. A similar sparse sampling approach, with short scanning periods after stimulus presentation and/or response, has been successfully used in previous fMRI language localizers (Deblaere et al., 2002; Wilson et al., 2017; Nettekoven et al., 2018).

Figure 1. Experimental design of the functional paradigms. For illustration purposes, only stimuli from the SYLL paradigm are presented in the baseline block.
Data Analysis
Behavioral Data
The participants’ auditory responses from both sessions were transcribed, except for one participant’s responses that were lost due to technical error. Response accuracy (in both sessions) and response time (in the first session) were analyzed. Response accuracy was assessed independently by two raters. In the experimental condition, a response was considered accurate if it was a grammatically correct and semantically appropriate sentence completion. In the baseline conditions, a response was considered accurate if the participant read and repeated a syllable/pseudoword without any phonological errors. Inter-rater reliability, as assessed using percent agreement and Cohen’s kappa (Cohen, 1960), was high (1st session: 98.36% and 0.76, respectively; 2nd session: 98.62% and 0.69, respectively). All inconsistencies were resolved by discussion between the two raters.
Response time was measured by one rater using Praat software (Boersma, 2001). Specifically, the rater measured time to response completion: that is, time from the beginning of the trial (start of stimulus presentation) until the end of overt response. To test whether time to response completion differed across experimental conditions, we conducted a repeated-measures ANOVA with subsequent pairwise comparisons. Mauchly’s test was used to check the assumption of sphericity and the Greenhouse–Geisser correction was applied to correct for its violation.
Activation Maps
MRI data were analyzed using SPM121 for MATLAB 2014b. Prior to data analysis, first eight volumes of each functional paradigm, corresponding to instructions and training blocks, were discarded. For data preprocessing, images of each participant were first manually reoriented to the AC-PC plane. Then, functional scans were re-aligned to correct for head motion. Participants with excessive head movement (more than 5 mm in any direction) were excluded from further analysis. Functional images were coregistered to the anatomical T1 image, followed by spatial normalization of images to the International Consortium of Brain Mapping (ICBM) space template–European brains (Mazziotta et al., 1995) based on segmentation into six tissue types (gray matter, white matter, cerebrospinal fluid, bone, soft tissue and air/background) defined by tissue probability maps in SPM12. This step was followed by spatial smoothing with an isotropic 8-mm Gaussian kernel.
Statistical analysis was performed separately for each paradigm and each session, resulting in four activation maps for each participant. In the first-level (individual-level) analysis, a high-pass filter with a cut-off period of 256 s was employed to remove slow signal drift. The model included two conditions: experimental (sentence completion) and baseline (SYLL or PW, depending on the paradigm). The duration of each event was set to 7 s. Six movement parameters obtained in re-alignment were entered as regressors. A canonical hemodynamic response function with no derivatives was used to model BOLD response. Model estimation was done using a restricted maximum likelihood fit. T-contrast maps were computed separately for each paradigm, subtracting activation in the baseline condition (SYLL or PW) from activation in the experimental condition.
Although the present paper focuses on individual localization of language-related areas, we also conducted second-level (group) statistical analysis of the first session, aiming to establish which brain networks were consistently activated across participants. The contrast maps from the first-level analyses were submitted to the second-level one-sample t-test. To visualize the results at different levels of statistical stringency, three types of statistical thresholding were applied to all group fMRI activation maps: (1) the most conservative voxel-wise family-wise error correction (FWE) based on Gaussian random field theory at α = 0.05, as implemented in SPM12; (2) a more liberal cluster correction at voxel-wise α = 0.001 and cluster-wise α = 0.05 FWE-corrected, as implemented in SPM12 (resulting in the minimum cluster size k = 173 for the SYLL paradigm and k = 213 for the PW paradigm); and (3) adaptive thresholding (AT) method proposed by Gorgolewski et al. (2012), which combines Gamma-Gaussian mixture modeling with topological FDR thresholding at α = 0.05. The AT method takes into account the strength of the signal: it generates lower thresholds when the signal is weak, resulting in fewer false negative clusters, and higher thresholds when the signal is strong, resulting in fewer false positive clusters, aiming to ensure an optimal balance between Type I and Type II error rate (Gorgolewski et al., 2012).
Anatomical labels for activation clusters were determined based on the Brainnetome atlas (Fan et al., 2016) implemented in the ICN_atlas toolbox (Kozák et al., 2017) in SPM12. The Brainnetome atlas was selected due to detailed parcelation, as it includes 246 regions in total. Cerebellar activations were additionally labeled using the AAL atlas (Tzourio-Mazoyer et al., 2002) implemented in the ICN_atlas toolbox (Kozák et al., 2017) in SPM12. The activation maps were visualized in MRIcroGL 1.2.22.
Assessing Individual-Level Activation in Language-Related Regions of Interest
Since the ultimate goal of the localizer was to individually localize critical language areas, we estimated how well the paradigms were able to activate them in each participant. We focused on the following set of language-related regions of interest (ROIs) in the left hemisphere: pars triangularis of the inferior frontal gyrus (IFG), pars opercularis of the IFG, posterior middle frontal gyrus (pMFG), supplementary motor area (SMA), posterior middle temporal gyrus (pMTG), posterior superior temporal gyrus (pSTG), angular gyrus, and supramarginal gyrus. These ROIs were chosen theoretically as the most relevant for the sentence completion task. The IFG is implicated in numerous linguistic functions including morphosyntactic processing (Hagoort, 2005; Den Ouden et al., 2019), lexical selection (Zyryanov et al., 2020) and articulatory encoding (Flinker et al., 2015). pMFG has been recently discussed as crucial for speech fluency (Hazem et al., 2021). SMA has long been associated with the motor aspect of speech (Krainik et al., 2001). pMTG is implicated in semantic processing (Davey et al., 2016) and language comprehension (Turken and Dronkers, 2011). pSTG is primarily involved in phonological processing (Buchsbaum et al., 2001; Graves et al., 2008). The angular and supramarginal gyri are crucial for verb argument structure processing (Thompson et al., 2007); besides, the angular gyrus is known as a semantic hub (Price et al., 2016). Additionally, this set of ROIs largely overlaps with the one suggested by Benjamin et al. (2017) as most clinically relevant in neurosurgical planning.
At each of the three statistical thresholds applied in first-level analyses, we calculated which percentage of the resulting ROIs was activated by the paradigm, using the Brainnetome atlas (Fan et al., 2016) implemented in the ICN_atlas toolbox (Kozák et al., 2017). The respective list of areas from the Brainnetome atlas is listed in Supplementary Table S1 and illustrated in Figure 2. The resulting values were visualized using the seaborn library3 in Python 3.7.
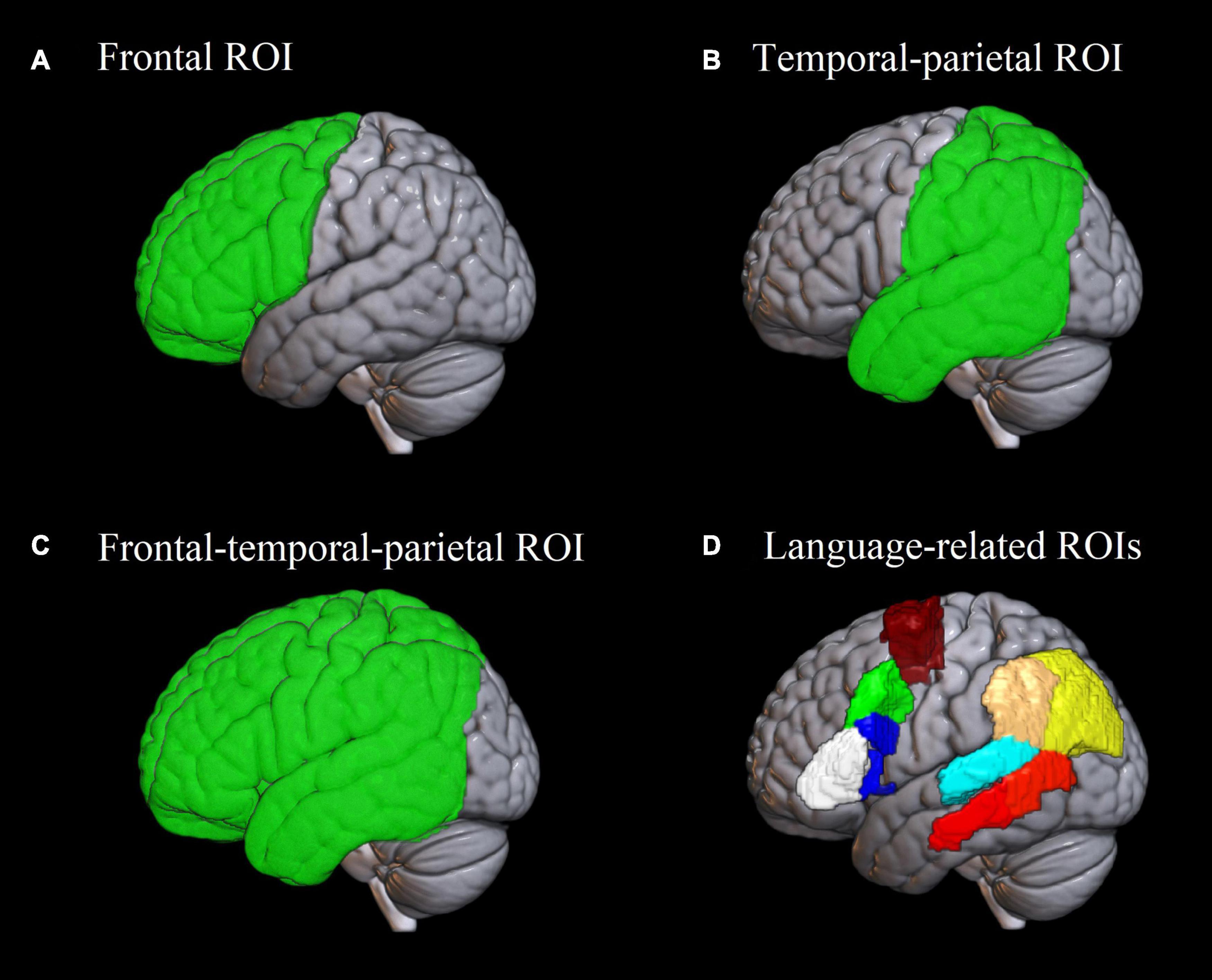
Figure 2. Regions of interest used in the analysis. (A–C) Frontal, temporal-parietal and frontal-temporal-parietal region of interest used in the analysis of Dice coefficients and lateralization indices. (D) Eight language-related regions of interest based on the Brainnetome atlas (Fan et al., 2016) implemented in the ICN_atlas toolbox (Kozák et al., 2017), used for assessing individual-level activation. White: pars triangularis of the inferior frontal gyrus; blue: pars opercularis of the inferior frontal gyrus; green: posterior middle frontal gyrus; burgundy: supplementary motor area; red: posterior middle temporal gyrus; cyan: posterior superior temporal gyrus; beige: supramarginal gyrus; yellow: angular gyrus.
To test how individual-level activation volume in the eight language-related ROIs was affected by baseline and statistical threshold, a separate repeated-measures ANOVA was conducted for each ROI. The ANOVAs were conducted on the number of significantly activated voxels in the ROI and tested the main effects of baseline and statistical threshold and their interaction. Mauchly’s test was used to check the assumption of sphericity. In case it was violated, Greenhouse–Geisser correction was applied. The Bonferroni correction was applied to correct for the number of statistical models, resulting in α = 0.00625.
Test–Retest Reliability
Consistency of localizer paradigms with regard to individual-level activation is crucial for clinical applications (Binder et al., 2008), so we estimated test-retest reliability of our paradigms. Following Wilson et al. (2017), we used the Dice coefficient (Rombouts et al., 1997) to quantify the similarity of language-related activation in the first and second session of each participant. The Dice coefficient indicated a degree of overlap between the participant’s activation maps in the first and second session and was calculated as follows: Dice = 2*Voverlap/(V1 + V2), where V1 and V2 denote the number of supra-threshold voxels in the first and second sessions of an individual and Voverlap is the total number of overlapping voxels. The overlap was calculated in Convert3D4, which is an extension of the ITK-SNAP tool (Yushkevich et al., 2006). The Dice coefficients can be interpreted as low (0.00 to 0.19), low-moderate (0.20 to 0.39), moderate (0.40 to 0.59), moderate-high (0.60 to 0.79) or high (0.80 to 1.00) (Wilson et al., 2017).
The Dice coefficients were calculated for each participant for the frontal, temporal-parietal and frontal-temporal-parietal regions (see Figure 2), separately for the SYLL and PW paradigm. Regions were defined using brain lobe masks available in the LI toolbox (Wilke and Lidzba, 2007) based on the atlas by Hammers et al. (2003). The frontal, temporal-parietal and frontal-temporal-parietal regions were selected because they correspond respectively to anterior language regions, posterior language regions and combination thereof, excluding the occipital lobe that is not relevant for the language function. To test what factors affected test-retest reliability, a repeated-measures ANOVA was conducted on Dice coefficients, testing the main effects of brain region, baseline and statistical threshold, as well as all interactions thereof. Mauchly’s test was used to check the assumption of sphericity. In case it was violated, Greenhouse–Geisser correction was applied.
Lateralization Indices
Finally, we evaluated the hemispheric lateralization of individual language-related activation. This analysis aimed to confirm the validity of the paradigms and estimate their reliability in establishing individual lateralization of language processing.
Lateralization indices were calculated with the LI toolbox for SPM (Wilke and Lidzba, 2007), based on the count and value of suprathreshold voxels and using adaptive thresholding. As implemented in the LI toolbox, adaptive thresholding uses averaged intensity of all voxels in the image as the internal threshold for a given participant, thus taking into account inter-subject variability of BOLD response. LI can take the values from +1 to –1, where +1 stands for full left lateralization of the activation, -1 indicates full right lateralization and 0 indicates bilateral activation. Similarly to Dice coefficients, LIs were calculated for the frontal, temporal-parietal and frontal-temporal-parietal regions (see Figure 2) for each participant individually, separately for each paradigm in each scanning session.
Given right-handedness of participants in our study, we expected to observe typical left-hemispheric dominance of language-related activation in the majority of participants. This outcome would confirm the validity of the paradigms. We also calculated Spearman’s correlation coefficients to assess the agreement of LIs in the two paradigms and two scanning sessions. Finally, we used a repeated-measures ANOVA to test how LIs were affected by baseline, region, number of session, and interactions thereof. Mauchly’s test was used to check the assumption of sphericity. In case it was violated, Greenhouse–Geisser correction was applied.
Results
Behavioral Results
Participants’ task performance was at ceiling. In the SYLL paradigm, the mean sentence completion accuracy was 96.1% (SD 3.1%, range 91.4–100.0%) in the first session and 98.4% (SD 1.6%, range 95.0–100.0%) in the second session. In the PW paradigm, the mean accuracy was 96.4% (SD 2.4%, range 93.1–100.0%) in the first session and 97.5% (SD 1.8%, range 94.9–100.0%) in the second session.
In the SYLL paradigm, the mean time to response completion was 3752 ms (SD 310, range 3176–4262 ms) in the experimental condition and 3834 ms (SD 681, range 2613–4698 ms) in the SYLL baseline condition. In the PW paradigm, the mean time to response completion was 3738 ms (SD 235, range 3315–4168 ms) in the experimental condition and 4160 ms (SD 335, range 3599–4756 ms) in the PW baseline condition. This proved that the response window (5 s) provided sufficient time for response in all conditions. A Greenhouse–Geisser-corrected repeated-measures ANOVA detected a significant effect of condition on time to response completion, F(1.26,20.17) = 4.24, p = 0.045. Pairwise comparisons indicated that the effect was driven by longer times to response completion in the PW baseline condition than in the experimental condition, p < 0.001. No other pairwise comparisons were significant.
Group-Level Activation Maps
Figure 3 demonstrates group-level activation maps from the first session produced at the three statistical thresholds. A full list of activation clusters comprising more than 100 voxels is presented in Supplementary Table S2 (SYLL paradigm) and Supplementary Table S3 (PW paradigm).
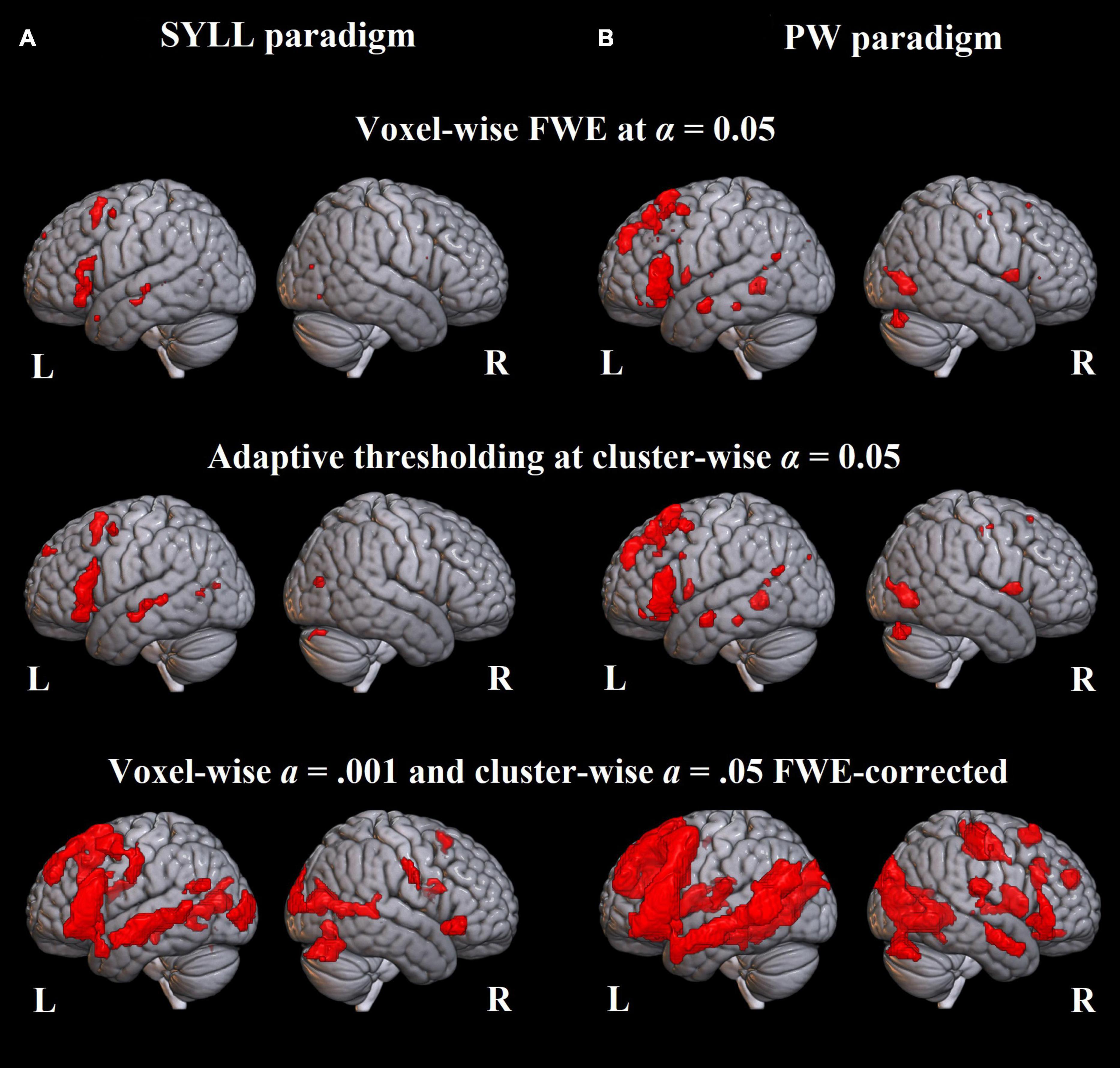
Figure 3. Language-related activation significant at the group level. (A) Paradigm with the syllable baseline. (B) Paradigm with the pseudoword baseline. Top: FWE correction for multiple comparisons at α = 0.05, middle: adaptive thresholding (AT) as implemented in Gorgolewski et al. (2012) at α = 0.05, bottom: cluster correction at voxel-wise α = 0.001 and cluster-wise α = 0.05 FWE-corrected.
At the most conservative statistical threshold (FWE correction; upper panel in Figure 3), both versions of the paradigm elicited significant group-level activation in the left inferior and superior frontal gyri. Additionally, the SYLL paradigm activated the orbital gyrus and insula and the PW paradigm activated the left middle temporal gyrus (MTG), left superior temporal gyrus (STG) and right cerebellum. With AT (Gorgolewski et al., 2012; middle panel in Figure 3), significant group-level activation in both paradigms extended to the left middle frontal gyrus (MFG). The activation additionally extended to the left MTG, left STG and right cerebellum in the SYLL paradigm and the orbital gyrus, insula, parts of occipital cortex and bilateral cerebellum in the PW paradigm. Finally, when using the most liberal cluster correction (bottom panel in Figure 3), activation in both paradigms extended to a wide network of left frontal, left temporal, bilateral occipital and bilateral cerebellar regions, particularly extensive with the PW paradigm.
For illustrative purposes, we also provide individual activation maps from three example participants (first session) in Supplementary Figures S1–S3.
Individual-Level Activation in Language-Related Regions of Interest
The violin plots in Figure 4 present the number of activated voxels in language-related ROIs across participants (as a percentage of the total number of voxels in the region). The respective numeric values are presented in Supplementary Table S4.
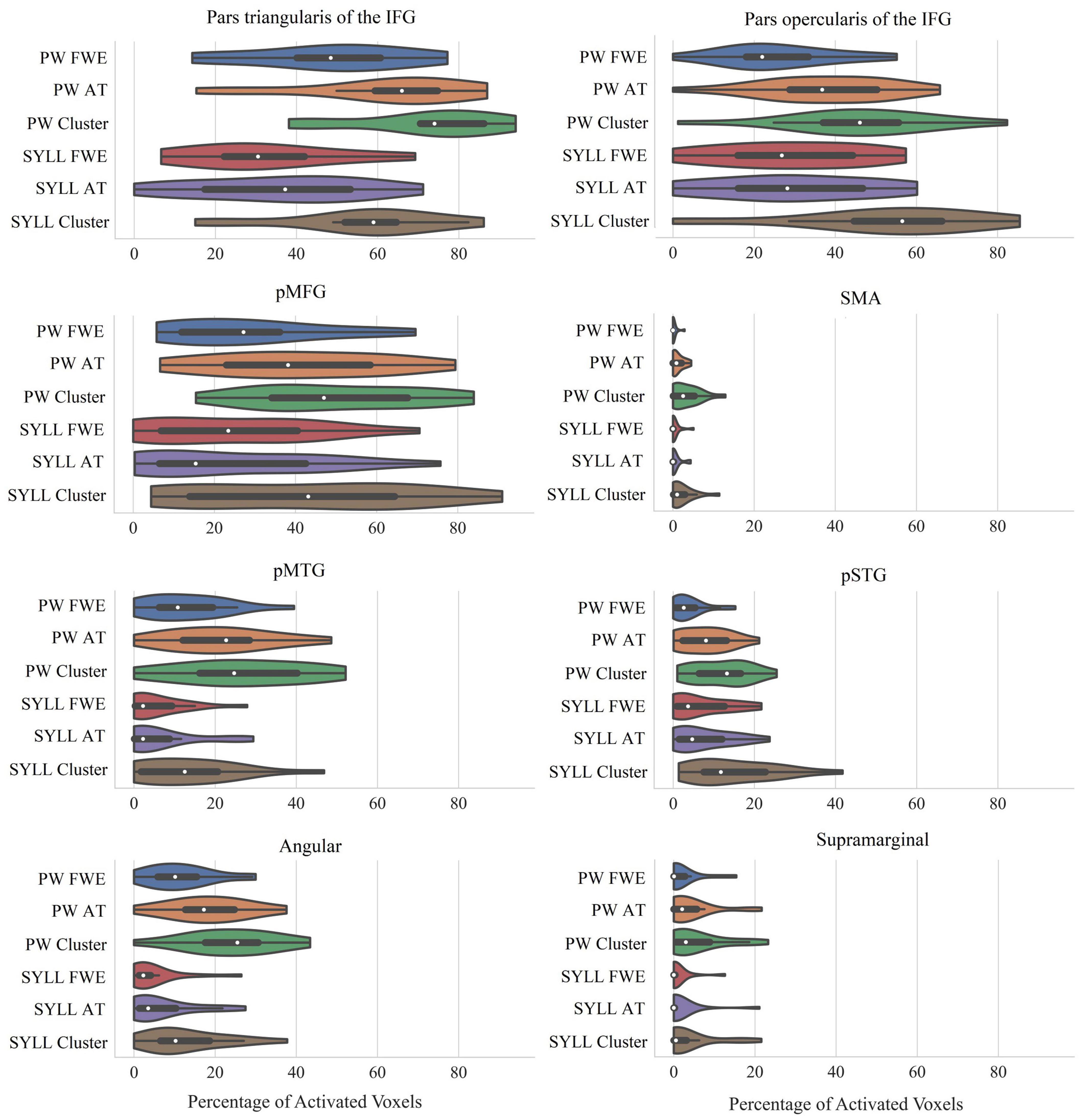
Figure 4. Percentage of significantly activated voxels in language-related ROIs depending on the paradigm (PW, pseudoword baseline; SYLL, syllable baseline) and threshold [FWE: FWE correction for multiple comparisons at α = 0.05; AT: adaptive thresholding as implemented in Gorgolewski et al. (2012) at α = 0.05; Cluster: cluster correction at voxel-wise α = 0.001 and cluster-wise α = 0.05 FWE-corrected]. The white dot in the middle of each “violin” represents the median value and the thick black bar in the center represents the interquartile range. IFG, inferior frontal gyrus; pMFG, posterior middle frontal gyrus; SMA, supplementary motor area; pMTG, posterior middle temporal gyrus; pSTG, posterior superior temporal gyrus; Angular, angular gyrus; Supramarginal, supramarginal gyrus.
As seen in Figure 4, individual-level activation was the most extensive in pars triangularis of the IFG (median activation ranging from 31 to 74% depending on the paradigm and threshold) and pars opercularis of the IFG (median activation ranging from 22 to 57%). Individual-level activation was also extensive in the pMFG (median activation ranging from 15 to 47%) and pMTG (median activation ranging from 2 to 25%). In other analyzed regions, individual-level activation was less extensive.
Bonferroni-corrected repeated-measures ANOVAs showed that individual-level activation volume was greater with the PW than SYLL baseline in three language-related ROIs: pars triangularis of the IFG, F(1,17) = 11.84, p = 0.003, pMTG, F(1,17) = 12.95, p = 0.002, and angular gyrus, F(1,17) = 23.45, p < 0.001. In the other five language-related ROIs, individual-level activation volume was not significantly affected by baseline. Expectedly, individual-level activation volume was significantly affected by statistical threshold in all language-related ROIs: pars triangularis of the IFG, F(1.37,23.28) = 68.21, p < 0.001, pars opercularis of the IFG, F(1.43,24.29) = 57.48, p < 0.001, pMFG, F(1.39,23.66) = 43.48, p < 0.001, SMA, F(1.32,22.45) = 26.54, p < 0.001, pMTG, F(2,34) = 32.97, p < 0.001, pSTG, F(2,34) = 47.85, p < 0.001, angular gyrus, F(2,34) = 45.34, p < 0.001, and supramarginal gyrus, F(2,34) = 13.33, p < 0.001. Post hoc pairwise comparisons showed that the main effects of statistical threshold were driven by two effects. First, in all ROIs, the cluster correction at voxel-wise α = 0.001 and cluster-wise α = 0.05 FWE-corrected yielded more significantly activated voxels than the other two statistical thresholds (all p < 0.001). Second, in three ROIs (pars triangularis of the IFG, pSTG, angular gyrus), adaptive thresholding as implemented in Gorgolewski et al. (2012) at α = 0.05 yielded more significantly activated voxels than the FWE correction at α = 0.05 (all p < 0.005). In pMTG, the latter effect was only significant with the PW baseline, driving a significant interaction between baseline and statistical threshold, F(2,34) = 6.87, p = 0.003. No other interactions between baseline and statistical threshold were significant.
Test–Retest Reliability
Mean Dice coefficients quantifying the overlap of individual activation in the first and second scanning session are presented in Table 1. Mean Dice coefficients ranged from 0.39 to 0.60, that is, from low-moderate to moderate-high. A Greenhouse–Geisser-corrected repeated-measures ANOVA demonstrated a significant effect of brain region, F(1.03,17.58) = 8.83, p = 0.008. Post hoc pairwise comparisons showed that Dice coefficients were significantly higher in the frontal (p = 0.009) and frontal-temporo-parietal (p = 0.005) than the temporo-parietal region. Dice coefficients were significantly higher with the PW than SYLL baseline, F(1,17) = 5.75, p = 0.028. Finally, there was a significant effect of statistical threshold, F(1.24,21.00) = 4.42, p = 0.041. Post hoc pairwise comparisons showed that Dice coefficients were higher with the most liberal statistical threshold (cluster correction at voxel-wise α = 0.001 and cluster-wise α = 0.05 FWE-corrected) relative to FWE correction for multiple comparisons at α = 0.05 (p < 0.001) and relative to the adaptive thresholding as implemented in Gorgolewski et al. (2012) at α = 0.05 (p = 0.037). No interactions were significant.
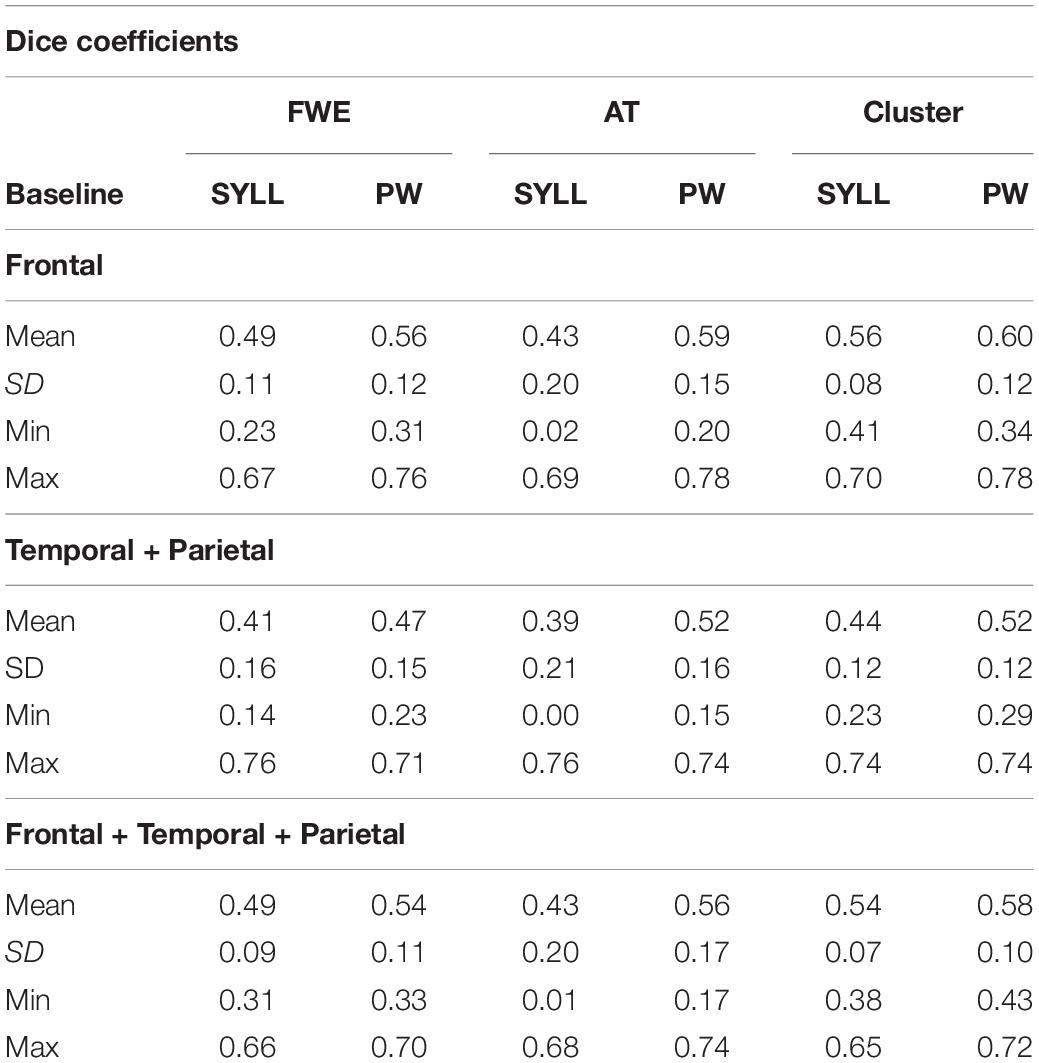
Table 1. Dice coefficients in the two paradigms (SYLL: syllable baseline vs. PW: pseudoword baseline) in three regions (frontal, temporal-parietal, and frontal-temporal-parietal) at three statistical thresholds [FWE, FWE correction for multiple comparisons at α = 0.05; AT, adaptive thresholding as implemented in Gorgolewski et al. (2012) at α = 0.05; Cluster, cluster correction at voxel-wise α = 0.001 and cluster-wise α = 0.05 FWE-corrected].
The range of Dice coefficients in Table 1, varying between 0 and 0.78, indicated substantial inter-individual variability. Low Dice coefficients around 0 resulted exclusively following the application of the AT method suggested by Gorgolewski et al. (2012). In some cases, no or almost no voxels survived the statistical threshold established by this method in one of the two participant’s sessions.
Lateralization Indices
Table 2 presents mean LIs for three regions (frontal, temporal-parietal and frontal-temporal-parietal) for the two paradigms (SYLL and PW) in the two scanning sessions. Table 2 also includes Spearman’s correlation coefficients examining the reproducibility of individual LI values across two sessions, as well as their agreement in the two paradigms.
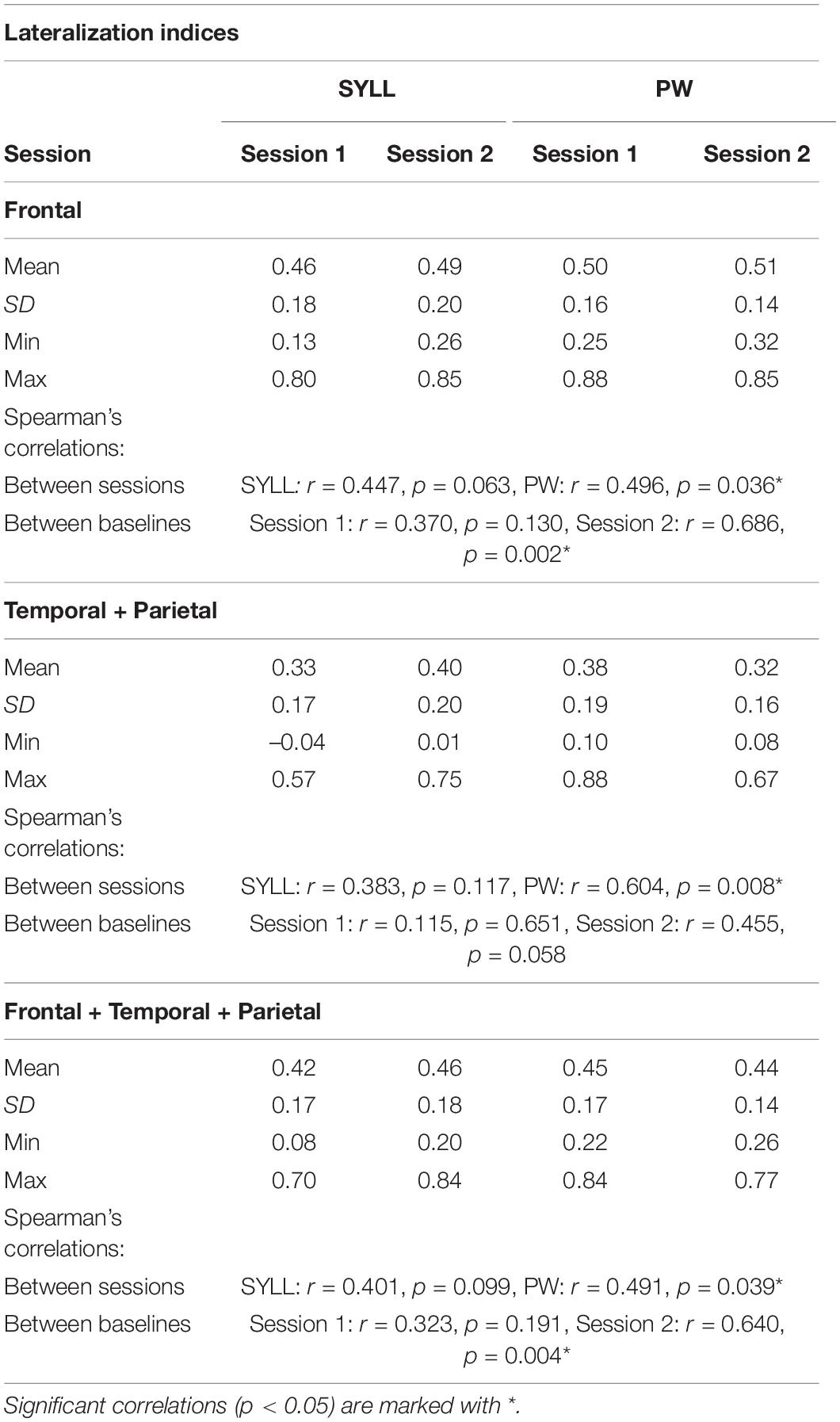
Table 2. Lateralization indices for each paradigm (SYLL: syllable baseline vs. PW: pseudoword baseline) in three regions (frontal, temporal-parietal, frontal-temporal-parietal) along with results of Spearman’s correlations between LIs in the two paradigms and scanning sessions.
Mean LIs in all regions across two scanning sessions showed left lateralization regardless of the paradigm (SYLL or PW), ranging from 0.32 to 0.51. Individual LIs ranged between very strong left-hemispheric dominance (0.88) and bilateral organization (–0.04). Minimum individual LIs showing bilateral organization were observed mostly in the temporal-parietal region in both sessions and for both paradigms.
With regard to reproducibility, LIs in the two experimental sessions were significantly correlated in the PW paradigm in all regions (all p < 0.05). In the SYLL paradigm, the correlation between LIs in the two experimental sessions in all regions remained at the level of a statistical trend. With regard to agreement between the two paradigms, LIs in the SYLL and PW paradigm were significantly correlated only in the frontal and frontal-temporal-parietal region in the second session (all p < 0.05). The correlations between LIs in the two paradigms in the first session and in the temporal-parietal region in the second session were not significant.
A repeated-measures ANOVA showed a significant three-way interaction between baseline, region and session, F(1.11,18.93) = 8.92, p = 0.006. To address this interaction, separate repeated-measures ANOVAs were performed for the SYLL and PW baseline [there was no main effect of baseline, F(1,17) = 0.06, p = 0.811]. With the SYLL baseline, a main effect of region was significant, F(1.12,18.99) = 15.88, p = 0.001, with higher LIs in the frontal than temporo-parietal (p = 0.001) or fronto-temporo-parietal region (p = 0.038) and in the fronto-temporo-parietal than temporo-parietal region (p < 0.001). No other main effects or interactions were significant. With the PW baseline, a two-way interaction of session and region was significant, F(1.08,18.31) = 6.71, p = 0.017, so separate repeated-measures ANOVAs were performed for the first and second session [there was no main effect of session, F(1,17) = 0.26, p = 0.618]. In the first session with the PW baseline, the main effect of region was significant, F(1.07,18.19) = 15.21, p = 0.001. Post hoc pairwise comparisons showed the same hierarchy of LIs as with the SYLL baseline: LIs were higher in the frontal than temporo-parietal (p = 0.001) and frontal-temporo-parietal region (p = 0.006) and in the frontal-temporo-parietal than temporo-parietal region (p = 0.001). In the second session with the PW baseline, the main effect of region was again significant but much greater, F(1.09,18.52) = 80.49, p < 0.001. Post hoc pairwise comparisons showed the same hierarchy of LIs as for the first session or for the SYLL baseline: LIs were higher in the frontal than temporo-parietal (p < 0.001) and fronto-temporo-parietal region (p < 0.006) and in the frontal-temporo-parietal than temporo-parietal region (p < 0.001). That is, the significant three-way interaction between baseline, region and session was driven by the main effect of region being the greatest in the second session with the PW baseline.
Discussion
We presented a new fMRI language localizer for preoperative language mapping in Russian-speaking individuals. Following the world’s best practices (Zacà et al., 2012; Barnett et al., 2014; Black et al., 2017; Połczyńska et al., 2017; Salek et al., 2017; Wilson et al., 2017; Unadkat et al., 2019), the paradigm used a sentence completion task that uniquely engages both language production and comprehension at the word and sentence level. The current study validated the localizer paradigm in a control group of neurologically healthy individuals. In this group, the paradigm successfully activated language-related ROIs, elicited expected left-hemispheric lateralization and showed test–retest reliability comparable to previous studies. Apart from demonstrating general validity and reliability of the paradigm, we compared two different baseline conditions (SYLL and PW), for the first time for the sentence completion task. All outcomes were reported at three statistical thresholds.
Activation of Language-Related Regions of Interest
At the group level, both versions of the localizer (with the SYLL and PW baseline) elicited significant activation in an expected network of language-related areas. At the most stringent statistical threshold (FWE correction at α = 0.05), both versions of the paradigm elicited significant activation in the left posterior inferior and posterior superior frontal gyri (differences in results with the SYLL and PW baseline are discussed in section “Comparison of baseline conditions”). The left IFG has been implicated in many linguistic processes engaged by the sentence completion task: sentence parsing (Hagoort, 2005), conceptual and lexical selection of the completing word (Robinson et al., 2010; Zyryanov et al., 2020), morphosyntactic inflection of the completing word (Den Ouden et al., 2019), and articulatory encoding (Flinker et al., 2015). Such multifaceted involvement of the left IFG in linguistic processes may explain why its activation was the most statistically robust. The posterior superior frontal gyrus (premotor to supplementary motor cortex) was likely activated as part of the dorsal route supporting articulation (Hickok and Poeppel, 2007) or speech initiation (Kinoshita et al., 2015; Dragoy et al., 2020).
At a more liberal statistical threshold [AT at α = 0.05 as implemented by Gorgolewski et al. (2012)], activation in both versions of the localizer (with the SYLL and PW baseline) extended to the left MFG and to a new cluster of activation encompassing the mid-posterior portions of the left MTG and superior temporal sulcus (STS). More superior and posterior portions of the temporal activation may pertain to phonological processing (Buchsbaum et al., 2001; Graves et al., 2008), whereas activation in the mid part of the MTG may reflect several components of semantic processing, such as storage of heteromodal semantic knowledge (Binder et al., 2009), linkage of word forms to meanings (Hickok and Poeppel, 2007; Bonilha et al., 2017), and semantic control when searching for a completing word (Davey et al., 2016).
Finally, at the most liberal statistical threshold (cluster correction at voxel-wise α = 0.001 and cluster-wise α = 0.05 FWE-corrected), activation extended to a broad left-lateralized frontotemporal and bilateral occipital network. Occipital activation may pertain to reading, including the linkage between visual word processing and phonological word representations (Richardson et al., 2011; Mano et al., 2013): although the baseline condition also required reading, it did not involve any linkage to word representations. Alternatively, the greater occipital activation in the experimental condition may reflect mental imagery of the sentence content (Pearson et al., 2015).
Therefore, group-level results proved that the sentence completion paradigm successfully activated both anterior and posterior areas implicated in language processing. This is in line with previous empirical work (Zacà et al., 2012; Barnett et al., 2014; Połczyńska et al., 2017; Salek et al., 2017; Wilson et al., 2017; Unadkat et al., 2019) and reviews (Black et al., 2017; Manan et al., 2020) promoting sentence-level tasks and particularly sentence completion for eliciting activation in a more comprehensive language network than with word-level tasks. However, significant group-level clusters may result from different individual-level patterns: consistent activation across all/most participants versus strong activation in fewer participants. For clinical use, it is most crucial whether the paradigm consistently elicits significant activation of language-related areas in each tested individual, so that individual maps of language-related areas can be routinely used by a neurosurgeon.
To test this, we analyzed individual-level activation in each participant’s first session in eight language-related ROIs. Mirroring the group-level findings, individual-level activation was the most consistent in pars triangularis of the IFG, followed by pars opercularis of the IFG, followed by the pMFG. Among these, pars triangularis of the IFG was to some extent activated in each participant (with an exception of the AT statistical thresholding with the SYLL baseline). As represented by the interquartile range of activation area, activation spanned one third to two thirds of this area in most participants. Similarly, most participants showed activation in about one fifth to one half of pars opercularis of the IFG and in the pMFG. In the vast majority of participants, significant individual-level activation was also present in the pMTG, followed by the pSTG and the angular gyrus. The successfully activated ROIs are listed by Benjamin et al. (2017) among critical language areas to be mapped before neurosurgery, so the paradigms proved suitable for localizing most clinically relevant areas.
On the other hand, most participants did not show significant individual-level activation in the SMA or supramarginal gyrus. The lack of consistent individual-level activation in the supramarginal gyrus was surprising, given that activation at the temporo-parietal junction is expected in sentence-level tasks (Połczyńska et al., 2017) and has sometimes also been found with word-level tasks (Roux et al., 2003; Stippich et al., 2007). Still, the majority of participants in the present study showed activation in the adjacent angular gyrus. The lack of consistent individual-level activation in the SMA may be driven by comparable articulatory demands in the experimental and baseline conditions. Non-automatized articulation of meaningless syllables and particularly pseudowords may place high demands on phonological-articulatory programming. Likely, these demands in the baseline condition are equal or higher compared to more automatized articulation of familiar words in the experimental condition, therefore neither the SYLL nor the PW paradigm systematically detected articulation-related activation in the SMA.
Lateralization of Language Processing
All participants in the present study were right-handed with no history of neurological disorders. Thus, we expected that the paradigm should elicit primarily left-hemispheric lateralization of language processing, with a certain degree of individual variability (Springer et al., 1999; Knecht et al., 2000). Indeed, mean LI values indicated left-hemispheric lateralization of task-related brain activity, with individual values ranging between bilateral organization and very strong left lateralization. Thus, the ability of the paradigm to detect hemispheric lateralization of language processing activity was confirmed. Numerically, the LI values (mean 0.32 to 0.51, depending on the brain region and baseline) were comparable to those in previous studies with neurologically healthy right-handed participants. For example, Deblaere et al. (2002) found individual LI values from –0.08 to 0.58 across four language tasks; Dodoo-Schittko et al. (2012) found mean LI values of 0.44 and 0.45 in a verb and antonym generation tasks respectively (for review, see Bradshaw et al., 2017).
Interestingly, language-related activity was significantly more strongly left-lateralized in the frontal (and, correspondingly, frontal-temporal-parietal) than temporal-parietal region. This is in line with Tailby et al. (2017) who also found stronger language lateralization in anterior than posterior brain regions in healthy individuals using three tasks: orthographic lexical retrieval, noun-verb generation and pseudoword rhyming. More broadly, this is consistent with contemporary models of auditory language processing (Hickok and Poeppel, 2007; Price, 2010; Peelle, 2012). Although our task did not involve auditory comprehension, our findings converge with them with regard to stronger left-hemispheric lateralization of language processing in the frontal than temporal lobe. For example, the dual-stream model (Hickok and Poeppel, 2007) postulates a bilaterally organized ventral stream, which primarily involves the temporal lobe and connects speech sounds to meanings, and a left-lateralized dorsal stream, which extends to the frontal lobe and maps speech sounds to articulatory networks. In the same vein, Peelle (2012) argues that phonological and lexical information are processed bilaterally in the temporal lobe, whereas sentence processing engages a left-lateralized pathway including the left IFG. Possibly, the same general pattern of stronger language lateralization in anterior brain regions applies beyond the auditory modality of language processing.
Test–Retest Reliability
Dice coefficients measuring the spatial overlap of significant activation in the individual’s first and second scanning session were in the moderate range: 0.39 to 0.60, depending on the ROI, baseline condition and statistical threshold. In the only previous study measuring Dice coefficients for the sentence completion task (Wilson et al., 2017), the coefficients indicated a smaller spatial overlap, ranging between 0.06 and 0.47 depending on the region of interest and statistical threshold. As a more specific example, at the statistical threshold of α = 0.001 with the minimum cluster size of 2 cm2, the mean Dice coefficient in the broadest examined region of interest [“supratentorial region” in Wilson et al. (2017) and the combination of frontal, temporal and parietal lobe in the present study] was 0.34 in Wilson et al. (2017) versus 0.43 or 0.56 with the SYLL and PW baseline respectively in the present study. The Dice coefficients in the present study were also comparable to those reported in previous studies for other language tasks in neurologically healthy participants, presented in Table 3, and to the mean overlap of 0.48 across a variety of tasks established in a meta-analysis by Bennett and Miller (2010).
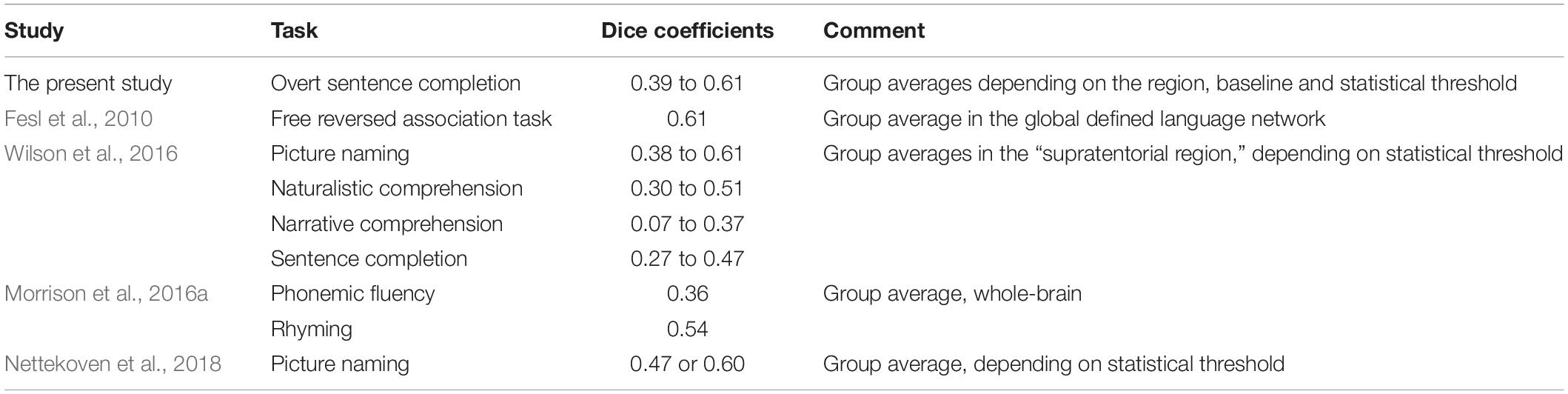
Table 3. Comparison of Dice coefficients to previous fMRI paradigms using language tasks in neurologically healthy participants.
Test–retest reliability was affected by the brain region and statistical threshold. Dice coefficients were significantly higher in the frontal (and, correspondingly, frontal-temporal-parietal) than temporal-parietal region. This is consistent with higher Dice coefficients in the frontal than temporal or parieto-occipital region of interest in a free reversed association task by Fesl et al. (2010). Conversely, Nettekoven et al. (2018) showed higher Dice coefficients for a picture naming task in the IFG than the STG. Possibly, this discrepancy could arise because Nettekoven et al. (2018) used smaller ROIs than here and in Fesl et al. (2010). With regard to the statistical threshold, Dice coefficients were higher with the most liberal than two more conservative statistical thresholds, in line with previous literature (Stevens et al., 2013; Wilson et al., 2017; Nettekoven et al., 2018).
Hemispheric lateralization of language-related activity also showed moderate test–retest reliability. LIs in the first and second scanning session showed either a significant moderate-to-strong correlation (with the PW baseline) or a statistical trend for a moderate correlation (with the SYLL baseline). This held true when LIs were calculated for the frontal region, temporal-parietal region, and combination thereof. The findings on moderate reliability of the paradigm in identifying both localization and hemispheric lateralization of language-related activity contribute to the literature on general test–retest reliability of fMRI (Bennett and Miller, 2010; Holiga et al., 2018; Elliott et al., 2020).
Comparison of Baseline Conditions
Each participant was administered two versions of the paradigm with different baselines: reading a sequence consisting of the same syllable and repeating the syllable once more (SYLL baseline) and reading a sequence of pseudowords and repeating any of them once (PW baseline). Both baselines are theoretically plausible for the sentence completion task, yet no previous studies have empirically investigated how their choice may affect the outcomes.
The SYLL and PW baselines showed a similar spatial distribution of significantly activated areas at the group level (see section “Activation of language-related ROIs”). Among minor differences in the spatial distribution, one may highlight somewhat more inferior temporal activation with the PW baseline: it largely extended to the inferior temporal sulcus and gyrus, whereas significant activation with the SYLL baseline encompassed more of the STS and STG. Possibly, this pattern emerged because the PW baseline exactly matched the experimental condition in phonological complexity. Therefore, their comparison yielded significant activations in more inferior temporal areas implicated in lexical-semantic processing (Binder et al., 2009; Davey et al., 2016) but not in more superior temporal areas enabling phonological processing (Buchsbaum et al., 2001; Graves et al., 2008).
With regard to the extent of activation, the area of significant group-level activation was somewhat greater with the PW than SYLL baseline across statistical thresholds. This was unexpected: we hypothesized that subtraction of the PW baseline should have yielded a smaller difference from the experimental condition because the PW baseline matched it closer in terms of phonological complexity and real-word neighbors and associations. One possible explanation are differences in how individual participants approached the SYLL baseline: for example, how accurately they tried to pronounce the length (number of vowels) in the string and whether they imposed prosody when reading. Possibly, such individual variability could introduce noise in the data, reducing the statistical power of comparison to the experimental condition. Another possible account is that the simpler SYLL baseline allowed more time and cognitive resources for non-task-related cognitive activity, which could also introduce noise in the data.
At the individual level, the paradigms with the SYLL and PW baseline also showed a very similar pattern. With both baselines, activation was most robust across individuals in the IFG and pMFG, followed by the pMTG, pSTG and the angular gyrus, whereas the basal temporal area and the supramarginal gyrus showed no activation in most participants (see section “Activation of language-related ROIs”). Mirroring the group-level results, areas of individual activation were numerically lower with the SYLL than PW baseline in most ROIs. This difference was significant in pars triangularis of the IFG, pMTG, and angular gyrus. With regard to hemispheric lateralization, the comparison of the SYLL and PW paradigms showed mixed results. On the one hand, LI values did not significantly differ between the two paradigms. On the other hand, they did not consistently show a significant correlation either, indicating a somewhat different pattern of lateralization elicited with the SYLL and PW baseline.
Finally, test–retest reliability, or spatial overlap between significant activation in the participant’s first and second scanning session, was significantly higher with the PW than SYLL baseline. Test-retest reliability of hemispheric lateralization was also higher with the PW baseline. With the PW baseline, the LIs in the first and second session showed a significant moderate-to-high correlation, whereas with the SYLL baseline, they remained at the level of a statistical trend for moderate correlation. This held true for the frontal region, temporal-parietal region and combination thereof.
To summarize, the PW baseline provided more robust activation, as reflected in somewhat more extensive significant activation and higher test–retest reliability. As discussed above, the cognitively simpler SYLL baseline may have allowed more time and cognitive resources for non-task-related cognitive activity or, alternatively, have provoked interindividual variability in the specifics of task performance. Both could introduce noise in the data and reduce statistical power compared to the more cognitively taxing PW baseline. On the other hand, this quantitative difference was not large, and the SYLL baseline appeared to have a qualitative advantage. Namely, posterior temporal activation with the SYLL baseline encompassed more superior areas than with the PW baseline. Damage to pSTG impairs phonological processing (Binder, 2015) and possibly word comprehension, although with some potential for neuroplasticity (Hillis et al., 2017), so its mapping is crucial.
Apart from potentially better mapping of the posterior temporal region, the SYLL baseline has the advantage of being cognitively simpler and thus more feasible in the clinical population. Here in the control group of neurologically healthy participants, task accuracy was at ceiling in both versions of the paradigm. However, time to response completion was significantly longer in the PW baseline condition (but not SYLL baseline condition) than in the experimental condition. First, this confounds the interpretation of results in the PW paradigm. Any significant group- and individual-level activation in the paradigm could be driven by different response timing and task difficulty in the experimental and baseline condition (Yarkoni et al., 2009) rather than by target linguistic processes. Second, this reflects cognitive difficulty of the PW baseline condition even for neurologically healthy participants, due to non-lexical reading and non-automated articulation of pseudowords. The cognitive difficulty of the PW baseline may present a particular problem in case of preoperative neuropsychological and linguistic deficits in patients with brain tumors (Ek et al., 2010; Racine et al., 2015) and epilepsy (Patrikelis et al., 2016). Thus, lower cognitive complexity and thus greater feasibility may present an important clinical advantage of the SYLL baseline, despite greater robustness of the PW baseline in the control group.
Comparison of Statistical Thresholds
We reported all measures and activation maps at three different statistical thresholds. The most conservative was the FWE correction for multiple comparisons at α = 0.05, followed by the AT method proposed by Gorgolewski et al. (2012) at α = 0.05, followed by the most liberal cluster correction at voxel-wise α = 0.001 and cluster-wise α = 0.05 FWE-corrected. Many previous studies have also reported results at multiple statistical thresholds (Dodoo-Schittko et al., 2012; Nadkarni et al., 2015; Morrison et al., 2016a; Wilson et al., 2017; Nettekoven et al., 2018), since there is no “gold standard” for statistical thresholding in individual or group-level fMRI analysis. Moreover, studies have shown that the statistical threshold may vastly impact metrics induced from fMRI analysis, such as LIs (Nadkarni et al., 2015) or test–retest reliability metrics (Stevens et al., 2016), so reporting results at only one threshold could be misleading.
In the present study, the spatial distribution of activation both at the group and individual level was expectedly similar across statistical thresholds, although some relevant clusters of activation only emerged at more liberal statistical thresholds. For example, significant group-level activation in the pSTG became evident at the two more liberal statistical thresholds, and significant group-level activation in the angular and particularly supramarginal gyrus mainly emerged at the most liberal statistical threshold.
At the individual level, participants highly varied in the extent of activation depending on the statistical threshold: the extent of activation that was present in some participants at the most stringent threshold only appeared in others at more liberal thresholds (Supplementary Table S4). This adds to the evidence for impossibility of using a one-for-all statistical threshold in individual preoperative mapping in clinical practice. Various methods have been proposed in previous literature for individualized statistical thresholding. They have been based, for example, on receiver operating characteristic reliability (Stevens et al., 2016), normalizing statistical maps to the local peak activation amplitude within a brain region (Voyvodic et al., 2009; Gross and Binder, 2014), thresholding based on a fixed percentage of brain activation rather than a statistical threshold (Wilson et al., 2017), and expert judgment by a clinician (American College of Radiology, 2014; Benjamin et al., 2017, 2018). In the present study, we reported the results using one method of individualized thresholding: the AT method by Gorgolewski et al. (2012), which is based on the combination of Gamma-Gaussian mixture modeling with topological FDR thresholding. The AT method did not alleviate individual variability in the extent of activation: the percentage of activation in language-related ROIs was not more homogeneous across participants when using the AT method than the two non-adaptive thresholding methods (Figure 4). For clinical practice, this means that the AT method would not solve the issue of largely variable activation strength across individuals that confounds the interpretation of the presence or absence of significant activation in an area. An important research direction, which was beyond the scope of the present study, would be to compare other methods of individualized statistical thresholding.
Test–retest reliability, as measured by Dice coefficients, was in the moderate range across statistical thresholds. Still, Dice coefficients were significantly higher with the most liberal statistical threshold compared to the two more conservative statistical thresholds, in line with previous literature (Stevens et al., 2013; Wilson et al., 2017; Nettekoven et al., 2018). With regard to LIs, these were calculated using adaptive thresholding and taking into account the values of suprathreshold voxels as implemented in the LI Toolbox for SPM (Wilke and Lidzba, 2007), so comparison of different statistical thresholds did not apply to this measure.
Limitations and Future Directions
The present study validated the fMRI language localizer in a control group of 18 neurologically healthy participants. The sample size is a limitation of the study: recent modeling studies (Cremers et al., 2017) suggest that samples of more than 20 participants are needed to localize even strong effects, whereas weaker and more diffuse effects require even greater sample sizes.
For full validation of the localizer, the crucial next step is to test it in the clinical group of presurgical patients with brain tumors and drug-resistant epilepsy. Data from a clinical sample will test the ability of the localizer to elicit activation in critical language-related areas in patients with different etiology and localization of pathological tissue and thus ultimately assess its clinical value. Data from a clinical sample would also provide the best test case for assessing the clinical value of different methods of individualized statistical thresholding (Voyvodic et al., 2009; American College of Radiology, 2014; Gross and Binder, 2014; Stevens et al., 2016; Benjamin et al., 2017, 2018; Wilson et al., 2017), which remained beyond the scope of the present study.
Finally, as a validation against the gold standard, the findings of the fMRI language localizer in the clinical group will need to be compared to the findings from intraoperative mapping using DES. So far, such comparisons between DES and fMRI language localizer protocols have yielded diverging results (Roux et al., 2003; Spena et al., 2010; Morrison et al., 2016b; for review, see De Witte and Mariën, 2013). Thus, it would be informative to validate our particular fMRI language localizer protocol against DES and thereby add to general evidence on the sensitivity and specificity of fMRI language localizer protocols for preoperative language mapping.
Data Availability Statement
The raw data supporting the conclusions of this article will be made available by the authors, without undue reservation.
Ethics Statement
The studies involving human participants were reviewed and approved by the HSE Committee on Interuniversity Surveys and Ethical Assessment of Empirical Research. The patients/participants provided their written informed consent to participate in this study.
Author Contributions
KE and SM contributed equally to the manuscript and wrote sections of the manuscript. OD conceptualized, designed, and supervised the study. ES and OD created linguistic materials. SM and OB implemented and tested the paradigm. KE, SM, OB, and AM collected the data. KE, SM, and AZ performed the data analysis. All authors contributed to manuscript revision, read and approved the submitted version.
Funding
The publication was supported by the grant for research centers in the field of AI provided by the Analytical Center for the Government of the Russian Federation (ACRF) in accordance with the agreement on the provision of subsidies (identifier of the agreement 000000D730321P5Q0002) and the agreement with HSE University No. 70-2021-00139.
Conflict of Interest
The authors declare that the research was conducted in the absence of any commercial or financial relationships that could be construed as a potential conflict of interest.
Publisher’s Note
All claims expressed in this article are solely those of the authors and do not necessarily represent those of their affiliated organizations, or those of the publisher, the editors and the reviewers. Any product that may be evaluated in this article, or claim that may be made by its manufacturer, is not guaranteed or endorsed by the publisher.
Acknowledgments
We would like to thank Evgenii Kalenkovich, Olga Buivolova, and Valeriya Zelenkova for their help with paradigm development, and all study participants for their contribution.
Supplementary Material
The Supplementary Material for this article can be found online at: https://www.frontiersin.org/articles/10.3389/fnhum.2022.791577/full#supplementary-material
Footnotes
- ^ http://www.fil.ion.ucl.ac.uk/spm/software/spm12/
- ^ https://www.nitrc.org/projects/mricrogl
- ^ https://seaborn.pydata.org/
- ^ http://www.itksnap.org/pmwiki/pmwiki.php?n=Downloads.C3D
References
Agarwal, S., Sair, H. I., Gujar, S., and Pillai, J. J. (2019). Language mapping with fMRI. Top. Magn. Reson. Imaging 28, 225–233. doi: 10.1097/rmr.0000000000000216
Almairac, F., Duffau, H., and Herbet, G. (2018). Contralesional macrostructural plasticity of the insular cortex in patients with glioma: a VBM study. Neurology 91, e1902–e1908. doi: 10.1212/WNL.0000000000006517
American College of Radiology (2014). ACR–ASNR–SPR Practice Parameter for the Performance of Functional Magnetic Resonance Imaging (fMRI) of the brain. Amended 2014 (Resolution 39). Reston, VA: American College of Radiology.
Barnett, A., Marty-Dugas, J., and McAndrews, M. P. (2014). Advantages of sentence-level fMRI language tasks in presurgical language mapping for temporal lobe epilepsy. Epilepsy Behav. 32, 114–120. doi: 10.1016/j.yebeh.2014.01.010
Benjamin, C. F., Walshaw, P. D., Hale, K., Gaillard, W. D., Baxter, L. C., Berl, M. M., et al. (2017). Presurgical language fMRI: mapping of six critical regions. Hum. Brain Mapp. 38, 4239–4255. doi: 10.1002/hbm.23661
Benjamin, C. F. A., Dhingra, I., Li, A. X., Blumenfeld, H., Alkawadri, R., Bickel, S., et al. (2018). Presurgical language fMRI: technical practices in epilepsy surgical planning. Hum. Brain Mapp. 39, 4032–4042. doi: 10.1002/hbm.24229
Bennett, C. M., and Miller, M. B. (2010). How reliable are the results from functional magnetic resonance imaging? Ann. N. Y. Acad. Sci. 1191, 133–155. doi: 10.1111/j.1749-6632.2010.05446.x
Binder, J. R. (2015). The Wernicke area: modern evidence and a reinterpretation. Neurology 85, 2170–2175. doi: 10.1212/WNL.0000000000002219
Binder, J. R., Desai, R. H., Graves, W. W., and Conant, L. L. (2009). Where is the semantic system? A critical review and meta-analysis of 120 functional neuroimaging studies. Cereb. Cortex 19, 2767–2796. doi: 10.1093/cercor/bhp055
Binder, J. R., Swanson, S. J., Hammeke, T. A., Morris, G. L., Mueller, W. M., Fischer, M., et al. (1996). Determination of language dominance using functional MRI: a comparison with the Wada test. Neurology 46, 978–984. doi: 10.1212/wnl.46.4.978
Binder, J. R., Swanson, S. J., Hammeke, T. A., and Sabsevitz, D. S. (2008). A comparison of five fMRI protocols for mapping speech comprehension systems. Epilepsia 49, 1980–1997. doi: 10.1111/j.1528-1167.2008.01683.x
Black, D. F., Vachha, B., Mian, A., Faro, S. H., Maheshwari, M., Sair, H. I., et al. (2017). American Society of Functional Neuroradiology-recommended fMRI paradigm algorithms for presurgical language assessment. Am. J. Neuroradiol. 38, E65–E73. doi: 10.3174/ajnr.A5345
Bleich-Cohen, M., Hendler, T., Kotler, M., and Strous, R. D. (2009). Reduced language lateralization in first-episode schizophrenia: an fMRI index of functional asymmetry. Psychiatry Res. Neuroimaging 171, 82–93. doi: 10.1016/j.pscychresns.2008.03.002
Bonilha, L., Hillis, A. E., Hickok, G., Den Ouden, D. B., Rorden, C., and Fridriksson, J. (2017). Temporal lobe networks supporting the comprehension of spoken words. Brain 140, 2370–2380. doi: 10.1093/brain/awx169
Bradshaw, A. R., Thompson, P. A., Wilson, A. C., Bishop, D., and Woodhead, Z. (2017). Measuring language lateralisation with different language tasks: a systematic review. PeerJ 5:e3929. doi: 10.7717/peerj.3929
Brennan, N. M. P., Whalen, S., De Morales Branco, D., O’Shea, J. P., Norton, I. H., and Golby, A. J. (2007). Object naming is a more sensitive measure of speech localization than number counting: converging evidence from direct cortical stimulation and fMRI. Neuroimage 37, S100–S108. doi: 10.1016/j.neuroimage.2007.04.052
Buchsbaum, B. R., Hickok, G., and Humphries, C. (2001). Role of left posterior superior temporal gyrus in phonological processing for speech perception and production. Cogn. Sci. 25, 663–678. doi: 10.1016/S0364-0213(01)00048-9
Cohen, J. (1960). A coefficient of agreement for nominal scales. Educ. Psychol. Meas. 20, 37–46. doi: 10.1177/001316446002000104
Cremers, H. R., Wager, T. D., and Yarkoni, T. (2017). The relation between statistical power and inference in fMRI. PLoS One 12:e0184923. doi: 10.1371/journal.pone.0184923
Davey, J., Thompson, H. E., Hallam, G., Karapanagiotidis, T., Murphy, C., De Caso, I., et al. (2016). Exploring the role of the posterior middle temporal gyrus in semantic cognition: integration of anterior temporal lobe with executive processes. Neuroimage 137, 165–177. doi: 10.1016/j.neuroimage.2016.05.051
De Guibert, C., Maumet, C., Ferré, J.-C., Jannin, P., Biraben, A., Allaire, C., et al. (2010). FMRI language mapping in children: a panel of language tasks using visual and auditory stimulation without reading or metalinguistic requirements. Neuroimage 51, 897–909. doi: 10.1016/j.neuroimage.2010.02.054f
De Witte, E., and Mariën, P. (2013). The neurolinguistic approach to awake surgery reviewed. Clin. Neurol. Neurosurg. 115, 127–145. doi: 10.1016/j.clineuro.2012.09.015
Deblaere, K., Backes, W. H., Hofman, P., Vandemaele, P., Boon, P. A., Vonck, K., et al. (2002). Developing a comprehensive presurgical functional MRI protocol for patients with intractable temporal lobe epilepsy: a pilot study. Neuroradiology 44, 667–673. doi: 10.1007/s00234-002-0800-4
Den Ouden, D., Malyutina, S., Basilakos, A., Bonilha, L., Gleichgerrcht, E., Yourganov, G., et al. (2019). Cortical and structural-connectivity damage correlated with impaired syntactic processing in aphasia. Hum. Brain Mapp. 40, 2153–2173. doi: 10.1002/hbm.24514
Dodoo-Schittko, F., Rosengarth, K., Doenitz, C., and Greenlee, M. (2012). Assessing language dominance with functional MRI: the role of control tasks and statistical analysis. Neuropsychologia 50, 2684–2691. doi: 10.1016/j.neuropsychologia.2012.07.032
Dragoy, O., Zyryanov, A., Bronov, O., Gordeyeva, E., Gronskaya, N., Kryuchkova, O., et al. (2020). Functional linguistic specificity of the left frontal aslant tract for spontaneous speech fluency: evidence from intraoperative language mapping. Brain Lang. 208:104836. doi: 10.1016/j.bandl.2020.104836
Duffau, H. (2012). The challenge to remove diffuse low-grade gliomas while preserving brain functions. Acta Neurochir. 154, 569–574. doi: 10.1007/s00701-012-1275-7
Eberhard, D. M., Simons, G. F., and Fennig, C. D. (2020). Ethnologue: Languages of the World, 23rd Edn. Dallas, TX: SIL International.
Ek, L., Almkvist, O., Wiberg, M. K., Stragliotto, G., and Smits, A. (2010). Early cognitive impairment in a subset of patients with presumed low-grade glioma. Neurocase 16, 503–511. doi: 10.1080/13554791003730634
Elliott, M. L., Knodt, A. R., Ireland, D., Morris, M. L., Poulton, R., Ramrakha, S., et al. (2020). What is the test-retest reliability of common task-functional MRI measures? New empirical evidence and a meta-analysis. Psychol. Sci. 31, 792–806. doi: 10.1177/0956797620916786
Fan, L., Li, H., Zhuo, J., Zhang, Y., Wang, J., Chen, L., et al. (2016). The Human Brainnetome Atlas: a new brain atlas based on connectional architecture. Cereb. Cortex 26, 3508–3526. doi: 10.1093/cercor/bhw157
Fedorenko, E., Hsieh, P. J., Nieto-Castañón, A., Whitfield-Gabrieli, S., and Kanwisher, N. (2010). New method for fMRI investigations of language: defining ROIs functionally in individual subjects. J. Neurophysiol. 104, 1177–1194. doi: 10.1152/jn.00032.2010
Fernández, G., Specht, K., Weis, S., Tendolkar, I., Reuber, M., Fell, J., et al. (2003). Intrasubject reproducibility of presurgical language lateralization and mapping using fMRI. Neurology 60, 969–975. doi: 10.1212/01.wnl.0000049934.34209.2e
Fesl, G., Bruhns, P., Rau, S., Wiesmann, M., Ilmberger, J., Kegel, G., et al. (2010). Sensitivity and reliability of language laterality assessment with a free reversed association task – a fMRI study. Eur. Radiol. 20, 683–695. doi: 10.1007/s00330-009-1602-4
Flinker, A., Korzeniewska, A., Shestyuk, A. Y., Franaszczuk, P. J., Dronkers, N. F., Knight, R. T., et al. (2015). Redefining the role of Broca’s area in speech. Proc. Natl. Acad. Sci. U.S.A. 112, 2871–2875. doi: 10.1073/pnas.1414491112
Gabel, N., Altshuler, D. B., Brezzell, A., Briceño, E. M., Boileau, N. R., Miklja, Z., et al. (2019). Health-related quality of life in adult low and high-grade glioma patients using the National Institutes of health patient-reported outcomes measurement information system (PROMIS) and Neuro-QOL assessments. Front. Neurol. 10:212. doi: 10.3389/fneur.2019.00212
Gorgolewski, K., Storkey, A. J., Bastin, M. E., and Pernet, C. R. (2012). Adaptive thresholding for reliable topological inference in single subject fMRI analysis. Front. Hum. Neurosci. 6:245. doi: 10.3389/fnhum.2012.00245
Graves, W. W., Grabowski, T. J., Mehta, S., and Gupta, P. (2008). The left posterior superior temporal gyrus participates specifically in accessing lexical phonology. J. Cogn. Neurosci. 20, 1698–1710. doi: 10.1162/jocn.2008.20113
Gross, W. L., and Binder, J. R. (2014). Alternative thresholding methods for fMRI data optimized for surgical planning. Neuroimage 84, 554–561. doi: 10.1016/j.neuroimage.2013.08.066
Grummich, P., Nimsky, C., Pauli, E., Buchfelder, M., and Ganslandt, O. (2006). Combining fMRI and MEG increases the reliability of presurgical language localization: a clinical study on the difference between and congruence of both modalities. Neuroimage 32, 1793–1803. doi: 10.1016/j.neuroimage.2006.05.034
Hagoort, P. (2005). On Broca, brain, and binding: a new framework. Trends Cogn. Sci. 9, 416–423. doi: 10.1016/j.tics.2005.07.004
Hakyemez, B., Erdogan, C., Yildirim, N., Bora, I., Bekar, A., and Parlak, M. (2006). Functional MRI in patients with intracranial lesions near language areas. Neuroradiol. J. 508, 306–312. doi: 10.1177/197140090601900306
Hall, D. A., Haggard, M. P., Akeroyd, M. A., Palmer, A. R., Summerfield, A. Q., Elliott, M. R., et al. (1999). “Sparse” temporal sampling in auditory fMRI. Hum. Brain Mapp. 7, 213–223. doi: 10.1002/(sici)1097-019319997:3<213::aid-hbm5<3.0.co;2-n
Hammers, A., Allom, R., Koepp, M. J., Free, S. L., Myers, R., Lemieux, L., et al. (2003). Three-dimensional maximum probability atlas of the human brain, with particular reference to the temporal lobe. Hum. Brain Mapp. 19, 224–247. doi: 10.1002/hbm.10123
Hazem, S. R., Awan, M., Lavrador, J. P., Patel, S., Wren, H. M., Lucena, O., et al. (2021). Middle frontal gyrus and area 55b: perioperative mapping and language outcomes. Front. Neurol. 12:646075. doi: 10.3389/fneur.2021.646075
Hervey-Jumper, S. L., Li, J., Lau, D., Molinaro, A. M., Perry, D. W., Meng, L., et al. (2015). Awake craniotomy to maximize glioma resection: methods and technical nuances over a 27-year period. J. Neurosurg. 123, 325–339. doi: 10.3171/2014.10.JNS141520
Hickok, G., and Poeppel, D. (2007). The cortical organization of speech processing. Nat. Rev. Neurosci. 8, 393–402. doi: 10.1038/nrn2113
Hilari, K., Wiggins, R., Roy, P., Byng, S., and Smith, S. (2003). Predictors of health-related quality of life (HRQL) in people with chronic aphasia. Aphasiology 17, 365–381. doi: 10.1080/02687030244000725
Hillis, A. E., Rorden, C., and Fridriksson, J. (2017). Brain regions essential for word comprehension: drawing inferences from patients. Ann. Neurol. 81, 759–768. doi: 10.1002/ana.24941
Holiga, Š, Sambataro, F., Luzy, C., Greig, G., Sarkar, N., Renken, R. J., et al. (2018). Test-retest reliability of task-based and resting-state blood oxygen level dependence and cerebral blood flow measures. PLoS One 13:e0206583. doi: 10.1371/journal.pone.0206583
Huettel, S., Song, A., and McCarthy, G. (2008). Functional Magnetic Resonance Imaging, 2nd Edn. Sunderland, MA: Sinauer Associates Inc.
Hund-Georgiadis, M., Lex, U., and von Cramon, D. Y. (2001). Language dominance assessment by means of fMRI: contributions from task design, performance, and stimulus modality. J. Magn. Reson. Imaging 13, 668–675. doi: 10.1002/jmri.1094
Ille, S., Sollmann, N., Hauck, T., Maurer, S., Tanigawa, N., Obermueller, T., et al. (2015). Combined noninvasive language mapping by navigated transcranial magnetic stimulation and functional MRI and its comparison with direct cortical stimulation. J. Neurosurg. 123, 212–225. doi: 10.3171/2014.9.JNS14929
Jansen, A., Menke, R., Sommer, J., Förster, A. F., Bruchmann, S., Hempleman, J., et al. (2006). The assessment of hemispheric lateralization in functional MRI—robustness and reproducibility. Neuroimage 33, 204–217. doi: 10.1016/j.neuroimage.2006.06.019
Jones, S. E., Mahmoud, S. Y., and Phillips, M. D. (2011). A practical clinical method to quantify language lateralization in fMRI using whole-brain analysis. Neuroimage 54, 2937–2949. doi: 10.1016/j.neuroimage.2010.10.052
Kinno, R., Ohta, S., Muragaki, Y., Maruyama, T., and Sakai, K. L. (2014). Differential reorganization of three syntax-related networks induced by a left frontal glioma. Brain 137, 1193–1212. doi: 10.1093/brain/awu013
Kinoshita, M., de Champfleur, N. M., Deverdun, J., Moritz-Gasser, S., Herbet, G., and Duffau, H. (2015). Role of fronto-striatal tract and frontal aslant tract in movement and speech: an axonal mapping study. Brain Struct. Funct. 220, 3399–3412. doi: 10.1007/s00429-014-0863-0
Knecht, S., Deppe, M., Dräger, B., Bobe, L., Lohmann, H., Ringelstein, E., et al. (2000). Language lateralization in healthy right-handers. Brain 123, 74–81. doi: 10.1093/brain/123.1.74
Kozák, L. R., van Graan, L. A., Chaudhary, U. J., Szabó, ÁG., and Lemieux, L. (2017). ICN_Atlas: automated description and quantification of functional MRI activation patterns in the framework of intrinsic connectivity networks. Neuroimage 163, 319–341. doi: 10.1016/j.neuroimage.2017.09.014
Krainik, A., Lehéricy, S., Duffau, H., Vlaicu, M., Poupon, F., Capelle, L., et al. (2001). Role of the supplementary motor area in motor deficit following medial frontal lobe surgery. Neurology 57, 871–878. doi: 10.1212/wnl.57.5.871
Lehéricy, S., Cohen, L., Bazin, B., Samson, S., Giacomini, E., Rougetet, R., et al. (2000). Functional MR evaluation of temporal and frontal language dominance compared with the Wada test. Neurology 54, 1625–1633. doi: 10.1212/WNL.54.8.1625
Litvinova, L., Pechenkova, E., Vlasova, R., Berezutskaya, Y., and Sinitsyn, V. (2012). “Lokalizatsiya zon, svyazannyh s vospriyatiem rechi: sopostavlenie tryokh prob dlya fMRT na material russkogo yazyka. [A comparison of three fMRI paradigms for mapping speech perception in Russian speakers],” in Proceedings of the International Symposium on Functional Neuroimaging: Basic Research and Clinical Applications. Abstracts, ed. S. Novikova (Moscow: MSUPE), 76–79.
Loddenkemper, T., Morris, H. H., and Möddel, G. (2008). Complications during the Wada test. Epilepsy Behav. 13, 551–553. doi: 10.1016/j.yebeh.2008.05.014
Loring, D. W., Meador, K. J., and Lee, G. P. (1992). “Criteria and validity issues in Wada assessment,” in The Neuropsychology of Epilepsy, ed. I. Benett (New York, NY: Plenum Press), 233–245. doi: 10.1007/978-1-4899-2350-9_11
Manan, H. A., Franz, E. A., and Yahya, N. (2020). Utilization of functional MRI language paradigms for pre-operative mapping: a systematic review. Neuroradiology 62, 353–367. doi: 10.1007/s00234-019-02322-w
Mano, Q. R., Humphries, C., Desai, R. H., Seidenberg, M. S., Osmon, D. C., Stengel, B. C., et al. (2013). The role of left occipitotemporal cortex in reading: reconciling stimulus, task, and lexicality effects. Cereb. Cortex 23, 988–1001. doi: 10.1093/cercor/bhs093
Mauler, J., Neuner, I., Neuloh, G., Fimm, B., Boers, F., Wiesmann, M., et al. (2017). Dissociated crossed speech areas in a tumour patient. Case Rep. Neurol. 9, 131–136. doi: 10.1159/000475882
Mazziotta, J. C., Toga, A. W., Evans, A., Fox, P., and Lancaster, J. (1995). A probabilistic atlas of the human brain: theory and rationale for its development. Neuroimage 2, 89–101. doi: 10.1006/nimg.1995.1012
Miró, J., Ripollés, P., López-Barroso, D., Vilà-Balló, A., Juncadella, M., de Diego-Balaguer, R., et al. (2014). Atypical language organization in temporal lobe epilepsy revealed by a passive semantic paradigm. BMC Neurol. 14:98. doi: 10.1186/1471-2377-14-98
Morrison, M. A., Tam, F., Garavaglia, M. M., Hare, G. M. T., Cusimano, M. D., Schweizer, T. A., et al. (2016b). Sources of variation influencing concordance between functional MRI and direct cortical stimulation in brain tumor surgery. Front. Neurosci. 10:461. doi: 10.3389/fnins.2016.00461
Morrison, M. A., Churchill, N. W., Cusimano, M. D., Schweizer, T. A., Das, S., and Graham, S. J. (2016a). Reliability of task-based fMRI for preoperative planning: a test-retest study in brain tumor patients and healthy controls. PLoS One 11:e0149547. doi: 10.1371/journal.pone.0149547
Nadkarni, T. N., Andreoli, M. J., Nair, V. A., Yin, P., Young, B. M., Kundu, B., et al. (2015). Usage of fMRI for pre-surgical planning in brain tumor and vascular lesion patients: task and statistical threshold effects on language lateralization. Neuroimage Clin. 7, 415–423. doi: 10.1016/j.nicl.2014.12.014
Nettekoven, C., Reck, N., Goldbrunner, R., Grefkes, C., and Weiß Lucas, C. (2018). Short- and long-term reliability of language fMRI. Neuroimage 176, 215–225. doi: 10.1016/j.neuroimage.2018.04.050
Newman, S. D., Twieg, D. B., and Carpenter, P. A. (2001). Baseline conditions and subtractive logic in neuroimaging. Hum. Brain Mapp. 14, 228–235. doi: 10.1002/hbm.1055
Ocklenburg, S., Hugdahl, K., and Westerhausen, R. (2013). Structural white matter asymmetries in relation to functional asymmetries during speech perception and production. Neuroimage 83, 1088–1097. doi: 10.1016/j.neuroimage.2013.07.076
Ojemann, G. A. (1979). Individual variability in cortical localization of language. J. Neurosurg. 50, 164–169. doi: 10.3171/jns.1979.50.2.0164
Ojemann, G. A. (1993). Functional mapping of cortical language areas in adults. Intraoperative approaches. Adv. Neurol. 63, 155–163.
Partovi, S., Jacobi, B., Rapps, N., Zipp, L., Karimi, S., Rengier, F., et al. (2012). Clinical standardized fMRI reveals altered language lateralization in patients with brain tumor. Am. J. Neuroradiol. 33, 2151–2157. doi: 10.3174/ajnr.A3137
Patrikelis, P., Gatzonis, S., Siatouni, A., Angelopoulos, E., Konstantakopoulos, G., Takousi, M., et al. (2016). Preoperative neuropsychological presentation of patients with refractory frontal lobe epilepsy. Acta Neurochir. 158, 1139–1150. doi: 10.1007/s00701-016-2786-4
Pearson, J., Naselaris, T., Holmes, E. A., and Kosslyn, S. M. (2015). Mental imagery: functional mechanisms and clinical applications. Trends Cogn. Sci. 19, 590–602. doi: 10.1016/j.tics.2015.08.003
Peelle, J. E. (2012). The hemispheric lateralization of speech processing depends on what “speech” is: a hierarchical perspective. Front. Hum. Neurosci. 6:309. doi: 10.3389/fnhum.2012.00309
Picht, T., Krieg, S. M., Sollmann, N., Rösler, J., Niraula, B., Neuvonen, T., et al. (2013). A comparison of language mapping by preoperative navigated transcranial magnetic stimulation and direct cortical stimulation during awake surgery. Neurosurgery 72, 808–819. doi: 10.1227/NEU.0b013e3182889e01
Pillai, J. J., and Zaca, D. (2011). Relative utility for hemispheric lateralization of different clinical fMRI activation tasks within a comprehensive language paradigm battery in brain tumor patients as assessed by both threshold-dependent and threshold-independent analysis methods. Neuroimage 54(Suppl. 1), S136–S145. doi: 10.1016/j.neuroimage.2010.03.082
Połczyńska, M., Japardi, K., Curtiss, S., Moody, T., Benjamin, C., Cho, A., et al. (2017). Improving language mapping in clinical fMRI through assessment of grammar. Neuroimage Clin. 15, 415–427. doi: 10.1016/j.nicl.2017.05.021
Pouratian, N., Bookheimer, S. Y., Rex, D. E., Martin, N. A., and Toga, A. W. (2002). Utility of preoperative functional magnetic resonance imaging for identifying language cortices in patients with vascular malformations. J. Neurosurg. 97, 21–32. doi: 10.3171/jns.2002.97.1.0021
Price, A. R., Peelle, J. E., Bonner, M. F., Grossman, M., and Hamilton, R. H. (2016). Causal evidence for a mechanism of semantic integration in the angular gyrus as revealed by high-definition transcranial direct current stimulation. J. Neurosci. 36, 3829–3838. doi: 10.1523/jneurosci.3120-15.2016
Price, C. J. (2010). The anatomy of language: a review of 100 fMRI studies published in 2009. Ann. N. Y. Acad. Sci. 1191, 62–88. doi: 10.1111/j.1749-6632.2010.05444.x
Racine, C. A., Li, J., Molinaro, A. M., Butowski, N., and Berger, M. S. (2015). Neurocognitive function in newly diagnosed low-grade glioma patients undergoing surgical resection with awake mapping techniques. Neurosurgery 77, 371–379. doi: 10.1227/NEU.0000000000000779
Richardson, F. M., Seghier, M. L., Leff, A. P., Thomas, M. S., and Price, C. J. (2011). Multiple routes from occipital to temporal cortices during reading. J. Neurosci. 31, 8239–8247. doi: 10.1523/JNEUROSCI.6519-10.2011
Robinson, G., Shallice, T., Bozzali, M., and Cipolotti, L. (2010). Conceptual proposition selection and the LIFG: neuropsychological evidence from a focal frontal group. Neuropsychologia 48, 1652–1663. doi: 10.1016/j.neuropsychologia.2010.02.010
Rodd, J. M., Davis, M. H., and Johnsrude, I. S. (2005). The neural mechanisms of speech comprehension: fMRI studies of semantic ambiguity. Cereb. Cortex 15, 1261–1269. doi: 10.1093/cercor/bhi009
Rofes, A., Mandonnet, E., de Aguiar, V., Rapp, B., Tsapkini, K., and Miceli, G. (2019). Language processing from the perspective of electrical stimulation mapping. Cogn. Neuropsychol. 36, 117–139. doi: 10.1080/02643294.2018.1485636
Rombouts, S. A., Barkhof, F., Hoogenraad, F. G., Sprenger, M., Valk, J., and Scheltens, P. (1997). Test-retest analysis with functional MR of the activated area in the human visual cortex. Am. J. Neuroradiol. 18, 1317–1322.
Roux, F. E., Boulanouar, K., Lotterie, J. A., Mejdoubi, M., LeSage, J. P., and Berry, I. (2003). Language functional magnetic resonance imaging in preoperative assessment of language areas: correlation with direct cortical stimulation. Neurosurgery 52, 1335–1347. doi: 10.1227/01.neu.0000064803.05077.40
Ruff, I. M. Petrovich Brennan, N. M., Peck, K. K., Hou, B. L., Tabar, V., Brennan, C. W., et al. (2008). Assessment of the language laterality index in patients with brain tumor using functional MR imaging: effects of thresholding, task selection, and prior surgery. Am. J. Neuroradiol. 29, 528–535. doi: 10.3174/ajnr.A0841
Ruge, M. I., Victor, J., Hosain, S., Correa, D. D., Relkin, N. R., Tabar, V., et al. (1999). Concordance between functional magnetic resonance imaging and intraoperative language mapping. Stereotact. Funct. Neurosurg. 72, 95–102. doi: 10.1159/000029706
Rumshiskaya, A., Vlasova, R., Litvinova, L., Pechenkova, E., and Mershina, E. (2014). “Combined analysis of two tasks improves localization of Wernicke’s area in patients with primary brain tumors,” in Poster Presented at the European Congress of Neuroradiology, Vienna. doi: 10.1594/ecr2014/C-1232
Rutten, G. J. M., Ramsey, N. F., Van Rijen, P. C., Alpherts, W. C., and Van Veelen, C. W. M. (2002). fMRI-determined language lateralization in patients with unilateral or mixed language dominance according to the Wada test. Neuroimage 17, 447–460. doi: 10.1006/nimg.2002.1196
Salek, K. E., Hassan, I. S., Kotrotsou, A., Abrol, S., Faro, S. H., Mohamed, F. B., et al. (2017). Silent sentence completion shows superiority localizing Wernicke’s area and activation patterns of distinct language paradigms correlate with genomics: prospective study. Sci. Rep. 7:12054. doi: 10.1038/s41598-017-11192-2
Sanjuan, A., Bustamante, J.-C., Forn, C., Ventura-Campos, N., Barros-Loscertales, A., Martinez, J.-C., et al. (2010). Comparison of two fMRI tasks for the evaluation of the expressive language function. Neuroradiology 52, 407–415. doi: 10.1007/s00234-010-0667-8
Satoer, D., Visch-Brink, E., Dirven, C., and Vincent, A. (2016). Glioma surgery in eloquent areas: can we preserve cognition? Acta Neurochi. 158, 35–50. doi: 10.1007/s00701-015-2601-7
Silva, M. A., See, A. P., Essayed, W. I., Golby, A. J., and Tie, Y. (2018). Challenges and techniques for presurgical brain mapping with functional MRI. Neuroimage Clin. 17, 794–803. doi: 10.1016/j.nicl.2017.12.008
Sollmann, N., Kubitscheck, A., Maurer, S., Ille, S., Hauck, T., Kirschke, J. S., et al. (2016). Preoperative language mapping by repetitive navigated transcranial magnetic stimulation and diffusion tensor imaging fiber tracking and their comparison to intraoperative stimulation. Neuroradiology 58, 807–818. doi: 10.1007/s00234-016-1685-y
Spena, G., Nava, A., Cassini, F., Pepoli, A., Bruno, M., D’Agata, F., et al. (2010). Preoperative and intraoperative brain mapping for the resection of eloquent-area tumors. A prospective analysis of methodology, correlation, and usefulness based on clinical outcomes. Acta Neurochir. 152, 1835–1846. doi: 10.1007/s00701-010-0764-9
Springer, J. A., Binder, J. R., Hammeke, T. A., Swanson, S. J., Frost, J. A., Bellgowan, P. S., et al. (1999). Language dominance in neurologically normal and epilepsy subjects: a functional MRI study. Brain 122, 2033–2046. doi: 10.1093/brain/122.11.2033
Stevens, M. T., Clarke, D. B., Stroink, G., Beyea, S. D., and D’Arcy, R. C. (2016). Improving fMRI reliability in presurgical mapping for brain tumours. J. Neurol. Neurosurg. Psychiatry 87, 267–274. doi: 10.1136/jnnp-2015-310307
Stevens, M. T. R., D’Arcy, R. C. N., Stroink, C. D. B., and Beyea, S. D. (2013). Thresholds in fMRI studies: reliable for single subjects? J. Neurosci. Methods 219, 312–323. doi: 10.1016/j.jneumeth.2013
Stippich, C., Rapps, N., Dreyhaupt, J., Durst, A., Kress, B., Nennig, E., et al. (2007). Localizing and lateralizing language in patients with brain tumors: feasibility of routine preoperative functional MR imaging in 81 consecutive patients. Radiology 243, 828–836. doi: 10.1148/radiol.2433060068
Stoppelman, N., Harpaz, T., and Ben-Shachar, M. (2013). Do not throw out the baby with the bath water: choosing an effective baseline for a functional localizer of speech processing. Brain Behav. 3, 211–222. doi: 10.1002/brb3.129
Suarez, R. O., Taimouri, V., Boyer, K., Vega, C., Rotenberg, A., Madsen, J. R., et al. (2014). Passive fMRI mapping of language function for pediatric epilepsy surgical planning: validation using Wada, ECS, and FMAER. Epilepsy Res. 108, 1874–1888. doi: 10.1016/j.eplepsyres.2014.09.016
Szaflarski, J. P., Holland, S. K., Jacola, L. M., Lindsell, C., Privitera, M. D., and Szaflarski, M. (2008). Comprehensive presurgical functional MRI language evaluation in adult patients with epilepsy. Epilepsy Behav. 12, 74–83. doi: 10.1016/j.yebeh.2007.07.015
Tailby, C., Abbott, D. F., and Jackson, G. D. (2017). The diminishing dominance of the dominant hemisphere: language fMRI in focal epilepsy. Neuroimage Clin 14, 141–150. doi: 10.1016/j.nicl.2017.01.011
Thivard, L., Hombrouck, J., Du Montcel, S. T., Delmaire, C., Cohen, L., Samson, S., et al. (2005). Productive and perceptive language reorganization in temporal lobe epilepsy. Neuroimage 24, 841–851. doi: 10.1016/j.neuroimage.2004.10.001
Thompson, C. K., Bonakdarpour, B., Fix, S. C., Blumenfeld, H. K., Parrish, T. B., Gitelman, D. R., et al. (2007). Neural correlates of verb argument structure processing. J. Cogn. Neurosci. 219, 1753–1767. doi: 10.1162/jocn.2007.19.11.1753
Turken, A. U., and Dronkers, N. F. (2011). The neural architecture of the language comprehension network: converging evidence from lesion and connectivity analyses. Front. Syst. Neurosci. 5:1. doi: 10.3389/fnsys.2011.00001
Tzourio-Mazoyer, N., Landeau, B., Papathanassiou, D., Crivello, F., Étard, O., Delcroix, N., et al. (2002). Automated anatomical labeling of activations in SPM using a macroscopic anatomical parcellation of the MNI MRI single-subject brain. Neuroimage 15, 273–289. doi: 10.1006/nimg.2001.0978
Unadkat, P., Fumagalli, L., Rigolo, L., Vangel, M. G., Young, G. S., Huang, R., et al. (2019). Functional MRI task comparison for language mapping in neurosurgical patients. J. Neuroimaging 29, 348–356. doi: 10.1111/jon.12597
Van Poppel, M., Wheless, J. W., Clarke, D. F., McGregor, A., McManis, M. H., Perkins, F. F. Jr., et al. (2012). Passive language mapping with magnetoencephalography in pediatric patients with epilepsy. J. Neurosurg. Pediatr. 10, 96–102. doi: 10.3171/2012.4.PEDS11301
Voyvodic, J. T., Petrella, J. R., and Friedman, A. H. (2009). fMRI activation mapping as a percentage of local excitation: consistent presurgical motor maps without threshold adjustment. J. Magn. Res. Imaging 29, 751–759. doi: 10.1002/jmri.21716
Wada, J., and Rasmussen, T. (1960). Intracarotid injection of sodium amytal for the lateralization of cerebral speech dominance. J. Neurosurg. 17, 266–282. doi: 10.3171/jns.2007.106.6.1117
Weng, H. H., Noll, K. R., Johnson, J. M., Prabhu, S. S., Tsai, Y. H., Chang, S. W., et al. (2018). Accuracy of presurgical functional MR imaging for language mapping of brain tumors: a systematic review and meta-analysis. Radiology 286, 512–523. doi: 10.1148/radiol.2017162971
Whalley, H. C., Gountouna, V. E., Hall, J., McIntosh, A. M., Simonotto, E., Job, D. E., et al. (2009). fMRI changes over time and reproducibility in unmedicated subjects at high genetic risk of schizophrenia. Psychol. Med. 39, 1189–1199. doi: 10.1017/S0033291708004923
Wilke, M., and Lidzba, K. (2007). LI-tool: a new toolbox to assess lateralization in functional MR-data. J. Neurosci. Methods 163, 128–136. doi: 10.1016/j.jneumeth.2007.01.026
Wilson, S. M., Bautista, A., Yen, M., Lauderdale, S., and Eriksson, D. K. (2017). Validity and reliability of four language mapping paradigms. Neuroimage Clin. 16, 399–408. doi: 10.1016/j.nicl.2016.03.015
Yarkoni, T., Barch, D. M., Gray, J. R., Conturo, T. E., and Braver, T. S. (2009). BOLD correlates of trial-by-trial reaction time variability in gray and white matter: a multi-study fMRI analysis. PLoS One 4:e4257. doi: 10.1371/journal.pone.0004257
Yushkevich, P. A., Piven, J., Hazlett, H. C., Smith, R. G., Ho, S., Gee, J. C., et al. (2006). User-guided 3D active contour segmentation of anatomical structures: significantly improved efficiency and reliability. Neuroimage 31, 1116–1128. doi: 10.1016/j.neuroimage.2006.01.015
Zacà, D., Nickerson, J. P., Deib, G., and Pillai, J. J. (2012). Effectiveness of four different clinical fMRI paradigms for preoperative regional determination of language lateralization in patients with brain tumors. Neuroradiology 54, 1015–1025. doi: 10.1007/s00234-012-1056-2
Zhang, N., Xia, M., Qiu, T., Wang, X., Lin, C. P., Guo, Q., et al. (2018). Reorganization of cerebro-cerebellar circuit in patients with left hemispheric gliomas involving language network: a combined structural and resting-state functional MRI study. Hum. Brain Mapp. 39, 4802–4819. doi: 10.1002/hbm.24324
Keywords: functional magnetic resonance imaging, presurgical mapping, language localizer paradigm, test–retest reliability of fMRI, language mapping, sentence completion
Citation: Elin K, Malyutina S, Bronov O, Stupina E, Marinets A, Zhuravleva A and Dragoy O (2022) A New Functional Magnetic Resonance Imaging Localizer for Preoperative Language Mapping Using a Sentence Completion Task: Validity, Choice of Baseline Condition, and Test–Retest Reliability. Front. Hum. Neurosci. 16:791577. doi: 10.3389/fnhum.2022.791577
Received: 08 October 2021; Accepted: 04 March 2022;
Published: 30 March 2022.
Edited by:
Lars Meyer, Max Planck Society, GermanyReviewed by:
Gaël Jobard, Université de Bordeaux, FrancePhilipp Kuhnke, Max Planck Institute for Human Cognitive and Brain Sciences, Germany
Copyright © 2022 Elin, Malyutina, Bronov, Stupina, Marinets, Zhuravleva and Dragoy. This is an open-access article distributed under the terms of the Creative Commons Attribution License (CC BY). The use, distribution or reproduction in other forums is permitted, provided the original author(s) and the copyright owner(s) are credited and that the original publication in this journal is cited, in accordance with accepted academic practice. No use, distribution or reproduction is permitted which does not comply with these terms.
*Correspondence: Svetlana Malyutina, smalyutina@hse.ru
†These authors have contributed equally to this work