The effects of robot-assisted gait training combined with non-invasive brain stimulation on lower limb function in patients with stroke and spinal cord injury: A systematic review and meta-analysis
- 1Department of Rehabilitation Medicine, Keio University School of Medicine, Tokyo, Japan
- 2Department of Physical Therapy, Graduate School of Health Sciences, Tokyo Metropolitan University, Tokyo, Japan
- 3Department of Artificial Environment, Safety, Environment and System Engineering, Graduate School of Environment and Information Sciences, Yokohama National University, Kanagawa, Japan
Objective: This study aimed to investigate the effect of robot-assisted gait training (RAGT) therapy combined with non-invasive brain stimulation (NIBS) on lower limb function in patients with stroke and spinal cord injury (SCI).
Data sources: PubMed, Cochrane Central Register of Controlled Trials, Ovid MEDLINE, and Web of Science were searched.
Study selection: Randomized controlled trials (RCTs) published as of 3 March 2021. RCTs evaluating RAGT combined with NIBS, such as transcranial direct current stimulation (tDCS) and repetitive transcranial magnetic stimulation (rTMS), for lower limb function (e.g., Fugl-Meyer assessment for patients with stroke) and activities (i.e., gait velocity) in patients with stroke and SCI were included.
Data extraction: Two reviewers independently screened the records, extracted the data, and assessed the risk of bias.
Data synthesis: A meta-analysis of five studies (104 participants) and risk of bias were conducted. Pooled estimates demonstrated that RAGT combined with NIBS significantly improved lower limb function [standardized mean difference (SMD) = 0.52; 95% confidence interval (CI) = 0.06–0.99] but not lower limb activities (SMD = −0.13; 95% CI = −0.63–0.38). Subgroup analyses also failed to find a greater improvement in lower limb function of RAGT with tDCS compared to sham stimulation. No significant differences between participant characteristics or types of NIBS were observed.
Conclusion: This meta-analysis demonstrated that RAGT therapy in combination with NIBS was effective in patients with stroke and SCI. However, a greater improvement in lower limb function and activities were not observed using RAGT with tDCS compared to sham stimulation.
Introduction
Many patients with stroke and spinal cord injury (SCI) suffer from motor and gait dysfunction due to lower limb paralysis. It was reported that about 290,000 individuals per year experience a stroke in Japan (Takashima et al., 2017). As common sequelae in stroke patients, mobility deficits associated with lower limb dysfunction seriously affect patients' functional independence and quality of life (van de Port et al., 2006; Tyson et al., 2007). As for SCI, the prevalence ranges from 280 to 1,298 cases per million people (Bickenbach, 2013), with a male-to-female sex ratio of 3–4:1. Similarly, patients with SCI usually have mild to moderate lower limb dysfunction and disability. More than half of such injuries occur before 30 years of age (Sekhon and Fehlings, 2001) and cause subsequent psychological stress to the patient and their caregivers because of disability at such an early age. Patients with lower limb motor paralysis due to stroke and SCI suffer from impaired motor and gait function. Improvement in gait function is strongly desired in patients with lower limb motor paralysis caused by stroke and SCI. However, 6 months after stroke onset, it is estimated that only about 30% of patients can recover to an ambulatory level (Jørgensen et al., 1995). More effective treatment for patients with stroke and SCI is desired.
The application of robotic technology for stroke (Krebs and Hogan, 2006; Oujamaa et al., 2009; Veerbeek et al., 2011; Mehrholz et al., 2020) and SCI (Mehrholz et al., 2012; Cheung et al., 2017) to improve motor function has increased in recent years. Robot-assisted gait training (RAGT) devices utilize electromechanically actuated motors that control movement and exert force on the joints or other parts of the lower limbs and are categorized as end-effector, exoskeleton, mobile, and ankle devices (Oujamaa et al., 2009). However, the efficacy of these devices is still under discussion and yet to be clarified. Some studies have shown a positive effect of RAGT on gait velocity and walking independence in both stroke and SCI (Krebs and Hogan, 2006; Oujamaa et al., 2009; Veerbeek et al., 2011; Mehrholz et al., 2012, 2020; Cheung et al., 2017), while others have found no effect (Mehrholz et al., 2012, 2020). Therefore, careful consideration of means to increase the effectiveness of RAGT is warranted.
Furthermore, as an example of rehabilitation in patients with lower limb motor paralysis, some studies have shown the effectiveness of non-invasive brain stimulation (NIBS), such as transcranial direct current stimulation (tDCS) and repetitive transcranial magnetic stimulation (rTMS). Multiple meta-analyses and systematic reviews have examined the efficacy of tDCS, rTMS, or spinal direct current stimulation on lower limb motor function related to gait and balance function in patients with stroke or SCI (Chieffo et al., 2016; Fleming et al., 2017; Li et al., 2018; Ghayour-Najafabadi et al., 2019; Tung et al., 2019; Abualait and Ibrahim, 2020; Elsner et al., 2020; Gowan and Hordacre, 2020). Although most meta-analyses have demonstrated the effectiveness of NIBS, some have shown limited effectiveness.
Thus, systematic reviews with meta-analyses have verified the effectiveness of RAGT alone or NIBS alone on lower limb motor function in patients with stroke and SCI. The combination of therapeutic exercise and NIBS holds promise for facilitating neuromodulation in subjects with corticospinal-tract lesions (Hiscock et al., 2008; Dimyan and Cohen, 2010; Tanaka et al., 2011; Benito et al., 2012; Sohn et al., 2013; Murray et al., 2015). We hypothesized that RAGT therapy combined with NIBS might be more effective than RAGT combined with sham stimulation in improving lower limb motor function related to gait function in patients with lower limb paralysis. In the present study, we conducted a systematic review with meta-analysis to demonstrate the effects of combined therapy with RAGT and NIBS in patients with stroke and SCI.
Methods
Search strategy
The literature review protocol was developed following the Preferred Reporting Items for Systematic Reviews and Meta-Analyses statement (Moher et al., 2009). Articles were assessed and collected from PubMed, Cochrane Central Register of Controlled Trials, Ovid MEDLINE, and Web of Science up to 3 March 2021.
Study selection
The primary search was conducted using the combined terms: “stroke” OR “spinal cord injury,” “lower limb*” OR “lower extremit*,” “transcranial magnetic stimulation” OR “transcranial electrical stimulation” OR “transcranial direct current stimulation” AND “robot*” OR “orthos*” OR “orthotic” OR “automat*” OR “computer-aided” OR “computer-assisted” OR “power-assist*.”
Additionally, the following parameters were used: clinical trials, randomized controlled trials (RCTs), and scientific articles written in English, with full text available. The publication date was not restricted; additional studies were identified by manual search, and duplicates were removed.
The inclusion criteria were as follows: (1) adult participants (age > 18 years); (2) outcomes, including the effects of NIBS, such as tDCS or rTMS, on lower limb motor function, RCT or randomized controlled crossover trial; (3) recruitment of more than five patients; (4) the same interventions between the experimental and control groups, except for tDCS or rTMS treatment in the experimental group; and (5) studies published in English. The exclusion criteria were as follows: (1) non-peer-reviewed articles and articles only describing protocols; (2) studies that met the eligibility criteria but lacked outcomes owing to a lack of e-mail reply from the author; (3) studies of patients with lower limb dysfunction which was caused by other diseases such as Parkinson's disease or amyotrophic lateral sclerosis.
Concerning the reference selection process and inclusion and exclusion criteria above, the potential articles were screened by two reviewers to remove irrelevant studies. Potentially eligible studies were chosen from the remainder if the full text was available.
From the final selected studies, data on the study design and subjects, intervention, methods, and reporting of information on the effectiveness of RAGT combined with NIBS were extracted from the selected articles to provide information on the results and effects that would be useful in clinical practice (Table 1).
Data extraction and quality assessment
The two researchers extracted data regarding the following parameters of the intervention (tDCS or rTMS) and control groups: number of participants, time since onset, severity, motor function at baseline as inclusion criteria, training period and protocol, robotic setting, and outcome measures.
The quality of the studies based on the inclusion and exclusion criteria was assessed using the Physiotherapy Evidence Database (PEDro) scale. The results of the two researchers were compared, and any discrepancies were evaluated by a third researcher and resolved through discussion.
Outcomes
Several different outcomes were used in each included study. In this study, we performed comprehensive meta-analyses of similar outcomes owing to low participant numbers in each included study. Therefore, the outcomes were classified based on the International Classification of Functioning, Disability, and Health (ICF) into two components: (1) body functions/structures and (2) activities/participation. If multiple outcome assessments of a study were classified in the same domain, the most comprehensive assessment was adopted. Outcomes after the intervention were used for the meta-analysis. If the data were missing, the authors were contacted.
Data synthesis and analysis
Results were pooled, and meta-analysis was conducted using Cochrane Collaboration's Review Manager software Version 5.4 (RevMan 5.4, The Nordic Cochrane Center, Copenhagen, Denmark). After pooling the software for calculation, the data are expressed using standard mean difference (SMD) and 95% confidence intervals (CI). Statistical heterogeneity was considered high if the I2 value was > 50%. P < 0.05 in the equivalent z test was considered statistically significant. In addition, the following subgroup analyses were conducted: (1) according to participants' characteristics: stroke or SCI; and (2) according to the type of NIBS: tDCS or rTMS.
Results
Study selection
A flowchart of the study selection process is illustrated in Figure 1. The characteristics of the included studies are listed in Table 1. After independent reviews by two researchers, five RCTs comparing the effects of RAGT therapy combined with tDCS or rTMS to sham stimulation on lower limb motor function in patients with stroke and SCI met the eligibility criteria.
Assessment of risk of bias
For the included RCTs, an assessment of the risk of bias was conducted. The PEDro scale scores for all included studies were between 10 and 11, indicating high quality. The patients were blinded in all studies. Among the studies, there was only one in which therapists were not blinded. Therefore, the potential risk of bias was low. Appropriate randomization was performed in all studies except one, in which participants were assigned in order. Allocation concealment and baseline similarity between groups for the most important prognostic indicators appeared to be present in all studies. The assessment results of the risk of bias using the PEDro score are shown in Supplementary Table 1.
Outcome selection
The five included RCTs used various outcomes (Table 1). Based on the ICF, these outcomes were classified into the following two subgroups: body functions and activities.
The subgroups of body functions included the following outcomes: (1) Fugl–Meyer assessment of the lower limbs, (2) Stroke Impact Scale 16, and (3) total motor score from the lower extremities of the American Spinal Injury Association Impairment Scale clinical examination. The subgroup of activities included the 10 m walk test.
Meta-analysis
The results of the meta-analysis showed that RAGT with NIBS exhibited a higher effectiveness than sham stimulation regarding lower limb function (SMD = 0.52, 95% CI = 0.06–0.99, n = 84, I2 = 0%; Figure 2) but not regarding lower limb activities (SMD = −0.13, 95% CI = −0.63–0.38, n = 62, I2 = 0%; Figure 3).
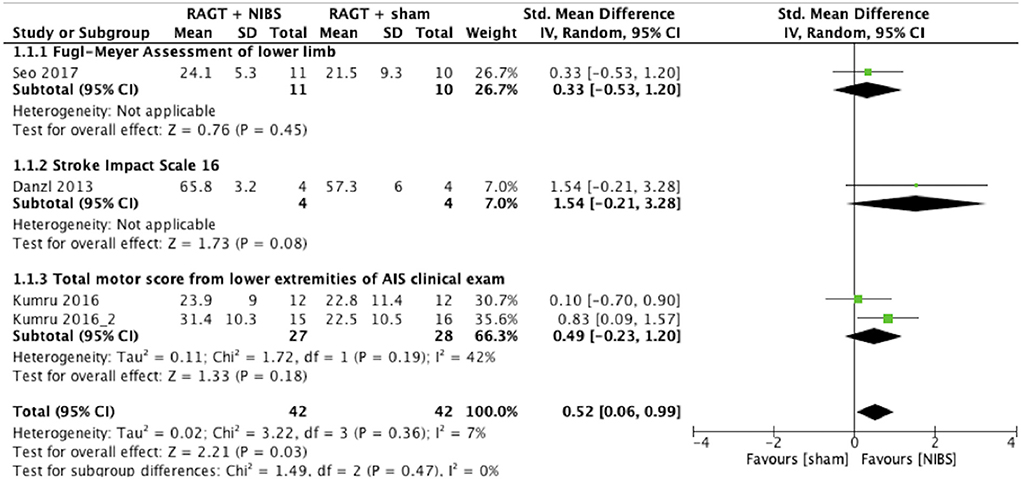
Figure 2. Forest plots: RAGT + NIBS vs. RAGT + sham with outcomes of lower limb body function based on the International Classification of Functioning, Disability, and Health. AIS, American Spinal Injury Association Impairment Scale; NIBS, non-invasive brain stimulation; RAGT, robot assisted gait training.
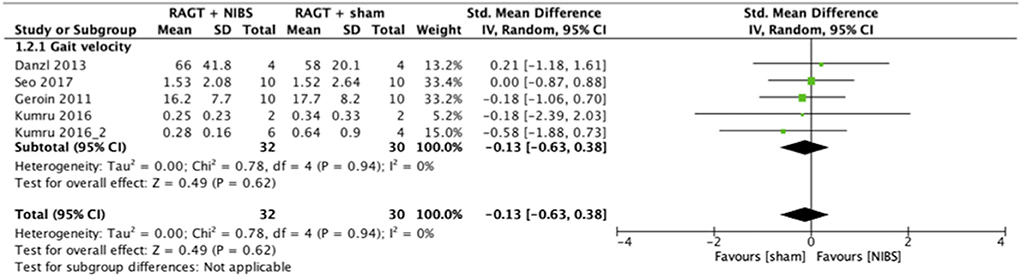
Figure 3. Forest plots: RAGT + NIBS vs. RAGT + sham with outcomes of activities (gait velocity) based on the International Classification of Functioning, Disability, and Health. NIBS, non-invasive brain stimulation; RAGT, robot assisted gait training.
The subgroup analyses focused on the participants' characteristics (stroke or SCI) and type of NIBS (tDCS or rTMS) for secondary outcome measures (Table 2). In the function and activities of the lower limbs, while subgroup analyses were performed separately for stroke and SCI, a greater improvement with RAGT with NIBS than with sham stimulation was not observed. A higher level of improvement in lower limb function was observed for RAGT with rTMS than with sham stimulation but not for RAGT with tDCS. In lower limb activities, while subgroup analyses were performed separately for tDCS and rTMS, a greater improvement with RAGT with NIBS than with sham stimulation was not observed. The subgroup analysis showed no significant differences between participants' characteristics or types of NIBS for body function and activities of the lower limbs.
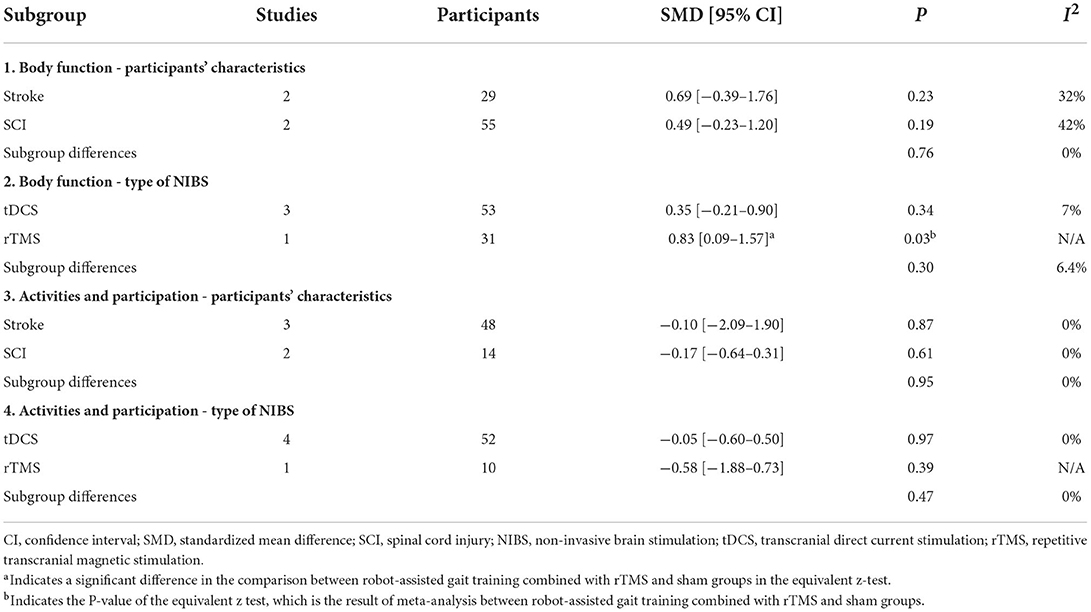
Table 2. Subgroup analysis of participants' characteristics (stroke or SCI) and type of NIBS (tDCS or rTMS).
Statistical heterogeneity
The statistical heterogeneity of all outcome measures was low to moderate (I2 < 50%) in this study (Figures 2, 3; Table 2). Therefore, the results of these meta-analyses were considered reliable.
Adverse effects
Two studies reported adverse effects. One study reported that eight subjects had slightly uncomfortable twitching of the facial muscles or speaking difficulty because of facial-muscle contraction during rTMS, and one subject had a mild headache 1 h after the first rTMS session. The other study reported that one subject in the tDCS group dropped out because of side effects, but no details were provided. Only one study did not report adverse effects.
Discussion
The present study demonstrated that RAGT therapy combined with NIBS had a significantly better effect compared to RAGT combined with sham stimulation on lower limb body function but not activities (gait velocity) in patients with stroke and SCI. However, in terms of the type of NIBS, there were no significant differences between tDCS and rTMS in body function and lower limb activities, and a greater improvement in lower limb function was not observed using RAGT with tDCS than with sham stimulation. The review showed that three of the five studies applied NIBS immediately before RAGT as pre-conditioning for after-effects (Danzl et al., 2013; Kumru et al., 2016b; Seo et al., 2017). Furthermore, four of the five studies used tDCS as the NIBS intervention (Geroin et al., 2011; Danzl et al., 2013; Kumru et al., 2016a; Seo et al., 2017). That is, the results of this review alone are insufficient to determine the efficacy of rTMS immediately before RAGT for patients with lower limb paralysis in improving lower limb function and activities.
The combination of therapeutic exercise and NIBS such as tDCS (Tanaka et al., 2011; Sohn et al., 2013; Murray et al., 2015) and rTMS (Hiscock et al., 2008; Dimyan and Cohen, 2010; Benito et al., 2012) holds promise for facilitating neuromodulation in subjects with corticospinal tract lesions. It is suggested that these effects are based on the principle of activity-dependent neuroplasticity. Therefore, the mechanism for the positive effect of combining the two different modalities could be a compound effect. The integrity and function of neural networks depend on sustained sensory input and, when interrupted, the brain undergoes multiple processes to correct the disruption (Dayan and Cohen, 2011; Hosp and Luft, 2011). Neuroplasticity leads to changes that facilitate the restoration of sensorimotor integration and output (Jenkins and Merzenich, 1987; Wall et al., 2002). These phenomena underlie mechanisms for long-term potentiation of motor learning and sensorimotor remapping in viable brain regions (Chen et al., 2011; Dalise et al., 2014). That is, NIBS might enhance sensorimotor functions by acting on these neuroplastic mechanisms. Both motor training and NIBS are associated with morphological dendritic plasticity changes within the primary motor cortex (M1) and cognitive brain areas, and these effects might explain the efficacy of combined treatment (Barbati et al., 2020; Cambiaghi et al., 2020, 2021).
However, in this study, there was no greater improvement in lower limb function and activities using RAGT with tDCS than with sham stimulation in patients with stroke and SCI. In NIBS, tDCS was the primary intervention in this study. In contrast, the effect size of the combination of rTMS and RAGT was large in patients with SCI (Kumru et al., 2016b). In a previous meta-analysis examining the effect of NIBS on patients with stroke, it was reported that the effect size of rTMS (Hedges' g = 0.46) was larger than that of tDCS (Hedges' g = 0.31) (O'Brien et al., 2018). The present study results also showed that the implementation of rTMS rather than of tDCS before or during RAGT might be effective in enhancing the effect of RAGT, at least in patients with SCI (Kumru et al., 2016b). However, since only one study of RAGT combined with rTMS was included in this meta-analysis, more studies related to RAGT combined with rTMS remain needed. The main significance of this study is therefore that it objectively shows that RAGT combined with tDCS, which has been expected to have effectiveness for lower limb function and activities in patients with stroke and SCI, has no effectiveness.
Applications using tDCS should consider the duration per session, electrode size, number, and placement. One study included in this meta-analysis (Geroin et al., 2011) only applied tDCS for 7 min, which might be too short to generate significant effects. Although this study was also included in the Cochrane review (Elsner et al., 2020) which investigated the effect of tDCS on improving activities of daily living and physical functioning in people after stroke, this is the only study that applied tDCS for <10 min. The guidelines state that shorter tDCS duration has shorter after-effects (Woods et al., 2016). Accordingly, this study was not included in the current meta-analysis of lower limb function due to the lack of outcome. However, it was included in the meta-analysis of activities that may be contributing factors to non-significant effects of tDCS. Furthermore, a previous study reported that smaller electrodes, compared to larger electrodes, may produce more focal current density, which could lead to more effective and localized neural modulation (Bastani and Jaberzadeh, 2013). In another study, the authors applied multi-channel electrodes (Otal et al., 2016). A computational modeling study that compared electrode placements targeting M1 (i.e., electrode placements for all studies included in this meta-analysis) and the cerebellum found that cerebellar stimulation produced substantially higher electric field strengths in the target area compared to M1 stimulation, suggesting that the cerebellum may indeed be a suitable target for tDCS (Gowan and Hordacre, 2020). Future tDCS studies should consider these factors.
Furthermore, more recently, studies on the effects of other NIBS, such as transcranial alternating current stimulation (tACS) and transcranial random noise stimulation (tRNS), have been reported (Terney et al., 2008; Moliadze et al., 2010; Saiote et al., 2013; Inukai et al., 2016; Nakazono et al., 2016). In particular, tRNS has been reported to increase the excitability of cortical primary motor areas more stable than tDCS and tACS (Inukai et al., 2016). Thus, various new NIBS algorithms have been developed. Rather than tDCS, different NIBS algorithms might further improve body function and activities, such as gait and velocity of the lower extremities, if performed during or just before RAGT. No study in which rTMS was applied during RAGT was included in this meta-analysis. Further RCTs are needed because rTMS or various new NIBS implementations during RAGT might be an effective intervention to increase lower motor function in patients with stroke and SCI.
Our study had several limitations that must be considered. It was difficult to compare the outcomes of all the included studies. This was because different studies used different clinical measurements to assess factors such as muscle strength and function and activities of daily living, which hindered their comparison. Thus, we comprehensively conducted the meta-analysis based on the ICF. As a result, statistical heterogeneity was low among the studies. The number of the studies included in the present study was only five RCTs. This might be not satisfactory as a meta-analysis. However, as there is no meta-analysis with including over 100 subjects in previous studies for the areas covered in this study (Byeon, 2020; Shen et al., 2020), the suggestion in the present study must be significant for the practitioners. Although the results of the subgroup analysis focused on the type of NIBS showed that three tDCS studies had no effectiveness and only one rTMS study had effectiveness for lower limb function, the results of this study alone may not be interpretable because of insufficient of studies. If more studies related to RAGT therapy combined with tDCS and rTMS are conducted in the future, it will become clear whether the present subgroup analysis results are due to mechanistic differences; such future studies would further enhance the significance of the findings in this study. This study is valuable for demonstrating this need.
Conclusion
This systematic review and meta-analysis demonstrated the efficacy of RAGT therapy in combination with NIBS for body function but not for activities of the lower limbs in patients with stroke or SCI with motor paralysis. However, in terms of the type of NIBS, there were no significant differences between tDCS and rTMS in body function and lower limb activities, and a greater improvement in lower limb function was not observed using RAGT with tDCS than with sham stimulation. Since only one study of RAGT combined with rTMS was included in this meta-analysis, additional future studies related to RAGT combined with rTMS or different NIBS implementations such as tACS and tRNS are warranted.
Data availability statement
The original contributions presented in the study are included in the article/Supplementary material, further inquiries can be directed to the corresponding author/s.
Author contributions
FK and MK were founded. FK managed the project and resources, supervision, conceptualization, partially writing—review, and editing. WK, SS, and RY contributed to data collection, synthesizing the data, and searching the literature. WK and partially all other authors wrote the article. All authors contributed to revising the manuscript and preparing the final version of the manuscript.
Funding
This study was supported by the Japan Agency for Medical Research and Development (Grant Nos. JP19he2202005 and JP19he2302006).
Acknowledgments
We would like to thank Editage (www.editage.com) for English language editing.
Conflict of interest
Authors FK and MK are the founders of INTEP Inc., a commercial company for the development of rehabilitation devices since July 2020. This company had no association with the device or setup used in the current study.
The remaining authors declare that the research was conducted in the absence of any commercial or financial relationships that could be construed as a potential conflict of interest.
Publisher's note
All claims expressed in this article are solely those of the authors and do not necessarily represent those of their affiliated organizations, or those of the publisher, the editors and the reviewers. Any product that may be evaluated in this article, or claim that may be made by its manufacturer, is not guaranteed or endorsed by the publisher.
Supplementary material
The Supplementary Material for this article can be found online at: https://www.frontiersin.org/articles/10.3389/fnhum.2022.969036/full#supplementary-material
Abbreviations
RAGT, robot-assisted gait training; NIBS, non-invasive brain stimulation; RCT, randomized controlled trial; tDCS, transcranial direct current stimulation; rTMS, repetitive transcranial magnetic stimulation; SMD, standardized mean difference; CI, confidence interval; SCI, spinal cord injury; PEDro scale, physiotherapy evidence database scale; ICF, International Classification of Functioning, Disability, and Health.
References
Abualait, T. S., and Ibrahim, A. I. (2020). Spinal direct current stimulation with locomotor training in chronic spinal cord injury. Saudi. Med. J. 41, 88–93. doi: 10.15537/smj.2020.1.24818
Barbati, S. A., Cocco, S., Longo, V., Spinelli, M., Gironi, K., Mattera, A., et al. (2020). Enhancing plasticity mechanisms in the mouse motor cortex by anodal transcranial direct-current stimulation: the contribution of nitric oxide signaling. Cereb. Cortex. 30, 2972–2985. doi: 10.1093/cercor/bhz288
Bastani, A., and Jaberzadeh, S. (2013). a-tDCS differential modulation of corticospinal excitability: the effects of electrode size. Brain Stimul. 6, 932–937. doi: 10.1016/j.brs.2013.04.005
Benito, J., Kumru, H., Murillo, N., Costa, U., Medina, J., Tormos, J. M., et al. (2012). Motor and gait improvement in patients with incomplete spinal cord injury induced by high-frequency repetitive transcranial magnetic stimulation. Top Spinal Cord. Inj. Rehabil. 18, 106–112. doi: 10.1310/sci1802-106
Bickenbach, J. (2013). International Perspectives On Spinal Cord Injury. Available online at: http://apps.who.int/iris/bitstream/10665/94190/1/9789241564663_eng.pdf?ua=1 (accessed July 28, 2017).
Byeon, H. (2020). Meta-analysis on the effects of transcranial direct current stimulation on naming of elderly with primary progressive aphasia. Int. J. Environ. Res. Public. Health. 17, 1095. doi: 10.3390/ijerph17031095
Cambiaghi, M., Cherchi, L., Masin, L., Infortuna, C., Briski, N., Caviasco, C., et al. (2021). High-frequency repetitive transcranial magnetic stimulation enhances layer II/III morphological dendritic plasticity in mouse primary motor cortex. Behav. Brain Res. 410, 113352. doi: 10.1016/j.bbr.2021.113352
Cambiaghi, M., Crupi, R., Bautista, E. L., Elsamadisi, A., Malik, W., Pozdniakova, H., et al. (2020). The effects of 1-Hz rTMS on emotional behavior and dendritic complexity of mature and newly generated dentate gyrus neurons in male mice. Int. J. Environ. Res. Public Health. 17, E4074. doi: 10.3390/ijerph17114074
Chen, J. L., Lin, W. C., Cha, J. W., So, P. T., Kubota, Y., and Nedivi, E. (2011). Structural basis for the role of inhibition in facilitating adult brain plasticity. Nat. Neurosci. 14,587–594. doi: 10.1038/nn.2799
Cheung, E. Y. Y., Ng, T. K. W., Yu, K. K. K., Kwan, R. L. C., and Cheing, G. L. Y. (2017). Robot-assisted training for people with spinal cord injury: a meta-analysis. Arch. Phys. Med. Rehabil. 98, 2320–2331.e12. doi: 10.1016/j.apmr.2017.05.015
Chieffo, R., Comi, G., and Leocani, L. (2016). Non-invasive neuromodulation in poststroke gait disorders: rationale, feasibility, and state of the art. Neurorehabil. Neural. Repair. 30, 71–82. doi: 10.1177/1545968315586464
Dalise, S., Ambrosio, F., and Modo, M. (2014). Brain plasticity and recovery in preclinical models of stroke. Arch. Ital. Biol. 152, 190–215. doi: 10.12871/00039829201442
Danzl, M. M., Chelette, K. C., Lee, K., Lykins, D., and Sawaki, L. (2013). Brain stimulation paired with novel locomotor training with robotic gait orthosis in chronic stroke: a feasibility study. NeuroRehabilitation 33, 67–76. doi: 10.3233/NRE-130929
Dayan, E., and Cohen, L. G. (2011). Neuroplasticity subserving motor skill learning. Neuron. 72, 443–454. doi: 10.1016/j.neuron.2011.10.008
Dimyan, M. A., and Cohen, L. G. (2010). Contribution of transcranial magnetic stimulation to the understanding of functional recovery mechanisms after stroke. Neurorehabil. Neural. Repair. 24, 125–135. doi: 10.1177/1545968309345270
Elsner, B., Kugler, J., Pohl, M., and Mehrholz, J. (2020). Transcranial direct current stimulation (tDCS) for improving activities of daily living, and physical and cognitive functioning, in people after stroke. Cochrane Database Syst. Rev. 11, CD009645. doi: 10.1002/14651858.CD009645.pub4
Fleming, M. K., Pavlou, M., Newham, D. J., Sztriha, L., and Teo, J. T. (2017). Non-invasive brain stimulation for the lower limb after stroke: what do we know so far and what should we be doing next? Disabil. Rehabil. 39, 714–720. doi: 10.3109/09638288.2016.1161835
Geroin, C., Picelli, A., Munari, D., Waldner, A., Tomelleri, C., and Smania, N. (2011). Combined transcranial direct current stimulation and robot-assisted gait training in patients with chronic stroke: a preliminary comparison. Clin. Rehabil. 25, 537–548. doi: 10.1177/0269215510389497
Ghayour-Najafabadi, M., Memari, A. H., Hosseini, L., Shariat, A., and Cleland, J. A. (2019). Repetitive transcranial magnetic stimulation for the treatment of lower limb dysfunction in patients poststroke: a systematic review with meta-analysis. J. Stroke Cerebrovasc. Dis. 28, 104412. doi: 10.1016/j.jstrokecerebrovasdis.2019.104412
Gowan, S., and Hordacre, B. (2020). Transcranial direct current stimulation to facilitate lower limb recovery following stroke: current evidence and future directions. Brain Sci. 10, E310. doi: 10.3390/brainsci10050310
Hiscock, A., Miller, S., Rothwell, J., Tallis, R. C., and Pomeroy, V. M. (2008). Informing dose-finding studies of repetitive transcranial magnetic stimulation to enhance motor function: a qualitative systematic review. Neurorehabil. Neural. Repair. 22, 228–249. doi: 10.1177/1545968307307115
Hosp, J. A., and Luft, A. R. (2011). Cortical plasticity during motor learning and recovery after ischemic stroke. Neural. Plast. 2011, 871296. doi: 10.1155/2011/871296
Inukai, Y., Saito, K., Sasaki, R., Tsuiki, S., Miyaguchi, S., Kojima, S., et al. (2016). Comparison of three non-invasive transcranial electrical stimulation methods for increasing cortical excitability. Front. Hum. Neurosci. 10, 668. doi: 10.3389/fnhum.2016.00668
Jenkins, W. M., and Merzenich, M. M. (1987). Reorganization of neocortical representations after brain injury: a neurophysiological model of the bases of recovery from stroke. Prog. Brain Res. 71, 249–266. doi: 10.1016/S0079-6123(08)61829-4
Jørgensen, H. S., Nakayama, H., Raaschou, H. O., and Olsen, T. S. (1995). Recovery of walking function in stroke patients: the copenhagen stroke study. Arch. Phys. Med. Rehabil. 76, 27–32. doi: 10.1016/S0003-9993(95)80038-7
Krebs, H. I., and Hogan, N. (2006). Therapeutic robotics: a technology push: stroke rehabilitation is being aided by robots that guide movement of shoulders and elbows, wrists, hands, arms and ankles to significantly improve recovery of patients. Proc IEEE Inst Electr. Electron. Eng. 94, 1727–1738. doi: 10.1109/JPROC.2006.880721
Kumru, H., Benito-Penalva, J., Valls-Sole, J., Murillo, N., Tormos, J. M., Flores, C., et al. (2016b). Placebo-controlled study of rTMS combined with Lokomat® gait training for treatment in subjects with motor incomplete spinal cord injury. Exp. Brain Res. 234, 3447–3455. doi: 10.1007/s00221-016-4739-9
Kumru, H., Murillo, N., Benito-Penalva, J., Tormos, J. M., and Vidal, J. (2016a). Transcranial direct current stimulation is not effective in the motor strength and gait recovery following motor incomplete spinal cord injury during Lokomat(®) gait training. Neurosci. Lett. 620, 143–147. doi: 10.1016/j.neulet.2016.03.056
Li, Y., Fan, J., Yang, J., He, C., and Li, S. (2018). Effects of repetitive transcranial magnetic stimulation on walking and balance function after stroke: a systematic review and meta-analysis. Am. J. Phys. Med. Rehabil. 97, 773–781. doi: 10.1097/PHM.0000000000000948
Mehrholz, J., Kugle,r, J., and Pohl, M. (2012). Locomotor training for walking after spinal cord injury. Cochrane Database Syst. Rev. 11, CD006676. doi: 10.1002/14651858.CD006676.pub3
Mehrholz, J., Thomas, S., Werner, C., Kugler, J., Pohl, M., and Elsner, B. (2020). Electromechanical-assisted training for walking after stroke. Cochrane Database Syst. Rev. 10, CD006185. doi: 10.1002/14651858.CD006185.pub5
Moher, D., Liberati, A., Tetzlaff, J., Altman, D. G., and P. R. I. S. M. A., Group (2009). Preferred reporting items for systematic reviews and meta-analyses: the PRISMA statement. PLoS Med. 6, e1000097. doi: 10.1371/journal.pmed.1000097
Moliadze, V., Antal, A., and Paulus, W. (2010). Boosting brain excitability by transcranial high frequency stimulation in the ripple range. J. Physiol. 588, 4891–4904. doi: 10.1113/jphysiol.2010.196998
Murray, L. M., Edwards, D. J., Ruffini, G., Labar, D., Stampas, A., Pascual-Leone, A., et al. (2015). Intensity dependent effects of transcranial direct current stimulation on corticospinal excitability in chronic spinal cord injury. Arch. Phys. Med. Rehabil. 96, S114–S121. doi: 10.1016/j.apmr.2014.11.004
Nakazono, H., Ogata, K., Kuroda, T., and Tobimatsu, S. (2016). Phase and frequency-dependent effects of transcranial alternating current stimulation on motor cortical excitability. PLoS ONE. 11, e0162521. doi: 10.1371/journal.pone.0162521
O'Brien, A. T., Bertolucci, F., Torrealba-Acosta, G., Huerta, R., Fregni, F., and Thibaut, A. (2018). Non-invasive brain stimulation for fine motor improvement after stroke: a meta-analysis. Eur. J. Neurol. 25, 1017–1026. doi: 10.1111/ene.13643
Otal, B., Dutta, A., Foerster, Á., Ripolles, O., Kuceyeski, A., Miranda, P. C., et al. (2016). Opportunities for guided multichannel non-invasive transcranial current stimulation in poststroke rehabilitation. Front. Neurol. 7, 21. doi: 10.3389/fneur.2016.00021
Oujamaa, L., Relave, I., Froger, J., Mottet, D., and Pelissier, J. Y. (2009). Rehabilitation of arm function after stroke. Literature review. Ann. Phys. Rehabil. Med. 52, 269–293. doi: 10.1016/j.rehab.2008.10.003
Saiote, C., Turi, Z., Paulus, W., and Antal, A. (2013). Combining functional magnetic resonance imaging with transcranial electrical stimulation. Front. Hum. Neurosci. 7, 435. doi: 10.3389/fnhum.2013.00435
Sekhon, L. H., and Fehlings, M. G. (2001). Epidemiology, demographics, and pathophysiology of acute spinal cord injury. Spine. 26, S2–12. doi: 10.1097/00007632-200112151-00002
Seo, H. G., Lee, W. H., Lee, S. H., Yi, Y., Kim, K. D., and Oh, B. M. (2017). Robotic-assisted gait training combined with transcranial direct current stimulation in chronic stroke patients: a pilot double-blind, randomized controlled trial. Restor. Neurol. Neurosci. 35, 527–536. doi: 10.3233/RNN-170745
Shen, Z., Li, Z., Ke, J., He, C., Liu, Z., Zhang, D., et al. (2020). Effect of non-invasive brain stimulation on neuropathic pain following spinal cord injury: a systematic review and meta-analysis. Medicine. 99, e21507. doi: 10.1097/MD.0000000000021507
Sohn, M. K., Jee, S. J., and Kim, Y. W. (2013). Effect of transcranial direct current stimulation on postural stability and lower extremity strength in hemiplegic stroke patients. Ann. Rehabil. Med. 37, 759–765. doi: 10.5535/arm.2013.37.6.759
Takashima, N., Arima, H., Kita, Y., Fujii, T., Miyamatsu, N., Komori, M., et al. (2017). Incidence, management and short-term outcome of stroke in a general population of 1.4 million Japanese - Shiga stroke registry. Circ. J. 81, 1636–1646. doi: 10.1253/circj.CJ-17-0177
Tanaka, S., Takeda, K., Otaka, Y., Kita, K., Osu, R., Honda, M., et al. (2011). Single session of transcranial direct current stimulation transiently increases knee extensor force in patients with hemiparetic stroke. Neurorehabil. Neural. Repair. 25, 565–569. doi: 10.1177/1545968311402091
Terney, D., Chaieb, L., Moliadze, V., Antal, A., and Paulus, W. (2008). Increasing human brain excitability by transcranial high-frequency random noise stimulation. J. Neurosci. 28, 14147–14155. doi: 10.1523/JNEUROSCI.4248-08.2008
Tung, Y. C., Lai, C. H., Liao, C. D., Huang, S. W., Liou, T. H., and Chen, H. C. (2019). Repetitive transcranial magnetic stimulation of lower limb motor function in patients with stroke: a systematic review and meta-analysis of randomized controlled trials. Clin. Rehabil. 33, 1102–1112. doi: 10.1177/0269215519835889
Tyson, S. F., Hanley, M., Chillala, J., Selley, A. B., and Tallis, R. C. (2007). The relationship between balance, disability, and recovery after stroke: predictive validity of the Brunel Balance Assessment. Neurorehabil. Neural. Repair. 21, 341–346. doi: 10.1177/1545968306296966
van de Port, I. G., Kwakkel, G., van Wijk,. I., and Lindeman, E. (2006). Susceptibility to deterioration of mobility long-term after stroke: a prospective cohort study. Stroke. 37, 167–171. doi: 10.1161/01.STR.0000195180.69904.f2
Veerbeek, J. M., Koolstra, M., Ket, J. C., van Wegen, E. E., and Kwakkel, G. (2011). Effects of augmented exercise therapy on outcome of gait and gait-related activities in the first 6 months after stroke: a meta-analysis. Stroke. 42, 3311–3315. doi: 10.1161/STROKEAHA.111.623819
Wall, J. T., Xu, J., and Wang, X. (2002). Human brain plasticity: an emerging view of the multiple substrates and mechanisms that cause cortical changes and related sensory dysfunctions after injuries of sensory inputs from the body. Brain Res. Rev. 39, 181–215. doi: 10.1016/S0165-0173(02)00192-3
Keywords: transcranial direct current stimulation, repetitive transcranial magnetic stimulation, robotics, stroke, spinal cord injury, lower limb
Citation: Kuwahara W, Sasaki S, Yamamoto R, Kawakami M and Kaneko F (2022) The effects of robot-assisted gait training combined with non-invasive brain stimulation on lower limb function in patients with stroke and spinal cord injury: A systematic review and meta-analysis. Front. Hum. Neurosci. 16:969036. doi: 10.3389/fnhum.2022.969036
Received: 14 June 2022; Accepted: 19 July 2022;
Published: 16 August 2022.
Edited by:
Akiyoshi Matsugi, Shijonawate Gakuen University, JapanReviewed by:
Sofia Rita Cardoso Fernandes, University of Lisbon, PortugalTadamitsu Matsuda, Juntendo University, Japan
Copyright © 2022 Kuwahara, Sasaki, Yamamoto, Kawakami and Kaneko. This is an open-access article distributed under the terms of the Creative Commons Attribution License (CC BY). The use, distribution or reproduction in other forums is permitted, provided the original author(s) and the copyright owner(s) are credited and that the original publication in this journal is cited, in accordance with accepted academic practice. No use, distribution or reproduction is permitted which does not comply with these terms.
*Correspondence: Fuminari Kaneko, f-kaneko@tmu.ac.jp