In situ spatiotemporal mapping of 3-hydroxy-3-methylglutaryl-CoA reductase (HMGCR) inhibitor in pineapple (Ananas comosus) fruit tissue by MALDI mass spectrometry imaging
- 1Center for Natural Drug Discovery and Development (CND3), University of San Agustin, Iloilo, Philippines
- 2JY Campos Centre, Del Monte Philippines Inc., Taguig, Philippines
- 3Center for Chemical Biology and Biotechnology (C2B2), University of San Agustin, Iloilo, Philippines
- 4Agricultural Biotechnology Research Center, Biotechnology Center in Southern Taiwan, Academia Sinica, Tainan, Taiwan
- 5Department of Chemistry, College of Liberal Arts, Sciences, and Education, University of San Agustin, Iloilo, Philippines
- 6Balik Scientist Program, Department of Science and Technology Philippine Council for Health Research and Development (DOST-PCHRD), Taguig, Philippines
- 7Department of Biology, College of Liberal Arts, Sciences, and Education, University of San Agustin, Iloilo, Philippines
Pineapple (Ananas comosus) fruit is a functional food that has become a part of healthy diets worldwide due to its nutrition and health benefits. Given this concept, the potential anti-hypercholesterolemic activity of pineapple fruit was assessed using in vitro HMG-CoA reductase (HMGCR) inhibition assay. Our findings show that pineapple fruit (PAF) extract showed HMGCR inhibition activity. These findings led to partial purification of PAF extract by gel filtration chromatography (GFC) whereby fraction PAF 06 exhibited 100.00% HMGCR inhibition at 10 mg/mL. Matrix assisted laser desorption ionization (MALDI) mass spectrometry profiling, HRMS, MS/MS, and dereplication identified N1, N10-diferuloylspermidine as potential HMGCR inhibitor in pineapple fruit. The MALDI mass spectrometry imaging (MSI) enabled the in situ spatiotemporal mapping of the HMGCR inhibitor, N1, N10-diferuloylspermidine, in the pineapple fruit tissue, with localization concentrated mainly in the tissue near the peel/shell and a gradual decrease towards the flesh and ovary. The MALDI MSI provided the first evidence of in situ spatial localization of N1, N10-diferuloylspermidine, a potential HMGCR inhibitor in pineapple while maintaining the cellular and structural features of the fruit tissues.
1 Introduction
Pineapple, Ananas comosus, is one of the most widely cultivated and economically important tropical fruits globally that is available as fresh or canned products (i.e., sliced or diced fruit and juice) (Fang et al., 2016; Yabor et al., 2021). As a functional food, pineapple has become a part of healthy diets worldwide since it provides nutrition and health benefits. Its nutrients include carbohydrates, dietary fiber, sugars, organic acids (such as citric and malic acids), ascorbic acid, niacin and thiamin as vitamins, minerals including potassium, magnesium, manganese, and copper, and a complex mixture of volatile oil compounds, which are associated with flavor and aroma (Wei et al., 2011; Mohd Ali et al., 2020). The health benefits of pineapple fruit include antioxidant, anti-inflammatory, anti-thrombotic, fibrinolytic, and antibacterial properties (Maurer, 2001; Chobotova et al., 2010; Ramli et al., 2017). These bioactive properties can be attributed to a significant amount of bromelains, a mixture of protein-digesting enzymes, which is regarded highly for applications in food, cosmetic, pharmaceutical, and clinical industries (Pavan et al., 2012; Jančič and Gorgieva, 2022). The cosmetic use of bromelain is due to its proteolytic activity, which is linked to its antibacterial activity against acne-causing pathogens such as Staphylococcus aureus and Propionibacterium acnes (Abbas et al., 2021). In addition, bromelain is promoted as a dietary supplement and an alternative therapeutic agent to non-steroidal anti-inflammatory drugs (NSAIDs) to reduce pain and inflammation (Brien et al., 2004; Hossain et al., 2015). Bromelain also decreases risk factors contributing to heart ailments, such as reducing blood clots and breaking down cholesterol plaques via its potent fibrinolytic activity (Metzig et al., 1999; Pavan et al., 2012).
While reports showed that bromelain from pineapple fruit affects cardiovascular and circulation due to its fibrinolytic activity, the scientific literature has a dearth of information on the phytochemicals or secondary metabolites in pineapple fruit that can reduce hypercholesterolemia, a metabolic disorder caused by an increase in blood cholesterol concentration. Hypercholesterolemia has become a worldwide public health issue because it contributes significantly to the severity and occurrence of cardiovascular diseases and other serious health problems (Koletzko, 2022). Treatment of hypercholesterolemia requires taking cholesterol-lowering drugs, such as statins (Stone, 1996). Statins, a class of medications that lower blood cholesterol levels by reducing the production of cholesterol by the liver, are among the most commonly prescribed drugs for regulating cholesterol levels. Statins are competitive inhibitors of hydroxyl-methyl glutaryl coenzyme-A reductase (HMGCR). This rate-limiting enzyme catalyzes the conversion of HMG-CoA to mevalonate in the cholesterol biosynthesis pathway and efficiently reduces the serum levels of low-density lipoprotein (LDL) (Salvamani et al., 2016). However, studies reveal that long-term use of statin drugs increases the risk of adverse effects, such as liver and muscle damage, in patients with multiple medical co-morbidities (Baskaran et al., 2015). One to ten percent of patients taking statin drugs were documented to experience myalgia. Moreover, hypothyroidism, polypharmacy, and alcohol abuse were determined to be the most common risk factors for statin-related myopathy (Ramkumar et al., 2016). Because of the potential adverse effects of statins, there is a need for an alternative therapeutic compound from functional food, such as pineapple fruit, that can lower blood cholesterol levels with less toxicity and free of side effects.
Preliminary studies show that plant extract of pineapple (A. comosus) blocks the uptake of cholesterol into CaCo-2 cells as well as inhibits the HMGCR inhibition activity by 46.8% at 100 μg/mL concentration (Duangjai et al., 2011). However, these studies on the HMGCR inhibitory activity focus specifically on pineapple leaves. Therefore, as part of our continuing research on bioactive secondary metabolites from functional foods (Suarez et al., 2021; Balida et al., 2022), we investigated the ripe fruit of MD-2 (syn. Gold Extra Sweet) Del Monte pineapple cultivar for 3-hydroxy-3-methylglutaryl-CoA reductase (HMGCR) inhibition activity. Herein, we describe the 1) in vitro HMGCR inhibition activities of PAF extracts and its gel filtration chromatography fractions, 2) the putative chemical identity of the HMGCR inhibitor by MALDI MS, HRMS and MS/MS, and 3) in situ spatiotemporal localization and distribution of the HMGCR inhibitor in the pineapple fruit tissue by MALDI mass spectrometry imaging.
2 Materials and methods
2.1 Sample collection
A total of 24 ripe pineapple fruits (cultivar: MD-2) of shell 1–2, i.e., green to yellow green from Del Monte Philippines, Inc. Plantation, Manolo Fortich, Bukidnon, Philippines were harvested and maintained at 4°C for 2 days.
2.2 Sample preparation
Before the collective extraction of fruit, dimensions of length, weight, and girth of the samples were measured. Next, the bottom slip and the crown of the fruit were discarded. Next, the core was removed from the flesh using a pineapple slicer, and the peel was removed using a knife. Finally, the flesh samples were cut into chunks, and the juice was extracted using Breville BJE820 at level 1 speed, approximately 6,500 rpm.
After fruit extraction, the samples were subjected to coarse filtration using eight layers of muslin cloth and two steps of cotton gravity filtration. Next, vacuum filtration was performed in four repeated steps using layers of well-stacked ordinary filter paper, cotton, and Whatman filter paper #1 to eliminate fibers further and clarify the extract. The clear fruit extract was distributed to 50 mL Falcon tubes and was stored at −80°C. After overnight freezing, the samples were lyophilized.
2.3 Gel filtration chromatography (GFC)
The lyophilized PAF extract was dissolved in distilled water at a concentration of 500 mg in 1 mL. The sample was filtered syringe (pore size: 0.2 μm) before loading through Sephadex G-75 resin (pore size: 40–120 μm). The column’s measured bed height and radius were 42.5 and 1.625 cm, respectively, with a column volume of 352.57 mL. Water was used as the mobile phase, and 30% of the bed volume was collected as void. The eluate was collected up to 140 fractions at approximately 5.5 mL per test tube at a 1.2 mL per minute flow rate. One hundred microliters of each collected fraction were transferred in a 96-well plate (4titude UltraVision™ Plate 96 Well) and profiled by UV-Vis using a microplate reader (BMG Labtech CLARIOStar®). Post-run calculations were performed in the MARS software, wherein the local maxima of the raw data were extracted at the highest sensitivity, and the scan was smoothed at three units to minimize the background noise. The λmax obtained for each of the fractions were plotted and then pooled into 11 major fractions for the PAF extract. The pooled fractions were frozen and freeze-dried until further analysis.
2.4 3-Hydroxy-3-methylglutaryl-CoA reductase (HMGCR) inhibition assay
The HMGCR inhibitory activity was determined using an HMGCR inhibition assay kit (Sigma-Aldrich, St. Louis, MO, United States). The assay was based on the spectrophotometric measurement of the decrease in absorbance at 340 nm, representing the oxidation of NADPH by the catalytic subunit of 3-hydroxy-3-methylglutaryl-CoA reductase (HMGCR) in the presence of the substrate HMG-CoA. All storage and reagent preparations were performed according to the protocol (CS1090, Sigma Aldrich, St. Louis, MO, United States).
Following the manufacturer’s protocol, assay buffer (×1) was added to each well, 184 μL for the blank, 182 μL for the activity control, and 181 μL for the inhibition control, and 162 μL for samples. Pravastatin (2.1 μg/mL, recommended concentration in the protocol), a clinically known antihypercholesterolemic drug was used as positive control. Freeze-dried pineapple extracts and partially purified fractions were dissolved in water to obtain its desired test concentration. The sample solution was added to its respective wells at 20 μL as the treatment. NADPH solution was added at 4 μL and 12 μL HMGCR substrate were also added to the treatments. The HMGCR enzyme (2 μL) was kept on ice until added to controls, and samples. The total volume of the reaction mixture was 200 μL. Using a plate reader (CLARIOStar® BMG LABTECH, Ortenberg, Germany), the absorbance at 340 nm was monitored every 20 s for up to 10 min. The assay was done in triplicates and three trials.
One unit will convert 1 μmol of NADPH to NADP+ per 1 min at 37°C. The inhibitory activity was calculated relative to the untreated HMGCR enzyme. A sample with HMGCR inhibitory activity of >80% was considered as active. The assay was performed in triplicates, three trials. In three trials, the pH of each reaction mixture was also determined using the Macherey-Nagel™ (MN) pH indicator.
2.5 Matrix-assisted laser desorption/ionization—time of flight—mass spectrometry (MALDI-TOF-MS) of HMGCR inhibitory active GFC fraction
The compounds in each GFC PAF fraction were profiled using MALDI-TOF mass spectrometry (Aotoflex Speed™ Bruker Daltonics). The spectra were then correlated with the reported HMGCR inhibitory activity of the GFC fractions.
The GFC PAF fractions (100 μg) were spotted on the MTP 384 target plate (Bruker Daltonics) mixed with α-cyano-4-hydroxycinnamic acid (CHCA, 10 mg in 75% MeOH) or 2,5-dihydroxybenzoic acid (DHB, 10 mg/mL) as a matrix and allowed to dry in vacuo. The mass ions were detected by Autoflex Speed MALDI-TOF mass spectrometry (Bruker Daltonics) in the linear positive mode range of m/z 200–20,000, and the laser frequency was 500 Hz with a laser power of 50%. There were three technical trial MS run per sample for data acquisition and validation. Mass calibration was performed using Protein and Peptide Calibration Standard for Mass Spectrometry (Bruker Daltonics).
2.6 Native polyacrylamide gel electrophoresis (PAGE)
Native PAGE experiment was performed to gain a preliminary peptide or protein profile of the extract and its corresponding GFC fractions, which aided us in determining whether the bioactivities were due to proteins or small metabolites.
Freeze-dried GFC PAF fractions were freshly prepared and centrifuged at ×20,000 g. An acrylamide gel was prepared and loaded into the gel cassettes to polymerize for about 1 h prior to transferring the cassette to the tank filled with 2.5 mM Tris and 92 mM glycine, pH 8.3 running buffer (Bio-Rad Mini-PROTEAN® Tetra Cell). An aliquot of the supernatant of the samples was obtained and added to the same amount of sample loading buffer. The mixture was then placed into the wells along with a protein marker (Bio-Rad Precision Plus Protein Dual Xtra Standard) for reference. The gels were subjected to electrophoresis until the protein marker reached the gel bottom. The gels were fixed for 1 h with 1:5 acetic acid/methanol, stained using 10% w/v Coomassie Brilliant Blue dye in 1:5 acetic acid/methanol, and destained with 1:4 acetic acid/methanol to reveal the protein bands.
2.7 Spatial distribution and localization of pineapple HMGCR inhibitor by matrix-assisted laser desorption/ionization—time of flight–mass spectrometry imaging (MALDI-TOF-MSI)
Fresh pineapple fruit (cultivar: MD-2) was cut according to its major parts, covering the core, flesh, and peel (Supplementary Figure S1). The pineapple fruit tissue cross sections were cut with approximately 3 cm width, 3 cm height, and 1 cm thickness. To maintain the tissue morphology and ensure precise tissue sectioning, each fresh fruit tissue block was immediately embedded in agarose (3% in ultra-pure water) and froze instantly in ultra-low temperature freezer (−80°C) overnight. Agarose has been successfully utilized as MSI-compatible embedding medium as highlighted by several studies (Gemperline et al., 2015; Dong et al., 2016). The frozen tissues were then cryosectioned at 50 μm thickness using cryostat (Leica CM 1950, Nussloch, Germany) cooled to −15°C. The thickness of current MSI studies on water rich plant tissues is set to ca. 50 μm, providing optimum MSI performance and practicality (Peukert et al., 2012; Dong et al., 2016). The tissue slices were swiftly and carefully mounted onto a MALDI plate to avoid folding and tearing in the tissue sections. The cut tissue was air dried prior to application of matrix. The 2,5-dihydroxybenzoic acid (DHB, 40 mg/mL, 70:30 (v:v) MeOH/H2O) as matrix of choice was applied using an automated sprayer (TM-Sprayer™, HTX Technologies, North Carolina, United States). The solvent of the sprayer was 50% MeOH at 80°C dry gas temperature, 10 psi N2 pressure, 0.1 mL/min flow rate, 1,250 mm/min velocity, and ten passes with 1 mm track passing space in CC pattern. The gas flow rate is 3.0 L/min, 30 s drying time with a nozzle height of 65 mm to ensure the even distribution of matrix on the fruit tissue. There were three cross-section tissues prepared to represent three (3) technical runs for MALDI MSI experiments.
MALDI-TOF mass spectrometry imaging data of the fruit tissue was acquired in the positive ion mode at a resolution range of m/z 200–7,000 Da with a spatial resolution of 200 μm at a laser energy setting of 1,000 Hz and a laser power of 70%. The area selected for imaging was defined using FlexImaging™ software (V3.0, Bruker Daltonics). Mass calibration was performed using Protein and Peptide Calibration Standard for Mass Spectrometry (Bruker Daltonics). Further, the raw data were processed and analyzed in FlexAnalysis™ software (V3.4, Bruker Daltonics) and ClinProTools (V3.0, Bruker Daltonics). Fruit tissue samples were also stained with toluidine blue O (0.02%) directly on the MALDI imaged tissues after the imaging analysis to profile its histological properties.
2.8 Putative identification of anti-hypercholesterolemic compound in pineapple fruit
High-resolution mass accuracy experiments were performed using the LTQ Orbitrap XL ETD mass spectrometer (Thermo Fisher Scientific, San Jose, CA) with a standard ESI ion source. Five μL of the sample was flow injected at a rate of 50 μL/min in 80% ACN/H2O (with 0.1% formic acid) by the Ultimate 3000 RSLC system from Dionex (Dionex Corporation, Sunnyvale, CA). Full-scan MS conditions were set at a mass range of m/z 100–2000 or 500–4,000 and a resolution of 60,000 at m/z 400. The target ions were sequentially isolated for MS/MS by LTQ. Electrospray voltage was maintained at 4 kV and the capillary temperature was set at 275°C. The MS/MS experiment was done at 35 V collision energy. The identity of the metabolites was based on a comparison with online databases such as METLIN™, PubChem, and ChemSpider. Fragmentation of the metabolite of interest was also generated for essential uses in structural elucidation and confirmation of the metabolite identity.
2.9 Statistical analysis
Results were expressed as mean ± standard deviation except for the initial HMGCR inhibition assay of pineapple fruit extract. The data were analyzed by one-way ANOVA followed by Tukey’s post hoc test for multiple comparisons using Graph Pad Prism® 9 software (H.J. Motulsky, Prism 9 Statistics Guide, GraphPad Software Inc., San Diego, 258 CA, United States, www.Graph Pad.com). The differences between groups were regarded as statistically significant at p ≤ 0.05.
3 Results
3.1 HMGCR inhibitory activity of pineapple fruit (PAF) extract
We hypothesized that the active HMGCR inhibitor compound in the crude PAF extract is very little, hence we tested the sample at 100 mg/mL compared to the positive control which is a pure pravastatin at 2.1 μg/mL. The calculated % HMGCR inhibitory activity, as shown in Figure 1 confirmed that PAF extract exhibited high HMGCR inhibition activity at 74.17% ± 6.80% (100 mg/mL) compared to the positive control pravastatin (56.46% ± 0.06%) at 2.1 μg/mL. During the assay, we noted that PAF extract is acidic at pH 4.0. We speculated that the acidity of the PAF extract have denatured the enzyme, thus demonstrating the observed HMGCR inhibition. To test this hypothesis, we tested PAF extract at 25 mg/mL while maintaining the acidity at pH 4.0. The assay showed no HMGCR inhibitory activity, thus confirming that enzyme denaturation is not the plausible cause of HMGCR inhibition (Supplementary Figure S2). This finding is supported by several reports revealing that the HMGCR optimal pH to convert HMG-CoA substrate to mevalonate ranges from 5.5 to 7.0 (Bochar et al., 1997; Rodwell et al., 2000). Notably, the HMGCR inhibitory activity was diminished at 25 mg/mL in PAF extract, i.e., four-fold lower than 100 mg/mL showing HMGCR activity, thus, indicating concentration dependent activity of PAF extract.
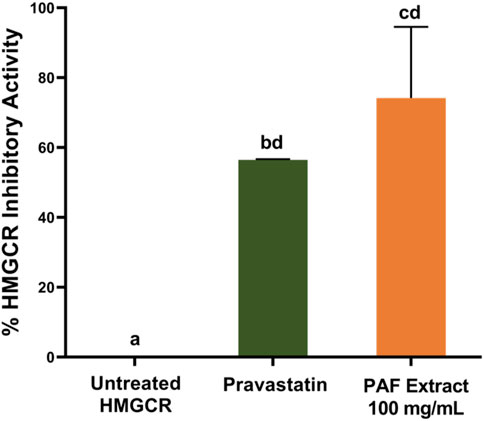
FIGURE 1. The HMGCR inhibition activity of pineapple fruit (PAF) extract at 100 mg/mL. Error bars represent the standard error of the mean. Different letters are differed significantly by Tukey’s multiple comparison test (p ≤ 0.05), (n = 3).
3.2 Gel filtration chromatography (GFC) and HMGCR inhibitory activity assay
The PAF extracts were semi-purified and separated according to their molecular size to determine which fractions contain the potential HMGCR inhibitor. Each batch of GFC purified PAF extract yielded 140 fractions of ca. 5.5 mL eluate volume. A total of five batches of PAF extract samples were purified by GFC. Since the GFC fractions collected were not pigmented, the eluates were profiled by UV-Vis spectrometry. The GFC fractions were pooled into 11 major fractions (namely, PAF 01 to 11) based on UV profile (Supplementary Figure S3). From the plot, fractions PAF 04 and PAF 05 were the only fractions that showed absorbance in the UV region. Fraction PAF 04 showed absorbance at 278–280 nm. The remaining PAF fractions showed absorbance in the visible range of the light spectrum. Interestingly, the bulk of the sample was distributed in fraction PAF 05, having the absorption maxima at 265–275 nm. Notably, there was also absorption at 400 nm–600 nm. However, the UV-Vis profile does not provide conclusive data for the identity of these compounds. Therefore, each GFC fraction was subjected to initial screening of HMGCR inhibition assay with its respective concentration, i.e., PAF 01–06 at 10 mg/mL and PAF 07–11 at 5 mg/mL. The latter concentration was due to limitation of the sample yield after GFC purification. In the HMGCR inhibition assay of GFC fractions, a sample is considered active if it shows >80% HMGCR inhibitory activity (see Materials and Methods Section). The results showed that GFC fractions displayed HMGCR inhibition assay at 10 mg/mL, indicating a 10-fold decrease in concentration to exhibit high HMGCR inhibition compared to the pineapple fruit (PAF) extract which was tested at 100 mg/mL. Notably, fractions PAF 03 and 06 demonstrated higher HMGCR inhibitory activity at 10 mg/mL compared to pravastatin (positive control, 2.1 μg/mL) as shown in Figure 2; Supplementary Figure S4. Fraction PAF 03, however, displayed different progress curve behavior than PAF 06 at 10 min reaction time, PAF 03 has weaker inhibitory activity relative to pravastatin and PAF 06 (Supplementary Figure S5). Perhaps the molecular sizes of these compounds found in this fraction affect the mechanisms of inhibition in which PAF 03 contains relatively larger molecules than PAF 06 based on its GFC eluting time. To highlight, PAF 06 showed 100% ± 0.72% HMGCR inhibitory activity, followed by fraction PAF 03 with 86.27% ± 1.09% (Supplementary Figure S4). From this information, the assay focused on the validation of HMGCR inhibition of PAF 06 and 03 to confirm our results. Interestingly, subsequent trials revealed that PAF 06 consistently demonstrates 100.00% inhibition against the HMGCR enzyme at 10 mg/mL concentration. Notably, PAF 07 exhibits HMGCR inhibition (82.6%) at 5 mg/mL concentration, which is half the concentration of PAF 06 at 10 mg/mL (Figure 2). This means that the putative HMGCR inhibitor could be present in PAF 07 in higher amount than in PAF 06.
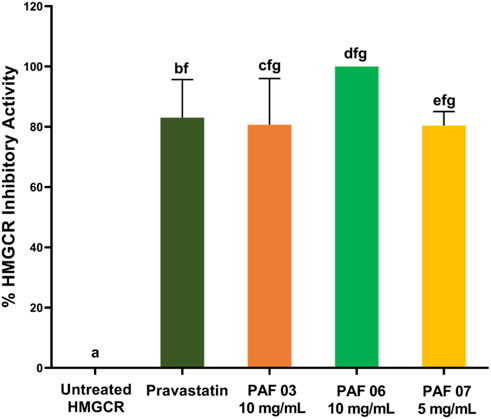
FIGURE 2. The HMGCR inhibition activities of the active GFC fractions of pineapple fruit (PAF) crude extracts. Error bars represent the standard error of the mean. Different letters are differed significantly by Tukey’s multiple comparison test (p ≤ 0.05), (n = 3).
We then sought if the HMGCR active fraction contains protein-like molecules, e.g., bromelain-like compounds. To check for the presence of proteins in the PAF fractions, we performed the native PAGE analysis. The PAF 03 that demonstrated HMGCR inhibition has displayed protein bands in PAGE gel. Interestingly, no protein bands were observed in PAF 06 and 07 that elicited the HMGCR inhibition (Supplementary Figure S6). The PAGE experiment assay findings indicate that the HMGCR inhibitory activities in fractions PAF 06 and 07 are not due to protein or peptides but small metabolites. Overall, the promising HMGCR inhibition activity of PAF 06 and 07 are associated with small secondary metabolites found in pineapple fruit. Therefore, this study is focused on the small molecules present in PAF extract that displayed the HMGCR inhibition.
3.3 Matrix-assisted laser desorption/ionization—time of flight—mass spectrometry (MALDI-TOF-MS) of active GFC fractions of pineapple fruit extract
The HMGCR inhibition assay revealed that GFC fractions (PAF 03, PAF 06, and PAF 07) contain the active metabolites responsible for the inhibition of HMGCR (>80% inhibition, Figure 2). Hence, it is imperative to investigate its chemical profile and screen for the potential identity of HMGCR inhibitors by mass spectrometry. Among all the PAF fractions, it is important to highlight that PAF 06 consistently exhibited the highest HMGCR inhibition activity at 100% (10 mg/mL). To find the mass ion (m/z) unique in the bioactive fraction PAF 06, we performed MALDI mass spectrometry using α-cyano-4-hydroxycinnamic acid (CHCA) as a matrix. Its MALDI mass spectrum was compared to a non-active fraction, PAF 05 and an HMGCR bioactive fraction, PAF 07 (Figure 3). Mass ions (m/z) from 30 to 380 were ubiquitous to the three fractions (PAF 05, 06, and 07) and therefore were eliminated in screening for the potential HMGCR inhibitor. Interestingly, m/z 498 [M + H]+ was found in high mass intensity in PAF 06 and was also found in PAF 07, a fraction with HMGCR inhibition (82.6%) at 5 mg/mL concentration (Figure 3) but not present in non-active fraction, PAF 05. This finding suggests that the compound at m/z 498 [M + H]+ plausibly is responsible for the observed HMGCR inhibition in PAF 06 and PAF 07.
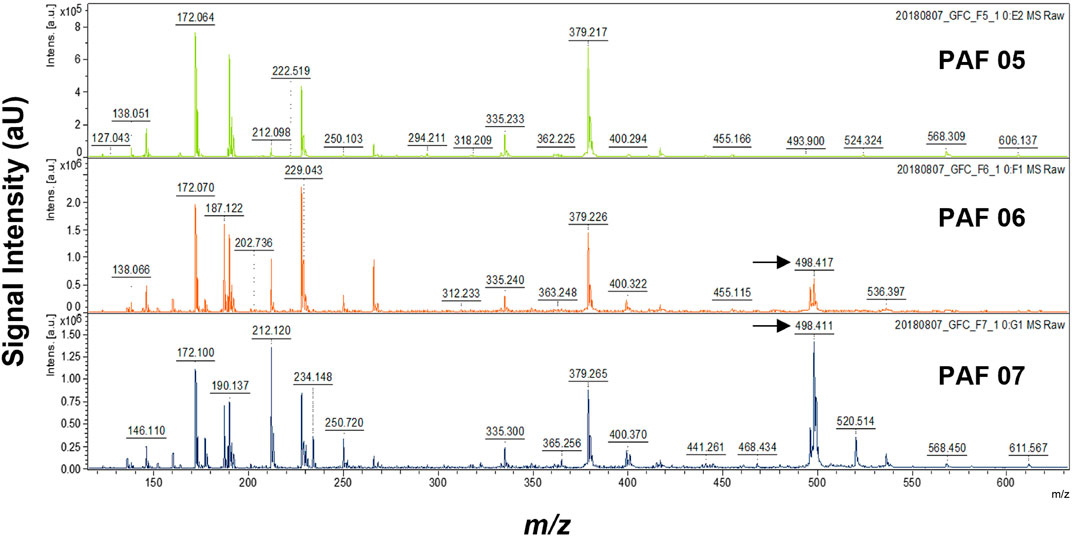
FIGURE 3. The MALDI-TOF MS spectra showing chemical profile of PAF 05 (inactive against HMGCR), PAF 06, and PAF 07 fractions (displayed inhibitory activity against HMGCR). The MALDI MS were obtained using α-cyano-4-hydroxycinnamic acid (CHCA, 10 mg in 75% MeOH) as the matrix. Each fraction has three trials of MS runs. Mass spectra (MS) showed the detection of small molecule with m/z 498 [M + H]+ (arrow) in bioactive fractions PAF 06 and 07.
We further optimized the chemical profiling of pineapple metabolites, this time using 2,5-dihydroxybenzoic acid (DHB) as matrix to narrow our investigation in targeting putative HMGCR inhibitors. In this experiment, we compared the MALDI mass spectra of PAF 03, 06, and 07, i.e., fractions with active HMGCR inhibition activity. The mass data demonstrated that m/z 498 [M + H]+ was present in PAF 06 and 07 (Figure 4). Therefore, changing of MALDI-TOF-MS matrix from α-cyano-4-hydroxycinnamic acid (CHCA) to dihydroxybenzoic acid (DHB) did not change the result in the presence of HMGCR inhibitor at m/z 498 [M + H]+. Another reason for using 2,5-dihydroxybenzoic acid (DHB) as a matrix is that several reports revealed that the DHB matrix is good at ionizing small metabolites (Snovida et al., 2008; 2006; Snovida and Perreault, 2007). Hence, we used 2,5-dihydroxybenzoic acid (DHB) as a matrix for subsequent experiments for MALDI MSI as described in Materials and Methods section.
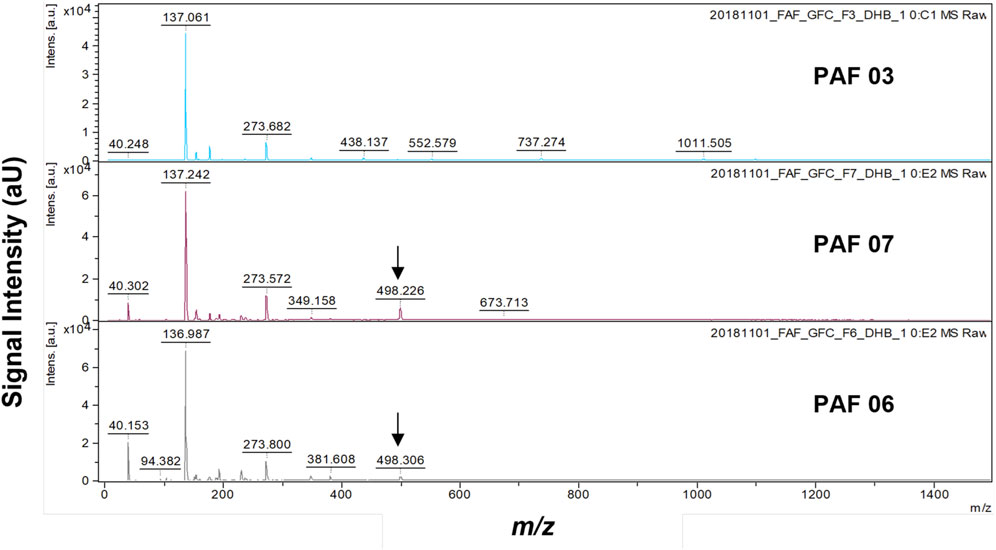
FIGURE 4. MALDI-TOF mass spectrometry chemical profile of PAF 03, 06, and 07 obtained using 2,5-dihydroxybenzoic acid (DHB) as the matrix. Each extract has three trials of MS runs. The arrow indicates the presence of m/z 498 [M + H]+.
While it is universally known that partially purified extract often contains many compounds, the mass at m/z 498 [M + H]+, present in bioactive PAF 06 and PAF 07 fractions, was not found in PAF 03 mass spectrum, indicating that different compounds may elicit the HMGCR inhibition in PAF 03 fraction. Plausibly due to larger molecules as supported by results and findings of gel filtration chromatography and the native PAGE experiments. Moreover, there were no m/z 498 [M + H]+ detected in mass spectra of non-active fractions (<80% Inhibition), i.e., PAF 01-02, 04-05, 10-11 (Supplementary Figure S7). Low mass intensity of m/z 498 [M + H]+ were detected in PAF 08-09, which is possibly contributory to the HMGCR inhibitory activities of these fractions. These findings further validate that compound with m/z 498 [M + H]+ is a potential HMGCR inhibitor in pineapple fruit extract.
3.4 Putative identification of anti-hypercholesterolemic compound in pineapple fruit
The metabolite with the mass ion of m/z 498 [M + H]+, which is the putative HMGCR inhibitor, was further analyzed via the high-resolution accurate mass and tandem mass spectrometry analysis (MS/MS) using LTQ Orbitrap XL ETD mass spectrometer (Thermo Fisher Scientific, San Jose, CA). In addition, dereplication using different online databases such as METLIN™, PubChem, and ChemSpider was also performed to assign putative identification on the target mass. In Figure 5A, the molecular ion peak at m/z 498.2599 [M + H]+ (predicted molecular formula: C27H36N3O6, calc. mass = 498.2604, and DBE = 12, mass error: 1.0 ppm) revealed that it corresponds to N1, N10-diferuloylspermidine (Saludes et al., 2022), whose identity was unambiguously confirmed by the fragment ions at m/z 177, 234, 248, 305, 322, and 481 by MS/MS analysis (Figure 5B) and reported MS/MS fragment pattern (Youhnovski et al., 1998; Steingass et al., 2015).
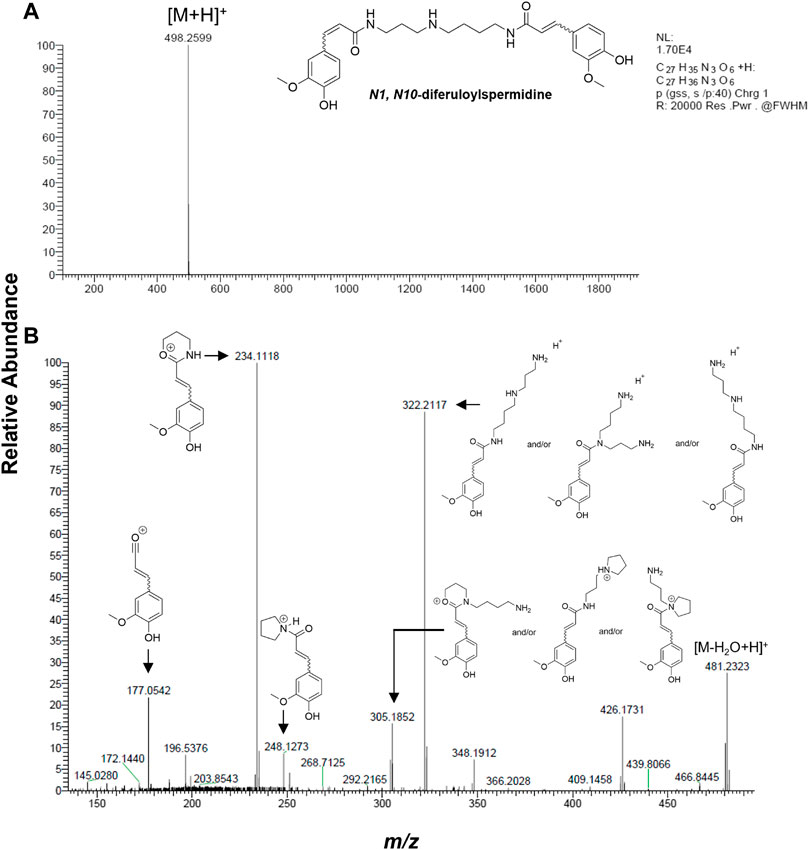
FIGURE 5. Identification of putative HMGCR inhibitor by HRMS and MS/MS. (A) Extracted ion mass spectrum and (B) MS/MS fragmentation of m/z 498.2607 [M + H]+ analyzed by LTQ Orbitrap LX ETD at 35 V collision energy and showing N1, N10-diferuloylspermidine as the molecule corresponding to the MS fragments (Youhnovski et al., 1998; Steingass et al., 2015).
3.5 In situ spatial distribution and localization of HMGCR inhibitor in pineapple fruit tissue by matrix-assisted laser desorption/ionization—time of flight—mass spectrometry imaging, MALDI-TOF-MSI
To establish the spatiotemporal distribution of N1, N10-diferuloylspermidine in pineapple fruit tissue, pineapple fruit cross sections that cover the core, flesh with ovary, and peel were first obtained (Supplementary Figure S1). This pie-shaped section provides better insight into the localization of metabolites as it covers a broader range of the fruit. Under the experimental conditions described in Material and Methods section, the mass ion peak m/z 498 [M + H]+ corresponding to N1, N10-diferuloylspermidine was readily detected and identified in the fruit tissue when using 2,5-dihydroxybenzoic acid (DHB) as a matrix. This mass ion m/z 498 [M + H]+ is abundantly distributed in the fruit tissue, concentrated mainly in the tissue near the peel/shell and gradually decreasing towards the flesh and ovary (Figure 6A; Supplementary Figure S8). Interestingly, N1, N10-diferuloylspermidine was not detected in the core region. This spatiotemporal distribution was validated by the anatomical visualization of peel/shell, ovary, and core tissues by toluidine blue O stain (Figure 6B; Supplementary Figure S8). This finding further supports that the HMGCR inhibition observed from PAF extract prepared mainly from the ripe fruit without the core, as described in Materials and Methods section, is linked to the presence of N1, N10-diferuloylspermidine.
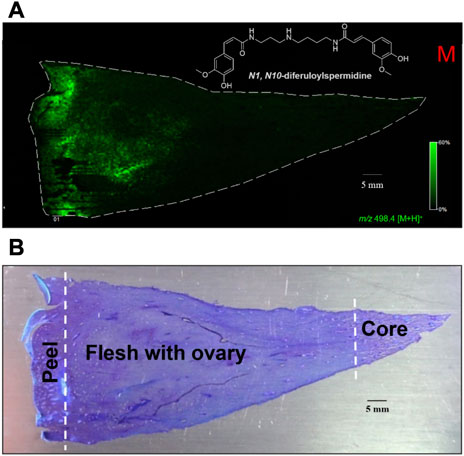
FIGURE 6. In situ spatial distribution and localization of HMGCR inhibitor, N1, N10-diferuloylspermidine in pineapple fruit tissue. (A) MALDI image showing the spatial distribution and localization of m/z 498.4 [M + H]+ (MALDI-TOF-MSI) using 2,5-dihydroxybenzoic acid (DHB) as matrix in cross section of pineapple fruit tissues (50 μm thickness) and (B) toluidine blue O (TBO)-stained pineapple fruit tissues after MALDI MSI experiment. The N1, N10-diferuloylspermidine is highly abundant in the tissue near the peel/shell and sparingly localized in the flesh and ovary.
4 Discussion
The use of plant materials as a source of biologically active substances has received much attention to the medical system, offering safety, availability, and economic benefits (Courdavault et al., 2021). In the Philippines, Ananas comosus (pineapple) has long been one of the most popular tropical fruits due to its high nutritional value and medicinal properties (Seenak et al., 2021). Several studies have reported pineapple as a rich source of biologically active compounds, providing powerful natural pharmacological activities (Dembitsky et al., 2011; Okafor et al., 2011; Bataglion et al., 2015; El-Shazly et al., 2018; Seenak et al., 2021).
In this study, the juice of pineapple fruit (PAF) was mechanically extracted and tested against HMG-CoA reductase (HMGCR) to evaluate its hypocholesterolemic activity. The analysis revealed that the crude extract of pineapple fruit possesses HMGCR inhibitory activity of 74% at 100 mg/mL. These findings led to partial purification of PAF extract by gel filtration chromatography (GFC) whereby fraction PAF 06 has consistently exhibited 100.00% HMGCR inhibition at 10 mg/mL, which is 10-fold decrease in concentration compared to the crude fruit extract, which was tested at 100 mg/mL indicating that the active HMGCR inhibitor is present in higher concentration. Aside from PAF 06, there were two GFC fractions, PAF 03 and PAF 07, that were also considered bioactive since they showed >80% HMGCR inhibitory activity.
To gain insights into the identity of metabolites in the active GFC PAF fractions, the chemical profile of the GFC fractions PAF 03, 06, and 07 was further investigated using mass spectrometry. MALDI-TOF-MS experiments revealed a distinct mass at m/z 498 [M + H]+ active fractions PAF 06 and PAF 07. High-resolution accurate mass and tandem mass spectrometry analysis (MS/MS) of m/z 498 [M + H]+, and dereplication using different natural product databases identified N1, N10-diferuloylspermidine as the putative hypocholesterolemic compound in the pineapple fruit. In this study however, the HRMS and MS/MS analyses of N1, N10-diferuloylspermidine would not be able to discern isomers with different olefin configurations (E or Z) in the feruloyl moiety unless authentic standards are available for direct comparisons. Hence, future investigation on the compound by NMR would be needed to firmly establish the structure. Moreover, an exploration of its mechanism of inhibition of HMGCR would be explored in the future, as typical inhibitors of HMGCR (like the statins) have a negative charge, whereas N1, N10-diferuloylspermidine bears a positive charge, which might lead to a different mode of inhibition.
The metabolite N1, N10-diferuloylspermidine is a conjugate compound of ferulic acid with spermidine. It belongs to phenolamides (PAs), also known as phenylamides or hydroxycinnamic acid amides. These are plant secondary metabolites that occur as conjugates of various hydroxycinnamic acids (e.g., coumaric, caffeic, or ferulic acids) with mono/polyamines (e. g., spermine, putrescine, or tyramine) (Martin-Tanguy et al., 1978; Bassard et al., 2010; Roumani et al., 2021). PAs provide significant roles in plant reproductive (flower) growth, differentiation, and development (Roumani et al., 2021). More importantly, these compounds were reported to play essential roles in plant defenses (Macoy et al., 2015). In pineapple, N1, N10-diferuloylspermidine was reported to be present in the fruit pulp and with an elevated concentration in the fruit peel/shell, which is linked to its role in defense against UV light (Steingass et al., 2015).
The potential health-promoting properties of PAs are wide and considered as health-promoting food ingredients since they occur in elevated concentrations in edible produce products such as eggplant (Solanum melongena L.), tomato (Solanum lycopersicum L.), cocoa (Theobroma cacao L.), and oat (Avena sativa L.) (Roumani et al., 2020). Their reported biological activities include hypotensive activity (Forero et al., 2016), anti-inflammatory (Kim et al., 2012), antioxidant (Kabanda, 2015), antimicrobial (Michalet et al., 2007), and anti-cancer (Rocha et al., 2012) properties together with activities protecting humans from metabolic ailments, and cardiovascular and neurodegenerative diseases (Xie et al., 2007). In the context of PAs as health promoting plant metabolite, the N1, N10-diferuloylspermidine in pineapple has potential to lower cholesterol biosynthesis via HMGCR inhibition activity.
MALDI-TOF mass spectrometry imaging was further employed to obtain a high-resolution image of pineapple plant tissue that shows the spatial distribution of its metabolites and to gain insights into the localization of the possible HMGCR inhibitor, N1, N10-diferuloylspermidine. MALDI mass spectrometry imaging was performed on the core and flesh parts of the fruit.
MALDI mass spectrometry imaging is a technique that provides visualization of the spatial distribution of multiple biomolecules, including small molecules, lipids, peptides, proteins, and endogenous compounds, in a thin tissue section without the aid of probes or antibodies while maintaining the structural integrity of the tissue (Cornett et al., 2007; Marques et al., 2014). MALDI MSI applicability has been shown to work suitably on bioactive compounds in medicinal plants and functional food (Marques et al., 2014; Dalisay et al., 2015; Wang et al., 2021; Kokesch-Himmelreich et al., 2022; Zhang et al., 2022). For example, Dalisay in Lewis lab at Washington State University previously demonstrated the use of MALDI mass spectrometry imaging on the medicinal plant, flax Linum usitatissimum, and identified unambiguously and mapped out the spatial localization of cancer/health protective lignin secoisolariciresinol diglucoside, SDG in the flax pod with seeds (Dalisay et al., 2015). It was shown that the anticancer lignan is stored in the seed coat of the flax seed. This work is the first demonstration of mapping the localization of anticancer plant metabolites, SDG and 6a-HMG SDG, in a thin tissue section of a flax capsule, without solvent extraction and purification.
We envisage the application of MALDI MSI as an analytical imaging technique to detect and determine the spatiotemporal distribution and localization in situ of N1, N10-diferuloylspermidine in pineapple fruit tissue. Although N1, N10-diferuloylspermidine was present in the pineapple fruit extracts by HPLC-MS and GC-MS (Steingass et al., 2015) there was no report on the spatial mapping of N1, N10-diferuloylspermidine in situ.
These MALDI mass spectrometry imaging findings are in agreement with previous reports that N1, N10-diferuloylspermidine contents in pineapple fruit tissues are elevated in peel extracts and found in lesser concentrations in pulp (flesh) extract (Steingass et al., 2015) which is linked for its role as fruit’s UV protectant. However, it is interesting to note in our MALDI MSI experiments that the UV protectant metabolite is also spatially located and distributed in the flesh region and ovary. Since the transition of the tissue in pineapple peel and flesh is not clearly defined as these are composed predominantly of parenchymal cells with unlignified cell walls (Smith and Harris, 1995), there is the plausible translocation of N1, N10-diferuloylspermidine towards the latter region and may imply a protective effect on the ovary for defense against biotic stress such as plant-pathogen interactions. This hypothesis is supported by reports that phenolamides are a class of plant metabolites associated with plant defenses against plant pathologies and immune responses (Roumani et al., 2021; Liu et al., 2022). To our knowledge, the MALDI MSI provided the first evidence of in situ spatial localization of N1, N10-diferuloylspermidine, a potential HMGCR inhibitor in pineapple while maintaining the cellular and structural features of the fruit tissues.
5 Conclusion
This study demonstrated the potential health promoting metabolite with HMGCR inhibitory activity from pineapple. Using an analytical workflow combined with an HMGCR inhibition assay, we deduced a metabolite present in the semi-purified pineapple fruit extract demonstrating in vitro inhibition of HMGCR. Unlike the enzyme bromelain, which is mainly associated with the known health benefits of pineapple, the N1, N10-diferuloylspermidine is a hydroxycinnamic acid amide and a small plant secondary metabolite eliciting HMGCR inhibitory activity. This result suggests that small secondary metabolites (such as flavonoids, hydroxycinnamic acid depsides, hydroxycinnamoyl glycosides, and others) present in pineapple fruit may possess potential bioactivities and health benefits. Future investigation and purification of these small secondary metabolites including the N1, N10-diferuloylspermidine currently underway will next establish their health benefits for cardiovascular diseases.
Moreover, our MALDI MSI experiments aided the in situ spatiotemporal localization and distribution of N1, N10-diferuloylspermidine in a complex biological space, thereby allowing us to investigate and understand the dynamic distribution/accumulation of secondary metabolites in fruit tissues. Our findings further emphasized continuing our efforts to identify and determine the spatial localization and distribution of hundreds of metabolites ranging from m/z 200–800 Da detected in this study by the MALDI mass spectrometry imaging experiment. This future work will provide insights into the spatiotemporal distribution and localization of secondary metabolites in an economically important functional food such as pineapple.
Data availability statement
The original contributions presented in the study are included in the article/Supplementary Material, further inquiries can be directed to the corresponding authors.
Author contributions
DD and JS conceived the study. AS, AJ, Y-LY, DD, and JS designed the experiments. AS and AJ performed the experiments. AS, AJ, DS, JO, MJ, DB, Y-LY, JS, and DD for data analysis and curation. AS, AJ, DS, JO, MJ, DB, Y-LY, JS, and DD writing—original draft presentation and writing—review and editing. DS, JO, MJ, Y-LY, JS, and DD for supervision, administration, and funding acquisition. All authors have read and agreed to the published version of the manuscript.
Funding
This project is funded by the Department of Science and Technology (DOST) through the Science for Change Program (S4CP)—Collaborative Research and Development to Leverage Philippine Economy (CRADLE) and monitored by DOST—Philippine Council for Health Research and Development (PCHRD) with Project No. 7788. The instruments used in this study were acquired through University of San Agustin’s Tuklas Lunas® Center grant by the Philippine Council for Health Research and Development (DOST-PCHRD). The mass spectrometry data were collected in Agriculture Biotechnology Research Center, Academia Sinica, Taiwan and supported by the National Science and Technology Council (NSTC), Taiwan (Grant number: MOST 104-2320-B-001-019-MY2).
Acknowledgments
DD and JS wish to acknowledge the Balik Scientist Program of the Philippines Department of Science and Technology (DOST), through the Philippine Council for Health Research and Development (PCHRD), for the opportunity to serve the Filipino community through science, technology, and innovation. The Balik (Filipino word for returning) Scientist Program (BSP) seeks highly trained Filipino scientists, technologists, experts, and professionals residing abroad to return to the Philippines and transfer their expertise to the local community for the acceleration of the scientific, agro-industrial, and economic development of the country. Special thanks to Miss Suzanne Yñion for the administrative support.
Conflict of interest
Authors DS, JO, and MJ were employed by the company Del Monte Philippines Inc.
The remaining authors declare that the research was conducted in the absence of any commercial or financial relationships that could be construed as a potential conflict of interest.
Publisher’s note
All claims expressed in this article are solely those of the authors and do not necessarily represent those of their affiliated organizations, or those of the publisher, the editors and the reviewers. Any product that may be evaluated in this article, or claim that may be made by its manufacturer, is not guaranteed or endorsed by the publisher.
Supplementary material
The Supplementary Material for this article can be found online at: https://www.frontiersin.org/articles/10.3389/fntpr.2023.1160541/full#supplementary-material
References
Abbas, S., Shanbhag, T., and Kothare, A. (2021). Applications of bromelain from pineapple waste towards acne. Saudi J. Biol. Sci. 28 (1), 1001–1009. doi:10.1016/j.sjbs.2020.11.032
Balida, L. A. P., Regalado, J. T. A., Teodosio, J. J. R., Dizon, K. A. H., Sun, Z., Zhan, Z. Q., et al. (2022). Antibiotic isoflavonoids, anthraquinones, and pterocarpanoids from pigeon pea (Cajanus cajan L.) seeds against multidrug-resistant Staphylococcus aureus. Metabolites 12 (4), 279. doi:10.3390/metabo12040279
Baskaran, G., Salvamani, S., Azlan, A., Ahmad, S. A., Yeap, S. K., and Shukor, M. Y. (2015). Hypocholesterolemic and antiatherosclerotic potential of Basella alba leaf extract in hypercholesterolemia-induced rabbits. Evid. Based Complement. Altern. Med. 2015, 1–7. doi:10.1155/2015/751714
Bassard, J. E., Ullmann, P., Bernier, F., and Werck-Reichhart, D. (2010). Phenolamides: Bridging polyamines to the phenolic metabolism. Phytochemistry 71 (16), 1808–1824. doi:10.1016/j.phytochem.2010.08.003
Bataglion, G. A., da Silva, F. M. A., Eberlin, M. N., and Koolen, H. H. F. (2015). Determination of the phenolic composition from Brazilian tropical fruits by UHPLC-MS/MS. Food Chem. 180, 280–287. doi:10.1016/j.foodchem.2015.02.059
Bochar, D. A., Brown, J. R., Doolittle, W. F., Klenk, H. P., Lam, W., Schenk, M. E., et al. (1997). 3-hydroxy-3-methylglutaryl coenzyme A reductase of sulfolobus solfataricus: DNA sequence, phylogeny, expression in Escherichia coli of the hmgA gene, and purification and kinetic characterization of the gene product. J. Bacteriol. 179 (11), 3632–3638. doi:10.1128/jb.179.11.3632-3638.1997
Brien, S., Lewith, G., Walker, A., Hicks, S. M., and Middleton, D. (2004). Bromelain as a treatment for osteoarthritis: A review of clinical studies. Evid. Based Complement. Altern. Med. 1 (3), 251–257. doi:10.1093/ecam/neh035
Chobotova, K., Vernallis, A. B., and Majid, F. A. A. (2010). Bromelain’s activity and potential as an anti-cancer agent: Current evidence and perspectives. Cancer Lett. 290 (2), 148–156. doi:10.1016/j.canlet.2009.08.001
Cornett, D. S., Reyzer, M. L., Chaurand, P., and Caprioli, R. M. (2007). MALDI imaging mass spectrometry: Molecular snapshots of biochemical systems. Nat. Methods 4 (10), 828–833. doi:10.1038/nmeth1094
Courdavault, V., O’Connor, S. E., Jensen, M. K., and Papon, N. (2021). Metabolic engineering for plant natural products biosynthesis: New procedures, concrete achievements and remaining limits. Nat. Prod. Rep. 38 (12), 2145–2153. doi:10.1039/d0np00092b
Dalisay, D. S., Kim, K. W., Lee, C., Yang, H., Rübel, O., Bowen, B. P., et al. (2015). Dirigent protein-mediated lignan and cyanogenic glucoside formation in flax seed: Integrated omics and MALDI mass spectrometry imaging. J. Nat. Prod. 78 (6), 1231–1242. doi:10.1021/acs.jnatprod.5b00023
Dembitsky, V. M., Poovarodom, S., Leontowicz, H., Leontowicz, M., Vearasilp, S., Trakhtenberg, S., et al. (2011). The multiple nutrition properties of some exotic fruits: Biological activity and active metabolites. Food Res. Int. 44 (7), 1671–1701. doi:10.1016/j.foodres.2011.03.003
Dong, Y., Li, B., Malitsky, S., Rogachev, I., Aharoni, A., Kaftan, F., et al. (2016). Sample preparation for mass spectrometry imaging of plant tissues: a review. Front. Plant Sci. 7, 60. doi:10.3389/fpls.2016.00060
Duangjai, A., Ingkaninan, K., and Limpeanchob, N. (2011). Potential mechanisms of hypocholesterolaemic effect of Thai spices/dietary extracts. Nat. Prod. Res. 25 (4), 341–352. doi:10.1080/14786411003754249
El-Shazly, S. A., Ahmed, M. M., Al-Harbi, M. S., Alkafafy, M. E., El-Sawy, H. B., and Amer, S. A. M. (2018). Physiological and molecular study on the anti-obesity effects of pineapple (Ananas comosus) juice in male Wistar rat. Food Sci. Biotechnol. 27 (5), 1429–1438. doi:10.1007/s10068-018-0378-1
Fang, J., Miao, C., Chen, R., and Ming, R. (2016). Genome-wide comparative analysis of microsatellites in pineapple. Trop. Plant Biol. 9 (3), 117–135. doi:10.1007/s12042-016-9163-6
Forero, D. P., Masatani, C., Fujimoto, Y., Coy-Barrera, E., Peterson, D. G., and Osorio, C. (2016). Spermidine derivatives in lulo (solanum quitoense lam.) fruit: Sensory (taste) versus biofunctional (ace-inhibition) properties. J. Agric. Food Chem. 64 (26), 5375–5383. doi:10.1021/acs.jafc.6b01631
Gemperline, E., Jayaraman, D., Maeda, J., Ané, J. M., and Li, L. (2015). Multifaceted investigation of metabolites during nitrogen fixation in medicago via high resolution MALDI-MS imaging and ESI-MS. J. Am. Soc. Mass Spectrom. 26 (1), 149–158. doi:10.1007/s13361-014-1010-0
Hossain, F. M., Akhtar, S., and Anwar, M. (2015). Nutritional value and medicinal benefits of pineapple. Int. J. Food Sci. Nutr. 4 (1), 84–88. doi:10.11648/j.ijnfs.20150401.22
Jančič, U., and Gorgieva, S. (2022). Bromelain and nisin: The natural antimicrobials with high potential in biomedicine. Pharmaceutics 14 (1), 76. doi:10.3390/pharmaceutics14010076
Kabanda, M. M. (2015). A theoretical study of the antioxidant properties of phenolic acid amides investigated through the radical-scavenging and metal chelation mechanisms. Eur. Food Res. Technol. 241 (4), 553–572. doi:10.1007/s00217-015-2484-0
Kim, E. O., Min, K. J., Kwon, T. K., Um, B. H., Moreau, R. A., and Choi, S. W. (2012). Anti-inflammatory activity of hydroxycinnamic acid derivatives isolated from corn bran in lipopolysaccharide-stimulated Raw 264.7 macrophages. Food Chem. Toxicol. 50 (5), 1309–1316. doi:10.1016/j.fct.2012.02.011
Kokesch-Himmelreich, J., Wittek, O., Race, A. M., Rakete, S., Schlicht, C., Busch, U., et al. (2022). MALDI mass spectrometry imaging: From constituents in fresh food to ingredients, contaminants and additives in processed food. Food Chem. 385, 132529. doi:10.1016/j.foodchem.2022.132529
Koletzko, B. (2022). 3.20 hypercholesterolemia. World Rev. Nutr. Diet. 124, 362–367. doi:10.1159/000517394
Liu, S., Jiang, J., Ma, Z., Xiao, M., Yang, L., Tian, B., et al. (2022). The role of hydroxycinnamic acid amide pathway in plant immunity. Front. Plant Sci. 13, 922119. doi:10.3389/fpls.2022.922119
Macoy, D. M., Kim, W. Y., Lee, S. Y., and Kim, M. G. (2015). Biotic stress related functions of hydroxycinnamic acid amide in plants. J. Plant Biol. 58 (3), 156–163. doi:10.1007/s12374-015-0104-y
Marques, J. V., Dalisay, D. S., Yang, H., Lee, C., Davin, L. B., and Lewis, N. G. (2014). A multi-omics strategy resolves the elusive nature of alkaloids in Podophyllum species. Mol. Biosyst. 10 (11), 2838–2849. doi:10.1039/C4MB00403E
Martin-Tanguy, J., Cabanne, F., Perdrizet, E., and Martin, C. (1978). The distribution of hydroxycinnamic acid amides in flowering plants. Phytochemistry 17 (11), 1927–1928. doi:10.1016/s0031-9422(00)88735-x
Maurer, H. R. (2001). Bromelain: Biochemistry, pharmacology and medical use. Cell Mol. Life Sci. 58 (9), 1234–1245. doi:10.1007/PL00000936
Metzig, C., Grabowska, E., Eckert, K., Rehse, K., and Maurer, H. R. (1999). Bromelain proteases reduce human platelet aggregation in vitro, adhesion to bovine endothelial cells and thrombus formation in rat vessels in vivo. Vivo 13 (1), 7–12.
Michalet, S., Cartier, G., David, B., Mariotte, A. M., Dijoux-franca, M. G., Kaatz, G. W., et al. (2007). N-Caffeoylphenalkylamide derivatives as bacterial efflux pump inhibitors. Bioorg Med. Chem. Lett. 17 (6), 1755–1758. doi:10.1016/j.bmcl.2006.12.059
Mohd Ali, M., Hashim, N., Abd Aziz, S., and Lasekan, O. (2020). Pineapple (Ananas comosus): A comprehensive review of nutritional values, volatile compounds, health benefits, and potential food products. Food Res. Int. 137, 109675. doi:10.1016/j.foodres.2020.109675
Okafor, O. Y., Erukainure, O. L., Ajiboye, J. A., Adejobi, R. O., Owolabi, F. O., and Kosoko, S. B. (2011). Modulatory effect of pineapple peel extract on lipid peroxidation, catalase activity and hepatic biomarker levels in blood plasma of alcohol-induced oxidative stressed rats. Asian Pac J. Trop. Biomed. 1 (1), 12–14. doi:10.1016/s2221-1691(11)60060-9
Pavan, R., Jain, S., Shraddha, S., and Kumar, A. (2012). Properties and therapeutic application of bromelain: A review. Biotechnol. Res. Int. 2012, 1–6. doi:10.1155/2012/976203
Peukert, M., Matros, A., Lattanzio, G., Kaspar, S., Abadía, J., and Mock, H. P. (2012). Spatially resolved analysis of small molecules by matrix-assisted laser desorption/ionization mass spectrometric imaging (MALDI-MSI). New Phytol. 193 (3), 806–815. doi:10.1111/j.1469-8137.2011.03970.x
Ramkumar, S., Raghunath, A., and Raghunath, S. (2016). Statin therapy: Review of safety and potential side effects. Acta Cardiol. Sin. 32 (6), 631–639. doi:10.6515/acs20160611a
Ramli, A. N. M., Aznan, T. N. T., and Illias, R. M. (2017). Bromelain: From production to commercialisation. J. Sci. Food Agric. 97 (5), 1386–1395. doi:10.1002/jsfa.8122
Rocha, L. D., Monteiro, M. C., and Teodoro, A. J. (2012). Anticancer properties of hydroxycinnamic acids - a review. Cancer Clin. Oncol. 1 (2), 109. doi:10.5539/cco.v1n2p109
Rodwell, V. W., Beach, M. J., Bischoff, K. M., Bochar, D. A., Darnay, B. G., Friesen, J. A., et al. (2000). 3-Hydroxy-3-methylglutaryl-CoA reductase. Methods Enzymol. 324, 259–280. doi:10.1016/s0076-6879(00)24237-7
Roumani, M., Besseau, S., Gagneul, D., Robin, C., and Larbat, R. (2021). Phenolamides in plants: An update on their function, regulation, and origin of their biosynthetic enzymes. J. Exp. Bot. 72 (7), 2334–2355. doi:10.1093/jxb/eraa582
Roumani, M., Duval, R. E., Ropars, A., Risler, A., Robin, C., and Larbat, R. (2020). Phenolamides: Plant specialized metabolites with a wide range of promising pharmacological and health-promoting interests. Biomed. Pharmacother. 131, 110762. doi:10.1016/j.biopha.2020.110762
Saludes, J. P., Dalisay, D. S., Suarez, A. F. L., Juanillo, A. A. B., Sison, D. C. D., Orajay, J. I., et al. (2022). Compositions for treating hypercholesterolemia and preparation methods thereof. Geneva, Switzerland: World Intellectual Property Organization. Application No. WO2022124917. Available at: https://patentscope2.wipo.int/search/en/detail.jsf;jsessionid=20032591A90D4FEF5725BD2112D81ACD?docId=WO2022124917&_cid=JP1-L4KZFU-77680-1.
Salvamani, S., Gunasekaran, B., Shukor, M. Y., Shaharuddin, N. A., Sabullah, M. K., and Ahmad, S. A. (2016). Anti-HMG-CoA reductase, antioxidant, and anti-inflammatory activities of Amaranthus viridis leaf extract as a potential treatment for hypercholesterolemia. Evid. Based Complement. Altern. Med. 2016, 1–10. doi:10.1155/2016/8090841
Seenak, P., Kumphune, S., Malakul, W., Chotima, R., and Nernpermpisooth, N. (2021). Pineapple consumption reduced cardiac oxidative stress and inflammation in high cholesterol diet-fed rats. Nutr. Metab. (Lond) 18 (1), 36–10. doi:10.1186/s12986-021-00566-z
Smith, B. C., and Harris, P. J. (1995). Polysaccharide composition of unlignified cell walls of pineapple [Ananas comosus (L.) Merr.] fruit. Plant Physiol. 107 (4), 1399–1409. doi:10.1104/pp.107.4.1399
Snovida, S. I., Chen, V. C., and Perreault, H. (2006). Use of a 2,5-dihydroxybenzoic acid/aniline MALDI matrix for improved detection and on-target derivatization of glycans: A preliminary report. Anal. Chem. 78 (24), 8561–8568. doi:10.1021/ac061375r
Snovida, S. I., and Perreault, H. (2007). A 2,5-dihydroxybenzoic acid/N,N-dimethylaniline matrix for the analysis of oligosaccharides by matrix-assisted laser desorption/ionization mass spectrometry. Rapid Commun. Mass Spectrom. 21 (22), 3711–3715. doi:10.1002/rcm.3265
Snovida, S. I., Rak-Banville, J. M., and Perreault, H. (2008). On the use of DHB/aniline and DHB/N,N-dimethylaniline matrices for improved detection of carbohydrates: Automated identification of oligosaccharides and quantitative analysis of sialylated glycans by MALDI-TOF mass spectrometry. J. Am. Soc. Mass Spectrom. 19 (8), 1138–1146. doi:10.1016/j.jasms.2008.04.033
Steingass, C. B., Glock, M. P., Schweiggert, R. M., and Carle, R. (2015). Studies into the phenolic patterns of different tissues of pineapple (Ananas comosus [L.] Merr.) infructescence by HPLC-DAD-ESI-MS (n) and GC-MS analysis. Anal. Bioanal. Chem. 407 (21), 6463–6479. doi:10.1007/s00216-015-8811-2
Stone, N. J. (1996). Lipid management: Current diet and drug treatment options. Am. J. Med. 101 (4), 40–49. doi:10.1016/s0002-9343(96)00319-1
Suarez, A. F. L., Tirador, A. D. G., Villorente, Z. M., Bagarinao, C. F., Sollesta, J. V. N., Dumancas, G. G., et al. (2021). The isorhamnetin-containing fraction of Philippine honey produced by the stingless bee tetragonula biroi is an antibiotic against multidrug-resistant Staphylococcus aureus. Molecules 26 (6), 1688. doi:10.3390/molecules26061688
Wang, J., Yang, E., Chaurand, P., and Raghavan, V. (2021). Visualizing the distribution of strawberry plant metabolites at different maturity stages by MALDI-TOF imaging mass spectrometry. Food Chem. 345, 128838. doi:10.1016/j.foodchem.2020.128838
Wei, C., Liu, S. H., Liu, Y. G., Lv, L. L., Yang, W. X., and Sun, G. M. (2011). Characteristic aroma compounds from different pineapple parts. Molecules 16 (6), 5104–5112. doi:10.3390/molecules16065104
Xie, W., Wang, W., Su, H., Xing, D., Cai, G., and Du, L. (2007). Hypolipidemic mechanisms of Ananas comosus L. Leaves in mice: Different from fibrates but similar to statins. J. Pharmacol. Sci. 103 (3), 267–274. doi:10.1254/jphs.fp0061244
Yabor, L., Gómez, D., Pérez-Bonachea, L., Martínez, J., Escalante, D., Garro, G., et al. (2021). Pineapple fruits from transgenic plants have limited differences on mesocarp biochemical component contents. Acta Physiol. Plant 43, 6–9. doi:10.1007/s11738-020-03181-2
Youhnovski, N., Bigler, L., Werner, C., and Hesse, M. (1998). On-Line coupling of high-performance liquid chromatography to atmospheric pressure chemical ionization mass spectrometry (HPLC/APCI-MS and MS/MS). The pollen analysis of Hippeastrum x hortorum (Amaryllidaceae). Helvetica Chim. Acta 81 (9), 1654–1671. doi:10.1002/(sici)1522-2675(19980909)81:9<1654::AID-HLCA1654>3.0.CO;2-T
Keywords: hypercholesterolemia, HMGCR inhibitor, pineapple, functional food, MALDI-TOF mass spectrometry imaging, N1, N10-diferuloylspermidine
Citation: Suarez AFL, Juanillo AAB, Sison DCD, Orajay JI, Javier MBB, Baladjay DMS, Yang Y-L, Saludes JP and Dalisay DS (2023) In situ spatiotemporal mapping of 3-hydroxy-3-methylglutaryl-CoA reductase (HMGCR) inhibitor in pineapple (Ananas comosus) fruit tissue by MALDI mass spectrometry imaging. Front. Nat. Prod. 2:1160541. doi: 10.3389/fntpr.2023.1160541
Received: 07 February 2023; Accepted: 31 March 2023;
Published: 24 April 2023.
Edited by:
Eng Shi Ong, Singapore University of Technology and Design, SingaporeReviewed by:
Xuezhi Bi, Bioprocessing Technology Institute (A∗STAR), SingaporeDaniel Pecoraro Demarque, Universidade de São Paulo, Brazil
Copyright © 2023 Suarez, Juanillo, Sison, Orajay, Javier, Baladjay, Yang, Saludes and Dalisay. This is an open-access article distributed under the terms of the Creative Commons Attribution License (CC BY). The use, distribution or reproduction in other forums is permitted, provided the original author(s) and the copyright owner(s) are credited and that the original publication in this journal is cited, in accordance with accepted academic practice. No use, distribution or reproduction is permitted which does not comply with these terms.
*Correspondence: Jonel P. Saludes, jsaludes@usa.edu.ph; Doralyn S. Dalisay, ddalisay@usa.edu.ph