Gut Microbiota and Type 1 Diabetes Mellitus: The Effect of Mediterranean Diet
- 1PoliTO BioMed Lab, Politecnico di Torino, Torino, Italy
- 2Dipartimento di Medicina Interna, Ospedale Madre Giuseppina Vannini, Rome, Italy
- 3Dipartimento di Scienze e Innovazione Tecnologica, Università del Piemonte Orientale, Alessandria, Italy
Type 1 diabetes mellitus (T1DM) is a chronic autoimmune disease resulting from a complex interplay between genetic susceptibility and environmental factors. Regarding the latter, gut microbiota has a pivotal role in the pathogenesis of T1DM, by affecting intestinal permeability, molecular mimicry, and modulating innate and adaptive immune system, as described in several previous studies. The composition of the gut microbiota is largely influenced by diet. Some observational studies have shown that a low fiber intake is associated with the development of many inflammatory and immune-mediated diseases. In this context, the Mediterranean diet (MD), which is based on high consumption of cereals (preferably as whole grains), legumes, nuts, vegetables, fruits, olive oil, and fish, could play a protective role. Many of the characteristic components of MD have functional characteristics with positive effects on health and well-being. Eating habits are the main significant determinants of the microbial multiplicity of the intestine and the food components influence both microbial populations and their metabolic activities from the early stages of life. Moreover, food metabolites influence the immune response. The intestine is considered the primary site where food metabolites mediate their effects, through epithelial integrity or mucosal immunity. The compromised epithelial integrity allows the translocation of bacteria and/or the diffusion of their products, such as food antigens and lipopolysaccharides, from the intestinal lumen to the tissues, which could enhance the stimulation of immune cells, contributing to the pathogenesis of autoimmune diseases, such as T1DM. The intake of a high amount of fiber and therefore of prebiotics with MD allows the microbiota to have a good microbial balance. Moreover, as more dietary fibers are ingested, a higher amount of short-chain fatty acids (SCFAs) is produced by anaerobic gut microbiota, promoting gut homeostasis, to which also contribute tryptophan metabolites and omega-3-fatty acids. Furthermore, the higher intake of polyunsaturated fatty acids and omega-3-fatty-acids contribute to a better metabolic control. In this review we report the relationship between gut microbiota and T1DM and we explore the effects of Mediterranean diet on microbiota as a potential therapeutic strategy, aimed at preventing or delaying progression of T1DM and its complications.
Introduction
Type 1 Diabetes Mellitus (T1DM) accounts for about 5–10% of all cases of diabetes (1) and is a chronic autoimmune disease resulting from a complex interplay between genetic susceptibility and environmental factors (2–4).
Up to 50% of genetic risk for T1DM is due to the presence of susceptibility loci within the human leukocyte antigen (HLA) regions (5–7), with a strong linkage between T1DM and genetic variations of the HLA region on the short arm of chromosome 6 (6p21) (4–6, 8, 9).
Non-HLA genes are also associated with T1DM, particularly the insulin gene region on the short arm of chromosome 11 (11p15) (4, 6) and other 58 genomic regions discovered with the genome-wide association studies (5, 6, 10). Most of these loci exert their effects acting at multiple levels in the immune system (8) and leading to an aberrant immune responsiveness (11).
Although genetic susceptibility contributes to the pathogenesis of T1DM, several non-genetic factors, such as environmental ones, are involved in the development of the disease (2).
Viral infections seem to have a pivotal role in the pathogenesis of T1DM (2, 12, 13), as reported in a systematic review and meta-analysis of the literature which showed an association between enterovirus infection and T1DM (14), and Coxsackievirus B1 was recently identified as a diabetogenic virus able to induce β-cell autoimmunity (15).
Other candidate environmental triggers include dietary factors, toxins and gut microbiota (12, 13, 16).
Many nutritional factors could contribute to the pathogenesis of T1DM (17, 18), such as cow's milk, animal protein, and fruit and berry juices (19, 20), while polyunsaturated omega-3 fatty acids have a protective role by modulating immune response (21, 22).
It has been recently supposed that gut microbiota, whose composition is largely influenced by diet (23), also plays an important role in the pathogenesis of T1DM, by affecting intestinal permeability, molecular mimicry, and modulating innate and adaptive immune system (24).
In this review we report the relationship between gut microbiota and T1DM, we explore the effects of diet, particularly of Mediterranean diet (MD), on microbiota, and we analyse its potential role as a therapeutic strategy, aimed at preventing or delaying progression of T1DM and its complications.
Gut Microbiota
Human gut microbiota mainly consists of about 1014 commensals bacteria (25) belonging to seven different divisions with a high predominance of Bacterioidetes (particularly Bacterioides and Prevotella) and Firmicutes, which constitute more of 90% of the total population (26, 27), but it also includes archaea, viruses, parasites, and fungi (28). According to recent studies, gut microbiota can be separated into three robust clusters, designated as “enterotypes,” identifiable by variation in the levels of one of three main genera: Bacteriodetes (enterotype 1), Prevotella (enterotype 2), and Ruminococcus (enterotype 3) (27).
Gut microbiota has several roles in human health, acting as a mucosal barrier against pathogens, contributing to improve the strengthening of microvilli, providing nutrients such as the degradation of non-digestible polysaccharides and the synthesis of vitamins, maintaining metabolic homeostasis and modulating systemic immune response (25, 28–32).
Gut microbiota composition is largely influenced by diet (23). Nutrients for the gut commensals bacteria derive from the fermentation of non-digestible dietary carbohydrates (30), leading to the production of gases, smaller amounts of organic acids and short chain fatty acids (SCFAs) (26, 30, 33), such as acetate, propionate and butyrate which perform a pivotal role in human health (33). Particularly, butyrate exerts its effects on the intestinal mucosa inducing mucin synthesis and promoting tight junctions formation (34), contributing in the maintenance of physiological gut permeability. Moreover, SCFAs, the main metabolic products of fermentation, are involved in the modulation of the immune system (35, 36) by regulating the production of pro-inflammatory cytokines and chemokines (35, 37) and T-cell function (36).
Gut Microbiota and T1DM
Several previous studies conducted in non-obese diabetic mice suggested a role of gut microbiota in the pathogenesis of T1DM (24, 38). Subsequent studies carried out on the human population also showed a strong association between gut dysbiosis and the pathogenesis in T1DM (39), suggesting that intestinal microbiota contributes to the development and progression of the disease (40). Gut dysbiosis, in fact, could contribute to the alteration of the balance between pro-inflammatory vs. tolerant signals, thus promoting the development of pancreatic autoimmunity (41).
As described by Murri et al. T1DM is associated with changes in the composition of gut microbiota (42). Particularly, it has been showed a significant difference in the amount of the two most abundant phyla of human gut microbiota, the Bacteriodes and the Firmicutes (43), with a large amount of Bacteriodes in T1DM (43, 44) which leads to an increase in Bacteriodes-to-Firmicutes ratio (45).
Although the alterations of gut microbiota contribute to the pathogenesis of T1DM, the exact mechanisms involved in this process are still unknown (46, 47). To date, it has been hypothesized that gut microbiota is involved in the regulation of intestinal mucosa permeability (48).
The large amount of Bacterioides contributes to the production of SCFAs other than butyrate (49), leading to a higher gut permeability (50) and thus contributing to the development of anti-islet cell autoantibodies (34, 51). As reported in previous studies, the increase in intestinal permeability observed in T1DM leads to microbial translocation into the circulatory system (39, 41), by inducing both a direct damage and an inflammatory immune-mediated damage of the pancreatic β-cells (52, 53).
To sustain the hypothesis of the interaction between gut dysbiosis, intestinal permeability, and host immune system, a recent study of fecal meta-proteomic showed that T1DM patients have increased intestinal inflammation, as expressed by higher levels of inflammatory proteins (galectin-3 and fibrillin-1), and altered gut permeability, as a consequence of a higher mucin degradation and lower butyrate production (54).
Nevertheless, it has been recently proposed a direct interaction between gut microbiota and pancreatic β-cells as suggested by the observation that the exocrine pancreas secretes into the intestinal tract both digestive enzymes and antimicrobial peptides (AMPs) (55), such as cathelicidins and defensins, which play a central role not only in protecting against infections but also in regulating gut microbiota (55–57) and in modulating the innate and adaptive immune system (58). Furthermore, as previously described, cathelicidin exerts trophic effect on pancreatic β-cells (57) and regulates its functions, attenuating inflammatory responses and β-cell death in diabetic subjects (59). As shown by Sun et al. gut microbiota contributes to the production of AMPs via SCFAs (60), thus gut dysbiosis may induce a lower levels of AMPc, particularly of cathelicidin and beta-defensin-1 (61), leading to the development of autoimmune diabetes (57, 60).
However, further studies are needed to better investigate the exact mechanism by which gut microbiota interacts with pancreatic β-cells and immune system.
Mediterranean Diet
The Mediterranean Diet (MD) was first described by Prof. Ancel Keys in the 1960s (62) following the results of the epidemiological study “Seven Countries' Study,” which showed a lower incidence of cardiovascular and oncological diseases in the populations of the Mediterranean area (63, 64).
MD can be considered not only a simple diet, but also a sustainable diet, because of its low environmental impact, its attention to food and nutrition safety, and to a healthy lifestyle (65). Concerning the benefits of the MD, several studies confirmed a protective role of the MD against the development of different chronic degenerative diseases, contributing to a lower mortality risk (66, 67).
MD is characterized by a high intake of cereals, legumes, vegetables, fruit, nuts, fish and an elevated consumption of olive oil, a moderate alcohol intake, and a low consumption of red meats and saturated fats (68). As reported in previous studies, in fact, the MD consists of a diet rich carbohydrates (55–60% of total energy, of which <15% are sugars), proteins (15% of total energy, with a prevalence of vegetables origin) and fibers (25 g/day), and low in lipids (30% of total energy, of which about 19% derive from monounsaturated fat, 5% from polyunsaturated fat, and <7% from saturated fat) (69–71). Relative to lipids, the main source of dietary fat in MD is represented by extra virgin olive oil (EVOO) (71, 72), whose consumption is associated with a reduction in the development of cardiovascular diseases (72–74) and, as recently shown, with the modulation of gut microbiota (74–76), contributing to a relative abundance of Firmicutes compared with Bacterioidetes (77). EVOO is predominantly composed by monounsaturated fatty acids (MUFAs), polyunsaturated fatty acids (PUFAs), such as linoleic and α-linolenic acids, and saturated fatty acids (SFA), mainly palmitic and stearic acids, and it also contains vitamin E and polyphenols, such as hydroxytyrosol (78). The beneficial effects of EVOO can also be associated with the presence of 3,3-dimethyl-1-butanol (DMB), a structural analog of choline, involved in the inhibition of trimethylamine N-oxide (TMAO) formation (79), a metabolite of phosphatidylcholine and L-carnitine, which production is mediated by gut microbiota (80) (Figure 1). As reported in several studies, higher levels of TMAO contribute to an increased cardiovascular risk (81, 82), and in type 1 diabetic subjects are associated with mortality, coronary events, stroke, heart failure and poor renal function (83).
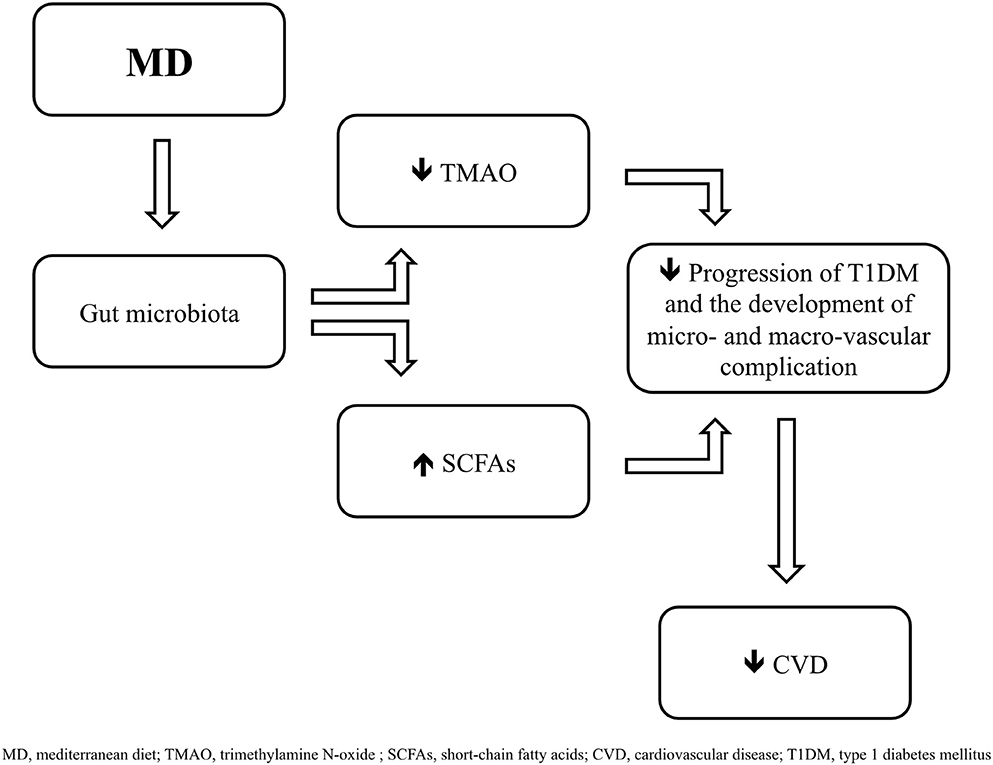
Figure 1. Mediterranean diet (MD) induces changes in gut microbiota by promoting the growing of taxa which contribute to a greater formation of short-chai fatty acids (SCFAs) and to a lower production of trimethylamine N-oxide (TMAO), leading to a delay in the progression of type 1 diabetes mellitus (T1DM) and in the development of cardiovascular complications.
MD is based on a large daily consumption of fiber (71), a very present element among plant foods, with different chemical structures, and resistant to human enzymatic degradation (84). Dietary fiber is distinguished in two main classes: polysaccharides, including cellulose and non-cellulose substances, the latter mainly represented by hemicellulose and pectin, and lignin (84). Moreover, fiber can be divided into soluble fiber, such as pectin, and insoluble ones (85).
Dietary fiber resists the action of the upper gastrointestinal human enzymes and reaches the large intestine where the local microbiota induces its fermentation, leading to the production of SCAFs (84, 86, 87) that, as described above, contribute to the energy metabolism of the host and modulate its immune system (88).
Some dietary fiber can be classified as prebiotics (89). Gibson G. R. and colleagues defined a prebiotic as “a non-digestible food ingredient that beneficially affects the host by selectively stimulating the growth and/or activity of one or a limited number of bacteria in the colon, and thus improves host health” (90). Subsequently, as shown by the same researcher (91), prebiotics has been defined as “a selectively fermented ingredient that allows specific changes, both in the composition and/or activity in the gastrointestinal microflora, that confer benefits” (86).
Prebiotics are composed of carbohydrates, mainly oligosaccharides, which resist to the digestion in the upper gastrointestinal tract and are fermented in the colon, producing SCFAs and favoring the growth of beneficial bacteria, particularly Bifidobacterium, Faecalibacterium prausnitzii, A. Muciniphila, and Ruminoccoccus bromii (28). The main target of prebiotics, in fact, are some intestinal commensal bacteria, such as Lactobacilli and Bifidobacteria, also known as probiotics (86, 92).
Finally, probiotics have been defined as “live microorganisms that confer a health benefit to the host, when administered in adequate doses” (93). In fact, probiotics can prevent intestinal infections by inhibiting the adhesion of pathogens to the intestinal mucosa, are involved in the maintenance of the physiological gut permeability through the increased production of mucin and the strengthen of tight junctions, and modulate innate and adaptive immune system (92, 94).
The beneficial effects of MD could also be related with its ability to impact the composition of the gut microbiota, leading to the production of several metabolites able to promote metabolic and molecular health (95–97). In this regard, it has been described that MD is associated with a lower E. coli fecal counts and a higher culture-based Bifidobacteria:E. coli ratio, increased levels of C. albicans, greater amount of SCFAs (98), and with a higher levels of Faecalibacterium prausnitzii and Clostridium cluster XVIa (99). According to Tindall et al. (100), further researches are needed to investigate the relationship between MD and gut enterotypes, although a study carried out by Wu et al. showed that Bacteroides “enterotype” was highly associated with animal protein and saturated fat consumption, while Prevotella “enterotype” was linked to high values of carbohydrates and simple sugars (101).
As described above, MD is characterized by a higher intake of plant foods (71), which contain microconstituents with significant biological activity, known as bioactive compounds or phytochemicals (102). According to Kitty et al. bioactive compounds are “extra-nutritional” constituents naturally present in small quantities in the food matrix (103) and include flavonoids, resveratrol, phytoestrogens, lycopene, phytosterols, dietary fibers, isothiocyanates, and monoterpenes (104). Most of these compounds have antioxidant activity (102), contributing in the prevention of chronic disease (102, 105).
Mediterranean Diet and T1DM
T1DM is associated with high cardiovascular risk (106), which is largely influenced by environmental factors, such as diet (107).
It is known that MD, rich in monounsaturated fat and fiber, is protective against cardiovascular risk (108). This observation led to the recommendation of a higher consumption of saturated fatty acids (SFA) and dietary fiber (109), as also suggested by several studies demonstrating an inverse association between dietary fiber and cardiovascular risk (110, 111). Moreover, a multicentric study carried out in a population of European type 1 diabetic patients examined the effects of SFA and dietary fiber intake on the onset of cardiovascular disease, and showed that an increased consumption of fiber, especially soluble fiber, can prevent both cardiovascular and all-cause mortality risk in these patients (112). Finally, a recent study confirmed that MD reduces cardiovascular risk but also proved that it improves glycemic control in type 1 diabetic patients (113).
Gut Microbiota, Mediterranean Diet, and T1DM
It has been proved that diet largely influences human gut microbiota (114, 115). MD, which recommends a grate intake of whole grains, fruit, vegetables, legumes, fiber and unsaturated fats, contributes to a favorable microbiota, and gut-related metabolomic profile (97, 116). In this regard, a study carried out by De Filippis et al. showed that a high-level adherence to the MD was associated with an increased amount of Prevotellaceae and a reduced Firmicutes-to-Bacteroidetes ratio, and with a greater levels of SCFAs and a lower concentrations of TMAO (97). Accordingly, a recent study carried out in an elderly population, showed that MD alters gut microbiota, promoting the growing of taxa such as Faecalibacterium prausnitzii, Bacteroides thetaiotaomicron, and Prevotella copri, which contribute to the formation of SCFAs (117).
Furthermore, MD-induced changes in gut microbiota promote a greater production of SCFAs, and particularly of butyrate, which exert an immunoregulatory effects (118), as described above, and regulate oxidative stress and inflammation in the gut and in others peripheral tissues (119, 120).
Gut microbiota is also modulated by probiotics, which contribute to maintenance of physiological intestinal permeability, thus interfering with autoimmune pancreatic β-cell destruction (121), as shown in a study carried out in a mice model of T1DM, in which the administration of Lactobacillus johnsonii delayed the development and the progression of the disease (122).
Conclusions
The interaction between diet, intestinal microbiota and the development and progression of T1DM could lead to the hypothesis that MD can modify both the onset and the progression of the disease by modulating the intestinal microflora and reducing the associated pro-inflammatory profile.
The high intake of fibers could especially contribute to a favorable ecology of the microbiota which increases the production of SCAFs, mainly of butyrate, leading to the maintenance of physiological intestinal permeability and thus regulating the response of the immune system. In addition, the large amount of fiber improves glycemic control and fat absorption, slowing the progression of the disease and delaying the development of cardiovascular complications.
Nevertheless, further researches are needed to evaluate the effect of MD on the gut microbiota of type 1 diabetic patients and to investigate the potential beneficial effects of a greater adherence to the MD in this population, to better comprehend its eventual prophylactic and therapeutic role. Moreover, it could be interesting to evaluate the effects of a higher intake of probiotics in type 1 diabetic subjects and in their microbiota, to better understand the role of probiotics, as therapeutic agents, in the modification of the composition of the human gut microbiota and in the onset and progression of diabetic disease.
To date, according with the data currently published, we recommend a greater adherence to MD, favoring a large consumption of dietary fiber and probiotics and we suggest more researches in this field, also to find new probiotic's formulations, with the aim of ameliorate the adhesion to the MD and to take healthy advantages of its consumption.
Author Contributions
CC, AV, and GC designed the review, performed the literature analysis, and wrote the manuscript. GC critically revised the text and gave a substantial scientific contribution. All authors approved the final version of the manuscript for publication.
Conflict of Interest
The authors declare that the research was conducted in the absence of any commercial or financial relationships that could be construed as a potential conflict of interest.
References
1. American Diabetes Association. Classification and diagnosis of diabetes: standards of medical care in diabetes −2020. Diabetes Care. (2020) 43 (Suppl. 1):S14–31. doi: 10.2337/dc20-S002
2. Ilonen J, Lempainen J, Veijola R. The heterogeneous pathogenesis of type 1 diabetes mellitus. Nat Rev Endocrinol. (2019) 15:635–50. doi: 10.1038/s41574-019-0254-y
3. Di Meglio LA, Evans-Molina C, Oram RA. Type 1 diabetes. Lancet. (2018) 391:2449–62. doi: 10.1016/S0140-6736(18)31320-5
4. Acharjee S, Ghosh B, Al-Dhubiab BE, Nair AB. Understanding type 1 diabetes: etiology and models. Can J Diabetes. (2013) 37:269–76. doi: 10.1016/j.jcjd.2013.05.001
5. Ounissi-Benkalha H, Polychronakos C. The molecular genetics of type 1 diabetes: new genes and emerging mechanisms. Trends Mol Med. (2008) 14:268–75. doi: 10.1016/j.molmed.2008.04.002
6. Nyaga DM, Vickers MH, Jefferies C, Perry JK, O'Sullivan JM. The genetic architecture of type 1 diabetes mellitus. Mol Cell Endocrinol. (2018) 477:70–80. doi: 10.1016/j.mce.2018.06.002
7. Bluestone JA, Herold K, Eisenbarth G. Genetics, pathogenesis and clinical interventions in type 1 diabetes. Nature. (2010) 464:1293–300. doi: 10.1038/nature08933
8. Concannon P, Rich SS, Nepom GT. Genetics of type 1A diabetes. N Engl J Med. (2009) 360:1646–54. doi: 10.1056/NEJMra0808284
9. Cerna M. Epigenetic regulation in etiology of type 1 diabetes mellitus. Int J Mol Sci. (2019) 21:36. doi: 10.3390/ijms21010036
10. Pociot F, Lernmark Å. Genetic risk factors for type 1 diabetes. Lancet. (2016) 387:2331–9. doi: 10.1016/S0140-6736(16)30582-7
11. Atkinson MA, Eisenbarth GS, Michels AW. Type 1 diabetes. Lancet. (2014) 383:69–82. doi: 10.1016/S0140-6736(13)60591-7
12. Rewers M, Ludvigsson J. Environmental risk factors for type 1 diabetes. Lancet. (2016) 387:2340–8. doi: 10.1016/S0140-6736(16)30507-4
13. Norris JM, Johnson RK, Stene LC. Type 1 diabetes-early life origins and changing epidemiology. Lancet Diabetes Endocrinol. (2020) 8:226–38. doi: 10.1016/S2213-8587(19)30412-7
14. Yeung WC, Rawlinson WD, Craig ME. Enterovirus infection and type 1 diabetes mellitus: systematic review and meta-analysis of observational molecular studies. BMJ. (2011) 342:d35. doi: 10.1136/bmj.d35
15. Laitinen OH, Honkanen H, Pakkanen O, Oikarinen S, Hankaniemi MM, Huhtala H, et al. Coxsackievirus B1 is associated with induction of β-cell autoimmunity that portends type 1 diabetes. Diabetes. (2014) 63:446–55. doi: 10.2337/db13-0619
16. Esposito S, Toni G, Tascini G, Santi E, Berioli MG, Principi N. Environmental factors associated with type 1 diabetes. Front Endocrinol. (2019) 10:592. doi: 10.3389/fendo.2019.00592
17. Virtanen SM. Dietary factors in the development of type 1 diabetes. Pediatr Diabetes. (2016) 17 (Suppl. 22):49–55. doi: 10.1111/pedi.12341
18. Nielsen DS, Krych Ł, Buschard K, Hansen CH, Hansen AK. Beyond genetics influence of dietary factors and gut microbiota on type 1 diabetes. FEBS Lett. (2014) 588:4234–43. doi: 10.1016/j.febslet.2014.04.010
19. Virtanen SM, Nevalainen J, Kronberg-Kippilä C, Ahonen S, Tapanainen H, Uusitalo L, et al. Food consumption and advanced β cell autoimmunity in young children with HLA-conferred susceptibility to type 1 diabetes: a nested case-control design. Am J Clin Nutr. (2012) 95:471–8. doi: 10.3945/ajcn.111.018879
20. Muntoni S, Cocco P, Aru G, Cucca F. Nutritional factors and worldwide incidence of childhood type 1 diabetes. Am J Clin Nutr. (2000) 71:1525–9. doi: 10.1093/ajcn/71.6.1525
21. Norris JM, Yin X, Lamb MM, Barriga K, Seifert J, Hoffman M, et al. Omega-3 polyunsaturated fatty acid intake and islet autoimmunity in children at increased risk for type 1 diabetes. JAMA. (2007) 298:1420–8. doi: 10.1001/jama.298.12.1420
22. Bi X, Li F, Liu S, Jin Y, Zhang X, Yang T, et al. ω-3 polyunsaturated fatty acids ameliorate type 1 diabetes and autoimmunity. J Clin Invest. (2017) 127:1757–71. doi: 10.1172/JCI87388
23. Leeming ER, Johnson AJ, Spector TD, Le Roy CI. Effect of diet on the gut microbiota: rethinking intervention duration. Nutrients. (2019) 11:2862. doi: 10.3390/nu11122862
24. Zheng P, Li Z, Zhou Z. Gut microbiome in type 1 diabetes: a comprehensive review. Diabetes Metab Res Rev. (2018) 34:e3043. doi: 10.1002/dmrr.3043
25. Putignani L, Del Chierico F, Petrucca A, Vernocchi P, Dallapiccola B. The human gut microbiota: a dynamic interplay with the host from birth to senescence settled during childhood. Pediatr Res. (2014) 76:2–10. doi: 10.1038/pr.2014.49
26. Adak A, Khan MR. An insight into gut microbiota and its functionalities. Cell Mol Life Sci. (2019) 76:473–93. doi: 10.1007/s00018-018-2943-4
27. Arumugam M, Raes J, Pelletier E, Le Paslier D, Yamada T, Mende DR, et al. Enterotypes of the human gut microbiome. Nature. (2011) 473:174–80. doi: 10.1038/nature09944
28. Jardine M. Nutrition considerations for microbiota health in diabetes. Diabetes Spectr. (2016) 29:238–44. doi: 10.2337/ds16-0003
29. Thursby E, Juge N. Introduction to the human gut microbiota. Biochem J. (2017) 474:1823–36. doi: 10.1042/BCJ20160510
30. Jandhyala SM, Talukdar R, Subramanyam C, Vuyyuru H, Sasikala M, Nageshwar Reddy D. Role of the normal gut microbiota. World J Gastroenterol. (2015) 21:8787–803. doi: 10.3748/wjg.v21.i29.8787
31. Sekirov I, Russell SL, Antunes LC, Finlay BB. Gut microbiota in health and disease. Physiol Rev. (2010) 90:859–904. doi: 10.1152/physrev.00045.2009
32. Hooper LV, Littman DR, Macpherson AJ. Interactions between the microbiota and the immune system. Science. (2012) 336:1268–73. doi: 10.1126/science.1223490
33. Rowland I, Gibson G, Heinken A, Scott K, Swann J, Thiele I, et al. Gut microbiota functions: metabolism of nutrients and other food components. Eur J Nutr. (2018) 57:1–24. doi: 10.1007/s00394-017-1445-8
34. Davis-Richardson AG, Triplett EW. A model for the role of gut bacteria in the development of autoimmunity for type 1 diabetes. Diabetologia. (2015) 58:1386–93. doi: 10.1007/s00125-015-3614-8
35. Vinolo MA, Rodrigues HG, Nachbar RT, Curi R. Regulation of inflammation by short chain fatty acids. Nutrients. (2011) 3:858–76. doi: 10.3390/nu3100858
36. Luu M, Visekruna A. Short-chain fatty acids: Bacterial messengers modulating the immunometabolism of T cells. Eur J Immunol. (2019) 49:842–8. doi: 10.1002/eji.201848009
37. Vinolo MA, Rodrigues HG, Hatanaka E, Sato FT, Sampaio SC, Curi R. Suppressive effect of short-chain fatty acids on production of proinflammatory mediators by neutrophils. J Nutr Biochem. (2011) 22:849–55. doi: 10.1016/j.jnutbio.2010.07.009
38. Wen L, Ley RE, Volchkov PY, Stranges PB, Avanesyan L, Stonebraker AC, et al. Innate immunity and intestinal microbiota in the development of Type 1 diabetes. Nature. (2008) 455:1109–13. doi: 10.1038/nature07336
39. Jamshidi P, Hasanzadeh S, Tahvildari A, Farsi Y, Arbabi M, Mota JF, et al. Is there any association between gut microbiota and type 1 diabetes? a systematic review. Gut Pathog. (2019) 11:49. doi: 10.1186/s13099-019-0332-7
40. Alkanani AK, Hara N, Gottlieb PA, Ir D, Robertson CE, Wagner BD, et al. Alterations in intestinal microbiota correlate with susceptibility to type 1 diabetes. Diabetes. (2015) 64:3510–20. doi: 10.2337/db14-1847
41. Paun A, Yau C, Danska JS. Immune recognition and response to the intestinal microbiome in type 1 diabetes. J Autoimmun. (2016) 71:10–8. doi: 10.1016/j.jaut.2016.02.004
42. Murri M, Leiva I, Gomez-Zumaquero JM, Tinahones FJ, Cardona F, Soriguer F, et al. Gut microbiota in children with type 1 diabetes differs from that in healthy children: a case-control study. BMC Med. (2013) 11:46. doi: 10.1186/1741-7015-11-46
43. Giongo A, Gano KA, Crabb DB, Mukherjee N, Novelo LL, Casella G, et al. Toward defining the autoimmune microbiome for type 1 diabetes. ISME J. (2011) 5:82–91. doi: 10.1038/ismej.2010.92
44. Nakamura Y, Nagai Y, Kobayashi T, Furukawa K, Oikawa Y, Shimada A, et al. Characteristics of gut microbiota in patients with diabetes determined by data mining analysis of terminal restriction fragment length polymorphisms. J Clin Med Res. (2019) 11:401–6. doi: 10.14740/jocmr3791
45. de Goffau MC, Luopajärvi K, Knip M, Ilonen J, Ruohtula T, Härkönen T, et al. Fecal microbiota composition differs between children with β-cell autoimmunity and those without. Diabetes. (2013) 62:1238–44. doi: 10.2337/db12-0526
46. Knip M, Siljander H. The role of the intestinal microbiota in type 1 diabetes mellitus. Nat Rev Endocrinol. (2016) 12:154–67. doi: 10.1038/nrendo.2015.218
47. Dedrick S, Sundaresh B, Huang Q, Brady C, Yoo T, Cronin C, et al. The role of gut microbiota and environmental factors in type 1 diabetes pathogenesis. Front Endocrinol. (2020) 11:78. doi: 10.3389/fendo.2020.00078
48. Ulluwishewa D, Anderson RC, McNabb WC, Moughan PJ, Wells JM, Roy NC. Regulation of tight junction permeability by intestinal bacteria and dietary components. J Nutr. (2011) 141:769–76. doi: 10.3945/jn.110.135657
49. den Besten G, van Eunen K, Groen AK, Venema K, Reijngoud DJ, Bakker BM. The role of short-chain fatty acids in the interplay between diet, gut microbiota, and host energy metabolism. J Lipid Res. (2013) 54:2325–40. doi: 10.1194/jlr.R036012
50. Brown CT, Davis-Richardson AG, Giongo A, Gano KA, Crabb DB, Mukherjee N, et al. Gut microbiome metagenomics analysis suggests a functional model for the development of autoimmunity for type 1 diabetes. PLoS ONE. (2011) 6:e25792. doi: 10.1371/journal.pone.0025792
51. Endesfelder D, Engel M, Davis-Richardson AG, Ardissone AN, Achenbach P, Hummel S, et al. Towards a functional hypothesis relating anti-islet cell autoimmunity to the dietary impact on microbial communities and butyrate production. Microbiome. (2016) 4:17. doi: 10.1186/s40168-016-0163-4
52. Bibbò S, Dore MP, Pes GM, Delitala G, Delitala AP. Is there a role for gut microbiota in type 1 diabetes pathogenesis? Ann Med. (2017) 49:11–22. doi: 10.1080/07853890.2016.1222449
53. Bosi E, Molteni L, Radaelli MG, Folini L, Fermo I, Bazzigaluppi E, et al. Increased intestinal permeability precedes clinical onset of type 1 diabetes. Diabetologia. (2006) 49:2824–7. doi: 10.1007/s00125-006-0465-3
54. Gavin PG, Mullaney JA, Loo D, Cao KL, Gottlieb PA, Hill MM, et al. Intestinal metaproteomics reveals host-microbiota interactions in subjects at risk for type 1 diabetes. Diabetes Care. (2018) 41:2178–86. doi: 10.2337/dc18-0777
55. Ahuja M, Schwartz DM, Tandon M, Son A, Zeng M, Swaim W, et al. Orai1-mediated antimicrobial secretion from pancreatic acini shapes the gut microbiome and regulates gut innate immunity. Cell Metab. (2017) 25:635–46. doi: 10.1016/j.cmet.2017.02.007
56. Miani M, Le Naour J, Waeckel-Enée E, Verma SC, Straube M, Emond P, et al. Gut microbiota-stimulated innate lymphoid cells support β-defensin 14 expression in pancreatic endocrine cells, preventing autoimmune diabetes. Cell Metab. (2018) 28:557–72. doi: 10.1016/j.cmet.2018.06.012
57. Pound LD, Patrick C, Eberhard CE, Mottawea W, Wang GS, Abujamel T, et al. Cathelicidin antimicrobial peptide: a novel regulator of islet function, islet regeneration, and selected gut bacteria. Diabetes. (2015) 64:4135–47. doi: 10.2337/db15-0788
58. Stenwall A, Ingvast S, Skog O, Korsgren O. Characterization of host defense molecules in the human pancreas. Islets. (2019) 11:89–101. doi: 10.1080/19382014.2019.1585165
59. Sun J, Xu M, Ortsäter H, Lundeberg E, Juntti-Berggren L, Chen YQ, et al. Cathelicidins positively regulate pancreatic β-cell functions. FASEB J. (2016) 30:884–94. doi: 10.1096/fj.15-275826
60. Sun J, Furio L, Mecheri R, van der Does AM, Lundeberg E, Saveanu L, et al. Pancreatic β-cells limit autoimmune diabetes via an immunoregulatory antimicrobial peptide expressed under the influence of the gut microbiota. Immunity. (2015) 43:304–17. doi: 10.1016/j.immuni.2015.07.013
61. Brauner H, Lüthje P, Grünler J, Ekberg NR, Dallner G, Brismar K, et al. Markers of innate immune activity in patients with type 1 and type 2 diabetes mellitus and the effect of the anti-oxidant coenzyme Q10 on inflammatory activity. Clin Exp Immunol. (2014) 177:478–82. doi: 10.1111/cei.12316
62. Keys A, Aravanis C, Blackburn H, Buzina R, Djordjevic BS, Dontas AS, et al. Seven Countries Study. A Multivariate Analysis of Death and Coronary Heart Disease. Cambridge, MA: Harvard University Press (1980).
63. Kromhout D, Menotti A, Blackburn H. Prevention of Coronary Heart Disease. Diet, Lifestyle and Risk Factors in the Seven Countries Study. Norwell, MA; Dordrecht: Kluwer (2002).
65. Dernini S, Berry EM, Serra-Majem L, La Vecchia C, Capone R, Medina FX, et al. Med diet 4.0: the Mediterranean diet with four sustainable benefits. Public Health Nutr. (2017) 20:1322–30. doi: 10.1017/S1368980016003177
66. Sofi F, Abbate R, Gensini GF, Casini A. Accruing evidence on benefits of adherence to the Mediterranean diet on health: an updated systematic review and meta-analysis. Am J Clin Nutr. (2010) 92:1189–96. doi: 10.3945/ajcn.2010.29673
67. Sofi F, Cesari F, Abbate R, Gensini GF, Casini A. Adherence to Mediterranean diet and health status: meta-analysis. BMJ. (2008) 337:a1344. doi: 10.1136/bmj.a1344
68. D'Alessandro A, De Pergola G. The Mediterranean diet: its definition and evaluation of a priori dietary indexes in primary cardiovascular prevention. Int J Food Sci Nutr. (2018) 69:647–59. doi: 10.1080/09637486.2017.1417978
69. Vitiello V, Germani A, Capuzzo Dolcetta E, Donini LM, Del Balzo V. The new modern Mediterranean Diet italian pyramid. Ann Ig. (2016) 28:179–86. doi: 10.7416/ai.2016.2096
70. Davis C, Bryan J, Hodgson J, Murphy K. Definition of the Mediterranean diet; a Literature Review. Nutrients. (2015) 7:9139–53. doi: 10.3390/nu7115459
71. Willett WC, Sacks F, Trichopoulou A, Drescher G, Ferro-Luzzi A, Helsing E, et al. Mediterranean diet pyramid: a cultural model for healthy eating. Am J Clin Nutr. (1995) 61 (Suppl. 6):1402S−6. doi: 10.1093/ajcn/61.6.1402S
72. Yubero-Serrano EM, Lopez-Moreno J, Gomez-Delgado F, Lopez-Miranda J. Extra virgin olive oil: More than a healthy fat. Eur J Clin Nutr. (2019) 72 (Suppl. 1):8–17. doi: 10.1038/s41430-018-0304-x
73. Estruch R, Ros E, Salas-Salvadó J, Covas MI, Corella D, Arós F, et al. Primary prevention of cardiovascular disease with a Mediterranean diet supplemented with extra-virgin olive oil or nuts. N Engl J Med. (2018) 378:e34. doi: 10.1056/NEJMoa1800389
74. Hidalgo M, Prieto I, Abriouel H, Cobo A, Benomar N, Gálvez A, et al. Effect of virgin and refined olive oil consumption on gut microbiota. Comparison to butter. Food Res Int. (2014) 64:553–9. doi: 10.1016/j.foodres.2014.07.030
75. Zhao Z, Shi A, Wang Q, Zhou J. High oleic acid peanut oil and extra virgin olive oil supplementation attenuate metabolic syndrome in rats by modulating the gut microbiota. Nutrients. (2019) 11:3005. doi: 10.3390/nu11123005
76. Prieto I, Hidalgo M, Segarra AB, Martínez-Rodríguez AM, Cobo A, Ramírez M, et al. Influence of a diet enriched with virgin olive oil or butter on mouse gut microbiota and its correlation to physiological and biochemical parameters related to metabolic syndrome. PLoS ONE. (2018) 13:e0190368. doi: 10.1371/journal.pone.0190368
77. Rodríguez-García C, Sánchez-Quesada C, Algarra I, Gaforio JJ. The high-fat diet based on extra-virgin olive oil causes dysbiosis linked to colorectal cancer prevention. Nutrients. (2020) 12:1705. doi: 10.3390/nu12061705
78. Marcelino G, Hiane PA, Freitas KC, Santana LF, Pott A, Donadon JR, et al. Effects of olive oil and its minor components on cardiovascular diseases, inflammation, and gut microbiota. Nutrients. (2019) 11:1826. doi: 10.3390/nu11081826
79. Wang Z, Roberts AB, Buffa JA, Levison BS, Zhu W, Org E, et al. Non-lethal inhibition of gut microbial trimethylamine production for the treatment of atherosclerosis. Cell. (2015) 163:1585–95. doi: 10.1016/j.cell.2015.11.055
80. Ufnal M, Zadlo A, Ostaszewski R. TMAO: A small molecule of great expectations. Nutrition. (2015) 31:1317–23. doi: 10.1016/j.nut.2015.05.006
81. Koeth RA, Wang Z, Levison BS, Buffa JA, Org E, Sheehy BT, et al. Intestinal microbiota metabolism of L-carnitine, a nutrient in red meat, promotes atherosclerosis. Nat Med. (2013) 19:576–85. doi: 10.1038/nm.3145
82. Obeid R, Awwad HM, Rabagny Y, Graeber S, Herrmann W, Geisel J. Plasma trimethylamine N-oxide concentration is associated with choline, phospholipids, and methyl metabolism. Am J Clin Nutr. (2016) 103:703–11. doi: 10.3945/ajcn.115.121269
83. Winther SA, Øllgaard JC, Tofte N, Tarnow L, Wang Z, Ahluwalia TS, et al. Utility of plasma concentration of trimethylamine N-Oxide in predicting cardiovascular and renal complications in individuals with type 1 diabetes. Diabetes Care. (2019) 42:1512–20. doi: 10.2337/dc19-0048
85. Mudgil D, Barak S. Composition, properties and health benefits of indigestible carbohydrate polymers as dietary fiber: a review. Int J Biol Macromol. (2013) 61:1–6. doi: 10.1016/j.ijbiomac.2013.06.044
86. Slavin J. Fiber and prebiotics: mechanisms and health benefits. Nutrients. (2013) 5:1417–35. doi: 10.3390/nu5041417
87. Capuano E. The behavior of dietary fiber in the gastrointestinal tract determines its physiological effect. Crit Rev Food Sci Nutr. (2017) 57:3543–64. doi: 10.1080/10408398.2016.1180501
88. O'Grady J, O'Connor EM, Shanahan F. Review article: dietary fibre in the era of microbiome science. Aliment Pharmacol Ther. (2019) 49:506–15. doi: 10.1111/apt.15129
89. Holscher HD. Dietary fiber and prebiotics and the gastrointestinal microbiota. Gut Microbes. (2017) 8:172–84. doi: 10.1080/19490976.2017.1290756
90. Gibson GR, Roberfroid MB. Dietary modulation of the human colonic microbiota: introducing the concept of prebiotics. J Nutr. (1995) 125:1401–12. doi: 10.1093/jn/125.6.1401
91. Gibson GR, Probert HM, Loo JV, Rastall RA, Roberfroid MB. Dietary modulation of the human colonic microbiota: updating the concept of prebiotics. Nutr Res Rev. (2004) 17:259–75. doi: 10.1079/NRR200479
92. Bermudez-Brito M, Plaza-Díaz J, Muñoz-Quezada S, Gómez-Llorente C, Gil A. Probiotic mechanisms of action. Ann Nutr Metab. (2012) 61:1601–74. doi: 10.1159/000342079
93. Reid G. Probiotics: definition, scope and mechanisms of action. Best Pract Res Clin Gastroenterol. (2016) 30:17–25. doi: 10.1016/j.bpg.2015.12.001
94. Liu Y, Tran DQ, Rhoads JM. Probiotics in disease prevention and treatment. J Clin Pharmacol. (2018) 58 (Suppl. 10):S164–79. doi: 10.1002/jcph.1121
95. Tosti V, Bertozzi B, Fontana L. Health benefits of the mediterranean diet: metabolic and molecular mechanisms. J Gerontol A Biol Sci Med Sci. (2018) 73:318–26. doi: 10.1093/gerona/glx227
96. Nagpal R, Shively CA, Register TC, Craft S, Yadav H. Gut microbiome-Mediterranean diet interactions in improving host health. F1000Res. (2019) 8:699. doi: 10.12688/f1000research.18992.1
97. De F, Pellegrini N, Vannini L, Jeffery IB, La Storia A, Laghi L, et al. High-level adherence to a Mediterranean diet beneficially impacts the gut microbiota and associated metabolome. Gut. (2016) 65:1812–21. doi: 10.1136/gutjnl-2015-309957
98. Mitsou EK, Kakali A, Antonopoulou S, Mountzouris KC, Yannakoulia M, Panagiotakos DB, et al. Adherence to the Mediterranean diet is associated with the gut microbiota pattern and gastrointestinal characteristics in an adult population. Br J Nutr. (2017) 117:1645–55. doi: 10.1017/S0007114517001593
99. Gutiérrez-Díaz I, Fernández-Navarro T, Salazar N, Bartolomé B, Moreno-Arribas MV, de Andres-Galiana EJ, et al. Adherence to a Mediterranean diet influences the fecal metabolic profile of microbial-derived phenolics in a Spanish cohort of middle-age and older people. J Agric Food Chem. (2017) 65:586–95. doi: 10.1021/acs.jafc.6b04408
100. Tindall AM, Petersen KS, Kris-Etherton PM. Dietary patterns affect the gut microbiome-the link to risk of cardiometabolic diseases. J Nutr. (2018) 148:1402–7. doi: 10.1093/jn/nxy141
101. Wu GD, Chen J, Hoffmann C, Bittinger K, Chen YY, Keilbaugh SA, et al. Linking long-term dietary patterns with gut microbial enterotypes. Science. (2011) 334:105–8. doi: 10.1126/science.1208344
102. Saura-Calixto F, Goñi I. Definition of the Mediterranean diet based on bioactive compounds. Crit Rev Food Sci Nutr. (2009) 49:145–52. doi: 10.1080/10408390701764732
103. Kitts DD. Bioactive substances in food: identification and potential uses. Can J Physiol Pharmacol. (1994) 72:423–34. doi: 10.1139/y94-062
104. Kris-Etherton PM, Hecker KD, Bonanome A, Coval SM, Binkoski AE, Hilpert KF, et al. Bioactive compounds in foods: their role in the prevention of cardiovascular disease and cancer. Am J Med. (2002) 113 (Suppl. 9B):71S−88. doi: 10.1016/S0002-9343(01)00995-0
105. Silva LBAR, Pinheiro-Castro N, Novaes GM, Pascoal GFL, Ong TP. Bioactive food compounds, epigenetics and chronic disease prevention: Focus on early-life interventions with polyphenols. Food Res Int. (2019) 125:108646. doi: 10.1016/j.foodres.2019.108646
106. Rawshani A, Rawshani A, Franzén S, Eliasson B, Svensson AM, Miftaraj M, et al. Range of risk factor levels: control, mortality, and cardiovascular outcomes in type 1 diabetes mellitus. Circulation. (2017) 135:1522–31. doi: 10.1161/CIRCULATIONAHA.116.025961
107. Leroux C, Brazeau AS, Gingras V, Desjardins K, Strychar I, Rabasa-Lhoret R. Lifestyle and cardiometabolic risk in adults with type 1 diabetes: a review. Can J Diabetes. (2014) 38:62–9. doi: 10.1016/j.jcjd.2013.08.268
108. Martínez-González MA, Gea A, Ruiz-Canela M. The mediterranean diet and cardiovascular health. Circ Res. (2019) 124:779–98. doi: 10.1161/CIRCRESAHA.118.313348
109. Rydén L, Standl E, Bartnik M, Van den Berghe G, Betteridge J, de Boer MJ, et al. Guidelines on diabetes, pre-diabetes, and cardiovascular diseases: executive summary. The task force on diabetes and cardiovascular diseases of the European society of cardiology (ESC) and of the European association for the study of diabetes (EASD). Eur Heart J. (2007) 28:88–136. doi: 10.1093/eurheartj/ehl260
110. Pereira MA, O'Reilly E, Augustsson K, Fraser GE, Goldbourt U, Heitmann BL, et al. Dietary fiber and risk of coronary heart disease: a pooled analysis of cohort studies. Arch Intern Med. (2004) 164:370–6. doi: 10.1001/archinte.164.4.370
111. Overby NC, Flaaten V, Veierød MB, Bergstad I, Margeirsdottir HD, Dahl-Jørgensen K, et al. Children and adolescents with type 1 diabetes eat a more atherosclerosis-prone diet than healthy control subjects. Diabetologia. (2007) 50:307–16. doi: 10.1007/s00125-006-0540-9
112. Schoenaker DA, Toeller M, Chaturvedi N, Fuller JH, Soedamah-Muthu SS, EURODIAB Prospective Complications Study Group. Dietary saturated fat and fibre and risk of cardiovascular disease and all-cause mortality among type 1 diabetic patients: the EURODIAB prospective complications study. Diabetologia. (2012) 55:2132–41. doi: 10.1007/s00125-012-2550-0
113. Zhong VW, Lamichhane AP, Crandell JL, Couch SC, Liese AD, The NS. Association of adherence to a Mediterranean diet with glycemic control and cardiovascular risk factors in youth with type I diabetes: the SEARCH nutrition ancillary study. Eur J Clin Nutr. (2016) 70:802–7. doi: 10.1038/ejcn.2016.8
114. Maslowski KM, Mackay CR. Diet, gut microbiota and immune responses. Nat Immunol. (2011) 12:5–9. doi: 10.1038/ni0111-5
115. Mejía-León ME, Barca AM. Diet, microbiota and immune system in type 1 diabetes development and evolution. Nutrients. (2015) 7:9171–84. doi: 10.3390/nu7115461
116. Gentile CL, Weir TL. The gut microbiota at the intersection of diet and human health. Science. (2018) 362:776–80. doi: 10.1126/science.aau5812
117. Ghosh TS, Rampelli S, Jeffery IB, Santoro A, Neto M, Capri M, et al. Mediterranean diet intervention alters the gut microbiome in older people reducing frailty and improving health status: the NU-AGE 1-year dietary intervention across five European countries. Gut. (2020) 69:1218–28. doi: 10.1136/gutjnl-2019-319654
118. Bailey MA, Holscher HD. Microbiome-mediated effects of the Mediterranean Diet on Inflammation. Adv Nutr. (2018) 9:193–206. doi: 10.1093/advances/nmy013
119. Bach Knudsen KE, Lærke HN, Hedemann MS, Nielsen TS, Ingerslev AK, Gundelund Nielsen DS, et al. Impact of diet-modulated butyrate production on intestinal barrier function and inflammation. Nutrients. (2018) 10:1499. doi: 10.3390/nu10101499
120. Canani RB, Costanzo MD, Leone L, Pedata M, Meli R, Calignano A. Potential beneficial effects of butyrate in intestinal and extraintestinal diseases. World J Gastroenterol. (2011) 17:1519–28. doi: 10.3748/wjg.v17.i12.1519
121. Gomes AC, Bueno AA, de Souza RG, Mota JF. Gut microbiota, probiotics and diabetes. Nutr J. (2014) 13:60. doi: 10.1186/1475-2891-13-60
Keywords: type 1 diabetes mellitus, gut microbiota, Mediterranean diet, short-chain fatty acids, fibers
Citation: Calabrese CM, Valentini A and Calabrese G (2021) Gut Microbiota and Type 1 Diabetes Mellitus: The Effect of Mediterranean Diet. Front. Nutr. 7:612773. doi: 10.3389/fnut.2020.612773
Received: 30 September 2020; Accepted: 02 December 2020;
Published: 13 January 2021.
Edited by:
Annalisa Terranegra, Sidra Medicine, QatarReviewed by:
Silvia Turroni, University of Bologna, ItalyClaudia Vetrani, University of Naples Federico II, Italy
Copyright © 2021 Calabrese, Valentini and Calabrese. This is an open-access article distributed under the terms of the Creative Commons Attribution License (CC BY). The use, distribution or reproduction in other forums is permitted, provided the original author(s) and the copyright owner(s) are credited and that the original publication in this journal is cited, in accordance with accepted academic practice. No use, distribution or reproduction is permitted which does not comply with these terms.
*Correspondence: Cinzia Myriam Calabrese, cinzia.calabrese@gmail.com
†These authors have contributed equally to this work