Onion anthocyanins: Extraction, stability, bioavailability, dietary effect, and health implications
- 1Horticulture Crop Processing (HCP) Division, ICAR-Central Institute of Post-Harvest Engineering & Technology (CIPHET), Punjab, India
- 2Post Graduate Department of Biotechnology, Khalsa College, Amritsar, Punjab, India
- 3Department of Processing and Food Engineering, Punjab Agricultural University, Ludhiana, Punjab, India
- 4College of Agriculture, Agriculture University, Jodhpur, Rajasthan, India
- 5Division of Environmental Soil Science, ICAR-Indian Institute of Soil Science (IISS), Bhopal, MP, India
- 6Department of Mycology and Microbiology, Tea Research Association-North Bengal Regional R & D Center, Nagrakata, West Bengal, India
- 7Agricultural Research Station (ARS), Agriculture University, Kota, Rajasthan, India
- 8Department of Horticulture, G.B. Pant University of Agriculture and Technology, Pantnagar, Uttarakhand, India
- 9Department of Botany, Bhaskaracharya College of Applied Sciences, University of Delhi, New Delhi, India
- 10Department of Biochemistry, Punjab Agricultural University, Ludhiana, Punjab, India
Anthocyanins are high-value compounds, and their use as functional foods and their natural colorant have potential health benefits. Anthocyanins seem to possess antioxidant properties, which help prevent neuronal diseases and thereby exhibit anti-inflammatory, chemotherapeutic, cardioprotective, hepatoprotective, and neuroprotective activities. They also show different therapeutic effects against various chronic diseases. Anthocyanins are present in high concentrations in onion. In recent years, although both conventional and improved methods have been used for extraction of anthocyanins, nowadays, improved methods are of great importance because of their higher yield and stability of anthocyanins. In this review, we compile anthocyanins and their derivatives found in onion and the factors affecting their stability. We also analyze different extraction techniques of anthocyanins. From this point of view, it is very important to be precisely aware of the impact that each parameter has on the stability and subsequently potentiate its bioavailability or beneficial health effects. We present up-to-date information on bioavailability, dietary effects, and health implications of anthocyanins such as antioxidant, antidiabetic, anticancerous, antiobesity, cardioprotective, and hepatoprotective activities.
Introduction
Anthocyanins are a class of plant phenolic pigments and dietary compounds that have a role in human diseases, and these water-soluble pigments are the largest group of plant pigments, contributing different colors, such as the purple, red, and blue, present in fruits, flowers, vegetables, and grains (1). Currently, more people are health-conscious of what they are eating, and as a result, public demand for synthetic pigments such as indigo carmine, Alura Red, and brilliant blue have decreased. Even the food processing and regulatory authorities are seeking to minimize the use of synthetic food dyes and colorants. Nowadays, extensive research focuses on natural colorants like anthocyanins and their health benefits in functional foods (2). Anthocyanins, beyond their bioactivity, are used in foods as natural colorants, fulfilling the public demand for clean labels in food products (3). Anthocyanins seem to possess antioxidant properties, which help prevent neuronal diseases and thereby exhibit anti-inflammatory, cardioprotective, chemotherapeutic, hepatoprotective, and neuroprotective activities, as well as therapeutic effects against other human diseases (4, 5). Because of various health and other benefits, anthocyanins have been accepted by most people as a feed ingredient in recent years. In addition, as a phytonutrient, anthocyanins have anti-mutation and antioxidant activities are important to human health (6). In nature, more than 600 structurally distinct anthocyanins have been identified and characterized (7). For a long time, for enhancing aesthetics and appearance, synthetic colorants have been used. However, because of regulatory concerns, decreasing demands for synthetic colorants, and increasing demands for natural colorants, the food industry and research have shifted toward natural alternatives (8, 9).
Onions are the most important and frequently cultivated vegetable in India as well as worldwide, and the color of the bulb due to flavonoid compounds is an economically important trait. Red- and white-colored bulbs are used for cooking and salad, respectively (10–12). Onion has diverse phytochemicals, such as anthocyanins, flavonoids, phenolic compounds, triterpenoids, and organosulfur compounds, and due to these compounds, it has antioxidant, antibacterial, antidiabetic, and anti-inflammatory activities (13–17). Anthocyanins are the flavonoid compounds responsible for the purple/red color of onion and are highly concentrated in the skin (18). The onion waste is also a rich source of anthocyanins and is reused in foods as bioactive ingredients (19). In red onion, different types of anthocyanins, such as cyanidin 3-laminariobioside, cyanidin mono- and diglucosides, petunidin glucoside, peonidin mono- and diglucosides, and 5-carboxypyranocyanidin 3-glucoside, have been reported (20). Dietary flavonoids found in onions play an important role in human health and nutrition, and studies have reported that onions display many activities including anticancer (21–23), antibacterial (24), hepatoprotective (25), antioxidant (26, 27), antiplatelet (28), immunoprotective (29), anti-cholelithogenic (30), antithrombotic (31), anti-inflammatory (32), and neuroprotective (33) properties. Onion wastes also exhibit inhibitory activity against oxidative stress and enzymes responsible for metabolic syndrome (34–36). Due to their high health benefits to humans, the anthocyanin content of red onion bulbs and skin is extracted during processing (37).
For anthocyanins, different extraction processes have been used, while nowadays, improved methods are used to obtain high yield and improved stability. Anthocyanins are unstable, so it needs procedure optimization to prevent oxidation. Various factors such as temperature, UV radiation, enzymes, pH, chelating metal ion, SO2, and ascorbic acid affect its stability, resulting in degradation and color change (38–42).
Therefore, the present review is aimed to deliver a synthesis method from the literature that discusses anthocyanins found in onions, their stability, and extraction technologies that preserve the anthocyanin content. Furthermore, we also discuss the application of anthocyanins in foods and their various implications on human health.
Anthocyanins found in onion and their stability
In many fruits and vegetables (including onion bulbs), flavonoids and anthocyanins, which are secondary metabolites, are responsible for their vivid colors. Researchers have recently been paying attention to phytochemicals because of their antioxidant properties (43–45). One of their best-described properties is their ability to inhibit free radicals created by cells or environmental factors (44). Despite this, the outermost layers of onions are often discarded while used in raw or in cooked forms, thereby losing a valuable antioxidant component. Onion bulbs and skin contain many bioactive substances, such as fructo-oligosaccharides (FOSs), organosulfur compounds (OSCs), thiosulfinates, polyphenols, and flavonoids (46–48). There are a variety of colors of onions, such as white, yellow, red, pink, orange, and gold (Figure 1), which are primarily due to the presence of two kinds of flavonoids, namely, anthocyanin and flavonols. Anthocyanin generates a wide diversity of colors ranging from red and orange to blue and violet, and flavonols like quercetin and its derivatives give yellow and brown colors to develop in the epidermal cells of scale leaves.
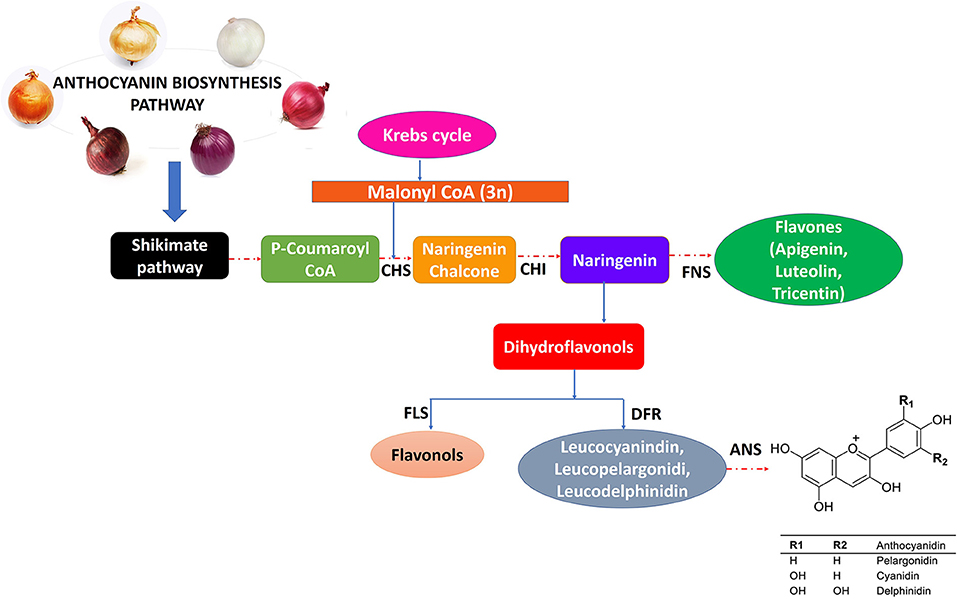
Figure 1. Variety of onion colors and anthocyanins biosynthesis pathway regulated by different enzymes.
Anthocyanins and flavonoids have distinct chemical structures, react with powerful free radicals, and possess antioxidants, anti-inflammatory, and anticancer properties and Alzheimer's and Parkinson's disease prevention properties (49–51). Research has been conducted on anthocyanins present in onions, leading to the identification of about 10 different types of anthocyanins. Among them, (3-(3-glucosyl-6-malonylglucoside), 3-(6”-malonylglucoside), 3-(3”-glucosylglucoside), and 3-glucoside of cyanidin) are primary anthocyanins (52). White onions have less anthocyanin than red onions. This may have resulted from anthocyanin contents found in each layer of onion. These facts show that red onions are a good source of anthocyanins (50). Among 25 anthocyanins found in red onions, cyanidin 3-O-glucoside is the primary anthocyanin present in the epidermal cells. However, derivatives of cyanidin and peonidin have also been reported. Approximately half of the red onion bulbs contain anthocyanins in the outer epidermis, but the inner epidermis contains smaller amounts (53). Despite being concentrated in the shell or skin, anthocyanin pigments are not present much in the edible portion of red onions. On the other hand, the highest concentrations of anthocyanins were found in the dry skin of red onions, which varied from 109 to 219 mg/100 g on average (50). In contrast to red onion, the white onion skin was reported to contain the lowest content of anthocyanin (0.75 mg/100 g), followed by yellow onion (9.64 mg/100 g) (54). Cyanidin 3-(6-malonylglucoside) (20.95 ± 0.60 mg/kg FW) is detected as major anthocyanin present in bulbs of red onion (Allium cepa L.) landrace “Krishnapuram” (KP) (55). The total anthocyanin content reported in onion was (28.6 mg/kg FW), and that of cyanidin 3-glucoside was 1.6 mg/kg FW) (56). Likewise, in Montoro ecotype, the cyanidin 3-glucoside content was 1.19 mg/kg FW (57). The different types of anthocyanins and their derivatives found in different types of onion are presented in Table 1, along with their various detection techniques.
Anthocyanins contain an anthocyanidin core that consists of a heterocyclic skeleton (Figure 2; called anthocyanidin or aglycon) and -OH or -OCH3 groups along with specific sugar or acylated sugar residues primarily at C3, C5, and/or C7 positions, and these can be modified further. It is thought that there are around 20 different core structures, which are named after the plants from which they were isolated: cyanidin, delphinidin, malvidin, pelargonidin, peonidin, and petunidin (Figure 3). As far as onions are concerned, they are frequently reported to contain cyanidin derivatives, as well as a few peonidin, pelargonidin, and delphinidin derivatives (66, 67). According to Bystricka et al., a variety of red onions contain anthocyanin glycosides, including cyanidin, peonidin, and pelargonidin (68). There has been some evidence that the edible portion of red onion contains about 250 mg/kg anthocyanins with cyanidin-3-glucoside as major components (44, 50, 54). The other derivatives of acylated and non-acylated anthocyanins, such as cyanidin mono- and diglucosides, peonidin mono- and diglucosides, petunidin glucoside, cyanidin 3-laminariobioside, and 5-carboxypyranocyanidin 3-glucoside, have been found in red onions (60).
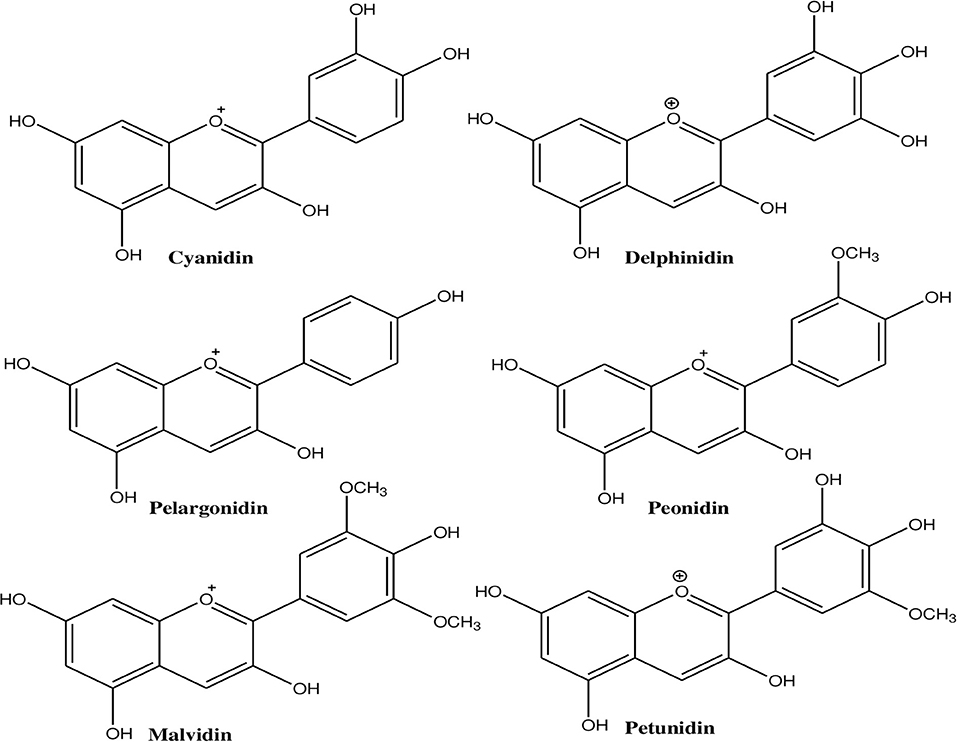
Figure 3. Different types of anthocyanins present in onion that contain -OH or -OCH3 groups at different positions.
Biosynthesis of anthocyanin occurs as part of a specific section of the flavonoid synthesis pathway, which is controlled on several levels. Through cinnamate-4-hydroxylase (C-4-H) and 4-coumaroyl CoA ligase (4-CL), phenylalanine is converted into cinnamic acid, which then undergoes conversion into 4-coumaryl CoA, an anthocyanin precursor. The next step is the condensation of one 4-coumaroyl CoA molecule and three malonyl CoA molecules using the chalcone synthase, which produces chalcones. In the final stage, a series of enzymatic reactions results in the synthesis of main anthocyanins. As anthocyanins are synthesized in the cytosol, they are transported to the vacuole, where they are stored in the form of color coalescences called anthocyanic vacuolar inclusions (53) (Figure 1). Various transcription factors and regulatory proteins are responsible for flavonoid biosynthesis (67). There is little information available on how flavonoids are regulated in onions. Recently, a report described the role of MYB1 as a transcription factor controlling anthocyanin biosynthesis in onions (69). The researchers also identified putative R2R3MYB genes in onions that are involved in the biosynthesis of anthocyanins and flavonols.
Factors affecting anthocyanin stability
Varying anthocyanin intensities and stability depend on several factors including the quantity and structure of anthocyanins, pH, temperature, light intensity, nature, and the existence of other pigments in conjunction with metal ions, enzymes, oxygen, sulfur dioxide, ascorbic acid, sugar, sugar metabolites, etc. (70). Based on structural activity studies, it was found that anthocyanins can be stabilized by the modification of their molecules through polymerization, cleavage, and derivatization. Anthocyanins are cleaved to produce colorless compounds, polymerization leads to browning, and derivatization produces colored compounds (71). Stabilizing reactions can be accomplished via glycosylation, methylation, and acylation, as well as anthocyanin aglycone synthesis (72). As anthocyanidins are hydroxylated, for instance, delphinidin pigments are shifted toward blue color, whereas when -OCH3 groups are glycosylated, red pigments with higher stability are synthesized (52). The stability is also enhanced by the acylation of the sugar moiety with aliphatic or aromatic acids. Numerous aromatic acids can be co-acylated, such as p-coumaric acid, gallic acid, sinapic acid, and ferulic acid, in addition to a wide variety of aliphatic acids, such as malic, tartaric, succinic, oxalic, and acetic acid (72, 73). Despite this, the colors formed by anthocyanins will also be affected by both the vacuolar environment and the structure created by anthocyanins.
pH
pH is a major factor that affects anthocyanins. Anthocyanins exist in six different states in the acidic vacuole of the plants. With pH 3 or less, the flavylium cation dominates and creates purple, orange, and red colors as the ionic nature of anthocyanins is found in different forms, which depend on pH. The flavylium form of anthocyanins is found at acidic pH = 1, and the quinoidal form is found at pH between 2 and 4, while the carbinol pseudobase form is found at pH between 5 and 6. Finally, at a pH higher than 7, anthocyanins will be degraded (52, 74–76). It has been shown that decreasing pH 2.8 in anthocyanin can increase the transfer of the structure to the flavylium cation, thereby improving stability; however, changing the pH of a liquid or gel could affect its sensory characteristics (77). It has been found that the color of anthocyanins can change because anthocyanins interact with other pigments and stack to form supermolecular structures, which stabilize at acidic pH of the colored forms and help in interaction with metal ions (53, 78, 79). Also, anthocyanin is much more soluble in water at lower pH as a result of the flavylium cation. Anthocyanins can undergo various acid–base, hydration, and tautomeric reactions when dissolved in water (80). With an increase in pH, the hydration reaction of the flavylium cation becomes more competitive with the proton transfer reactions involving acidic hydroxyl groups (60). In a pH range of 5-6, chalcone and carbinol pseudobase appear, and both are colorless (81). In addition, at a pH higher than 7, anthocyanins undergo degradation, depending on the substituent groups. Due to anthocyanin stability at low pH levels, foods with low pH levels are ideal for using anthocyanins. The presence of hydroxyl or methoxyl groups is responsible for the stability of anthocyanins. In neutral pH, mono- and diglycoside derivatives are more stable, while aglycones are not stable because in mono- and diglycoside derivatives of the sugar moieties will avoid the degradation of anthocyanins (82, 83). In short, the stability of anthocyanins decreases with an increasing number of hydroxyl groups, while it increases with increasing methylation and acylation, thus improving the stability of anthocyanins (84).
Temperature
Another important factor that influences the anthocyanin stability is temperature. Heat generally affects the anthocyanin stability through the activation of degradation, causing native enzymes. With an increase in temperature, there are a variety of mechanisms through which anthocyanins can be damaged and lost such as glycosylation, cleavage, nucleophilic attack by water, and polymerization (85). Although anthocyanins are weak substrates for enzymes, it has been demonstrated that B-glucosidase can affect anthocyanin persistence by generating anthocyanidins that can be further oxidized, by polyphenol oxidase (PPO) and/or peroxidase (POD) (86, 87). According to Jeya Krithika et al., anthocyanin in onion peels degrades when the temperature reaches a maximum of 100°C. Anthocyanin also interacts with objects due to its light sensitivity (60). Therefore, light is a major factor that influences the strength of anthocyanin. A recent study by Chithiraikannu et al. revealed that half of the pigments were destroyed when onion peels were placed in darkness for 135 days at 20°C, suggesting that acylation played a protective role (60). It was proven that an enhanced adjustment of the particle of anthocyanin in pH 1 buffer can increase absorbance because of the excitation of flavylium cations. Since anthocyanins are unsaturated molecules, they are susceptible to degradation by oxygen in two different ways: by direct oxidation and by enzyme-mediated decomposition (88). By contrast, some researchers found that phenols and anthocyanins in food increase during the first 7 days of cold storage if it is stored in an oxygen-enriched environment at a low temperature, during the initial days of storage (89). These enzymes cause anthocyanins to lose their solubility and transform them into colorless compounds, thus losing the pigment intensity of color (70). In addition, different plant regions and types of plants exhibit different amounts of anthocyanin depending on the regulatory molecules (precursors and enzymes) of biosynthesis and degradation pathways (52, 70, 90). Due to high temperature, anthocyanins undergo degradation by different mechanisms such as polymerization, glycosylation nucleophilic attack of water, and cleavage (85, 91). Therefore, with the increasing temperature, the degradation of anthocyanins occurs (92). The acylated form of anthocyanins has more stability than the non-acylated form at higher temperatures (93). However, heat treatment for a short period of time inhibits the enzymes and improves the stability of anthocyanins (94, 95) while at the same time prevents the thermal degradation of anthocyanins by decreasing the pH value. Furthermore, the decrease in oxygen concentration prevents the thermal degradation of anthocyanins (96, 97).
Extraction technology of anthocyanins
The anthocyanin extraction methods are broadly classified into two groups: first, conventional methods such as maceration, soaking, and heat-assisted extraction, which are easy to use and do not require much instrumentation but have limitations such as the possibility of solvents in the extract, toxicity of solvents, low yield, and thermal deterioration of anthocyanins; second, advanced technologies such as microwave-assisted extraction (MAE), high-pressure liquid extraction (HPLE), supercritical fluid extraction (SFE), ultrasound-assisted extraction (UAE), pulsed electric field extraction (PEFE), enzyme-assisted extraction (EAE), and high-voltage electrical discharge (HVED) (Figure 4), which are sophisticated but have advantages such as high stability of anthocyanins, lower or zero use of solvents, higher yield, and lower energy cost (38, 39, 41, 42, 64, 98–101).
Conventional methods
Conventional methods depend on the solubilizing capacity of solvents and for anthocyanins, ethanol, water, methanol, acetone, or mixtures thereof used. Anthocyanin is an unstable compound, so for stabilizing, weak acids such as citric acid, formic acid, or acetic acid are added in the solvent because anthocyanins are more stable at acidic pH. In addition to the solvents, the yield of anthocyanin depends on the size of the solid powder, time, temperature, and solvent-to-solid ratio. The anthocyanins are extracted by different polar solvents because of polar characteristics of anthocyanins, and selection of suitable solvents such as acetone, water, methanol alcohol, acidified methanol, or acidified acetonitrile is a crucial step because anthocyanins are highly reactive molecules (102–104). Zhang et al. reported using 0.1% hydrochloric acid in an 8:2 ratio of ethanol and water and found a higher concentration of anthocyanins and antioxidant activities in red, yellow, and white onions (50). Using the acidified ethanol and water, it was possible to extract a higher anthocyanin content of 22 mg/g dry material (105). Chandrasekhar et al. reported that using 50% (v/v) ethanol in water, the maximum anthocyanin content of 381.1 mg/L was extracted, while the higher anthocyanin content was recovered using acetone (425.9 mg/ L) and 1% HCl in methanol (419.7 mg/L) because of more polar solvents (106). Musso et al. observed that when the extract with water ethanol was stirred for half an hour at 300 rpm, the anthocyanin contents found in the alcoholic and aqueous extracts were 4 and 0.6 mg/L, respectively (107). Galvao et al. also observed that in a magnetic stirred batch with water, methanol, isopropanol, and ethanol at a controlled temperature of 25 C for a period of 3 h, the anthocyanin contents were found to be 50.37, 19.44, 0.77, and 0.76 mg/ L, respectively (108). Stirring in an orbital shaker with acidified water (0.05 M H3PO4) at 90 rpm for 12 h, the anthocyanin concentration was 32.05 mg/L (109). The conditions were optimized to extract anthocyanins with ethanol (9.1% v/v) acidified to pH 3 using citric acid because in acid pH, stabilization of the anthocyanin structure is high. It is concluded that with mild temperatures, the amounts of anthocyanins increased, while with longer time and high ethanol concentration, it decreased because longer time led to breakdown of cyanidin-3-O-glucoside compounds (110). Backes et al. also observed that using a mixture of ethanol (100% v/v) acidified to pH 3 and citric acid because in acidic pH, the degradation of anthocyanins is less because the structure of anthocyanins is more stable (111). The author revealed the yield of anthocyanin contents increased with the increase in ethanol concentration and temperature.
Improved methods
To overcome the disadvantages of conventional extraction methods, improved methods have been introduced for extraction. These methods are mainly focused on higher yield, environment-friendly, and industrial use. Among these improved methods are microwave-assisted extraction (MAE), high-pressure liquid extraction (HPLE), supercritical fluid extraction (SFE), ultrasound-assisted extraction (UAE), pulsed electric field extraction (PEFE), enzyme-assisted extraction (EAE), and high-voltage electrical discharge (HVED).
Microwave-assisted extraction (MAE)
The MAE method uses radiation in the microwave region such as electromagnetic radiation and to rupture the cell wall (112–114). Thus, the extract easily comes out of the cell to the solvent. Different conditions should be optimized during the MAE process, such as microwave power, liquid-to-solid ratio, time, particle size, and temperature. Xue et al. reported that the highest anthocyanin content was found at a temperature of 50.75°C (115), and Sun et al. also found the lowest degradation of anthocyanins was at a temperature of 53.3°C (116). Xue et al. found that the highest anthocyanin content was observed at 8-s irradiation time and a temperature of 50°C (117). The anthocyanins were found maximum (1,023.39 mg/ kg) at the conditions of a solid-to-water ratio of 1.3 g/ml, 800 W of microwave power, and 8 min of extraction time. These results indicated that reduction in extraction time enhances the extract concentration due to lower degradation of anthocyanins (118). Recently, the same results were also revealed by Nguyen et al., who found that the reduction in extraction time enhances the anthocyanin content. They found the optimal condition for maximum anthocyanin content (73.89 mg/L) was 60% ethanol concentration, 600 W of microwave power, a solid-to-solvent ratio of 1.30, and 2-min extraction time (119). Using ethanol as a solvent (100%; pH 3), optimized anthocyanin extraction conditions were a temperature of 62°C, time of extraction of 300 s, and 100:5 (mL/g) liquid-to-solid ratio. The anthocyanin content found was 411 mg/g of DW, and they also concluded that anthocyanins are more stable at lower pH (111). Farzaneh and Carvalho also optimized the conditions using water as a solvent at a temperature of 50°C, a time for extraction of 114 s, and a 30:1 (mL/g) liquid-to solid-ratio; the anthocyanin content found was 273.3 mg/L (120).
Ultrasound-assisted extraction (UAE)
The UAE method is based on ultrasound force that causes cavitation and breaks the cell wall (121, 122). The extraction of anthocyanin in the UAE method depends on different parameters such as temperature, solvent composition, time, the liquid-to-solid ratio, particle size, moisture content in the matrix, pulse cycle, and ultrasound power (110, 113, 123–126). The results obtained show a better yield (32 mg/g) of anthocyanin extracted from jabuticaba (110), which is in line with the results of Backes et al. and Demirdoven et al. that showed a higher yield of anthocyanin obtained by using the UAE method from red cabbage and fig peels, respectively (111, 127). Demirdoven et al. extracted anthocyanins using ethanol, water, and formic acid in an ultrasound bath operating at 37 kHz frequency. They extracted 11.92% more anthocyanins than those extracted using conventional methods at optimal conditions 40°C of temperature, 75 min of extraction time, and 45% of ethanol concentration. So, the anthocyanins were extracted at low temperature and low solvent concentration because at higher temperature, the degradation of anthocyanins is high (127). Ghavidel et al. also optimized the conditions for the extraction of anthocyanins using an ultrasonic power of 35 kHz, and the extracted concentration of anthocyanins was 9.468158 mg/L, with an ethanol-to-hydrochloric acid (15:85) at 60.94°C for 40 min (128). The anthocyanin yield was maximum (20.9 mg/L) using water as a solvent at 30 min of extraction at 15°C and 100 W using the UAE method because higher temperature and longer time of extraction degraded anthocyanins (124, 129, 130). Ravanfar et al. also revealed the optimal condition for extraction using water as a solvent at a different temperature, extraction time, ultrasonic power, and ultrasonic pulse duration for maximum anthocyanin yield (60 mg/L): power of 100 W, 15°C of temperature, 90 min of extraction time, and pulsation mode of 30 s (131). Using ethanol as solvent (34.47%) and anthocyanin extraction conditions optimized at a temperature of 35°C, time for extraction of 24 min, 100:5 (mL/g) liquid-to-solid ratio, the anthocyanin content was 32 mg/g of the extract (110).
Pressurized liquid extraction (PLE)
PLE is based on the use of solvents at different temperatures and pressure. The extraction yield of anthocyanins depends on different parameters such as temperature, static time, pressure, and the number of cycles (132–135). Wang et al. reported a higher yield of anthocyanins (8.15 mg/g) at 70 bar and 130°C during 90 min by using the PLE method (136). Kang et al. also found a maximum yield of anthocyanins at 100 bar and 130°C for 3 min from blueberries (74). Another method based on PLE is the high-pressure liquid extraction (HPLE) method, which is based on high pressure and keeps the solvent above its boiling point (100). In the HPLE method, the recovery efficiency mainly depends on the solvent, pressure, temperature, and the solvent-to-solid ratio (41). The optimal condition for maximum recovery of anthocyanins was found at 384 MPa pressure, for 15 min with 35% (v/v) ethanol (137) and increased with time from 15.1 to 39.4% from 5 to 15 min (138). Arapitsas and Turner, extracted anthocyanins with water + ethanol + formic acid in conditions temperature 80–120°C, sample amount 1–3 g, extraction time 6–11 min at a pressure of 50 bar, and anthocyanin yield was 662 μg/g of the sample. They revealed that the best conditions for anthocyanin extraction was at 2.5 g of sample, 7 min of extraction time, 99°C of temperature at 50 bar of pressure, and solvent composition of 94/5/1(v/v/v) of water/ethanol/formic acid (139). Pereira et al. also optimized the conditions for extraction of anthocyanins and found 10.21 mg malvidin-3-O-glucoside/g DW at an ethanol concentration of 50%, reactor volume of 50 mL, extraction time of 220 min, solvent flow of 5 g/min, temperature of 100°C, and pressure of 100 bar (140).
Pulsed electric field extraction (PEFE)
PEFE application is based on the increase in the electropermeabilization process to enhance the cell membrane permeability by an electrical force. The recovery of anthocyanins in the PEFE method depends on the electric field strength, the exposition time, initial temperature, and the total specific energy (42, 141–144). Thus, Gagneten et al. observed the effect of the temperature at 10 or 22°C and found higher extraction recovery at 22°C (145). Many studies have observed that recovery of anthocyanins increased with increase in the pulse number and pulse intensity (146–148). In the PEF method using water as a solvent with the conditions as a temperature of 22°C, electric field strength of 2.5 kV/cm, and specific energy of 15.63 J/g, the anthocyanin concentration was 44 to 889 μg/mL. Total anthocyanin extraction increased by 2.12 times because the non-acylated forms of anthocyanins have high proportion than the control (149).
Enzyme-assisted extraction (EAE)
In the enzyme-assisted extraction (EAE) method, pectinases, proteases, cellulases, and hemicelluloses have been used to accelerate and enhance the recovery of pigments from different matrices (150, 151). EAE has higher efficiency than the conventional methods for the extraction of pigments, and extraction depends on different conditions such as pH, the composition of the enzymatic mixture, temperature, liquid-to-solid ratio, and time (150–154). In EAE, using pectinex containing pectinase, cellulose, and hemicellulase at pH of 3.5, temperature of 45°C, liquid-to-solid ratio of 10:1, and hydrolysis time of 120 min, the anthocyanin concentration was 675 mg/100 g of the extract (151, 155). Li et al. also optimized the conditions using pectinase enzyme at pH of 5.9, temperature of 45°C, liquid-to-solid ratio of 20:1, and hydrolysis time of 58 min, anthocyanin concentration was 6.04 mg/g of sample (156).
Supercritical fluid extraction (SFE)
SFE efficiency depends on different conditions and needs to be optimized during the extraction process, and these conditions are pressure, amount of co-solvent, temperature, particle size, extraction time, moisture content, the flow rate of CO2, and the liquid-to-solid ratio (157–164). Maran et al. optimized the conditions for maximum recovery and found a higher yield at 50°C and 2 g/min solvent flow rate (163). Jiao et al. showed a higher yield of anthocyanins and antioxidant activity were obtained by using the SFE method than by using conventional extraction methods (164). Jio and Kermanshahi optimized the extraction conditions for the extraction of anthocyanins with a flow rate of 10 mL/min and found the anthocyanin concentration was 16.7–57.7%, which was more than that obtained using conventional methods (164). Researchers optimized the extraction conditions using acidified water under pressurized CO2 at different temperatures, extraction times, and pressure levels and found the optimal conditions at 60°C, 10 Mpa of pressure, and extraction time of 20 min yielded higher anthocyanin contents. They reported that the reduction in extraction time helps in increased efficiency of anthocyanin extractions (165). SFE with CO2 and co-solvent water is used for the extraction of anthocyanins. The anthocyanin yield was 25 mg/g of DW at pressure of 450 bar, flow rate of 15 min static time, and 20 min dynamic time at 10 mL/min (164). Maran et al. also optimized the extraction of anthocyanins with ethanol co-solvent, and the anthocyanin concentration found was 231.28 mg/100 g of the raw material at a pressure of 162 bar and a flow rate of 2 g/min (163).
Applications in foods and health benefits
As a necessary consequence, several studies on the delivery of anthocyanins from various sources into foods and their products like beverages, dairy products, soft drinks, jams, confectionery, and more have been carried out in recent years (42, 166, 167). Recent studies on in vitro experimental systems using anthocyanin-rich extracts or purified anthocyanins have confirmed high potential to applications in foods. Protection against liver damage, improvement in eye sight, significant reduction in blood pressure, strong anti-inflammatory and antimicrobial activities, and suppression of human cancer cell proliferation are all demonstrable benefits (Figure 5). Also, Figure 5 demonstrates that whenever there is any inflammation in the body or any chronic disease, parasitic infection, or microbial infection, it causes oxidative stress due to release of free radical species in the body. When anthocyanins are used, they act as an antioxidant and neutralize the free radicals and thereby decrease the oxidative stress.
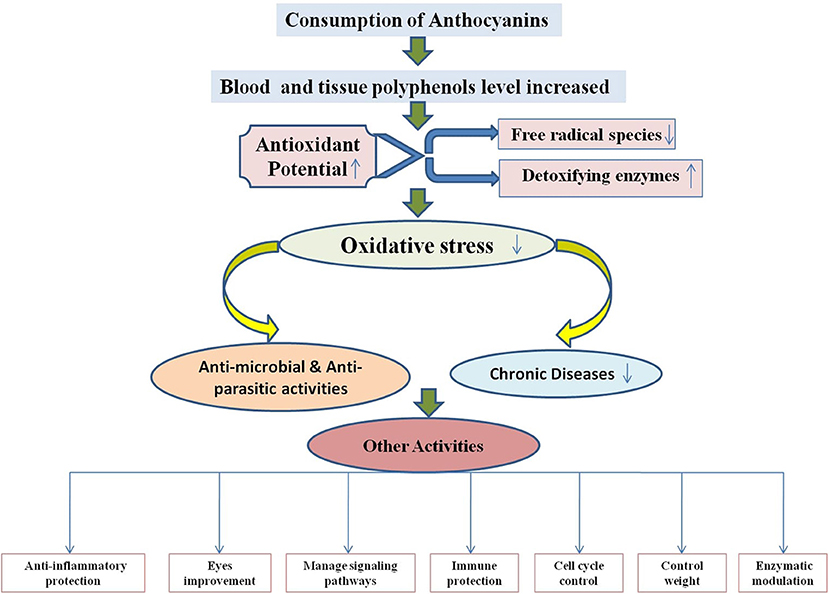
Figure 5. Mechanism of antioxidant potential of anthocyanins and reduction of chronic diseases, microbial, and parasitic activities.
Bioavailability and dietary effects of anthocyanins
Food extracts high in anthocyanins have been used in the development of dietary food supplements. Anthocyanins extracted from purple corn are used as an antioxidant dietary supplement are good health promoters. Anthocyanin is a bioactive component found in foods used as an appetite stimulant, phyto-pharmaceutical drug, and choleretic agent and for treatment of many other diseases (168). Most studies on the bioavailability of anthocyanins indicate that it is absorbed rapidly following consumption (about 0.22 to 2 h) and that it is excreted within 6 h (169, 170). In vitro studies on humans and animals show that anthocyanins are absorbed in their intact glycosidic form, unlike other flavonoids (171–173). Evidence shows that <1% of consumed anthocyanins are detected in the urine or plasma of humans (174–176). The bioavailability of anthocyanin as a nutraceutical is critical for maintaining good health and disease prevention like malvidin-3-glucoside, and cyanidin-3-glucoside is well-documented among major anthocyanins. Peonidin glycosides have the highest relative bioavailability, followed by cyanidin, malvidin, delphinidin, and petunidin glycosides of red wine anthocyanins (177, 178). Recently, anthocyanins are encapsulated with different dietary fibers such as gum arabic (179) or β-cyclodextrin (180) for their low release of anthocyanins during digestion. The encapsulation techniques enhanced the bioavailability of anthocyanins in the colon and delayed the release (181, 182). A study demonstrated the bioavailability and absorption of malvidin-3-glucoside anthocyanins, and it was observed that anthocyanins were found in the plasma and urine after 3 and 6 h of ingestion of red wine and red grape juice, respectively (183). Another finding also observed anthocyanins in the urine of volunteers who consumed 218 mg anthocyanins via 300 ml red wine and found that anthocyanins peaked in urine after 6 h of consumption. Recently, through 13C-tracer methodology, methylated and sulfo-conjugated metabolites were identified in urine and helped in the identification of degradation products during circulation through urine (184). Anthocyanins help reduce cholesterol levels and marketed as dietary supplements (185). Additionally, blue wheat bran was also used as dietary supplements and processed to produce an anthocyanin-rich blue wheat powder (186).
Anthocyanins are absorbed rapidly in the oral cavity of humans and appeared in the bloodstream after consumption, and degradation started in mouth. Absorption and degradation processes depend on oral microbiota (187). Anthocyanins have the ability to cross the blood–brain barrier and were observed in the endoepithelial cells, but not in certain tissues. Nowadays, different health organization suggested consumption of colorful vegetables and fruits because they provide essential nutrients and bioactive compounds (188, 189).
Physiological and health implications
There has been much research on anthocyanins that shows their therapeutic and food applications. As previously demonstrated, anthocyanins have a wide range of health and therapeutic effects (Figure 6). Table 2 presents the biological activities of anthocyanins in different systems and their health effects.
Antioxidant effects
Antioxidants in the diet can help reduce the generation of reactive oxygen species (ROS) and free radicals, lowering the risk of cancers and heart diseases. The antioxidant properties of anthocyanins are superior to well-known antioxidants such as butylated hydroxyl anisole (BHA) (212, 213). In addition to the ability of anthocyanin compounds to act as radical scavengers, the possess the ability to attack 2,2-diphenyl-1-picrylhydrazyl (DPPH) free radicals. DPPH is a stable free radical as the majority of its electrons are distributed over the whole molecule (214, 215). It has been suggested that the differences in DPPH-scavenging capacities of delphinidins and cyanidin-based anthocyanins are due to their molecular structure, which can determine their antioxidant properties and their bioactivity. For example, there are several natural antioxidants, but their amounts are low or they are primarily located in the pericarp and the embryo, which almost get destroyed in the processing (216). Using a genetic engineering technique called biofortification, we can generate different varieties with high anthocyanin contents, and this may help in the prevention of diseases (217). Antioxidant activity has been reported in different onion landraces such as “Cipolla di Giarratana” (218), “Vatikiotiko” (219), “Tropea” (220), and “Bianca di Pompei” (221). Vijayalakshmi et al. reported that onion extracts protect erythrocytes effectively (23%), against oxidative damage induced by hypochlorous acid in human erythrocytes (55), and Tedesco et al. also found onion extract inhibits hemolysis of blood cells induced by hypochlorous acid (222). In recent years, there has been an increase in interest in foods and plants containing antioxidant properties. The phytochemical compounds present in vegetables and colored fruits with these capacities are vitamins C and E, carotenoids, and flavonoids. These pigments, which are the most important groups of flavonoids, act as acidic compounds because they contain flavylium ions (AH+). As a result, their antioxidant activity is directly influenced by their structure (78, 222–226). According to Aprile et al. (227), the fully matured stage of olive, which is the best harvest time to obtain a table olive, is rich in phenolic compounds, anthocyanins, and other health-promoting nutrients. These compounds are used as antioxidants and anti-inflammatory agents and for preventive measures against several pathological states, such as cardiovascular diseases and tumors (6). In another study by Kim et al. using liquid chromatography and ultra-high-performance liquid chromatography, in combination with electrospray ionization and quadrupole time-of-flight, the antioxidant activity and anthocyanin composition of 12 cultivars of mulberry fruit were investigated and found major anthocyanins like cyanidin-3-O-glucoside and cyanidin-3-O-rutinoside showed significantly higher antioxidant activity (228).
Anticarcinogenic effects
In the past, hundreds of compounds including naturally occurring compounds and drugs have been identified as potential chemopreventive agents. Among them, compounds that naturally have the capability of inducing differentiation/apoptosis of cancer cells are the primary anticancer and chemopreventative agents (229). The potential for chemoprevention of cancer has been identified in hundreds of compounds, including drugs and naturally occurring components. According to Liu et al., raspberries inhibit the proliferation of hepatocellular liver carcinoma in a dose-dependent manner (230). Based on their research, Bonesi et al. found that cyanidin-3-glucoside causes apoptosis and cytodifferentiation in several leukemic cell lines (231).
Antiobesity and antidiabetic properties
Evidence suggests that a diet low in fat and high in fruits and vegetables reduces the risk of obesity and type-2 diabetes because they are rich in polyphenol compounds, a condition associated with insulin resistance. This condition occurs when insulin fails to effectively stimulate glucose transport in skeletal muscles and fat and fails to suppress hepatic glucose production (232). Anthocyanin has been shown to have lipid-lowering and antioxidant properties as blueberries can inhibit the early inflammatory response in adipose tissue to protect against whole-body insulin resistance and improve glycemia (233). Anthocyanins also play a role in insulin secretion. It protects pancreatic beta cells, from glucose-induced oxidative stress. The anthocyanin extract from colored rice increased the gene expression of genes associated with insulin secretion in rat pancreatic beta cells (234). According to the results, both anthocyanins and anthocyanidins stimulate insulin secretion, and delphinidin-3-glucoside was the most potent compound and significantly affected insulin secretion in comparison to untreated control cells at glucose concentrations of 4 and 10 mol/L (235). Diabetes mellitus (DM) is a chronic metabolic disease characterized by high blood sugar levels (236). Approximately 415 million adults between the ages of 20 and 79 years had diabetes mellitus in 2015, according to the International Diabetes Federation (IDF) (237). Over the past two decades, many efforts have been made to develop natural and less toxic antidiabetic agents. Scientists have been searching for new antidiabetic compounds made from natural sources to minimize side effects (238). Foods that are rich in bioactive compounds such as alkaloids, phenols, flavonoids, saponins, polysaccharides, terpenoids, glycosides, and xanthones can be consumed daily (239). Examples of food containing anthocyanins are berries such as cranberries, chokeberries, blackberries, gooseberries, black grape, bilberries, blueberries, red raspberries, blackcurrants, redcurrants, strawberry, pomegranates, apples, nectarine, peaches, plum radish, and plums; vegetables as red onion, red cabbage, eggplant, and purple potatoes; and seeds as black beans (240).
Cardioprotective roles of anthocyanins
The aging of the cardiovascular system involves endothelial dysfunction, intimal hyperplasy, and arterial stiffness, which may lead to arterial arteriosclerosis and atherosclerosis (241). Nutritional interventions are promising approaches to slow down the aging process of the cardiovascular system (241, 242). As a prerequisite for understanding the mechanisms of action of fruits and vegetables, it is imperative to identify bioactive compounds responsible for such beneficial effects and to demonstrate causality using accredited end points. It is also essential to study how such compounds are absorbed, distributed, metabolized, and excreted by healthy humans. Until now, polyphenols are the most promising class of food bioactive compounds found in fruits and vegetables (243, 244). Blueberries are not just a rich source of polyphenols, such as anthocyanin (ACN) but also contain flavonoids, flavonols, and phenolic acids, along with fiber, vitamins, and minerals (245). According to the Nurses' Health Study II, consuming large quantities of blueberries and strawberries, as well as large quantities of anthocyanin (as calculated per food frequency questionnaire), was associated with a lower risk of myocardial infarction (246). Even though these results suggest that anthocyanin intake with blueberries could lower cardiovascular risk, epidemiological data inherently only provide associative evidence, further limited by the lack of biomarkers of intake. Rodriguez et al. in their study suggested that anthocyanin metabolites contribute to healthy cardiovascular aging through mediating biological activities of blueberries and also induced gene expression in a manner that inhibits inflammation and reduces the risk of cardiovascular disease (247). Studies to be conducted in future will enhance our understanding of the mechanisms of action of individual metabolites, establish general structure–function relationships, and identify relevant interactions.
Hepatoprotective role of anthocyanins
The hepatotoxic compounds such as carbon tetrachloride (CCl4), allyl alcohol, 1-naphthyl isocyanate, and thioacetamide are proven to cause liver tissue necrosis in various areas of the liver in experimental medicine (248). While molecular CCl4 is not toxic, its hepatotoxicity arises from the formation of trichloromethyl radicals (*CCl3) and trichloromethyl peroxide of the radicals (CCl3O) following liver metabolism (249, 250). Romero et al. studied the effects of CCl4 intoxication on protein synthesis (251). Therefore, the total protein content can be considered as a useful index of cellular dysfunction in liver disorders. In a recent study, the ethyl acetate fraction of Justicia spicigera was evaluated for its efficiency against CCl4-induced liver damage. As indicated by the attenuation of liver function indices and improvement in markers of oxidative stress, ethyl acetate (EA) fraction protects the liver in a pathological condition (252). However, such types of studies have not been undertaken for onion-related anthocyanins and can be investigated in future research projects.
Conclusion
Recently, anthocyanins from onion are gaining popularity because of diverse health-promoting effects and natural colorants as natural antioxidants. The extraction methods of anthocyanins from onion need optimized conditions because of the sensitivity of anthocyanins. However, the extracted anthocyanins are susceptible to degradation effects by different factors such as pH, light, temperature, enzymes, and oxygen. Although the advanced technology showed a better potential to retain anthocyanins, the use of advanced methods needs further investigation toward industrial application, and due to the high cost of extraction, research could focus on assessing the economic viability of the methods.
Anthocyanins can be helpful in treatment of diseases and show different preventive activities such as antioxidant, antidiabetic, anticancerous, antiobesity, cardioprotective, and hepatoprotective properties. In order to extend the efficiency of anthocyanins to prevent diseases, new directions of research involve the incorporation of anthocyanins into targeted delivery systems that prevents the degradation of anthocyanins, enhances the bioavailability, and provides the stability in different conditions. Thus, different delivery systems have been developed such as dietary fiber-based, emulsions, microcapsules, or liposomes to enhance the bioavailability, and the effects produced by anthocyanins would be stronger. However, future studies are needed to focus on how to reduce the negative impact of each factor that influences the stability of anthocyanins.
Author contributions
MKS and MS contributed to the organization, writing, and composed of the manuscript. JT and AKP reviewed the manuscript. KK, S, DKY, YT, HR, and MR wrote the sections of manuscript. All authors contributed to the article and approved the submitted version.
Conflict of interest
The authors declare that the research was conducted in the absence of any commercial or financial relationships that could be construed as a potential conflict of interest.
Publisher's note
All claims expressed in this article are solely those of the authors and do not necessarily represent those of their affiliated organizations, or those of the publisher, the editors and the reviewers. Any product that may be evaluated in this article, or claim that may be made by its manufacturer, is not guaranteed or endorsed by the publisher.
Abbreviations
AURKA, aurora kinase A; Akt, protein kinase B; BIRC5, baculoviral IAP repeat containing 5; C4H, cinnamate-4-hydroxylase; 4-CL, coumaroyl CoA ligase; COX-2, cyclooxygenase-2; DPPH, 2, 2′-diphenyl-1-picrylhydrazyl; EAE, enzyme-assisted extraction; EGF, Epidermal growth factor; ERK1/2, extracellular signal-regulated kinase 1/2; HPLC, high-performance liquid chromatography; HPLC/DAD-ESI/MS, high-performance liquid chromatography with diode array and mass spectrometry; HPLC- MS, high-performance liquid chromatography–mass spectrometry; HVED, high-voltage electrical discharge; HPLE, high-pressure liquid extraction; iNOS, inducible nitric oxide synthase; MAE, microwave-assisted extraction; NF-kB, nuclear factor kappa-light chain-enhancer of activated B cells; ORAC, oxygen radical absorbance capacity methods; PTGS2, prostaglandin-endoperoxide synthase 2; SFE, supercritical fluid extraction; PEFE, pulsed electric fields; UHPLC, ultrahigh-performance liquid chromatography; UAE, ultrasound-assisted extraction.
References
1. Alvarez-Suarez JM, Cuadrado C, Redondo IB, Giampieri F, Gonzalez-Paramas AM, Santos-Buelga C, et al. Novel approaches in anthocyanin research-Plant fortification and bioavailability issues. Trends Food Sci Technol. (2021) 1:49. doi: 10.1016/j.tifs.2021.01.049
2. Swer TL, Chauhan K, Paul PK, Mukhim C. Evaluation of enzyme treatment conditions on extraction of anthocyanins from Prunus nepalensis L. Int J Biol Macromol. (2016) 92:867–71. doi: 10.1016/j.ijbiomac.2016.07.105
3. Chung C, Rojanasasithara T, Mutilangi W, Mc- Clements DJ. Stabilization of natural colors and nutraceuticals: inhibition of anthocyanin degradation in model beverages using polyphenols. Food Chem. (2016) 212:596–603. doi: 10.1016/j.foodchem.2016.06.025
4. Putta S, Yarla NS, Peluso I, Tiwari DK, Reddy GV, Giri PV, et al. Anthocyanins: multi-target agents for prevention and therapy of chronic diseases. Curr Pharm Des. (2017) 23:6321–46. doi: 10.2174/1381612823666170519151801
5. Ren KW, Li YH, Wu G, Ren JZ, Lu HB, Li ZM, et al. Quercetin nanoparticles display antitumor activity via proliferation inhibition and apoptosis induction in liver cancer cells. Int J Oncol. (2017) 50:1299–311. doi: 10.3892/ijo.2017.3886
6. Li D, Wang P, Luo Y, Zhao M, Chen F. Health benefits of anthocyanins and molecular mechanisms: update from recent decade. Crit Rev Food Sci Nutr. (2017) 57:1729–41. doi: 10.1080/10408398.2015.1030064
7. Saigo T, Wang T, Watanabe M, Tohge T. Diversity of anthocyanin and proanthocyanin biosynthesis in land plants. Curr Opin Plant Biol. (2020) 55:93–9. doi: 10.1016/j.pbi.2020.04.001
8. Sehrawat R, Panesar PS, Swer TL, Kumar A. Response surface methodology (RSM) mediated interaction of media concentration and process parameters for the pigment production by Monascuspurpureus MTCC using solid state fermentation. Pigment Resin Technol. (2017) 46:14–20. doi: 10.1108/PRT-08-2015-0077
9. Sehrawat R, Panesar PS, Panesar R, Kumar A. Biopigment produced by Monascuspurpureus MTCC in submerged and solid state fermentation: a comparative study. Resin Technol. (2017) 46:425–31. doi: 10.1108/PRT-10-2016-0095
10. Chinnappareddy LRD, Khandagale K, Chennareddy A, Ramappa VG. Molecular markers in the improvement of Allium crops. Czech J Genet Plant Breed. (2013) 49:131–9. doi: 10.17221/111/2013-CJGPB
11. Hassan NHS. Consumer preference implications on onion suppliers. International Int J Econ Manag. (2015) 4:281. doi: 10.4172/2162-6359.1000281
12. Veitch NC, Grayer RJ. Flavonoids and their glycosides, including anthocyanins. Nat Prod Rep. (2011) 28:1626–95. doi: 10.1039/c1np00044f
13. Bisen PS, Emerald M. Nutritional and therapeutic potential of garlic and onion (Allium sp.). Curr Nutr Food Sci. (2016) 12:190–9. doi: 10.2174/1573401312666160608121954
14. Putnik P, Gabric D, Roohinejad S, Barba FJ, Granato D, Lorenzo JM, et al. Bioavailability and food production of organosulfur compounds from edible Allium species. In: FJ Barba, JMA Saraiva, G Cravotto, JMBTIT, NTP Lorenzo, editors, Bioaccessibility and Bioavailability of Nutrients and Bioactive Compounds, In Innovative Thermal and Non-Thermal Processing, Bioaccessibility and Bioavailability of Nutrients and Bioactive Compounds Woodhead Publishing Series in Food Science, Technology and Nutrition. Sawston: Woodhead Publishing (2019). p. 293–308. doi: 10.1016/B978-0-12-814174-8.00010-X
15. Mai NT, Dung DT, Nga TT, Xuan VT, Doan VV, Tai BH, et al. Triterpenoid glycosides from the rhizomes of Allium ascalonicum and their anoctamin-1 inhibitory activity. Nat Prod Res. (2020) 21:1–9. doi: 10.1080/14786419.2020.1713122
16. Mobin L, Haq MA, Ali R, Naz S, Saeed SG. Antibacterial and antioxidant potential of the phenolic extract and its fractions isolated from Allium ascalonicum (onion) peel. Nat Prod Res. (2021) 2021:1948040. doi: 10.1080/14786419.2021.1948040
17. Prakash P, Radha M, Kumar N, Kumari S, Prakash S, Rathour M, et al. Therapeutic uses of wild plants by rural inhabitants of maraog region in District Shimla, Himachal Pradesh, India. Horticulturae. (2021) 7:343. doi: 10.3390/horticulturae7100343
18. Metrani R, Singh J, Acharya PK, Jayaprakasha G, Patil SB. Comparative metabolomics profiling of polyphenols, nutrients and antioxidant activities of two red onion (Allium cepa L). Cultivars Plants. (2020) 9:1077. doi: 10.3390/plants9091077
19. Ren F, Nian Y, Perussello CA. Effect of storage. Food processing and novel extraction technologies on onions flavonoid content: a review. Int Food Res J. (2020) 132:108953. doi: 10.1016/j.foodres.2019.108953
20. Slimestad R, Fossen T, Vagen IM. Onions: a source of unique dietary flavonoids. J Agric Food Chem. (2007) 55:10067–80. doi: 10.1021/jf0712503
21. Nishiumi S, Miyamoto S, Kawabata K, Ohnishi K, Mukai R, Murakami A, et al. Dietary flavonoids as cancer-preventive and therapeutic biofactors. Front Biosci. (2011) 3:1332–62. doi: 10.2741/229
22. Ren F, Reilly K, Gaffney M, Kerry JP, Hossain M, Rai DK, et al. Evaluation of polyphenolic content and antioxidant activity in two onion varieties grown under organic and conventional production systems. J Sci Food Agric. (2017) 97:2982–90. doi: 10.1002/jsfa.8138
23. Wang R, Yang L, Li S, Ye D, Yang LQ, Liu Z, et al. Quercetin inhibits breast cancer stem cells via downregulation of aldehyde dehydrogenase 1A1 (ALDH1A1), chemokine receptor type 4 (CXCR4), mucin 1 (MUC1), and epithelial cell adhesion molecule (EpCAM). Med Sci Monit. (2018) 24:412–20. doi: 10.12659/MSM.908022
24. Cushnie TT, Lamb AJ. Recent advances in understanding the antibacterial properties of flavonoids. Int J Antimicrob Agents. (2011) 38:99–107. doi: 10.1016/j.ijantimicag.2011.02.014
25. Peng Z, Gong X, Yang Y, Huang L, Zhang Q, Zhang P, et al. Hepatoprotective effect of quercetin against LPS/d-GalN induced acute liver injury in mice by inhibiting the IKK/NFkappaB and MAPK signal pathways. Int Immunopharmacol. (2017) 52:281–9. doi: 10.1016/j.intimp.2017.09.022
26. Lee B, Jung JH, Kim HS. Assessment of red onion on antioxidant activity in rat. Food Chem Toxicol. (2012) 50:3912–9. doi: 10.1016/j.fct.2012.08.004
27. Lee KA, Kim KT, Kim HJ, Chung MS, Chang PS, Park H, et al. Antioxidant activities of onion (Allium cepa L.) peel extracts produced by ethanol. Hot water, and subcritical water extraction. Food Sci Biotechnol. (2014) 23:615–21. doi: 10.1007/s10068-014-0084-6
28. Ro JY, Ryu JH, Park HJ, Cho HJ. Onion (Allium cepa L.) peel extract has anti-platelet effects in rat platelets. Springer Plus. (2015) 4:17. doi: 10.1186/s40064-015-0786-0
29. Kumar VP, Venkatesh YP. Alleviation of cyclophosphamide induced immunosuppression in Wistar rats by onion lectin (Allium cepaagglutinin). J Ethnopharmacol. (2016) 186:280–8. doi: 10.1016/j.jep.2016.04.006
30. Reddy RRL, Srinivasan K. Dietary fenugreek and onion attenuate cholesterol gallstone formation in lithogenicdietfed mice. Int J Exp Pathol. (2011) 92:308–19. doi: 10.1111/j.1365-2613.2011.00782.x
31. Lee SM, Moon J, Chung JH, Cha YJ, Shin MJ. Effect of quercetin-rich onion peel extracts on arterial thrombosis in rats. Food Chem Toxicol. (2013) 57:99–105. doi: 10.1016/j.fct.2013.03.008
32. Khajah MA, Orabi KY, Hawai S, Sary HG, El-Hashim AZ. Onion bulb extract reduces colitis severity in mice via modulation of colonic inflammatory pathways and the apoptotic machinery. J Ethnopharmacol. (2019) 241:112008. doi: 10.1016/j.jep.2019.112008
33. Singh T, Goel RK. Neuroprotective effect of Allium cepa L. in aluminium chloride induced neurotoxicity. Neurotoxicology. (2015) 49:1–7. doi: 10.1016/j.neuro.2015.04.007
34. Ogi K, Sumitani H. Elucidation of an α-glucosidase inhibitor from the peel of Allium Cepa by principal component analysis. Biosci Biotechnol Biochem. (2019) 83:751–4. doi: 10.1080/09168451.2018.1564619
35. Jaiswal N, Rizvi SI. Amylase inhibitory and metal chelating effects of different layers of onion (Allium Cepa L.) at two different stages of maturation in vitro. Ann Phytomed. (2017) VI:45–50. doi: 10.21276/ap.2017.6.1.6
36. Lesjak M, Beara I, Simin N, Pintac D, Majkic T, Bekvalac K, et al. Antioxidant and anti-inflammatory activities of quercetin and its derivatives. J Funct Foods. (2018) 40:68–75. doi: 10.1016/j.jff.2017.10.047
37. Viera VB, Piovesan N, Rodrigues JB, Mello RO, Prestes RC, dos Santos RC, et al. Extraction of phenolic compounds and evaluation of the antioxidant and antimicrobial capacity of red onion skin (Allium cepa L.). Int Food Res J. (2017) 24:990–9.
38. Kumara K, Srivastava S, Sharanagatb VS. Ultrasound assisted extraction (UAE) of bioactive compounds from fruit and vegetable processing by-products: a review. UltrasonSonochem. (2021) 70:105325. doi: 10.1016/j.ultsonch.2020.105325
39. Bagade SB, Patil M. Recent advances in microwave assisted extraction of bioactive compounds from complex herbal samples: a review. Crit Rev Anal Chem. (2021) 51:138–49. doi: 10.1080/10408347.2019.1686966
40. Zhang C, Li X, Zhan Z, Cao L, Zeng A, Chang G, et al. Transcriptome sequencing and metabolism analysis reveals the role of cyanidin metabolism in dark-red onion (Allium cepa L) bulbs. Sci Rep. (2018) 8:14109. doi: 10.1038/s41598-018-32472-5
41. Martin J, Asuero AG. High hydrostatic pressure for recovery of anthocyanins: effects, performance, and applications. Sep Purif Rev. (2021) 50:159–76. doi: 10.1080/15422119.2019.1632897
42. Ranjha MMAN, Kanwal R, Shafique B, Arshad RN, Irfan S, Kieliszek M, et al. A critical review on pulsed electric field: a novel technology for the extraction of phytoconstituents. Molecules. (2021) 26:4893. doi: 10.3390/molecules26164893
43. Ongkowijoyo P, Luna-Vital D, Gonzalez A, de Mejia E. Extraction techniques and analysis of anthocyanins from food sources by mass spectrometry: an update. Food Chem. (2018) 250:113–26. doi: 10.1016/j.foodchem.2018.01.055
44. Sidhu JS, Ali M, Al-Rashdan A, Ahmed N. Onion (Allium cepa L.) is potentially a good source of important antioxidants. J Food Sci Technol. (2019) 56:1811–9. doi: 10.1007/s13197-019-03625-9
45. Kumar M, Radha H, Devi S, Prakash S, Rathore M, Thakur S, et al. Ethnomedicinal plants used in the health care system: survey of the mid hills of Solan District, Himachal Pradesh, India. Plants. (2021) 10:1842. doi: 10.3390/plants10091842
46. Putnik P, Gabri D, Roohinejad S, Barba FJ, Granato D, Mallikarjunan K, et al. An overview of organosulfur compounds from Allium spp.: from processing and preservation to evaluation of their bioavailability. Antimicrobial, and anti-inflammatory properties. Food Chem. (2019) 276:680–91. doi: 10.1016/j.foodchem.2018.10.068
47. Sagar NA, Pareek S, Gonzalez-Aguilar GA. Quantification of flavonoids, total phenols and antioxidant properties of onion skin: a comparative study of fifteen Indian cultivars. J Food Sci Technol. (2020) 57:2423–32. doi: 10.1007/s13197-020-04277-w
48. Sagar NA, Pareek S. Fortification of multigrain flour with onion skin powder as a natural preservative: effect on quality and shelf life of the bread. Food Chem. (2021) 41:100992. doi: 10.1016/j.fbio.2021.100992
49. Yin DD, Yuan RY, Wu Q, Li SS, Shao S, Xu YJ, et al. Assessment of flavonoids and volatile compounds in tea infusions of water lily flowers and their antioxidant activities. Food Chem. (2015) 187:20–8. doi: 10.1016/j.foodchem.2015.04.032
50. Lin S, Zhang P, Deng Y, Xu A, Lu S, Wu C, et al. Quantification and analysis of anthocyanin and flavonoids compositions, and antioxidant activities in onions with three different colors. Int J Integr Agric. (2016) 9:2175–81. doi: 10.1016/S2095-3119(16)61385-0
51. Prakash P, Radha M, Kumar A, Pundir S, Puri S, Prakash N, et al. Documentation of commonly used ethnoveterinary medicines from wild plants of the high mountains in Shimla District, Himachal Pradesh, India. Horticulturae. (2021) 7:351. doi: 10.3390/horticulturae7100351
52. Khoo HE, Azlan A, Tang ST, Lim SM. Anthocyanidins and anthocyanins: colored pigments as food, pharmaceutical ingredients, and the potential health benefits. Food Nutr Res. (2017) 61:1361779. doi: 10.1080/16546628.2017.1361779
53. Collings DA. Anthocyanin in the vacuole of red onion epidermal cells quenches other fluorescent molecules. Plants. (2019) 8:120596. doi: 10.3390/plants8120596
54. Ren F, Zhou S. Phenolic components and health beneficial properties of onions. Agriculture. (2021) 11:90872. doi: 10.3390/agriculture11090872
55. Vijayalakshmi G, Raja M, Naik ML, Carbone V, Russo GL, Sha Valli Khan PS, et al. Determination of antioxidant capacity and flavonoid composition of onion (Allium cepa L.) landrace ‘Krishnapuram' bulb using HPLC-ESI-ITMS. J Biosci. (2021) 46:58. doi: 10.1007/s12038-021-00183-1
56. Perez-Gregorio MR, Garcia-Falcon MS, Simal-Gandara J, Rodrigues AS, Almeida DPF. Identification and quantification of flavonoids in traditional cultivars of red and white onions at harvest. J Food Compos Anal. (2010) 23:592–8. doi: 10.1016/j.jfca.2009.08.013
57. Tedesco I, Carbone V, Spagnuolo C, Minasi P, Russo GL. Identification and quantification of flavonoids from two southern Italian cultivars of Allium cepa L. tropea (red onion) and montoro (copper onion), and their capacity to protect human erythrocytes from oxidative stress. J Agric Food Chem. (2015) 63:5229–38. doi: 10.1021/acs.jafc.5b01206
58. Fredotovic Z, Sprung M, Soldo B, Ljubenkov I, Budic-Leto I, Bilusic T, et al. Chemical composition and biological activity of Allium cepa L. and Allium × cornutum (Clementi ex Visiani 1842) methanolicextracts. Molecules. (2017) 22:448. doi: 10.3390/molecules22030448
59. Ren S, Jimenez-Flores R, Giusti MM. The interactions between anthocyanin and whey protein: a review. Compr Rev Food Sci Food Saf. (2021) 20:5992–6011. doi: 10.1111/1541-4337.12854
60. Jeya Krithika S, Sathiyasree B, Beniz Theodore E, Chithiraikannu R, Gurushankar K. Optimization of extraction parameters and stabilization of anthocyanin from onion peel. Crit Rev Food Sci Nutr. 62:2560–7. doi: 10.1080/10408398.2020.1856772
61. Oancea S, Radu M, Olosutean H. Development of ultrasonic extracts with strong antioxidant properties from red onion wastes. Rom Biotechnol Lett. (2020) 25:1320–7. doi: 10.25083/rbl/25.2/1320.1327
62. Gonzalez-de-Peredo AV, Vazquez-Espinosa M, Espada-Bellido E, Ferreiro-Gonzalez M, Carrera C, Barbero GF, et al. Development of optimized ultrasound-assisted extraction methods for the recovery of total phenolic compounds and anthocyanins from onion bulbs. Antioxidants. (2021) 2021:antiox10111755. doi: 10.3390/antiox10111755
63. Park MJ, Ryu DH, Cho JY, Ha IJ, Moon JS, Kang YH, et al. Comparison of the antioxidant properties and flavonols in various parts of Korean red onions by multivariate data analysis. Hortic Environ Biotechnol. (2018) 59:919–27. doi: 10.1007/s13580-018-0091-2
64. Zhang QW, Lin LG, Ye WC. Techniques for extraction and isolation of natural products: a comprehensive review. Chin Med. (2018) 13:20. doi: 10.1186/s13020-018-0177-x
65. Wu X, Prior RL. Identification and characterization of anthocyaninsby high-performance liquid chromatography-electrosprayionization-tandem mass spectrometry in common foods in the United States: Vegetables, nuts, and grains. J Agric Food Chem. (2005) 53:3101–13. doi: 10.1021/jf0478861
66. Duangjit J, Welsh K, Wise ML, Bohanec B, Havey MJ. Genetic analyses of anthocyanin concentrations and intensity of red bulb color among segregating haploid progenies of onion. Mol Breed. (2014) 34:75–85. doi: 10.1007/s11032-014-0018-2
67. Khandagale K, Gawande S. Genetics of bulb colour variation and flavonoids in onion. In J Hortic Sci Biotechnol. (2019) 4:522–32. doi: 10.1080/14620316.2018.1543558
68. Bystricka J, Kavalcova P, Musilova J, Tomas J, Toth T, Orsak M, et al. Selenium and its influence on the content of polyphenol compounds in onion (Allium cepa L.). J Microbiol Biotechnol Food Sci. (2015) 4:23–6. doi: 10.15414/jmbfs.2015.4.special3.23-26
69. Schwinn KE, Ngo H, Kenel F, Brummell DA, Albert NW, McCallum JA, et al. The Onion (Allium cepa L.) R2R3-MYB gene MYB1 regulates anthocyanin biosynthesis. Front Plant Sci. 7:1865. doi: 10.3389/fpls.2016.01865
70. Enaru B, Dreţcanu G, Pop TD, Stanila A, Diaconeasa Z. Anthocyanins: factors affecting their stability and degradation. Antioxidants. (2021) 10:121967. doi: 10.3390/antiox10121967
71. Arruda HS, Silva EK, Peixoto Araujo NM, Pereira GA, Pastore GM, Marostica Junior MR, et al. Anthocyanins recovered from agri-food by-products using innovative processes: trends, challenges, and perspectives for their application in food systems. Molecules. (2021) 9:2632. doi: 10.3390/molecules26092632
72. Alappat B, Alappat J. Anthocyanin pigments: beyond aesthetics. Molecules. (2020) 25:5500. doi: 10.3390/molecules25235500
73. Saha S, Singh J, Paul A, Sarkar R, Khan Z, Banerjee K, et al. Anthocyanin profiling using UV-Vis spectroscopy and liquid chromatography mass spectrometry. J AOAC Int. (2020) 103:23–39. doi: 10.5740/jaoacint.19-0201
74. Kang HJ, Ko MJ, Chung MS. Anthocyanin structure and pH dependent extraction characteristics from blueberries (Vaccinium corymbosum) and chokeberries (Aronia melanocarpa) in subcritical water state. Foods. (2021) 10:527. doi: 10.3390/foods10030527
75. Castaneda-Ovando A, Pacheco-Hernandez MDL, Paez-Hernandez ME, Rodriguez JA, Galan-Vidal CA. Chemical studies of anthocyanins: a review. Food Chem. (2009) 113:859–71. doi: 10.1016/j.foodchem.2008.09.001
76. Pina F, Melo MJ, Laia CAT, Parola AJ, Lima JC. Chemistry and applications of flavylium compounds: a handful of colours. Chem Soc Rev. (2011) 41:869–908. doi: 10.1039/C1CS15126F
77. Cortez R, Luna-Vital DA, Margulis D, Gonzalez de Mejia E. Natural pigments: Stabilization methods of anthocyanins for food applications. Compr Rev Food Sci Food Saf. (2017) 16:180–98. doi: 10.1111/1541-4337.12244
78. Dangles O, Fenger JA. The chemical reactivity of anthocyanins and its consequences in food science and nutrition. Molecules. (2018) 23:1970. doi: 10.3390/molecules23081970
79. Sasaki N, Nakayama T. Achievements and perspectives in biochemistry concerning anthocyanin modification for blue flower coloration. Plant Cell Physiol. (2015) 56:pcu097. doi: 10.1093/pcp/pcu097
80. Gull A, Sheikh MA, Kour J, Zehra B, Zargar I, Wani A, et al. Anthocyanins. CRC press (2022). doi: 10.1016/B978-0-323-89779-2.00018-1
81. Ramalingam R, Palmurugan S, Shanmugapriya D. Effect of temperature, light, ph on the stability of anthocyanin pigments in Cocculus Hirsutus fruits. Int J Multidiscip Res Mod Educ. (2016) 2:91–96. doi: 10.5281/zenodo.61818
82. Fleschhut J, Kratzer F, Rechkemmer G, Kulling SE. Stability and biotransformation of various dietary anthocyanins in vitro. Eur J Nutr. (2006) 45:7–18. doi: 10.1007/s00394-005-0557-8
83. Weber F, Larsen LR. Influence of fruit juice processing on anthocyanin stability. Food Res Int. (2017) 100:354–65. doi: 10.1016/j.foodres.2017.06.033
84. Fei P, Zeng F, Zheng S, Chen Q, Hu Y, Cai J, et al. Acylation of blueberry anthocyanins with maleic acid: improvement of the stability and its application potential in intelligent color indicator packing materials. Dye Pigment. (2020) 184:108852. doi: 10.1016/j.dyepig.2020.108852
85. Rodriguez-Amaya DB. Update on natural food pigments - a mini-review on carotenoids, anthocyanins, and betalains. Int Food Res J. (2019) 124:200–5. doi: 10.1016/j.foodres.2018.05.028
86. Liu Y, Tikunov Y, Schouten RE, Marcelis LFM, Visser RGF, Bovy A. Anthocyanin biosynthesis and degradation mechanisms in Solanaceous vegetables: a review. Front Chem. (2018) 6:52. doi: 10.3389/fchem.2018.00052
87. Marszałek K, Wozniak Ł, Kruszewski B, Skapska S. The effect of high pressure techniques on the stability of anthocyanins in fruit and vegetables. Int J Mol Sci. (2017) 18:20277. doi: 10.3390/ijms18020277
88. Danişman G, Arslan E, Toklucu AK. Kinetic analysis of anthocyanin degradation and polymeric colour formation in grape juice during heating. Czech J Food Sci. (2015) 33:103–8. doi: 10.17221/446/2014-CJFS
89. Kammerer DR. 3-Anthocyanins. In: R Carle and B Schweiggert, editors, Wood head Publishing Series in Food Science, Technology and Nutrition. Sawston: Wood Head Publishing (2016). p. 61–80.
90. Belwal T, Pandey A, Bhatt ID, Rawal RS. Optimized microwave assisted extraction (MAE) of alkaloids and polyphenols from Berberis roots using multiple-component analysis. Sci Rep. (2020) 10:917. doi: 10.1038/s41598-020-57585-8
91. Aprodu I, Milea SA, Enachi E, Rapeanu G, Bahrim G, Stanciuc N, et al. Thermal degradation kinetics of anthocyanins extracted from purple maize flour extract and the effect of heating on selected biological functionality. Foods. (2020) 9:1593. doi: 10.3390/foods9111593
92. Laleh GH, Frydoonfar H, Heidary R, Jameei R, Zare S. The effect of light, temperature, pH and species on stability of anthocyanin pigments in four Berberis species. Pak J Nutr. (2005) 5:90–2. doi: 10.3923/pjn.2006.90.92
93. Turker N, Aksay S, Ekiz HI. Effect of storage temperature on the stability of anthocyanins of a fermented black carrot (Daucus carota var. L) Beverage: Shalgam. J Agric Food Chem. (2004) 52:3807–13. doi: 10.1021/jf049863s
94. Deylami MZ, Rahman RA, Tan CP, Bakar J, Olusegun L. Effect of blanching on enzyme activity, color changes, anthocyanin stability and extractability of mangosteen pericarp: a kinetic study. J Food Eng. (2016) 178:12–9. doi: 10.1016/j.jfoodeng.2016.01.001
95. He J. Isolation of Anthocyanin Mixtures from Fruits and Vegetables and Evaluation of Their Stability, Availability and Biotransformation in the Gastrointestinal Tract, (Ph.D. Thesis), The Ohio State University, Columbus, OH, United States (2008).
96. Rein M. Copigmentation Reactions and Color Stability of Berry Anthocyanins, (Ph.D. Dissertation), University of Helsinki, Helsinki, Finland (2005). p. 87.
97. Sipahli S, Mohanlall V, Mellem J. Stability and degradation kinetics of crude anthocyanin extracts from H. sabdariffa. Food Sci Technol. (2017) 37:209–15. doi: 10.1590/1678-457x.14216
98. Navas MJ, Jimenez-Moreno AM, Martin Bueno J, Saez-Plaza P, Asuero AG. Analysis and antioxidant capacity of anthocyanin pigments. Part IV: extraction of anthocyanins. Crit Rev Anal Chem. (2012) 42:313–42. doi: 10.1080/10408347.2012.680343
99. Yang W, Guo Y, Liu M, Chen X, Xiao X, Wang S, et al. Structure and function of blueberry anthocyanins: a review of recent advances. J Funct Foods. (2022) 88:104864. doi: 10.1016/j.jff.2021.104864
100. Azmir J, Zaidul ISM, Rahman MM, Sharif KM, Mohamed A, Sahena F, et al. Techniques for extraction of bioactive compounds from plant materials: a review. J Food Eng. (2013) 117:426–36. doi: 10.1016/j.jfoodeng.2013.01.014
101. Xi J. Ultrahigh pressure extraction of bioactive compounds from plants- a review. Crit Rev Food Sci Nutr. (2017) 57:1097–106. doi: 10.1080/10408398.2013.874327
102. Kerio LC, Wachira FN, Wanyoko JK, Rotich MK. Characterization of anthocyanins in Kenyan teas: extraction and identification. Food Chem. (2012) 131:31–8. doi: 10.1016/j.foodchem.2011.08.005
103. Karaaslan NM, Yaman M. Determination of anthocyanins in cherry cranberry by high-performance liquid chromatography-electrospray ionization-mass spectrometry. Eur Food Res Technol. (2016) 242:127–35. doi: 10.1007/s00217-015-2524-9
104. Nankar AN, Dungan B, Paz N, Sudasinghe N, Schaub T, Holguin FO, et al. Quantitative and qualitative evaluation of kernel anthocyanins from southwestern United States blue corn. J Sci Food Agri. (2016) 96:4542–52. doi: 10.1002/jsfa.7671
105. Garcia-Mendoza MP, Espinosa-Pardo FA, Baseggio AM, Barbero GF, Junior MRM, Rostagno MA, et al. Extraction of phenolic compounds and anthocyanins from jucara (Euterpe edulis Mart.) residues using pressurized liquids and supercritical liquids. J Supercrit Fluids. (2017) 119:9–16. doi: 10.1016/j.supflu.2016.08.014
106. Chandrasekhar J, Madhusudhan MC, Raghavarao KSMS. Extraction of anthocyanins from red cabbage and purification using adsorption. Food Bioproducts Processing. (2012) 90:615–23. doi: 10.1016/j.fbp.2012.07.004
107. Musso YS, Salgado PR, Mauri AN. Smart gelatin films prepared using red cabbage (Brassica oleracea L.) extracts as solvent. Food Hydrocolloids. (2019) 89:674–81. doi: 10.1016/j.foodhyd.2018.11.036
108. Galvao AC, Souza PP, Robazza WS, França CAL. Capacity of solutions involving organic acids in the extraction of the anthocyanins present in jabuticaba skins (Myrciariacauliflora) and red cabbage leaves (Brassica oleracea). J Food Sci Technol. (2020) 57:3995–4002. doi: 10.1007/s13197-020-04430-5
109. Valencia-Arredondo JA, Hernandez-Bolio GI, Ceron-Montes GI, Castro-Munoz R, Yanez-Fernandez J. Enhanced process integration for the extraction, concentration and purification of di-acylated cyanidin from red cabbage. Separation Purification Technol. (2020) 238:116492. doi: 10.1016/j.seppur.2019.116492
110. Albuquerque BR, Pinela J, Barros L, Oliveira MBPP, Ferreira ICFR. Anthocyanin-rich extract of jabuticaba epicarp as a natural colorant: Optimization of heat- and ultrasound-assisted extractions and application in a bakery product. Food Chem. (2020) 316:126364. doi: 10.1016/j.foodchem.2020.126364
111. Backes E, Pereira C, Barros L, Prieto MA, Genena AK, Barreiro MF, et al. ICFR Recovery of bioactive anthocyanin pigments from Ficus carica L. peel by heat. Microwave, and ultrasound based extraction techniques. Food Res Int. (2018) 113:197–209. doi: 10.1016/j.foodres.2018.07.016
112. Belwal T, Ezzat SM, Rastrelli LI, Bhatt D, Daglia M, Baldi A, et al. A critical analysis of extraction techniques used for botanicals: trends, priorities, industrial uses and optimization strategies. Trends Anal Chem. (2018) 100:82–102. doi: 10.1016/j.trac.2017.12.018
113. Xu DP, Li Y, Meng X, Zhou T, Zhou Y, Zheng J, et al. Natural antioxidants in foods and medicinal plants: extraction, assessment and resources. Int J Mol Sci. (2017) 18:96. doi: 10.3390/ijms18010096
114. Ardestani SB, Sahari MA, Barzegar M. Effect of extraction and processing conditions on organic acids of barberry fruits. J Food Biochem. (2015) 39:554–65. doi: 10.1111/jfbc.12158
115. Xue H, Xu H, Wang X, Shen L, Liu H, Liu C, et al. Effects of microwave power on extraction kinetic of anthocyanin from blueberry powder considering absorption of microwave energy. J Food Qual. (2018) 2018:9680184. doi: 10.1155/2018/9680184
116. Sun Y, Xue HK, Liu CH, Liu C, Su XL, Zheng XZ, et al. Comparison of microwave assisted extraction with hot reflux extraction in acquirement and degradation of anthocyanin from powdered blueberry. Int J Agric Biol. (2016) 9:186–99. doi: 10.3965/j.ijabe.20160906.2724
117. Xue H, Tan J, Fan L, Li Q, Cai XJ. Optimization microwave-assisted extraction of anthocyanins from cranberry using response surface methodology coupled with genetic algorithm and kinetics modelanalisis. J Food Process Eng. (2021) 44:13688. doi: 10.1111/jfpe.13688
118. Netramai S, Kijchavengkul T, Samsudin H, Lertsiri S. Enhanced extraction of anthocyanins from red cabbage (Brassica oleraces) using microwave assisted extraction. In: Paper Presented at the 21st Food Innovation Asia Conference. Bangkok (2019).
119. Nguyen H, Le Ngoc T, PhanThiKieu L, Tran Thanh T, Mai Huynh C. Bioactive compounds from red cabbage by microwave-assisted extraction: anthocyanins, total phenolic compounds and the antioxidant activity. Asian Life Sci. (2020) 12:172–84. Available online at: https://innovareacademics.in/journals/index.php/ijpps/article/view/6645
120. Farzaneh V, Carvalho IS. Modelling of microwave assisted extraction (MAE) of anthocyanins (TMA). J Appl Res Med Aromat Plants. (2017) 6:92–100. doi: 10.1016/j.jarmap.2017.02.005
121. Morata A, Escott C, Loira I, Lopez C, Palomero F, Gonzalez C, et al. Emerging non-thermal technologies for the extraction of grape anthocyanins. Antioxidants. (2021) 10:1863. doi: 10.3390/antiox10121863
122. Sang J, Ma Q, Li B, Li CQ. An approach for extraction, purification, characterization and quantitation of acylated anthocyanins from Nitrariatangutorun Bobr. Fruit. J Food Meas Charact. (2018) 12:45–55. doi: 10.1007/s11694-017-9615-1
123. Carrera C, Aliano-Gonzalez MJ, Valaityte M, Ferreiro-Gonzalez M, Barbero GF, Palma M, et al. A novel ultrasound-assisted extraction method for the analysis of anthocyanins in potatoes (Solanum tuberosum L). Antioxidants. (2021) 10:1375. doi: 10.3390/antiox10091375
124. Ravanfar R, Tamadon AM, Niakousari M. Optimization of ultrasound assisted extraction of anthocyanins from red cabbage using Taguchi design method. J Food Sci Technol. (2015) 52:8140–7. doi: 10.1007/s13197-015-1880-6
125. Agcam E, Akyildiz A, Balasubramaniam VM. Optimization of anthocyanins extraction from black carrot pomace with thermosonication. Food Chem. (2017) 237:461–70. doi: 10.1016/j.foodchem.2017.05.098
126. Espada-Bellido E, Ferreiro-González M, Carrera C, Palma M, Barroso CG, Barbero GF. Optimization of the ultrasound assisted extraction of anthocyanins and total phenolic compounds in mulberry (Morusnigra) pulp. Food Chem. (2017) 219:23–32. doi: 10.1016/j.foodchem.2016.09.122
127. Demirdoven A, Ozdogan K, Erdogan-Tokatli K. Extraction of anthocyanins from red cabbage by ultrasonic and conventional methods: optimization and evaluation. J Food Biochem. (2015) 39:491–500. doi: 10.1111/jfbc.12153
128. Ghavidel RA, Sheikholeslami Z, Ahmadi S. Optimization of extraction the red cabbage extract with ultrasound technology, assisted by response surface method. Int J Biosci. (2015) 6:94–100. doi: 10.12692/ijb/6.3.94-100
129. Zhu Z, Guan Q, Kouba M, Barba FJ, Roohineja S, Cravotto G, et al. HPLC-DAD-ESI-MS2 analytical profile of extracts obtained from purple sweet potato after green ultrasound-assisted extraction. Food Chem. (2017) 215:391–400. doi: 10.1016/j.foodchem.2016.07.157
130. Li S, Lei D, Zhu Z, Cai J, Manzoli M, Jicsinszky L, et al. Complexation of maltodextrin-based inulin and green tea polyphenols via different ultrasonic pretreatment Ultrasonics Sonochem. (2021) 74:105568. doi: 10.1016/j.ultsonch.2021.105568
131. Ravanfar R, Moein M, Niakousari M, Tamaddon A. Extraction and fractionation of anthocyanins from red cabbage: ultrasonic-assisted extraction and conventional percolation method. J Food Measur Characterization. (2018) 12:2271. doi: 10.1007/s11694-018-9844-y
132. Cai Z, Qu Z, Lan Y, Zhao S, Ma X, Wan Q, et al. Ultrasound-assisted, and accelerated-solvent extractions of anthocyanins from purple sweet potatoes. Food Chem. (2016) 197:266–72. doi: 10.1016/j.foodchem.2015.10.110
133. Sousa Sabino LBd, AlvesFilho EG, Fernandes FAN, de Brito ES, da Silva Junior IJ. Optimization of pressurized liquid extraction and ultrasound methods for recovery of anthocyanins present in jambolan fruit (Syzygiumcumini L.). Food Bioprod Process. (2021) 127:77–89. doi: 10.1016/j.fbp.2021.02.012
134. Sharifi A, Mortazav SA, Maskooki A, Niakousari M, Elhamirad AH. Optimization of subcritical water extraction of bioactive compounds from barberry fruit (Berberis vulgaris) by using response surface methodology. Int J Agric Crop Sci. (2013) 6:89–96. doi: 10.22101/jrifst.2014.06.15.312
135. Teixeira RF, Benvenutti L, Burin VM, Gomes TM, Ferreira SRS, Ferreira-Zielinski AA, et al. An eco-friendly pressure liquid extraction method to recover anthocyanins from broken black bean hulls. Innov Food Sci Emerg Technol. (2021) 67:102587. doi: 10.1016/j.ifset.2020.102587
136. Wang Y, Ye Y, Wang L, Yin W, Liang J. Antioxidant activity and subcritical water extraction of anthocyanin from raspberry process optimization by response surface methodology. Food Chem. (2021) 44:101394. doi: 10.1016/j.fbio.2021.101394
137. Fernandes L, Casal SIP, Pereira JA, Ramalhosa E, Saraiva JA. Optimization of high pressure bioactive compounds extraction from pansies (Viola wittrockiana) by response surface methodology. High Press Res. (2017) 37:415–29. doi: 10.1080/08957959.2017.1347925
138. Briones-Labarca V, Giovagnoli-Vicuna C, Chacana-Ojeda M. High pressure extraction increases the antioxidant potential and in vitro bio-accessibility of bioactive compounds from discarded blueberries. CYTA J Food. (2019) 17:622–63. doi: 10.1080/19476337.2019.1624622
139. Arapitsas P, Turner C. Pressurized solvent extraction and monolithic column- HPLC/DAD analysis of anthocyanins in red cabbage. Talanta. (2008) 74:1218–23. doi: 10.1016/j.talanta.2007.08.029
140. Pereira DTV, Tarone AG, Cazarin CBB, Barbero GF, Martínez JJ. Pressurized liquid extraction of bioactive compounds from grape marc. J Food Eng. (2019) 240:105–13. doi: 10.1016/j.jfoodeng.2018.07.019
141. Ngamwonglumlert L, Devahastin S, Chiewchan N. Natural colorants: pigment stability and extraction yield enhancement via utilization of appropriate pretreatment and extraction methods. Crit Rev Food Sci Nutr. (2017) 57:3243–59. doi: 10.1080/10408398.2015.1109498
142. Fincan M. Potential application of pulsed electric fields for improving extraction of plant pigments. In Handb Electropozration, Miklavcic, D, Ed, Springer: Cham, Germany, Chapter. (2017) 9:2171–92. doi: 10.1007/978-3-319-32886-7_34
143. Zderic A, Zondervan E. Polyphenol extraction from fresh tea leaves by pulsed electric field: a study of mechanisms. Chem Eng Res Des. (2016) 109:586–92. doi: 10.1016/j.cherd.2016.03.010
144. Buchmann L, Mathys A. Perspective on pulsed electric field treatment in the bio-based industry. Front Bioeng Biotechnol. (2019) 7:265. doi: 10.3389/fbioe.2019.00265
145. Gagneten M, Leiva G, Salvatori D, Schebor C, Olaiz N. Optimization of pulsed electric field treatment for the extraction of bioactive compounds from blackcurrant. Food Bioproc Tech. (2019) 12:1102–9. doi: 10.1007/s11947-019-02283-1
146. Loncaric A, Celeiro M, Jozinovic A, Jelinic J, Kovac T, Jokic S, et al. Green extraction methods for extraction of polyphenolic compounds from blueberry pomace. Foods9. (2020) 1521. doi: 10.3390/foods9111521
147. Lamanauskas N, Bobinait R, Satkauskas S, Viskelis P, Pataro G, Ferrari G, et al. Pulsed electric field-assisted juice extraction of frozen/thawed blueberries. Zemdirbyste. (2015) 102:59–66. doi: 10.13080/z-a.2015.102.007
148. Taiebirad F, Bakhshabadi H, Rashidzadeh SH. Optimization of extraction of bioactive compounds from seedless barberry fruit using pulsed electric field pretreatment. Iran J Sci Technol Trans A Sci. (2021) 18:305–17. doi: 10.52547/fsct.18.114.305
149. Gachovska T, Cassada D, Subbiah J, Hanna M, Thippareddi H, Snow D, et al. Enhanced anthocyanin extraction from red cabbage using pulsed electric field processing. J Food Sci. (2010) 75:323–9. doi: 10.1111/j.1750-3841.2010.01699.x
150. Meini MR, Cabezudo I, Boschetti CE, Romanini D. Recovery of phenolic antioxidants from Syrah grape pomace through the optimization of an enzymatic extraction process. Food Chem. (2019) 283:257–64. doi: 10.1016/j.foodchem.2019.01.037
151. Lotfi L, Kalbasi-Ashtari A, Hamedi M, Ghorbani F. Effects of enzymatic extraction on anthocyanins yield of saffron tepals (Crocos sativus) along with its color properties and structural stability. J Food Drug Anal. (2015) 23:210–8. doi: 10.1016/j.jfda.2014.10.011
152. Binaschi M, Duserm Garrido G, Cirelli C, Spigno G. Biotechnological strategies to valorise grape pomace for food applications. Chem Eng Trans. (2018) 64:367–72. doi: 10.3303/CET1864062
153. Vardakas AT, Shikov VT, Dinkova RH, Mihalev KM. Optimisation of the enzyme-assisted extraction of polyphenols from saffron (Crocus sativus L.) tepals. Acta Sci Pol Technol Aliment. (2021) 20:359–67. doi: 10.17306/J.AFS.2021.0954
154. Serea D, Rapeanu G, Constantin OE, Bahrim GE, Stanciuc N, Croitoru C. Ultrasound and enzymatic assisted extractions of bioactive compounds found in red grape skins babeascaneagra (Vitis vinifera) variety. Ann Univ Dunarea Jos Galati Fascicle VI. (2021) 45:9–25. doi: 10.35219/foodtechnology.2021.1.01
155. Zhua Z, Li S, He J, Thirumdas R, Montesano D, Barba FJ, et al. Enzyme-assisted extraction of polyphenol from edible lotus (Nelumbo nucifera) rhizome knot: Ultra-filtration performance and HPLC-MS2 profile. Food Res Int. (2018) 111:291–8. doi: 10.1016/j.foodres.2018.05.047
156. Li Y, Tao F, Wang Y, Cui K, Cao J, Cui C, et al. Process optimization for enzymatic assistedextraction of anthocyanins from the mulberry wine residue. IOP Conf Series Earth Environ Sci. (2020) 559:012011. doi: 10.1088/1755-1315/559/1/012011
157. Pazir F, Kocak E, Turan F, Ova G. Extraction of anthocyanins from grape pomace by using supercritical carbon dioxide. J Food Process Preserv. (2021) 45:14950. doi: 10.1111/jfpp.14950
158. Idham Z, Nasir HM, Yunus MAC, Yian LN, Peng WL, Hassan H, et al. Optimisation of supercritical CO2 extraction of red colour from roselle (Hibiscus Sabdariffa Linn.) calyces. Chem Eng Trans. (2017) 56:871–6. doi: 10.3303/CET1756146
159. Babova O, Occhipinti A, Capuzzo A, Maffei ME. Extraction of bilberry (Vaccinium myrtillus) antioxidants using supercritical / subcritical CO2 and ethanol as co-solvent. J Supercrit Fluids. (2016) 107:358–63. doi: 10.1016/j.supflu.2015.09.029
160. Lopez-Padilla A, Ruiz-Rodriguez A, Florez CER, Barrios DMR, Reglero G, Fornari T, et al. Vaccinium meridionale Swartz supercritical CO2 extraction: effect of process conditions and scaling up. Materials. (2016) 9:519. doi: 10.3390/ma9070519
161. Talmaciu AI, Volf I, Popa VI. Supercritical fluids and ultrasound assisted extractions applied to spruce bark conversion. Environ Eng Manag J. (2015) 14:615–23. doi: 10.30638/eemj.2015.068
162. Wozniak L Marszalek K Skapska S Jedrzejczak R. The application of supercritical carbon dioxide and ethanol for the extraction of phenolic compounds from chokeberry pomace. Appl Sci. 7:322. doi: 10.3390/app7040322
163. Maran JP, Priya B, Manikandan S. Modeling and optimization of supercritical fluid extraction of anthocyanin and phenolic compounds from Syzygiumcumini fruit pulp. J Food Sci Technol. (2014) 51:1938–46. doi: 10.1007/s13197-013-1237-y
164. Jiao G, Kermanshahi A. Extraction of anthocyanins from haskap berry pulp using supercritical carbon dioxide: influence of co-solvent composition and pretreatment. LWT Food Sci Technol. (2018) 98:237–44. doi: 10.1016/j.lwt.2018.08.042
165. Xu Z, Wu J, Zhang Y, Hu X, Liao X, Wang Z, et al. Extraction of anthocyanins from red cabbage using high pressure CO2. Bioresour Technol. (2010) 101:7151–7. doi: 10.1016/j.biortech.2010.04.004
166. Giusti MM, Wrolstad RE. Anthocyanins. Characterization and measurement with UV-visible spectroscopy. In: RE Wrolstad, SJ Schwartz, editors, Curr. Protoc. Food Anal. Chem. New York, NY: John Wiley & Sons (2001). p. 2.1–2.13. doi: 10.1002/0471142913.faf0102s00
167. Bridle P, Timberlake CF. Anthocyanins as natural food colours-selected aspects. Food Chem. (1997) 58:103–9. doi: 10.1016/S0308-8146(96)00222-1
168. Biolink Group [homepage on the internet]. Sandnes. NO: Biolink Group, AS. Available online at: http//www.biolink.no/products/professional-dietarysupplement-article86-111.html (accessed October 15, 2009).
169. Aura AM, Martin-Lopez P, O'Leary KA, Williamson G, Oksman-Caldentey KM, Poutanen K, et al. In vitro metabolism of anthocyanins by human gut microflora. Eur J Nut. (2005) 44:133–42. doi: 10.1007/s00394-004-0502-2
170. Talavera S, Felgines C, Texier O, Besson C, Lamaison JL, Remesy C, et al. Anthocyanins are efficiently absorbed from the stomach in anesthetized rats. J Nut. (2003) 133:4178–82. doi: 10.1093/jn/133.12.4178
171. Matsumoto H, Inaba H, Kishi M, Tominaga S, Hirayama M, Tsuda T, et al. Orally administered delphinidin-3-rutinoside and cyanidin-3- rutinoside are directly absorbed in rats and humans and appear in the blood as the intact forms. J Agric Food Chem. (2001) 49:1546–51. doi: 10.1021/jf001246q
172. Miyazawa T, Nakagawa K, Kudo M, Muraishi K, Someya K. Direct intestinal absorption of red fruit anthocyanins, cyanidin-3-glucoside and cyanidin-3-5-diglucoside, into rats and humans. J Agric Food Chem. (1999) 47:1083–91. doi: 10.1021/jf9809582
173. Krishnan V, Awana M, Samota MK, Warwate SI, Kulshreshtha A, Ray M, et al. Pullulanase activity: a novel indicator of inherent resistant starch in rice (Oryza sativa. L). Int J Biol Macromol. (2020) 152:1213–23. doi: 10.1016/j.ijbiomac.2019.10.218
174. Felgines C, Talavera S, Texier O, Gil-Izquierdo A, Lamaison JL, Remesy C, et al. Blackberry anthocyanins are mainly recovered from urine as methylated and glucuronidated conjugates in humans. J Agric Food Chem. (2005) 53:7721–7. doi: 10.1021/jf051092k
175. Kay CD, Mazza G, Holub BJ. Anthocyanins exist in the circulation primarily as metabolites in adult men. J Nutr. (2005) 135:2582–8. doi: 10.1093/jn/135.11.2582
176. Nielsen ILF, Dragsted LO, Ravn-Haren G, Freese R, Rasmussen SE. Absorption and excretion of black currant anthocyanins in humans and watanabe heritable hyperlipidemic rabbits. J Agric Food Chem. (2003) 51:2813–20. doi: 10.1021/jf025947u
177. Frank T, Netzel M, Strass G, Bitsch R, Bitsch I. Bioavailability of anthocyanidin-3-glucosides following consumption of red wine and red grape juice. Can J PhysiolPharmacol. (2003) 81:423–35. doi: 10.1139/y03-038
178. Kaur S, Thukral SK, Kaur P, Samota MK. Perturbations associated with hungry gut microbiome and postbiotic perspectives to strengthen the microbiome health. Future Food. (2021) 4:100043. doi: 10.1016/j.fufo.2021.100043
179. Huang Y, Zhou WB. Microencapsulation of anthocyanins through two-step emulsification and release characteristics during in vitro digestion. Food Chem. (2019) 278:357–63. doi: 10.1016/j.foodchem.2018.11.073
180. Fernandes A, Rocha M, Santos L, Brás J, Oliveira J, Mateus N, et al. Blackberry anthocyanins: β-Cyclodextrin fortification for thermal and gastro- intestinal stabilization. Food Chem. (2018) 245:426–31. doi: 10.1016/j.foodchem.2017.10.109
181. Wu Y, Han Y, Tao Y, Li D, Xie G, Show PL, et al. In vitro gastrointestinal digestion and fecal fermentation reveal the effect of different encapsulation materials on the release, degradation and modulation of gut microbiota of blueberry anthocyanin extract. Food Res Int. (2020) 132:109098. doi: 10.1016/j.foodres.2020.109098
182. Sharif N, Khoshnoudi-Nia S, Jafari SM. Nano/microencapsulation of anthocyanins; a systematic review and meta-analysis. Food Res Int. (2020) 132:109077. doi: 10.1016/j.foodres.2020.109077
183. Bub A, Watzl B, Heeb D, Rechkemmer G, Briviba K. Affiliations Expand. Malvidin-3-glucoside bioavailability in humans after ingestion of red wine. Dealcoholized red wine and red grape juice. Eur J Nut. (2001) 40:113–20. doi: 10.1007/s003940170011
184. Czank C, Cassidy A, Zhang Q, Morrison DJ, Preston T, Kroon PA, et al. Human metabolism and elimination of the anthocyanin, cyanidin-3-glucoside: a 13C-tracer study. Am J Clin Nut. (2013) 97:995–1003. doi: 10.3945/ajcn.112.049247
185. Pharmanex [homepage on the internet]. Provo, UT: Pharmanex, Inc. Available online at: http://www.pharmanex.com/corp/product/solutions/cholestin.shtml (accessed October 15, 2009).
186. Abdel-Aal SM, Abou-Arab A, Gamel TH, Hucl P, Young JC, Rabalski I, et al. Fractionation of blue wheat anthocyanin compounds and their contribution to antioxidant properties. J Agric Food Chem. (2008) 56:11171–7. doi: 10.1021/jf802168c
187. Kamonpatana K, Giusti MM, Chitchumroonchokchai C, Moreno Cruz M, MRiedl K, Kumar P, et al. Susceptibility of anthocyanins to ex vivo degradation in human saliva. Food Chem. (2012) 135:738–47. doi: 10.1016/j.foodchem.2012.04.110
188. Wallace TC, Blumberg JB, Johnson EJ, Shao A. Dietary bioactives: establishing a framework for recommended intakes. Adv Nut. (2015) 6:1–4. doi: 10.3945/an.114.007294
189. Sebastian RS, Wilkinson Enns C, Goldman JD, Martin CL, Steinfeldt LC, Murayi T, et al. A new database facilitates characterization of flavonoid intake, sources, and positive associations with diet quality among US adults. J Nutr. (2015) 145:1239–48. doi: 10.3945/jn.115.213025
190. Cheplick S, Kwon Y, Bhowmik P, Shetty K. Phenolic-linked variation in strawberry cultivars for potential dietary management of hyperglycemia and related complications of hypertension. Bioresour Technol. (2010) 101:404–13. doi: 10.1016/j.biortech.2009.07.068
191. Shim SH, Kim JM, Choi CY, Kim CY, Park KH. Ginkgo biloba extract and bilberry anthocyanins improve visual function in patients with normal tension glaucoma. J Med Food. (2012) 15:818–23. doi: 10.1089/jmf.2012.2241
192. Ahmad A, Kaleem M, Ahmed Z, Shafiq H. Therapeutic potential of flavonoids and their mechanism of action against microbial and viral infections-a review. Int Food Res J. (2015) 77:221–35. doi: 10.1016/j.foodres.2015.06.021
193. Wang LS, Hecht SS, Carmella SG, Yu N, Larue B, Henry C, et al. Anthocyanins in black raspberries prevent esophageal tumors in rats. Cancer Prev Res. (2009) 2:84–93. doi: 10.1158/1940-6207.CAPR-08-0155
194. Toufektsian MC, Lorgeril Md, Nagy N, Salen P, Donati MB, Giordano L, et al. Chronic dietary intake of plant-derived anthocyanins protects the rat heart against ischemia-reperfusion injury. J Nutr. (2008) 138:747–52. doi: 10.1093/jn/138.4.747
195. Jang H, Ha U-S, Kim S-J, Yoon B-I, Han D-S, Yuk S-M, et al. Anthocyanin extracted from black soybean reduces prostate weight and promotes apoptosis in the prostatic hyperplasia-induced rat model. J Agri Food Chem. (2010) 58:12686–91. doi: 10.1021/jf102688g
196. Knobloch TJ, Uhrig LK, Pearl DK, Casto BC, Warner BM, Clinton SK, et al. Suppression of proinflammatory and pro-survival biomarkers in oral cancer patients consuming a black raspberry phytochemical-rich troche. Cancer Prev Res. (2016) 9:159–71. doi: 10.1158/1940-6207.CAPR-15-0187
197. Probst Y. A review of the nutrient composition of selected Rubus berries. Nutr Food Sci. (2015) 45:242–54. doi: 10.1108/NFS-07-2014-0063
198. Skrovankova S, Sumczynski D, Mlcek J, Jurikova T, Sochor J. Bioactive compounds and antioxidant activity in different types of berries. Int J Mol Sci. (2015) 16:24673–706. doi: 10.3390/ijms161024673
199. Cho E, Chung EY, Jang HY, Hong OY, Chae HS, Jeong YJ, et al. Anti-cancer effect of cyanidin-3- glucoside from mulberry via caspase-3 cleavage and DNA fragmentation in vitro and in vivo. Anticancer Agents Med Chem. (2017) 17:1519–25. doi: 10.2174/1871520617666170327152026
200. Shi N, Riedl KM, Schwartz SK, Zhang X, Clinton SK, Chen T, et al. Efficacy comparison of lyophilised black raspberries and combination of celecoxib and PBIT in prevention of carcinogen-induced oesophageal cancer in rats. J Funct Foods. (2016) 27:11. doi: 10.1016/j.jff.2016.08.044
201. Genskowsky E, Puente LA, Perez-Alvarez JA, Fernandez-Lopez J, Munoz LA, Viuda-Martos M, et al. Determination of polyphenolic profile, antioxidant activity and antibacterial properties of maqui [Aristotelia chilensis (Molina) Stuntz] a Chilean blackberry. J Sci Food Agric. (2016) 96:4235–42. doi: 10.1002/jsfa.7628
202. Li D, Zhang Y, Liu Y, Sun R, Xia M. Purified anthocyanin supplementation reduces dyslipidemia, enhances antioxidant capacity, and prevents insulin resistance in diabetic patients. J Nutr. (2015) 145:742–8. doi: 10.3945/jn.114.205674
203. Koh ES, Lim JH, Kim MY, Chung S, Shin SJ, Choi BS, et al. Anthocyanin-rich Seoritae extract ameliorates renal lipotoxicity via activation of AMP activated protein kinase in diabetic mice. J Transl Med. (2015) 13:203. doi: 10.1186/s12967-015-0563-4
204. Wu T, Yu Z, Tang Q, Song H, Gao Z, Chen W, et al. Honeysuckle anthocyanin supplementation prevents diet-induced obesity in C57BL/6 mice. Food Funct. (2013) 4:1654–61. doi: 10.1039/c3fo60251f
205. Orqueda ME, Torres S, Zampini IC, Cattaneo F, Fernandez Di A, Pardo EM, et al. Integral use of Argentinean Solanum betaceum red fruits as functional food ingredient to prevent metabolic syndrome: effect of in vitro simulated gastroduodenal digestión. Heliyon. (2020) 6:3387. doi: 10.1016/j.heliyon.2020.e03387
206. Zhou L, Xiec M, Yanga F, Liu J. Antioxidant activity of high purity blueberry anthocyanins and the effects on human intestinal microbiota. Food Sci Technol. (2020) 117:628101. doi: 10.1016/j.lwt.2019.108621
207. Gowd V, Bao T, Chen W. Antioxidant potential and phenolic profile of blackberry anthocyanin extract followed by human gut microbiota fermentation. Int Food Res J. (2019) 120:523–33. doi: 10.1016/j.foodres.2018.11.001
208. Gowd V, Karim N, Xie L, Shishir MRI, Xu Y, Chen W, et al. In vitro study of bioaccessibility, antioxidant, and α-glucosidase inhibitory effect of pelargonidin-3-O-glucoside after interacting with beta-lactoglobulin and chitosan/pectin. Int J Biol Macromol. (2020) 154:380–9. doi: 10.1016/j.ijbiomac.2020.03.126
209. Drobiova H, Thomson M, Al-Qattan K, Shalaby RP, Al-Amin Z, Ali M, et al. Garlic increases antioxidant levels in diabetic and hypertensive rats determined by a modified peroxidase method. Evid Based Complement Altern Med. (2011) 8:703049. doi: 10.1093/ecam/nep011
210. Murayyan AI, Manohar CM, Hayward G, Neethirajan S. Antiproliferative activity of Ontario grown onions against colorectal adenocarcinoma cells. Int Food Res J. (2017) 96:12–8. doi: 10.1016/j.foodres.2017.03.017
211. Bahram-Parvar M, Lim LT. Fresh-cut onion: a review on processing, health benefits, and shelf-life. Compr Rev Food Sci Food Saf . (2018) 17:290–308. doi: 10.1111/1541-4337.12331
212. Awana M, Jain N, Samota MK, Rani K, Kumar A, Ray M, et al. Protein and gene integration analysis through proteome and transcriptome brings new insight into salt stress tolerance in pigeonpea (Cajanus cajan L.). Int J Biol Macromol. (2020) 164:3589–602. doi: 10.1016/j.ijbiomac.2020.08.223
213. AbouZid S, Ahmed H. Brief review on applications of continuous-wave electron paramagnetic resonance spectroscopy in natural product free radical research. In Stud Nat Prod Chem. (2020) 66:355–69. doi: 10.1016/B978-0-12-817907-9.00013-1
214. Radha M, Kumar S, Puri A, Pundir SP, Bangar S, Changan P, et al. Evaluation of nutritional, phytochemical, and mineral composition of selected medicinal plants for therapeutic uses from cold desert of Western Himalaya. Plants. (2021) 10:1429. doi: 10.3390/plants10071429
215. Wang W, Li Y, Dang P, Zhao S, Lai D, Zhou L, et al. Rice secondary metabolites: structures, roles, biosynthesis, and metabolic regulation. Molecules. (2018) 23:3098. doi: 10.3390/molecules23123098
216. Farre G, Blancquaert D, Capell T, Van Der Straeten D, Christou P, Zhu C, et al. Engineering complex metabolic pathways in plants. Annu Rev Plant Biol. (2014) 65:187–223. doi: 10.1146/annurev-arplant-050213-035825
217. Riggi E, Avola G, Siracusa L, Ruberto G. Flavonol content and biometrical traits as a tool for the characterization of Cipolla di Giarratana: a traditional Sicilian onion landrace. Food Chem. (2013) 140:810–6. doi: 10.1016/j.foodchem.2012.10.134
218. Petropoulos SA, Fernandes A, Barros L, Ferreira ICFR, Ntatsi G. Morphological nutritional and chemical description of Vatikiotiko an onion local landrace from Greece. Food Chem. (2015) 182:156–63. doi: 10.1016/j.foodchem.2015.03.002
219. Liguori L, Califano R, Albanese D, Raimo F, Matteo MD. Chemical composition and antioxidant properties of five white onion (Allium cepa L.) landraces. J Food Qual. (2017) 1:9. doi: 10.1155/2017/6873651
220. Kay CD, Pereira-Caro G, Ludwig IA, Clifford MN, Crozier A. Anthocyanins and flavanones are more bioavailable than previously perceived: a review of recent evidence. Annu Rev Food Sci Technol. (2017) 8:155–80. doi: 10.1146/annurev-food-030216-025636
221. Lila MA, Burton-Freeman B, Grace M, Kalt W. Unraveling anthocyanin bioavailability for human health. Annu Rev Food Sci Technol. (2016) 7:375–93. doi: 10.1146/annurev-food-041715-033346
222. Fang J. Bioavailability of anthocyanins. Drug Metab Rev. (2014) 46:508–20. doi: 10.3109/03602532.2014.978080
223. Lingua MS, Fabani MP, Wunderlin DA, Baroni MV. From grape to wine: changes in phenolic composition and its influence on antioxidant activity. Food Chem. (2016) 208:228–38. doi: 10.1016/j.foodchem.2016.04.009
224. Martin J, Kuskoski EM, Navas MJ, Asuero AG. Antioxidant capacity of anthocyanin pigments. In Flavonoids-From Biosynthesis to Human Health, Justino, J, Ed, Science, Technology and Medicine Open Access Publisher: Rijeka, Croatia, Chapter. (2017) 11:205–55. doi: 10.5772/67718
225. Aprile A, Negro C, Sabella E, Luvisi A, Nicoli F, Nutricati E, et al. Antioxidant activity and anthocyanin contents in olives (cv cellina di nardò) during ripening and after fermentation. Antioxidants. (2019) 8:138. doi: 10.3390/antiox8050138
226. Kim I, Lee J. Variations in anthocyanin profiles and antioxidant activity of 12 genotypes of mulberry (Morus spp.) fruits and their changes during processing. Antioxidants. (2020) 9:242. doi: 10.3390/antiox9030242
227. Knez Hrncic M, Spaninger E, Kosir IJ, Knez Z, Bren U. Hop compounds: extraction techniques, chemical analyses, anti-oxidative, antimicrobial, and anticarcinogenic effects. Nutrients. (2019) 11:257. doi: 10.3390/nu11020257
228. Wu TH, Wang PW, Lin TY, Yang PM, Li WT, Yeh CT, et al. Antioxidant properties of red raspberry extract alleviate hepatic fibrosis via inducing apoptosis and transdifferentiation of activated hepatic stellate cells. Biomed Pharmacother. (2021) 144:112284. doi: 10.1016/j.biopha.2021.112284
229. Bonesi M, Leporini M, Tenuta MC, Tundis R. The role of anthocyanins in drug discovery: recent developments. Curr Drug Discov Technolo. (2020) 17:286–98. doi: 10.2174/1570163816666190125152931
230. Calvano A, Izuora K, Oh EC, Ebersole JL, Lyons TJ, Basu A, et al. Dietary berries, insulin resistance and type 2 diabetes: an overview of human feeding trials. Food Funct. (2019) 10:6227–43. doi: 10.1039/C9FO01426H
231. Nunes S, Vieira P, Gomes P, Viana SD, Reis F. Blueberry as an attractive functional fruit to prevent (pre) diabetes progression. Antioxidants. (2021) 10:1162. doi: 10.3390/antiox10081162
232. Kang GG, Francis N, Hill R, Waters DLE, Blanchard CL, Santhakumar AB, et al. Coloured rice phenolic extracts increase expression of genes associated with insulin secretion in rat pancreatic insulinoma β-cells. Int J Mol Sci. (2020) 21:3314. doi: 10.3390/ijms21093314
233. Carrizzo A, Lizio R, Pietro PDi, Ciccarelli M, Damato A, Venturini E, et al. Healthberry 865 and its related, specific, single anthocyanins exert a direct vascular action, modulating both endothelial function and oxidative stress. Antioxidants. (2021) 10:1191. doi: 10.3390/antiox10081191
234. Les F, Casedas G, Gomez C, Moliner C, Valero MS, Lopez V, et al. The role of anthocyanins as antidiabetic agents: from molecular mechanisms to in vivo and human studies. J Physiol Biochem. (2020) 20:739. doi: 10.1007/s13105-020-00739-z
235. Zheng Y, Ley SH, Hu FB. Global aetiology and epidemiology of type 2 diabetes mellitus and its complications. Nat Rev Endocrinol. (2017) 14:88–98. doi: 10.1038/nrendo.2017.151
236. Jayawardena R, Ranasinghe P, Galappatthy P, Malkanthi R, Constantine GR, Katulanda P. Effects of zinc supplementation on diabetes mellitus: a systematic review and meta-analysis. DiabetolMetab Syndr4. (2012) 13. doi: 10.1186/1758-5996-4-13
237. Isvoranu C, Rugina D, Socaciu C. Plants and natural compounds with antidiabetic action. Not Bot Horti Agrobot Cluj Napoca. (2012) 40:314–25. doi: 10.15835/nbha4017205
238. Belwal T, Nabavi SF, Habtemariam S. Dietary anthocyanins and insulin resistance: when food becomes a medicine. Nutrients. (2017) 9:1111. doi: 10.3390/nu9101111
239. Heiss C, Rodriguez-Mateos A, Kelm M. Central role of eNOS in the maintenance of endothelial homeostasis. Antioxid Redox Signal. (2015) 22:1230–42. doi: 10.1089/ars.2014.6158
240. Heiss C, Sansone R, Karimi H, Krabbe M, Schuler D, Rodriguez-Mateos A, et al. European Union 7th Framework Program. Impact of cocoa flavanol intake on age dependent vascular stiffness in healthy men: a randomized, controlled, double masked trial. Age. (2015) 37:9794. doi: 10.1007/s11357-015-9794-9
241. Rodriguez-Mateos A, Vauzour D, Krueger CG, Shanmuganayagam D, Reed J, Calani L, et al. Bioavailability, bioactivity and impact on health of dietary flavonoids and related compounds: an update. Arch Toxicol. (2014) 88:1803–53. doi: 10.1007/s00204-014-1330-7
242. Del Rio D, Rodriguez-Mateos A, Spencer JP, Tognolini M, Borges G, Crozier A, et al. Dietary (poly) phenolics in human health: structures, bioavailability, and evidence of protective effects against chronic diseases. Antioxid Redox Signal. (2013) 18:1818–92. doi: 10.1089/ars.2012.4581
243. Rodriguez-Mateos A, Cifuentes-Gomez T, Tabatabaee S, Lecras C, Spencer JP. Procyanidin, anthocyanin, and chlorogenic acid contents of highbush and lowbush blueberries. J Agric Food Chem. (2012) 60:5772–8. doi: 10.1021/jf203812w
244. Cassidy A, Mukamal KJ, Liu L, Franz M, Eliassen AH, Rimm EB, et al. High anthocyanin intake is associated with a reduced risk of myocardial infarction in young and middle-aged women. Circulation. (2013) 127:188–96. doi: 10.1161/CIRCULATIONAHA.112.122408
245. Rodriguez-Mateos A, Istas G, Boschek L, Feliciano RP, Mills CE, Boby C, et al. Circulating anthocyanin metabolites mediate vascular benefits of blueberries: insights from randomized controlled trials, metabolomics, and nutrigenomics. J Gerontol A Biol Sci. (2019) 74:967–76. doi: 10.1093/gerona/glz047
246. Chen TM, Subeq YM, Lee RP, Chiou TW, Hsu BG. Single dose intravenous thioacetamide administration as a model of acute liver damage in rats. Int J Exp Pathol. (2008) 89:223–31. doi: 10.1111/j.1365-2613.2008.00576.x
247. Liang X, Zhang J, Guo F, Wei L, Zhou Q. Oxidative stress and inflammatory responses in the liver of swamp eel (Monopterus albus) exposed to carbon tetrachloride. Aquaculture. (2018) 496:232–8. doi: 10.1016/j.aquaculture.2018.07.026
248. Ritesh KR, Suganya A, Dileepkumar HV, Rajashekar Y, Shivanandappa T. A single acute hepatotoxic dose of CCl4 causes oxidative stress in the rat brain. Toxicol Rep. (2015) 2:891–5. doi: 10.1016/j.toxrep.2015.05.012
249. Romero FJ, Bosch-Morell F, Romero MJ, Jareno EJ, Romero B, Marin N, et al. Lipid peroxidation products and antioxidants in human disease. Environ Health Perspect. (1998) 106:1229–34. doi: 10.1289/ehp.98106s51229
250. Awad NE, Bdelkawy MAA, Hamed MA, Souleman AM, Abdelrahman EH, Ramadan NS. Antioxidant and hepatoprotective effects of Justicia spicigera ethyl acetate fraction and characterization of its anthocyanin content. Int J Pharm Pharm Sci. 7:91–96.
251. Mollavali M, Bolandnazar SA, Schwarz D, Rohn S, Riehle P, Zaare Nahandi F, et al. Flavonol glucoside and antioxidant enzyme biosynthesis affected by mycorrhizal fungi in various cultivars of onion (Allium cepa L.). J Agri Food Chem. (2016) 64:71–7. doi: 10.1021/acs.jafc.5b04791
Keywords: anthocyanins, bioavailability, dietary effects, extraction techniques, health effects
Citation: Samota MK, Sharma M, Kaur K, Sarita , Yadav DK, Pandey AK, Tak Y, Rawat M, Thakur J and Rani H (2022) Onion anthocyanins: Extraction, stability, bioavailability, dietary effect, and health implications. Front. Nutr. 9:917617. doi: 10.3389/fnut.2022.917617
Received: 11 April 2022; Accepted: 17 June 2022;
Published: 27 July 2022.
Edited by:
Dragan Milenkovic, University of California, Davis, United StatesReviewed by:
Juliane Viganó, State University of Campinas, BrazilMadhuresh Dwivedi, National Institute of Technology Rourkela, India
Copyright © 2022 Samota, Sharma, Kaur, Sarita, Yadav, Pandey, Tak, Rawat, Thakur and Rani. This is an open-access article distributed under the terms of the Creative Commons Attribution License (CC BY). The use, distribution or reproduction in other forums is permitted, provided the original author(s) and the copyright owner(s) are credited and that the original publication in this journal is cited, in accordance with accepted academic practice. No use, distribution or reproduction is permitted which does not comply with these terms.
*Correspondence: Mahesh Kumar Samota, mahesh.samota@icar.gov.in