Probiotic Supplementation Prevents the Development of Ventilator-Associated Pneumonia for Mechanically Ventilated ICU Patients: A Systematic Review and Network Meta-analysis of Randomized Controlled Trials
- 1Department of Emergency Medicine, Affiliated Hospital of Xuzhou Medical University, Xuzhou, China
- 2Laboratory of Morphology, Xuzhou Medical University, Xuzhou, China
- 3Department of Critical Care Medicine, Changshu Hospital Affiliated to Nanjing University of Chinese Medicine, Changshu, China
- 4Jiangsu Provincial Institute of Health Emergency, Xuzhou Medical University, Xuzhou, China
- 5Department of Critical Care Medicine, The Affiliated Wuxi No. 2 People’s Hospital of Nanjing Medical University, Wuxi, China
Background: Ventilator-associated pneumonia (VAP) is one of the common critical complications of nosocomial infection (NI) in invasive mechanically ventilated intensive care unit (ICU) patients. The efficacy of total parenteral nutrition (TPN), enteral nutrition and/or adjuvant peripheral parenteral nutrition (EPN) supplemented with or without probiotic, prebiotic, and synbiotic therapies in preventing VAP among these patients has been questioned. We aimed to systematically and comprehensively summarize all available studies to generate the best evidence of VAP prevention for invasive mechanically ventilated ICU patients.
Methods: Randomized controlled trials (RCTs) for the administration of TPN, EPN, probiotics-supplemented EPN, prebiotics-supplemented EPN, and synbiotics-supplemented EPN for VAP prevention in invasive mechanically ventilated ICU patients were systematically retrieved from four electronic databases. The incidence of VAP was the primary outcome and was determined by the random-effects model of a Bayesian framework. The secondary outcomes were NI, ICU and hospital mortality, ICU and hospital length of stay, and mechanical ventilation duration. The registration number of Prospero is CRD42020195773.
Results: A total of 8339 patients from 31 RCTs were finally included in network meta-analysis. The primary outcome showed that probiotic-supplemented EPN had a higher correlation with the alleviation of VAP than EPN in critically invasive mechanically ventilated patients (odds ratio [OR] 0.75; 95% credible intervals [CrI] 0.58–0.95). Subgroup analyses showed that probiotic-supplemented EPN prevented VAP in trauma patients (OR 0.30; 95% CrI 0.13–0.83), mixed probiotic strain therapy was more effective in preventing VAP than EPN therapy (OR 0.55; 95% CrI 0.31–0.97), and low-dose probiotic therapy (less than 1010 CFU per day) was more associated with lowered incidence of VAP than EPN therapy (OR 0.16; 95% CrI 0.04–0.64). Secondary outcomes indicated that synbiotic-supplemented EPN therapy was more significantly related to decreased incidence of NI than EPN therapy (OR 0.34; 95% CrI 0.11–0.85). Prebiotic-supplemented EPN administration was the most effective in preventing diarrhea (OR 0.05; 95% CrI 0.00–0.71).
Conclusion: Probiotic supplementation shows promise in reducing the incidence of VAP in critically invasive mechanically ventilated patients. Currently, low quality of evidence reduces strong clinical recommendations. Further high-quality RCTs are needed to conclusively prove these findings.
Systamatic Review Registration: [https://www.crd.york.ac.uk/prospero/display_record.php?ID=CRD42020195773], identifier [CRD42020195773].
Introduction
Description of the Condition
Ventilator-associated pneumonia (VAP) is the most common fatal complication of nosocomial infection (NI) in intensive care unit (ICU) patients requiring invasive mechanical ventilation (MV) (1, 2). A high incidence of VAP is reported in critically ill patients with major traumatic brain injuries and lung contusion (3, 4), chronic obstructive pulmonary disease (5), and acute respiratory distress syndrome (6). Especially in critically ill patients undergoing extracorporeal membrane oxygenation during invasive MV, the incidence of VAP is as high as 35% (7). VAP is associated with increased duration of invasive MV, ICU length of stay (LOS) (1), hospital cost (8, 9), and risk of dying (10, 11).
The development of VAP involves dysbiosis and failure of host immune response (12). Gut dysbiosis is common in critically ill patients (13), especially in invasive mechanically ventilated patients (1) who are generally subjected to various types of stress, such as shock, trauma, and bleeding (14). These insults to the gut deteriorate beneficial commensal bacteria and promote the overgrowth of pathogens (15). In addition, antibiotic therapies exacerbate gut dysbiosis (16, 17). Beneficial commensal bacteria play critical roles in the maintenance of the intestinal barrier and host immunity (18, 19). Gut dysbiosis weakens the intestinal barrier, which comprises physical, secretory, and immunological barriers, by producting cytokines, mucins, and antimicrobial peptides through intestinal epithelial cells. These secretions in turn impair host immunity (12, 20). Hence, gut dysbiosis is believed to be involved in the occurrence of VAP in critically ill patients under invasive MV (12, 14).
Recommendation of the Intervention
Microbes beneficial to hosts exert therapeutic effects on sites distant from habitats (21). Dietary interventions involving probiotic, prebiotic, and synbiotic supplementation can alleviate gut dysbiosis and enhance host immunity (22–24). Thus, in the European Union, probiotic and prebiotic supplements are recommended for critically ill patients to help maintain commensal microbiota (25). The American Society for Parenteral and Enteral Nutrition (A.S.P.E.N.) (26) and Canadian Clinical Practice Guidelines (CCPG) (19) recommend the use of probiotic and prebiotic supplementation to prevent dysbiosis in patients with critical illnesses. In addition, enteral nutrition and/or adjuvant peripheral parenteral nutrition (EPN) is more effective in protecting the intestinal barrier than total parenteral nutrition (TPN) (27, 28). The reducing effects of EPN on infectious morbidity as compared with those of TPN are well documented in meta-analyses involving a variety of populations with critical illnesses (29–33). Therefore, guidelines recommend the use of enteral nutrition for nutritional support therapy in critically ill patients and consider the use of supplemental parenteral nutrition (26, 30).
Controversy of the Intervention
The use of these therapies to prevent VAP remains highly controversial. Previous randomized controlled trials (RCTs) (14, 34–37) and meta-analyses (38–42) based on high-quality RCTs suggested that probiotics, prebiotics, and synbiotics are associated with VAP prevention. Promising data seemed to support that probiotic or prebiotic supplementation is a strategy for preventing VAP. However, evidence needs to be considered when evaluating the benefits of probiotics for VAP prevention. Large multicenter RCTs (including 2653 critically ill patients requiring invasive MV) evaluated the effects of probiotics and showed no significant reduction in the incidence of VAP (43). In addition, probiotic or prebiotic supplements do not seem to be associated with a reduction in VAP in patients with trauma (44–46) or other critically illness (47–54). TPN did not exhibit lower efficacy than EPN in VAP treatment in patients in patients with shock (55), acute organophosphate poisoning (56), and other critically illnesses (57, 58). Our previous network meta-analysis (NMA) on NI in critically ill patients suggested that TPN and EPN supplemented with or without probiotic, prebiotic, and synbiotic therapies did not significantly prevent the incidence of VAP (59). Moreover, evidence has highlighted the higher risk of bacterial and fungal translocation resulting from synbiotic and probiotic therapies in critical patients with damaged intestinal mucosa and immunodeficiency (60–62). These patients even showed an increased risk of mortality (63). Thus, the guidelines only recommend probiotic therapy based on evidence-based medicine for surgical and medical patient populations (26).
Importance of This Review
No study has compared the efficacy of TPN and EPN supplemented with or without probiotic, prebiotic, and synbiotic therapies in preventing VAP in critically ill patients under invasive MV. The traditional meta-analysis did not evaluate the risks and benefits of mixed treatments (64). Given that their efficacy in preventing VAP is uncertain, we conducted this systematic review and NMA to evaluate and rank probiotic-supplemented EPN, prebiotic-supplemented EPN, synbiotic-supplemented EPN, EPN, and TPN therapies in terms of their efficacy in preventing VAP in critically invasive mechanically ventilated patients. The effects of these therapies on NI, ICU LOS, duration of MV, mortality, and other clinically important outcomes were investigated. This study is intended to provide an evidence-based medical basis for exploring efficient and safe strategies for preventing or relieving VAP.
Methods
Approval
This study has been registered in Prospero (65), with number CRD42020195773.
Eligibility Criteria for Considering Studies
Types of Studies
We included only full-text published RCTs in this NMA.
Types of Participants
Adult ICU patients (≥16 years) who underwent invasive MV.
Types of Interventions
We included studies comparing two or more of the five therapies (probiotic-supplemented EPN, prebiotic-supplemented EPN, synbiotic-supplemented EPN, EPN, and TPN). Probiotic therapies include mixed strains or a single strain, different routes of administration, and different dosage regimens.
Types of Outcome Measures
The primary outcome was the incidence of VAP. Secondary outcomes were incidence of NI, incidence of bloodstream infections (BSIs), incidence of urinary tract infection (UTI), incidence of diarrhea, hospital and ICU mortality, duration of MV, and hospital and ICU LOS.
Exclusion Criteria
We excluded controlled clinical trials, quasi-RCTs, interrupted time series studies, controlled before and after studies, cluster-RCTs, and cross-over studies. Studies that used the same therapy in two study groups were excluded. Studies that were not published as full-text reports or did not report outcome variables or duplicate publications were also excluded.
Search Strategy for Identifying Studies
Electronic Searches
We have systematically searched clinical trials from 2000 to 2021 in the electronic databases of Cochrane (CENTRAL), Embase, Pubmed, and Web of Science. Searching was not restricted by language. According to the relevant term combination proposed by Cochrane for RCT-related systematic reviews, a special search strategy was developed for each database (66).
We used the search MeSH terms “critically ill” OR “intensive care unit” OR “mechanical ventilation” AND “synbiotics” OR “probiotics” OR “prebiotics” OR “enteral nutrition” OR “parenteral nutrition” AND “ventilator-associated pneumonia” combined with RCTs for searching relevant literature. The search strategy is described in Supplementary File 1.
Searching Other Resources
We searched Google Scholar to identify gray literature relevant to this topic and searched completed trials (latest search, 31 December 2021) in the following registers: ClinicalTrials.gov1; Chinese Clinical Trial Register2; World Health Organization (WHO) International Clinical Trials Registry Platform (ICTRP3); ISRCTN4; and Australian New Zealand Clinical Trials Registry5.
Data Collection and Analysis
Study Selection
Three investigators independently selected studies according to the inclusion criteria. Any discrepancies among investigators were resolved through consensus and arbitration within the review team.
Definition of Interventions and Outcomes
The definitions of probiotics, prebiotics, and synbiotics were obtained from the expert consensus document of the International Scientific Association for Probiotics and Prebiotics (ISAPP). Synbiotic is a mixture comprising live microorganisms and substrate(s) selectively utilized by host microorganisms that confers a health benefit on hosts (67). Probiotics are live microorganisms that may confer health benefits on hosts, when administered in adequate amounts (68). By contrast, prebiotics are substrates that are selectively utilized by host microorganisms and confer a health benefit (23). All outcomes were based on the definitions used in the primary study. Hospital mortality was presumed when mortality had an unspecified location.
Data Extraction
Three investigators independently extracted all the available data by using the Cochrane ARI Group’s data extraction form. The data included demographic information, intervention details, and data of primary and secondary outcomes. The following data were extracted from each study: author, published year, language, institution, funding, demographic information of participants (age range and gender), inclusion and exclusion criteria, methodological design (methods of blinding, allocation concealment, and randomization), intervention, treatment comparison (details of strains, dosage regimen, duration, and route of administration), and result (incidence of VAP and secondary outcomes). Discrepancies among investigators were resolved through consensus and arbitration within the review team. When necessary, we contacted the original authors to clarify unclear data and information on methodological quality.
Risk of Bias Assessment
The methodological quality of each included study was evaluated according to the recommended approach in Cochrane reviews (69). The risk of bias (ROB) was evaluated in seven domains. Each domain was classified as high, unclear, or low and was adjudicated within each study. A study had low ROB when it had no high ROB domain or had only three or low unclear domains. A study was classified as moderate ROB when it had a high ROB domain or more than three unclear domains. All other studies were classified as high ROB. Three independent evaluators performed an assessment and reached a consensus on the results. The results were represented in a ROB table.
Measures of Treatment Effect
Unit of Analysis Issues
The unit of analysis was each participant in a trial.
Data Synthesis
Dichotomous outcomes were measured using the proportions of variables and estimated using odds ratio (OR). Continuous outcomes were measured using means and standard deviations and estimated using mean difference (MD). We expressed data as an OR or MD with 95% credible interval (CrI). A Bayesian random-effects model was conducted to assess the study effect sizes and synthesize evidence for overall outcomes. Dichotomous outcomes and continuous outcomes used binomial likelihood and normal likelihood, respectively. The first 20,000 iterations were annealing, and the subsequent 30,000 iterations were sampled. Continuous iterations were increased when the potential scale reduction factor was not close to 1.0 (70). We will conduct the surface under the cumulative ranking curve (SUCRA) for all outcomes to obtain a comprehensive ranking of each therapy (71).
Assessment of Heterogeneity
The amount of heterogeneity variance of pairwise and network was calculated and conveyed using I2 statistic (72, 73). Heterogeneity was considered statistically significant when the I2 statistic was more than 50%, and the possible sources of heterogeneity for each outcome were discussed through subgroup analysis.
Assessment of Inconsistency
Global and local methods, such as design-by-treatment tests and node splitting, were used in evaluating network consistency (74, 75). Inconsistency between direct and indirect comparison evidence was assumed when the P-value was less than 0.05.
Assessment of Transitivity
The transitivity of network was assured by limiting the number of critically ill patients. The distributions of clinical variables, such as age and severity of illness at baseline, were used in investigating the transitivity assumption of NMA (71).
Subgroup Analysis
Subgroup analyses including population, disease severity, dose, strains, the timing of initial nutrition, and quality, were used in assessing the impacts of key factors on the primary outcome and elucidating the source of possible heterogeneity. Specific populations, such as general ICU and trauma patients, were analyzed. In healthy people, the number of obligate anaerobes was around log10 colony-forming units (CFU)/g of feces (1010) on average (76). In some countries of the European Union and North America, the minimum recommended dose of probiotics was the minimum number of viable cells administered per day (109 CFU) (77, 78). Therefore, according to the daily dose of enteral probiotics, we divided the subgroups into high-dose (probiotics of more than 1010 CFU per day) and low-dose (probiotics of less than 1010 CFU per day) groups. For the subgroup analysis of strains, we evaluated studies that used only Lactobacillus rhamnosus GG as probiotics and mixed strains as probiotics. Moreover, we divided the subgroups into high-severity of illness and low-severity illness groups according to the Acute Physiology And Chronic Health Evaluation (APACHE) II score of 20 at baseline or Simplified Acute Physiology Score (SPSA) II score of 35 at baseline (10, 79–81). According to the ROB results, the study was divided into high-quality (only low ROB) and low-quality (moderate and high ROB) groups. We divided the timing of initial nutrition according to Europe and American guidelines: within 24 h, within 48 h, and beyond 48 h (82, 83).
Sensitivity Analysis
Sensitivity analysis was performed using the datasets of recruited centers (multicenter or single-center), quality of study (low and high quality), and diagnostic criteria of VAP (excluding studies with questionable diagnostic criteria). When the factors influencing the conclusions were identified, the potential causes of uncertainty were explored.
Quality Assessment
We assessed the quality of evidence for each network estimate according to the Grading of Recommendations Assessment, Development, and Evaluation (GRADE) system (84). The following criteria were evaluated sequentially: study limitation, imprecision, inconsistency, indirectness, and publication bias. Study limitations were evaluated based on the assessment of ROB and the contribution matrix of network estimates. Imprecision was assessed according to the OR point estimate and confidence interval (CI). Inconsistency was assessed in accordance with heterogeneity and incoherence. Indirectness was evaluated in accordance with the transitivity of the network. Comparison-adjusted funnel plots were used in assessing publication bias (85, 86). The level of evidence was classified as high, moderate, low, or very low (84). The details of quality assessment are presented in Supplementary File 8.
Statistical Software
R software (version 3.6.1) and Stata (version 14.0) were used for statistical analysis. The former was used (Netmeta 1.1–0 package, gemtc 0.8–2, and rjags 4–10 package) for Bayesian NMA, and the latter was used in drawing the comparison-adjusted funnel plots and network plots of the network. R software (ggplot2 3.2.1 package) was also used in drawing SUCRA graphics.
Results
Description of Included Studies
Characteristics related to each included study are presented in Tables 1, 2.
Results of the Search
A total of 9825 articles were identified, of which 121 articles were potentially eligible articles. Overall, 31 RCTs were included after full texts were retrieved (Figure 1).
Included Studies
Thirty-one studies were published between 2001 and 2021 in 16 countries and comprised 8339 patients. Among them, 29 articles were published in English, and one article each was published in Chinese and Arabic. Fourteen trials (45%) recruited patients from Asia; fourteen trials (45%), from Europe; two (6%) trials, from Americas; and one (4%) trial, from Oceania. Sample size ranged from 33 to 2650, with a mean of 132 participants (SD = 300). The mean age was 53 years (SD = 11). In total, 5167 (62%) males were included in the sample population. One study (4%) randomly assigned participants to three groups. Ten studies (32%) were multicenter studies, seventeen (55%) were double-blind studies, and eleven (35%) were open-label studies. The most included diseases were general diseases in the ICU, followed by sepsis or septic shock, severe multiple trauma, severe stroke, brain trauma, and poisoning. The mean APACHE II score was 21 (SD = 9), the mean SAPS II score was 46 (SD = 11), and the mean GCS score was 8 (SD = 1). The details were presented in Table 1.
Sixteen trials compared probiotics-supplemented EPN with EPN, seven trials compared EPN with TPN, five trials compared synbiotics-supplemented EPN with EPN, four trials comparing prebiotics-supplemented EPN with EPN, and one trial compared synbiotics-supplemented EPN with prebiotics-supplemented EPN. No trial compared synbiotics-supplemented EPN with probiotics-supplemented EPN, synbiotics-supplemented EPN with TPN, probiotics-supplemented EPN with TPN, probiotics-supplemented EPN with prebiotics-supplemented EPN, and prebiotics-supplemented EPN with TPN. The dose of probiotics varied from 6 × 107 CFU per day to 9 × 1011 CFU per day. Participants in the majority of trials received initial nutritional support therapy within 72 h. The details were presented in Table 2.
A total of 25 studies reported VAP, 12 reported NI, 9 reported BSIs; 12 reported UTI, and 15 reported diarrhea. Fourteen reported ICU mortality, eighteen reported hospital mortality, twenty-five reported ICU LOS, seventeen reported hospital mortality, and eighteen reported duration of MV. Data for all outcomes was presented in Table 3.
The diagnostic criteria for VAP included Centers for Disease Control and Prevention (CDC) criteria (87), American College of Chest Physicians (ACCP) clinical Criteria (88), American Thoracic Society (ATS) and Infectious Diseases Society of America (IDSA) criteria (89), clinical pulmonary infection score (CPIS) criteria (90), and diagnostic criteria of clinical symptoms, laboratory and chest radiographic tests, and pathogenic bacteria (Supplementary Table 2.1). The diagnostic criteria for NI, BSIs and UTI used the CDC criteria or criteria of combined clinical symptoms, laboratory tests and pathogenic bacteria (Supplementary Tables 2.2, 2.3). NI included pneumonia, BSIs, catheter-related bloodstream infections (CRBSI), UTI, sepsis, surgical infections, wound infections, skin or soft-tissue infection, and other infections (Supplementary Table 2.5). The diagnostic criteria for diarrhea were duration, frequency, weight, and combination of consistency and frequency (Supplementary Table 2.4).
Risk of Bias in Included Studies
Twenty (65%) of 31 trials were rated as low ROB, six (19%) trials were deemed high, and five (16%) were considered moderate (Figures 2, 3). Adequately random sequence generation was performed in 23 trials, unclear in four trials, and high risk in four trials. Adequate allocation concealment was reported in 24 trials, and unclear in seven trials. Blinding of participants and personnel was adequately reported in 24 trials, unclear in six trials, and high risk in one trial. Blinding of outcome assessors was adequately reported in four trials, and unclear in seven trials. Twenty-eight trials adequately addressed incomplete outcome data, and we considered two “high” risk, and the remaining study was rated as “unclear.” We did not have sufficient information to assess selective reporting bias because the protocols for the included studies were not available. Therefore, all studies were rated as “low” risk. Twenty-five trials were rated as “low” risk, five trials were rated as “unclear,” and one trial was rated as “high” risk.
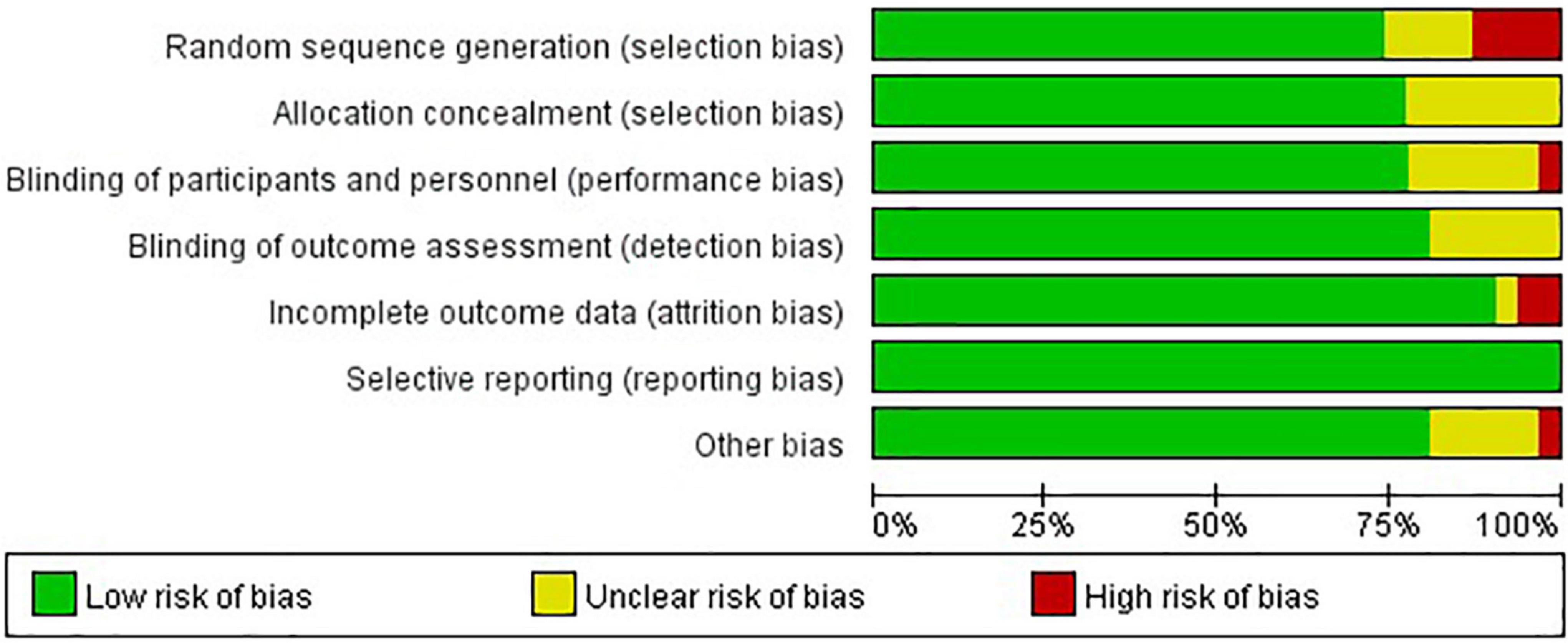
Figure 2. Risk of bias assessment graph for included studies. Review authors’ judgments (low, unclear, and high) for each risk of bias item shown as percentages across all included studies.
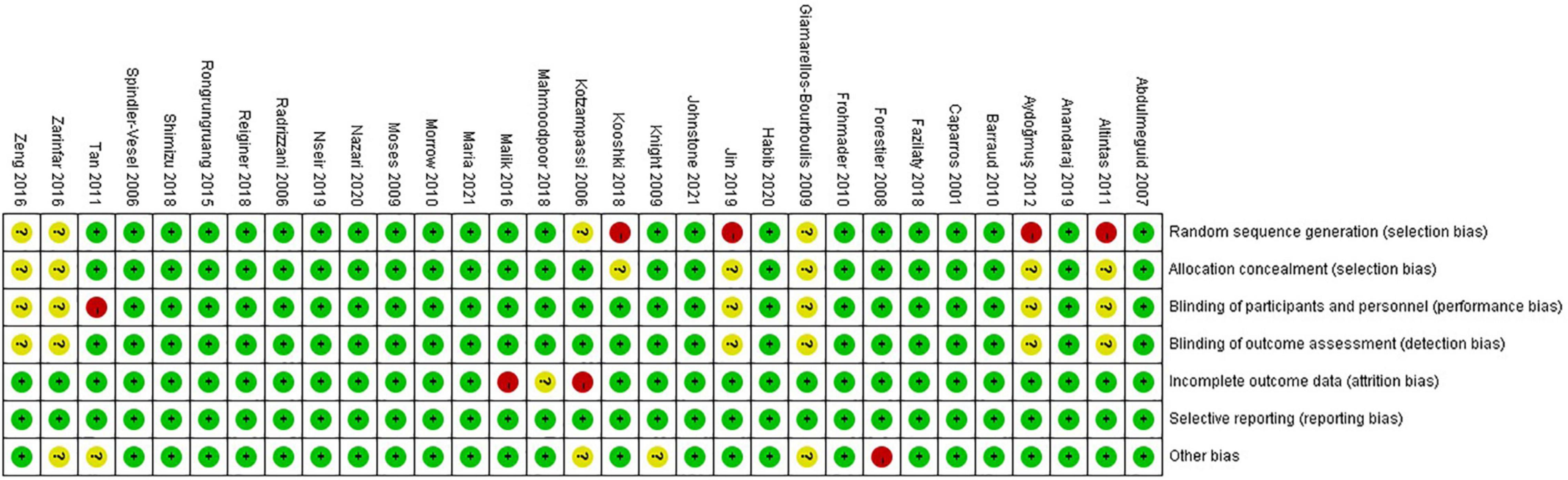
Figure 3. Risk of bias summary for included studies. Studies were classified as having low ROB if none was rated as high ROB, and three or less were rated as unclear risk. Studies had moderate ROB if one was rated as high ROB or none was rated as high ROB but four or more were rated as unclear risk. All other cases were assumed to pertain to high ROB.
Effects of Interventions in the Network
Primary Outcome (Incidence of Ventilator-Associated Pneumonia)
The analysis of the primary outcome was based on 25 studies comprising 7721 patients. A head-to-head trial between EPN and any other intervention was obtained, but no study directly compared synbiotic-supplemented EPN therapy with TPN, prebiotic-supplemented EPN, and probiotic-supplemented EPN therapies in the network (Figure 4). Probiotic-supplemented EPN therapy was more significantly associated with a low incidence of VAP than EPN therapy (OR 0.75; 95% CrI 0.58–0.95), whereas synbiotic-supplemented EPN (OR 0.66; 95% CrI 0.37–1.15), prebiotic-supplemented EPN (OR 1.14; 95% CrI 0.63–1.98), and TPN (OR 1.01; 95% CrI 0.67–1.54) therapies were not correlated to a low incidence of VAP (Table 4). The SUCRA ranking curve showed that synbiotic-supplemented EPN and probiotic-supplemented EPN therapies were the top two treatments for VAP prevention (Figure 5).
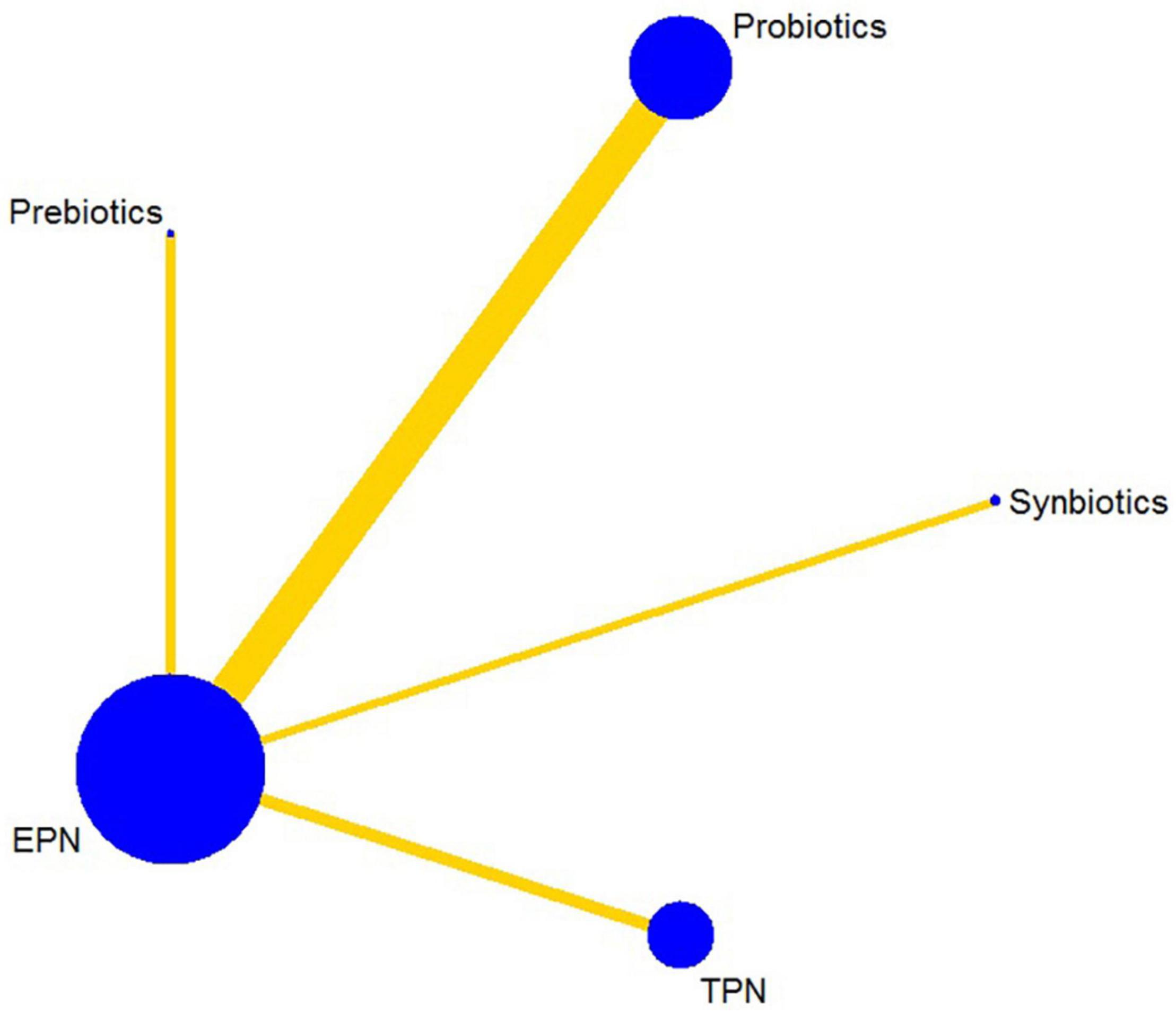
Figure 4. Network plot of all intervention comparisons for ventilator-associated pneumonia. The size of the nodes corresponds to the total number of participants that study the treatments. The (directly) comparable treatments are linked with a line. The thickness of the line corresponds to the standard error of trials that study this comparison. The colors of the line correspond to the quality of trials that study this comparison. Moderate risk of bias [yellow]. EPN, enteral nutrition and/or adjuvant peripheral parenteral nutrition. TPN, total parenteral nutrition.
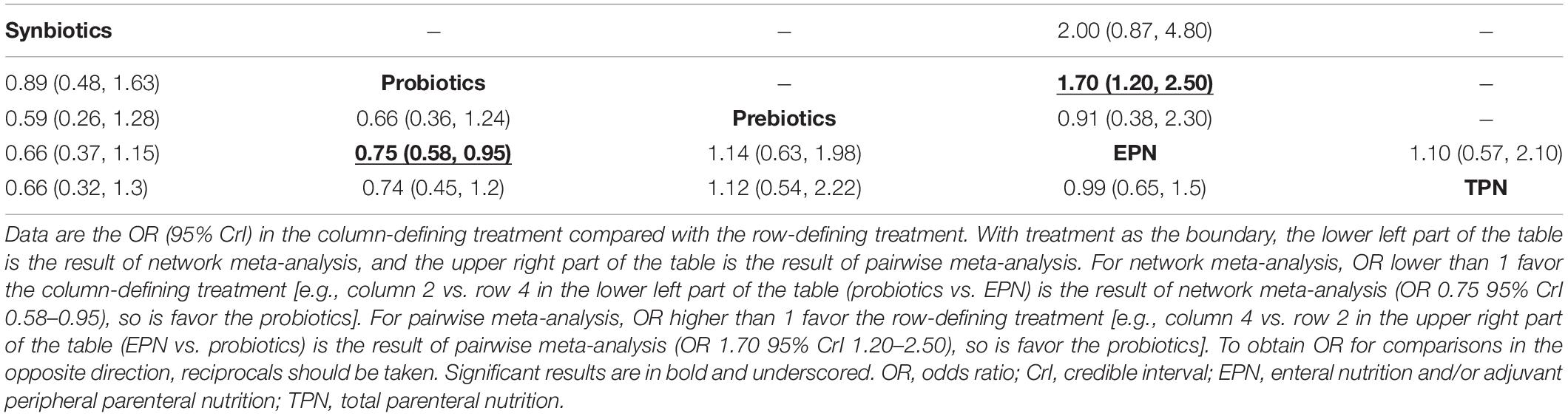
Table 4. Results from pairwise meta-analysis and network meta-analysis on ventilator-associated pneumonia.
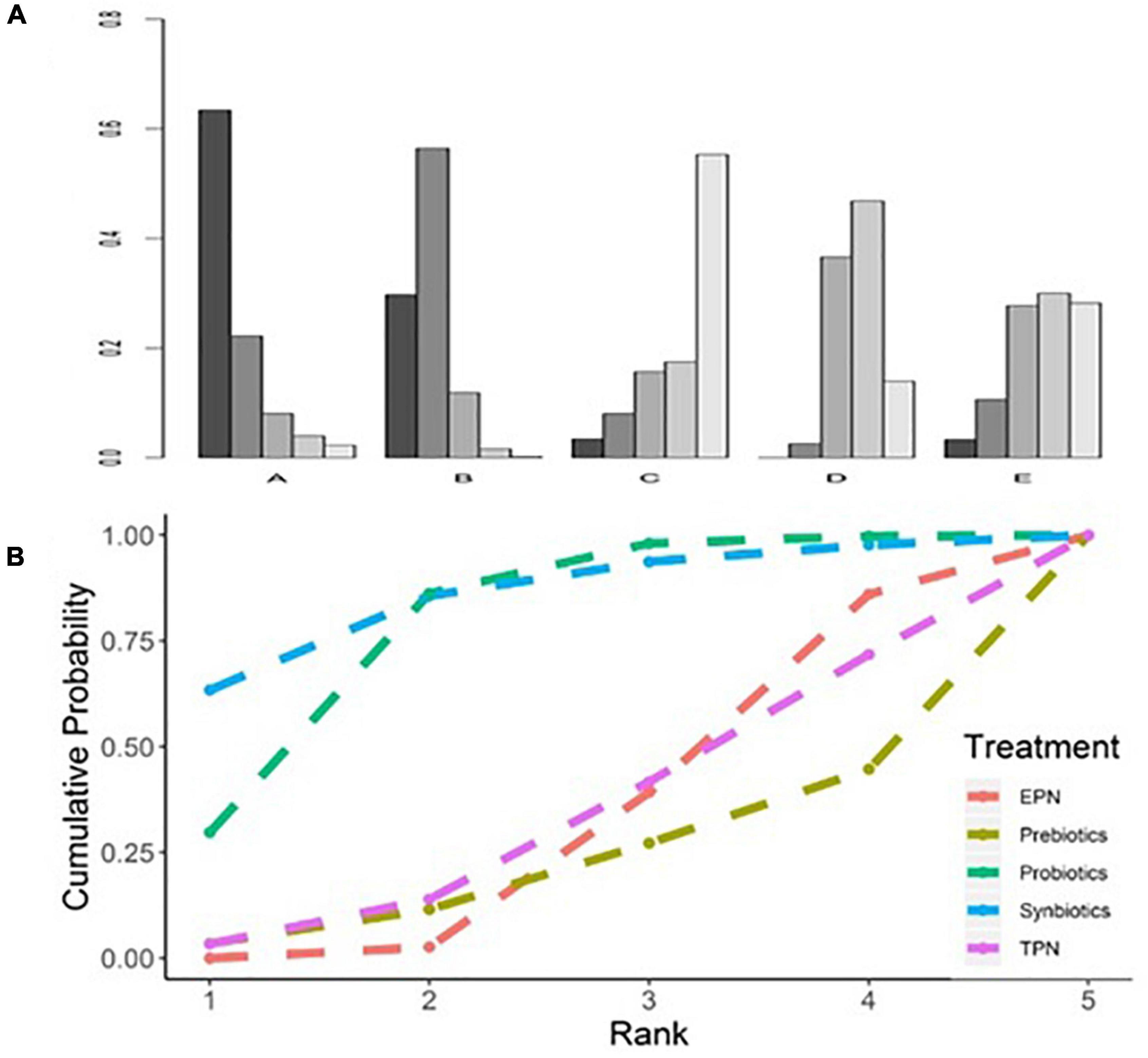
Figure 5. Rankogram and SUCRA ranking curve for ventilator-associated pneumonia. (A) Rankogram for ventilator-associated pneumonia. A = Synbiotics. B = Probiotics. C = Probiotics. D = EPN. E = TPN. (B) SUCRA ranking for ventilator-associated pneumonia. The number on the X-axis represents the rank. As the number goes up, the rating goes down. EPN, enteral nutrition and/or adjuvant peripheral parenteral nutrition. TPN, total parenteral nutrition.
Secondary Outcomes
Analyses of NI, BSIs, UTI, and diarrhea outcomes were based on 12 studies (including 6183 patients), 9 studies (including 5939 patients), 12 studies (including 6198 patients), and 15 studies (including 6439 patients), respectively. Analyses of hospital and ICU mortality outcomes were based on 18 studies (including 6998 patients) and 14 studies (including 6586 patients), respectively. Analyses of hospital LOS, ICU LOS, and MV duration were based on 17 studies (including 7021 patients), 25 studies (including 7817 patients), and 18 studies (including 4520 patients), respectively.
A head-to-head trial between EPN therapy and any other intervention was found in all networks. No study directly compared probiotic-supplemented EPN therapy with TPN and synbiotic-supplemented EPN therapies in networks of NI, BSIs, UTI, ICU mortality, ICU LOS, and MV duration. Similarly, prebiotic-supplemented EPN therapy was not directly compared with TPN and probiotic-supplemented EPN therapies in these networks (Supplementary File 4).
In terms of preventing the development of NI, synbiotic-supplemented EPN therapy was more effective than EPN therapy (OR 0.19; 95% CrI 0.08–0.47). In terms of preventing diarrhea, prebiotic-supplemented EPN therapy was more effective than EPN therapy (OR 0.05; 95% CrI 0.00–0.71). Synbiotic-supplemented EPN, probiotic-supplemented EPN, prebiotic-supplemented EPN, and TPN therapies were not correlated with the low incidence of BSIs and UTI, or the low mortality of hospital and ICU, compared with EPN therapy. Similarly, these therapies were not correlated with the shorter length of hospital stay, length of ICU stay, and duration of MV, compared with EPN therapy.
The SUCRA ranking curve showed that synbiotic-supplemented EPN therapy ranked first among all therapies in networks of NI, BSIs, UTI, ICU mortality, ICU LOS, and MV duration. Prebiotic-supplemented EPN therapy ranked first among all therapies in networks of diarrhea and hospital mortality. Probiotic-supplemented EPN therapy ranked first among all therapies in the network of hospital LOS. Figure 6 and Supplementary Files 3, 9 detail the results.
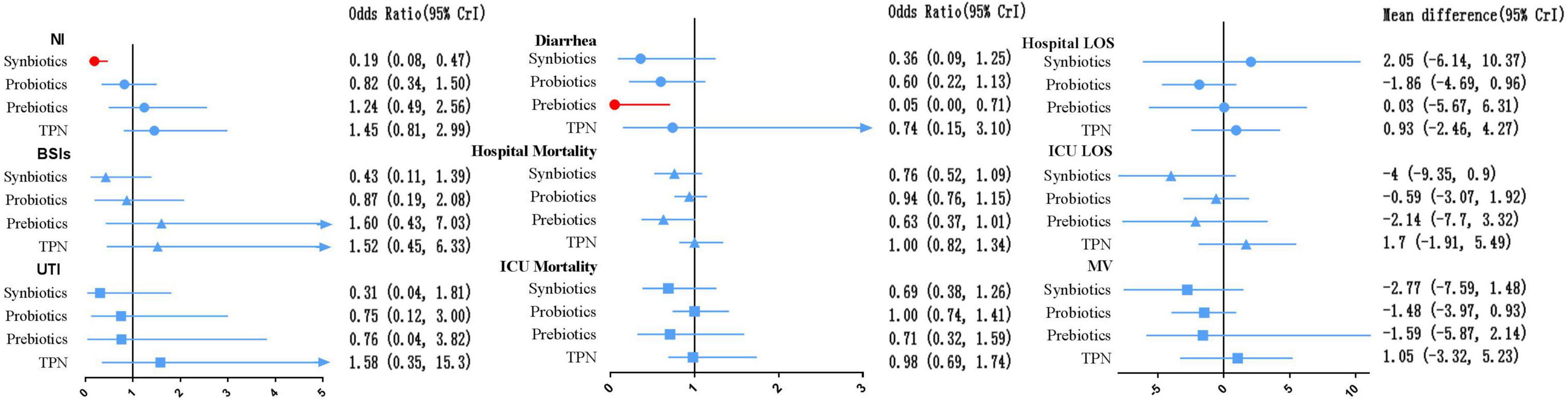
Figure 6. Forest plot of the effect estimate for each active intervention vs. EPN on secondary outcomes. Estimates are presented as odds ratios (OR) or mean difference (MD) and 95% CrI. OR < 1 favor the treatment. MD < 0 favor the treatment. BSIs, bloodstream infections; CrI, credible interval; EPN, enteral nutrition and/or adjuvant peripheral parenteral nutrition; LOS, length of stay; MV, duration of mechanical ventilation; NI, nosocomial infections; TPN, total parenteral nutrition; UTI, urinary tract infection.
Direct Meta-analysis
Table 4 shows the results of the pairwise on VAP. The data of pairwise meta-analysis for all secondary outcomes are shown in Supplementary File 3.
Heterogeneity, Inconsistency and Transitivity
Heterogeneity analyses revealed moderate-to-high global heterogeneity for VAP incidence (I2 = 73.43%), NI incidence (I2 = 58.27%), diarrhea incidence (I2 = 84.83%), hospital LOS (I2 = 74.57%), ICU LOS (I2 = 83.60%) and MV duration (I2 = 90.55%) (Supplementary Figure 5.1).
Global inconsistency and inconsistencies between indirect and direct comparisons were not found for all outcomes (Supplementary Figure 5.2).
Transitivity assessment for primary outcome showed that the mean age among all comparisons was the same (Supplementary Figure 6.1). In addition, the mean severity of illness at baseline showed a relatively high mean APACHE II score for the comparison between prebiotics and EPN (Supplementary Figure 6.2).
Subgroup Analyses for the Incidence of Ventilator-Associated Pneumonia
Analyses in the subgroups of general ICU patients and trauma patients subgroup were based on 19 studies (including 7250 patients), and 6 studies (including 471 patients), respectively. Analyses in the subgroups of higher disease severity and lower disease severity were based on 11 studies (including 6061 patients) and 11 studies (including 1506 patients), respectively. Analyses in the subgroups of only Lactobacillus and a mixture of strains were based on 14 studies (including 6256 patients), and 19 studies (including 4504 patients), respectively. Analyses in the subgroups of low-dose and high-dose were based on 16 studies (including 3917 patients), and 16 studies (including 6783 patients), respectively. Analysis in the subgroup of high-quality studies was based on 16 studies (including 6864 patients). Analyses in the subgroups of nutrition therapy within 24 h, nutrition therapy within 48 h, and nutrition therapy beyond 48 h were based on 9 studies (including 3808 patients), 15 studies (including 4205 patients), and 4 studies (including 2841 patients), respectively.
In terms of trauma patients, probiotic-supplemented EPN therapy was significantly associated with a low incidence of VAP, in contrast to EPN therapy (OR 0.30; 95% CrI 0.13–0.83). Similarly, probiotic-supplemented EPN therapy was significantly associated with a low incidence of VAP in the routine low-dose subgroup (OR 0.17; 95% CrI 0.03–0.73) and the mixed probiotic strain subgroup (OR 0.55; 95% CrI 0.31–0.97), in contrast to EPN therapy. In terms of other subgroups, synbiotic-supplemented EPN, probiotic-supplemented EPN, prebiotic-supplemented EPN, and TPN therapies were not associated with a low incidence of VAP, in contrast to EPN therapy. Similarly, these therapies were not correlated with the shorter length of hospital stay, length of ICU stay, and duration of MV, compared with EPN therapy (Table 5).
Sensitivity Analyses for the Incidence of Ventilator-Associated Pneumonia
Analyses of studies with low-moderate ROB and studies with robust diagnostic criteria for VAP were based on 20 studies (including 7439 patients), and 23 studies (including 7596 patients), respectively. Analyses of multicentric studies and single-center studies were based on 8 studies (including 5920 patients) and 17 studies (including 1801 patients), respectively. In terms of studies with low-moderate ROB and studies with robust diagnostic criteria for VAP, probiotic-supplemented EPN therapy remained significantly associated with low VAP incidence, in contrast to EPN therapy, whereas synbiotic-supplemented EPN, prebiotic-supplemented EPN, and TPN therapies were not correlated with a low incidence of VAP. No significant differences were found among the five therapies for incidence of VAP in multicentric studies and single-center studies. The data are shown in Supplementary File 10.
Grades of Recommendation, Assessment, Development and Evaluation Assessments
Publication bias was found in the incidence of VAP and NI, hospital mortality, hospital and ICU LOS, and MV duration (Supplementary File 7).
In summary, the GRADE scores of the relative therapeutic effects and ranking for VAP suggested that the certainty of evidence varied. Comparisons between synbiotic-supplemented EPN and probiotic-supplemented EPN therapies, synbiotic-supplemented EPN and prebiotic-supplemented EPN therapies, and probiotics-supplemented EPN and TPN therapies were high, whereas those between synbiotics-supplemented EPN and EPN therapies and between prebiotic-supplemented EPN and TPN therapies were low. Moreover, the comparison between probiotic-supplemented EPN and TPN therapies was very low, and other comparisons were moderate. The ranking of treatment was low. Downgrading was due to imprecision, publication bias, or inconsistency (Table 6). Supplementary File 8 presents the GRADE and ranking of treatment for all secondary outcomes.
Discussion
Summary of Main Results
This systematic review evaluated the effects of TPN and EPN supplemented with or without probiotic, prebiotic, and synbiotic therapies on VAP, using 31 RCTs (including 8339 patients). Overall, the results of NMA indicated that probiotic supplementation was significantly associated with increased incidence of VAP in critically invasive mechanically ventilated patients. This result was consistent with previous RCTs (14, 34, 36, 37, 91, 92) and meta-analysis (38–42). Subgroup analysis showed that probiotic supplementation therapy significantly prevented the incidence of VAP in trauma patients. Mixed strains and low-dose probiotic therapies were associated with a low incidence of VAP. Moreover, this NMA found that synbiotic supplementation therapy was significantly related to decreased incidence of NI and prebiotics supplementation were the most effective in preventing diarrhea.
Applicability of Evidence
The availability of evidence that probiotic supplementation alleviates VAP in critically ill patients was influenced by several complex risk factors. The possible underlying mechanism areas were discussed below: First, probiotic supplementation may maintain the intestinal microbiota. Probiotic therapy increases the number of intestinal microbiota while increasing their genus groups and promoting the growth of other microbiota (14). Second, probiotic supplementation increases the nutritional support of host epithelial cells. Probiotic supplementation significantly increases the levels of short-chain fatty acids, especially acetate, which provides an additional energy source for intestinal epithelial cells and may attenuate the occurrence of VAP (93). Third, probiotic supplements maintain the intestinal epithelial barrier. Probiotic supplementation may inhibit the release of enteric toxins and maintain tight connections by promoting an increase in acetate and lactate levels (94). Finally, probiotics regulate innate and adaptive immune systems, which in turn promote extra-intestinal organ function and reduce systemic inflammation (94).
Disagreements With Other Studies
Notably, the primary finding was inconsistent with the results of previous meta-analysis (59, 95, 96) and RCTs (43, 44, 46, 49, 50, 52, 54, 97), which showed that probiotics cannot alleviate VAP in invasive mechanically ventilated patients. The reasons that probiotic supplementation did not improve VAP were complex, and we believe that the following reasons can be discussed: First, the principal limitation of these studies stems from small sample size. The sample sizes used by Tan et al. (44), Habib et al. (46), and Jin et al. (97) were all less than 100. Increasing the population size may provide additional information about the prevention of VAP (98). In addition, Barraud et al. (50) showed that 740 patients are needed to demonstrate the benefits of probiotics, but their study was prematurely stopped after an interim analysis because of safety concerns. Only 167 patients were actually enrolled in the study. Second, some large clinical trials used a single probiotic strain. Only Lactobacillus rhamnosus was used by Johnstone et al. (43) and Anandaraji et al. (54). Accumulating evidence shows that different strains of probiotics exert beneficial effects through multiple mechanisms and have synergistic effects when supplemented as combinations of strains (99). Third, the improving effects of probiotics may vary with the study population. Jin et al. (97) showed that probiotics prevented the incidence of VAP in stroke patients, Tan et al. (44) showed an opposite result in patients with traumatic brain injuries. Therefore, the effect of critically ill population heterogeneity on probiotics should be fully considered.
Analysis of Subgroup Results
The results of the subgroup analysis were as follows: First, probiotic-supplemented therapy had a significant effect on preventing the incidence of VAP in trauma patients and ranked first. Major traumatic injuries influence intestinal microbiota inhibiting the proliferation of beneficial commensal bacteria by inducing the overgrowth of pathogenic bacteria (100), and leading to changes in immune function after trauma (4). Starting probiotic therapy immediately can minimize dysbiosis. Probiotics may play a potential suppressive role in gut inflammation by strengthening intestinal mucosal and epithelial barriers and antagonizing the colonization of virulent species (101). Future high-quality RCTs with large sample sizes should focus on trauma patients. Second, mixed probiotic strain therapy was associated with low VAP. Gut dysbiosis involves loss of diversity and abundance and altered metabolic capacity of a flora. The depletion of beneficial commensal bacteria such as Bifidobacterium and Lactobacillus was significantly associated with these effects (17). Probiotic strains had a greater effect on ecological performance possibly because of competitive interactions among the members of the microbiota (102). Some clinical therapeutic benefits are likely derived from shared mechanisms, suggesting that probiotic effects may be subspecies specific, species-specific, or genus specific (99, 103). Third, low-dose probiotic therapy was associated with a lower incidence of VAP. The physiologically required administration of probiotics seems to be associated with a low incidence of infectious complications, whereas the administration of excessive probiotic microorganisms led to an increase in infectious complications due to bacteremia and fungemia in critically ill adults, postoperative, and immuno-compromised patients (22). Hence, in our view, the safety of probiotics in future RCTs should be explored, particularly in specialized critically ill patients. Furthermore, disease severity at baseline has found no association between probiotic-supplemented EPN therapy and a low incidence of VAP as compared to EPN therapy. Good scoring systems should be able to predict the severity of disease and functional status, be well-calibrated, validated and highly discernible, and be applicable to all the general population of critically ill patients. However, APACHE II and SAPS II scoring predictions are very complex, requiring multiple variables to calculate their score (104). Their cut-offs, sensitivity, and specificity for predicting the mortality risk of ICU patients vary with patient populations (79). In addition, they are weak to measure organ dysfunction (105) and are affected by lead-time bias and treatment (106, 107). For these reasons, the above results should be interpreted with caution.
Analysis for Secondary Outcomes
Results of analysis for secondary outcomes were as follows: In terms of preventing the incidence of NI, synbiotic-supplemented EPN therapy showed a better effect than EPN therapy. However, these findings may be inconclusive. Although these results were consistent with our previous results (59), they should be interpreted with caution. The analysis involved only three small-sample studies involving synbiotics. Among them, Spindler-Vesel et al.’s (108) and Kotzampassi et al.’s studies (109) involving trauma patients and Shimizu et al.’s study (14) involving sepsis patients suggested that synbiotics supplementation can alleviate NI. They indicated that synbiotic-supplemented therapy should be considered to prevent NI in specific invasive mechanically ventilated patients. Additionally, in terms of preventing the incidence of diarrhea, prebiotics-supplemented EPN therapy showed a better effect than EPN therapy. However, these findings may be equally inconclusive and should be interpreted with caution. The diagnostic criteria for diarrhea were based on the original literature, which was based on duration, frequency, weight, and the combination of consistency and frequency. Given that ensuring consistency among different definitions is difficult (110), the incidence of diarrhea varied according to the diagnostic criterion used for calculations. In addition, owing to the limited number of studies, we were unable to perform further grouping analysis.
Strengths of This Network Meta-analysis
This study had four strengths. Firstly, this is the first NMA that is based on a Bayesian framework and that assessed the relative effectiveness of different symbiotic regimens for alleviating VAP in critically invasive mechanically ventilated patients. The results of this study can help clinicians identify differences in relative efficacy among treatments without head-to-head comparison. Second, this study offered the most updated assessment of symbiotic therapy for patients with critically invasive mechanically ventilated patients. A structured search strategy was used in retrieving all published studies since 2000, focusing on symbiotic therapy for improving VAP. Third, this study examined the largest number of studies on symbiotic therapy (31 RCTs) from 16 countries in Europe, America, Asia, and Africa and enrolled 8339 patients. Fourth, this study evaluated several relevant important clinical outcomes among critically invasive mechanically ventilated patients, including the incidence of NI, the incidence of BSIs, the incidence of UTI, the incidence of diarrhea, mortality, LOS, and MV duration. Hence, our study is of great value to clinicians exploring the characteristics of different therapies. Lastly, sensitivity and subgroup analysis provided evidence of the robustness of estimates.
Limitations of This Network Meta-analysis
This study had several limitations. First, patients with sepsis, shock, severe multiple injuries, severe traumatic brain injury, severe stroke, acute organophosphorus poisoning, and general ICU were enrolled in this study. These patients presented significant differences in pathogenic factors, pathophysiology, and clinical manifestations. Second, the definitions of VAP in the included studies varied. Ensuring the consistency of different definitions was difficult. Given that these criteria vary in sensitivity and accuracy for diagnosing VAP (111), reliance upon different criteria may result in potential variations in the incidence of VAP (2). Some included studies did not provide an accurate definition of secondary outcomes, such as incidence of diarrhea, and were inconsistent because they were vague (112) and subject to different interpretations (110). Third, variations in dose, genus, species, strain, and duration of probiotics were found among the studies. Furthermore, the gastrointestinal conditions of patients before the treatment could contribute to the heterogeneity of the patients and affect the treatment efficacy. The aforementioned four limitations were common among the studies in this field, which may contribute to the slight heterogeneity (13, 40, 113, 114). These heterogeneities could not be eliminated by statistical methods. To decrease these heterogeneities among the studies, whenever possible, we performed subgroup analysis based on population, disease severity, dose, strains, the timing of initial nutrition, and study quality. Nevertheless, more subgroup analyses were limited by the small number of studies. The results of subgroup analysis showed that these heterogeneities were significantly decreased. Similarly, sensitivity analyses of studies with low-moderate ROB and studies with robust diagnostic criteria for VAP showed that the conclusions concerning the overall effects of probiotics were robust. Overall, even the Cochrane review, which is internationally recognized as the gold standard of evidence-based information in health care, could not eliminate the effect of these heterogeneities (113, 114). Because of this, none of the recommendations that advocated probiotics supplementation in critically ill patients in evidence-based guidelines were actually based on high-quality evidence. Clinical evidence surrounding the impact of the aforementioned limitations on the treatment effects of probiotics is the focus of this field, and future clinical studies and reviews should focus on addressing this issue.
Beyond this, other potential limitations should also be concerned: Nearly 90% of all included studies were from Europe and Asia, and thus our ability to generalize the results of this study to all patient populations was limited. Antibiotic consumption and length of antibiotic therapy were not assessed because of inadequate information and inconsistent reporting across trials. Based on the GREAD system, confidence in the estimates was low or very low, restricting the interpretation and evaluation in further clinical practice.
Suggestions and Perspectives
In further works, the mechanisms of probiotics and hosts need to be further explored and clarified. Well-conducted, large-scale, multicenter, concealed, and stratified RCTs focus on specific populations, such as trauma, sepsis, and high infectious risk or high antimicrobial exposure patients, are needed to confirm these findings. Factors, including probiotic genus, species, strain, optimal dose, route, and duration of administration, should be carefully considered in the evaluation of the effectiveness in alleviating VAP.
Postbiotics are inanimate microorganisms and/or their components confer health benefits on hosts (115). They improve the immune system in animal experiments and may play important roles in antimicrobial and targeted anti-inflammatory activities and immune response modulation and influence intestinal secretion and movement. Moreover, they exert beneficial metabolic effects through interactions with dietary components. We are looking forward to high-quality human clinical RCTs to provide the ultimate proof (115).
Conclusion
Based on pooled results, this study suggests that probiotic supplementation shows promise in reducing the incidence of VAP in critically invasive mechanically ventilated patients. Probiotic supplementation for trauma patients, as well as routine low-dose and mixed probiotic strains supplementation, seems to be plausible. Currently, low quality of evidence reduces strong clinical recommendations. Further high-quality RCTs are needed to conclusively prove these findings.
Data Availability Statement
The original contributions presented in this study are included in the article/Supplementary Material, further inquiries can be directed to the corresponding author/s.
Author Contributions
CL and NX had the idea for and designed the study. NX supervised the study. CL, NX, JM, FL, and JC did search clinical trials, study select, data extract, and statistical analysis. CL wrote the manuscript. All authors contributed to acquisition, analysis, interpretation of data, revised the report, and approved the final version before submission.
Funding
This study was funded by the Project of Xuzhou Applied and Basic Research (KC19016).
Conflict of Interest
The authors declare that the research was conducted in the absence of any commercial or financial relationships that could be construed as a potential conflict of interest.
Publisher’s Note
All claims expressed in this article are solely those of the authors and do not necessarily represent those of their affiliated organizations, or those of the publisher, the editors and the reviewers. Any product that may be evaluated in this article, or claim that may be made by its manufacturer, is not guaranteed or endorsed by the publisher.
Supplementary Material
The Supplementary Material for this article can be found online at: https://www.frontiersin.org/articles/10.3389/fnut.2022.919156/full#supplementary-material
Supplementary File 1 | Search strategy.pdf.
Supplementary File 2 | Diagnostic criteria for outcomes.pdf.
Supplementary File 3 | Results of meta-analysis of pairwise comparisons and network.pdf.
Supplementary File 4 | Network plot of all intervention comparisons.pdf.
Supplementary File 5 | Assessment of heterogeneity and inconsistency in networks.pdf.
Supplementary File 6 | Assessment of transitivity in networks for primary outcome.pdf.
Supplementary File 7 | Comparison-adjusted funnel plot for the network.pdf.
Supplementary File 8 | GRADE for each outcome in networks.pdf.
Supplementary File 9 | Treatment ranking and SUCRA ranking curve for each outcome.pdf.
Supplementary File 10 | Sensitivity analyses for the risk of primary outcome.pdf.
Abbreviations
BSIs, bloodstream infections; CENTRAL, Cochrane Central Register for Controlled Trials; CFU, colony-forming units; CRBSI, catheter-related bloodstream infections; CrI, credible interval; DB, double-blind; EN, enteral nutrition; EPN, enteral nutrition and/or adjuvant peripheral parenteral nutrition; GCS, Glasgow coma scale; GRADE, Grades of Recommendation, Assessment, Development and Evaluation; ICU, intensive care unit; LOS, length of stay; MC, multicenter; MD, mean difference; MV, mechanical ventilation; NI, nosocomial infection; NMA, network meta-analysis; NR, not reported; OP, open study; OR, odds ratio; PN, parenteral nutrition; PRISMA, Preferred Reporting Items for Systematic Review and Meta-analysis; PROSPERO, prospective register of systematic reviews; RCTs, randomized controlled trial studies; SB, single-blind; SC, single-center; SD, standard deviation; SUCRA, surface under the cumulative ranking curve; TPN, total parenteral nutrition; UTI, urinary tract infection; VAP, ventilator-associated pneumonia.
Footnotes
- ^ https://clinicaltrials.gov/
- ^ http://www.chictr.org.cn/index.aspx
- ^ http://www.who.int/ictrp/en/
- ^ https://www.isrctn.com/
- ^ http://www.anzctr.org.au/
References
1. Papazian L, Klompas M, Luyt CE. Ventilator-associated pneumonia in adults: a narrative review. Intensive Care Med. (2020) 46:888–906. doi: 10.1007/s00134-020-05980-0
2. Fernando SM, Tran A, Cheng W, Klompas M, Kyeremanteng K, Mehta S, et al. Diagnosis of ventilator-associated pneumonia in critically ill adult patients-a systematic review and meta-analysis. Intensive Care Med. (2020) 46:1170–9. doi: 10.1007/s00134-020-06036-z
3. Cook A, Norwood S, Berne J. Ventilator-associated pneumonia is more common and of less consequence in trauma patients compared with other critically ill patients. J Trauma. (2010) 69:1083–91. doi: 10.1097/TA.0b013e3181f9fb51
4. Koulenti D, Tsigou E, Rello J. Nosocomial pneumonia in 27 ICUs in Europe: perspectives from the EU-VAP/CAP study. Eur J Clin Microbiol. (2017) 36:1999–2006. doi: 10.1007/s10096-016-2703-z
5. Rouze A, Cottereau A, Nseir S. Chronic obstructive pulmonary disease and the risk for ventilator-associated pneumonia. Curr Opin Crit Care. (2014) 20:525–31. doi: 10.1097/MCC.0000000000000123
6. Forel JM, Voillet F, Pulina D, Gacouin A, Perrin G, Barrau K, et al. Ventilator-associated pneumonia and ICU mortality in severe ARDS patients ventilated according to a lung-protective strategy. Crit Care. (2012) 16:R65. doi: 10.1186/cc11312
7. Grasselli G, Scaravilli V, Di Bella S, Biffi S, Bombino M, Patroniti N, et al. Nosocomial infections during extracorporeal membrane oxygenation: incidence, etiology, and impact on patients’ outcome. Crit Care Med. (2017) 45:1726–33. doi: 10.1097/CCM.0000000000002652
8. Zimlichman E, Henderson D, Tamir O, Franz C, Song P, Yamin CK, et al. Health care-associated infections: a meta-analysis of costs and financial impact on the US health care system. JAMA Intern Med. (2013) 173:2039–46. doi: 10.1001/jamainternmed.2013.9763
9. Kollef KE, Schramm GE, Wills AR, Reichley RM, Micek ST, Kollef MH. Predictors of 30-day mortality and hospital costs in patients with ventilator-associated pneumonia attributed to potentially antibiotic-resistant gram-negative bacteria. Chest. (2008) 134:281–7. doi: 10.1378/chest.08-1116
10. Melsen WG, Rovers MM, Groenwold RHH, Bergmans DCJJ, Camus C, Bauer TT, et al. Attributable mortality of ventilator-associated pneumonia: a meta-analysis of individual patient data from randomised prevention studies. Lancet Infect Dis. (2013) 13:665–71. doi: 10.1016/S1473-3099(13)70081-1
11. Bekaert M, Timsit JF, Vansteelandt S, Depuydt P, Vesin A, Garrouste-Orgeas M, et al. Attributable mortality of ventilator-associated pneumonia: a reappraisal using causal analysis. Am J Respirat Cri Care Med. (2011) 184:1133–9. doi: 10.1164/rccm.201105-0867OC
12. Rello J, Schrenzel J, Tejo AM. New insights into pneumonia in patients on prolonged mechanical ventilation: need for a new paradigm addressing dysbiosis. J Brasil Pneumol. (2021) 47:e20210198. doi: 10.36416/1806-3756/e20210198
13. Szychowiak P, Villageois-Tran K, Patrier J, Timsit JF, Ruppe E. The role of the microbiota in the management of intensive care patients. Ann Intensive Care. (2022) 12:3. doi: 10.1186/s13613-021-00976-5
14. Shimizu K, Yamada T, Ogura H, Mohri T, Kiguchi T, Fujimi S, et al. Synbiotics modulate gut microbiota and reduce enteritis and ventilator-associated pneumonia in patients with sepsis: a randomized controlled trial. Crit Care. (2018) 22:239. doi: 10.1186/s13054-018-2167-x
15. Wischmeyer PE, McDonald D, Knight R. Role of the microbiome, probiotics, and ‘dysbiosis therapy’ in critical illness. Curr Opin Crit Care. (2016) 22:347–53. doi: 10.1097/MCC.0000000000000321
16. Karacaer F, Hamed I, Özogul F, Glew RH, Özcengiz D. The function of probiotics on the treatment of ventilator-associated pneumonia (VAP): facts and gaps. J Med Microbiol. (2017) 66:1275–85. doi: 10.1099/jmm.0.000579
17. Duan H, Yu L, Tian F, Zhai Q, Fan L, Chen W. Antibiotic-induced gut dysbiosis and barrier disruption and the potential protective strategies. Crit Rev Food Sci Nutr. (2020) 62:1427–52. doi: 10.1080/10408398.2020.1843396
18. Isakow W, Morrow LE, Kollef MH. Probiotics for preventing and treating nosocomial infections: review of current evidence and recommendations. Chest. (2007) 132:286–94. doi: 10.1378/chest.06-2156
19. Morrow LE, Wischmeyer P. Blurred lines: dysbiosis and probiotics in the ICU. Chest. (2017) 151:492–9. doi: 10.1016/j.chest.2016.10.006
20. Klingensmith NJ, Coopersmith CM. The gut as the motor of multiple organ dysfunction in critical illness. Crit Care Clin. (2016) 32:203–12. doi: 10.1016/j.ccc.2015.11.004
21. Reid G, Abrahamsson T, Bailey M, Bindels LB, Bubnov R, Ganguli K, et al. How do probiotics and prebiotics function at distant sites. Benef Microb. (2017) 8:521–33. doi: 10.3920/BM2016.0222
22. Suez J, Zmora N, Segal E, Elinav E. The pros, cons, and many unknowns of probiotics. Nat Med. (2019) 25:716–29. doi: 10.1038/s41591-019-0439-x
23. Gibson GR, Hutkins R, Sanders ME, Prescott SL, Reimer RA, Salminen SJ, et al. Expert consensus document: the international scientific association for probiotics and prebiotics (ISAPP) consensus statement on the definition and scope of prebiotics. Nat Rev Gastroenterol Hepatol. (2017) 14:491–502. doi: 10.1038/nrgastro.2017.75
24. Roberfroid M, Gibson GR, Hoyles L, McCartney AL, Rastall R, Rowland I, et al. Prebiotic effects: metabolic and health benefits. Br J Nutr. (2010) 104(Suppl. 2):S1–63. doi: 10.1017/S0007114510003363
25. Smug LN, Salminen S, Sanders ME, Ebner S. Yoghurt and probiotic bacteria in dietary guidelines of the member states of the European Union. Benef Microb. (2014) 5:61–6. doi: 10.3920/BM2013.0050
26. McClave SA, Taylor BE, Martindale RG, Warren MM, Johnson DR, Braunschweig C, et al. Guidelines for the provision and assessment of nutrition support therapy in the adult critically ill patient: society of critical care medicine (SCCM) and American society for parenteral and enteral nutrition (A.S.P.E.N.). JPEN J Parenter Enteral Nutr. (2016) 40:159–211. doi: 10.1177/0148607115621863
27. Ammori BJ. Importance of the early increase in intestinal permeability in critically ill patients. Eur J Surg. (2002) 168:660–1. doi: 10.1080/11024150201680019
28. Kang W, Kudsk KA. Is there evidence that the gut contributes to mucosal immunity in humans? JPEN J Parenter Enteral Nutr. (2007) 31:246–58. doi: 10.1177/0148607107031003246
29. Braunschweig CL, Levy P, Sheean PM, Wang X. Enteral compared with parenteral nutrition: a meta-analysis. Am J Clin Nutr. (2001) 74:534–42. doi: 10.1093/ajcn/74.4.534
30. Heyland DK, Dhaliwal R, Drover JW, Gramlich L, Dodek P. Canadian critical care clinical practice guidelines committee. Canadian clinical practice guidelines for nutrition support in mechanically ventilated, critically ill adult patients. JPEN J Parenter Enteral Nutr. (2003) 27:355–73. doi: 10.1177/0148607103027005355
31. Gramlich L, Kichian K, Pinilla J, Rodych NJ, Dhaliwal R, Heyland DK. Does enteral nutrition compared to parenteral nutrition result in better outcomes in critically ill adult patients? A systematic review of the literature. Nutrition. (2004) 20:843–8. doi: 10.1016/j.nut.2004.06.003
32. Peter JV, Moran JL, Phillips-Hughes J. A meta-analysis of treatment outcomes of early enteral versus early parenteral nutrition in hospitalized patients. Crit Care Med. (2005) 33:213–220;discussion 260–211. doi: 10.1097/01.CCM.0000150960.36228.C0
33. Simpson F, Doig GS. Parenteral vs. enteral nutrition in the critically ill patient: a meta-analysis of trials using the intention to treat principle. Intensive Care Med. (2005) 31:12–23. doi: 10.1007/s00134-004-2511-2
34. Nazari B, Amani L, Ghaderi L, Gol MK. Effects of probiotics on prevalence of ventilator-associated pneumonia in multitrauma patients: a randomized clinical trial. Trauma Month. (2020) 25:262–8.
35. Kooshki A, Khazaei Z, Zarghi A, Rad M, Tabaraie Y. Prebiotic prophylaxis of ventilator-associated pneumonia: a randomized clinical trial. Biomed Res Ther. (2018) 5:2287–95. doi: 10.15419/bmrat.v5i5.442
36. Morrow LE, Kollef MH, Casale TB. Probiotic prophylaxis of ventilator-associated pneumonia: a blinded, randomized, controlled trial. Am J Respir Crit Care Med. (2010) 182:1058–64. doi: 10.1164/rccm.200912-1853OC
37. Giamarellos-Bourboulis EJ, Bengmark S, Kanellakopoulou K, Kotzampassi K. Pro- and synbiotics to control inflammation and infection in patients with multiple injuries. J Trauma. (2009) 67:815–21. doi: 10.1097/TA.0b013e31819d979e
38. Barraud D, Bollaert PE, Gibot S. Impact of the administration of probiotics on mortality in critically ill adult patients: a meta-analysis of randomized controlled trials. Chest. (2013) 143:646–55. doi: 10.1378/chest.12-1745
39. Siempos I, Ntaidou T, Falagas M. Impact of the administration of probiotics on the incidence of ventilator-associated-pneumonia: a meta-analysis of randomized controlled trials. Crit Care Med. (2010) 38:3. doi: 10.1097/CCM.0b013e3181c8fe4b
40. Manzanares W, Lemieux M, Langlois PL, Wischmeyer PE. Probiotic and synbiotic therapy in critical illness: a systematic review and meta-analysis. Crit Care. (2016) 19:262. doi: 10.1186/s13054-016-1434-y
41. Zhao J, Li LQ, Chen CY, Zhang GS, Cui W, Tian BP. Do probiotics help prevent ventilator-associated pneumonia in critically ill patients? A systematic review with meta-analysis. ERJ Open Res. (2021) 7:00302–2020. doi: 10.1183/23120541.00302-2020
42. Batra P, Soni KD, Mathur P. Efficacy of probiotics in the prevention of VAP in critically ill ICU patients: an updated systematic review and meta-analysis of randomized control trials. J Intensive Care. (2020) 8:81. doi: 10.1186/s40560-020-00487-8
43. Johnstone J, Meade M, Lauzier F, Marshall J, Duan E, Dionne J, et al. Effect of probiotics on incident ventilator-associated pneumonia in critically ill patients: a randomized clinical trial. JAMA. (2021) 326:1024–33.
44. Tan M, Zhu JC, Du J, Zhang LM, Yin HH. Effects of probiotics on serum levels of Th1/Th2 cytokine and clinical outcomes in severe traumatic brain-injured patients: a prospective randomized pilot study. Crit Care. (2011) 15:R290. doi: 10.1186/cc10579
45. Fazilaty Z, Chenari H, Shariatpanahi ZV. Effect of β-glucan on serum levels of IL-12, hs-CRP, and clinical outcomes in multiple-trauma patients: a prospective randomized study. Ulus Travma Acil Cerrahi Derg. (2018) 24:287–93. doi: 10.5505/tjtes.2017.34514
46. Habib T, Kassem AB, Ahmed I. Early probiotics in preventing ventilator-Associated pneumonia after multiple trauma. Asian J Pharm Clin Res. (2020) 13:83–5. doi: 10.22159/ajpcr.2020.v13i10.38114
47. Caparros T, Lopez J, Grau T. Early enteral nutrition in critically ill patients with a high-protein diet enriched with arginine, fiber, and antioxidants compared with a standard high-protein diet. The effect on nosocomial infections and outcome. J Parenter Enteral Nutr. (2001) 25:299–309. doi: 10.1177/0148607101025006299
48. Forestier C, Guelon D, Cluytens V, Gillart T, Sirot J, De Champs C. Oral probiotic and prevention of Pseudomonas aeruginosa infections: a randomized, double-blind, placebo-controlled pilot study in intensive care unit patients. Crit Care. (2008) 12:R69. doi: 10.1186/cc6907
49. Knight DJ, Gardiner D, Banks A, Snape SE, Weston VC, Bengmark S, et al. Effect of synbiotic therapy on the incidence of ventilator associated pneumonia in critically ill patients: a randomised, double-blind, placebo-controlled trial. Intensive Care Med. (2009) 35:854–61. doi: 10.1007/s00134-008-1368-1
50. Barraud D, Blard C, Hein F, Marcon O, Cravoisy A, Nace L, et al. Probiotics in the critically ill patient: a double blind, randomized, placebo-controlled trial. Intensive Care Med. (2010) 36:1540–7. doi: 10.1007/s00134-010-1927-0
51. Rongrungruang Y, Krajangwittaya D, Pholtawornkulchai K, Tiengrim S, Thamlikitkul V. Randomized controlled study of probiotics containing Lactobacillus casei (Shirota strain) for prevention of ventilator-associated pneumonia. J Med Assoc Thailand. (2015) 98:253–9.
52. Zeng J, Wang CT, Zhang FS, Qi F, Wang SF, Ma S, et al. Effect of probiotics on the incidence of ventilator-associated pneumonia in critically ill patients: a randomized controlled multicenter trial. Intensive Care Med. (2016) 42:1018–28. doi: 10.1007/s00134-016-4303-x
53. Mahmoodpoor A, Hamishehkar H, Asghari R, Abri R, Shadvar K, Sanaie S. Effect of a probiotic preparation on ventilator-associated pneumonia in critically ill patients admitted to the intensive care unit: a prospective double-blind randomized controlled trial. Nutr Clin Pract. (2018) 34:156–62. doi: 10.1002/ncp.10191
54. Anandaraj AM, Pichamuthu KK, Hansdak SG, Samuel P, Irodi A, Valsa S, et al. A randomised controlled trial of lactobacillus in the prevention of ventilator associated pneumonia. J Clin Diagnostic Res. (2019) 13:21–4. doi: 10.7860/JCDR/2019/42325.13084
55. Reignier J, Boisramé-Helms J, Brisard L, Lascarrou JB, Ait Hssain A, Anguel N, et al. Enteral versus parenteral early nutrition in ventilated adults with shock: a randomised, controlled, multicentre, open-label, parallel-group study (NUTRIREA-2). Lancet. (2018) 391:133–43.
56. Moses V, Mahendri NV, John G, Peter JV, Ganesh A. Early hypocaloric enteral nutritional supplementation in acute organophosphate poisoning – a prospective randomized trial. Clin Toxicol. (2009) 47:419–24. doi: 10.1080/15563650902936664
57. Aydoğmuş MT, Tomak Y, Tekin M, Katı I, Hüseyinoğlu U. Glutamine supplemented parenteral nutrition to prevent ventilator-associated pneumonia in the intensive care unit. Balkan Med J. (2012) 29:414–8. doi: 10.5152/balkanmedj.2012.043
58. Altintas ND, Aydin K, Turkoglu MA, Abbasoglu O, Topeli A. Effect of enteral versus parenteral nutrition on outcome of medical patients requiring mechanical ventilation. Nutr Clin Pract. (2011) 26:322–9. doi: 10.1177/0884533611405790
59. Li C, Liu L, Gao Z, Zhang J, Chen H, Ma S, et al. Synbiotic therapy prevents nosocomial infection in critically ill adult patients: a systematic review and network meta-analysis of randomized controlled trials based on a bayesian framework. Front Med (Lausanne). (2021) 8:693188. doi: 10.3389/fmed.2021.693188
60. Didari T, Solki S, Mozaffari S, Nikfar S, Abdollahi M. A systematic review of the safety of probiotics. Expert Opin Drug Saf. (2014) 13:227–39. doi: 10.1517/14740338.2014.872627
61. Pinto G, Lima L, Pedra T, Assumpção A, Morgado S, Mascarenhas L. Bloodstream infection by Saccharomyces cerevisiae in a COVID-19 patient receiving probiotic supplementation in the ICU in Brazil. Access Microbiol. (2021) 3:000250. doi: 10.1099/acmi.0.000250
62. Van den Nieuwboer M, Brummer RJ, Guarner F, Morelli L, Cabana M, Claasen E. The administration of probiotics and synbiotics in immune compromised adults: is it safe? Benef Microb. (2015) 6:3–17. doi: 10.3920/BM2014.0079
63. Besselink MG, van Santvoort HC, Buskens E, Boermeester MA, van Goor H, Timmerman HM, et al. Probiotic prophylaxis in predicted severe acute pancreatitis: a randomised, double-blind, placebo-controlled trial. Lancet. (2008) 371:651–9. doi: 10.1016/S0140-6736(08)60207-X
64. Lu G, Ades AE. Combination of direct and indirect evidence in mixed treatment comparisons. Stat Med. (2004) 23:3105–24. doi: 10.1002/sim.1875
65. Li C, Gao Z, Zhang J, Chen H, Ma S, Mo M, et al. Effectiveness of Probiotic, Prebiotic, and Synbiotic Therapies in Improving Ventilator-Associated Pneumonia in Critically ill Adult:A Systematic Review and Network Meta-Analysis of Randomized Controlled Trials Based on a Bayesian Framework. (2020). Available online at: https://www.crd.york.ac.uk/prospero/display_record.php?ID=CRD42020195773 (accessed August 6, 2020).
66. Higgins J, Thomas J. Cochrane Handbook for Systematic Reviews of Interventions Version 5.1.0. (2012). p. 2012. Available online at: http://handbook.cochrane.org (accessed December 15, 2012).
67. Swanson KS, Gibson GR, Hutkins R, Reimer RA, Reid G, Verbeke K, et al. The International Scientific Association for Probiotics and prebiotics (ISAPP) consensus statement on the definition and scope of synbiotics. Nat Rev Gastroenterol Hepatol. (2020) 17:687–701. doi: 10.1038/s41575-020-0344-2
68. Hill C, Guarner F, Reid G, Gibson GR, Merenstein DJ, Pot B, et al. Expert consensus document. The international scientific association for probiotics and prebiotics consensus statement on the scope and appropriate use of the term probiotic. Nat Rev Gastroenterol Hepatol. (2014) 11:506–14. doi: 10.1038/nrgastro.2014.66
69. Higgins JPTAD, Sterne JAC. Chapter 8: assessing risk of bias in included studies. In: Higgins JPT, Green S editors. Cochrane Handbook for Systematic Reviews of Interventions Version 5.1.0. (New York, NY: Wiley) (2011).
70. Gelman A, Rubin DB. Markov chain monte carlo methods in biostatistics. Stat Methods Med Res. (1996) 5:339–55. doi: 10.1177/096228029600500402
71. Salanti G, Ades AE, Ioannidis JP. Graphical methods and numerical summaries for presenting results from multiple-treatment meta-analysis: an overview and tutorial. J Clin Epidemiol. (2011) 64:163–71. doi: 10.1016/j.jclinepi.2010.03.016
72. Rhodes KM, Turner RM, Higgins JP. Predictive distributions were developed for the extent of heterogeneity in meta-analyses of continuous outcome data. J Clin Epidemiol. (2015) 68:52–60. doi: 10.1016/j.jclinepi.2014.08.012
73. Turner RM, Jackson D, Wei Y, Thompson SG, Higgins JP. Predictive distributions for between-study heterogeneity and simple methods for their application in Bayesian meta-analysis. Stat Med. (2015) 34:984–98. doi: 10.1002/sim.6381
74. Dias S, Welton NJ, Caldwell DM, Ades AE. Checking consistency in mixed treatment comparison meta-analysis. Stat Med. (2010) 29:932–44. doi: 10.1002/sim.3767
75. Higgins JP, Jackson D, Barrett JK, Lu G, Ades AE, White IR. Consistency and inconsistency in network meta-analysis: concepts and models for multi-arm studies. Res Synth Methods. (2012) 3:98–110. doi: 10.1002/jrsm.1044
76. Bian LNS, Asahara T. Effects of the continuous intake of Lactobacillus casei strain Shirota-fermented milk on risk management of long-term inpatients at health service facilities for the elderly. Int J Probiot Prebiot. (2011) 6:123–31.
77. Health Canada. Accepted Claims About the Nature of Probiotic Microorganisms in Food. Ottawa, ON: Health Canada (2009).
78. Ministero della Salute. Commissione Unica per la Nutrizione e la Dietetica. Guidelines on Probiotics and Prebiotics. Viale Giorgio Ribotta: Ministero della Salute (2013).
79. Sungono V, Hariyanto H, Soesilo TEB, Adisasmita AC, Syarif S, Lukito AA, et al. Cohort study of the APACHE II score and mortality for different types of intensive care unit patients. Postgrad Med J. (2021) 8:postgradmedj-2021-140376. doi: 10.1136/postgradmedj-2021-140376
80. Zhou XY, Ben SQ, Chen HL, Ni SS. A comparison of APACHE II and CPIS scores for the prediction of 30-day mortality in patients with ventilator-associated pneumonia. Int J Infect Dis. (2015) 30:144–7. doi: 10.1016/j.ijid.2014.11.005
81. Colussi G, Perrotta G, Pillinini P, Dibenedetto AG, Da Porto A, Catena C, et al. Prognostic scores and early management of septic patients in the emergency department of a secondary hospital: results of a retrospective study. BMC Emerg Med. (2021) 21:152. doi: 10.1186/s12873-021-00547-8
82. Compher C, Bingham AL, McCall M, Patel J, Rice TW, Braunschweig C, et al. Guidelines for the provision of nutrition support therapy in the adult critically Ill patient: American society for parenteral and enteral nutrition. JPEN J Parenter Enteral Nutr. (2022) 46:12–41. doi: 10.1002/jpen.2267
83. Singer P, Blaser AR, Berger MM, Alhazzani W, Calder PC, Casaer MP, et al. ESPEN guideline on clinical nutrition in the intensive care unit. Clin Nutr. (2019) 38:48–79. doi: 10.1016/j.clnu.2018.08.037
84. Song F, Harvey I, Lilford R. Adjusted indirect comparison may be less biased than direct comparison for evaluating new pharmaceutical interventions. J Clin Epidemiol. (2008) 61:455–63. doi: 10.1016/j.jclinepi.2007.06.006
85. Chaimani A, Higgins JP, Mavridis D, Spyridonos P, Salanti G. Graphical tools for network meta-analysis in STATA. PLoS One. (2013) 8:e76654. doi: 10.1371/journal.pone.0076654
86. Salanti G, Del Giovane C, Chaimani A, Caldwell DM, Higgins JP. Evaluating the quality of evidence from a network meta-analysis. PLoS One. (2014) 9:e99682. doi: 10.1371/journal.pone.0099682
87. Horan TC, Andrus M, Dudeck MA. CDC/NHSN surveillance definition of health care-associated infection and criteria for specific types of infections in the acute care setting. Am J Infect Control. (2008) 36:309–32. doi: 10.1016/j.ajic.2008.03.002
88. Grossman RF, Fein A. Evidence-based assessment of diagnostic tests for ventilator-associated pneumonia. Executive summary. Chest. (2000) 117(Suppl. 42):177S–81S. doi: 10.1378/chest.117.4_suppl_2.177S
89. Society AT. America IDSo: Guidelines for the management of adults with hospital-acquired, ventilator-associated, and healthcare-associated pneumonia. Am J Respir Crit Care Med. (2005) 171:388–416. doi: 10.1164/rccm.200405-644ST
90. Pugin J, Auckenthaler R, Mili N, Janssens JP, Lew PD, Suter PM. Diagnosis of ventilator-associated pneumonia by bacteriologic analysis of bronchoscopic and nonbronchoscopic “Blind” bronchoalveolar lavage fluid. Am Rev Respir Dis. (1991) 143:1121–9. doi: 10.1164/ajrccm/143.5_Pt_1.1121
91. Zarinfar N, Sharafkhah M, Amiri M, Rafeie M. Probiotic effects in prevention from ventilator-associated pneumonia. Koomesh. (2016) 17:803–13.
92. Tsilika M, Thoma G, Aidoni Z, Tsaousi G, Fotiadis K, Stavrou G, et al. A four-probiotic preparation for ventilator-associated pneumonia in multi-trauma patients: results of a randomized clinical trial. Int J Antimicrob Agent.s (2022) 59:106471. doi: 10.1016/j.ijantimicag.2021.106471
93. Asahara T, Shimizu K, Nomoto K, Hamabata T, Ozawa A, Takeda Y. Probiotic bifidobacteria protect mice from lethal infection with Shiga toxin-producing Escherichia coli O157:H7. Infect Immun. (2004) 72:2240–7. doi: 10.1128/IAI.72.4.2240-2247.2004
94. Davison JM, Wischmeyer PE. Probiotic and synbiotic therapy in the critically ill: State of the art. Nutrition. (2019) 59:29–36. doi: 10.1016/j.nut.2018.07.017
95. Petrof EO, Dhaliwal R, Manzanares W, Johnstone J, Cook D, Heyland DK. Probiotics in the critically ill: a systematic review of the randomized trial evidence. Crit Care Med. (2012) 40:3290–302. doi: 10.1097/CCM.0b013e318260cc33
96. Wang J, Liu KX, Ariani F, Tao LL, Zhang J, Qu JM. Probiotics for preventing ventilator-associated pneumonia: a systematic review and meta-analysis of high-quality randomized controlled trials. PLoS One. (2013) 8:e83934. doi: 10.1371/journal.pone.0083934
97. Jin X, Sin Y, Yuan B, Wang H, Liu H, Chen Y, et al. The effect of early enteral nutrition supplemented with probiotics on ventilator-associated pneumonia in patients with severe stroke. Chin J Microecol. (2019) 31:174–8.
98. Hurley JC. Profound effect of study design factors on ventilator-associated pneumonia incidence of prevention studies: benchmarking the literature experience. J Antimicrob Chemother. (2008) 61:1154–61. doi: 10.1093/jac/dkn086
99. Sanders ME, Benson A, Lebeer S, Merenstein DJ, Klaenhammer TR. Shared mechanisms among probiotic taxa: implications for general probiotic claims. Curr Opin Biotechnol. (2018) 49:207–16. doi: 10.1016/j.copbio.2017.09.007
100. Fatema MD, Patel BK, Patel K, Bhatia M, Lee CN, Moochhala SM. Gut microbiota dysbiosis as a target for improved post-surgical outcomes and improved patient care: a review of current literature. Shock. (2021) 55:441–54. doi: 10.1097/SHK.0000000000001654
101. Mahajan C, Khurana S, Kapoor I, Sokhal S, Kumar S, Prabhakar H, et al. Characteristics of gut microbiome after traumatic brain injury. J Neurosurg Anesthesiol. (2021): doi: 10.1097/ANA.0000000000000789
102. Krumbeck JA, Rasmussen HE, Hutkins RW, Clarke J, Shawron K, Keshavarzian A, et al. Probiotic Bifidobacterium strains and galactooligosaccharides improve intestinal barrier function in obese adults but show no synergism when used together as synbiotics. Microbiome. (2018) 6:121. doi: 10.1186/s40168-018-0494-4
103. Cotoia A, Spadaro S, Gambetti G, Koulenti D, Cinnella G. Pathogenesis-targeted preventive strategies for multidrug resistant ventilator-associated pneumonia: a narrative review. Microorganisms. (2020) 8:821. doi: 10.3390/microorganisms8060821
104. Vincent J-L, Moreno R. Clinical review– scoring systems in the critically ill. Crit Care. (2010) 14:207. doi: 10.1186/cc8204
105. Naqvi IH, Mahmood K, Ziaullaha S, Kashif SM, Sharif A. Better prognostic marker in ICU – APACHE II, SOFA or SAP II! Pak J Med Sci. (2016) 32:1146–51. doi: 10.12669/pjms.325.10080
106. Breslow MJ, Badawi O. Severity scoring in the critically ill: part 1–interpretation and accuracy of outcome prediction scoring systems. Chest. (2012) 141:245–52. doi: 10.1378/chest.11-0330
107. Zimmerman JE, Kramer AA. A history of outcome prediction in the ICU. Curr Opin Crit Care. (2014) 20:550–6. doi: 10.1097/MCC.0000000000000138
108. Spindler-Vesel A, Bengmark S, Fracs F, Vovk I, Cerovic O, Kompan L. Synbiotics prebiotics glutamine or peptide in early enteral nutrition. J Parenter Enteral Nutr. (2006) 31:119–26. doi: 10.1177/0148607107031002119
109. Kotzampassi K, Giamarellos-Bourboulis EJ, Voudouris A, Kazamias P, Eleftheriadis E. Benefits of a synbiotic formula (Synbiotic 2000Forte) in critically Ill trauma patients: early results of a randomized controlled trial. World J Surg. (2006) 30:1848–55. doi: 10.1007/s00268-005-0653-1
110. Bliss DZGP, Settle RG. Defining and reporting diarrhea in tube-fed patients-what a mess! Am J Clin Nutr. (1992) 55:753–9. doi: 10.1093/ajcn/55.3.753
111. Gunalan A, Sistla S, Sastry AS, Venkateswaran R. Concordance between the national healthcare safety network (NHSN) surveillance criteria and clinical pulmonary infection score (CPIS) criteria for diagnosis of ventilator-associated pneumonia (VAP). Indian J Crit Care Med. (2021) 25:296–8. doi: 10.5005/jp-journals-10071-23753
112. de Brito-Ashurst I, Preiser JC. Diarrhea in critically ill patients: the role of enteral feeding. JPEN J Parenter Enteral Nutr. (2016) 40:913–23. doi: 10.1177/0148607116651758
113. Bo L, Li J, Tao T, Bai Y, Ye X, Hotchkiss RS, et al. Probiotics for preventing ventilator-associated pneumonia. Cochrane Database Syst Rev. (2014) 10:CD009066.
114. Parker EA, Roy T, D’Adamo CR, Wieland LS. Probiotics and gastrointestinal conditions: an overview of evidence from the Cochrane Collaboration. Nutrition. (2018) 45:125–134e111. doi: 10.1016/j.nut.2017.06.024
115. Salminen S, Collado MC, Endo A, Hill C, Lebeer S, Quigley EMM, et al. The international scientific association of probiotics and prebiotics (ISAPP) consensus statement on the definition and scope of postbiotics. Nat Rev Gastroenterol Hepatol. (2021) 18:649–67. doi: 10.1038/s41575-021-00440-6
116. Radrizzani D, Bertolini G, Facchini R, Simini B, Bruzzone P, Zanforlin G, et al. Early enteral immunonutrition vs. Parenteral nutrition in critically ill patients without severe sepsis: a randomized clinical trial. Intensive Care Med. (2006) 32:1191–8. doi: 10.1007/s00134-006-0238-y
117. Abdulmeguid AM, Hassan A. Enteral versus parenteral nutrition in mechanically ventilated patients. Neurol Croatica. (2007) 56:15–24.
118. Frohmader TJ, Chaboyer WP, Robertson IK, Gowardman J. Decrease in frequency of liquid stool in enterally fed critically ill patients given the multispecies probiotic VSL#3: a pilot trial. Am J Crit Care. (2010) 19:e1–11. doi: 10.4037/ajcc2010976
119. Malik AA, Rajandram R, Tah PC, Hakumat-Rai VR, Chin KF. Microbial cell preparation in enteral feeding in critically ill patients: a randomized, double-blind, placebo-controlled clinical trial. J Crit Care. (2016) 32:182–8. doi: 10.1016/j.jcrc.2015.12.008
Keywords: critical illness, probiotic, ventilator-associated pneumonia, mechanical ventilation, network meta-analysis
Citation: Li C, Lu F, Chen J, Ma J and Xu N (2022) Probiotic Supplementation Prevents the Development of Ventilator-Associated Pneumonia for Mechanically Ventilated ICU Patients: A Systematic Review and Network Meta-analysis of Randomized Controlled Trials. Front. Nutr. 9:919156. doi: 10.3389/fnut.2022.919156
Received: 13 April 2022; Accepted: 21 June 2022;
Published: 08 July 2022.
Edited by:
Caili Fu, National University of Singapore Suzhou Research Institute (NUSRI), ChinaCopyright © 2022 Li, Lu, Chen, Ma and Xu. This is an open-access article distributed under the terms of the Creative Commons Attribution License (CC BY). The use, distribution or reproduction in other forums is permitted, provided the original author(s) and the copyright owner(s) are credited and that the original publication in this journal is cited, in accordance with accepted academic practice. No use, distribution or reproduction is permitted which does not comply with these terms.
*Correspondence: Jiawei Ma, mjw081X@163.com; Nana Xu, xnn@xzhmu.edu.cn
†These authors have contributed equally to this work and share first authorship