Biochemical and antioxidant activity of wild edible fruits of the eastern Himalaya, India
- ICAR Research Complex for NEH Region, Umiam, India
The eastern Himalayas, one of the important hotspots of global biodiversity, have a rich diversity of wild edible fruit trees. The fruits of these tree species have been consumed by the tribal people since time immemorial. However, there is limited information available on the biochemical and antioxidant properties of the fruits. Therefore, the present investigation was undertaken to study the physico-chemical and antioxidant properties of the nine most important wild fruit trees. Among the species, Pyrus pashia had the maximum fruit weight (37.83 g), while the highest juice (43.72%) and pulp content (84.67%) were noted in Haematocarpus validus and Myrica esculenta, respectively. Maximum total soluble solids (18.27%), total sugar (11.27%), moisture content (88.39%), ascorbic acid content (63.82 mg/100 g), total carotenoids (18.47 mg/100 g), and total monomeric anthocyanin (354.04 mg/100 g) were recorded in H. validus. Docynia indica had the highest total phenolic content (19.37 mg GAE/g), while H. validus recorded the highest total flavonoids and flavanol content. The antioxidant activities of the different fruits ranged from 0.17 to 0.67 IC50 for DPPH activity and 3.59–13.82 mg AAE/g for FRAP. These fruits had attractive pigmentation of both pulp and juice and were a good potential source for the extraction of natural edible color in the food industry. The fruits also possess high market prices; Prunus nepalensis fetched $ 34.10–$ 141.5 per tree. Therefore, these fruits are rich sources of antioxidants, pigments and have a high market value for livelihood and nutritional security.
1. Introduction
The eastern Himalayan region of India has a diverse agro-climate, ranging from tropical to alpine, and receives very high rainfall. The region is an important part of the Indo-Myanmar biodiversity hotspot of the world (1). The diverse agro-climatic conditions of this region offer immense scope for the evolution and development of different wild edible species. Of the 800 wild edible tree species found in India, about 300 are consumed by the hill populace of this region alone. Therefore, the region is considered a reservoir of several crop species, including wild relatives, grown naturally in the forests and also in the backyards of the local tribes. The economy of this region is basically rural based; agriculture and allied sectors play a predominant role. The fruits collected from the forest as well as from their own land are consumed locally and also sold in local markets at a premium price. Recently, several trainings and demonstrations have been conducted by various government agencies to impart knowledge and skills to the local people, resulting in improved resource utilization and entrepreneurial skills. Several value-added products, such as wine, vinegar, jam, jelly squash, RTS, pickles, etc., of these wild fruits are prepared and marketed by self-help groups (SHGs) and entrepreneurs. However, the availability of value-added products on the market is still lacking due to poor commercial production.
These fruit plants are found in tropical, subtropical, and temperate regions of the Indian subcontinent, East Asian nations, South East Asian nations, and European nations, which suggests that they are adaptable to a wider range of environments (Table 1). These fruits have been important constituents of diet and health care and have contributed significantly to the livelihood security of the local people over centuries (2). Locally, these crops are also used to extract natural pigments (3); and ethnobotanical uses of fruits in the treatment of cancer (4); fever, cough, and jaundice, gastrointestinal, respiratory, and cardiovascular (5); anti-obesity (6); cognitive boosting properties, liver health, and reducing fatty liver buildup (7); leaves of Pyrus pashia were used as fodder (8) and the Monpa community of Tawang, Arunachal Pradesh, India, used extracts of P. pashia as butter tea beverages (9). This could be due to the nutraceutical properties of these fruits. It has been proven that ingestion of natural antioxidants from fruit sources, such as polyphenols, have significant anticarcinogenic, antipyretic, anticoagulant, anti-inflammatory, and hypoglycemic properties (10). This might be attributed to the powerful antioxidant capacity of polyphenols and their additives, and their synergistic effects with associated bioactive constituents. Such constituents provide protection to the cellular system against oxidative impairment, which consequently reduces the oxidative stress in the human body (11). In addition, fruit-based natural antioxidants are also fascinating because of their safety and wide applications in the cosmetic, pharmaceutical, and food industries as alternative sources to synthetic antioxidants (12). In spite of these potential applications, research on these crops is at an infant stage, although morphological characterization of some fruit crops, such as Prunus nepalensis, Elaeagnus latifolia, Pyrus pashia, and other wild edible fruits has been described (13–15). However, their nutraceutical properties have not yet been scientifically assessed. Hence, the present study was carried out to determine the biochemical and antioxidant properties of popular wild edible fruit trees grown in the eastern Himalayas of India. The information generated could lead to a better understanding of the potential functional food sources and an increased consumption of these fruits. This, in turn, could have a significant impact on the most vulnerable tribal population’s long-term economic, nutritional, and health system in the near future.
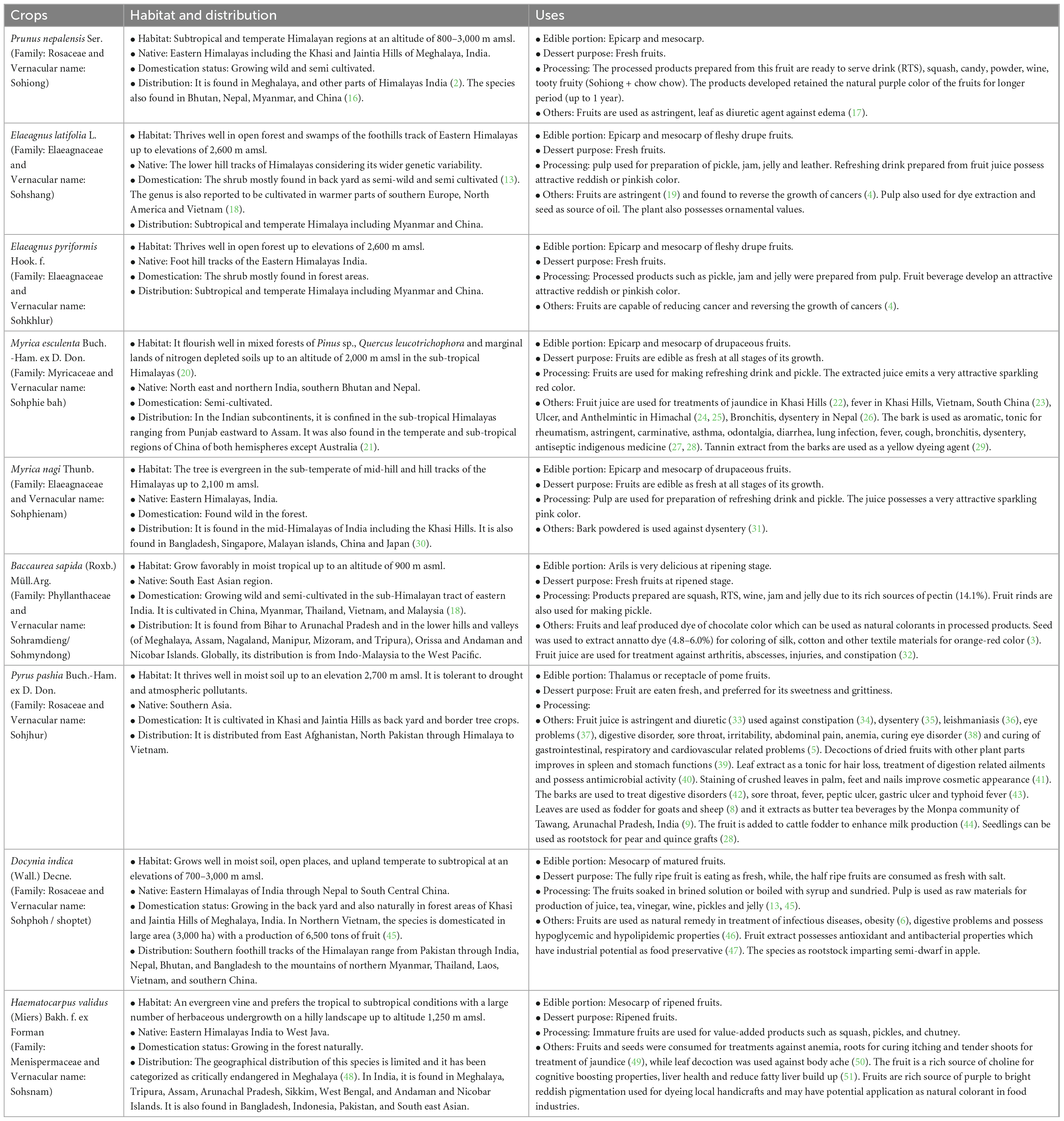
Table 1. Habitat, distribution, and utilization of wild edible fruit crops of the eastern Himalayas, India.
2. Materials and methods
2.1. Materials and experimental site
The fruits of wild edible plant species such as Baccaurea sapida (Roxb.) Müll. Arg., Docynia indica (Wall.) Decne., Elaeagnus latifolia L., E. pyriformis Hook. f., Haematocarpus validus (Miers) Bakh. f. ex Forman., Myrica esculenta Buch. -Ham. ex D. Don., Myrica nagi Thunb., Prunus nepalensis Ser., and Pyrus pashia Buch.-Ham. ex D. Don. grown in the forests and/or backyards, were collected for the study (Figure 1). The collection was made from various locations in the region, particularly the Khasi Hills, Jaintia Hills, Ri Bhoi, and Garo Hills, distributed between 20.1–26.5°N latitude and 85.49–92.52°E longitude with altitude ranging from 100 to 2,000 m amsl (Figure 2). The collected fruits were analyzed for different biochemical and functional attributes at the ICAR Research Complex for the North Eastern Hill Region, Umiam, Meghalaya, India, during 2019–2020.
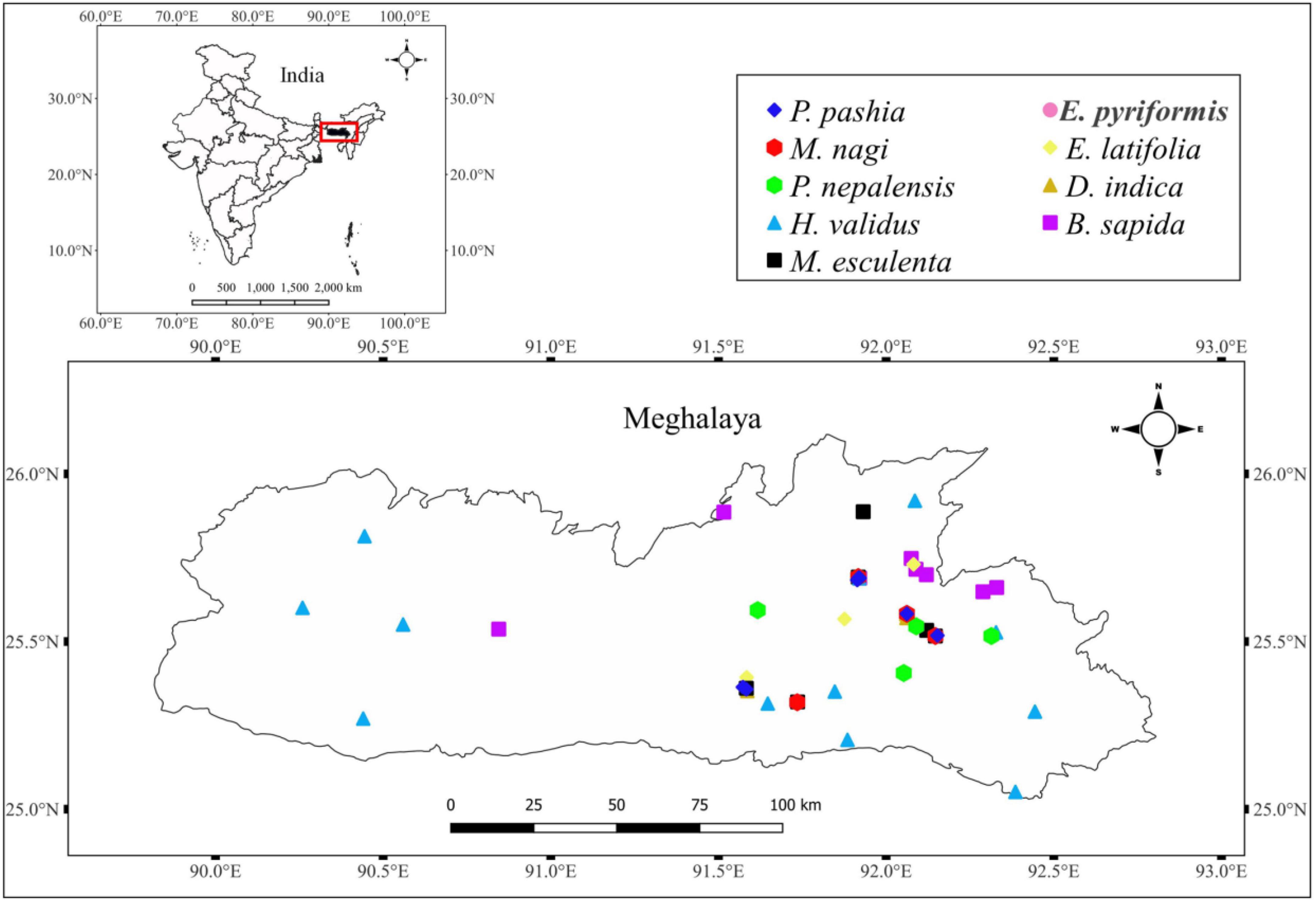
Figure 2. Collection sites of wild edible fruits grown in the eastern Himalayas, India (Generated by subjected the global positioning system (GPS) data to quantum geographic information system (QGIS) version 3.20.1).
2.2. Quantitative analysis
Twenty-five ripe fruits of each species were used for carrying out all the physical and biochemical analyses. Fruit samples were harvested at an appropriate maturity. The harvested fruits were washed with distilled water, wiped with tissue paper, and kept at room temperature for 10 min to remove the adhering water before analysis. The parameters, viz., fruit, and seed weights, were determined using an electronic balance (Adair Dutt-1620C). Fruit length and diameter were measured using a digital caliper (Code 1108-150). The pulp recovery percentage was estimated using the following formula:
2.3. Determination of biochemical attributes
Biochemical parameters such as total soluble solids (TSS) were determined using a hand-held refractometer (HI 96801) and titratable acidity, ascorbic acid, reducing sugars, and total sugars were analyzed according to Rangana (52). The moisture content of the fruits was determined gravimetrically as per the method of Akter et al. (53) and Raaf et al. (54). The fresh fruit samples were weight before and after drying in a hot air oven (thermostatically controlled, Model–IC7). About 20 g finely shredded fresh sample was placed in a clean and dried crucible with a cover, and accurately weighed on an electronic weighing balance (Model–AUX220). The samples were dried in the oven at 105°C for 24 h or until a constant weight was achieved for two consecutive weights. Following drying, the crucible was cooled in a desiccator. The moisture content (MC) was calculated as below and expressed as a percentage.
Where W1–fresh weight
W2–dried weight
2.4. Measurement of total carotenoids
Total carotenoids were determined as per the method of Chen et al. (55). The extraction of carotenoids was carried out according to the method developed by Chen et al. (55). Pulp (10 g) was placed in a vessel, protected from light, and mixed with 50 mL of extraction solvent (hexane/acetone/ethanol: 70:15:15, v/v/v). The mixture was stirred for 1 h using an orbital shaker. About 5 mL of a 40% KOH in methanolic solution were added, and the solution was saponified at 25°C in the dark for 2 h. Subsequently, 30 mL of hexane were added, the mixture was shaken vigorously, and the upper layer was collected. The lower layer was extracted twice, and the supernatant was also collected and filtered through sodium sulfate powder to remove traces of water. The supernatant obtained was pooled and stored at −80°C under a nitrogen atmosphere (99.9% purity) in the dark until analysis. The total carotenoid content of the extracts was measured using a UV-Visible spectrophotometer at 450 nm. A calibration curve (0–50 ppm) was prepared using β-carotene as the standard and the results were expressed as mg β-carotene/100 g sample.
2.5. Determination of functional attributes and antioxidant activity
2.5.1. Preparation of fruit extract
The pulp (5 g) of each fruit was grinded, and 50 mL of aqueous methanol was added at ambient temperature. The mixture was incubated for 1 h at room temperature with continuous magnetic stirring at 200 rpm and centrifuged at 1,000 g for 20 min. The supernatant was collected and stored at −20°C until analysis. The aliquot was used for assessments of total phenolic content, total monomeric anthocyanins, total flavonoids, total flavonol, DPPH free radical scavenging capacity, and FRAP reducing power.
2.5.2. Determination of total phenolic content
The crude extracts were estimated for total phenolic content using the Folin–Ciocalteu procedure as per the method of Singleton and Rossi (56). About 1 mL of the extract was transferred to 2 mL of Folin–Ciocalteu reagent (1:10 v/v distilled water). After 10 min, 1.6 mL (7.5%) of sodium carbonate was added. The mixture was vortexed for 15 s before being left to stand for 30 min at room temperature to develop its color. The absorption was measured at 743 nm in a UV-visible spectrophotometer (Model: UV 3200). The concentration of polyphenols in samples was derived from a standard curve of Gallic acid, and the total phenolic content was expressed as Gallic acid equivalents (GAE) in mg/g of pulp.
2.5.3. Determination of total monomeric anthocyanin content
Total monomeric anthocyanin was determined as per the procedure of Giusti and Wrolstad (57); Lako et al. (58). About 0.4 mL of the extract solution was taken, and 3.6 mL of the corresponding buffer; pH 1.0 buffer (potassium chloride 0.025 M) and pH 4.5 buffer (sodium acetate, 0.4 M) was added. The absorbance of each solution was taken against a blank in a cuvette with a 1 cm path length at 510 nm and 700 nm using a UV-Visible spectrophotometer. Total monomeric anthocyanin pigment concentration was expressed as cyanidin-3-glucoside equivalents (mg cyd-3-gluE/100 g) as follows:
Where A = (A510nm – A700nm) pH 1.0 – (A510nm – A700nm) pH 4.5
MW (molecular weight) = 449.2 g/mol for cyanidin-3-glucoside (cyd-3-glu)
DF = dilution factor established in D
l = pathlength in cm
ε = 26,900 molar extinction coefficients for cyd-3-glu; and 1,000 = factor for conversion from g to mg.
2.5.4. Measurement of total flavonoids
The total flavonoid content of extracts was estimated using Aluminum chloride (AlCl3) colourimetric assay as previously described by Zhishen et al. (56). About 0.3 mL of 5% NaNO2 was added to 1 mL extract. After 5 min, 0.3 mL of 10% AlCl3.6H2O was added, and incubated for 5 min. About 2 mL NaOH (1M) was added, and the final volume of the solution was adjusted to 5 mL with distilled water. After 15 min of incubation, the mixture turned to pink and the absorbance was measured at 510 nm (UV-visible spectrophotometer, Model: UV 3200). Total flavonoid content was presented as mg quercetin equivalent per gram (mg QE/g).
2.5.5. Determination of total flavonols
Total flavonols in the fruit sample extracts were determined according to the method of Miliauskas et al. (59). 2 mL of 2% AlCl3 and 6 mL (5.0%) sodium acetate solutions were added to 2.0 mL of extract. The mixture was incubated at 25°C for 2.5 h and absorption at 440 nm (UV-visible spectrophotometer, Model-UV 3200) was read. Total flavonol content was expressed as quercetin equivalent (mg QE/g).
2.5.6. Measurement of DPPH free radical scavenging activity
The free radical scavenging activity of the fruit extracts was estimated with the DPPH (1, 1-diphenyl-2- picrylhydrazyl) method (60). Ascorbic acid was used as a reference standard. 100 μL of aliquot was transferred to test tubes, to which 3.9 mL of freshly prepared DPPH solution (25 mg/L in methanol) were added. The mixtures were then thoroughly mixed and allowed to stand for 30 min. The absorbance was measured at 517 nm (UV-visible spectrophotometer, Model: UV 3200). The percent scavenging activity of DPPH was calculated using the following formula:
Where, Ac is the absorbance of the control reaction and At is the absorbance of the sample of the extracts. The antioxidant activity of the extract was expressed as IC50 (the concentration of fruit sample required to decrease the absorption at 517 nm by 50%). The IC50 value was expressed as the concentration in milligram of extract per mL that inhibited the formation of DPPH radicals by 50%.
2.5.7. Measurement of FRAP reducing power
The reducing power of the extracts was assessed as per the method of Oyaizu (61). About 100 μL of fruit extracts were mixed with phosphate buffer (2.5 mL, 0.2 M, pH 6.6) and 1% potassium ferricyanide (2.5 mL). This mixture was incubated at 50°C for 20 min, to which 2.5 mL aliquots of trichloroacetic acid (10%) was added. The content was centrifuged at 3,000 rpm for 10 min. The upper layer of the solution (2.5 mL) was extracted and mixed with 2.5 mL of distilled water and 0.5 mL of freshly prepared ferric chloride solution (0.1%). Then the measurement of absorbance was recorded at 700 nm (UV-visible spectrophotometer, Model: UV 3200) and the reducing power was expressed in terms of ascorbic acid equivalent (AAE) in milligram per gram of extract (mg AAE/g).
2.6. Color, season of availability and market price of fruits
Color measurements of ripened fruits of different fruit tree species were carried out using a Color Hunter meter (HunterLab Color Quest XE). The instrument was calibrated using the black and white tiles. The value was expressed as L* values indicated lightness (black, L* = 0 and white, L* = 100), a* values indicated redness-greenness (red, a* = 100 and green, a* = −100), b* values indicated yellowness-blueness (yellow, b* = 100 and blue, b* = −100). The observation was replicated thrice for each sample. Observations were taken at the base, middle, and apex of fruits at an equidistant space under the aperture of the color meter. Through image analysis, an Android application (Color Grab version 3.9.2) was used to determine the color of fruit juice. A local market survey in Shillong city and 10 weekly markets in Khasi and Jaintia Hills were conducted. Informants (60 no.) were randomly selected among the local vendors and farmers for data collection on period of fruit availability in the markets and the market price of fruits. The selection of key informants was done with the help of village workers and elders as per the ethnoecological methods of Martin (62). The yield of fruits per tree was determined by counting the number of fruits per tree at harvest and multiplying it by its fruit weight, expressed in kg per tree.
2.7. Statistical analysis
The replicated (three of each parameter) data were analyzed using statistical package for the social sciences (SPSS) (Version 14.0) software, and the data were presented as mean ± SE using one-way ANOVA (p < 0.05) of Tukey’s HSD (honestly significant difference) test. The possible relationship between antioxidant compounds and antioxidant activity was analyzed through Pearson’s correlation coefficient. Using quantum geographic information system (QGIS) version 3.20.1, a map of the collection sites was created subjecting the global positioning system (GPS) data.
3. Results and discussion
3.1. Physico-chemical characteristics
The biochemical traits of fruits contribute to the consumer’s perception of quality traits, including those associated with taste, mouth feel, and appearance. The results revealed a significant variation among the fruit morphological and biochemical characteristics of different wild edible fruit species (p < 0.05, Table 2). The maximum fruit length ranged from 4.38 cm in H. validus to 1.52 cm in M. nagi; fruit diameter (1.23 cm in M. nagi to 4.39 cm in P. pashia); fruit circumference (12.38 cm in P. pashia to 3.64 cm in M. nagi); fruit weight (7.32 g in E. pyriformis to 37.83 g in P. pashia); fruit volume (39.89 cm3 in P. pashia to 7.32 cm3 in E. pyriformis); juice content (21.22% in M. nagi to 43.72% in H. validus) and pulp content (56.69% in B. sapida to 84.67% in M. esculenta). The significant differences in fruit physical characteristics indicated greater variability among fruit crops. The maximum fruit weight was observed in P. pashia, followed by D. indica, H. validus, and E. latifolia; juice content was recorded in H. validus, followed by M. esculenta, B. sapida, and E. pyriformis; and pulp content (>70%) in M. esculenta, followed by M. nagi, P. pashia, P. nepalensis, D. indica, and H. validus. Similarly, there was a significant difference (p < 0.05) among fruits for biochemical attributes as given in Table 3. The moisture content was the highest in H. validus (88.39 ± 1.85%) and the lowest in P. pashia (73.75 ± 1.88%). The determination of moisture content in food is considered to be one of the most important assays since moisture greatly influences the physical properties and stability of the food (63). The total soluble solids (TSS) was the maximum in H. validus (18.27 ± 1.49%) and the minimum in M. esculenta (5.83 ± 0.30%). The titratable acidity was the highest in M. esculenta (3.32 ± 0.06%), followed by E. latifolia (2.68 ± 0.04%) and the lowest in P. pashia (0.31 ± 0.03%). Total sugar ranged from 3.26 ± 0.05% in E. latifolia to 11.27 ± 1.26% in H. validus. Reducing sugar content ranged from 1.32 ± 0.03% to 7.38 ± 0.54%, the minimum was recorded in E. latifolia and the maximum in H. validus. Our results indicated that the fruits of H. validus, P. nepalensis, B. sapida, E. latifolia, M. esculenta, and D. indica contained higher levels of TSS and acidity. TSS and acidity are the two important factors for determining the quality traits in a fruit, which also influence the taste, sweetness, and also act as an indicator of the maturity of the fruit and its suitability for processing. This was indicated by a strong relationship between TSS and total sugar (0.711**), ascorbic acid (0.838**), total monomeric anthocyanin (0.732**), total carotenoids (0.407*), total flavonoids (0.479**), and total flavonol (0.532**). Similar observations have been reported by Canan et al. (64). Hence, the fruits rich in TSS and acidity were found suitable for fresh consumption as well as processing and value addition (65), and can be promoted for different value-added products such as ready to serve (RTS), wine, etc. as a cottage industry.
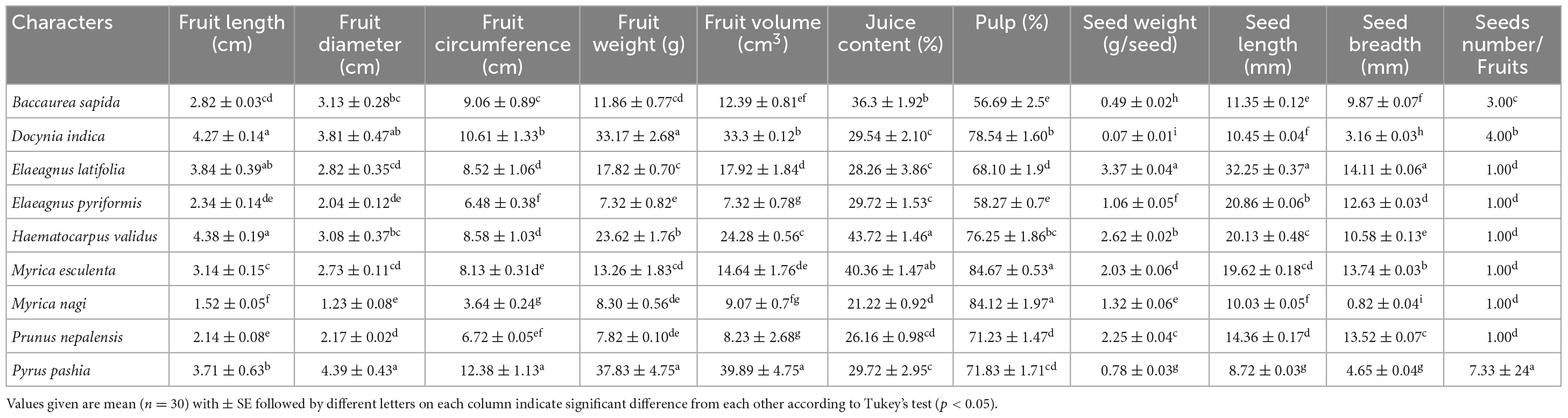
Table 2. Fruits and seed physical characteristics of promising wild edible fruits grown in the eastern Himalayas, India.
3.2. Functional attributes and antioxidant activity
3.2.1. Ascorbic acid content
Ascorbic acid is regarded as the most important antioxidant vitamin. However, it cannot be synthesized by humans due to the lack of gulonolactone oxidase enzyme, and a deficiency of dietary ascorbate results in clinical syndrome and scurvy (66). Hence, supplementing the diet with ascorbic acid-rich foods is very vital. In this study, the ascorbic acid content of wild edible fruits had significant variations (Figure 3A). The highest ascorbic acid content was recorded in H. validus (63.82 mg/100 g pulp) and the lowest in P. pashia (9.62 mg/100 g pulp). These results agree with the reports of Contreras-Calderón et al. (67) on the variability of vitamin C content in several wild edible fruits. Furthermore, the finding demonstrated that these wild edible fruits have a higher vitamin-C content than commercially available major fruits: Citrus sinensis (10.13 ± 0.10 mg/100 g), Ananas comosus (6.40 ± 0.18 mg/100 g), Malus domestica (7.94 ± 0.13 mg/100 g), and Prunus persica (5.92 ± 0.12 mg/100 g). However, they had a lesser content than the richest known sources of vitamin C, such as Psidium guajava (198.05–221.47 mg/100 g), Phyllanthus emblica (375.68 mg/100 g), and Emblica officinalis (756.32 mg/100 g) (68–70). Our results showed that the ascorbic acid content of E. latifolia was lower than that reported from Sikkim by Dasila and Singh (71). This variation may be attributed to different analytical methods, as reported by Dias et al. (72). The E. pyriformis reported in this study had a lower ascorbic acid content than that reported from Manipur (20.10 mg/100 g) by Khomdram et al. (70). The reason for variations may be due to the unique genetic make-up among genotypes and environmental factors (73), and differences in soil physico-chemical attributes such as pH, nutrients, and agro-ecology (74). A variation in the pH of the soil is known to determine the availability of nutrients to the roots and their uptake, which could be influenced by soil geology and climatic factors (75). A significant positive correlation of ascorbic acid with total soluble solids (0.838**) and total sugar (0.784**) was observed (Table 4). A high positive correlation of ascorbic acids with sugars was due to the recurring and elaborate interactions between organic acids and sugars (76), which may be associated with the synthesis of ascorbic acid from glucose (77). Ascorbic acid also showed a significant negative correlation with total antioxidant activity (−0.397*) which was represented by the IC50 of DPPH and analyzed by Pearson’s correlation coefficient (r). It is well-established that lower IC50 values indicate high antioxidant activity (78). Therefore, an increase in the ascorbic acid content will enhance the antioxidant activity of these fruits, as shown by the lower IC50 of DPPH value. Our findings suggested that ascorbic acid may be one of the factors contributing to antioxidant properties, as evidenced by their positive relationship in a variety of other food sources (79). Hence, the daily consumption of these fruit crops will enrich the diet and act as an additional or alternative source of ascorbic acid.
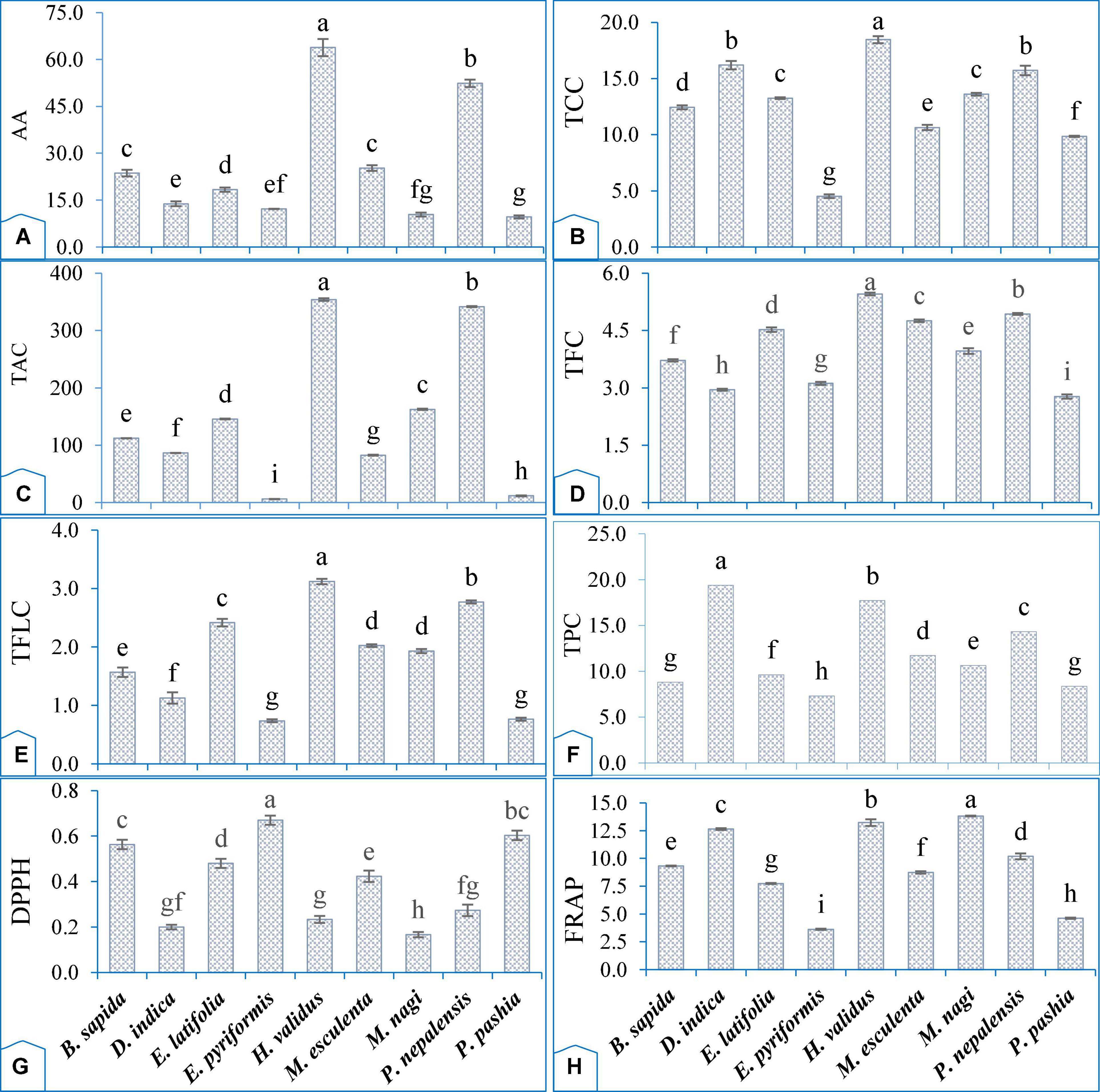
Figure 3. Functional attributes of wild edible fruits grown in the eastern Himalayas, India. (A) Ascorbic acid content (AA, mg/100 g fw); (B) total carotenoids content (TCC, mg/100 g fw); (C) total monomeric anthocyanins content (TAC, mg/100 g fw); (D) total flavonoids content (TFC, mg QE/g); (E) total flavonol content (TFLC, mg QE/g); (F) total phenol content (TPC, mg GAE/g); (G) DPPH antioxidants capacity (DDPH, IC50 value mg/mL); (H) FRAP antioxidants capacity (FRAP, mg AAE/g) content in wild edible fruits. IC50 ascorbic acid (0.012 ± 0.002). Mean value of three replications (each replication consisted 10 fruits) with ± S.E followed by different letters on each bar indicate significant difference from each other according to Tukey’s test (p < 0.05).
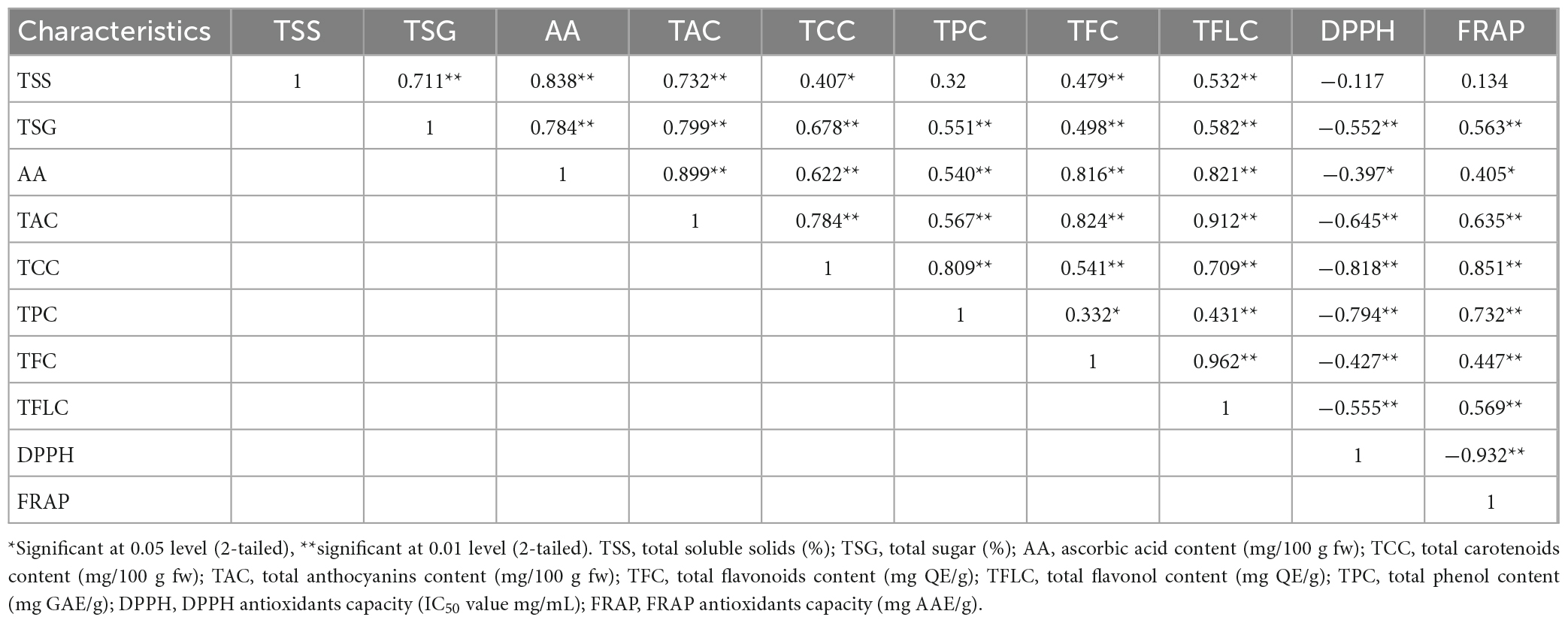
Table 4. Pearson’s correlation coefficient (r) among biochemical and antioxidant activities of wild edible fruits grown in the eastern Himalayas, India.
3.2.2. Total carotenoid content
Carotenoids as antioxidant compounds are known to be present in several fruit crops, and the dietary intake of carotenoid-rich foods has been reported to retard cancer, cardiovascular disease, and several other ailments in humans (80). Results showed that the total carotenoids content of different wild edible fruit species varied significantly (p < 0.05; Figure 3B). H. validus recorded the highest total carotenoids (18.47 mg/100 g pulp), followed by D. indica, and the lowest total carotenoids (4.52 mg/100 g pulp) were noted in E. pyriformis. The fruits of H. validus, D. indica, and P. nepalensis contain higher total carotenoids than mangoes [4,926.76–14,942.46 μg/100 g fw, (81)] and cashews [0.4 mg/100 g fw, (82)]. The variations in genetic make-up among the species may be the cause of the variations in total carotenoids. Dias et al. (72) have also reported the great influences of varieties, maturity, cultural management, environment, postharvest care, storage conditions, and analytical methods on the formation of secondary metabolites, including the total carotenoid content in fruit crops. The high carotenoid content of these fruits is an important indicator of their quality and high nutritional value (83). In addition, our study also found a strong negative correlation between total carotenoid content and DPPH (−0.818**). The presence of high level of total carotenoids in the fruits of H. validus, P. nepalensis, D. indica, B. sapida, E. latifolia, and M. esculenta indicates their powerful ability to scavenge oxygen free radicals and active oxygen. The previous study (84) revealed that carotenoid scavenging ability would increase due to an increase in the lipophilicity of carotenoid. Lycopene was effective in reducing Fe (III) to Fe (II), given the fact that lycopene contains 11 conjugated double bonds (84). Although lycopene content was not analyzed in our study, Dasila and Singh (71) found that it was 2.5 times higher in E. latifolia (2.06 ± 0.38 mg/100 g) than β-carotene (0.83 ± 0.02 mg/100 g). Our results also indicated that the a* value (redness) of the peel (31.5 ± 3.90a) and juice (23.4 ± 1.9a) of E. latifolia were the highest among these wild edible fruits (Table 5). It is well established that the red color of certain fruits and vegetables, such as tomato, pink grapefruit, red grapes, watermelon, and red guava, is due to the presence of lycopene (85). Therefore, lycopene may be one of the major pigments responsible for the red color in the fruits of E. latifolia.
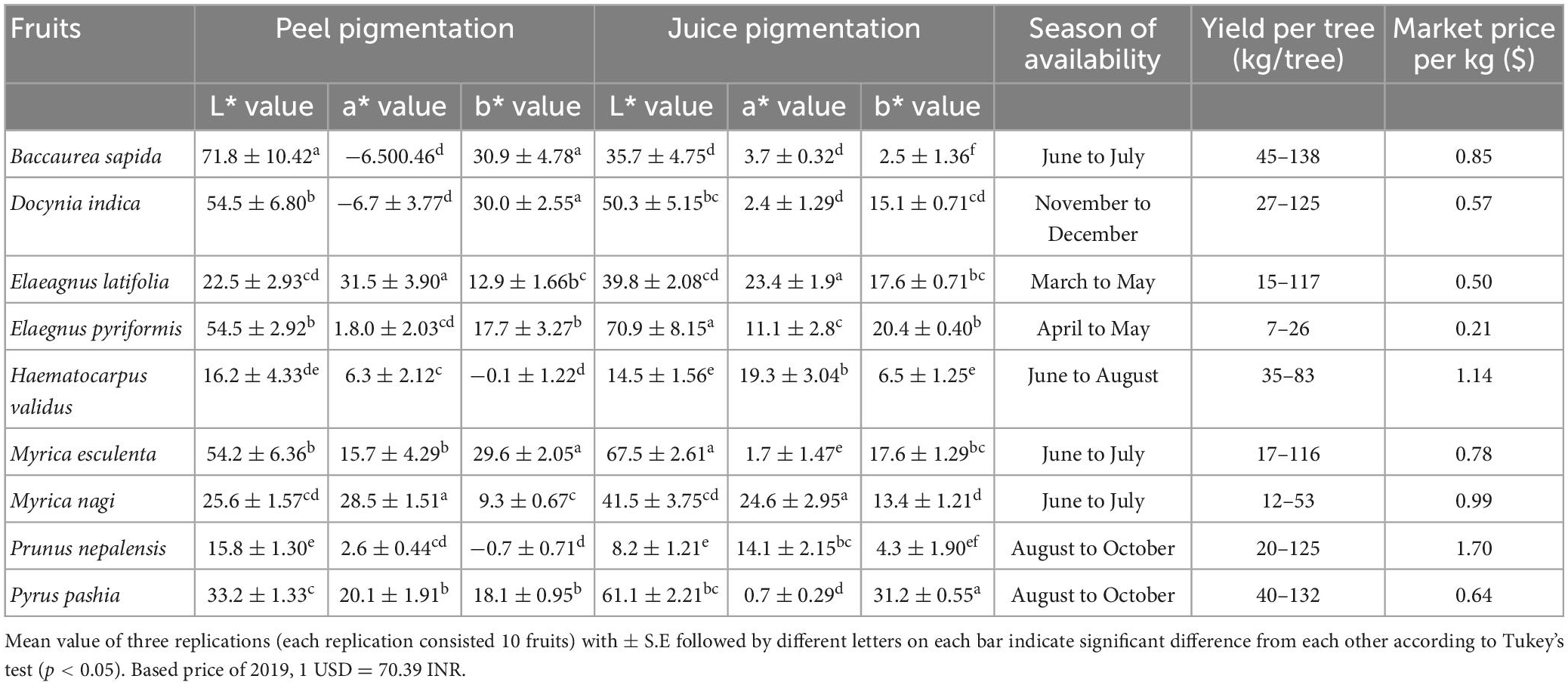
Table 5. Color, season of availability and market price of wild edible fruits grown in the eastern Himalayas, India.
3.2.3. Total monomeric anthocyanin content
Anthocyanins are water-soluble and vacuolar pigments found in most species in the plant kingdom. Its accumulation mostly occurs on flowers and fruits, which impart an attractiveness to the fruit, hence; it is considered a color indicator and a natural colorant (86). It also plays a role in preventing, ameliorating, and scrubbing oxidative stress, thus retarding several diseases and physiological malfunctions (87). A significant variation (p < 0.05) in total monomeric anthocyanin content was observed among wild edible fruits (Figure 3C). The highest total monomeric anthocyanin content was recorded in H. Validus (354.04 mg/100 g), followed by P. nepalensis (341.70 mg/100 g) and the lowest was found in E. pyriformis (6.02 mg/100 g). These wild fruits contain more total monomeric anthocyanin than commercial fruit cultivars such as sweet cherry cv. Black Gold [44.19 ± 1.38 mg/100 g fw, (88)], red currants (12.14 ± 0.87 mg/100 g fw), black currant (287.78 ± 0.08 mg/100 g fw) (89), purple tomato [20.73 ± 2.86 mg/100 g fw, (90)], and guava [0.40–0.69 mg/100 g, (82)]. The varied total anthocyanin levels between species indicate genetic variations in the synthesis of these bioactive substances. Horbowicz et al. (86) have also reported the considerable variation in anthocyanin content of the fruits among different species or cultivars within the same species. This difference in anthocyanin content among these fruits might be due to the effects of genetics, agro-ecological conditions such as pH, light, temperature, and horticultural practices (91). In our study, it was also observed that the fruits with higher anthocyanin content, such as H. Validus (−0.7 ± 0.71) and P. nepalensis (−0.1 ± 1.22) had the lowest b* value (Table 5). Similarly, the lowest L* values were recorded in the darkest colored fruits (H. Validus, 16.2 ± 4.33 and P. nepalensis, 15.8 ± 1.30). In the previous study by Muzolf-Panek and Waskiewicz (92), it was revealed that the effect of variety was predominant in fruit peel color and that the darkest table grapes had the lowest L* values, indicating blue-black and violet-black peel in varieties of table grapes. According to Ponder et al. (93), anthocyanins are responsible for the specific dark blue color of fruit berries, and the darker the fruit, the more anthocyanins it contains. Therefore, the dark purple and blue fruit color of H. validus and P. nepalensis might be due to their high anthocyanin content. Furthermore, a significant inverse relationship (−0.645**) between total monomeric anthocyanin content and DPPH demonstrated their high antioxidant properties. Similarly, Katiresh et al. (94) found that anthocyanins in Sesbania sesban had high antioxidant activity and were effective at scavenging free radical DPPH. Structurally, monomeric anthocyanin possesses loose structures that are easier to undergo oxidation and thus will exhibit better antioxidant activity compared to non-monomeric anthocyanin (95). This is also in agreement with Castaneda-Ovando et al. (96), who claimed that the molecule that donates a free electron (ionization potential) or hydrogen atoms (bond dissociation energy) to the reactive free radicals is often the best antioxidant, and increasing the stability of the anthocyanin will reduce its antioxidant stability. As a result, consuming fruits with high concentrations of these compounds may provide protection to the body against various illnesses (97).
3.2.4. Total flavonoids and flavonols
Flavonoids and flavonols are naturally occurring phenolic compounds found in fruits, vegetables, and/or medicinal plants. They have significant biological effects and exhibit promising antioxidant activity due to their ability to effectively scavenge reactive oxygen species. Dietary flavonoids are recognized for their antioxidant potential, antiproliferative effects, and protective effects on lipids and vital cells against oxidative damage. These properties also play a significant role in the prevention of cardiovascular disease, inflammation, and antiproliferative or anticancer activities (98). A significant variation was also recorded in total flavonoids and flavonol content among different wild fruit species (p < 0.05, Figures 3D, E). Total flavonoid content values ranged from 2.77 ± 0.06 mg QE/g (P. pashia) to 5.46 ± 0.04 mg QE/g (H. validus). Similarly, total flavonol content also varied significantly among the studied fruits, being the maximum in H. validus (3.12 ± 0.05 mg QE/g) and the minimum in E. pyriformis (0.74 ± 0.03 mg QE/g). These fruits contained a higher level of flavonoids than most of the plants reported by Fouad et al. (99) and also higher concentrations of flavonols than Prunus mahaleb [1.24 ± 0.06 g/kg, (100)]. This variation in total flavonoids and flavonol content among different fruit species could be due to various intrinsic and extrinsic factors, such as genetic and environmental factors. Our results revealed a strong negative correlation of total flavonoids content (−0.794**) and total flavonol content (−0.427**) with DPPH content, which indicates that flavonoids and flavonols play an important role in the antioxidant activity of these fruits. Our study is in line with that of Chandra et al. (101), who reported that 32% of the antioxidant activity in crops was contributed by flavonoids, which constitute a major group of antioxidant compounds and act as primary antioxidants (102). The redox properties of total flavonoids were due to the unique positions of OH ortho (C-3′ and C-4′) and oxo functional groups (C-4) in flavonoids (103). Therefore, fruit trees such as H. validus, M. esculenta, B. Sapida, M. nagi, E. latifolia, D. indica, and P. nepalensis are rich in flavonoids and flavonol content, suggesting their consumption can help people meet their nutritional needs and protect them from developing a variety of degenerative diseases.
3.2.5. Total phenolic content
Plant-derived phenolic compounds are a diverse group of secondary metabolites that interact with reactive oxygen species to prevent oxidative damage, thereby aiding plant defense mechanisms and protecting humans from a variety of degenerative diseases (104). Our results indicated the presence of significant variations (p < 0.05) in total phenolic content among fruits in the following descending order: D. indica (19.37 ± 0.07 mg GAE/g) followed by H. validus, P. nepalensis, M. esculenta, M. nagi, E. latifolia, B. sapida, P. pashia, and E. Pyriformis (7.32 ± 0.11 mg GAE/g) (Figure 3F). Interestingly, fruits like D. indica, H. validus, P. nepalensis, M. esculenta, M. nagi, and E. latifolia had higher total phenolic content than the commercial crops of the region, such as pineapple (47.9 mg GAE/100 g), banana (7.2 ± 0.5–18.9 ± 1.4 mg GAE/g dw), and papaya (57.6 mg GAE/100 g) (105, 106). These fruits are comparable with the known richest sources of total phenolic content, such as Aonla (944.85–4,969.50 mg/100 g pulp), which are grown locally (107). According to Robards et al. (108), phenolic compounds exhibit heterogeneity in distribution and concentration across and within plant species. Furthermore, the higher phenol accumulation in the fruits under our study might depend on several factors, viz., agroclimatic conditions, organ, plant developmental stage, and their interaction with the genotype (10). The presence of different concentrations of sugars, carotenoids, or ascorbic acid, as well as extraction methods, may influence the amount of phenolics (109). Czyczyło-Mysza et al. (110) also suggested the importance of both additive and epistatic gene effects on total phenolic content in species, which affect other adaptation traits of the species. Total phenolic content had a positive correlation with total sugar (0.551**), ascorbic acid, DPPH (−0.794**), total monomeric anthocyanin, total carotenoids, total flavonoids, and total flavonol. According to Fitriansyah et al. (111), if the r value is −0.61 ≤ r ≤ −0.9723, it showed a high negative correlation, which indicates that TPC had a strong negative correlation with DPPH. It is well established that phenolic compounds are important plant constituents with redox properties responsible for antioxidant activity. The higher the TPC, the greater is the total antioxidant activity of these fruits as demonstrated by low IC50 of DPPH. Our study exhibits that TPC was one of the major contributory compounds for antioxidant activity, which was also confirmed by Nariya et al. (112) for their scavenging ability due to their unique hydroxyl groups. This indicates that these wild food resources are highly nutritious and rich sources of bioactive compounds, and their consumption will further help improve nutrition.
3.2.6. DPPH free radical scavenging activity
The free radical chain reaction is widely accepted as the most important mechanism of lipid peroxidation. Radical scavengers terminate the peroxidation chain reaction by directly counteracting and quenching peroxide radicals. The capacity of polyphenols to transport labile H atoms to radicals is a probable mechanism of antioxidant protection, which can be assessed universally and rapidly using DPPH. Furthermore, DPPH is the most common and cost-effective way to determine the free radical scavenging capacity of natural products, which are major factors in biological damage caused by oxidative stress (113). Our results revealed a significant variation (p < 0.05) in DPPH free radical scavenging activity among the studied fruits (Figure 3G), and it ranges from (0.17 ± 0.01 IC50 mg/mL) in M. nagi to 0.67 ± 0.02 IC50 mg/mL in E. pyriformis. The present results showed lesser values than those recorded in commercial fruits such as grapes (0.79 ± 0.34 IC50 mg/mL), pineapple (0.83 ± 0.24 IC50 mg/mL), and guava (1.71 ± 0.61 IC50 mg/mL) (79). The antioxidant capacity of fruits and vegetables was influenced by factors such as genetic makeup, maturity, and other environmental factors such as sunlight exposure, soil, and the gene-environment interaction (10). According to Matuszewska et al. (78), the lower IC50 values of DPPH indicate a high level of antioxidant activity, which means that these fruits, viz., M. nagi, D. indica, H. validus, and P. nepalensis with a low IC50 value can scavenge the DPPH radicals to form a stable reduced DPPH molecule. The high accumulation of total sugar, ascorbic acid, total monomeric anthocyanin, total carotenoids, total phenolics, total flavonoids, and total flavonol content increases the antioxidant activity, as demonstrated by the lower IC50 of DPPH value in our result, which agreed with the finding of Sundaramoorthy and Packiam (114). Therefore, the high antioxidant activity of these fruits might be due to a strong negative correlation of different compounds with IC50 DPPH. Previous studies have also found that the antioxidant activity in plant tissue was mainly due to the unusual redox properties of not just one particular compound but also of different bioactive compounds including TPC, tannin, anthocyanin, TFC, phenols, alkaloids, and pro-anthocyanins (115, 116). The antioxidant effect is due to the ability of compounds in the plant extract to transfer electrons or hydrogen atoms to neutralize radicals of DPPH and form neutral DPPH molecules (117). Hence, it is clear from our results that these fruit crops had a greater potential for radical scavenging compounds with proton-donating abilities.
3.2.7. FRAP reducing power
FRAP antioxidants capacity is a simple and inexpensive assay that offers a putative index of the potential antioxidant activity of plant materials. Principally, the FRAP assay treats the antioxidants in the sample as reductants in a redox-linked colourimetric reaction. The reducing power assay, i.e., the transformation of Fe3+ to Fe2+ in the presence of either the extract or the standard (ascorbic acid), is a measure of reducing capability (79). A significant variation (p < 0.05) of FRAP reducing power was observed among the underutilized fruits studied, and it ranged from 3.63 ± 0.05 mg AAE/g in E. pyriformis to 13.82 ± 0.04 mg AAE/g in M. nagi (Figure 3H). These results indicated higher FRAP values in these fruits than in the other wild fruits reported (0.0518 ± 0.49 to 0.111 ± 0.00 mg AAE/g) by Mahadkar et al. (118) from central India. The differences in antioxidant content between species may be due to genetics and environmental factors, as well as their interactions. Our result showed a strong positive correlation of FRAP value with total sugar (0.563**), ascorbic acid (0.405*), total monomeric anthocyanin (0.635**), total carotenoids (0.851**), total phenolics (0.732**), total flavonoids (0.447**), total flavonol (0.569**), and inversely related to DPPH (IC50, −0.932**) (Table 4). The finding that the DPPH and FRAP assays of fruit extracts were highly correlated agrees with the work of Szydłowska-Czerniak et al. (119) and is consistent with the view that the two assays share a similar mechanistic basis, viz., transfer of electrons from the antioxidant to reduce an oxidant, as proposed by Huang et al. (120). The total carotenoids and total phenolic content were the major compounds contributing to the antioxidant activity in our study. Previous report in amla fruits (Emblica officinalis Gaertn) showed that carotenoids had reduction potential lower than 0.44 V, allowing them to reduce Fe (III) to Fe (II) while also being oxidized and acting as antioxidants (84). Similarly, the phenolic compounds largely contribute to the antioxidant activities of these species and therefore could play an important role in the beneficial effects of these fruits. Several studies have found that phenolic compounds are major antioxidant constituents in selected plants and that there are direct relationships between their antioxidant activity and total phenolic content (103). The antioxidant properties of phenolic compounds are directly linked to their unique structure, which allows them to act as reducing agents, hydrogen donors, and singlet oxygen quenchers (121). This demonstrated that most of these underutilized fruits have strong reducing capabilities as compared to other fruit crops, which might be due to the presence of high total carotenoids, total phenolic content, and other functional compounds that are responsible for their antioxidant activity (122). In general, among the fruits, M. nagi showed relatively stronger FRAP activity than other fruits.
3.3. Color, season of availability and market price of fruits
These fruits have appealing pigments, both in the peel and juice, as evidenced by a significant variation in value of L*, a* and b* in both peel and juice color (p < 0.05, Table 5). B. sapida had the highest peel L* value (71.80 ± 10.42a) and b* value (30.90 ± 4.78a), while E. latifolia had the highest peel a* value (31.50 ± 3.90a). Similarly, the yellowness and redness of these fruits were found to be higher than many of the Indian commercial mango varieties (123). The color variation (L*, a, b) among the wild edible fruit trees might be due to a genetic effect. It is well established that the L* value is a suitable indicator of darkening that arises either from increasing pigment concentrations or from oxidative browning reactions (124). Furthermore, the higher a* and b* values added a decorative effect toward the consumer’s preference. These fruits can be a good potential source for the extraction of natural edible color that is required in the food industry. As per Deka et al. (125), the products prepared from P. nepalensis, such as squash and jam, develop an attractive color and remain stable for 1 year (Figure 4). They have also prepared ready-to-serve (RTS) products and cherry wine from P. nepalensis fruits, which impart a unique natural purple color (13). The suitability of products for processing and extracting natural color helps stabilize the market price. The results also showed that the season of availability of fruits varies from plant to plant. The fruits of these wild edible fruit plants were found to be available throughout the year, with the exception of January and February. The different harvesting periods of these wild edible fruits ensure the year-round availability of these fruits, and particularly during the lean season when other fruits are not available, they provide supplementary food and nutritional security in the region. The yield of wild edible fruit trees varies between 7–26 kg per tree in E. pyriformis and 45–138 kg per tree in B. sapida. The variation in season of fruit availability and yield among wild edible fruit trees might be due to the contribution of genetic makeup and the growing environment (126). Similarly, the market price of fruits ranged from $ 0.21 per kg in E. pyriformis to $ 1.7 per kg in P. nepalensis. The variation in market price among wild edible fruits might be due to the contribution of fruit quality factors such as taste, TSS-acidity blend, peel appearance, etc., which determine the appealability and preferences amongst consumers. Tarancon et al. (127) also reported that the consumer’s perception of fruit quality is exclusively based on appearance. The market price of the fruits of P. nepalensis was about $34.10–$141.5 per tree, which highlights the high potential for income generation from these wild fruit trees. Therefore, expansion of the commercial area under these crops and their utilization may offer an additional source of income, employment generation, and livelihood improvement.
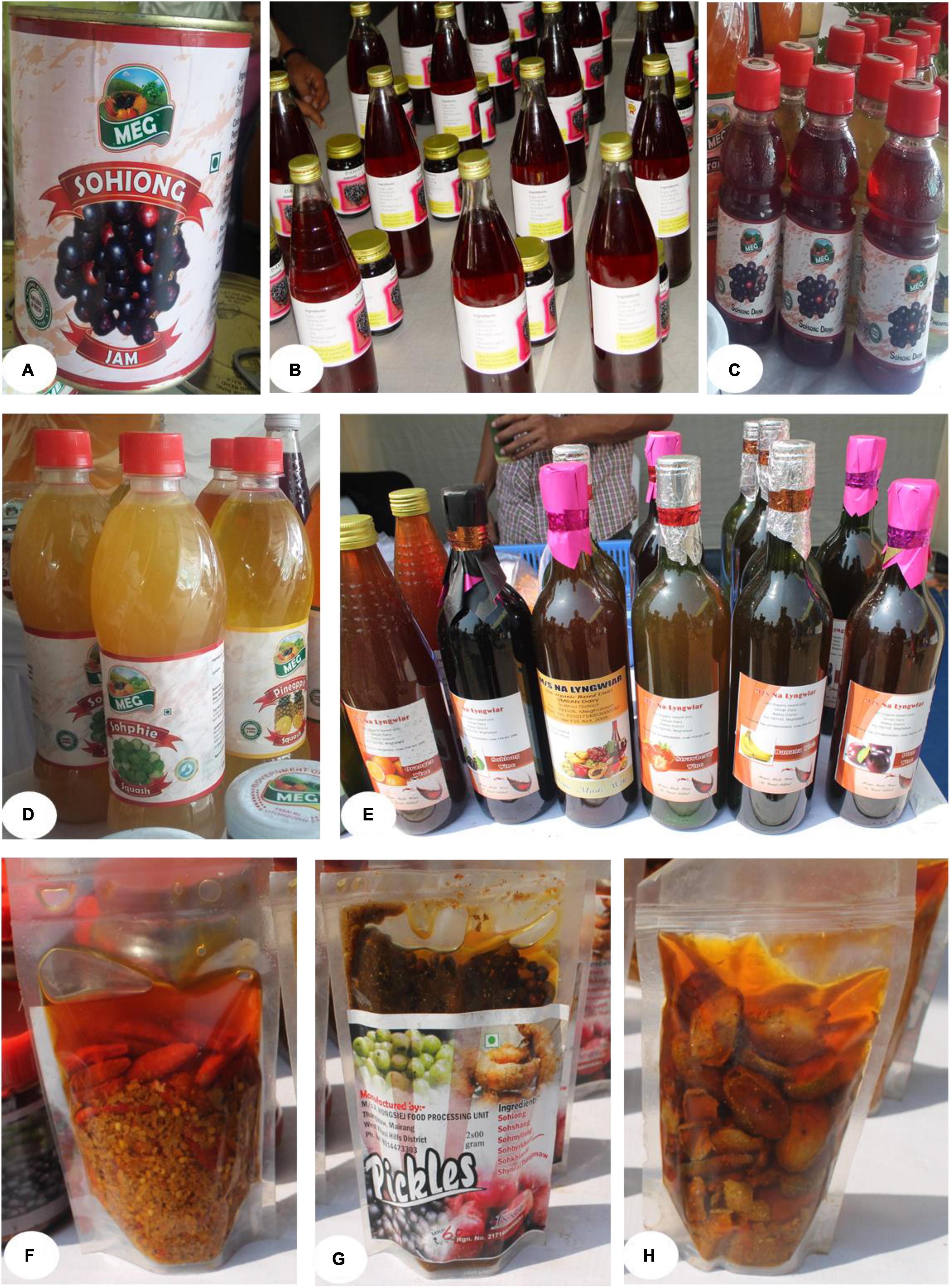
Figure 4. Different products developed by farmers from wild edible fruits grown in the eastern Himalayas, India. (A) Jam of Sohiong (P. nepalensis Ser.); (B) Squash of P. nepalensis; (C) Juice of P. nepalensis; (D) Juice of Sohphie (M. esculenta); (E) Wine of P. nepalensis; (F) pickle of Sohphoh (D. indica); (G) Mixed pickles of P. nepalensis and E. latifolia; (H) pickle of Sohshang (E. latifolia).
4. Conclusion
About 12% of the world’s population lives in mountainous regions. Wild edible fruits have been consumed by the mountainous populace since time immemorial. Many of these genetic resources, however, have become rare and endangered as a result of overexploitation in their natural habitat and a lack of consumer understanding of their antioxidant and biochemical values. Our results would aid in a proper understanding of the potential uses and antioxidant activities of wild edible fruit trees. Therefore, it is concluded that:
Wild fruits such as H. validus, P. nepalensis, B. sapida, E. latifolia, M. esculenta, and D. indica are high in total soluble solids, total sugar, and acidity. These fruits have the potential to be used as supplementary bases in the fruit processing industry.
The high antioxidant activities such as ascorbic acid, total phenolic content, total flavonoid, total flavonol, DPPH free scavenging capacity, and FRAP reducing power in H. validus, P. nepalensis, M. esculenta, and M. nagi suggest their potential as sources of bioactive compounds.
These fruits can be used to extract attractive natural colors and to make high-value processed products such as jams, squash, pickles, and wine.
A proper understanding of the biochemical and antioxidant properties of these fruits will help in their sustainable utilization and conservation.
Data availability statement
The original contributions presented in this study are included in the article/supplementary material, further inquiries can be directed to the corresponding author.
Author contributions
HR: laboratory studies, design of the figures, and writing the manuscript. VV: data analysis and interpretation of finding. HT, V, RS, and KB: editing of the manuscript. SA, MD, LC, and BM: correction of manuscript. JM, ARS: data collections, laboratory studies, and design of the figures. SH and VM: discussion of the results, critical feedback, and providing help in shaping the content, and evaluation of the manuscript. All authors contributed to the article and approved the submitted version.
Acknowledgments
We are thankful to the Director, ICAR Research Complex for NEH Region, Umiam, Meghalaya–793 103 for help throughout the entire experiment. We are also grateful to farmers for helping during survey and sharing of fruit samples for analysis under the Institute Project.
Conflict of interest
The authors declare that the research was conducted in the absence of any commercial or financial relationships that could be construed as a potential conflict of interest.
Publisher’s note
All claims expressed in this article are solely those of the authors and do not necessarily represent those of their affiliated organizations, or those of the publisher, the editors and the reviewers. Any product that may be evaluated in this article, or claim that may be made by its manufacturer, is not guaranteed or endorsed by the publisher.
References
1. Myers N, Mittermeier RA, Mittermeier CG, da Fonseca GAB, Kent J. Biodiversity ‘Hotspots’ for conservation priorities. Nature. (2000) 403:853–8. doi: 10.1038/35002501
2. Rymbai H, Roy AR, Deshmukh NA, Jha AK, Shimray W, War GF, et al. Analysis study on potential underutilized edible fruit genetic resources of the foothills track of Eastern Himalayas, India. Gen Res Crop Evol. (2016) 63:125–39. doi: 10.1007/s10722-015-0342-3
3. Abdullah ATM, Hossain MA, Bhuiyanv MK. Propagation of Latkan (Baccaurea sapida Muell.Arg.) by mature stem cutting. Res J Agric Biol Sci. (2005) 1:129–34.
4. Jabeen A, Sharma A, Gupta I, Kheraldine H, Vranic S, Al Farsi HF, et al. Elaeagnus angustifolia plant extract inhibits epithelial-mesenchymal transition and induces apoptosis via HER2 inactivation and JNK pathway in HER2-Positive breast cancer cells. Molecules. (2020) 25:4240. doi: 10.3390/molecules25184240
5. Janbaz KH, Ahsan MZ, Saqib F, Imran I, Zia-Ul-Haq M, Rashid MA, et al. Scientific basis for use of Pyrus pashia Buch.- Ham. ex D. Don. fruit in gastrointestinal, respiratory and cardiovascular ailments. PLoS One. (2015) 10:e0118605. doi: 10.1371/journal.pone.0118605
7. Alex BK, Koshy EP, Thomas G, Sharma N. Haematocarpus validus (Miers) Bakh.f.ex Forman (Khoon phal): an ethnomedicinal fruit crop rich in choline. SSRN Electron J. (2018) 59:168–78. doi: 10.2139/ssrn.3298649
8. Thapa B, Walker DH, Sinclair FL. Indigenous knowledge of the feeding value of tree fodder. Anim Feed Sci Tech. (1997) 67:97–l14. doi: 10.1016/S0377-8401(96)01129-7
9. Tsering J, Gogoi BJ, Tag H. Ethnobotany and phytochemical analysis of Pyrus pashia leaves. Int J Pharm Sci Res. (2012) 3:2721–5.
10. Shan S, Huang X, Shah MH, Abbasi AM. Evaluation of polyphenolics content and antioxidant activity in edible wild fruits. Biomed Res Int. (2019) 11:1381989. doi: 10.1155/2019/1381989
11. Wolfe KL, Liu RH. Structure-activity relationships of flavonoids in the cellular antioxidant activity assay. J Agric Food Chem. (2008) 56:8404–11. doi: 10.1021/jf8013074
12. Moure A, Cruz JM, Franco D, Domínguez JM, Sineiro J, Domínguez H, et al. Natural antioxidants from residual sources. Food Chem. (2001) 72:145–71. doi: 10.1016/S0308-8146(00)00223-5
13. Rymbai H, Patel RK, Deshmukh NA, Jha AK, Verma VK. Physical and biochemical content of indigenous underutilized Sohiong (Prunus nepalensiss Ser.) fruit in Meghalaya, India. Int J Minor Fruits Med Aromat Plants. (2016) 2:54–6.
14. Rymbai H, Deshmukh NA, Roy AR, Roy SS, Jha AK. Floral morphology of Eleaegnus latifolia L. Ind J Hortic. (2017) 74:340–5. doi: 10.5958/0974-0112.2017.00068.8
15. Rymbai H, Deshmukh NA, Talang HD, Jha AK. Physico-chemical variation in fruits of Pyrus pashia genotypes. Int J Minor Fruits Med Aromat Plants. (2019) 5:11–4. doi: 10.13140/RG.2.2.14758.88645
16. Fruitipedia. SOHIONG_Prunus nepalensis. (2022). Available online at: http://www.fruitipedia.com/2018/12/sohiong_prunus-nepalensis (accessed August 25, 2022).
17. Agrahar-Murugkar D, Subbulakshmi G. Nutritive values of wild edible fruits, berries, nuts, roots and spices consumed by the Khasi tribes of India. Ecol Food Nutr. (2005) 44:207–23. doi: 10.1016/j.foodchem.2004.03.042
18. Hanelt P. The mansfeld’s encyclopedia of agricultural and horticultural crops. Institute of Plant Genetics and Crop Plant Research (IPK) Edn. Berlin: Springer (2001).
20. Bhatt ID, Rawal RS, Dhar U. Improvement in seed germination of Myrica esculenta Buch.-Ham. ex D. Don-a high value tree species of Kumaun Himalaya, India. Seed Sci Technol. (2000) 28:597–605.
22. Kumar JK, Sinha AK. Resurgence of natural colourants: a holistic view. Nat Prod Res. (2004) 18:59–84. doi: 10.1080/1057563031000122112
23. Jeeva S, Lyndem FG, Sawian JT, Laloo RC, Mishra BP. Myrica esculenta Buch.–Ham. ex D. Don.–a potential ethnomedicinal species in a subtropical forest of Meghalaya, northeast India. Asian Pac J Trop Biomed. (2011) 1:S174–7. doi: 10.1016/S2221-1691(11)60150-0
24. Nainwal P, Kalra K. Study on the wound activity potential on the aqueous extract of the bark of Myrica esculenta Buch. & Ham. Int J Pharm Clin Res. (2009) 1:85–7.
25. Pala NA, Negi AK, Todaria NP. Traditional uses of medicinal plants of Pauri Garhwal, Uttrakhand. Nat Sci. (2010) 8:57–61.
26. Gaire BP, Subedi L. Medicinal plant diversity and their pharmacological aspects of Nepal Himalayas. Pharmacogn J. (2011) 3:6–17. doi: 10.5530/pj.2011.25.2
27. Kirtikar KR, Basu BD. Indian medicinal plants. Vol. III. Dehradun: Bishen Singh and Mahendra Pal Singh Edn (1984). p. 1664–6.
28. Rai N, Asati BS, Patel RK, Patel KK, Yadav DS. Underutilized horticultural crops in north eastern region. ENVIS Bull Himal Ecol. (2005) 13:19–29.
29. Sun B, Ricardo-da-Silva JM, Spranger I. Critical factors of vanillin assay for catechins and proanthocyanidins. J Agric Food Chem. (1998) 46:4267–74. doi: 10.1021/jf980366j
31. Maikhuri RK, Gangwar AK. Ethnobiological notes on the Khasi and Garo tribes of Meghalaya, Northeast India. Econ Bot. (1993) 47:345–57. doi: 10.1007/BF02907348
32. Lin YF, Yi Z, Zhao YH. Chinese dai medicine colorful illustrations. Kunming: Yunnan National Publishing House (2003). p. 380.
33. Meyers KJ, Watkins CB, Pritts MP, Liu RH. Antioxidant and antiproliferative activities of strawberries. J Agric Food Chem. (2003) 51:6887–92. doi: 10.1021/jf034506n
34. Abbasi AM, Khan MA, Khan N, Shah MH. Ethnobotanical survey of medicinally important wild edible fruits species used by tribal communities of lesser Himalayas-Pakistan. J. Ethnopharm. (2013) 148:528–36.
35. Chettri N, Sharma E, Lama SD. Non-timber forest produces utilization, distribution and status in a trekking corridor of Sikkim, India. Lyonia. (2005) 81:93–108.
36. Khandelwal R, Paliwal S, Chauhan R, Anees A. Phytochemical screening of hexane soluble fraction of Pyrus pashia fruits. Orient J Chem. (2008) 24:773.
37. Lama YC, Ghimire SK, Aumeeruddy-Thomas Y. Medicinal plants of dolpo. amchis’ knowledge and conservation. Katmandu: WWF Nepal Program (2001). p. 167.
38. Saklani S, Chandra S. In vitro antimicrobial activity nutritional profile of medicinal plant of Garhwal, Himalaya. Int J Pharm Sci Res. (2012) 3:268–72.
39. Jiangsu New Medical College. Dictionary of chinese traditional medicine. Shanghai: Shanghai People’s Press (1986).
40. Gulia KS. Human ecology of Sikkim: A case study of upper rangit basin. New Delhi: Gyan Publishing House (2005).
41. Shinwari MI, Khan MA. Indigenous use of medicinal trees and shrubs of Margalla Hills National Park, Islamabad. Pakistan J For. (1998) 48:63–90.
42. Prajapati ND, Purohit SS, Sharma AK, Kumar T. A handbook of medicinal plants: A complete source book. (2003). p. 554–554.
43. Shrestha PM, Dhillion SS. Medicinal plant diversity and use in the highlands of Dolakha district, Nepal. J Ethnopharm. (2003) 86:81–96.
45. Tiep HV, Thuong PH, Nguyen L, Lua HT, Thuan VV, Kieu LT, et al. Domestication of docynia indica in Vietnam. For Trees Livelih. (2018) 27:230–42.
46. Vivek K, Mishra S, Sasikumar R. Effect of ultra-sonication on postharvest quality parameters and microbial load on Docynia indica. Sci Hortic. (2017) 225:163–70.
47. Shende KM, Singh NI, Negi PS. Phytochemical characterization and biological activities of Docynia indica (Wall) fruit extracts. J Mol Genet Med. (2016) 10:1–5.
48. Singh B, Bedi YS. Rediscovery, taxonomic history and extended enumeration of Haematocarpus validus Bakh. f. ex Forman (Menispermaceae) to Indo-Myanmar biodiversity hotspot. Natl Acad Sci Lett. (2016) 39:383–7. doi: 10.1007/s40009-016-0483-8
49. Khatun MJM, Mahfuzur RM, Rahim MA, Mirdah MH. Study on the taxonomy and nutritional status of Lalgula (Haematocarpus validus): a promising endemic ethnic fruit of Bangladesh. Intl J Sustain Agric Technol. (2014) 10:1–4.
50. Kumar K, Kumar B, Selvun T, Sajibala B, Jairaj RSC, Mehrotra S, et al. Ethnobotanical heritage of Nicobarese tribe. J Econ Taxon Bot. (2006) 30:331–48.
51. Blessymole AK, Koshy EP, Thomas G. Antioxidant profile of Haematocarpus validus (miers) bakh. F. Ex forman (Khoonphal) leaf and fruit: a medicinally important rare ethnic fruit crop. Plant Archives. (2018) 18:2031–6.
52. Rangana S. Handbook of analysis and quality control for fruit and vegetable products. 2nd Edn. New Delhi: Tata McGraw Hill Publishing Co. Ltd (1997).
53. Akter S, Shahriar SMS, Akter F, Morshed S, Islam MN. Study on chemical composition of fresh Mymensingh and Barishal hog-plum (Spondius mangifera) and developed leather and jelly and sensory evaluation. J Environ Sci Nat Resour. (2012) 5:29–36. doi: 10.3329/jesnr.v5i2.14598
54. Raaf A, Putra TW, Mulana F, Syamsuddin Y, Supardan MD. Investigation of kinetics of amla (Emblica officinalis) fruit drying process. S Afr J Chem Eng. (2022) 41:10–6. doi: 10.1016/j.sajce.2022.03.011
55. Chen JP, Tai CY, Chen BH. Improved liquid chromatographic method for determination of carotenoids in Taiwanese mango (Mangifera indica L.). J Chromatogr A. (2004) 1054:261–8. doi: 10.1016/s0021-9673(04)01406-2
56. Zhishen J, Mengcheng T, Jianming W. The determination of flavonoid contents in mulberry and their scavenging effects on superoxide radicals. Food Chem. (1999) 64:555–59.
57. Giusti MM, Wrolstad RE. Characterization and measurement of anthocyanins by UV-visible spectroscopy. Curr Protoc Food Anal Chem. (2001) 1:F1–2. doi: 10.1021/jf0478096
58. Lako J, Trenerry VC, Wahlqvist M, Naiya W. Phytochemical flavonols, carotenoids, and the antioxidant properties of a wide selection of Fijian fruit, vegetables and other readily available foods. Food Chem. (2007) 101:1727–41. doi: 10.1016/j.foodchem.2006.01.031
59. Miliauskas G, Venskutonis PR, Van Beek TA. Screening of radical scavenging activity of some medicinal plants and aromatic plant extract. Food Chem. (2004) 85:231–7. doi: 10.1016/j.foodchem.2003.05.007
60. Blois MS. Antioxidant determinations by the use of a stable free radical. Nature. (1958) 181:1199–200. doi: 10.1038/1811199a0
61. Oyaizu M. Studies on product of browning reaction prepared from glucose amine. Jap J Nutri. (1986) 44:307–15. doi: 10.5264/eiyogakuzashi.44.307
62. Martin GJ. Ethnobotany: A Method manual. London: Chapman and hall (1995). doi: 10.1007/978-1-4615-2496-0
63. Bradley RL Jr. Moisture and total solids analysis. In: S Nielsen editor. Food Analysis. New York, NY: Springer Science and Business Media (2010). doi: 10.1007/978-1-4419-1478-1_6
64. Canan I, Gündoǧdu1 M, Seday U, Oluk CA, Karaşahin Z, Eroǧlu EC, et al. Determination of antioxidant, total phenolic, total carotenoid, lycopene, ascorbic acid, and sugar contents of Citrus species and mandarin hybrids. Turk J Agric For. (2016) 40:894–9. doi: 10.3906/tar-1606-83
65. Walkowiak-Tomczak D, Reguła J, Łysiak G. Physico-chemical properties and antioxidant activity of selected plum cultivars fruit. Acta Sci Pol Technol Aliment. (2008) 7:15–22.
66. Paciolla C, Fortunato S, Dipierro N, Paradiso A, De Leonardis S, Mastropasqua L, et al. Vitamin C in plants: from functions to biofortification. Antioxidants. (2019) 8:519. doi: 10.3390/antiox8110519
67. Contreras-Calderón J, Calderón-Jaimes L, Guerra-Hernández E, García-Villanova B. Antioxidant capacity, phenolic content and vitamin C in pulp, peel and seed from 24 exotic fruits from Colombia. Food Res Int. (2011) 44:2047–53. doi: 10.1016/j.foodres.2010.11.003
68. Gupta S, Prakash J. Studies on Indian leafy vegetables for their antioxidant activity. Plant Food Hum Nutr. (2009) 64:39–45. doi: 10.1007/s11130-008-0096-6
69. Deshmukh NA, Rymbai H, Jha AK, Lyngdoh P, Malhotra SK. Effect of thinning time and fruit spacing on fruit maturity, yield, size, peel colour and quality attributes of peach cv, Flordasun. Ind J Hortic. (2017) 74:45–50. doi: 10.5958/0974-0112.2017.00012.3
70. Khomdram S, Barthakur S, Devi GS. Biochemical and molecular analysis of wild endemic fruits of the Manipur region of India. Int. J. Fruit Sci. (2014) 14:253–66. doi: 10.1080/15538362.2013.818483
71. Dasila K, Singh S. Bioactive compounds and biological activities of Elaegnus latifolia L.: An underutilized fruit of North-East Himalaya, India. South Afri Bot. (2021) 145:177–85. doi: 10.1016/j.sajb.2021.07.020
72. Dias MG, Borge GIA, Kljak K, Mandić AI, Mapelli-Brahm P, Olmedilla-Alonso B, et al. European database of carotenoid levels in foods. factors affecting carotenoid content. Foods. (2021) 10:912. doi: 10.3390/foods10050912
73. Walker PG, Viola R, Woodhead M, Jorgensen L, Gordon SL, Brennan RM, et al. Ascorbic acid content of blackcurrant fruit is influenced by both genetic and environmental factors. Funct Plant Sci Biotechnol. (2009) 4:40–52. doi: 10.1002/jsfa.7864
74. Dewanti FD, Pujiasmanto B, Sukendah S, Yunus A. Analysis of ascorbic acid content (vitamin C) of purslane (Portulaca oleracea L.) at various altitudes in East Java, Indonesia. IOP Conf Ser Earth Environ Sci. (2021) 637:012074. doi: 10.1088/1755-1315/637/1/012074
75. Suksri A. Some agronomic and physiological aspects in growing crops in Northeast Thailand. 1st Edn. Khon Kaen: Khon Kaen University Press (1999).
76. Ramaiya SD, Bujang JS, Zakaria MH, Kinga WS, Sahrira MAS. Sugars, ascorbic acid, total phenolic content and total antioxidant activity in passion fruit (Passiflora) cultivars. J Sci Food Agric. (2012) 93:1198–205. doi: 10.1002/jsfa.5876
78. Matuszewska A, Jaszek M, Stefaniuk D, Ciszewski T, Matuszewski Ł. Anticancer, antioxidant, and antibacterial activities of low molecular weight bioactive subfractions isolated from cultures of wood degrading fungus Cerrena unicolor. PLoS One. (2018) 13:e0197044. doi: 10.1371/journal.pone.0197044
79. Singh S, Singh DR, Salim KM, Srivastava A, Singh LB, Srivastava RC. Estimation of proximate composition, micronutrients and phytochemical compounds in traditional vegetables from Andaman & Nicobar Islands. Int J Food Sci Nutri. (2012) 62:765–73. doi: 10.3109/09637486.2011.585961
80. Machlin LJ. Critical assessment of the epidemiological data concerning the impact of antioxidant nutrients on cancer and cardiovascular disease. Crit Rev Food Sci Nutr. (1995) 35:41–50. doi: 10.1080/10408399509527684
81. Rymbai H. Growth, flowering and fruiting characteristics of mango (Mangifera indica L.) hybrids. Ph.D. thesis. New Delhi: Indian Agricultural Research Institute (2012).
82. Batista PF, de Lima MAC, Alves RE, Façanha RV. Bioactive compounds and antioxidant activity in tropical fruits grown in the lower-middle São Francisco Valley. Rev Ciência Agron. (2018) 49:616–23. doi: 10.5935/1806-6690.20180070
83. Lu W, Shi Y, Wang R, Su D, Tang M, Liu Y, et al. Antioxidant activity and healthy benefits of natural pigments in fruits: a review. Int J Mol Sci. (2021) 22:4945. doi: 10.3390/ijms22094945
84. Fidrianny I, Puspitasari N, Singgih M. Antioxidant activities, total flavonoid, phenolic, carotenoid of various shells extract from four species of legumes. Asian J Pharm Sci. (2014) 7:42–6.
85. Kong KW, Khoo HE, Prasad KN, Ismail A, Tan CP, Rajab NF. Revealing the power of the natural red pigment lycopene. Molecules. (2010) 15:959–87. doi: 10.3390/molecules15020959
86. Horbowicz M, Kosson R, Grzesiuk A, Dębski H. Anthocyanins of fruits and vegetables-their occurrence, analysis and role in human nutrition. J Fruit Ornam Plant Res. (2008) 68:5–22. doi: 10.2478/v10032-008-0001-8
87. Andersen ØM, Jordheim M. Basic anthocyanin chemistry and dietary sources. In: TC Wallace, MM Giusti editors. Anthocyanins in health and disease. Boca Raton, FL: CRC Press (2013). doi: 10.1201/b15554-3
88. Oancea S, Draghici O, Ketney O. Changes in total anthocyanin content and antioxidant activity in sweet cherries during frozen storage, and air-oven and infrared drying. Fruits. (2016) 71:281–8. doi: 10.1051/fruits/2016025
89. Nour V, Trandafir I, Ionica ME. Ascorbic acid, anthocyanins, organic acids and mineral content of some black and red currant cultivars. Fruits. (2011) 66:353–62. doi: 10.1051/fruits/2011049
90. Di Gioia F, Tzortzakis N, Rouphael Y, Kyriacou MC, Sampaio SL, Ferreira ICFR, et al. Grown to be blue—antioxidant properties and health effects of colored vegetables. part ii: leafy, fruit, and other vegetables. Antioxidants. (2020) 9:97. doi: 10.3390/antiox9020097
91. Ma Y, Zhao M, Wu H, Yuan C, Li H, Zhang Y. Effects of fruit bagging on anthocyanin accumulation and related gene expression in peach. J Am Soc Hort Sci. (2021) 146:217–23. doi: 10.21273/JASHS05019-20
92. Muzolf-Panek M, Waskiewicz A. Relationship between phenolic compounds, antioxidant activity and color parameters of red table grape skins using linear ordering analysis. Appl Sci. (2022) 12:6146. doi: 10.3390/app12126146
93. Ponder A, Hallmann E, Kwolek M, Tober SD, Kazimierczak R. Genetic differentiation in anthocyanin content among berry fruits. Curr Issues Mol Biol. (2021) 43:36–51. doi: 10.3390/cimb43010004
94. Katiresh M, Suganya P, Saravanakumar M. Antioxidant effect of Sesbania sesban flower extract. Int J Ph Sci. (2011) 3:1307–12.
95. Wrolstad RE, Durst RW, Lee J. Tracking color and pigment changes in anthocyanin products. Trends Food Sci Technol. (2005) 16:423–8. doi: 10.1093/treephys/tpv148
96. Castaneda-Ovando A, Pacheco-Hernandez ML, Paez-Hernandez ME, Rodriguez JA, Galan-Vidal CA. Chemical studies of anthocyanins: a review. Food Chem. (2009) 113:859–71. doi: 10.1016/j.foodchem.2008.09.001
97. Khoo HE, Azlan A, Teng ST, Lim SM. Anthocyanidins and anthocyanins: coloured pigments as food, pharmaceutical ingredients, and the potential health benefits. Food Nutr. (2017) 61:1361779. doi: 10.1080/16546628.2017.1361779
98. Kopustinskiene DM, Jakstas V, Savickas A, Bernatoniene J. Flavonoids as anticancer agents. Nutrients. (2020) 12:457. doi: 10.3390/nu12020457
99. Fouad A, Ahmed A, Rehab F, Mohammed A. Proximate compositions, phytochemical constituents, antioxidant activities and phenolic contents of seed and leaves extracts of Egyptian leek (Allium ampeloprasum var. kurrat). Eur J Chem. (2013) 4:185-190. doi: 10.5155/eurjchem.4.3.185-190.711
100. Blando F, Albano C, Liu Y, Nicoletti I, Corradini D, Tommasi N, et al. Polyphenolic composition and antioxidant activity of the under-utilised Prunus mahaleb L. fruit. J Sci Food Agric. (2015) 96:2641–9. doi: 10.1002/jsfa.7381
101. Chandra S, Khan S, Avula B, Lata H, Yang MH, ElSohly MA, et al. Assessment of total phenolic and flavonoid content, antioxidant properties, and yield of aeroponically and conventionally grown leafy vegetables and fruit crops: a comparative study. Evid-Based Complement Altern Med. (2014) 2014:253875. doi: 10.1155/2014/253875
102. Adesegun SA, Fajana A, Orabueze CI, Coker HAB. Evaluation of antioxidant properties of Phaulopsis fascisepala CB Cl.(Acanthaceae). Evid-Based Complement Altern Med. (2009) 6:227–31. doi: 10.1093/ecam/nem098
103. Heim K, Tagliaferro A, Bobilya D. Flavonoid antioxidants: chemistry, metabolism and structure-activity relationship. J Nutr Biochem. (2002) 13:572–84. doi: 10.1016/S0955-2863(02)00208-5
104. Wangcharoen W, Phimphilai S. Chlorophyll and total phenolic contents, antioxidant activities and consumer acceptance test of processed grass drinks. J Food Sci Technol. (2016) 53:4135–40. doi: 10.1007/s13197-016-2380-z
105. Luximon-Ramma A, Bahorun T, Crozier A. Antioxidant actions and phenolic and vitamin C contents of common Mauritian exotic fruits. J Sci Food Agric. (2003) 83:496–502. doi: 10.1002/jsfa.1365
106. Bennett RN, Shiga TM, Hassimotto NMA, Rosa EAS, Lajolo FM, Cordenunsi BR. Phenolics and antioxidant properties of fruit pulp and cell wall fractions of postharvest banana (Musa acuminata Juss.) Cultivars. J Agric Food Chem. (2010) 58:7991–8003. doi: 10.1021/jf1008692
107. Singh AK, Singh PP, Singh S, Bhargava R, Makwana P. Variability in morphological and physico-chemical traits of aonla (Emblica officinalis) genotypes collected from north-eastern region of India. Indian J Agric Sci. (2016) 86:992–7.
108. Robards K, Prenzlera PD, Tucker G, Swatsitang P, Glover W. Phenolic compounds and their role in oxidative processes in fruits. Food Chem. (1999) 66:401–36. doi: 10.1016/S0308-8146(99)00093-X
109. Aryal S, Baniya MK, Danekhu K, Kunwar P, Gurung R, Koirala N. Total phenolic content, flavonoid content and antioxidant potential of wild vegetables from Western Nepal. Plants. (2019) 8:96. doi: 10.3390/plants8040096
110. Czyczyło-Mysza I, Cyganek K, Dziurka K, Quarrie S, Skrzypek E, Marcińska I, et al. Genetic Parameters and QTLs for total phenolic content and yield of wheat mapping population of CSDH lines under drought stress. Int J Mol Sci. (2019) 20:6064. doi: 10.3390/ijms20236064
111. Fitriansyah SN, Fidrianny I, Ruslan K. Correlation of total phenolic, flavonoid and carotenoid content of Sesbania sesban (L. Merr) leaves extract with DPPH scavenging activities. Int J Pharmacogn Phytochem Res. (2017) 9:89–94.
112. Nariya P, Bhalodia N, Shukla V, Acharya R, Nariya M. In vitro evaluation of antioxidant activity of Cordia dichotoma (Forst f.) bark. Ayu. (2013) 34:124–8. doi: 10.4103/0974-8520.115451
113. Prior RL, Wu X, Schaich K. Standardized methods for the determination of antioxidant capacity and phenolics in food and dietary supplements. J Agric Food Chem. (2005) 53:4290–302. doi: 10.1021/jf0502698
114. Sundaramoorthy PMK, Packiam KP. In vitro enzyme inhibitory and cytotoxic studies with Evolvulus alsinoides (Linn.) Linn. Leaf extract: a plant from Ayurveda recognized as Dasapushpam for the management of Alzheimer’s disease and diabetes mellitus. BMC Complement Med Altern Med Ther. (2020) 20:129. doi: 10.1186/s12906-020-02922-7
115. Mukherjee PK, Maity N, Nema NK, Sarkar BK. Bioactive compounds from natural resources against skin aging. Phytomedicine. (2011) 19:64–73. doi: 10.1016/j.phymed.2011.10.003
116. Muflihah YM, Gollavelli G, Ling YC. Correlation study of antioxidant activity with phenolic and flavonoid compounds in 12 Indonesian indigenous herbs. Antioxidants. (2021) 10:1530.
117. Munteanu IG, Apetrei C. Analytical methods used in determining antioxidant activity: a review. Int J Mol Sci. (2021) 22:3380. doi: 10.3390/ijms22073380
118. Mahadkar S, Jadhav V, Deshmukh S. Antioxidant activity of some promising wild edible fruits. Der Chemica Sin. (2013) 4:165–9.
119. Szydłowska-Czerniak A, Tułodziecka A, Szłyk E. A silver nanoparticle-based method for determination of antioxidant capacity of rapeseed and its products. Analyst. (2012) 137:3750–9. doi: 10.1039/c2an35326a
120. Huang D, Ou B, Prior RL. The chemistry behind antioxidant capacity assays. J Agric Food Chem. (2005) 53:1841–56. doi: 10.1021/jf030723c
121. Rice-Evans C, Miller N, Paganga G. Antioxidant properties of phenolic compounds. Trends Plant Sci. (1997) 2:152–9. doi: 10.1016/S1360-1385(97)01018-2
122. Khabade VK, Lakshmeesh NB, Roy S. Comparative study on antioxidant and anti-inflammatory properties of three colored varieties of Capsicum annuum. Int J Fundam Appl Sci. (2012) 1:51–4.
123. Sethi S, Srivastav M, Samuel DVK, Singh AK, Dubey AK, Singh G. Evaluation of newly developed mango (Mangifera indica) hybrids for their storage behaviour and peel colour. Ind J Agric Sci. (2011) 81:252–5.
124. Keshek MH, Omar MN, Elsisi SF. Simulation of mass transfer from peaches during cool store and its effect on some quality properties. Misr J Agric Eng. (2019) 36:259–82. doi: 10.21608/MJAE.2019.94455
125. Deka BC, Patel RK, Rymbai H, Thirugnanavel A, Deshmukh NA, Ngachan S. Bulletin: Sohiong and Sohshang: Two lesser known fruits of Northeast India. Umiam: ICAR Research Complex for NEH Region (2014).
126. Chagné D, Dayatilake D, Diack R, Oliver M, Ireland H, Watson A, et al. Genetic and environmental control of fruit maturation, dry matter and firmness in apple (Malus × domestica Borkh.). Hortic Res Sep. (2014) 17:14046. doi: 10.1038/hortres.2014.46
Keywords: wild edible fruits, bioactive compounds, pigmentation, processing, value addition, livelihood, diversity, conservation
Citation: Rymbai H, Verma VK, Talang H, Assumi SR, Devi MB, Vanlalruati, Sangma RHCH, Biam KP, Chanu LJ, Makdoh B, Singh AR, Mawleiñ J, Hazarika S and Mishra VK (2023) Biochemical and antioxidant activity of wild edible fruits of the eastern Himalaya, India. Front. Nutr. 10:1039965. doi: 10.3389/fnut.2023.1039965
Received: 08 September 2022; Accepted: 31 January 2023;
Published: 01 March 2023.
Edited by:
Subhash Babu, Indian Agricultural Research Institute (ICAR), IndiaReviewed by:
Manish Srivastav, Indian Agricultural Research Institute (ICAR), IndiaVivek Yadav, Northwest A&F University, China
Copyright © 2023 Rymbai, Verma, Talang, Assumi, Devi, Vanlalruati, Sangma, Biam, Chanu, Makdoh, Singh, Mawleiñ, Hazarika and Mishra. This is an open-access article distributed under the terms of the Creative Commons Attribution License (CC BY). The use, distribution or reproduction in other forums is permitted, provided the original author(s) and the copyright owner(s) are credited and that the original publication in this journal is cited, in accordance with accepted academic practice. No use, distribution or reproduction is permitted which does not comply with these terms.
*Correspondence: Heiplanmi Rymbai, rymbaihort@gmail.com