Polyphenols: Natural food grade biomolecules for treating neurodegenerative diseases from a multi-target perspective
- 1School of Basic Medical Sciences, Yunnan University of Chinese Medicine, Kunming, China
- 2The First Clinical Medical School, Yunnan University of Chinese Medicine, Kunming, Yunnan, China
- 3The Second Clinical Medical School, Yunnan University of Chinese Medicine, Kunming, Yunnan, China
As natural functional bioactive ingredients found in foods and plants, polyphenols play various antioxidant and anti-inflammatory roles to prevent the development of disease and restore human health. The multi-target modulation of polyphenols provides a novel practical therapeutic strategy for neurodegenerative diseases that are difficult to treat with traditional drugs like glutathione and cholinesterase inhibitors. This review mainly focuses on the efficacy of polyphenols on ischemic stroke, Parkinson's disease and Alzheimer's disease, including in vivo and in vitro experimental studies. It is further emphasized that polyphenols exert neuroprotective effects primarily through inhibiting production of oxidative stress and inflammatory cytokines, which may be the underlying mechanism. However, polyphenols are still rarely used as medicines to treat neurodegenerative diseases. Due to the lack of clinical trials, the mechanism of polyphenols is still in the stage of insufficient exploration. Future large-scale multi-center randomized controlled trials and in-depth mechanism studies are still needed to fully assess the safety, efficacy and side effects of polyphenols.
Introduction
Neurodegenerative diseases are a heterogeneous group of diseases characterized by irreversible, progressive degeneration and death of neuronal cells (1). At present, the cause of this kind of disease is still unclear and cannot completely cured yet, which poses a severe challenge to human health and a substantial economic burden. Neurons present in the body can maintain cellular homeostasis by dealing with different stressors (2). However, two conditions usually lead to neuronal death. The first one is when multiple stressors accumulate and exceed the cell's ability to recover, various damages to cells will eventually lead to neuronal death. The formation of higher-order aggregates is a significant cause of neuronal stressor, and eventually triggers consequent cytotoxic events and cell death (3–6). Another cause of neuronal death is the traumatic events, such as ischemic stroke, which usually leads to a massive decrease in neuronal function in the affected area and subsequently induces acute neuronal cell death (7). In recent years, the improvement of neurodegenerative diseases through natural medicines such as polyphenols has increasingly become a research hotspot.
Polyphenols are secondary plant metabolites with highly diversified chemical structures (8). They are the largest class of plant chemicals. The basic structure of polyphenols is to connect at least one aromatic ring with one or more hydroxyl functional groups (i.e., several hydroxyl groups on the aromatic ring). Thousands of such polyphenol structures have been identified in plants and foods, mainly including flavonoids (60%), phenolic acids (30%), and other polyphenols, such as stilbene and lignans (8, 9). Flavonoids can be divided into anthocyanins, flavan-3-oil, flavonoids, flavanone, and other flavonoid subclasses. Phenolic acid exists in free form in fruits and vegetables, and they often exist in a conjugated state in bran and shell (10–12). Wine and red wine contain stilbene in other polyphenols, and lignans are found in many grains, such as sesame (13, 14). Polyphenols, as a powerful antioxidant, can play an essential role in the treatment of oxidative stress and neuroinflammation-related diseases. It has been found that supplementing antioxidant vitamins and enzymes, such as vitamins C, E, carotene, and other antioxidants, can protect the organism against external stimuli, reduce and eliminate the level of reactive oxygen species (ROS) (14, 15). Recently, the side effects of traditional drugs used to treat neurodegenerative diseases, such as cholinesterase inhibitors and NMDA antagonists, have become more widely recognized (16). Compared to other drugs, polyphenolic natural medicines are often found in our daily diet, and they have fewer side effects. Many plant foods that are common in our daily lives contain polyphenols, such as tea, cocoa, fruits, and vegetables. In addition to this, polyphenols have also been found in traditional Chinese medicine (17).
The preventive and therapeutic effects of polyphenols on neurodegenerative diseases have been investigated in previous studies (18–20). Although there are relatively many studies on polyphenols, the underlying core mechanism of polyphenols in neurodegenerative diseases is still unclear. This review will focus on the effects of polyphenols on neurodegenerative diseases, including cerebral ischemic stroke (CIS), Parkinson's disease (PD), and Alzheimer's disease (AD), which covers both in vitro and in vivo studies. Furthermore, the underlying mechanisms that polyphenols exert neuroprotective effects are also reviewed.
Effects and mechanisms of polyphenols in the treatment of neurodegenerative diseases
As shown in Figure 1, the pathogenesis of neurodegenerative diseases, as well as the core target and underlying mechanism of polyphenols in the treatment of neurodegenerative diseases are described, including cerebral ischemic stroke (CIS), Parkinson's disease (PD), and Alzheimer's disease (AD). Table 1 further illustrates the type of polyphenols, and the effects and mechanism in vitro and in vivo experiments.
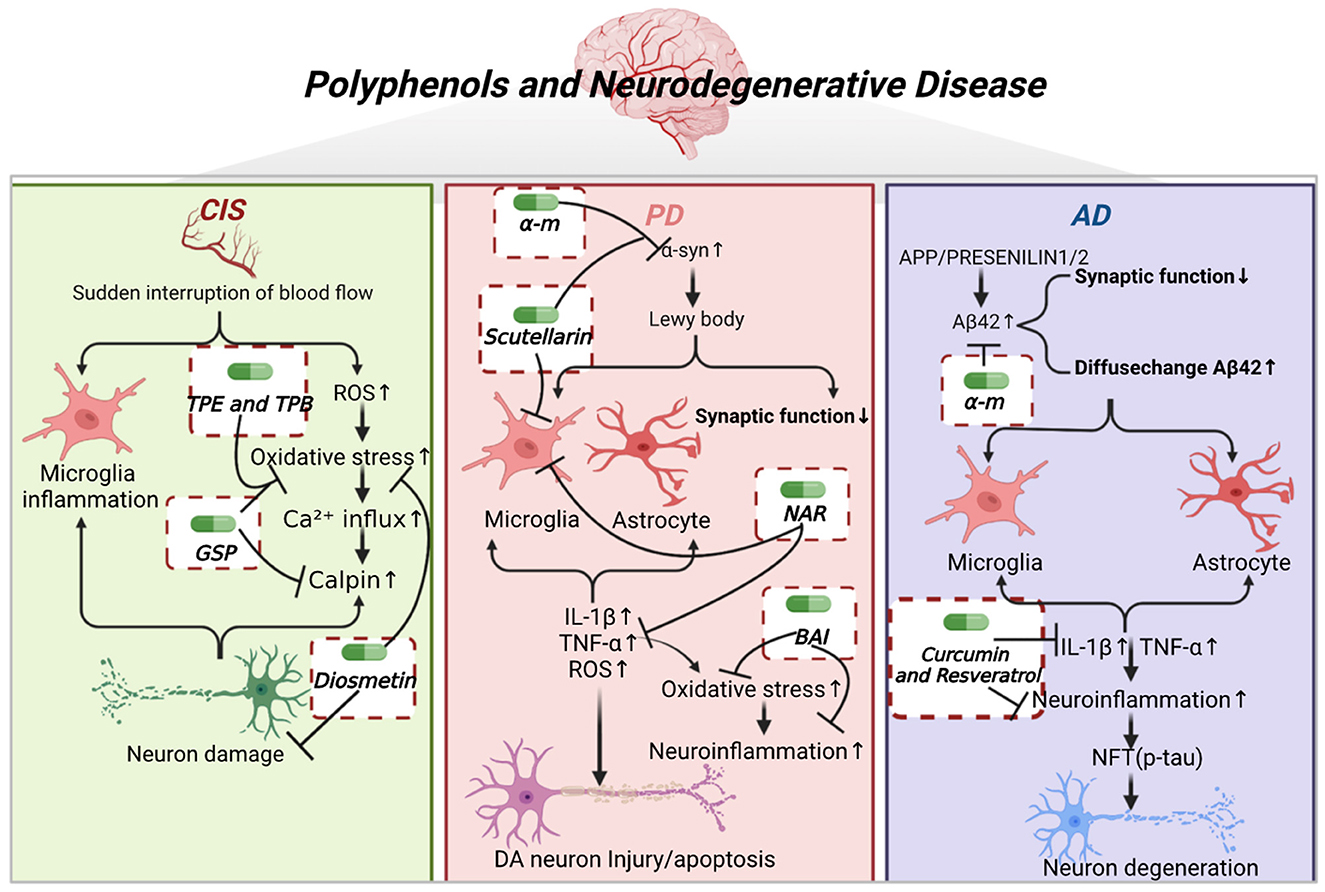
Figure 1. Polyphenols and neurodegenerative diseases. CIS is caused by the sudden interruption of blood flow to the brain, leading to brain cell death and nerve damage. Subsequently, it causes microglia activation, elevates ROS, inward calcium flow, and calmodulin release, leading to neuronal death. TPE, TPB, GSP, and diosmetin inhibited oxidative stress. GSP also inhibited calpain activation, and diosmetin exerted neuroprotective effects. PD is caused by the aggregation of α-syn proteins into Lewy bodies, leading to decreased synaptic function, and further activation of microglia and astrocytes. This leads to the release of inflammatory factors such as IL-β, TNF-α, and ROS, causing elevated levels of oxidative stress, neuroinflammation and damage or apoptosis of DA neurons. α-M and scutellarin inhibited the aggregation of α-syn oligomers. Scutellarin and NAR inhibited the activation of microglia. NAR inhibited the release of inflammatory factors. BAI inhibited the oxidative stress and neuroinflammation. AD is caused by the deposition of Aβ42 aggregates, which activates microglia and astrocytes to release inflammatory factors and leads to neuroinflammation as well as NFT. α-M inhibited the aggregation of Aβ42 oligomers. Curcumin and resveratrol inhibited the release of inflammatory factors and neuroinflammation, thereby attenuating neuronal degeneration.
Cerebral ischemic stroke
Cerebral ischemic stroke is one of the leading causes of disability in the world's population. CIS is caused by the sudden interruption of blood flow to the brain, leading to brain cell death and neuronal damage (35). Because of the poor regenerative capacity of the adult brain, neuronal damage is almost impossible to reverse (36). Therefore, it is essential to reduce the loss of neurons or tissues after CIS (37). Cerebral ischemia/reperfusion (I/R) injury is an important risk factor for stroke because the process of I/R may further aggravate the initial ischemic injury. For this reason, the pathogenesis of CIS has not been fully clarified, and there is a lack of effective treatments (38).
In vitro studies
Oxidative stress caused by excessive ROS production is closely related to the pathogenesis of CIS. At the same time, oxidative stress is one of the most important processes in brain I/R injury and the main risk factor for neural cell apoptosis (39). Excessive ROS production leads to neuronal dysfunction through a variety of mechanisms, including inflammation, cell apoptosis and necrosis (40), which is considered to be the main factor leading to brain damage (41, 42). Resveratrol is a natural plant antitoxin, which has neuroprotective, antioxidant, anti-cancer, and anti-inflammatory properties (43–45). A study has found that resveratrol exerts its protective effects against the damage of oxygen glucose deprivation and reperfusion (OGD/R), at least in part, by promoting mitophagy (23). Resveratrol attenuates OGD/R-induced oxidative stress and preserves mitochondrial function, exerting neuroprotective effects through PINK1/Parkin-mediated mitophagy. Grape seed proanthocyanidins (GSP) are one of the complex flavonoid polymers. Many studies show that GSP have anti-inflammatory, anti-apoptotic, antioxidant, and free radical scavenging properties (29, 46, 47). One study established an OGD/R model using primary brain cell cultures (48). They found that GSP could prevent the damages of OGD/R, inhibit OGD/R-induced cell death and improve cell viability. Pretreatment with GSP can efficiently prevent the cells from almost all inflammatory factors. Another experiment identified the main biological activity of TPE and TPB by high performance liquid chromatography (HPLC) as phenolic acids after grading and purification of crude extracts (21). It was found that TPE and TPB facilitated the translocation of nuclear factor erythroid-2 related factor 2 (Nrf2) to the nucleus and enhanced Nrf2 expression in the nucleus, restored OGD/R-induced oxidative damage in BV2 microglial cells. Pelargonium is a polyphenol extracted from phyllanthus amarus, which has a wide range of biological and pharmaceutical activities, including antioxidant, anti-inflammatory, antithrombotic, and other biological activities. In addition, pelargonium has been found to activate the nuclear factor erythroid-2 related factor 2/heme oxygenase-1 (Nrf2/HO-1) signaling pathway and protect PC12 cells from cytotoxicity, as well as oxidative stress and apoptosis caused by OGD/R (22).
In vivo studies
Two-month-old male SD rats were modeled by middle cerebral artery occlusion (MACO) (24), and rehabilitation training plus resveratrol was used for treatment. It was found that resveratrol improved the recovery of neurological and motor function in MCAO rats through the silent mating type information regulation 2 homolog-1 (Sirt1) signaling pathway, and activated brain-derived neurotrophic factor/tyrosine kinase receptor b (BDNF/TrkB) signaling pathways. By modeling the I/R injury of male Wistar rats (48), it was found that the treatment of GSP reduced the size of cerebral infarct and clearly improved both behavioral and overall activities of rats as it allowed to restore rearing and crossing scores to near control level. Another study demonstrated that the TPE and TPB alleviated the reduction of antioxidant enzyme activity in cerebral ischemia-reperfusion injury, and exerted protective effects in MCAO mice by inhibiting apoptosis and stimulating the Nrf2/HO-1 signaling pathway (21). Geraniin can reduce brain I/R damage by inhibiting oxidative stress (22). Geraniin can activate Nrf2/HO-1 signal pathway, reduce I/R damage of the middle cerebral artery occlusion-reperfusion (MCAO/R) in rats, significantly increase the number of surviving neurons, and inhibit oxidative stress induced by MCAO/R.
Therefore, polyphenols can inhibit oxidative stress to prevent neurons against the damage of ROS, exerting neuroprotective effects on CIS.
Parkinson's disease
Parkinson's disease (PD), a progressive motor dysfunction, is defined as a significant loss and misfolding of dopaminergic (DA) neurons in the substantia nigra (SN), α-Synuclein (α-Syn) aggregation in louis corpuscles, as well as motor dysfunction (static tremor, rigidity, and motor retardation) and non-motor symptoms (autonomic nervous dysfunction, cognitive impairment, depression, REM sleep behavior, etc.) that appears several years before the onset of the motor phenotype (49–54). Studies have found that most PD patients are sporadic, and the rest are mostly related to gene mutations caused by mitochondrial dysfunction. About 50% of early-onset PD patients have mutations in Parkin (55–57).
In vitro studies
α-Syn is one of the first genes found to be associated with PD. Research proves that α-Syn and Nrf2 deficiency aggravates protein aggregation, neuroinflammation, and neuronal death (58). A study has found that scutellarin can effectively inhibit metal-induced and uninduced α-Syn's fibrosis (25), and stabilize partially folded α-Syn intermediate to form an SDS-resistant high-order oligomer. Baicalin (BAI) is an important flavonoid compound. Another study by human cell line pLVX-Tet3G-α-synuclein SH-SY5Y found that BAI could protect DA neurons against ROS and decrease C/EBPβ and α-Syn expression in pLVX-Tet3G-α-synuclein SH-SY5Y cells (59). Alpha-mangostin (AM), a polyphenolic xanthone obtained from Garcinia Mangostana L, can activate the autophagy in PC12 cells, playing roles in clearance of α-Syn (28). Chlorogenic acid (CGA) is a polyphenolic compound with antioxidant and anti-inflammatory properties. CGA initiated the SIRT1/NF-κB signaling pathway and inhibited OGD/R-induced inflammation, oxidative stress, and neuronal apoptosis by upregulating MIR497HG to suppress miR-29b-3p expression (60). Naringin (NAR) is a natural flavone contained in citrus fruits and grapefruit, which has a lot of pharmacological activities. A study has proved that NAR protects DA neurons from lipopolysaccharide (LPS)-induced neurotoxicity by inhibiting the activation of inflammatory corpuscle signals of microglial NOD-like receptor pyrin domain containing 3 (NLRP3) and the subsequent release of proinflammatory factors (27).
In vivo studies
A study of rotenone-induced male C57BL/6J mice proved that AM improved the behavioral deficiency induced by rotenone (28), and offset the oxidative stress in striatum and cortex, and decreased the degradation of DA neurons. DA can regulate innate immunity and inhibit systemic inflammation through different subtypes of dopamine receptor (DR) (61). DA also inhibits neuroinflammation in the brain through astrocyte DR2 and downstream signal transduction (62). Neuroinflammation is related to DA neurodegeneration and is a critical factor in the pathogenesis and progression of PD (63–66). A study modeled adult male C57BL/6 mice using LPS (67), and found that BAI could inhibit the activation of hippocampal glial cells and cytokine release, inhibit SIRT1 and downregulate the expression of high mobility group protein 1 (HMGB1) in microglial cells, preventing LPS-induced cognitive dysfunction and neuroinflammation, and producing neuroprotective effects. Another study modeled the male C57BL/6 mice via 1-methyl-4-phenyl-1,2,3,6-tetrahydropyridine (MPTP) (59), and found BAI protected dopaminergic neurons and rescued motor dysfunction. Chlorogenic acid (CGA) could downregulate rotenone-induced phosphorylated α-Syn levels by upregulating PI3K/AKT signaling pathway and inactivating GSK-3β via GLP-1, which improved rotenone-induced dopaminergic neurodegeneration and α-Syn accumulation in the substantia nigra, and enhanced striatal dopaminergic mean density of nerve fibers and eventually prevented rotenone-induced motor and cognitive impairments (26). NAR regulates PQ-induced DRD2, DAT, LRRK2, SNCA, β-linked protein, cystathionine-3 and BDNF genes, alleviates the loss of dopaminergic neurons (68). By modeling adult male Sprague-Dawley rats through LPS (27), it has been shown that NAR ameliorates LPS-induced decrease in TH protein expression and inhibits the activation of microglia and NLRP3 inflammasome, thus protecting DA neurons.
Therefore, it has been evidenced that polyphenols can disintegrate upstream α-Syn aggregation and inhibit the further development of PD. Moreover, polyphenols can also inhibit inflammation, reduce oxidative stress, play a neuroprotective role in regulating downstream events to protect DA neurons, and hinder the progression of PD.
Alzheimer's disease
AD is a neurodegenerative disease, which is the most common type of dementia. AD can lead to progressive cognitive decline, irreversible loss of memory and cognitive function, and interfere with daily activities. Its main characteristics are Aβ deposition of aggregates in extracellular amyloid plaques (senile plaques), followed by degenerated neurons containing neurofibrillary tangles (NFT), which are mainly composed of hyperphosphorylated microtubule-associated protein Tau and synaptic loss (1, 2, 69–73).
In vitro studies
The occurrence of AD is closely related to neuroinflammation. Some studies have found that neuroinflammation and microglia activation exist in the early stage of AD (74). In the AD model, microglia and astrocytes have been proven to produce various proinflammatory cytokines (3–5, 75). Resveratrol is a polyphenol commonly found in grape skins (Vitaceae). More and more studies have shown that resveratrol has anti-inflammatory, antioxidant, and anti-diabetes effects and can improve cognitive decline. Resveratrol has been proven to exert inhibitory activity on neuroinflammation and can inhibit the release of proinflammatory factors from microglia and astrocytes (76–78). Microglia can eliminate Aβ sediment and activate phagocytosis to restore tissue homeostasis (79, 80). However, when activation becomes chronic, microglia release excessive cytotoxic mediators, including proinflammatory cytokines, chemokines, complement components, ROS, and nitrogen species, which will cause neuronal cell degeneration (81, 82). Curcumin was first isolated from Curcuma longa L. in 1870 as a low molecular weight polyphenol compound. Studies have shown that curcumin has many beneficial pharmacological effects, including anti-cancer, anti-virus, anti-arthritis, anti-oxidative stress, anti-inflammatory, and neuroprotective properties (83). Inhibiting inflammation-promoting factors released by microglia are important targets of curcumin in treating AD. It has been shown that curcumin and its analogs cur 6 and cur 16 inhibit the secretion of pro-inflammatory mediators IL-1β and TNF-α released by microglia after stimulation of HMW Aβ42Os (32). The senile plaque can induce AD, and the “amyloid cascade hypothesis” is the most accepted hypothesis of AD etiology (2). Aβ42 oligomer is a soluble and diffusible Aβ species that play a key role in synaptic loss and synaptic damage in individuals with mild cognitive decline and can further trigger synaptotoxicity and neurotoxicity, glial cell proliferation, and activation, inflammation, or cell death (3–7, 84). Aβ also has the ability to bind to pattern recognition receptors on glial cells (including astrocytes and microglia), and it contributes to the progression and severity of AD (32, 85, 86). α-Mangostin (α-M) is a kind of polyphenol flavone from polygonatumodoratum, which has been proven to be effective for clearness of Aβ. It was found that α-M favored Aβ fiber generation, decomposition, uptake, and degradation, which reduced Aβ neurotoxicity induced by oligomer and prevented LPS-induced neuroinflammation (30).
In vivo studies
A study has investigated 90 AD patients by using a randomized double-blind method (87). Compared with the donepezil hydrochloride group, the resveratrol (RES) group achieved a significantly higher efficacy rate, MMSE score and FIM score, and the clinical indicators and ADAS cog score were significantly lower. There was no significant difference in the total incidence of adverse reactions. It proves that polyphenols have obvious effects on the treatment of AD diseases, including improvement of the inflammatory factor, and promotion of the cognitive function and prognosis (87).
Thus, polyphenols inhibit the formation and degradation of senile plaques in AD, thereby protecting the function of synapses. In addition, polyphenols play a role in inhibiting the release of inflammatory factors and neuroinflammation in AD, preventing the formation of NFTs and neuronal death, thus inhibiting the progression of AD.
Perspectives and conclusion
Polyphenols show a significant neuroprotective effect, but the lack of understanding of the underlying mechanism limits its clinical application. This minireview makes a comprehensive investigation to reveal the role and mechanism of polyphenols in the treatment of neurodegenerative diseases, including in vitro and in vivo studies. It has been found that polyphenols, as multi-target drugs, play a crucial role in inhibiting the formation of pathological products, such as oxidative stress and inflammatory factors. Polyphenols have two advantages as drugs for the treatment of neurodegenerative diseases. Firstly, polyphenols are derived from natural plants and can be obtained in daily diet. They have fewer side effects and are suitable for long-term use. Secondly, polyphenols can treat neurodegenerative diseases through multiple targets, which is undoubtedly crucial for the therapy of heterogeneous diseases. However, polyphenols are currently rarely used as drugs for the treatment of neurodegenerative diseases. Future large-scale multi-center randomized controlled trials and in-depth mechanism studies are still needed to fully evaluate the safety, effectiveness and possible side effects of polyphenols as therapeutic agents.
Author contributions
All authors listed have made a substantial, direct, and intellectual contribution to the work and approved it for publication.
Funding
This study was supported by the National Natural Science Foundation of China (31960178 and 82160923), Applied Basic Research Programs of Science and Technology Commission Foundation of Yunnan Province (2019FA007), Key Laboratory of Traditional Chinese Medicine for Prevention and Treatment of Neuropsychiatric Diseases, Yunnan Provincial Department of Education, Scientific Research Projects for High-level Talents of Yunnan University of Chinese Medicine (2019YZG01), Young Top-Notch Talent in 10,000 Talent Program of Yunnan Province (YNWR-QNBJ-2019-235), National Science and Technology Innovation 2030 Major Program (2021ZD0200900), Yunnan Key Research and Development Program (202103AC100005), and Yunnan Province Fabao Gao Expert Workstation Construction Project (202105AF150037), and Yunnan Province Qingguo Wang Expert Workstation Construction Project (202005AF150017).
Conflict of interest
The authors declare that the research was conducted in the absence of any commercial or financial relationships that could be construed as a potential conflict of interest.
Publisher's note
All claims expressed in this article are solely those of the authors and do not necessarily represent those of their affiliated organizations, or those of the publisher, the editors and the reviewers. Any product that may be evaluated in this article, or claim that may be made by its manufacturer, is not guaranteed or endorsed by the publisher.
References
1. Perl DP. Neuropathology of Alzheimer's disease. Mt Sinai J Med. (2010) 77:32–42. doi: 10.1002/msj.20157
2. Selkoe DJ, Hardy J. The amyloid hypothesis of Alzheimer's disease at 25 years. EMBO Mol Med. (2016) 8:595–608. doi: 10.15252/emmm.201606210
3. Masliah E, Mallory M, Alford M, DeTeresa R, Hansen LA, McKeel DW, et al. Altered expression of synaptic proteins occurs early during progression of Alzheimer's disease Neurology. (2001) 56:127–9. doi: 10.1212/WNL.56.1.127
4. Scheff SW, Price DA, Schmitt FA, DeKosky ST, Mufson EJ. Synaptic alterations in Ca1 in mild alzheimer disease and mild cognitive impairment. Neurology. (2007) 68:1501–8. doi: 10.1212/01.wnl.0000260698.46517.8f
5. Shankar GM, Walsh DM. Alzheimer's disease: synaptic dysfunction and abeta. Mol Neurodegener. (2009) 4:48. doi: 10.1186/1750-1326-4-48
6. Gong Y, Chang L, Viola KL, Lacor PN, Lambert MP, Finch CE, et al. Alzheimer's disease-affected brain: presence of oligomeric a beta ligands (addls) suggests a molecular basis for reversible memory loss. Proc Natl Acad Sci U S A. (2003) 100:10417–22. doi: 10.1073/pnas.1834302100
7. Peineau S, Taghibiglou C, Bradley C, Wong TP, Liu L, Lu J, et al. Ltp inhibits Ltd in the hippocampus via regulation of Gsk3beta. Neuron. (2007) 53:703–17. doi: 10.1016/j.neuron.2007.01.029
8. Manach C, Scalbert A, Morand C, Rémésy C, Jiménez L. Polyphenols: food sources and bioavailability. Am J Clin Nutr. (2004) 79:727–47. doi: 10.1093/ajcn/79.5.727
9. Neveu V, Perez-Jiménez J, Vos F, Crespy V, du Chaffaut L, Mennen L, et al. Phenol-explorer: an online comprehensive database on polyphenol contents in foods. Database. (2010) 2010:bap024. doi: 10.1093/database/bap024
10. Kim K-H, Tsao R, Yang R, Cui SW. Phenolic acid profiles and antioxidant activities of wheat bran extracts and the effect of hydrolysis conditions. Food Chem. (2006) 95:466–73. doi: 10.1016/j.foodchem.2005.01.032
11. Adom KK, Liu RH. Antioxidant activity of grains. J Agric Food Chem. (2002) 50:6182–7. doi: 10.1021/jf0205099
12. Chandrasekara A, Shahidi F. Content of insoluble bound phenolics in millets and their contribution to antioxidant capacity. J Agric Food Chem. (2010) 58:6706–14. doi: 10.1021/jf100868b
13. Pandey KB, Rizvi SI. Plant polyphenols as dietary antioxidants in human health and disease. Oxid Med Cell Longev. (2009) 2:270–8. doi: 10.4161/oxim.2.5.9498
14. Tsao R. Chemistry and biochemistry of dietary polyphenols. Nutrients. (2010) 2:1231–46. doi: 10.3390/nu2121231
15. Rana A, Samtiya M, Dhewa T, Mishra V, Aluko RE. Health benefits of polyphenols: a concise review. J Food Biochem. (2022) 46:e14264. doi: 10.1111/jfbc.14264
16. Rountree SD, Chan W, Pavlik VN, Darby EJ, Siddiqui S, Doody RS. Persistent treatment with cholinesterase inhibitors and/or memantine slows clinical progression of Alzheimer disease. Alzheimers Res Ther. (2009) 1:7. doi: 10.1186/alzrt7
17. Cheng YC, Sheen JM, Hu WL, Hung YC. Polyphenols and oxidative stress in atherosclerosis-related ischemic heart disease and stroke. Oxid Med Cell Longev. (2017) 2017:8526438. doi: 10.1155/2017/8526438
18. Potì F, Santi D, Spaggiari G, Zimetti F, Zanotti I. Polyphenol health effects on cardiovascular and neurodegenerative disorders: a review and meta-analysis. Int J Mol Sci. (2019) 20:351. doi: 10.3390/ijms20020351
19. Griñán-Ferré C, Bellver-Sanchis A, Izquierdo V, Corpas R, Roig-Soriano J, Chillón M, et al. The pleiotropic neuroprotective effects of resveratrol in cognitive decline and Alzheimer's disease pathology: from antioxidant to epigenetic therapy. Ageing Res Rev. (2021) 67:101271. doi: 10.1016/j.arr.2021.101271
20. Aryal S, Skinner T, Bridges B, Weber JT. The pathology of Parkinson's disease and potential benefit of dietary polyphenols. Molecules. (2020) 25:19. doi: 10.3390/molecules25194382
21. Lin K, Zhou M, Leng C, Tao X, Zhou R, Li Y, et al. Neuroprotective effect of polyphenol extracts from terminalia chebula retz. against cerebral ischemia-reperfusion injury. Molecules. (2022) 27:449. doi: 10.3390/molecules27196449
22. Yang Y, He B, Zhang X, Yang R, Xia X, Chen L, et al. Geraniin protects against cerebral ischemia/reperfusion injury by suppressing oxidative stress and neuronal apoptosis via regulation of the Nrf2/Ho-1 Pathway. Oxid Med Cell Longev. (2022) 2022:2152746. doi: 10.1155/2022/2152746
23. Ye M, Wu H, Li S. Resveratrol alleviates oxygen/glucose deprivation/reoxygenation-induced neuronal damage through induction of mitophagy. Mol Med Rep. (2021) 23:11. doi: 10.3892/mmr.2020.11711
24. Shi N, Zhu C, Li L. Rehabilitation training and resveratrol improve the recovery of neurological and motor function in rats after cerebral ischemic injury through the sirt1 signaling pathway. Biomed Res Int. (2016) 2016:1732163. doi: 10.1155/2016/1732163
25. Zaidi FK, Deep S. Scutellarin inhibits the uninduced and metal-induced aggregation of α-synuclein and disaggregates preformed fibrils: implications for Parkinson's disease. Biochem J. (2020) 477:645–70. doi: 10.1042/BCJ20190705
26. Sharma N, Soni R, Sharma M, Chatterjee S, Parihar N, Mukarram M, et al. Chlorogenic acid: a polyphenol from coffee rendered neuroprotection against rotenone-induced Parkinson's disease by Glp-1 secretion. Mol Neurobiol. (2022) 59:6834–56. doi: 10.1007/s12035-022-03005-z
27. Chen C, Wei YZ, He XM Li DD, Wang GQ Li JJ, et al. Naringenin produces neuroprotection against lps-induced dopamine neurotoxicity via the inhibition of microglial Nlrp3 inflammasome activation. Front Immunol. (2019) 10:936. doi: 10.3389/fimmu.2019.00936
28. Parekh P, Sharma N, Sharma M, Gadepalli A, Sayyed AA, Chatterjee S, et al. Ampk-dependent autophagy activation and alpha-synuclein clearance: a putative mechanism behind alpha-mangostin's neuroprotection in a rotenone-induced mouse model of Parkinson's disease. Metab Brain Dis. (2022) 37:2853–70. doi: 10.1007/s11011-022-01087-1
29. Wang QQ, Gao H, Yuan R, Han S, Li XX, Tang M. et al. Procyanidin A2, a polyphenolic compound, exerts anti-inflammatory and anti-oxidative activity in lipopolysaccharide-stimulated Raw2647 cells. PLoS ONE. (2020) 15:e0237017. doi: 10.1371/journal.pone.0237017
30. Hu X, Liu C, Wang K, Zhao L, Qiu Y, Chen H, et al. Multifunctional anti-Alzheimer's disease effects of natural xanthone derivatives: a primary structure-activity evaluation. Front Chem. (2022) 10:842208. doi: 10.3389/fchem.2022.842208
31. Chen Y, Bian Y, Wang JW, Gong TT, Ying YM, Ma LF, et al. Effects of α-mangostin derivatives on the Alzheimer's disease model of rats and their mechanism: a combination of experimental study and computational systems pharmacology analysis. ACS Omega. (2020) 5:9846–63. doi: 10.1021/acsomega.0c00057
32. De Lorenzi E, Franceschini D, Contardi C, Di Martino RMC, Seghetti F, Serra M, et al. Modulation of amyloid α-induced microglia activation and neuronal cell death by curcumin and analogues. Int J Mol Sci. (2022) 23:81. doi: 10.3390/ijms23084381
33. Pupyshev AB, Belichenko VM, Tenditnik MV, Bashirzade AA, Dubrovina NI, Ovsyukova MV, et al. Combined induction of mtor-dependent and mtor-independent pathways of autophagy activation as an experimental therapy for Alzheimer's disease-like pathology in a mouse model. Pharmacol Biochem Behav. (2022) 217:173406. doi: 10.1016/j.pbb.2022.173406
34. Wang H, Jiang T, Li W, Gao N, Zhang T. Resveratrol attenuates oxidative damage through activating mitophagy in an in vitro model of Alzheimer's disease. Toxicol Lett. (2018) 282:100–8. doi: 10.1016/j.toxlet.2017.10.021
35. Barthels D, Das H. Current advances in ischemic stroke research and therapies. Biochim Biophys Acta Mol Basis Dis. (2020) 1866:165260. doi: 10.1016/j.bbadis.2018.09.012
36. Liu J, He J, Huang Y, Hu Z. Resveratrol has an overall neuroprotective role in ischemic stroke: a meta-analysis in rodents. Front Pharmacol. (2021) 12:795409. doi: 10.3389/fphar.2021.795409
37. Wu MY, Yiang GT, Liao WT, Tsai AP, Cheng YL, Cheng PW, et al. Current mechanistic concepts in ischemia and reperfusion injury. Cell Physiol Biochem. (2018) 46:1650–67. doi: 10.1159/000489241
38. Caprio FZ, Sorond FA. Cerebrovascular disease: primary and secondary stroke prevention. Med Clin North Am. (2019) 103:295–308. doi: 10.1016/j.mcna.2018.10.001
39. Liao S, Apaijai N, Chattipakorn N, Chattipakorn SC. The possible roles of necroptosis during cerebral ischemia and ischemia / reperfusion injury. Arch Biochem Biophys. (2020) 695:108629. doi: 10.1016/j.abb.2020.108629
40. Gasparovic AC, Zarkovic N, Bottari SP. Biomarkers of nitro-oxidation and oxidative stress. Current Opinion Toxicol. (2018) 7:73–80. doi: 10.1016/j.cotox.2017.10.002
41. Jurcau A, Ardelean AI. Oxidative stress in ischemia/reperfusion injuries following acute ischemic stroke. Biomedicines. (2022) 10:3. doi: 10.3390/biomedicines10030574
42. Rodrigo R, Fernández-Gajardo R, Gutiérrez R, Matamala JM, Carrasco R, Miranda-Merchak A, et al. Oxidative stress and pathophysiology of ischemic stroke: novel therapeutic opportunities. CNS Neurol Disord Drug Targets. (2013) 12:698–714. doi: 10.2174/1871527311312050015
43. Celotti E, Ferrarini R, Zironi R, Conte LS. Resveratrol content of some wines obtained from dried valpolicella grapes: recioto and amarone. J Chromatogr A. (1996) 730:47–52. doi: 10.1016/0021-9673(95)00962-0
44. Pany S, Majhi A, Das J. Pkc Activation by Resveratrol Derivatives with Unsaturated Aliphatic Chain. PLoS One. (2012) 7:e52888. doi: 10.1371/journal.pone.0052888
45. Das J, Pany S, Majhi A. Chemical modifications of resveratrol for improved protein kinase C alpha activity. Bioorg Med Chem. (2011) 19:5321–33. doi: 10.1016/j.bmc.2011.08.008
46. Wang EH Yu ZL, Ping GF, Zhai S. Grape seed procyanidin extract attenuate sodium fluoride-induced oxidative damage and apoptosis in rat kidneys. Biomed Environ Sci. (2020) 33:454–7. doi: 10.3967/bes2020.061
47. Zeng YX, Wang S, Wei L, Cui YY, Chen YH. Proanthocyanidins: components, pharmacokinetics and biomedical properties. Am J Chin Med. (2020) 48:813–69. doi: 10.1142/S0192415X2050041X
48. Safwen K, Selima S, Mohamed E, Ferid L, Pascal C, Mohamed A, et al. Protective effect of grape seed and skin extract on cerebral ischemia in rat: implication of transition metals. Int J Stroke. (2015) 10:415–24. doi: 10.1111/ijs.12391
49. Trudler D, Nash Y, Frenkel D. New insights on Parkinson's disease genes: the link between mitochondria impairment and neuroinflammation. J Neural Transm. (2015) 122:1409–19. doi: 10.1007/s00702-015-1399-z
50. Dong AQ, Yang YP, Jiang SM, Yao XY Qi D, Mao CJ, et al. Pramipexole inhibits astrocytic nlrp3 inflammasome activation via Drd3-dependent autophagy in a mouse model of Parkinson's disease. Acta Pharmacol Sin. (2022). doi: 10.1038/s41401-022-00951-1
51. Cheng X-Y, Mao C-J, Wang Y-L, Liu C-F. Gastrointestinal symptoms of Parkinson's disease: a systematic review from pathogenesis to management. Adv Neurol. (2022) 1:1–16. doi: 10.36922/an.v1i1.9
52. Simon DK, Tanner CM, Brundin P. Parkinson disease epidemiology, pathology, genetics, and pathophysiology. Clin Geriatr Med. (2020) 36:1–12. doi: 10.1016/j.cger.2019.08.002
53. Fathi M, Vakili K, Yaghoobpoor S, Qadirifard MS, Kosari M, Naghsh N, et al. Pre-Clinical studies identifying molecular pathways of neuroinflammation in Parkinson's disease: a systematic review. Front Aging Neurosci. (2022) 14:855776. doi: 10.3389/fnagi.2022.855776
54. Kempuraj D, Thangavel R, Fattal R, Pattani S, Yang E, Zaheer S, et al. Mast cells release chemokine Ccl2 in response to parkinsonian toxin 1-methyl-4-phenyl-pyridinium (Mpp(+)). Neurochem Res. (2016) 41:1042–9. doi: 10.1007/s11064-015-1790-z
55. Lücking CB, Dürr A, Bonifati V, Vaughan J, De Michele G, Gasser T, et al. Association between early-onset Parkinson's disease and mutations in the parkin gene. N Engl J Med. (2000) 342:1560–7. doi: 10.1056/NEJM200005253422103
56. Kitada T, Asakawa S, Hattori N, Matsumine H, Yamamura Y, Minoshima S, et al. Mutations in the parkin gene cause autosomal recessive juvenile parkinsonism. Nature. (1998) 392:605–8. doi: 10.1038/33416
57. Wasner K, Smajic S, Ghelfi J, Delcambre S, Prada-Medina CA, Knappe E, et al. Parkin deficiency impairs mitochondrial DNA dynamics and propagates inflammation. Mov Disord. (2022) 37:1405–15. doi: 10.1002/mds.29025
58. Lastres-Becker I, Ulusoy A, Innamorato NG, Sahin G, Rábano A, Kirik D, et al. α-Synuclein expression and Nrf2 deficiency cooperate to aggravate protein aggregation, neuronal death and inflammation in early-stage Parkinson's Disease. Hum Mol Genet. (2012) 21:3173–92. doi: 10.1093/hmg/dds143
59. Lei K, Shen Y, He Y, Zhang L, Zhang J, Tong W, et al. Baicalin represses C/Ebpβ via its antioxidative effect in Parkinson's disease. Oxid Med Cell Longev. (2020) 2020:8951907. doi: 10.1155/2020/8951907
60. Fan Y, Li Y, Yang Y, Lin K, Lin Q, Luo S, et al. Chlorogenic acid prevents microglia-induced neuronal apoptosis and oxidative stress under hypoxia-ischemia environment by regulating the Mir497hg/Mir-29b-3p/Sirt1 axis. Dis Markers. (2022) 2022:1194742. doi: 10.1155/2022/1194742
61. Wu Y, Hu Y, Wang B, Li S, Ma C, Liu X, et al. Dopamine uses the Drd5-Arrb2-Pp2a signaling axis to block the Traf6-mediated Nf-Kb pathway and suppress systemic inflammation. Mol Cell. (2020) 78:42–56. doi: 10.1016/j.molcel.2020.01.022
62. Shao W, Zhang SZ, Tang M, Zhang XH, Zhou Z, Yin YQ, et al. Suppression of neuroinflammation by astrocytic dopamine D2 receptors via αb-crystallin. Nature. (2013) 494:90–4. doi: 10.1038/nature11748
63. Ransohoff RM. How neuroinflammation contributes to neurodegeneration. Science. (2016) 353:777–83. doi: 10.1126/science.aag2590
64. Hirsch EC, Hunot S. Neuroinflammation in Parkinson's disease: a target for neuroprotection? Lancet Neurol. (2009) 8:382–97. doi: 10.1016/S1474-4422(09)70062-6
65. Labzin LI, Heneka MT, Latz E. Innate immunity and neurodegeneration. Annu Rev Med. (2018) 69:437–49. doi: 10.1146/annurev-med-050715-104343
66. Guan Y, Han F. Key Mechanisms and potential targets of the Nlrp3 inflammasome in neurodegenerative diseases. Front Integr Neurosci. (2020) 14:37. doi: 10.3389/fnint.2020.00037
67. Li Y, Liu T, Li Y, Han D, Hong J, Yang N, et al. Baicalin ameliorates cognitive impairment and protects microglia from lps-induced neuroinflammation via the Sirt1/Hmgb1 pathway. Oxid Med Cell Longev. (2020) 2020:4751349. doi: 10.1155/2020/4751349
68. Ahmad MH, Fatima M, Ali M, Rizvi MA, Mondal AC. Naringenin alleviates paraquat-induced dopaminergic neuronal loss in Sh-Sy5y cells and a rat model of Parkinson's disease. Neuropharmacology. (2021) 201:108831. doi: 10.1016/j.neuropharm.2021.108831
69. García-Blanco A, Baquero M, Vento M, Gil E, Bataller L, Cháfer-Pericás C. Potential oxidative stress biomarkers of mild cognitive impairment due to alzheimer disease. J Neurol Sci. (2017) 373:295–302. doi: 10.1016/j.jns.2017.01.020
70. Li NM, Liu KF, Qiu YJ, Zhang HH, Nakanishi H, Qing H. Mutations of beta-amyloid precursor protein alter the consequence of Alzheimer's disease pathogenesis. Neural Regen Res. (2019) 14:658–65. doi: 10.4103/1673-5374.247469
71. Zheng W, Su Z, Liu X, Zhang H, Han Y, Song H, et al. Modulation of functional activity and connectivity by acupuncture in patients with alzheimer disease as measured by resting-state Fmri. PLoS ONE. (2018) 13:e0196933. doi: 10.1371/journal.pone.0196933
72. Cacace R, Sleegers K, Van Broeckhoven C. Molecular genetics of early-onset Alzheimer's disease revisited. Alzheimers Dement. (2016) 12:733–48. doi: 10.1016/j.jalz.2016.01.012
73. Orr ME, Sullivan AC, Frost B. A.brief overview of tauopathy: causes, consequences, and therapeutic strategies. Trends Pharmacol Sci. (2017) 38:637–48. doi: 10.1016/j.tips.2017.03.011
74. Femminella GD, Dani M, Wood M, Fan Z, Calsolaro V, Atkinson R, et al. Microglial activation in early alzheimer trajectory is associated with higher gray matter volume. Neurology. (2019) 92:e1331–43. doi: 10.1212/WNL.0000000000007133
75. Wasilewski D, Villalba-Moreno ND, Stange I, Glatzel M, Sepulveda-Falla D, Krasemann S. Reactive astrocytes contribute to Alzheimer's disease-related neurotoxicity and synaptotoxicity in a neuron-astrocyte co-culture assay. Front Cell Neurosci. (2021) 15:739411. doi: 10.3389/fncel.2021.739411
76. Potter KA, Buck AC, Self WK, Callanan ME, Sunil S, Capadona JR. The effect of resveratrol on neurodegeneration and blood brain barrier stability surrounding intracortical microelectrodes. Biomaterials. (2013) 34:7001–15. doi: 10.1016/j.biomaterials.2013.05.035
77. Wang F, Cui N, Yang L, Shi L, Li Q, Zhang G, et al. Resveratrol rescues the impairments of hippocampal neurons stimulated by microglial over-activation in vitro. Cell Mol Neurobiol. (2015) 35:1003–15. doi: 10.1007/s10571-015-0195-5
78. Zhao H, Wang Q, Cheng X, Li X, Li N, Liu T, et al. inhibitive effect of resveratrol on the inflammation in cultured astrocytes and microglia induced by Aβ(1-42). Neuroscience. (2018) 379:390–404. doi: 10.1016/j.neuroscience.2018.03.047
79. Hanisch UK, Kettenmann H. Microglia: active sensor and versatile effector cells in the normal and pathologic brain. Nat Neurosci. (2007) 10:1387–94. doi: 10.1038/nn1997
80. Parkhurst CN, Yang G, Ninan I, Savas JN, Yates JR 3rd, Lafaille JJ, et al. Microglia promote learning-dependent synapse formation through brain-derived neurotrophic factor. Cell. (2013) 155:1596–609. doi: 10.1016/j.cell.2013.11.030
81. Hickman SE, Allison EK, El Khoury J. Microglial dysfunction and defective beta-amyloid clearance pathways in aging Alzheimer's disease mice. J Neurosci. (2008) 28:8354–60. doi: 10.1523/JNEUROSCI.0616-08.2008
82. Doens D, Fernández PL. Microglia receptors and their implications in the response to amyloid β for Alzheimer's disease pathogenesis. J Neuroinflammation. (2014) 11:48. doi: 10.1186/1742-2094-11-48
83. Pulido-Moran M, Moreno-Fernandez J, Ramirez-Tortosa C, Ramirez-Tortosa M. Curcumin and health. Molecules. (2016) 21:264. doi: 10.3390/molecules21030264
84. Braak H, Braak E. Morphological criteria for the recognition of Alzheimer's disease and the distribution pattern of cortical changes related to this disorder. Neurobiol Aging. (1994) 15:355–6. doi: 10.1016/0197-4580(94)90032-9
85. Heneka MT, Carson MJ, El Khoury J, Landreth GE, Brosseron F, Feinstein DL, et al. Neuroinflammation in Alzheimer's disease. Lancet Neurol. (2015) 14:388–405. doi: 10.1016/S1474-4422(15)70016-5
86. Varnum MM, Ikezu T. The classification of microglial activation phenotypes on neurodegeneration and regeneration in Alzheimer's disease brain. Arch Immunol Ther Exp. (2012) 60:251–66. doi: 10.1007/s00005-012-0181-2
Keywords: polyphenols, antioxidant, anti-inflammation, neurodegenerative diseases, efficacy, mechanism
Citation: Li Z, Zhao T, Shi M, Wei Y, Huang X, Shen J, Zhang X, Xie Z, Huang P, Yuan K, Li Z, Li N and Qin D (2023) Polyphenols: Natural food grade biomolecules for treating neurodegenerative diseases from a multi-target perspective. Front. Nutr. 10:1139558. doi: 10.3389/fnut.2023.1139558
Received: 23 January 2023; Accepted: 13 February 2023;
Published: 28 February 2023.
Edited by:
Leo Veenman, Consorzio per Valutazioni Biologiche e Farmacologiche, ItalyReviewed by:
Ufuk Okkay, Atatürk University, TürkiyeCopyright © 2023 Li, Zhao, Shi, Wei, Huang, Shen, Zhang, Xie, Huang, Yuan, Li, Li and Qin. This is an open-access article distributed under the terms of the Creative Commons Attribution License (CC BY). The use, distribution or reproduction in other forums is permitted, provided the original author(s) and the copyright owner(s) are credited and that the original publication in this journal is cited, in accordance with accepted academic practice. No use, distribution or reproduction is permitted which does not comply with these terms.
*Correspondence: Zhaofu Li, lzf0817@126.com; Ning Li,
598122514@qq.com; Dongdong Qin,
qindong108@163.com
†These authors have contributed equally to this work