Development of a highly sensitive colloidal gold semiquantitative method for the determination of difenoconazole residues in citrus
- 1Hebei Key Laboratory of Quality & Safety Analysis-Testing for Agro-Products and Food, Hebei North University, Zhangjiakou, Hebei, China
- 2Hainan Inspection and Detection Center of Modern Agriculture, Haikou, Hainan, China
- 3Beijing Kwinbon Technology Co., Ltd., Beijing, China
- 4Beijing Engineering Research Centre of Food Safety Immunodetection, Beijing, China
Introduction: Difenoconazole (DIFE) is a common pesticide used in citrus cultivation; excessive intake can cause neurological damage to the organism, and the existing colloidal gold immunochromatographic test strips cannot meet the requirements for the detection of citrus samples.
Methods: Difenoconazole test strip was prepared based on the colloidal gold immunochromatographic technique (GICT), and its application in citrus samples was investigated; with colloidal gold (CG) as the probe, the optimization of GICT parameters, and the determination of reaction method, the immunochromatographic test strips for the detection of DIFE in citrus was developed, and the limit of detection (LOD), specificity, accuracy, and stability of the test strips were verified.
Results: The results showed that the visual detection limit of the prepared colloidal gold immunochromatographic test strips was 0.2 mg/kg and the quantitative range was 0.06–0.6 mg/kg, and the test strips could specifically identify DIFE and have no cross-reaction with other common triazole pesticides. The detection method established in this study was verified by the GC–MS method, and the detection results achieved good consistency (R2 > 0.98).
Conclusion: The test strips developed in this study have good performance and can be used for highly sensitive detection of citrus samples.
1 Introduction
Difenoconazole (DIFE) is a broad-spectrum, high-efficiency triazole systemic fungicide, which inhibits the growth of fungi by inhibiting the germination of pathogen spores, leading to their death, and has a preventive effect on vegetables, fruit trees, cereals, and other crops, with high adhesion and low degradability (1). In view of the high risk of DIFE to fish (2) and mammals (3) and its high levels in indoor dust (4) and water bodies (5), to prevent excessive intake of DIFE by humans, the residue limit of DIFE has been clearly set in GB.2763–2021 National Food Safety Standard - Maximum Residue Limit of Pesticides in Foods (6) issued by China, which is 0.01 mg/kg per person as the tolerable daily intake. Citrus is the main fruit crop, with an annual production of more than 100 million tons, and the production of oranges and tangerines alone reaches more than 50 million tons (7), making it one of the most vital cash crops in the world (8). Citrus is the most exported fruit in China (9), but with the continuous increase in citrus commercial planting area and yield, citrus diseases and pests, especially diseases, have increased gradually, and the problem of citrus simultaneously suffering from multiple diseases appears which seriously affects industrial development. DIFE is a specific agent for the prevention and treatment of some common diseases, such as citrus anthrax, citrus scab, and citrus sarcoidosis, but there are great food safety hazards associated with the high rate of DIFE exceedance due to excessive spraying, so testing DIFE residues in citrus is necessary.
At present, some studies have reported that classical chemical technologies employed for monitoring the difenoconazole residue, such as gas chromatography-tandem mass spectrometry (GC–MS) (10), the limit of detection (LOD) for difenoconazole was 0.02 mg/kg. The trace detection method for the determination of residues of difenoconazole in a plant sample by high-performance liquid chromatography–tandem mass spectrometry (HPLC-MS/MS) was developed (11). The detection limits for difenoconazole were 0.0002–0.0004 mg/kg, and the quantitation limits were 0.0044–0.011 mg/kg in citrus leaves and whole fruits. An effective method was developed to determine difenoconazole residues in pollen and honey of litchi by modified QuEChERS-HPLC-MS/MS (12). The LODs of difenoconazole were 0.25 μg/kg and 0.50 μg/kg. Although the chemical analytical methods are accurate, they are limited to laboratory analysis. Correct experimental results still need complex sample preparation methods, long detection time, and well-skilled personnel (13).
In addition to instrumental analysis methods, immunological methods have also been used to determine difenoconazole residues since such techniques are simple and sensitive (14). Immunoassays have been widely used to detect various analytes in biomedicine, food, and the environment because of the advantages of being simple, fast, and economical (15). Chen (16) established an indirect competitive enzyme-linked immunosorbent assay (ic-ELISA). Under this condition, the linear detection range (IC20 ~ IC80) of difenoconazole was 0.49 ~ 3.90 ng / mL, and the LOD was 0.33 ng/mL. A surface-enhanced Raman spectroscopy (SERS) has been reported to detect difenoconazole in banana (17). The results indicated a detection limit of 0.16 mg/kg in banana. However, the long reaction time, tedious operating procedures, and the need for specialized equipment have limited the application of ELISAs in field testing (18).
Compared with other detection methods, the GICT has low requirements on the purity of the sample, does not require complex sample pre-treatment, and is simple to operate during the experiment. It is less dependent on the instrument and is suitable for public use. In addition, it has low cost, short detection time, intuitive detection results, and can be detected on-site (19). At present, GICT is widely used in many fields such as food (20–22), clinical medicine (23, 24), environmental analysis (25, 26), and biology (27). However, in the application of rapid detection in food, trace substances to be detected usually exist in complex food matrices, which pose great challenges to traditional CG-ICA in terms of sensitivity and matrix tolerance (28). The extraction of pesticide residues in samples with organic solvents affects the chromatographic effect of the NC membrane and causes environmental pollution (29). The report on the analysis of DIFE based on the colloidal gold immunochromatographic technique is that Tingting Cui (30) used the colloidal gold immunochromatographic method to detect DIFE in fruits and vegetables, resulting in a detection limit of the test strip at 0.5 mg/kg, which could not meet the detection of citrus samples. Cai (31) reduced the sensitivity of aflatoxin B1 from 0.5 μg/L to 0.05 μg/L based on the lyophilized gold reaction form, with a 10-fold increase in sensitivity. The lyophilized gold was the gold standard antibody dispensed in the microtiter plate. In the reflective form of lyophilized gold, the gold-labeled antibody was fully recognized with the antigen, and the amount of antigen binding to the T-line was reduced, resulting in a lighter T-line. Since the binding site of the gold-labeled antibody with the secondary antibody was different from the antigen–antibody binding site, the reflective form of lyophilized gold did not affect the color development effect of the C-line, thus achieving the purpose of reducing the detection limit (32).
In this study, a semiquantitative colloidal gold immuno-chromatographic test strip analysis method was established with citrus as the sample and compared with the detection method specified in the national standard (33), demonstrating that the developed colloidal gold test strips were accurate, rapid, convenient, conducive to ecological protection, and could be used for the quantitative detection of large batches of samples.
2 Materials and methods
2.1 Materials
DIFE, Triadimenol, Triadimefon, Tebuconazole, and Myclobutanil standards (≥99%) were purchased from Dr. Ehrenstorfer, Germany; potassium carbonate, sucrose, phosphate, sodium carbonate, trisodium citrate (C6H5Na3O7), chloroauric acid (HAuCl4), and bovine serum albumin (BSA) were purchased from Sinopharm Chemical Reagent Co., Ltd.; sheep anti-mouse IgG, difenoconazole antigen, and difenoconazole monoclonal antibody were prepared by Beijing Bontem Biotechnology Co., Ltd.; nitrocellulose membrane CN140 was purchased from Millipore, USA; sample pads, gold standard pads, blotting paper, and backing plate were purchased from Shanghai Gold Standard Biotechnology Co., Ltd.; Citrus samples were purchased from supermarkets in Changping District, Beijing, China.
ESJ110-4A electronic balance (0.01 mg) was purchased from Shenyang Longteng Electronics Co., Ltd.; electrothermal magnetic stirrer was purchased from WIGGENS, Germany; SP-756P UV–Vis spectrophotometer was purchased from Shanghai Spectrum; H2100R high-speed frozen centrifuge was purchased from Hunan Xiangli Scientific Instruments Co., Ltd.; HM3035 gold spray film scribing instrument, CTS300 CNC cutting machine, and ZQ2402 microcomputer automatic chopping machine were purchased from Shanghai Gold Standard Biotechnology Co., Ltd.; GT-710 nano gold immunoassay reader was purchased from Beijing Kwinbon Technology Co., Ltd.; high-performance liquid chromatograph (UltiMate3000) was purchased from Thermo Fisher, USA; JSM 6701F scanning electron microscope was purchased from Japan Electronics.
2.2 Methods
2.2.1 Preparation of colloidal gold
The traditional trisodium citrate method (34) was used for the preparation: 1 mL of 1% concentration of the chloroauric acid solution was added to 98 mL of ultrapure water with stirring at a constant speed until boiling, followed by the rapid addition of 2 mL of 1.5% trisodium citrate solution; the rotational speed was accelerated and the solution was kept boiling for approximately 10 min, during which the solution gradually changed from colorless to yellow and finally to purple red. After 10 min, the heating was stopped, and the rotational speed was maintained until the solution temperature decreased to normal temperature, followed by filtrating with a 0.22-μm filter, and the solution was sealed with sealing film.
2.2.2 Identification of colloidal gold
The colloidal gold used the observation method, transmission electron microscopy method, and ultraviolet–visible spectrophotometer for identification. First of all, the color of colloidal gold was clear, transparent, and no particulate matter appears was observed; it indicated that colloidal gold was successfully fired. After a period of time, whether there was wall hanging, precipitation, flocculent, and other phenomena in the bottle was observed; if not, it indicated that the preparation of colloidal gold was stable. Then, the wavelength scanning of the gold particles was carried out by the ultraviolet–visible spectrophotometer to observe the peak width and the highest absorption peak, according to which the size and shape of the gold particles were calculated. Finally, scanning electron microscope (SEM) observation was the most intuitive way to determine the size and state of colloidal gold particles.
2.2.3 Preparation of gold standard antibodies
The key for gold nanoparticles to couple with antibodies and form a stable colloidal state lies in the pH of the colloidal gold solution, which is generally adjusted with HCl solution or K2CO3 solution. When the solution pH is adjusted to near the antibody isoelectric point, on the one hand, the gold nanoparticles are in equilibrium with the electrostatic and hydrophobic properties of the antibody proteins so that the nanoparticles remain colloidal and no aggregation or sedimentation phenomenon occurs; on the other hand, the antibody can be maximally adsorbed on the surface of gold nanoparticles, thus reducing the specific or non-specific binding of gold nanoparticles to other proteins and improving the gold standard antibody specificity (35).
2.2.3.1 Optimal labeling pH
In a centrifuge tube, 1 mL of colloidal gold solution was added, and the pH of the solution was adjusted with 0.2 mol/L K2CO3. Subsequently, 10 μL of DIFE antibody was added into each tube, and the solution was mixed and stood for 1 h at room temperature, and then 200 μL of 10% NaCl was added and stood for 2 h. The color change of the colloidal gold solution was observed. K2CO3 with less dosage and no color change in the solution is the pH optimum.
2.2.3.2 Optimal amount of labeled protein
In a centrifuge tube, 1 mL of colloidal gold solution was taken, and 1.5 μL of 0.2 mol/L K2CO3 solution was added; 0.5, 1, 1.5, 2, 2.5, and 3 μL of DIFE antibodies were added, respectively. The solution was left at room temperature for 1 h, while 200 μL of 10% NaCl was added and left overnight to observe the color change of the colloidal gold solution. The optimal amount of protein labeling was determined by the small amount of protein and no color change in the solution.
2.2.3.3 Optimal blocking solution
In a centrifuge tube, 2 mL of colloidal gold solution was taken, 3 μL of 0.2 mol/L K2CO3 solution was added, and then the determined DIFE antibody was added and allowed to stand for 10 min, waiting for the gold particles to couple with the protein. To ensure the specificity of the test strips and prevent non-specific binding, the other binding sites of the gold particles should be blocked with a blocking solution. In the centrifuge tube, 10% BSA, BSAH, and PEG 20000 were added, shaken well, and left for 10 min. The supernatant was removed by centrifugation at a speed of 10,000 r/min for 5 min at 4°C, the precipitation was retained, and 200 μL suspension was added and shaken for further use. The optimal blocking solution was judged by the color development status of the T and C lines.
2.2.4 Reaction method determination
The basic materials of the GICT system based on AuNFs as probes are shown in Figure 1A.
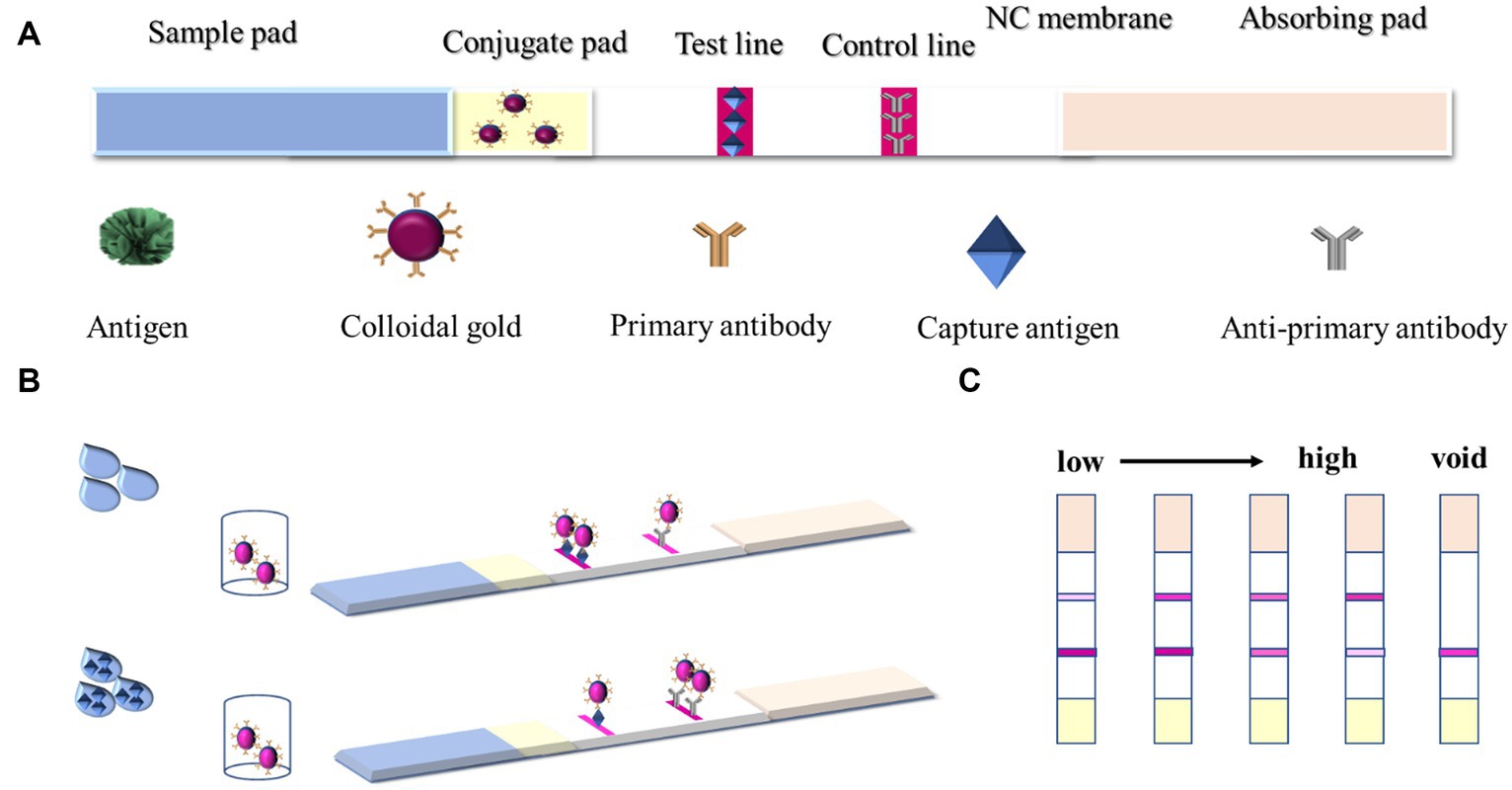
Figure 1. (A) Materials of the GICT system; (B) Colloidal gold immunochromatographic strip results; and (C) result determination.
GICT consists of four top-down parts: absorbent paper, NC film, gold standard pad, and sample pad. The gold standard pad was the glass fiber pad carrying the gold standard antibody, and the sprayed gold was the gold standard antibody evenly sprayed on the gold standard pad. The DIFE standard or sample solution was dropped into the prepared sample pad, and the results were provided within 10 min through capillary action and immune reaction. The test strips were prepared using the principle of competitive inhibition. When there is no DIFE in the sample or its concentration is below the LOD, the AuNFs-labeled mAb would bind to the coating antigen on the T line and the goat anti-mouse IgG on the C line, which generates the darker red T line and the red C line. With a gradual increase in the amount of DIFE in the test sample, the binding between AuNFs-labeled mAb and coating antigen is gradually inhibited by DIFE, so the red T line would gradually become lighter (Figure 1B). Determined results are shown in Figure 1C.
To prepare test strips that could satisfy citrus detection, the two methods were compared. The optimal reaction method was judged by comparing the inhibition ratio of standards at different concentrations. The AuNFs were prepared according to Table 1.
2.2.5 Determination of colloidal gold immunochromatographic test strip results
To the prepared lyophilized microtiter reagent, 100 μL of sample extract to be tested or diluted difenoconazole extract was added; after 5 min incubation, all of them were pipetted into the prepared test strips, and then the test strips were observed 10 min later to determine the results, as demonstrated in Figure 1B. If no or undetectable concentration was present in the sample, the antigen on the T-line bound to most of the gold standard antibodies and the colors of the T and C lines were all red. With the concentration of DIFE in the extract increasing gradually, the DIFE in the extract reacted with the gold standard antibodies preferentially, making the T-line color gradually lighter and the C-line color gradually darker, and when the C-line color was significantly darker than that of the T-line, the result was judged as positive; If the C-line did not show color, the test strip was invalid.
2.3 Sample testing
It was found in the pre-experiment that the CB solution was more suitable as a buffer for detecting pesticide residues in citrus, and it was particularly necessary to design a rational pre-treatment method due to the large difference in odor, rich pigmentation, high moisture content, and the great influence of sample matrix in citrus samples. In this experiment, six pre-treatment methods were designed using extraction solution, two-fold dilution, and centrifugation, from which the pre-treatment method with the highest inhibition rate was selected as the optimal pre-treatment method.
2.4 Test strip performance evaluation
2.4.1 Determination of standard curve and detection limit
The blank citrus was used as the sample to add the DIFE standard, which was diluted by extract and configured into 11 gradients of spiked samples of 0, 0.01, 0.02, 0.03, 0.06, 0.15, 0.3, 0.6, 1.2, 1.5, and 1.8 mg/kg, and titrated on the test strips after pre-treatment. The standard curve was developed by reading the test strips after the reaction, and the interval with a good gradient in the standard curve was selected to make the quantitative curve. The final added concentrations of DIFE in citrus blank samples were 0, 0.1, 0.2, 0.3, 0.5, and 1 mg/kg, and each level was repeated five times. The samples were extracted according to the method in Section 1.3.1, and the concentration at which the C-line showed significantly darker than the T-line in the naked eye test was the naked eye detection limit of the test strip.
2.4.2 Specificity and stability evaluation
Triadimenol, Triadimefon, Tebuconazole, Cyclobutanil, and other common triazole pesticides were configured to 10 mg/kg and tested with test strips to observe the color development of C and T lines to determine whether the test strips showed cross-reactivity.
The prepared test strips were stored at 45°C, 37°C, and 4°C and taken out for testing 30 days later. The stability of the test strips was determined by observing the test results.
2.4.3 Comparison experiments
The blank samples were prepared into 20 positive samples containing different concentrations of DIFE, which were mixed into other 20 blank samples for testing, and the samples were examined simultaneously by GC–MS/MS and colloidal gold immunochromatography for DIFE residues to determine the differences of the methods.
Another positive sample containing 0.1, 0.2, and 0.6 mg/kg DIFE was prepared, and 10 parallel tests were conducted for each level, and the fortified recoveries and coefficients of variation were calculated from the actual measured values, thereby judging the accuracy of the test strips.
Pre-treatment method of GC–MS method: The samples were processed according to GB23200.8–2016 and determined by gas chromatography–mass spectrometry analyzer (GC–MS).
The validation results were used to evaluate the sensitivity, specificity, accuracy, and consistency of the colloidal gold method with the reference method by Technical Specification for the Evaluation of Rapid Food Testing Methods.
3 Results and analysis
3.1 Quality identification of colloidal gold
First, by scanning electron microscopy (SEM), it was known that the particles were uniform in size, with a size of 25 ± 2 nm (Figure 2A). After a period of storage at normal temperature, no flocculent substances appeared, indicating that the colloidal gold structure was stable. Second, according to the UV–visible spectrophotometry, the particle size and dispersion state of gold particles determined that the maximum absorption peak was at 524 nm (Figure 2B), and the narrow peak width indicated that the gold particles were well dispersed, and the particle size of the gold particles was about 22 nm by calculation. Finally, the colloidal gold solution was observed by the naked eye, which was pink, clear, and transparent without blocky substance (Figure 2C), illustrating that the colloidal gold was prepared successfully.
3.2 Preparation of gold standard antibodies
3.2.1 Determination of the optimal pH for labeling
The initial colloidal gold solution pH was 4.22, with 0.2 mol/L K2CO3 to adjust the solution pH to 4.22, 5, 6, 7, 8, and 9, respectively. To each tube, 10 μL DIFE antibody was added and mixed. After adding the NaCl solution, the antibody surface charge was massively neutralized, resulting in protein precipitation. In addition, the number of water molecules on the protein surface determined its solution degree. When salt ions were added to the solution, water molecules had a greater affinity for salt ions than proteins, making protein solubility further reduced. If the affinity between gold particles and protein was high and the stable state of the protein was not easily destroyed, there was less protein precipitation phenomenon. After the test, it was found that when pH was 6, the color of the colloidal gold solution tended to be stable without precipitation or discoloration, so the optimal pH in this experiment was 6.
3.2.2 Determination of the optimal labeling amount
Similarly, using the salinization method, 0.5, 1, 1.5, 2, 2.5, and 3 μL of DIFE antibodies were added, respectively. When there were more antibodies in the solution that could not be adsorbed on the surface of gold particles, the phenomenon of protein precipitation aggregation would also occur. It was determined that the solution color tended to be stable when the addition of antibody was 1.5 μL/mL. Therefore, the optimal amount of antibody labeling was 1.5 μL/mL.
3.2.3 Identification of gold standard antibodies
As can be seen by SEM (Figure 3A), successful labeling could be judged due to the non-uniform particle size caused by the selective attachment of antibodies to the gold particles in different crystal orientations during the coupling process. It can be seen from Figure 3B that the wavelength of the gold particles labeled with antibody was shifted, and the change of the maximum absorption peak implied the change of the structure of the material to be tested, indicating that the protein and gold particles interacted with each other, and the protein adhered to the surface of the gold particles, resulting in the change of the refractive index, from which the gold standard antibody labeling could be preliminarily proved successful.
3.2.4 Reaction method
It can be seen from the table that the inhibition rate of the lyophilized gold form of the test strip was 91.47% when the concentration of the standard was 0.1 mg/kg, which was higher than that of the sprayed gold form (78.77%), explaining that the binding rate of the gold standard antibody to the antigen in the extract was higher; therefore, lyophilized gold was chosen as the reaction form of the test strip.
3.3 Test strip performance test
3.3.1 Determination of sample pre-treatment method
A total of 2 g blank citrus samples were accurately weighed into a 10 mL centrifuge tube, and the extraction solution was added to set the concentration as 0.2 mg/mL of DIFE spiked samples. The samples were prepared according to Table 2, and 100 μL extraction solution was taken for double parallel test strip assay, thus taking the optimal reaction conditions. After calculation, 2 g citrus samples were extracted by adding 2 mL of the CB solution, mixed by vortex for 1 min, put into a centrifuge, 3,000 r/min for 5 min, and 1 mL of the supernatant plus 3 mL extraction solution was taken as the optimal pre-treatment method.
3.3.2 Quantitative curve and detection limit analysis
The LOD is the minimum concentration or amount of a substance to be measured that can be detected from a sample by a particular analytical method within a given confidence level. The standard curve was drawn by double parallel test strip assay with 10 different gradients of mass fractions of DIFE as the horizontal coordinates and the T/C values of the test strip instrument as the vertical coordinates, as shown in Figure 4A. The interval with a good linear range in the standard curve was selected for curve fitting, as shown in Figure 4B, 0.06–0.6 mg/kg was used for linear fitting, and the regression equation was y = −0.3449x + 2.2796, R2 = 0.9906, and a quantitative detection range of 0.06–0.6 mg/kg.
The test results are shown in Figure 5. The citrus substrate had no significant effect on the test strip after 3-fold dilution, and the minimum concentration of the C-line significantly deeper than the T-line was 0.2 mg/kg, so the naked eye detection limit of the test strip was determined to be 0.2 mg/kg, that is, when the sample concentration in citrus was ≥0.2 mg/kg, the test strip was positive and the general test strip ODT/ODC was ≤0.6.
3.3.3 Specificity and stability analysis
As shown in Figure 6, after the cross-reaction test, it was found that there was no significant cross-reaction between DIFE and other commonly used triazole pesticides, and the test strip results were all negative. Because the concentration of the DIFE standard was too high, the gold-labeled antibody was sufficient with the antigen in the standard, and the gold-labeled antibody lacked the binding site with the T-line antigen, and the T-line was not colored. Therefore, the test strips could be considered to have good specificity.
After 30 days of destructive experiments, the final measured results are shown in Figure 7. After being stored at three temperature conditions for 30 consecutive days, the C-line showed color indicating that the test strips were still effective and the results were consistent, explaining that the test strips were stable and could be used for long-term storage at room temperature.
3.3.4 Comparison experiments
The results of 10 parallel tests for each spiked level of the same batch of test strips are shown in Table 3. The spiked recoveries of the prepared test strips were in the range of 66.5–76%, and the coefficients of variation were 8.92–11.94%, which met the assay requirements.
Comparison experiment results: Among the 40 prepared samples, 10 were randomly selected for blind testing, whose results are shown in Table 4. Within the limit of quantitation, the relative deviation of the two results was in the range of 4.02–27.69%, with a false positive rate of 0 and a false negative rate of 0. The correlation analysis of the two test results is shown in Figure 8. The linear equation of the test strip and the GC–MS test results are Y = 1.2022x − 0.022, R2 = 0.9864, with good linear correlation. The test results showed that the results of the well-prepared colloidal gold immunochromatographic test strips for DIFE were accurate and reliable, and could be well applied to the screening of DIFE residues in citrus.
4 Conclusion
In this experiment, a quantitative and rapid method for determining DIFE residues in citrus has been established. Compared with the detection limit of 0.5 mg/kg of the test strip created by Tingting Cui for the detection of DIFE, through the freeze-dried gold method, this method increased the detection limit by 2.5 times, resulting in a detection limit of the test strip at 0.2 mg/kg, a quantitative detection range of 0.06–0.6 mg/kg, and a reaction time of 10 min, which can meet the national standard for the detection of DIFE residues in citrus fruits. Compared with the GC–MS, the test strip was accurate, reliable, and had good specificity. No organic reagent was added to the extract, which prevented environmental pollution and was friendly to the environment. In the future, the sample extraction solution will be further explored, the extraction efficiency of DIFE and the pre-treatment method will be optimized, and the test strips with more convenient operation and higher sensitivity will be prepared.
Data availability statement
The original contributions presented in the study are included in the article/supplementary material, further inquiries can be directed to the corresponding authors.
Author contributions
RW: Writing – original draft, Writing – review & editing. MG: Writing – review & editing, Writing – original draft. YaL: Data curation, Writing – original draft. WZ: Software, Writing – original draft. KZ: Methodology, Writing – original draft. YZ: Validation, Writing – original draft. CY: Investigation, Writing – original draft. YuL: Funding acquisition, Resources, Writing – review & editing. JW: Funding acquisition, Resources, Writing – review & editing. YW: Funding acquisition, Methodology, Resources, Visualization, Writing – review & editing.
Funding
The author(s) declare financial support was received for the research, authorship, and/or publication of this article. The study was supported by the Key Research and Development Program Projects of Hebei (20325501D, 18227138D), the Heibei Open-field Vegetable Innovation Team of Modern Agro-industry Technology Research System (HBCT2024140209), and the Research on high-throughput detection technology and early warning decision-making platform for non-targeted pesticide residues in vegetables in Hainan (ZDYF2022XDNY232).
Acknowledgments
The authors would like to thank all the reviewers who participated in the review and the editor for their assistance during the preparation of this manuscript.
Conflict of interest
YW was employed by Beijing Kwinbon Technology Co., Ltd.
The remaining authors declare that the research was conducted in the absence of any commercial or financial relationships that could be construed as a potential conflict of interest.
Publisher’s note
All claims expressed in this article are solely those of the authors and do not necessarily represent those of their affiliated organizations, or those of the publisher, the editors and the reviewers. Any product that may be evaluated in this article, or claim that may be made by its manufacturer, is not guaranteed or endorsed by the publisher.
References
1. Man, YL, Stenrød, M, Wu, C, Almvik, M, Holten, R, Clarke, JL, et al. Degradation of difenoconazole in water and soil: kinetics, degradation pathways, transformation products identification and ecotoxicity assessment. J Hazard Mater. (2021) 418:126303. doi: 10.1016/J.JHAZMAT.2021.126303
2. Fan, RQ, Zhang, WJ, Jia, L, Li, LZ, Zhao, J, Zhao, ZM, et al. Combined developmental toxicity of the pesticides difenoconazole and dimethomorph on embryonic zebrafish. Toxins. (2021) 13:854–67. doi: 10.3390/toxins13120854
3. Khwanes, SA, Mohamed, RA, Ibrahim, KA, Abd el-Rahman, HA, et al. Ginger reserves testicular spermatogenesis and steroidogenesis in difenoconazole-intoxicated rats by conducting oxidative stress, apoptosis and proliferation. Andrologia. (2022) 54:e14241–54. doi: 10.1111/and.14241
4. Liu, J, Wan, YJ, Jiang, Y, Xia, W, He, ZY, Xu, SQ, et al. Occurrence of azole and strobilurin fungicides in indoor dustin three cities from China. Environ Pollut. (2022) 304:119168–8. doi: 10.1016/j.envpol.2022.119168
5. Liu, FX, Li, XQ, Bello, BK, Zhang, TM, Yang, HT, Wang, K, et al. Difenoconazole causes spleen tissue damage and immune dysfunction of carp through oxidative stress and apoptosis. Ecotoxicol Environ Saf. (2022) 237:113563–99179. doi: 10.1016/j.ecoenv.2022.113563
6. National Health Commission of the People’s Republic of China, Ministry of Agriculture and Rural Affairs of the People’s Republic of China, State Administration for Market Regulation . GB 2763— 2021 national food safety standard maximum residue limits for pesticides in food. Beijing: China Agriculture Press (2021).
7. Khamsaw, P, Sangta, J, Chaiwan, P, Rachtanapun, P, Sirilun, S, Sringarm, K, et al. Bio-circular perspective of Citrus fruit loss caused by pathogens: occurrences, active ingredient recovery and applications. Horticulturae. (2022) 8:748–8. doi: 10.3390/horticulturae8080748
8. Papoutsis, K, Mathioudakis, MM, Hasperué, JH, and Ziogas, V. Non-chemical treatments for preventing the postharvest fungal rotting of citrus caused by Penicillium digitatum (green mold) and Penicillium italicum (blue mold). Trends Food Sci Technol. (2019) 86:479–91. doi: 10.1016/j.tifs.2019.02.053
9. Fu, SJ, and Zhang, FA. Bbrief statistical analysis of China's export of fresh citrus fruits in 2019. Rome: Food and Agriculture Organization (2020).
10. Souza, LP, Faroni, LRDA, Heleno, FF, Pinto, FG, Queiroz, MELP, Prates, LHF, et al. Difenoconazole and linuron dissipation kinetics in carrots under open-field conditions. Ecotoxicol Environ Saf. (2019) 168:479–85. doi: 10.1016/j.ecoenv.2018.10.070
11. Song, QM, Wang, Y, Tang, SY, Meng, XR, Wang, F, Hu, DY, et al. Enantioselective analysis and degradation studies of four stereoisomers of Difenoconazole in Citrus by chiral liquid chromatography-tandem mass spectrometry. J Agric Food Chem. (2021) 69:501–10. doi: 10.1021/acs.jafc.0c05938
12. WANG, S, LIU, Y, SUN, H, du, L, XU, N, et al. Determination of myclobutanil and difenoconazole residues in pollen and honey of litchi by high performance liquid chromatography-tandem mass spectrometry. Chin J Chromatogr. (2018) 36:17–22. doi: 10.3724/SP.J.1123.2017.09034
13. Hassan, MM, Zareef, M, Jiao, TH, Liu, SS, Xu, Y, Viswadevarayalu, A, et al. Signal optimized rough silver nanoparticle for rapid SERS sensing of pesticide residues in tea. Food Chem. (2021) 338:127796. doi: 10.1016/j.foodchem.2020.127796
14. Liu, J, Xu, XX, Wu, AH, Song, SS, Xu, LG, Xu, CL, et al. Rapid and sensitive determination of difenoconazole in cucumber and pear samples using an immunochromatographic assay. Food Biosci. (2022) 47:101745. doi: 10.1016/J.FBIO.2022.101745
15. Sang, P, Hu, Z, Cheng, Y, Yu, H, Xie, YF, Yao, WR, et al. Nucleic acid amplification techniques in immunoassay: an integrated approach with hybrid performance. J Agric Food Chem. (2021) 69:5783–97. doi: 10.1021/ACS.JAFC.0C07980
16. Chen, ZH, Zhang, YY, Yang, JS, Zeng, DP, He, Y, Xu, ZL, et al. Highly sensitive immunoassay of difenoconazole in fruits and environmental water samples. J Instrum Anal. (2023) 42:768–74. doi: 10.19969/j.fxcsxb.23022801
17. Du, WY, Ma, D, Li, B, Yin, QC, Cao, N, Tan, MN, et al. Rapid detection of difenoconazole and chlorpyrifos residues in banana by surface enhanced Raman spectroscopy. Food Chem. (2023) 14:238–44. doi: 10.19812/j.cnki.jfsq11-5956/ts.2023.08.031
18. Liu, X, Li, J, Huang, Y, Zhang, Z, Lin, Q, Xia, P, et al. Rapid and sensitive detection of quizalofop-p-ethyl by gold nanoparticle-based lateral flow immunoassay in agriproducts and environmental samples. Sci Total Environ. (2023) 857:159427. doi: 10.1016/J.SCITOTENV.2022.159427
19. Liu, GY, Lu, M, Huang, XD, Li, TF, Xu, DH, et al. Application of gold-nanoparticle colorimetric sensing to rapid food safety screening. Sensors. (2018) 18:4166–6. doi: 10.3390/s18124166
20. Xu, X, Guo, L, Wu, AH, Liu, LQ, Kuang, H, Xu, LG, et al. Rapid and sensitive detection of flubendiamide in grapes and tomatoes using a colloidal gold immunochromatography assay. Food Addit Contam Part A Chem Anal Control Expo Risk Assess. (2022) 39:1843–54. doi: 10.1080/19440049.2022.2120635
21. Lin, L, Xu, XX, Song, SS, Liu, LQ, Kuang, H, Wang, ZY, et al. A colloidal gold immunochromatographic strip for quantitative detection of azoxystrobin in vegetables. New J Chem. (2021) 45:9002–9. doi: 10.1039/D1NJ01191J
22. Xin, YR, Yang, RL, Qu, Y, Liu, HF, Feng, YS, Li, L, et al. Novel, highly sensitive, and specific assay to monitor acute myocardial infarction (AMI) by the determination of cardiac troponin I (cTnI) and heart-type fatty acid binding protein (H-FABP) by a colloidal gold-based immunochromatographic test strip. Anal Lett. (2021) 54:1329–50. doi: 10.1080/00032719.2020.1802594.374
23. Mao, MX, Chen, XJ, Cai, YN, Yang, HJ, Zhang, CZ, Zhang, Y, et al. Accelerated and signal amplified nanozyme-based lateral flow assay of acetamiprid based on bivalent triple helix aptamer. Sens Actuators B: Chem. (2023) 378:133148. doi: 10.1016/J.SNB.2022.133148
24. Xiao, XM, Zhou, QT, Zhu, JH, Sun, L, Zhang, H, Sun, YC, et al. False-positive colloidal gold-based immunochromatographic strip assay reactions for antibodies to SARS-CoV-2 in patients with autoimmune diseases. Ann Transl Med. (2021) 9:534–4. doi: 10.21037/atm-20-6509
25. Qi, M, Huo, JQ, Li, ZF, He, C, Li, DY, Wang, YX, et al. On-spot quantitative analysis of dicamba in field waters using a lateral flow immunochromatographic strip with smartphone imaging. Anal Bioanal Chem. (2020) 412:1–12. doi: 10.1007/s00216-020-02833-z
26. Sun, WM . Research on immunoassay techniques for carbofuran and the metabolite residues in agricultural products and water [master's thesis]. Haikou (HN): Hainan University (2021).
27. Xifeng, W, Mengfan, Q, Kai, Z, Guowu, Z, Jing, L, Lixia, W, et al. Development and evaluation of a colloidal gold immunochromatographic assay based on recombinant protein CatL1D for serodiagnosis of sheep fasciolosis. J Helminthol. (2020) 94:E98. doi: 10.1017/S0022149X19000919
28. Chen, YY, Lu, HX, Shi, JL, Zhu, JL, Wang, HF, et al. An improved up-conversion nanoparticles-based immunochromatographic assay for rapid detection of zearalenone in cereals. Food Chem. (2023) 412:135555. doi: 10.1016/j.foodchem.2023.135555
29. Ma, L, Zhao, Y, Chen, JB, Zhao, L, et al. Rapid detection of chlorothalonil residues in blueberry based on colloidal gold immunochromatographic assay. Chin J Pestic Sci. (2023) 25:435–43. doi: 10.16801/j.issn.1008-7303.2022.0133
30. Cui, TT, Zhu, LL, Tan, C, Wang, J, Wang, ZQ, Wan, YP, et al. Development of difenoconazole colloidal gold immunochromatographic strip and its application in vegetables. Food Ind. (2022) 43:175–9.
31. Cai, F . Study of improved Immunechromatographic lateral flow strip for accurate detection of aflatoxin B1[master's thesis]. Hefei: HeFei University of Technology (2020).
32. Li, J . Effect of different-sized spherical gold nanoparticles on the sensitivity of an immunochromatog raphic assay [master's thesis]. Nanchang: Nan Chang University (2016).
33. National Health and Family Planning Commission of the People’s Republic of China, Ministry of Agriculture and Rural Affairs of the People’s Republic of China, State Administration for Market Regulation . National food safety standards—Determination of 500 pesticides and related chemicals residues in fruits and vegetables gas chromatography-mass spectrometry. Beijing: Standards Press of China (2017).
34. Iqbal, M, Usanase, G, Oulmi, K, Aberkane, F, Bendaikha, T, Fessi, H, et al. Preparation of gold nanoparticles and determination of their particles size via different methods. Mater Res Bull. (2016) 79:97–104. doi: 10.1016/j.materresbull.2015.12.026
Keywords: difenoconazole, detection limit, gold nanoparticles, colloidal gold immunochromatographic technique, rapid diagnosis strips
Citation: Wang R, Gong M, Liu Y, Zhu W, Zhang K, Zhao Y, Yin C, Liu Y, Wang J and Wan Y (2024) Development of a highly sensitive colloidal gold semiquantitative method for the determination of difenoconazole residues in citrus. Front. Nutr. 11:1341219. doi: 10.3389/fnut.2024.1341219
Edited by:
Fuguo Xing, Chinese Academy of Agricultural Sciences, ChinaReviewed by:
Guangyang Liu, Chinese Academy of Agricultural Sciences, ChinaYing Shang, Kunming University of Science and Technology, China
Copyright © 2024 Wang, Gong, Liu, Zhu, Zhang, Zhao, Yin, Liu, Wang and Wan. This is an open-access article distributed under the terms of the Creative Commons Attribution License (CC BY). The use, distribution or reproduction in other forums is permitted, provided the original author(s) and the copyright owner(s) are credited and that the original publication in this journal is cited, in accordance with accepted academic practice. No use, distribution or reproduction is permitted which does not comply with these terms.
*Correspondence: Yuan Liu, bfxyliuyuan@126.com; Jian Wang, xuanyuanjian0228@126.com; Yuping Wan, wyp@kwinbon.com
†These authors have contributed equally to this work and share first authorship