Protein C Pathway in Paediatric and Neonatal Sepsis
- 1National Children's Research Centre, Dublin, Ireland
- 2Department of Paediatrics, Trinity Translational Medicine Institute, Trinity College Dublin, Dublin, Ireland
- 3Department of Paediatrics, Children's Health Ireland at Tallaght, Dublin, Ireland
- 4Trinity Research in Childhood Centre, Dublin, Ireland
- 5Department of Haematology, Children's Health Ireland at Crumlin, Dublin, Ireland
- 6Department of Neonatology, Children's Health Ireland at Crumlin, Dublin, Ireland
- 7Department of Paediatrics, Coombe Women's and Infant's University Hospital, Dublin, Ireland
Protein C plays a major role in the physiological regulation of coagulation pathways through inactivation of factor Va, factor VIIIa, and plasminogen activator inhibitor. Protein C is involved in the control of inflammation during sepsis, by inhibiting release of pro-inflammatory cytokines, thereby controlling neutrophil, and monocyte effects on injured tissue. Recombinant human activated protein C (rhAPC) reduced mortality in adult sepsis in earlier studies but had no significant benefit in more recent trials. Protein C levels are reduced during paediatric and neonatal sepsis, which may play a major role in the development of disseminated intravascular thrombosis, purpura fulminans, and multiorgan dysfunction. The role of protein C in paediatric sepsis requires further clinical and immunological evaluation to define the patient subgroups who may benefit from this therapy. Newer versions of rhAPC are under development with less risk of haemorrhage potentially broadening the scope of this intervention.
Introduction
Protein C was first isolated by Johan Stenflo from bovine plasma in 1976 and named “C” because it was the third protein eluted from DEAE-Sepharose (1). It is a 62-kD, two-chain glycoprotein encoded by the PROC (protein C, inactivator of coagulation factors Va and VIIIa) gene, located on the second chromosome (2q13-q14). Protein C is an endogenous vitamin K-dependent glycoprotein that circulates in plasma as an inactive zymogen (2). The concentration of protein C in a healthy term infant ranges from 25 to 40 IU/dl and achieves adult levels after puberty (65-135 IU/dl) (3). Protein C has a half-life of 7-10 h. It is activated by thrombin bound to the cell surface glycoprotein thrombomodulin (TM) (4) to generate the anticoagulant activated protein C (APC), which has a half-life of only approximately 30 min in adult plasma. This TM-APC pathway plays an important role in maintaining homeostasis. Protein C activation by the thrombin-TM complex is enhanced by binding to the endothelial protein C receptor (EPCR) (5, 6).
Activated protein C (APC) mediates its anticoagulant function by cleaving and inactivating cofactors Factor Va (7) and FVIIIa (8). APC inactivation of FVa in plasma is dependent on protein S (9). Protein S is an anticoagulant plasma protein and a member of the protein C anticoagulant pathway, there it acts as a cofactor for APC. Protein S mediates its APC cofactor activity by enhancing the inactivation of activated coagulation FVa and FVIIIa (10).
Both protein S and procofactor FV act as cofactors to APC in inactivation of FVIIIa (11). In addition to its anticoagulant functions, APC has recently been shown to indirectly mediate protective intracellular signalling on multiple cell types. These “cytoprotective” properties result in endothelial barrier stabilisation (12), inhibition of inflammation (13–15) and apoptosis (16, 17). APC cytoprotective signalling on endothelial cells is mediated primarily by binding of APC to EPCR followed by cleavage of protease activated receptor 1 (PAR1) and PAR3 by the active site of APC (10, 12, 15–20). Alternative mechanisms for APC signalling on different cell types have also been proposed which are EPCR-independent and PAR-dependent (15) and EPCR and PAR1-independent (14).
In this narrative review the search terms: protein c, activated protein c and sepsis were used across all age-groups and included all levels of evidence in Pubmed. Adult studies were included due to the paucity of paediatric and neonatal research in this area and to explain mechanisms of action.
Protein C Deficiency
Deficiency of protein C can be classified into two types; Type 1 describes a low level of Protein C with normal function in the circulation, which can be hereditary or acquired. In type 2, there is a normal level of protein C with abnormal function, which also can be hereditary or acquired. The hereditary form of type 1 protein C deficiency is rare genetic disorder. In heterozygote form, the activity levels of protein C are approximately 50% of normal and have a variable phenotypic expression from asymptomatic to recurrent venous thrombosis and abortion (21). The incidence of venous thromboembolism is 0.52 per 100 patient-years (22). Homozygous protein C deficiency is a rare autosomal recessive disorder with an estimated incidence of 1 in 0.5-1 million live births. Neonates may develop disseminated intravascular coagulation (DIC), purpura fulminans, vitreal vein thrombosis (absent red reflex and blindness), or perinatal ischemic stroke (23–25). The natural course is fatal unless diagnosed early and rapidly managed with replacement therapy, rhAPC. Long term management includes anticoagulant therapy with or without rhAPC supplementation. Factor V Leiden is an example of a hereditary type 2 protein C deficiency, in which there is an inability of Protein C to degrade Factor V, resulting in hypercoagulability (26). Acquired Protein C deficiency is caused by increased consumption of protein C during sepsis, hepatic synthetic dysfunction, and administration of vitamin K antagonists or as a complication of prematurity (25).
Protein C and Inflammation
APC diminishes both the excessive coagulation and inflammatory responses in sepsis, ultimately decreasing mortality (27). In an animal model of E. coli infection, Taylor et al. have shown that treatment with APC protects against a lethal inflammatory response and the inhibition of protein C activation exacerbates this response (28). Protein C-deficient mice with sepsis exhibit hypotension, DIC, elevated inflammatory mediators, neutrophil adhesion to the microvascular endothelium, and loss of protective endothelial and epithelial cell barriers (29). In a double-blinded placebo-controlled trial, 16 normal adults were randomised to receive either rhAPC or normal saline, to investigate the effect of rhAPC in reducing human endotoxin–induced pulmonary inflammation. There was decreased neutrophil chemotaxis following exposure to rhAPC in vitro, but no difference was detected in gene expression, kinase activation, cytokine release, cell survival, or apoptosis of neutrophils (30). Inhibition of the protein C pathway increasesendothelial cell injury, cytokine elaboration and leukocyte extravasations in response to endotoxin, processes that are decreased by infusion of APC. The production of inflammatory cytokines [tumour necrosis factor (TNF)-α, Interleukin (IL)-1β, and IL-6] are inhibited by APC. It also limits the rolling of neutrophils on injured endothelium by binding selectins such as CD11b/CD18 (Mac-1), a β2 integrin involved in neutrophil signalling and cell adhesion (31).
In addition to its role in anticoagulation, APC is barrier-protective, pro-survival and has anti-inflammatory properties (32). APC is protective in ischemia-reperfusion injury by inhibition of the nucleotide-binding domain(NOD)-like receptor protein C (NLRP3) inflammasome through mammalian target of rapamycin complex-1 (mTORC1), demonstrating a potential novel way to therapeutically utilise APC (33). Neonates with sepsis have an increased risk of death if PC activity is ≤ 10%, suggesting the potential of using PC as a predictive marker of outcome in neonatal sepsis (34).
The Protein C anticoagulant pathway plays an important role in preventing microvascular thrombosis (35) and is initiated when thrombin binds to TM on the surface of the endothelium (36) (Figure 1). Protein C binds EPCR, augmenting protein C activation by the thrombin-TM complex more than 10-fold in vivo (37) and is shed from the endothelium by inflammatory mediators and thrombin. EPCR binds to activated neutrophils in a process that involves proteinase 3 and CD11b/CD18 and inhibits leukocyte extravasation. EPCR can translocate from the plasma membrane to the nucleus where it redirects gene expression, which possibly accounts for APCs ability to modulate the inflammatory responses in the endothelium (29). TNF-α and other inflammatory mediators can down-regulate EPCR and TM. APC inhibits TNF-α release from monocytes and blocks leukocyte adhesion to selectins. In addition to its classic role as a feedback inhibitor of thrombin, APC suppresses Macrophage Migratory Inhibitory Factor (MIF) and TNF-α mediated effects in the endothelial cell by down-regulating NFκB sub-units and subsequent inhibition of inflammatory cell adhesion (13). Treatment of endothelial cells with rhAPC blocks the induction of apoptosis and modulates up-regulation of the anti-apoptotic genes A1, endothelial nitric oxide synthase (eNOS) and inhibitor of apoptosis protein (38).
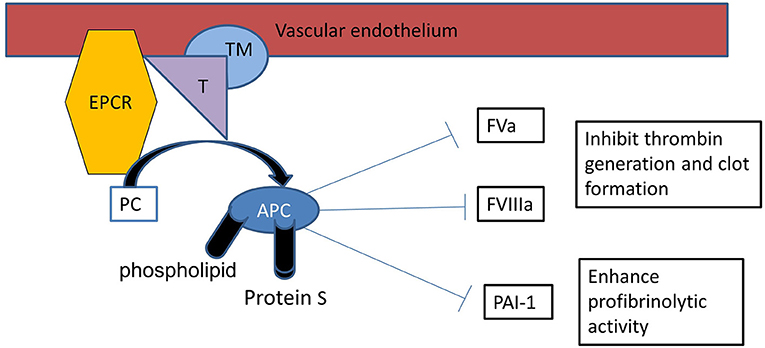
Figure 1. Protein C activation: Protein C binds with thrombin (T)-thrombomodulin (TM) complex on endothelial surface with it is receptor endothelial protein C receptor (EPCR), resulting in activation of protein C (APC). APC proteolytically inactivates FVa and FVIIIa, therefore APC is an important inhibitor of the clotting cascade. APC also inhibits plasminogen activator inhibitor-1 (PAI-1) enhancing profibrinolytic activity.
APC inhibits neutrophil activation in spinal cord injury and contributes to decreased ischaemic cord damage (39). Increased transcriptional expression of lung inflammatory mediators (TNF-α, IL-1β, and IL-6) in response to hyperoxia is attenuated with APC administration and associated with decreased acute lung injury (40). APC is protective in the liver and kidney after liver ischemia and reperfusion (41).
Protein C as Treatment for Sepsis
Activated protein C has a major role in control of coagulation and inflammation during sepsis. Tissue injury and exposure of tissue factor in sepsis results in the activation of the coagulation cascade and thrombin generation (Figure 2). Activated phagocytic cells stimulate inflammatory cytokines mediating altered protein transcription resulting in the development of systemic inflammation. Systemic inflammation increases the synthesis of the prothrombotic proteins factor VIII, von Willebrand factor (vWF) and fibrinogen, with decreased synthesis of the regulatory proteins of the coagulation cascade-antithrombin, protein C and protein S (42, 43).
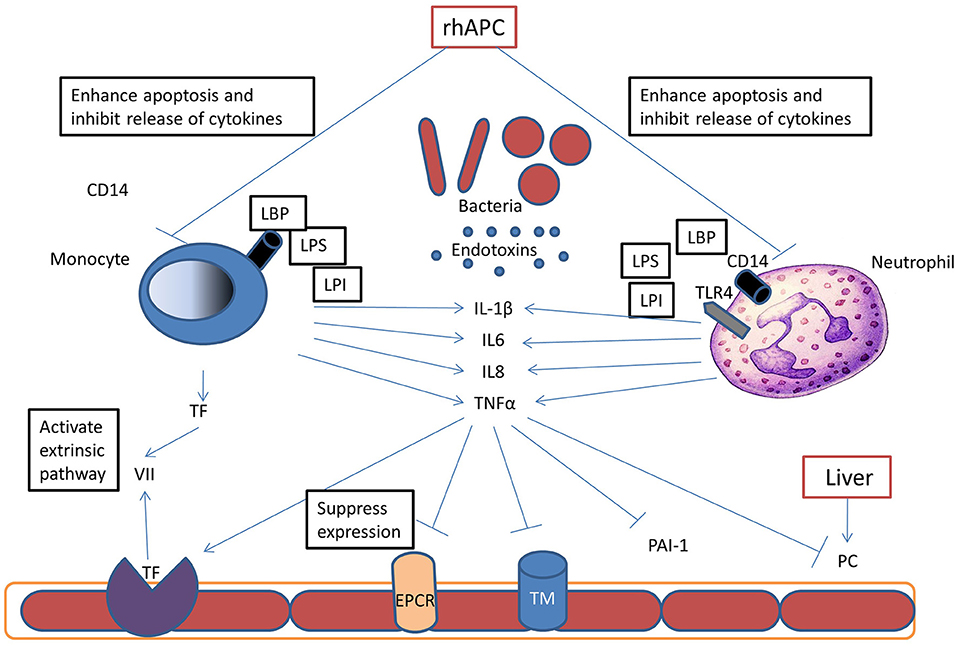
Figure 2. Pathophysiology of Protein C in Sepsis: during sepsis, bacteria release endotoxins (LPS, LPI, LBP), these endotoxins bind to different receptors on neutrophils and monocytes and stimulate inflammatory cytokine release. These cytokines promote thrombin generation by upregulation of tissue factor (TF) expression on monocytes and endothelium. Inflammatory cytokines also inhibit protein C synthesis in the liver and reduce thrombomodulin (TM) and endothelial protein C receptor (EPCR) expression, and thereby inhibit APC formation. Recombinant human activated protein C (rhAPC) enhances neutrophils and monocytes apoptosis and therefore inhibits cytokines release and reducing tissue damage.
Clinical studies investigating the safety and efficacy of APC in the treatment of sepsis primarily use either recombinant human activated protein C (rhAPC or drotrecogin alpha activated) or plasma-derived protein C (Table 1). Decreased protein C levels (<50% of normal) have been associated with a poor outcome in adult severe sepsis (53). In the PROWESS (Recombinant Human Activated Protein C Worldwide Evaluation in Severe Sepsis) trial, 1690 patients diagnosed with severe sepsis were randomised in a double blind, placebo-controlled, multicentre study. They were assigned to either rhAPC or placebo. Patients on rhAPC had protein C activity less than the lower limit of normal (81%) in the 24 h before starting treatment. At day 28, 30.8% of the placebo group and 24.7% of the rhAPC group had died (p = 0.005) in the non-stratified analysis. There was a reduction in mortality for all individual organisms, especially Streptococcus pneumoniae infection (RR, 0.56; 95% CI, 0.35-0.88) (31). In the ADDRESS (the Administration of rhAPC Drotrecogin Alfa Activated in Early Stage Severe Sepsis Study Group) study, 2,640 adult patients diagnosed with severe sepsis with at least one organ dysfunction and with low risk of death were randomised in a double-blind, placebo-controlled, multicentre trial. There was no statistical difference in outcomes between placebo and treatment groups (17 vs. 18.5%; p = 0.34) (45). The RESPOND [Research Evaluating Serial Protein C levels in Severe Sepsis Patients on Drotrecogin alfa (activated)] study was a phase II double blind study, using endogenous protein C levels (pre-treatment, treatment and post-treatment) as a biomarker to guide the dose and duration of treatment with rhAPC. Four hundered and eighty eight patients with protein C deficiency and sepsis induced multi-organ dysfunction started treatment within 36 h of the first sepsis-induced organ dysfunction. All patients received rhAPC for 24 h and then were randomised to either a further standard 72 h of rhAPC or high dose with infusion duration dependent on their protein C level reaching the normal range. The latter strategy resulted in a greater increase in protein C levels than standard therapy. The data shows that protein C may be an effective biomarker for patients with severe sepsis and who may respond to rhAPC (54).
The EXPRESS (Early PREdiction of Severe Sepsis) study was a randomised, double blind, phase 4 equivalence design trial (n = 1,194) of adults with severe sepsis comparing rhAPC in combination with either heparin (low molecular weight or unfractionated heparin) or placebo. Both heparin and placebo groups had similar occurrences of severe bleeding and 28-day mortality rate (28.3 vs. 31.9%; p = 0.08). Patients receiving placebo had higher mortality (35.6 vs. 26.9%; p = 0.005) compared to a subgroup of patients in the heparin group. Significant differences were observed for bleeding events (heparin v placebo: n = 105 v 78; p = 0.049), and for ischemic stroke (heparin v placebo: n = 3 v 12; p = 0.02) (55, 56).
The Cochrane review of rhAPC in sepsis found four eligible studies with 4,911 patients (including 477 children). The risk of death was not reduced by rhAPC in adult patients at 28 days (p = 0.50). In two studies, there was no association between the effectiveness of APC and degree of severity of sepsis, although rhAPC was associated with significant risk of bleeding (p = 0.02) and they concluded that there was insufficient evidence to support the use of human rhAPC in children or adults with severe sepsis (44). Marti et al. published similar recommendations in a further Cochrane review in 2012 (44).
A multicentre, randomised, double blind, placebo-controlled study of 193 adults with severe sepsis and vasopressor-dependent hypotension receiving either an extended rhAPC infusion or placebo found no difference in mortality, organ dysfunction, and duration of hypotension (57). The extended infusion group had a lower percentage change in D-dimers (p < 0.001) with increase in plasma protein C activity levels. There was no difference in serious adverse events including bleeding events.
The PROWESS-SHOCK study was a placebo-controlled adult trial of rhAPC in SIRS. All-cause mortality at 28-days was the primary outcome and was not reduced by rhAPC. This prompted the withdrawal of rhAPC (Xigris) from the commercial market by Eli-Lilly (44, 56).
Protein C and Paediatric Sepsis
The incidence of paediatric sepsis in the United States is 56 per 100,000 per year, and 50% of those children have an underlying disease. The annual cost is estimated at 2 billion dollars (58). Two thousand children are admitted to paediatric intensive care units in the United Kingdom annually with presumed sepsis with 20% mortality (46, 59). Protein C administration to children with febrile neutropenia significantly decreased the duration of the episode but there was no association between bacteraemia and protein C, soluble ECPR, soluble TM, and fibrin degradation products (60). In paediatric patients with septic shock, very low levels of protein C activity are associated with increased mortality (61). In children <1 year of age the protein C promoter genotype (CG-CG genotype) was associated with increased susceptibility to and development of meningococcal septicaemia with lower blood pressure and need for adrenergic support (62).
The RESOLVE study (REsearching severe Sepsis and Organ dysfunction in children: a global perspectiVE) was a randomised placebo-controlled trial, to investigate the efficacy and safety of rhAPC in children. Children aged between 38 weeks corrected gestational age to 17 years with suspected or proven infection and systemic inflammation, sepsis-induced cardiovascular and respiratory organ dysfunction within 12 h before entering the study were included. Exclusion criteria included a high risk of intracranial bleeding, end stage liver or renal disease, platelet count <30,000 or those expected to die before completion of the study. This study enrolled 477 children from 102 study sites in 18 countries who received either placebo or rhAPC (24 microgram/kg/h for 96 h). The primary endpoint for efficacy was the Composite Time to Complete Organ Failure Resolution (CTCOFR) score of three organ systems: cardiovascular, respiratory, and renal. There were no significant differences in either CTCOFR score (p = 0.72) or 28 day-mortality (p = 0.93) between patients on rhAPC compared with placebo. There were no significant differences between groups in the incidence of serious bleeding. Four out of five patients in the rhAPC group with a central nervous system bleeding event during infusion were <60 days of age and weighed <4 kg (46, 59).
The apparently conflicting results between the children in RESOLVE and adults in PROWESS with sepsis may be attributed to the baseline characteristics, trial design and statistical power or to the differences in the paediatric and adult pathophysiology of sepsis (46). In the RESOLVE study, patients with the most severe coagulation abnormalities appeared to benefit most from rhAPC and may be an important subgroup for further RCTs. This study was the largest paediatric sepsis study undertaken when published but heterogeneity of patient population and sepsis management between centres may have altered the results and larger PICU networks are required. Enrolment of few patients in many centres might have resulted in a “first patient effect,” endpoints such as cardiovascular and respiratory failure could be affected by local weaning practice. Different management in intensive care units within and between different countries might also affect endpoints reliability and numerical imbalances in some baseline characteristics because of comparatively small size. The rhAPC group had more severe disease such as more organ dysfunction, acute respiratory distress syndrome, DIC and higher paediatric risk mortality III (PRISM II). The RESOLVE study was stopped early after the second interim analysis as there was no significant difference in the primary efficacy or safety endpoints (46).
In a retrospective, multicentre study of 94 paediatric patients treated with protein C concentrate for purpura fulminans, 79.8% showed improvement, 13.8% were unchanged and 6.4% deteriorated. Skin grafts were required in 9.6% and amputation in 5.3%. The non-survivor group had lower protein C levels (p < 0.05), increased coagulopathy (p = 0.01) and longer interval to the start of protein C concentrate (p = 0.03) (63).
Protein C concentrate, used as an adjunctive therapy, improved coagulopathy in a prospective open-label study of eight children with severe meningococcal disease (47). In a study of Protein C concentrate administration, with daily dose adjustment to maintain plasma protein C activity range of 0.8-1.2 IU/ml, to 12 patients (3 months to 27 years old) with meningococcal septic shock, DIC, widespread purpura and skin necrosis and acquired low protein C levels, there were no deaths although the expected mortality from the Glasgow meningococcal septicaemia prognostic score and the paediatric risk of mortality score predicted a minimum mortality of 80 and 57%, respectively (50). White et al. also found a significant reduction in morbidity and mortality in patients (n = 36; age 0.3-72 years) with severe meningococcaemia treated with protein C (51).
A reduction in limb amputation rate, compared to previous reports (16-23 vs. 50%), was observed in a study of non-activated plasma derived protein C in 30 paediatric patients (median age 2 years) with sepsis, (49).
Protein C and Neonatal Sepsis
Sepsis is one of the major causes of death in neonates with an incidence of 1-10 per 1,000 live births and a mortality rate of 15-50%. Protein C is decreased in healthy newborns and further diminished with delivery by uncomplicated elective caesarean section (64). Neonatal sepsis and shock are also associated with a significant reduction in plasma protein C and S levels (65). Protein C concentrate replacement therapy improved coagulopathy in 18 preterm and term neonates with severe sepsis or septic shock, coagulopathy, and acquired protein C deficiency (52).
In their Cochrane review of protein C use in neonates with severe sepsis, Kylat et al. found insufficient data to support the use of rhAPC. A cautious approach was suggested for the use of rhAPC due to the high incidence of bleeding with its use, as severe sepsis in preterm infants is commonly associated with bleeding problems and intraventricular haemorrhage. The use of rhAPC was not recommended outside the confines of randomised controlled trials (66). Infants with neonatal encephalopathy (NE) have an altered inflammatory response in terms of neutrophil reactive oxygen species (ROS) and Toll-like receptor (TLR)-4 expression. This response was reduced following treatment with APC, demonstrating the potential use of APC as an adjuvant therapy in NE (67).
Protein C as Biomarker in Sepsis
Protein C level may be a good biomarker in sepsis. In the RESPOND study, protein C levels were used to modify therapy with increased dose and duration of rhAPC in severe deficiency (54). In a multicentre study of adult patients with severe sepsis, at baseline and 44 h, lower protein C levels were associated with increased 30 day-mortality (p = 0.19 and p = 0.04 respectively), increased incidence of shock (p = 0.01; p < 0.001) and a reduction in the number of ventilator-free days (p = 0.02; p = 0.03) (53).
In 187 patients (term newborn to <18 years old) in the ENHANCE (drotrecogin alfa (activated) in children with severe sepsis) study, there was a significant association between increased 28-day mortality and decreased protein C level following rhAPC administration (p < 0.001). The change in protein C level from baseline was predictive of survival (68). In a paediatric study of protein C concentrate in purpura fulminans, non survivors had lower protein C levels (p < 0.05), increased coagulopathy (p = 0.01) and an increased interval between admission and commencing protein C concentrate (p = 0.03) (47). In 73 patients (1 month-15 years) with septic shock, there was significantly lower protein C activity in non-survivors (p = 0.002) (61). Protein C levels did not differ between paediatric febrile neutropenia with and without bacteraemia in 73 paediatric oncology patients in a prospective cohort study (69).
In 40 neonates (1-28 days of age) with low birth weight, severe sepsis and organ dysfunction, plasma protein C activity levels were lower in non-survivors vs. survivors (p < 0.001). The positive predictive value of the protein C level for mortality was 90.9%. Each 1% rise in protein C activity levels decreased the hazard of dying by 5% Infants with congenital anomalies, birth asphyxia or blood products received before protein C assay were excluded (70).
In a prospective observational study of protein C and antithrombin III levels in neonates with late onset sepsis, mean birth weight 1.86 (range 0.7-4.2) kg, gestational age 32.7 (range 25-41) weeks, age 14.5 (8–172) days, there were statistical differences in AT III (p = 0.003) and protein C activity levels (p = 0.002) between survivors and non-survivors. Low protein C activity levels also significantly correlated with the risk of death in neonates with sepsis (p = 0.0016) (71). El Beshlawy et al. studied the effect of sepsis on protein C, S and ATIII levels in term neonates (30 septic and 30 normal controls). Blood samples were taken 72-96 h after birth and the physiological inhibition system including protein C, S and ATIII was markedly decreased in all septic neonates, compared to controls (p < 0.001). DIC developed and death occurred in 33.3% of cases; necrotizing enterocolitis in 40% of cases; rectal bleeding in 33.3%; haematuria in 20% of cases; haematemesis in 26.7% of cases; intracranial haemorrhage in 23.3% of cases, and convulsions in 23.3% (Table 2) (53).
Activated Protein C: Side Effects
Treatment with rhAPC has been associated with an increased risk of bleeding. The PROWESS study reported serious bleeding events in 3.5% of rhAPC treated patients, compared with 2% of those on placebo (p = 0.06) (31). Patients with activated internal bleeding, haemorrhagic stroke within 3 months, intracranial/intraspinal surgery or severe head trauma within 2 months, trauma with increased risk of life-threatening bleeding, presence of an epidural catheter and intracranial neoplasm or mass lesion or evidence of cerebral herniation were excluded (31). In the ADDRESS study, the rate of serious bleeding was greater in the rhAPC group than placebo group both during infusion (2.4 vs. 1.2%, p = 0.02) and the 28-day study period (3.9 vs. 2.2%, p = 0.01). Four (0.3%) patients had CNS bleeding during rhAPC infusion vs. 3 (0.2%) in the placebo group (45). In the ENHANCE study, the risk of bleeding related to administration of rhAPC was 3.2% (68). Fumagalli et al. demonstrated that adverse events associated with rhAPC occur with a frequency of 5% or greater including ecchymosis (23.0%), headache (30.9%), and pain (5.8%) primarily at the site of venepuncture. rhAPC antibody formation was uncommon (<1%) after a single course of therapy (72). In the EVAA dose finding study, rhAPC was safe and well tolerated by adult patients with sepsis (27).
A mutant APC generated with three alanine mutations (3K3A-APC) has been created with potent neuroprotective properties and reduced anticoagulant activity (73). 3K3A-APC crosses the intact blood-brain barrier through EPCR-mediated transport and may have direct action on neurons contributing to the observed neuroprotection in vivo. The creation of mutant APC with less anticoagulant activity may increase the utility of APC in sepsis especially in populations vulnerable to haemorrhage.
There are also many alternative compounds to rhAPC that are currently in Phase III clinical trials which include TFPI, TNF-antibody fragment, antithrombin III and platelet-activating factor acetylhydrolase (74), although TFI and antithrombin have been ineffective against severe sepsis and septic shock (75, 76).
Other Inflammatory Conditions
Acute Lung Injury
A recent double-blind placebo-controlled study in a human model of endotoxin-induced pulmonary inflammation, inhalation of activated protein C significantly reduced lung inflammation and leukocyte infiltration (30). In awake sheep, rhAPC alleviates endotoxin-induced lung injury as characterised by improved oxygenation and reversal of pulmonary hemodynamic and volumetric changes, as well as by reversal of pulmonary hemodynamic and volumetric changes (77). Inhaled protein C may attenuate acute pulmonary inflammation and inhaled APC improved oxygenation and lung aeration in a sheep model of endotoxin-induced acute lung injury but did not alter haemodynamics. Further research is required on the effectiveness of the inhaled route (78).
Coronavirus disease 2019 (COVID-19) has quickly spread across the world and caused a significant burden on healthcare. Patients hospitalised with COVID-19 may present with sepsis, pneumonia and acute respiratory disease syndrome (ARDS) (79). It has been established that COVID-19 patients with pneumonia have defects in their thrombin coagulation system, causing microthrombosis and multi-organ failure (79, 80). PAR-1 antagonists have been shown to be effective in a mouse model of pneumonia, and Jose and Manuel hypothesised that targeting it, may be effective in the management of thromboembolism (81, 82). Therefore, the use of therapies targeting key components of the thrombin coagulation system have the potential to control microthrombosis in the fight against COVID-19 (82).
Brain Injury
Activated protein C (APC) protects neurons and endothelium from ischemic injury. Early administration of wild-type APC to mice with traumatic brain injury results in decreased lesion volume and improved functional outcome (83), while late administration of an APC variant with reduced anticoagulant function has been shown to be more neuroprotective than wild type APC, and has shown to significantly improve functional recovery and with no increased risk of bleeding (84). Activated drotrecogin-alfa, a form of human recombinant APC, is currently being studied in patients with ischemic stroke. Non-anticoagulant 3K3A-APC exhibits greater neuroprotective efficacy with no risk for bleeding compared with drotrecogin-alfa activated (73). APC attenuates the lipopolysaccharide (LPS)-induced protein expression of inflammatory cytokines, TNF-α and IL-6in neonatal rat brains (85). A single intraperitoneal injection of rhAPC to neonatal rats had similar protective and anti-inflammatory effects against maternally administered LPS. APC may therefore provide protection against an endotoxin-evoked inflammatory response and white matter injury in the developing rat brain.
Independent of its anticoagulant activity, APC acts directly on cells and can alter gene expression profiles, inhibit apoptosis, and down-regulate inflammation mediating neuroprotection. These effects require protease-activated receptor-1 and the endothelial protein C receptor. In an in vitro model involving hypoxia-induced apoptosis of human brain endothelial cells, protease-activated receptor-1 and endothelial protein C receptor is required for APC to exert its anti-apoptotic effects. In these cells, APC attenuates the hypoxia-induced increases in p53 messenger RNA and protein, reduces pro-apoptotic Bax, and increases anti-apoptotic Bcl-2, and therefore inhibits mitochondrial-dependent apoptosis. Murine ischemic stroke model studies have provided in vivo evidence for the physiologic roles of protease-activated receptor-1 and endothelial protein C receptor in the neuroprotective activities of APC (86). The protein C pathway is also thought to be critically involved in chronic inflammatory disorders such as inflammatory bowel disease and renal inflammation (87, 88).
Other Disease Models
APC administration has been shown to be beneficial in neurological conditions besides stroke, slowing disease progression and prolonging survival in a mouse model of amyotrophic lateral sclerosis (ALS) (89) and demonstrating therapeutic efficacy in a mouse multiple sclerosis model (90). APC also attenuates glomerular and endothelial injury in diabetic mice (91) and has been shown to be beneficial in a mouse model of inflammatory bowel disease (92).
Future Directions for Activated Protein C
The “holy grail” would be to develop a recombinant human APC which has improved ability to mediate protective signalling, but which has minimal potential to cause bleeding. “Second generation” recombinant APC variants have been designed, which have similar signalling properties to wild type APC but reduced anticoagulant properties (93–95). Mosnier et al. (93) generated a recombinant APC variant with impaired FVa substrate recogition due to mutation of its FVa-binding exosite. Harmon et al. have described an APC mutant with attenuated protein S cofactor function (94). Another group engineered an APC variant in which a disulfide bond was introduced which virtually ablated its anticoagulant function (95). However, until recently no means of enhancing APC protective signalling has been reported.
Ní Ainle et al. characterised novel recombinant APC variants with greatly enhanced cytoprotective signalling function but limited anticoagulant activity (96). One of the recombinant variants described in this study, APC-L38D/N329Q, represents the first example of an APC molecule with enhanced cytoprotective function but attenuated anticoagulant function. These novel recombinant molecules are predicted to have enhanced therapeutic potential compared to existing recombinant APC preparations when used in the treatment of systemic inflammatory conditions. A recombinant human form of endogenous activated protein C (drotrecogin alfa) was recently approved by the Food and Drug Administration for adults with severe sepsis who have a high risk of death. It possesses anticoagulant, profibrinolytic, and anti-inflammatory properties (74).
In the setting of cancer, staging systems are used to stratify patients based on risk and potential benefit from treatments (97). This approach will be key for the management of sepsis as patients are highly heterogenous in terms of the cause of sepsis, disease severity and associated co-morbidities (97). Therefore, a lack of recognition of septic patient heterogeneity in clinical trials may mask therapeutic effects of a therapy. For instance, the PROWESS trial showed reduced mortality in severe sepsis patients and the clinical trials preceding this did not, but these patients presented with a low risk of mortality to begin with. APC causes a reduction in reactive oxygen species (ROS) production after induction with LPS in adults but no difference in paediatric intensive care unit (PICU) patients. These differences in immune response to APC could explain conflicting results between the RESOLVE and PROWESS trials (98). Future studies must shift their focus to developing validated patient stratification methods if there is any hope for management of sepsis (97). This must take into account the above-mentioned factors such as the severity of disease, patient age, the initial source of infection, varying degrees of SOFA score, APC levels at baseline and the timing of treatment. The heterogeneity of sepsis is also influenced within trial centres and their sepsis criteria or definitions (99), all of these factors combined will provide a more precise clinical understanding of sepsis and outcomes. The definitions and clinical criteria made by the Sepsis-3 group provides a guideline to the progress of this (100). Similarly, these challenges are described by McGovern et al., within a neonatal population (101).
Conclusion
Protein C levels have been used as biomarker in paediatric and adult sepsis and low protein C levels predict the risk of morbidity and mortality. Replacement therapy with rhAPC in adult sepsis reduced mortality in early studies but these results could not be replicated in more recent trials. In addition, the RESOLVE study in paediatric sepsis did not show a similar improvement in morbidity and mortality. Although activated protein C has promise for sepsis management, the development of newer preparations with decreased coagulability while retaining anti-inflammatory properties are required. Immune profiling of neonates and children treated in randomised controlled trials of rhAPC will also be important to evaluate the individualised immune responses at each stage of sepsis from proinflammatory to the compensatory anti-inflammatory response to allow treatment optimisation with medications such as rhAPC. In addition, studies with long-term outcomes beyond 28 days are vital to evaluate the effect on neurodevelopmental outcome. Therefore, large clinical trials are required to elucidate the effectiveness of rhAPC in reducing mortality and adverse outcomes in children with severe sepsis.
Author Contributions
HE conducted the research and wrote the manuscript. EJM supervised the work and co-wrote the manuscript. MO, EM, BN, and IR assisted in writing and revision of the manuscript. All authors contributed to the article and approved the submitted version.
Funding
This work was supported by National Children's Research Centre, Crumlin, Dublin, Ireland.
Conflict of Interest
The authors declare that the research was conducted in the absence of any commercial or financial relationships that could be construed as a potential conflict of interest.
Publisher's Note
All claims expressed in this article are solely those of the authors and do not necessarily represent those of their affiliated organizations, or those of the publisher, the editors and the reviewers. Any product that may be evaluated in this article, or claim that may be made by its manufacturer, is not guaranteed or endorsed by the publisher.
References
1. Stenflo J. A new vitamin K-dependent protein. Purification from bovine plasma and preliminary characterization. J Biol Chem. (1976) 251:355–63 doi: 10.1016/S0021-9258(17)33886-3
2. Rezaie AR. Regulation of the protein C anticoagulant and antiinflammatory pathways. Curr Med Chem. (2010) 17:2059–69. doi: 10.2174/092986710791233706
3. Bertina RM. Protein C deficiency and venous thrombosis–the search for the second genetic defect. Thromb Haemost. (2000) 83:360–1. doi: 10.1055/s-0037-1613820
4. Esmon CT. Thrombomodulin as a model of molecular mechanisms that modulate protease specificity and function at the vessel surface. FASEB J. (1995) 9:946–55. doi: 10.1096/fasebj.9.10.7615164
5. Stearns-Kurosawa DJ, Kurosawa S, Mollica JS, Ferrell GL, Esmon CT. The endothelial cell protein C receptor augments protein C activation by the thrombin-thrombomodulin complex. Proceed Natl Acad Sci USA. (1996) 93:10212–6. doi: 10.1073/pnas.93.19.10212
6. Taylor FB Jr, Peer GT, Lockhart MS, Ferrell GL, Esmon CT. Endothelial cell protein C receptor plays an important role in protein C activation in vivo. Blood. (2001) 97:1685–8. doi: 10.1182/blood.V97.6.1685
7. Walker FJ, Sexton PW, Esmon CT. The inhibition of blood coagulation by activated Protein C through the selective inactivation of activated Factor V. Biochim Biophys Acta. (1979) 571:333–42. doi: 10.1016/0005-2744(79)90103-7
8. Fay PJ, Smudzin TM, Walker FJ. Activated protein C-catalyzed inactivation of human factor VIII and factor VIIIa. Identification of cleavage sites and correlation of proteolysis with cofactor activity. J Biol Chem. (1991) 266:20139–145. doi: 10.1016/S0021-9258(18)54901-2
9. Dahlbäck B, Villoutreix BO. Regulation of blood coagulation by the protein C anticoagulant pathway: novel insights into structure-function relationships and molecular recognition. Arterioscler Thromb Vasc Biol. (2005) 25:1311–20. doi: 10.1161/01.ATV.0000168421.13467.82
10. Ahnstrom HMJ, Andersson K, Canis E, Norstrom Y, Yu B, Dahlback M, et al. Activated protein C cofactor function of protein S: a novel role for a gamma-carboxyglutamic acid residue. Blood. (2011) 117:6685–93. doi: 10.1182/blood-2010-11-317099
11. Váradi K, Rosing J, Tans G, Pabinger I, Keil B, Schwarz HP. Factor V enhances the cofactor function of protein S in the APC-mediated inactivation of factor VIII: influence of the factor VR506Q mutation. Thromb Haemost. (1996) 76:208–14. doi: 10.1055/s-0038-1650556
12. Feistritzer C, Riewald M. Endothelial barrier protection by activated protein C through PAR1-dependent sphingosine 1-phosphate receptor-1 crossactivation. Blood. (2005) 105:3178–84. doi: 10.1182/blood-2004-10-3985
13. White BM, Schmidt C, Murphy W, Livingstone D, O'Toole M, Lawler L, et al. Activated protein C inhibits lipopolysaccharide-induced nuclear translocation of nuclear factor kappaB (NF-kappaB) and tumour necrosis factor alpha (TNF-alpha) production in the THP-1 monocytic cell line. Br J Haematol. (2000) 110:130–4. doi: 10.1046/j.1365-2141.2000.02128.x
14. Cao C, Gao Y, Li Y, Antalis TM, Castellino FJ, Zhang L. The efficacy of activated protein C in murine endotoxemia is dependent on integrin CD11b. J Clin Invest. (2010) 120:1971–80. doi: 10.1172/JCI40380
15. Yang XV, Banerjee Y, Fernández JA, Deguchi H, Xu X, Mosnier RT, et al. Activated protein C ligation of ApoER2 (LRP8) causes Dab1-dependent signaling in U937 cells. Proc Natl Acad Sci USA. (2009) 106:274–9. doi: 10.1073/pnas.0807594106
16. Joyce DE, Grinnell BW. Recombinant human activated protein C attenuates the inflammatory response in endothelium and monocytes by modulating nuclear factor-kappaB. Crit Care Med. (2002) 30(5 Suppl):S288–93. doi: 10.1097/00003246-200205001-00019
17. Cheng T, Dong L, Griffin JH, Fernández JA, Castellino F, Elliot D, et al. Activated protein C blocks p53-mediated apoptosis in ischemic human brain endothelium and is neuroprotective. Nat Med. (2003) 9:338–42. doi: 10.1038/nm826
18. Riewald RJ, Petrovan M, Donner A, Mueller BM, Ruf W. Activation of endothelial cell protease activated receptor 1 by the protein C pathway. Science. (2002) 296:1880–2. doi: 10.1126/science.1071699
19. Mosnier LO, Griffin JH. Inhibition of staurosporine-induced apoptosis of endothelial cells by activated protein C requires protease-activated receptor-1 and endothelial cell protein C receptor. Biochem J. (2003) 373:65–70. doi: 10.1042/bj20030341
20. Burnier L, Mosnier LO. Novel mechanisms for activated protein C cytoprotective activities involving noncanonical activation of protease-activated receptor 3. Blood. (2013) 122:807–16. doi: 10.1182/blood-2013-03-488957
21. Samlaska CP, James WD. Superficial thrombophlebitis. I. Primary hypercoagulable states. J Am Acad Dermatol. (1990) 22:975–89. doi: 10.1016/0190-9622(90)70139-9
22. Bucciarelli FR, Rosendaal P, Tripodi A, Mannucci PM, De Stefano VG, et al. Risk of venous thromboembolism and clinical manifestations in carriers of antithrombin, protein C, protein S deficiency, or activated protein C resistance: a multicenter collaborative family study. Arterioscler Thromb Vasc Biol. (1999) 19:1026–33. doi: 10.1161/01.ATV.19.4.1026
23. Estellés AI, Garcia-Plaza A, Dasí J, Aznar M, Duart G, Sanz JL, et al. Severe inherited homozygous protein C deficiency in a newborn infant. Thromb Haemost. (1984) 52:53–6. doi: 10.1055/s-0038-1661136
24. Seligsohn U, Berger A, Abend M, Rubin L, Attias D, Zivelin A, et al. (1984) Homozygous protein C deficiency manifested by massive venous thrombosis in the newborn. N Engl J Med. (1984) 310:559–62. doi: 10.1056/NEJM198403013100904
25. Goldenberg NA, Manco-Johnson MJ. Protein C deficiency. Haemophilia. (2008) 14:1214–21. doi: 10.1111/j.1365-2516.2008.01838.x
26. Bertina RM, Koeleman BP, Koster T, Rosendaal FR, Dirven RJ, de Ronde H, et al. Mutation in blood coagulation factor V associated with resistance to activated protein C. Nature. (1994) 369:64–7. doi: 10.1038/369064a0
27. Bernard GR, Ely EW, Wright TJ, Fraiz J, Stasek JE Jr, Russell JA, et al. Safety and dose relationship of recombinant human activated protein C for coagulopathy in severe sepsis. Crit Care Med. (2001) 29:2051–9. doi: 10.1097/00003246-200111000-00003
28. Taylor FB Jr, Chang A, Esmon CT, D'Angelo A, Vigano-D'Angelo S, Blick KE. Protein C prevents the coagulopathic and lethal effects of Escherichia coli infusion in the baboon. J Clin Invest. (1987) 79:918–25. doi: 10.1172/JCI112902
29. Castellino FJ, Ploplis VA. The protein C pathway and pathologic processes. J Thromb Haemost. (2009) 7(Suppl 1):140–5. doi: 10.1111/j.1538-7836.2009.03410.x
30. Nick JA, Coldren CD, Geraci MW, Poch KR, Fouty BW, O'Brien J, et al. Recombinant human activated protein C reduces human endotoxin-induced pulmonary inflammation via inhibition of neutrophil chemotaxis. Blood. (2004) 104:3878–85. doi: 10.1182/blood-2004-06-2140
31. Bernard GR, Vincent JL, Laterre PF, LaRosa SP, Dhainaut JF, Lopez-Rodriguez A, et al. Efficacy and safety of recombinant human activated protein C for severe sepsis. N Engl J Med. (2001) 344:699–709. doi: 10.1056/NEJM200103083441001
32. Shahzad K, Kohli S, Al-Dabet MM, Isermann B. Cell biology of activated protein C. Curr Opin Hematol. (2019) 26:41–50. doi: 10.1097/MOH.0000000000000473
33. Nazir S, Ihsan G, Mohanad Al-Dabet M, Elwakiel A, Kohli S, Ghosh S, et al. Cytoprotective activated protein C averts Nlrp3 inflammasome-induced ischemia-reperfusion injury via mTORC1 inhibition. Blood. (2017) 130:2664–77. doi: 10.1182/blood-2017-05-782102
34. Lauterbach R, Wilk B, Bocheńska A, Hurkała J, Radziszewska R. Nonactivated protein C in the treatment of neonatal sepsis: a retrospective analysis of outcome. Pediatr Infect Dis J. (2016) 35:967–71. doi: 10.1097/INF.0000000000001247
35. Esmon CT. Crosstalk between inflammation and thrombosis. Maturitas. (2004) 47:305–14. doi: 10.1016/j.maturitas.2003.10.015
36. Xu J, Esmon NL, Esmon CT. Reconstitution of the human endothelial cell protein C receptor with thrombomodulin in phosphatidylcholine vesicles enhances protein C activation. J Biol Chem. (1999) 274:6704–10. doi: 10.1074/jbc.274.10.6704
37. Esmon CT. The interactions between inflammation and coagulation. Br J Haematol. (2005) 131:417–30. doi: 10.1111/j.1365-2141.2005.05753.x
38. Joyce DE, Gelbert L, Ciaccia A, DeHoff B, Grinnell BW. Gene expression profile of antithrombotic protein c defines new mechanisms modulating inflammation and apoptosis. J Biol Chem. (2001) 276:11199–203. doi: 10.1074/jbc.C100017200
39. Hirose K, Okajima K, Taoka Y, Uchiba M, Tagami H, Nakano K, et al. Activated protein C reduces the ischemia/reperfusion-induced spinal cord injury in rats by inhibiting neutrophil activation. Ann Surg. (2000) 232:272–80. doi: 10.1097/00000658-200008000-00018
40. Husari AW, Khayat A, Awdeh H, Hatoum H, Nasser M, Mroueh SM, et al. Activated protein C attenuates acute lung injury and apoptosis in a hyperoxic animal model. Shock. (2010) 33:467–72. doi: 10.1097/SHK.0b013e3181c69213
41. Park SW, Chen SW, Kim M, D'Agati VD, Lee HT. Human activated protein C attenuates both hepatic and renal injury caused by hepatic ischemia and reperfusion injury in mice. Kidney Int. (2009) 76:739–50. doi: 10.1038/ki.2009.255
42. Faust SN, Heyderman RS, Levin M. Coagulation in severe sepsis: a central role for thrombomodulin and activated protein C. Crit Care Med. (2001) 29 (7 Suppl):S62-7; discussion S67-8. doi: 10.1097/00003246-200107001-00022
43. El Beshlawy A, Alaraby I, Abou Hussein H, Abou-Elew HH, Mohamed Abdel Kader MS. Study of protein C. protein S, and antithrombin III in newborns with sepsis. Pediatr Crit Care Med. (2010) 11:52–9. doi: 10.1097/PCC.0b013e3181c59032
44. Martí-Carvajal AJ, Solà I, Gluud C, Lathyris D, Cardona AF. Human recombinant protein C for severe sepsis and septic shock in adult and paediatric patients. Cochr Database Syst Rev. (2012) 12:Cd004388. doi: 10.1002/14651858.CD004388.pub6
45. Abraham PF, Laterre E, Garg R, Levy H, Talwar D, Trzaskoma BL, et al. Drotrecogin alfa (activated) for adults with severe sepsis and a low risk of death. N Engl J Med. (2005) 353:1332–41. doi: 10.1056/NEJMoa050935
46. Nadel S, Goldstein B, Williams MD, Dalton H, Peters M, Macias WL, et al. Drotrecogin alfa (activated) in children with severe sepsis: a multicentre phase III randomised controlled trial. Lancet. (2007) 369:836–43. doi: 10.1016/S0140-6736(07)60411-5
47. Ettingshausen CE, Veldmann A, Beeg T, Schneider W, Jäger G, Kreuz W. Replacement therapy with protein C concentrate in infants and adolescents with meningococcal sepsis and purpura fulminans. Semin Thromb Hemost. (1999) 25:537–41. doi: 10.1055/s-2007-994962
48. Rintala OP, Seppälä E, Kotilainen P, Pettilä V, Rasi V. Protein C in the treatment of coagulopathy in meningococcal disease. Crit Care Med. (1998) 26:965–8. doi: 10.1097/00003246-199805000-00038
49. Piccin A, O' Marcaigh A, Mc Mahon C, Murphy C, Okafor I, Marcheselli L, et al. Non-activated plasma-derived PC improves amputation rate of children undergoing sepsis. Thromb Res. (2014) 134:63–7. doi: 10.1016/j.thromres.2014.04.019
50. Smith OP, White B, Vaughan D, Rafferty M, Claffey L, Lyons B, et al. Use of protein-C concentrate, heparin, and haemodiafiltration in meningococcus-induced purpura fulminans. Lancet. (1997) 350:1590–3. doi: 10.1016/S0140-6736(97)06356-3
51. White B, Livingstone W, Murphy C, Hodgson A, Rafferty M, Smith OP. An open-label study of the role of adjuvant hemostatic support with protein C replacement therapy in purpura fulminans-associated meningococcemia. Blood. (2000) 96:3719–24. doi: 10.1182/blood.V96.12.3719.h8003719_3719_3724
52. Decembrino L, D'Angelo A, Manzato F, Solinas A, Tumminelli F, De Silvestri A, et al. Protein C concentrate as adjuvant treatment in neonates with sepsis-induced coagulopathy: a pilot study. Shock. (2010) 34:341–5. doi: 10.1097/SHK.0b013e3181e7623e
53. Yan SB, Helterbrand JD, Hartman DL, Wright TJ, Bernard GR. Low levels of protein C are associated with poor outcome in severe sepsis. Chest. (2001) 120:915–22. doi: 10.1378/chest.120.3.915
54. Vangerow B, Shorr AF, Wyncoll D, Janes J, Nelson DR, Reinhart K. The protein C pathway: implications for the design of the RESPOND study. Crit Care. (2007) 11(Suppl 5):S4. doi: 10.1186/cc6155
55. Levi M, Levy M, Williams MD, Douglas I, Artigas A, Antonelli M, et al. Prophylactic heparin in patients with severe sepsis treated with drotrecogin alfa (activated). Am J Respir Crit Care Med. (2007) 176:483–90. doi: 10.1164/rccm.200612-1803OC
56. Silva E, de Figueiredo LF, Colombari F. Prowess-shock trial: a protocol overview and perspectives. Shock. (2010) 34(Suppl 1):48–53. doi: 10.1097/SHK.0b013e3181e7e97b
57. Dhainaut JF, Antonelli M, Wright P, Desachy A, Reignier J, Lavoue S, et al. Extended drotrecogin alfa (activated) treatment in patients with prolonged septic shock. Intensive Care Med. (2009) 35:1187–95. doi: 10.1007/s00134-009-1436-1
58. Watson RS, Carcillo JA, Linde-Zwirble WT, Clermont G, Lidicker J, Angus DC. The epidemiology of severe sepsis in children in the United States. Am J Respir Crit Care Med. (2003) 167:695–701. doi: 10.1164/rccm.200207-682OC
60. Giordano P, Cecinati V, Delvecchio GC, Arcamone G, De Leonardis F, Grassi M, et al. Successful treatment with Protein C of febrile neutropenia in pediatric patients during cancer therapy. Immunopharm Immunotoxic. (2009) 31:293–8. doi: 10.1080/08923970802552415
61. Samransamruajkit R, Hiranrat T, Prapphal N, Sritippayawan S, Deerojanawong J, Poovorawan Y. Levels of protein C activity and clinical factors in early phase of pediatric septic shock may be associated with the risk of death. Shock. (2007) 28:518–23. doi: 10.1097/shk.0b013e318054de02
62. Binder A, Endler G, Rieger S, Geishofer G, Resch B, Mannhalter C, et al. Protein C promoter polymorphisms associate with sepsis in children with systemic meningococcemia. Hum Genet. (2007) 122:183–90. doi: 10.1007/s00439-007-0392-5
63. Veldman A, Fischer D, Wong FY, Kreuz W, Sasse M, Eberspächer B, et al. Human protein C concentrate in the treatment of purpura fulminans: a retrospective analysis of safety and outcome in 94 pediatric patients. Crit Care. (2010) 14:R156. doi: 10.1186/cc9226
64. Franzoi M, Simioni P, Luni S, Zerbinati P, Girolami A, Zanardo V. Effect of delivery modalities on the physiologic inhibition system of coagulation of the neonate. Thromb Res. (2002) 105:15–8. doi: 10.1016/S0049-3848(01)00407-8
65. Román J, Velasco F, Fernandez F, Fernandez M, Villalba R, Rubio V, et al. Protein C. protein S and C4b-binding protein in neonatal severe infection and septic shock. J Perinat Med. (1992) 20:111–6. doi: 10.1515/jpme.1992.20.2.111
66. Kylat RI, Ohlsson A. Recombinant human activated protein C for severe sepsis in neonates. Cochr Database Syst Rev. (2006) Cd005385. doi: 10.1002/14651858.CD005385.pub2
67. Eliwan HO, Watson RW, Aslam S, Regan I, Philbin B, O'Hare FM, et al. Neonatal brain injury and systemic inflammation: modulation by activated protein C ex vivo. Clin Exp Immunol. (2015) 179:477–84. doi: 10.1111/cei.12453
68. Goldstein B, Nadel S, Peters M, Barton R, Machado F, Levy H, et al. ENHANCE: results of a global open-label trial of drotrecogin alfa (activated) in children with severe sepsis. Pediatr Crit Care Med. (2006) 7:200–11. doi: 10.1097/01.PCC.0000217470.68764.36
69. Kassam AK, Chan A, Dzolganovski B, Constantin J, Ramphal R, et al. No association between protein C levels and bacteremia in children with febrile neutropenia. J Pediatr Hematol Oncol. (2009) 31:647–50. doi: 10.1097/MPH.0b013e3181b1ec89
70. Venkataseshan S, Dutta S, Ahluwalia J, Narang A. Low plasma protein C values predict mortality in low birth weight neonates with septicemia. Pediatr Infect Dis J. (2007) 26:684–8. doi: 10.1097/INF.0b013e3180f616f0
71. Lauterbach R, Pawlik D, Radziszewska R, Wozniak J, Rytlewski K. Plasma antithrombin III and protein C levels in early recognition of late-onset sepsis in newborns. Eur J Pediatr. (2006) 165:585–9. doi: 10.1007/s00431-006-0139-7
72. Fumagalli R, Mignini MA. The safety profile of drotrecogin alfa (activated). Crit Care. (2007) 11:S6. doi: 10.1186/cc6157
73. Wang Y, Thiyagarajan M, Chow N, Singh I, Guo H, Davis TP, et al. Differential neuroprotection and risk for bleeding from activated protein C with varying degrees of anticoagulant activity. Stroke. (2009) 40:1864–9. doi: 10.1161/STROKEAHA.108.536680
74. Healy DP. New and emerging therapies for sepsis. Ann Pharmacother. (2002) 36:648–54. doi: 10.1345/aph.1A283
75. Sne N. Ondiveeran HK, Fox-Robichaud A. Tifacogin (Chiron Corp/Pharmacia Corp). IDrugs. (2002) 5:91–7.
76. Warren BL, Eid A, Singer P, Pillay SS, Carl P, Novak I, et al. Caring for the critically ill patient. High-dose antithrombin III in severe sepsis: a randomized controlled trial. JAMA. (2001) 286:1869–78. doi: 10.1001/jama.286.15.1869
77. Waerhaug VN, Kuklin K, Kirov MY, Sovershaev MA, Langbakk B, Ingebretsen OC, et al. Recombinant human activated protein C attenuates endotoxin-induced lung injury in awake sheep. Crit Care. (2008) 12:R104. doi: 10.1186/cc6985
78. Liu K, Mark D, Looney R, Matthay MA. Inhaled activated protein C: a novel therapy for acute lung injury? Crit Care. (2009) 13:150. doi: 10.1186/cc7869
79. Zhou F, Yu T, Du R, Fan G, Liu Y, Liu Z, et al. Clinical course and risk factors for mortality of adult inpatients with COVID-19 in Wuhan, China: a retrospective cohort study. Lancet. (2020) 395:1054–62. doi: 10.1016/S0140-6736(20)30566-3
80. Tang N, Li D, Wang X, Sun Z. Abnormal coagulation parameters are associated with poor prognosis in patients with novel coronavirus pneumonia. J Thromb Haemost. (2020) 18:844–7. doi: 10.1111/jth.14768
81. José R, Williams A, Sulikowski M, Brealey D, Brown J, Chambers R. Regulation of neutrophilic inflammation in lung injury induced by community-acquired pneumonia. Lancet. (2015) 385(Suppl 1):S52. doi: 10.1016/S0140-6736(15)60367-1
82. Jose RJ, Manuel A. COVID-19 cytokine storm: the interplay between inflammation and coagulation. Lancet Respir Med. (2020) 8:e46-7. doi: 10.1016/S2213-2600(20)30216-2
83. Petraglia AL, Marky AH, Walker C, Thiyagarajan M, Zlokovic BV. Activated protein C is neuroprotective and mediates new blood vessel formation and neurogenesis after controlled cortical impact. Neurosurgery. (2010) 66:165-71; discussion 171-2. doi: 10.1227/01.NEU.0000363148.49779.68
84. Walker CT, Marky AH, Petraglia AL, Ali T, Chow N, Zlokovic BV. Activated protein C analog with reduced anticoagulant activity improves functional recovery and reduces bleeding risk following controlled cortical impact. Brain Res. (2010) 1347:125–31. doi: 10.1016/j.brainres.2010.05.075
85. Yesilirmak DC, Kumral A, Baskin H, Ergur BU, Aykan S, Genc S, et al. Activated protein C reduces endotoxin-induced white matter injury in the developing rat brain. Brain Res. (2007) 1164:14–23. doi: 10.1016/j.brainres.2007.04.083
86. Deane R, LaRue B, Sagare AP, Castellino FJ, Zhong Z, Zlokovic BV. Endothelial protein C receptor-assisted transport of activated protein C across the mouse blood-brain barrier. J Cereb Blood Flow Metab. (2009) 29:25–33. doi: 10.1038/jcbfm.2008.117
87. Danese S, Vetrano S, Zhang L, Poplis VA, Castellino FJ. The protein C pathway in tissue inflammation and injury: pathogenic role and therapeutic implications. Blood. (2010) 115:1121–30. doi: 10.1182/blood-2009-09-201616
88. McDonnell CJ, Soule EE, Walsh PT, O'Donnell JS, Preston RJS. The immunoregulatory activities of activated protein C in inflammatory disease. Semin Thromb Hemost. (2018) 44:167–75. doi: 10.1055/s-0037-1608910
89. Zhong Z, Ilieva H, Hallagan L, Bell R, Singh I, Paquette N, et al. Activated protein C therapy slows ALS-like disease in mice by transcriptionally inhibiting SOD1 in motor neurons and microglia cells. J Clin Invest. (2009) 119:3437–49. doi: 10.1172/JCI38476
90. Han MH, Hwang SI, Roy DB, Lundgren DH, Price JV, Ousman SS, et al. Proteomic analysis of active multiple sclerosis lesions reveals therapeutic targets. Nature. (2008) 451:1076–81. doi: 10.1038/nature06559
91. Isermann IA, Vinnikov B, Madhusudhan T, Herzog S, Kashif M, Blautzik J, et al. Activated protein C protects against diabetic nephropathy by inhibiting endothelial and podocyte apoptosis. Nat Med. (2007) 13:1349–58. doi: 10.1038/nm1667
92. Scaldaferri F, Sans M, Vetrano S, Graziani C, De Cristofaro R, Gerlitz B, et al. Crucial role of the protein C pathway in governing microvascular inflammation in inflammatory bowel disease. J Clin Invest. (2007) 117:1951–60. doi: 10.1172/JCI31027
93. Mosnier LO, Gale AJ, Yegneswaran S, Griffin JH. Activated protein C variants with normal cytoprotective but reduced anticoagulant activity. Blood. (2004) 104:1740–4. doi: 10.1182/blood-2004-01-0110
94. Harmon RJ, Preston S, Ni Ainle F, Johnson JA, Cunningham MS, Smith OP, et al. Dissociation of activated protein C functions by elimination of protein S cofactor enhancement. J Biol Chem. (2008) 283:30531–9. doi: 10.1074/jbc.M802338200
95. Bae JS, Yang L, Manithody C, Rezaie AR. Engineering a disulfide bond to stabilize the calcium-binding loop of activated protein C eliminates its anticoagulant but not its protective signaling properties. J Biol Chem. (2007) 282:9251–9. doi: 10.1074/jbc.M610547200
96. Ní Ainle F, O'Donnell JS, Johnson JA, Brown L, Gleeson EM, Smith OP, et al. Activated protein C N-linked glycans modulate cytoprotective signaling function on endothelial cells. J Biol Chem. (2011) 286:1323–30. doi: 10.1074/jbc.M110.159475
97. Marshall JC. The staging of sepsis: understanding heterogeneity in treatment efficacy. Crit Care. (2005) 9:626–8. doi: 10.1186/cc3907
98. Eliwan HO, Watson WRG, Regan I, Philbin B, O'Hare FM, Strickland T, et al. Pediatric intensive care: immunomodulation with activated protein C ex vivo. Front Pediatr. (2019) 7:386. doi: 10.3389/fped.2019.00386
99. Leligdowicz A, Matthay MA. Heterogeneity in sepsis: new biological evidence with clinical applications. Crit Care. (2019) 23:80. doi: 10.1186/s13054-019-2372-2
100. Singer CS, Deutschman M, Seymour CW, Shankar-Hari M, Annane D, Bauer M, et al. The Third International Consensus definitions for sepsis and septic shock (Sepsis-3). JAMA. (2016) 315:801–10. doi: 10.1001/jama.2016.0287
Keywords: activated protein C, paediatric sepsis, neonatal sepsis, rhAPC, coagulation
Citation: Eliwan H, Omer M, McKenna E, Kelly LA, Nolan B, Regan I and Molloy EJ (2022) Protein C Pathway in Paediatric and Neonatal Sepsis. Front. Pediatr. 9:562495. doi: 10.3389/fped.2021.562495
Received: 30 July 2020; Accepted: 20 December 2021;
Published: 02 February 2022.
Edited by:
Marzia Duse, Sapienza University of Rome, ItalyReviewed by:
Yutaka Umemura, Osaka General Medical Center, JapanLovro Lamot, University of Zagreb, Croatia
Copyright © 2022 Eliwan, Omer, McKenna, Kelly, Nolan, Regan and Molloy. This is an open-access article distributed under the terms of the Creative Commons Attribution License (CC BY). The use, distribution or reproduction in other forums is permitted, provided the original author(s) and the copyright owner(s) are credited and that the original publication in this journal is cited, in accordance with accepted academic practice. No use, distribution or reproduction is permitted which does not comply with these terms.
*Correspondence: Eleanor J. Molloy, eleanor.molloy@tcd.ie