Transient Early Fine Motor Abnormalities in Infants Born to COVID-19 Mothers Are Associated With Placental Hypoxia and Ischemia
- 1Department of Gynaecology and Obstetrics, Zhongnan Hospital of Wuhan University, Wuhan, China
- 2Hubei Clinical Research Center for Prenatal Diagnosis and Birth Health, Wuhan, China
- 3Department of Preventive Medicine, Northwestern University Feinberg School of Medicine, Chicago, IL, United States
- 4Department of Clinical Laboratory, Center for Gene Diagnosis, and Program of Clinical Laboratory, Zhongnan Hospital of Wuhan University, Wuhan, China
- 5Department of Pathology, Zhongnan Hospital of Wuhan University, Wuhan, China
- 6Department of Pediatrics, Zhongnan Hospital of Wuhan University, Wuhan, China
- 7Institute of Precision Medicine, Jining Medical University, Jining, China
Background: Long-term effects of Coronavirus Disease 2019 (COVID-19) on infants born to infected mothers are not clear. Fine motor skills are crucial for the development of infant emotional regulation, learning ability and social skills.
Methods: Clinical information of 100 infants born to 98 mothers (COVID-19 n = 31, non-COVID-19 n = 67) were collected. Infants were follow-up up to 9 months post-partum. The placental tissues were examined for SARS-CoV-2 infection, pathological changes, cytokines, and mtDNA content.
Results: Decreased placental oxygen and nutrient transport capacity were found in infected pregnant women. Increased IL-2, IL-6, TNF-α, and IFN-γ were detected in trophoblast cells and maternal blood of COVID-19 placentas. Elevated early fine motor abnormal-ities and increased serum TNI (troponin I) levels at delivery were observed in infants born to mothers with COVID-19. Increased abnormal mitochondria and elevated mtDNA content were found in the placentas from infected mothers. The placental mtDNA content of three infants with abnormal DDST were increased by 4, 7, and 10%, respectively, compared to the mean of the COVID-19 group. The Maternal Vascular Malperfusion (MVM), elevated cytokines and increased placental mtDNA content in mothers with COVID-19 might be associated with transient early fine motor abnormalities in infants. These abnormalities are only temporary, and they could be corrected by daily training.
Conclusions: Babies born to COVID-19 mothers with mild symptoms appeared to have little or no excess long-term risks of abnormal physical and neurobehavioral development as compared with the infants delivered by non-COVID-19 mothers.
Introduction
Coronavirus Disease 2019 (COVID-19) is a respiratory tract infection caused by severe acute respiratory syndrome coronavirus 2 (SARS-CoV-2) and has resulted in a global pandemic (1). A number of studies have described the clinical characteristics and outcomes of COVID-19 pregnancies and their babies (2). In the United States alone, over 84,000 pregnant women have been infected with SARS-CoV-2 as of April 12th, 2021 (3). Our previous observational study suggested that there was no evidence for intrauterine infection caused by vertical transmission in COVID-19 women in late pregnancy at the beginning of the pandemic in China (4). To date, no serious adverse outcomes have been reported in neonates born to SARS-CoV-2-positive mothers (2). Some reports in both adults and children have associated COVID-19 with a variety of central and peripheral neurological insults (5).
Whether gestational exposure to SARS-CoV-2 infection could have a long-term impact on postnatal growth and neurobehavioral development has not been investigated. Of particular interest to us is fine motor development, which is an important component for children general growth and successful participation in daily activities. Children with a history of transient neurological abnormalities are at a higher risk for poorer cognitive and academic skills than those with normal neurological findings during their 1st year of school (6). Transient neurodevelopmental abnormalities in infancy are also associated with developmental abnormalities at 2–3 years of age and learning abnormalities at 5 years of age (7). Calame et al. also found an increased rate of delayed language development and behavioral abnormalities at age 3 in children with transient neurologic abnormalities (8). These results suggested that long-term impact on infants' neurodevelopment after COVID-19 needs to be investigated with more cases, multidimensional indicators and longer time follow-up. Previous studies have found that infants manifesting fine motor problems could benefit from early intervention to improve their development and to prevent further complications (9–12). Therefore, it is of great importance to monitor fine motor development in infants born to COVID-19 mothers that may provide urgently needed information for possible early intervention in affected children.
Physiologically, the placenta serves a myriad of functions that support fetal growth and survival (13). In the cell, mitochondria play a central role in maintaining oxidative homeostasis and reactive oxide species (ROS) production (14). Restricted nutrients, oxidative stress, and inflammation may impair mitochondrial oxidative phosphorylation, leading to reduced ATP and excessive ROS (15, 16). Since the newborn brain accounts for almost 60% of total body oxygen and glucose consumption (17), this level of high-energy demand makes brain development particularly susceptible to impairment from nutritional shortfalls (18). Moreover, brain glucose requirement is associated inversely to body growth from infancy to puberty (18). To compensate for defective mitochondrial functions, the placenta could undergo metabolic adaptations by promoting mitochondrial biogenesis (15). As a matter of fact, elevated mtDNA copy numbers in the placentas have been associated with reduced birthweight, suggesting that mtDNA might be a potential biomarker for placental insufficiency (19, 20).
With the on-going global pandemic, the impact of COVID-19 on pregnant women, newborns have received worldwide attention. The Maternal Vascular Malperfusion (MVM) was found in placentas of mothers with COVID-19 (21–23). Under the MVM, whether there is mitochondrial dysfunction in the placentas of pregnant women with COVID-19 and whether these abnormalities are associated with developmental outcomes in infants remain unclear. Specifically, in the current study, taking advantage of our clinical resources, we explored the placental pathology and mitochondrial function of COVID-19 in pregnancy, examined the developmental outcomes of affected infants after the follow-up of ~9 months, and evaluated their correlations.
Materials and Methods
Study Design and Participants
The study design is shown in Figure 1. A total of 98 pregnant women (COVID-19, n = 31; non-COVID-19 pneumonia, n = 18; controls, n = 49) and 100 newborns were recruited at Zhongnan Hospital of Wuhan University, China during 01/2020-1/2021. SARS-CoV-2 infection was confirmed with both RT-PCR test and serum antibody assay according to the Chinese Novel Coronavirus Pneumonia Prevention and Control Program (7th edition) (24). Among the 18 non-COVID-19 pregnancies with pneumonia, there were 2 cases of influenza A infection, 4 cases of both influenza A and B infections, 5 cases of bacterial infections, the rest 7 cases had no pathogenic confirmation. The 49 controls were pregnant women matched for age, gestational age at delivery (GAD), and underlying illnesses including pregnancy-induced hypertension (PIH) and gestational diabetes mellitus (GDM). All pregnant women delivered by cesarean section and newborns were transferred to the Neonatal Intensive Care Unit (NICU) to reduce the risk of SARS-CoV-2 infection (4). This study was reviewed and approved by the Medical Ethical Committee of Zhongnan Hospital of Wuhan University (Approval Number: 2020080H).
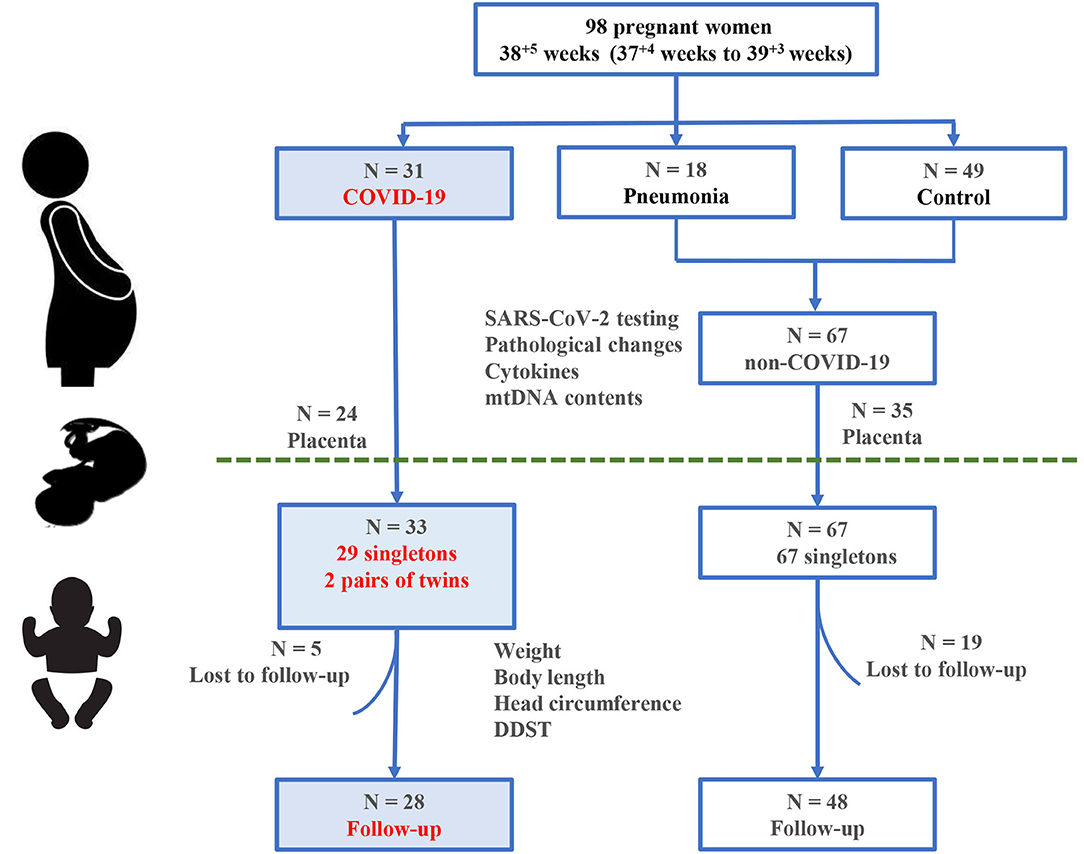
Figure 1. Study design and participants. A total of 98 pregnant women and 100 infants born to them are included in this study.
Clinical Data Collection
Demographic and clinical information were collected from medical records. Laboratory testing included routine blood cell counts, coagulation function, liver function, renal function, serum electrolytes, myocardial markers, lipids, inflammatory markers and cytokines.
Body weight and length are the most important and sensitive indicators that reflect children's growth and development, while the head circumference reflects the degree of development of the brain and skull. Therefore, weight, body length and head circumference were measured by a neonatologist. The standard growth curve was plotted according to the 2009 reference standards from the Ministry of Health of China (25). The development of a premature infant was evaluated with corrected age. The Denver Developmental Screening Test (DDST, 2nd Edition) (26) was used to evaluate neurodevelopmental levels for all infants in this study.
Parent-Guided Postnatal Training and Intervention
To improve fine motor performance for infants with fine motor skills abnormalties by the DDST, parents were advised to train their babies for at least 5 months, including visual motor perception (e.g., picking up the rattle) and fine motor training-based intervention (e.g., throwing, catching, opening hands, touching, grasping, and bimanual task). The frequency was 5–10 min once for 3–5 times per day (9, 27). Additionally, the infants were asked to be breast-feeding and lived in the enriched environments (28, 29).
Placental Characterization and Determination of mtDNA Copy Numbers
The pathologies of placentas were examined according to the Amsterdam Placental Workshop Group Consensus Statement (30). The MVM includes pathological features such as placental infarction, decidual vascular disease, accelerated villous maturation, and villous infarction (30). We collected 10 placentas from COVID-19 pregnant women with infection duration ≥7 days and 10 placentas from matched non-COVID-19 pregnant women as described in Study Design and Participants. Supplementary Table S7 summarizes their clinical and pathological findings. The placental tissues were examined for SARS-CoV-2 infection using the RT-PCR with the Daan Gene Kit (Guangzhou, China).
The H&E staining was used to observe the pathological features of placentas, following the standard protocol (31). Placental interhemal membrane (IHM) is a trilaminar barrier that is made up of syncytiotrophoblast, cytotrophoblast and fetal capillary endodermis cells. Thicker IHM may hamper transport efficiency of nutrients especially oxygen. Even slight thickening of the placental IHM may reduce the placental oxygen diffusion capacity significantly (32). The average thickness of the placental IHM was measured along the direction of the test line, from intersections between the test line and the fetal capillaries to the closest maternal blood space through the orthogonal intercepts method (33).
Cytokine levels in the placental tissues were performed by the Enzyme Linked Immunosorbent Assay (ELISA) with commercial ELISA kits (Beijing 4A Biotech Co., Ltd) according to the instructions. The concentrations of cytokines were calculated according to standard curves by a special program for the evaluation of ELISA results. Transmission electron microscopy (Hitachi TEM system) was used to detected mitochondrial morphology. The qPCR that simultaneously targeted a single-copy nuclear gene (β2-microglobulin, β2M) and the tRNA Leu (UUR) gene in mtDNA was used to quantify mtDNA copy numbers (34).
Statistical Analysis
All statistical analyses were performed using the SPSS 21.0 (SPSS Inc., Chicago, IL, USA), or under the R Statistical Computing Environment (v 3.6.2). The one-way ANOVA or the Student's t-test were used to compare the differences in normally distributed data. The Mann-Whitney-test was used to assess the differences in skewed data. The Chi-square-test was conducted to test the categorical variables. Linear mixed-effect models with fixed mean and random intercept were implemented to model the within-subject data and determine whether age, sex, and COVID-19 infection status were able to predict the physical development of infants. P-value < 0.05 was used for statistical significance. The Bonferroni correction was used for evaluating multiple comparisons.
Results
Baseline Clinical Features of Mothers
Table 1 summarizes the clinical characteristics of the pregnancies (n = 98). The mean age, BMI, GAA, gravidity and parity did not differ across the three groups (COVID-19, n = 31; non-COVID-19 pneumonia, n = 18; controls, n = 49). The baseline medical conditions of the COVID-19 pregnancies included PIH (19.4%), GDM (12.9%), cardiovascular diseases (3.2%), liver dysfunction (3.2%), hepatitis B virus carrier (16.1%), hepatitis E virus carrier (3.2%), and influenza infection (9.7%). No significant difference in the comorbidities was found across different groups, except for hepatitis B virus carrier and influenza infection. Of note, main clinical manifestations of the COVID-19 pregnancies included fever (58.1%), cough (41.9%), muscle soreness (9.7%), dyspnea (1.4%), and diarrhea (1.4%), and were similar to a previous study (35). All of the pregnant women were moderate disease. Only 22 cases (71.0 %) had typical symptoms of SARS-COV-2 infection, the rest of 9 patients (29.0%) showed typical images of multiple patchy ground-glass shadows in lungs.
Laboratory findings were available from all of the 98 pregnant women (Supplementary Table S1). Elevated myocardial enzymes, severe coagulopathy, and abnormal liver function were common features of the COVID-19 pregnant women (Supplementary Table S1). In particular, serum lactate dehydrogenase (LDH) was significantly elevated in the COVID-19 group relative to the women with non-COVID-19 pneumonia. Additionally, the incidence of abnormal LDH in the COVID-19 group (7/25) was higher compared to those with non-COVID-19 pneumonia (0/19) (P = 0.01; Supplementary Table S2).
Baseline Clinical Features of Newborns
We combined the controls and those infants from those mothers with non-COVID-19 pneumonia into a single “non-COVID-19” group, because their clinical features appeared to be comparable (Table 2). All 100 live births (58 male and 42 female neonates), among whom 33 babies (29 singletons and 2 pairs of twins) were born to 31 women with COVID-19 (Table 2). After removing the neonates from mothers with PIH and GDM, the average birthweight of newborns in the COVID-19 group was significantly lower than those infants in the non-COVID-19 group (3.03 ± 0.13 kg vs. 3.29 ± 0.06 kg, P = 0.03). All neonates in the COVID-19 group were tested negative for SARS-CoV-2. In addition, all babies showed negative results when they underwent serum SARS-CoV-2 antibody assay at the age of 1 month. Chest X-ray was performed in 25 newborns born to COVID-19 mothers who were immediately transferred to the NICU after birth. One infant born to a COVID-19 mother had patchy shadow in the middle lobe of right lung in the first exam, but negative in the replicate tests.
Overall, the laboratory testing results of the COVID-19 group had a significantly lower levels of white blood cells (P = 0.02), lymphocytes (P = 0.005), and aminotransferase (P = 0.03). Notably, the median of serum cardiac troponin I (TNI) showed ~2-fold increase in newborns in the COVID-19 group (20.32 ± 3.41 pg/mL vs. 9.66 ± 1.27 pg/mL, P = 0.007) (Supplementary Table S3), reflecting a strikingly different rate of abnormal TNI (20%, 5/25) in the COVID-19 group vs. (0%, 0/23) in the non-COVID-19 group (P = 0.05; Supplementary Table S4). Overall, the average birthweight of newborns was significantly decreased in the COVID-19 group when excluding maternal complications.
Development Outcomes in Infants
In total, 76 infants (COVID-19, n = 28; non-COVID-19, n = 48, including 11 infants born to mothers with non-COVID-19 pneumonia mothers) were followed up between March and November, 2020, with the median follow-up of 5 months. The main reason for loss of follow-up was parental decline to further participate in the study.
No significant differences in clinical and developmental parameters were found between the two groups, including sex, GAD, birthweight, body length and head circumference at birth. The proportion of preterm showed no difference between the two groups (17.9 vs. 14.3%) (Supplementary Table S5).
The Physical Development Index (i.e., weight, body length, and head circumference) of infants were collected and compared. Table 3 presents results from a linear mixed-effect model predicting physical development of infants. The growth curve of weight, body length and head circumference showed a similar trend in the COVID-19 group compared to the non-COVID-19 group (P > 0.05; Figures 2B,C). In female infants, the COVID-19 group had 0.45 kg lower weight, 2.44 cm shorter in body length and 1.01 cm smaller in head circumference as compared with the non-COVID-19 group (All P < 0.05). In contrast, there was no significant difference in physical development between the two groups among male infants (Table 3).
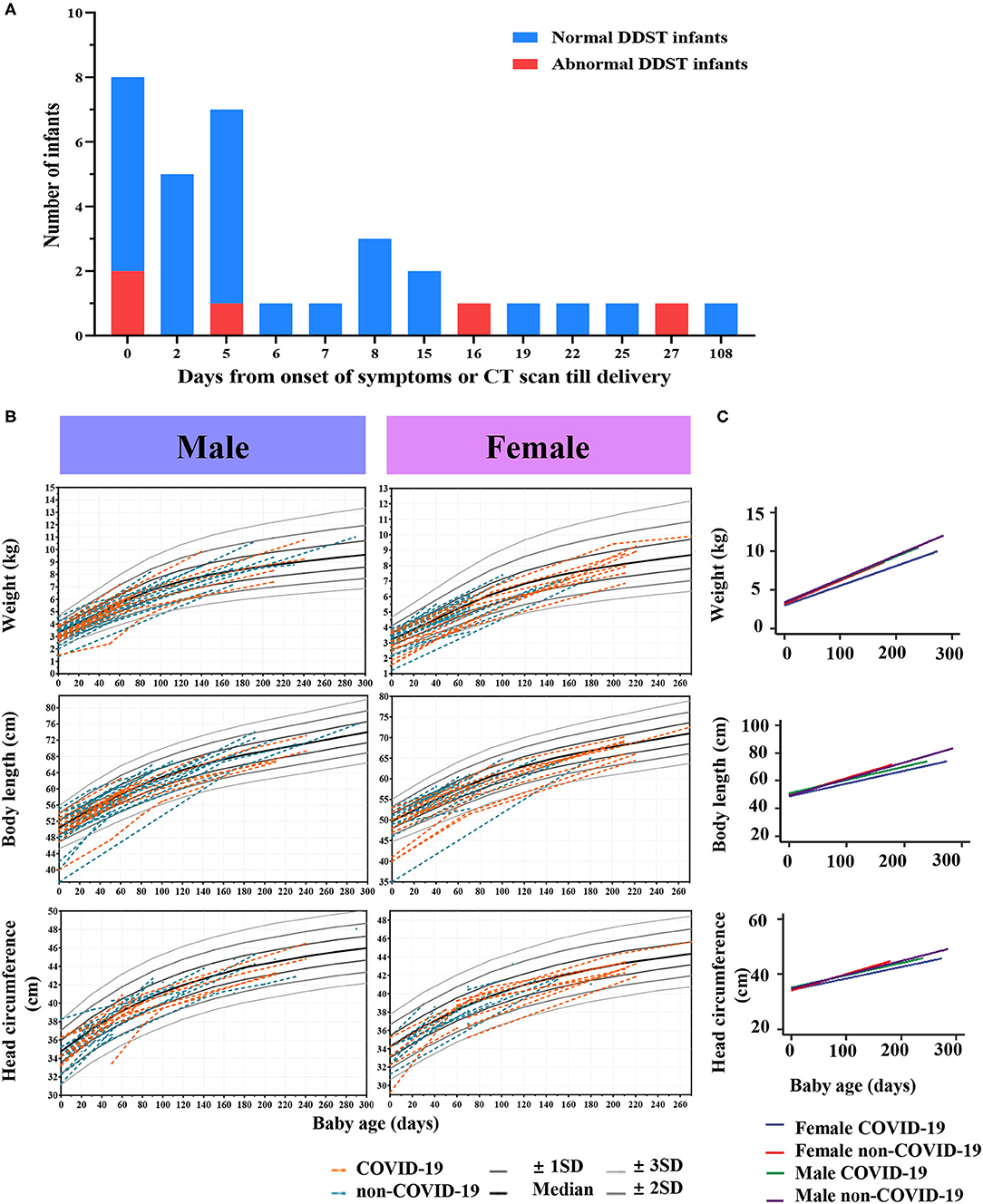
Figure 2. Follow-up outcomes of infants. (A) Distributions of infants with normal or abnormal DDST delivered by COVID-19 mothers. (B) Infants' growth curves of weight, body length and head circumference in the COVID-19 group and the non-COVID-19 group. (C) Linear mixed-effect models with fixed mean and random intercept were developed to model the within-subject data and determine whether age, sex, and COVID-19 infection status were able to predict the physical development of infants.
We observed fine motor abnormalities in five infants born to mothers with COVID-19, significantly higher than in the control group (15.2%, 5/28 vs. 2.1%, 1/48, P = 0.02) (Figure 2A). The maternal-infant demographic and clinical findings in infants with abnormal DDST are listed in Supplementary Table S6. The 5 infants in the COVID-19 group showing neurodevelopmental delay were full-term, normal in birthweight, and their mothers had no complications such as PIH or GDM. Notably, these 5 infants showed delay in “Fine motor-adaptive” domain at the age of 1–2 months (Table 4). Parents of these infants were asked to perform parent-guided postnatal training and intervention. Specifically, case 9 and case 11 passed the examinations in the second DDST at the age of 6–7 months. Additionally, case 3, 9, and 11 passed examinations at the age of 12–13 months, indicating that the observed neurodevelopmental delay would be short-term effects that could be corrected by postnatal training.
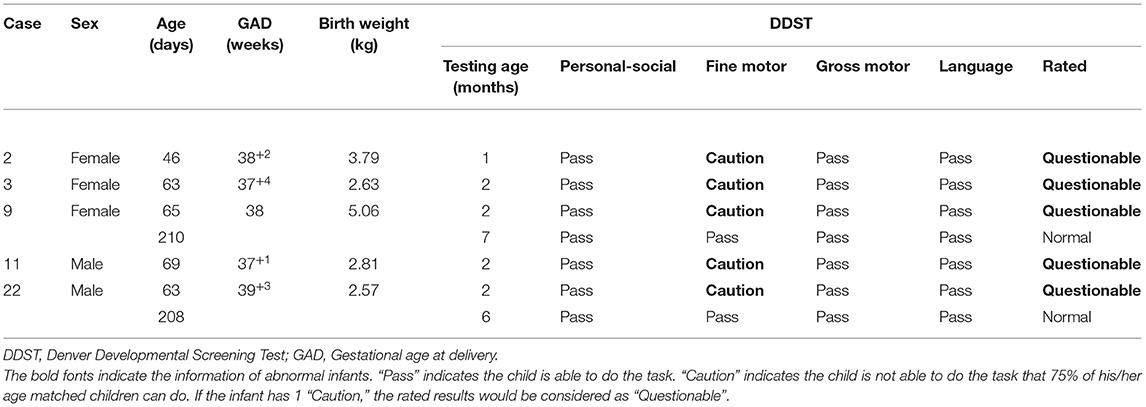
Table 4. Demographic and clinical findings in the 5 infants in the COVID-19 group with a diagnosis of neurodevelopmental delay by the DDST.
Maternal Vascular Malperfusion Were Present in COVID-19 Placentas
We observed that more than 5% of placental infarcts were observed in the sagittal surface of the COVID-19 placentas (Figure 3A). The rate of increased intervillous fibrin was higher in the COVID-19 group (7/10 vs. 0/10, P = 0.03) (Figure 3D, Supplementary Table S8). Besides, increased intervillous thrombus (4/10, Figure 3B), syncytial knots (5/10, Figure 3C), decidual arteriopathy (6/10, Figure 3E) and villous infarction (6/10, Figure 3F) were also observed in COVID-19 pregnancies, but showed no statistical differences between the two groups (Supplementary Table S8). Additionally, we also observed chorangiosis in COVID-19 placentas (Figure 3G). Of note, we found that the placental IHM thickness significantly increased in the COVID-19 group (P = 0.001, Figure 3H). These results supported that hypoxia and ischemia were present in COVID-19 placentas.
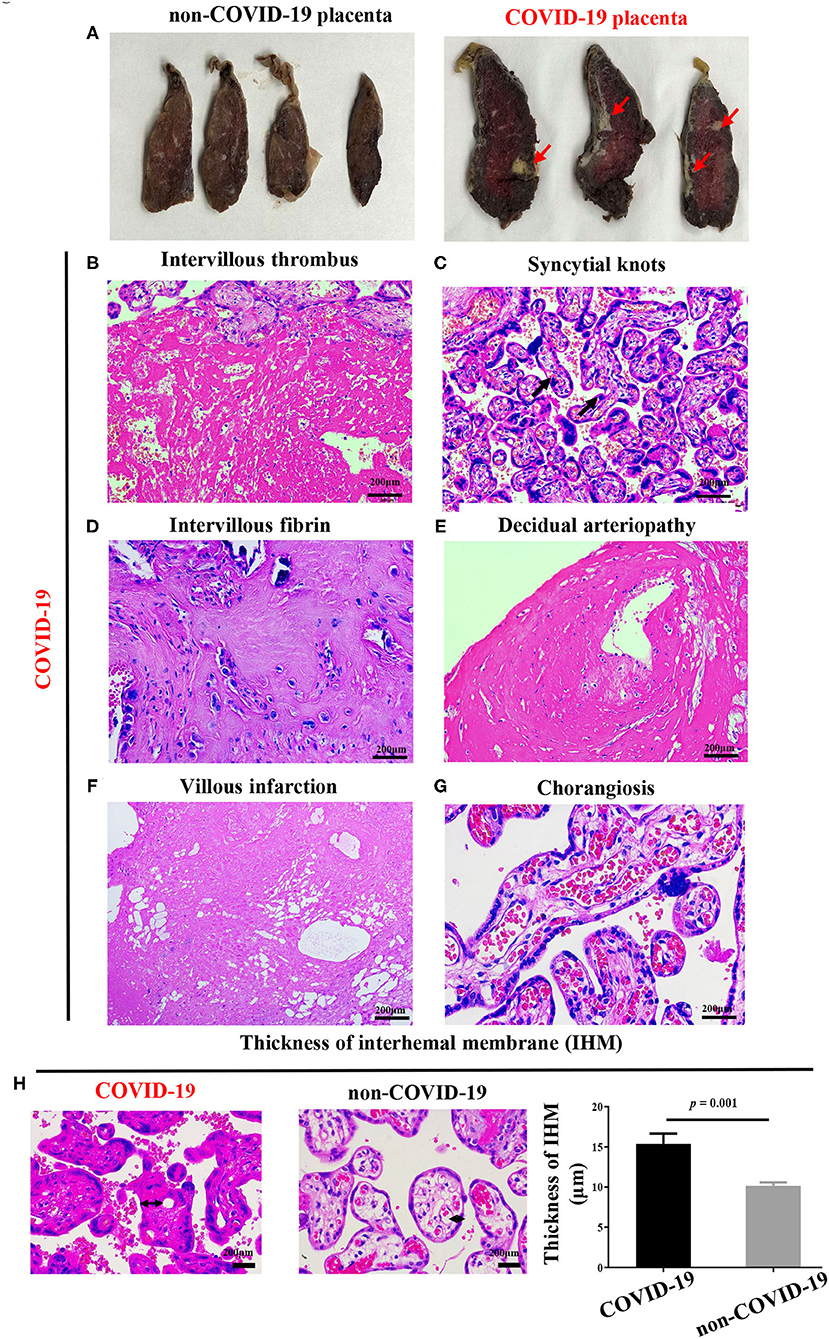
Figure 3. Pathological changes of COVID-19 placentas. (A) Sagittal plane of COVID-19 placentas showing hypoxia and infarction, (B–H) H&E stains of COVID-19 placentas showing (B) intervillous thrombus (C) syncytial knots and (D) intervillous fibrin, (E) decidual arteriopathy, (F) villous infarction, (G) chorangiosis, and (H) increased thickness of IHM, as compared with non-COVID-19 placentas, indicating placental oxygen and nutrient transport capacity are decreased. P-values were derived from the Student's t-test.
Increased Cytokines in COVID-19 Placentas
Because placental ischemia also stimulates the release of cytokines, we further explored the level and location of cytokines. Compared with that in the non-COVID-19 group, the levels of Interleukin-2 (IL-2), Interleukin-6 (IL-6), Interferon-gamma (IFN-γ) and tumor necrosis factor-alpha (TNF-α) were significantly increased in the COVID-19 group (P < 0.05; Table 5). Immunofluorescent staining further revealed that IFN-γ was co-localized with the maternal blood in the placentas (Figures 4A,D), but TNF-α was primarily co-localized with placental trophoblast cells in the COVID-19 group (Figures 4B,E). In addition, our immunofluorescent staining also showed co-localization of IL-2 with IL-6 was primarily in maternal blood in COVID-19 placentas (Figures 4C,F). These results indicated that hypoxia and ischemia in COVID-19 placentas could promote cytokines production by placental trophoblast cells.
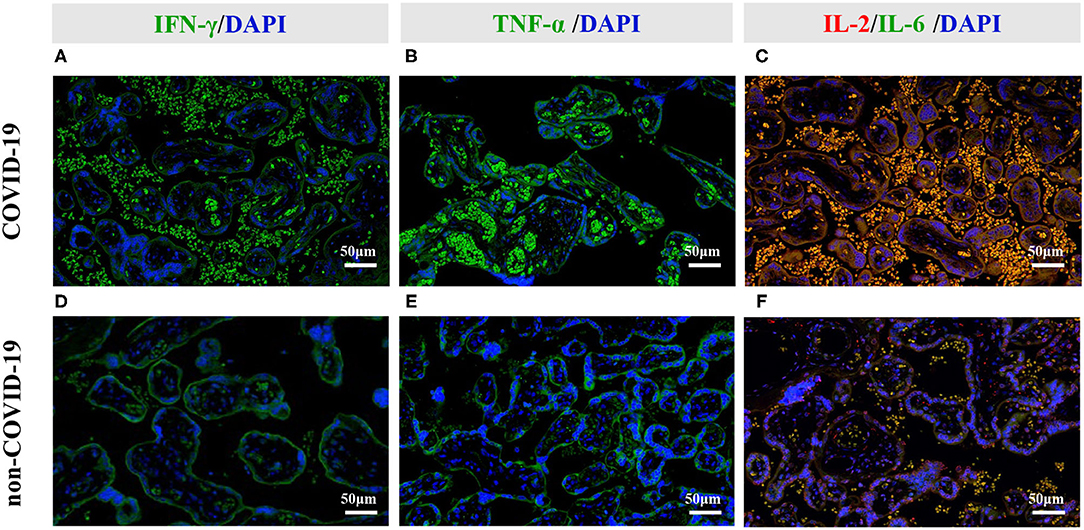
Figure 4. Representative photomicrographs of the immunofluorescent staining of IL-2, IL-6, IFN- γ, and TNF-α in the placental tissues. (A–C) Immunofluorescent staining of COVID-19 placentas. (D–F) Immunofluorescent staining of non-COVID-19 placentas. (A,D) Increased IFN-γ signal was primarily co-localized with maternal blood in COVID-19 placentas. (B,E) Increased TNF-α signal was primarily co-localized with placental trophoblast cells in COVID-19 placentas. (C,F) Increased IL-2 and IL-6 signals were primarily co-localized with the maternal blood in COVID-19 placentas.
Increase of Abnormal Mitochondria and mtDNA Content
As shown in Figures 5A–D, the proportion of abnormal mitochondria was increased in the villous syncytiotrophoblast of COVID-19 pregnant, indicating that mitochondrial dysfunction likely occurred in the placentas from infected women. The placental mtDNA content was also found to be elevated in COVID-19 mothers, as compared with the non-COVID-19 controls (4.66 ± 0.07 vs. 4.19 ± 0.06, P = 6 × 10−5) (Figure 5C).
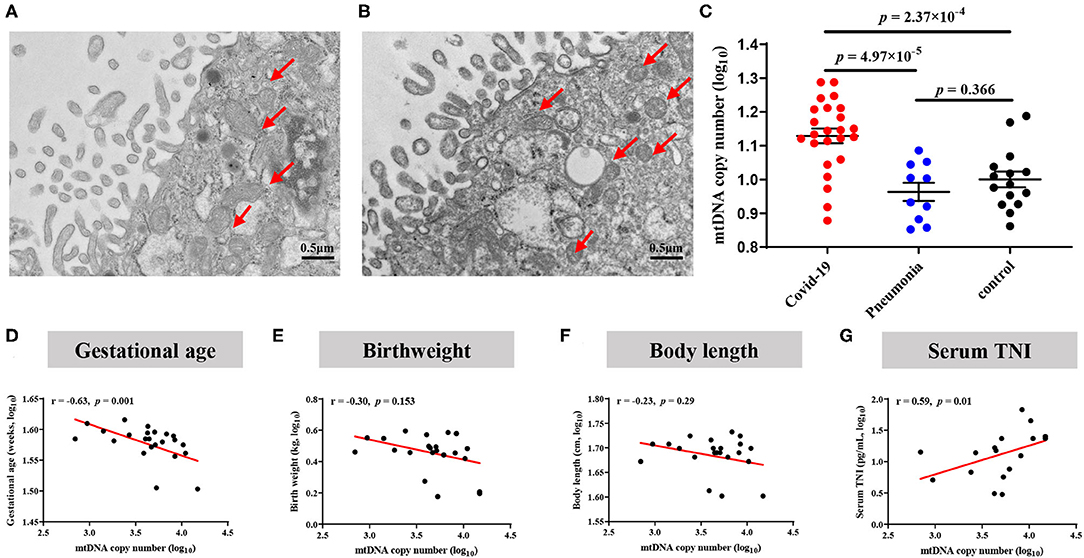
Figure 5. Alterations of mitochondrial morphology and mtDNA copy numbers in placentas. (A) Normal mitochondrial morphology in healthy placentas. (B) Elevated abnormal mitochondria in COVID-19 placentas. (C) Comparison of placental mtDNA copy numbers across COVID-19, pneumonia and Control group. P-values were derived from the Student's t-test. (D–G) COVID-19 pregnant women with increased placental mtDNA were associated with decreased birthweight. (D) Association of placental mtDNA and gestational age. (E) Association between placental mtDNA and birthweight. (F) Association between placental mtDNA and body length. (G) Association between placental mtDNA and neonatal serum TNI.
Association Between Placental mtDNA Content and Early Physical and Neurobehavioral Development
To investigate whether the increase of placental mtDNA content is associated with offspring health, we explored the correlation between placental mtDNA content and offspring outcome. We only found that the copy number of placental mtDNA was associated with GAD (r = −0.65, P = 0.001) and TNI (r = 0.59, P = 0.01) in the COVID-19 group (Figures 5D–G). Three infants with abnormal DDST results demonstrated placental mtDNA content increasing by 4, 7, and 10%, respectively, compared to the mean of the COVID-19 group.
Discussion
In the current study, we reported that poor maternal vascular perfusion, elevated cytokines production, increased abnormal mitochondria and elevated mtDNA copy numbers in the placentas of COVID-19 mothers. Early fine motor abnormalities which could be corrected in 6–7 months were elevated in the COVID-19 group compared to the non-COVID-19 group, supporting that neurodevelopmental delay might be short-term effects. Nevertheless, a similar trend of growth curve was shown in the COVID-19 and non-COVID-19 infants in the follow-up, indicating limited effects of COVID-19 on growth parameters after birth. In addition, mtDNA copy numbers appeared to be negatively associated with birthweight and GAD, and positively associated with infant serum TNI. Notably, infants with higher mtDNA content in placentas had a higher abnormal rate for the DDST at 1–2 months of age.
Significantly, poor MVM was the most significant feature associated with COVID-19 in the current study. Moreover, placental IHM thickening was also found in COVID-19 pregnancies that could influence placental oxygen and nutrient transport capacity. The MVM and chronic histiocytic intervillositis with trophoblast necrosis were found in COVID-19 placentas in several case reports (21, 22, 36, 37). Taken together, our findings provided support for the MVM in placentas from COVID-19 mothers, which could directly affect the fetal development in utero (38, 39).
It is also established that placental hypoxia may alter mitochondrial functions and mtDNA content, which eventually lead to fetal growth restriction (40). It is not clear whether mitochondrial functions and placental mtDNA would be changed in COVID-19 pregnant women, and their potential association with developmental delay. In agreement with previous reports, we found that the proportion of abnormal mitochondria was elevated in COVID-19 placentas. It is reasoned that to compensate for defective mitochondrial functions, their copy numbers would increase to fulfill energy demand for pregnancy maintenance under the placental hypoxia and ischemia status (15).
The so-called “cytokine storm” was observed in severe COVID-19 cases. Specifically, IFN-γ, IL-6, TNF-α, IL-10, IL-1, IL-5, and IL-8 were the main mediators behind cytokine storm (41, 42). Thus, evaluation of cytokine profiles in COVID-19 placentas attracted our attention. In severe COVID-19 cases, viral proteins may induce pro-inflammatory cytokines (43, 44). It is reported that IL-2, IL-6, TNF-α, and IFN-γ are increased in peripheral blood of COVID-19 pregnant and the level of IFN-γ is positively correlated with disease severity in pregnant women with COVID-19 (45). In the COVID-19 placentas, some studies observed elevated IL-5 levels as well as decreased IL-7 and TNF-related apoptosis-inducing ligand levels. In particular, the level of IFN-γ was higher in placentas that tested positive for both viral nucleic acids and proteins relative to placentas that tested positive only for the viral proteins (46). However, significantly higher levels of IL-2, IL-6, TNF-α, and IFN-γ were found in the pregnant women with COVID-19 infection. The locations of these cytokines were primarily in trophoblast cells and the maternal blood in placentas. Of note, under the placental hypoxia and ischemia condition, IL-6 and TNF-α were associated with fetal behaviors and neuro-pathologies (47). Additionally, fetal immune response to the increased postnatal level of IFN-γ was associated with the degree of white matter damage in the brain (48). Therefore, our observation of the elevated cytokines in COVID-19 placentas could provide novel insights into the link with a higher rate of abnormal DDST in infants.
The baseline and laboratory characteristics of COVID-19 pregnant women were consistent with previous studies (2, 49, 50). In our study, there are 5 pregnant women infected with hepatitis B. We collected the results of second liver two half-and-half detect and HBV-DNA from medical records, and found that the HBV-DNA copy number of all 5 pregnant women were under 1 × 103 U/mL. And theses pregnant women were recommended to the HBV mother-to-child blocking clinic of our hospital. None of the newborns were infected with HBV at birth. Previous studies have found that pregnant women infected with HBV increased the infant's risk of infection (51, 52). But there did not appear to be any reported effects on the infant's physical or neurodevelopment. Our follow-up results also suggested that the physical development of the infants delivered by these five pregnant women was normal and there was no DDST abnormality. A large-scale systematic review and meta-analysis showed that low birthweight and preterm birth were more probable in pregnant woman with COVID-19, and low birthweight was one of the most prevalent infant complications (50). In our study, the average birthweight of infants was slightly lower in the COVID-19 group, the median and rate of abnormal TNI in newborns were also significantly increased in the COVID-19 group. Previous studies suggested that TNI can be used not only as a marker for neonatal myocardial injury, but also a marker for neonatal hypoxic ischemic encephalopathy (53, 54). Our findings indicated that neonates born to COVID-19 mothers might be at risk for intrauterine ischemia and hypoxia.
In addition, the copy number of placental mtDNA has been functioned as a potential biomarker for placental insufficiency and intrauterine growth restriction (55–57). The placental mitochondrial DNA copy number has been associated with reduced birthweight in women with placental malaria (58). Here, we found that the placental mtDNA content was associated with GAD and birthweight. Consistently, the placental mtDNA content of preterm delivery was also higher than term delivery in COVID-19 pregnant women, reflecting the trend of different incidence of preterm delivery between the two groups. Furthermore, the placental mtDNA was positively correlated with TNI levels in newborns. Taken together, these findings indicated that the elevated placental mtDNA in COVID-19 was compensating for infant nutrient and oxygen requirements due to poor maternal vascular perfusion.
The three infants with abnormal DDST at 1–2 months of age passed the examinations later, supporting that neurodevelopmental delay might be short-term effects which could be corrected. Moreover, the placental mtDNA content of infants with abnormal DDST was increased compared to the mean of all infants from COVID-19 mothers. A previous study has shown that the placental mtDNA content was associated with performance of intelligence quotient in childhood, emphasizing the importance of the intrauterine environment for intelligence and the role of placental mitochondrial functions (59). Overall, our findings revealed the significance of placental mitochondrial functions during fetal brain development in utero.
In this study, we reported a link between placental mitochondrial function and early physical and neurobehavioral development in infants delivered by COVID-19 mothers. Importantly, the relationship between placental mitochondria functions and infant development reported here was based on ~9 months follow-up, our on-going observations would enhance our understanding of any long-lasting consequences due to COVID-19. There are some limitations in the current study. Our study was based on a cohort of 31 COVID-19 pregnant women in Wuhan. The relatively small sample size may lead to a bias. Of course, we cannot exclude the effect of the time of DDST administration. Future studies with a larger study population and longer-term follow-up information will provide a clearer picture of the mitochondrial dysfunction and infant outcomes for affected infants.
Conclusions
The MVM, increased cytokines, and mtDNA content of placentas in mothers with COVID-19 appear to be associated with transient early fine motor abnormalities in infants. Our findings suggest that infants born to mothers with COVID-19 need developmental screening test in the early life, and provide evidence that could guide the prenatal and postnatal considerations.
Data Availability Statement
The raw data supporting the conclusions of this article will be made available by the authors, without undue reservation.
Ethics Statement
The studies involving human participants were reviewed and approved by Medical Ethical Committee of Zhongnan Hospital of Wuhan University. Written informed consent to participate in this study was provided by the participants' legal guardian/next of kin.
Author Contributions
WZ, S-ML, and YZ: concept and design, critical revision of the manuscript for important intellectual content, and supervision. H-YL, JG, TW, GY, DZ, PY, and XY: acquisition, analysis, or interpretation of data. H-YL, JG, and YC: performing experiments. CZ: fitting model. H-YL and JG: statistical analysis. H-YL, JG, CZ, WZ, S-ML, and YZ: drafting of the manuscript. YZ: obtained funding. All authors approved the final manuscript.
Funding
This study was supported partially by Hubei Provincial Science and Technology Department Novel Coronavirus Pneumonia Emergency Science and Technology Project (Grant No. 2020FCA011), Wuhan Novel Coronavirus Pneumonia Emergency Science and Technology Tackling Key Project (Grant No. 2020020201010011), Health Commission of Hubei Province Scientific Research Project (Grant No. WJ2019C002).
Conflict of Interest
The authors declare that the research was conducted in the absence of any commercial or financial relationships that could be construed as a potential conflict of interest.
Publisher's Note
All claims expressed in this article are solely those of the authors and do not necessarily represent those of their affiliated organizations, or those of the publisher, the editors and the reviewers. Any product that may be evaluated in this article, or claim that may be made by its manufacturer, is not guaranteed or endorsed by the publisher.
Acknowledgments
The authors thank all patients and clinical staff at Zhongnan Hospital of Wuhan University, China. We would like to appreciate Professor Yang Guan (Department of Pathology, Renmin Hospital, Wuhan University) and Doctor Hong Cao (Department of Pathology, Zhongnan Hospital of Wuhan University) for critical pathology review.
Supplementary Material
The Supplementary Material for this article can be found online at: https://www.frontiersin.org/articles/10.3389/fped.2021.793561/full#supplementary-material
References
1. Wu Z, McGoogan MJ. Characteristics of and important lessons from the coronavirus disease 2019 (COVID-19) outbreak in China: summary of a report of 72 314 cases from the chinese center for disease control and prevention. JAMA. (2020) 323:1239–42. doi: 10.1001/jama.2020.2648
2. Wastnedge EAN, Reynolds RM, van Boeckel SR, Stock SJ, Denison FC, Maybin JA, et al. Critchley: pregnancy and COVID-19. Physiol Rev. (2021) 101:303–18. doi: 10.1152/physrev.00024.2020
4. Chen H, Guo J, Wang C, Luo F, Yu X, Zhang W, et al. Zhang: clinical characteristics and intrauterine vertical transmission potential of COVID-19 infection in nine pregnant women: a retrospective review of medical records. Lancet. (2020) 395:809–15. doi: 10.1016/S0140-6736(20)30360-3
5. Lin JE, Asfour A, Sewell TB, Hooe B, Pryce P, Earley C, et al. Geneslaw: neurological issues in children with COVID-19. Neurosci Lett. (2021) 743:135567. doi: 10.1016/j.neulet.2020.135567
6. Harmon HM, Taylor HG, Minich N, Wilson-Costello D. Hack M. Early school outcomes for extremely preterm infants with transient neurological abnormalities. Dev Med Child Neurol. (2015) 57:865–71. doi: 10.1111/dmcn.12811
7. PeBenito R, Santello MD, Faxas TA, Ferretti C. Fisch BC. Residual developmental disabilities in children with transient hypertonicity in infancy. Pediatr Neurol. (1989) 5:154–60. doi: 10.1016/0887-8994(89)90064-7
8. Calame A, Reymond-Goni I, Maherzi M, Roulet M, Marchand C, Prod'hom SL. Psychological and neurodevelopmental outcome of high risk newborn infants. Helv Paediatr Acta. (1976) 31:287–97.
9. Blauw-Hospers CH. Hadders-Algra M. A systematic review of the effects of early intervention on motor development. Dev Med Child Neurol. (2005) 47:421–32. doi: 10.1017/S0012162205000824
10. Zeng N. Ayyub M. Effects of physical activity on motor skills and cognitive development in early childhood: a systematic review. Biomed Res Int. (2017) 2017:2760716. doi: 10.1155/2017/2760716
11. Morgan C, Darrah J, Gordon AM, Harbourne R, Spittle A, Johnson R, et al. Fetters: effectiveness of motor interventions in infants with cerebral palsy: a systematic review. Dev Med Child Neurol. (2016) 58:900–9. doi: 10.1111/dmcn.13105
12. Spittle A, Orton J, Anderson PJ, Boyd R, Doyle WL. Early developmental intervention programmes provided post hospital discharge to prevent motor and cognitive impairment in preterm infants. Cochrane Database Syst Rev. (2015) 11:Cd005495. doi: 10.1002/14651858.CD005495.pub4
13. Coyne CB, Lazear MH. Zika virus - reigniting the TORCH. Nat Rev Microbiol. (2016) 14:707–15. doi: 10.1038/nrmicro.2016.125
14. Erusalimsky JD, Moncada S. Nitric oxide and mitochondrial signaling: from physiology to pathophysiology. Arterioscler Thromb Vascular Biol. (2007) 27:2524–31. doi: 10.1161/ATVBAHA.107.151167
15. Holland O, Dekker Nitert M, Gallo LA, Vejzovic M, Fisher JJ, Perkins VA. Review: placental mitochondrial function and structure in gestational disorders. Placenta. (2017) 54:2–9. doi: 10.1016/j.placenta.2016.12.012
16. Cherry AD, Piantadosi AC. Regulation of mitochondrial biogenesis and its intersection with inflammatory responses. Antioxid Redox Signal. (2015) 22:965–76. doi: 10.1089/ars.2014.6200
17. Bastian TW, Rao R, Tran PV, Georgieff KM. The effects of early-life iron deficiency on brain energy metabolism. Neurosci Insights. (2020) 15:2633105520935104. doi: 10.1177/2633105520935104
18. Kuzawa CW, Chugani HT, Grossman LI, Lipovich L, Muzik O, Hof PR, et al. Metabolic costs and evolutionary implications of human brain development. Proc Natl Acad Sci USA. (2014) 111:13010–5. doi: 10.1073/pnas.1323099111
19. Mandò C, De Palma C, Stampalija T, Anelli GM, Figus M, Novielli C, et al. Placental mitochondrial content and function in intrauterine growth restriction and preeclampsia. Am J Physiol Endocrinol Metab. (2014) 306:E404–13. doi: 10.1152/ajpendo.00426.2013
20. Lattuada D, Colleoni F, Martinelli A, Garretto A, Magni R, Radaelli T, et al. Higher mitochondrial DNA content in human IUGR placenta. Placenta. (2008) 29:1029–33. doi: 10.1016/j.placenta.2008.09.012
21. Baergen RN, Heller SD. Placental pathology in covid-19 positive mothers: preliminary findings. Pediatr Dev Pathol. (2020) 23:177–80. doi: 10.1177/1093526620925569
22. Gao L, Ren J, Xu L, Ke X, Xiong L, Tian X, et al. Placental pathology of the third trimester pregnant women from COVID-19. (2021) 16:8. doi: 10.1186/s13000-021-01067-6
23. Shanes ED, Mithal LB, Otero S, Azad HA, Miller ES, Goldstein AJ. Placental pathology in COVID-19. Am J Clin Pathol. (2020) 154:23–32. doi: 10.1093/ajcp/aqaa089
25. M. o. H. o. China: Reference standards for the growth and development of children under 7 years old in China. Beijing (2009).
26. Frankenburg WK, Dodds J, Archer P, Shapiro H, Bresnick B. The Denver II: a major revision and restandardization of the Denver. Dev Screen Test Pediatr. (1992) 89:91–7.
27. Smits-Engelsman BC, Blank R, van der Kaay AC, Mosterd-van der Meijs R, Vlugt-van den Brand E, Polatajko HJ, et al. Efficacy of interventions to improve motor performance in children with developmental coordination disorder: a combined systematic review and meta-analysis. Dev Med Child Neurol. (2013) 55:229–37. doi: 10.1111/dmcn.12008
28. Dee DL, Li R, Lee LC, Grummer-Strawn ML. Associations between breastfeeding practices and young children's language and motor skill development. Pediatrics. (2007) 119(Suppl. 1):S92–8. doi: 10.1542/peds.2006-2089N
29. Morgan C, Novak I, Badawi N. Enriched environments and motor outcomes in cerebral palsy: systematic review and meta-analysis. Pediatrics. (2013) 132:e735–46. doi: 10.1542/peds.2012-3985
30. Khong TY, Mooney EE, Ariel I, Balmus NC, Boyd TK, Brundler MA, et al. Sampling and definitions of placental lesions: Amsterdam placental workshop group consensus statement. Arch Pathol Lab Med. (2016) 140:698–713. doi: 10.5858/arpa.2015-0225-CC
31. Feldman AT, Wolfe D. Tissue processing and hematoxylin and eosin staining. Methods Mol Biol. (2014) 1180:31–43. doi: 10.1007/978-1-4939-1050-2_3
32. Mayhew TM, Jackson MR, Haas DJ. Microscopical morphology of the human placenta and its effects on oxygen diffusion: a morphometric model. Placenta. (1986) 7:121–31. doi: 10.1016/S0143-4004(86)80003-0
33. Jensen EB, Gundersen HJ, Osterby R. Determination of membrane thickness distribution from orthogonal intercepts. J Microsc. (1979) 115:19–33. doi: 10.1111/j.1365-2818.1979.tb00149.x
34. Venegas V, Wang J, Dimmock D, Wong JL. Real-time quantitative PCR analysis of mitochondrial DNA content. Curr Protoc Hum Genet. (2011) Chapter 19, Unit 19.7. doi: 10.1002/0471142905.hg1907s68
35. Allotey J, Stallings E, Bonet M, Yap M, Chatterjee S, Kew T, et al. Clinical manifestations, risk factors, and maternal and perinatal outcomes of coronavirus disease 2019 in pregnancy: living systematic review and meta-analysis. BMJ. (2020) 370:m3320. doi: 10.1136/bmj.m3320
36. Poisson TM, Pierone G Jr. Placental pathology and fetal demise at 35 weeks of gestation in a woman with SARS-CoV-2 infection: a case report. Case Rep Womens Health. (2021) 30:e00289. doi: 10.1016/j.crwh.2021.e00289
37. Schwartz DA, Baldewijns M, Benachi A, Bugatti M, Collins RRJ, De Luca D, et al. Vivanti: chronic histiocytic intervillositis with trophoblast necrosis are risk factors associated with placental infection from coronavirus disease 2019 (COVID-19) and intrauterine maternal-fetal severe acute respiratory syndrome coronavirus 2 (S2ARS-CoV-2) transmission in liveborn and stillborn infants. Arch Pathol Lab Med. (2021) 145:517–28. doi: 10.5858/arpa.2020-0771-SA
38. Burton GJ, Jauniaux E. Pathophysiology of placental-derived fetal growth restriction. Am J Obstet Gynecol. (2018) 218:S745–61. doi: 10.1016/j.ajog.2017.11.577
39. Yockey LJ, Iwasaki A. Interferons and proinflammatory cytokines in pregnancy and fetal development. Immunity. (2018) 49:397–412. doi: 10.1016/j.immuni.2018.07.017
40. Colson A, Sonveaux P, Debiève F, Sferruzzi-Perri NA. Adaptations of the human placenta to hypoxia: opportunities for interventions in fetal growth restriction. Hum Reprod Update. (2021) 27:531–69. doi: 10.1093/humupd/dmaa053
41. Nile SH, Nile A, Qiu J, Li L, Jia X, Kai G. COVID-19: pathogenesis, cytokine storm and therapeutic potential of interferons. Cytokine Growth Factor Rev. (2020) 53:66–70. doi: 10.1016/j.cytogfr.2020.05.002
42. Ragab D, Salah Eldin H, Taeimah M, Khattab R, Salem R. The COVID-19 cytokine storm; what we know so far. Front Immunol. (2020) 11:1446. doi: 10.3389/fimmu.2020.01446
43. Blanco-Melo D, Nilsson-Payant BE, Liu WC, Uhl S, Hoagland D, Møller R, et al. Imbalanced host response to SARS-CoV-2 drives development of COVID-19. Cell. (2020) 181:1036–45.e9. doi: 10.1016/j.cell.2020.04.026
44. Camiolo M, Gauthier M, Kaminski N, Ray A, Wenzel ES. Expression of SARS-CoV-2 receptor ACE2 and coincident host response signature varies by asthma inflammatory phenotype. J Allergy Clin Immunol. (2020) 146:315–324.e7. doi: 10.1016/j.jaci.2020.05.051
45. Tanacan A, Yazihan N, Erol SA, Anuk AT, Yucel Yetiskin FD, Biriken D, et al. The impact of COVID-19 infection on the cytokine profile of pregnant women: a prospective case-control study. Cytokine. (2021) 140:155431. doi: 10.1016/j.cyto.2021.155431
46. Wu H, Liao S, Wang Y, Guo M, Lin X, Wu J, et al. Zhang: molecular evidence suggesting the persistence of residual SARS-CoV-2 and immune responses in the placentas of pregnant patients recovered from COVID-19. Cell Prolif. (2021) 54:e13091. doi: 10.1111/cpr.13091
47. Warrington JP, Drummond HA, Granger JP, Ryan JM. Placental ischemia-induced increases in brain water content and cerebrovascular permeability: role of TNF-α. Am J Physiol Regul Integr Comp Physiol. (2015) 309:R1425–31. doi: 10.1152/ajpregu.00372.2015
48. Hansen-Pupp I, Harling S, Berg AC, Cilio C, Hellström-Westas L, Ley D. Circulating interferon-gamma and white matter brain damage in preterm infants. Pediatr Res. (2005) 58:946–52. doi: 10.1203/01.PDR.0000182592.76702.E8
49. Knight M, Bunch K, Vousden N, Morris E, Simpson N, Gale C, et al. Kurinczuk: characteristics and outcomes of pregnant women admitted to hospital with confirmed SARS-CoV-2 infection in UK: national population based cohort study. BMJ. (2020) 369:m2107. doi: 10.1136/bmj.m2107
50. Jafari M, Pormohammad A, Sheikh Neshin SA, Ghorbani S. Clinical characteristics and outcomes of pregnant women with COVID-19 and comparison with control patients: a systematic review and meta-analysis. Rev Med Virol. (2021) 31:1–16. doi: 10.1002/rmv.2208
51. Nayagam S, Thursz M, Sicuri E, Conteh L, Wiktor S, Low-Beer D, et al. Requirements for global elimination of hepatitis B: a modelling study. Lancet Infect Dis. (2016) 16:1399–408. doi: 10.1016/S1473-3099(16)30204-3
52. Dionne-Odom J, Cozzi GD, Franco RA, Njei B, Tita ATN. Treatment and prevention of viral hepatitis in pregnancy. Am J Obstet Gynecol. (2021) S0002-9378(21)00998-4. doi: 10.1016/j.ajog.2021.09.002
53. Neves AL, Henriques-Coelho T, Leite-Moreira A, Areias CJ. Cardiac injury biomarkers in paediatric age: are we there yet? Heart Fail Rev. (2016) 21:771–81. doi: 10.1007/s10741-016-9567-2
54. Lipshultz SE, Rifai N, Sallan SE, Lipsitz SR, Dalton V, Sacks DB, et al. Predictive value of cardiac troponin T in pediatric patients at risk for myocardial injury. Circulation. (1997) 96:2641–8. doi: 10.1161/01.CIR.96.8.2641
55. Naha R, Anees A, Chakrabarty S, Naik PS, Pandove M, Pandey D, et al. Placental mitochondrial DNA mutations and copy numbers in intrauterine growth restricted (IUGR) pregnancy. Mitochondrion. (2020) 55:85–94. doi: 10.1016/j.mito.2020.08.008
56. Manna S, McCarthy C, McCarthy PF. Placental ageing in adverse pregnancy outcomes: telomere shortening, cell senescence, mitochondrial dysfunction. Oxidat Med Cell Long. (2019) 2019:3095383. doi: 10.1155/2019/3095383
57. Guitart-Mampel M, Juarez-Flores DL, Youssef L, Moren C, Garcia-Otero L, Roca-Agujetas V, et al. Mitochondrial implications in human pregnancies with intrauterine growth restriction and associated cardiac remodeling. J Cell Mol Med. (2019) 23:3962–73. doi: 10.1111/jcmm.14282
58. Oktavianthi S, Fauzi M, Trianty L, Trimarsanto H, Bowolaksono A, Noviyanti R, et al. Placental mitochondrial DNA copy number is associated with reduced birth weight in women with placental malaria. Placenta. (2019) 80:1–3. doi: 10.1016/j.placenta.2019.03.005
Keywords: COVID-19, fine motor skills, placental mtDNA, infants, physical and neurobehavioral development
Citation: Liu H-Y, Guo J, Zeng C, Cao Y, Ran R, Wu T, Yang G, Zhao D, Yang P, Yu X, Zhang W, Liu S-M and Zhang Y (2022) Transient Early Fine Motor Abnormalities in Infants Born to COVID-19 Mothers Are Associated With Placental Hypoxia and Ischemia. Front. Pediatr. 9:793561. doi: 10.3389/fped.2021.793561
Received: 12 October 2021; Accepted: 29 November 2021;
Published: 06 January 2022.
Edited by:
Salvatore Savasta, University of Pavia, ItalyReviewed by:
Alice Bonuccelli, Pisana University Hospital, ItalyAlessandro Borghesi, Fondazione Ospedale San Matteo (IRCCS), Italy
Copyright © 2022 Liu, Guo, Zeng, Cao, Ran, Wu, Yang, Zhao, Yang, Yu, Zhang, Liu and Zhang. This is an open-access article distributed under the terms of the Creative Commons Attribution License (CC BY). The use, distribution or reproduction in other forums is permitted, provided the original author(s) and the copyright owner(s) are credited and that the original publication in this journal is cited, in accordance with accepted academic practice. No use, distribution or reproduction is permitted which does not comply with these terms.
*Correspondence: Wei Zhang, wei.zhang1@northwestern.edu; Song-Mei Liu, smliu@whu.edu.cn; Yuanzhen Zhang, zhangyuanzhen@whu.edu.cn
†These authors have contributed equally to this work