Acid base and metabolic parameters of the umbilical cord blood and cerebral oxygenation immediately after birth
- 1Division of Neonatology, Department of Paediatrics and Adolescent Medicine, Medical University of Graz, Graz, Austria
- 2Research Unit for Neonatal Micro- and Macrocirculation, Department of Paediatrics and Adolescent Medicine, Medical University of Graz, Graz, Austria
Objective: Aim was to investigate whether acid-base and metabolic parameters obtained from arterial umbilical cord blood affect cerebral oxygenation after birth in preterm neonates with respiratory support and in term neonates without respiratory support.
Study design: This was a post-hoc analysis of secondary outcome parameters of a prospective observational study including preterm neonates with and term neonates without respiratory support. Non-asphyxiated neonates with cerebral oxygenation measured with near-infrared spectroscopy during the first 15 min and with blood gas analyses from arterial umbilical cord blood were included. Arterial oxygen saturation (SpO2) and heart rate (HR) were monitored with pulse oximetry. Potential correlations were investigated between acid-base and metabolic parameters (pH-value, bicarbonate, base-excess, and lactate) and crSO2/cFTOE 5 min after birth.
Results: Seventy-seven neonates were included: 14 preterm neonates with respiratory support (mean gestational age [GA] 31.4 ± 4.1 weeks; mean birth weight [BW] 1,690 ± 640 g) and 63 term neonates without respiratory support (GA 38.7 ± 0.8 weeks; BW 3,258 ± 443 g). Mean crSO2 5 min after birth was 44.0% ± 24.2% in preterm and 62.2% ± 20.01% in term neonates. Mean cFTOE 5 min after birth was 0.46 ± 0.06 in preterm and 0.27 ± 0.19 in term neonates. In preterm neonates with respiratory support higher lactate was significantly associated with lower crSO2 and SpO2 and tended to be associated with higher cFTOE. In term neonates without respiratory support no significant correlations were found.
Conclusion: In non-asphyxiated preterm neonates with respiratory support, lactate levels were negatively associated with crSO2 and SpO2, whereas in term neonates without respiratory support no associations were observed.
1 Introduction
The transition from fetus to newborn represents a highly complex physiological process (1). Initial clinical assessment of the newborn is routinely performed using the Apgar score introduced by Virginia Apgar in 1953 (2). Especially in preterm infants, monitoring by pulse oximeter and/or electrocardiogram is recommended to ensure continuous monitoring of heart rate (HR) and arterial oxygen saturation (SpO2) (3–5).
However, brain monitoring is not routinely used yet, even though the brain is the organ, which might be mostly affected by hypoxia. Brain function of the newborn immediately after birth is usually assessed just by clinical evaluation of muscle tone and reflexes (3). Monitoring systems such as Doppler ultrasonography and electroencephalography are of limited value due to their lack of feasibility during immediate neonatal transition (3, 6). Near-infrared spectroscopy (NIRS) represents a method with increasing importance to measure cerebral oxygenation. Based on different absorption spectra of oxygenated and deoxygenated hemoglobin regarding the emitted infrared light, relative changes of these parameters can be measured and cerebral regional oxygen saturation (crSO2) can be derived. Further, cerebral fractional tissue oxygen extraction (cFTOE) can be calculated using the equation (SpO2-crSO2)/SpO2 (7). This non-invasive continuous monitoring of crSO2 represents a mixed arterial, capillary, and particularly venous saturation and reflects the balance between oxygen supply and oxygen consumption of the brain (6, 8). Within the first minutes after birth, crSO2 has been shown to rise to a plateau earlier than peripheral arterial oxygen saturation in term infants, even if the data on this is not fully conclusive (8, 9). In addition, crSO2-guided therapy in extremely immature neonates results in a significant reduction in cerebral hyperoxia and hypoxia, although this is not associated with a significantly lower incidence of death and brain injury (8, 10, 11).
crSO2 depends on cerebral blood flow (CBF), which is a result of cerebral perfusion pressure (CPP) and cerebral vascular resistance (CVR), on cerebral oxygen consumption (cVO2) and on arterial oxygen content (CaO2), which mainly depends on arterial oxygen saturation and hemoglobin level (Hb). Several of these parameters are influenced by acid-base balance (pH, HCO3, BE and lactate) (12).
Acid-base parameters of arterial umbilical cord blood such as pH, HCO3, BE and lactate provide information about the metabolic status of neonates at birth. Severe metabolic acidosis—defined by a pH below 7.0 and a base deficit above 12 mmol/L—is associated with increased neonatal mortality and morbidity (13). Acid-base parameters obtained from venous umbilical cord blood show higher pH values than arterial umbilical cord blood due to the placental origin, whereas samples from heel capillary blood usually indicate lower pH values than arterial umbilical cord blood because of poor peripheral microcirculation (14–16).
The aim of the present study was to analyze the possible impact of acid-base status, measured from a blood sample of the umbilical artery at birth on cerebral oxygenation 5 min after birth in non-asphyxiated preterm neonates with respiratory support and in stable term neonates without respiratory support.
2 Material and methods
2.1 Design
This was a post-hoc-analysis of secondary outcome parameters of prospective observational studies conducted from October 2015 to September 2018 at the Division of Neonatology, Department of Pediatrics and Adolescent Medicine, Medical University of Graz, Austria. The Regional Ethics Committee approved the study and written parental consent was obtained before birth (EC number: 27–465 ex 14/15).
2.2 Inclusion and exclusion criteria
Preterm neonates with respiratory support and stable term neonates without respiratory support delivered by Cesarean section, who were included in the prospective observational study were eligible. Neonates with missing data of the monitoring parameters or acid-base and metabolic parameters were excluded. Neonates with major congenital malformations, and laboratory signs of asphyxia (umbilical artery pH <7.0, BE >12 mml/L or Lactate >2.9 mmol/L) were excluded.
2.3 Measurements during immediate transition
After delivery, the neonates were immediately taken to the resuscitation desk and placed supine under an overhead heater. Plastic wraps were used to prevent hypothermia in preterm neonates <29 weeks' gestation. Neonatal stabilization was performed according to neonatal resuscitation guidelines (4, 5) by specialized resuscitation teams (neonatologist/experienced resident and nurse) who were not involved in the study. Respiratory support was provided by continuous positive airway pressure or positive pressure ventilation using a T-piece device (Neopuff Infant Resuscitator, Fisher & Paykel Healthcare, Auckland, New Zealand). Oxygen levels were titrated according to neonatal resuscitation guidelines (4, 5). SpO2 and HR were monitored by a pulse oximetry probe (IntelliVue MP30 Monitor, Philips, Amsterdam, The Netherlands) placed around the right wrist/hand. CrSO2 was measured using an INVOS 5100 monitor (Covidien, Minnesota, USA) with a neonatal sensor fixed on the left forehead of each neonate. Averaging time of SpO2, HR and crSO2 was 8 s and values were stored every second. cFTOE was calculated with the following formula: (SpO2-crSO2)/SpO2. Cerebral oxygenation was monitored during the first 15 min after birth. Measurements were recorded by the multi-channel system alpha-trace digital MM (BEST Medical Systems, Vienna, Austria) for subsequent analyses. The acid-base and metabolic parameters from arterial umbilical cord blood were analysed with a blood gas analyser (ABL 800 Flex; Fa.Drott, Wiener Neustadt, Austria).
2.4 Statistical analysis
Demographic and clinical data are presented as mean ± standard deviation (SD) or median with interquartile range (IQR), as appropriate. Data of preterm neonates with respiratory support and term neonates without respiratory support were compared using t-test for independent samples, Mann-Whitney-U or Chi-square test as appropriate.
Mean of values of minute 5 after birth of SpO2, HR, crSO2 and cFTOE values were correlated to pH, bicarbonate (HCO3), base excess (BE) and lactate using Spearman's rank correlation coefficient or Pearson's correlation, as appropriate. This time-point was chosen to be close to the blood sample time-point. The analyses were performed in an explorative sense. Therefore, no correction for multiple testing was performed.
A p-value <0.05 was considered statistically significant. The statistical analyses were performed using IBM SPSS Statistics 27.0.0 (IBM Corporation, Armonk, NY, USA).
3 Results
Out of 224 eligible neonates 77 were included (Figure 1): 14 preterm neonates with respiratory support and 63 stable term neonates without respiratory support. Most neonates were excluded due to missing NIRS data at minute five or missing acid-base and metabolic parameters of umbilical artery. Demographic data of preterm and term neonates are presented in Table 1.
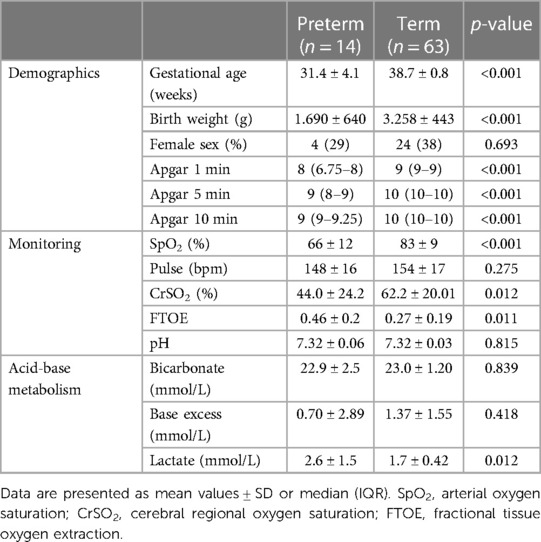
Table 1. Demographics, monitoring data 5 min after birth and acid base metabolism of the umbilical artery in preterm neonates with respiratory support and stable term neonates without respiratory support.
Concerning monitoring parameters (Table 1) preterm neonates receiving respiratory support showed significantly lower values of crSO2 and SpO2 and higher values in cFTOE than term neonates without respiratory support, whereas there was no statistically significant difference in HR. Besides lactate levels, which were statistically significantly higher in preterm neonates with respiratory support, no statistically significant differences were observed regarding pH, HCO3 or BE between the two groups (Table 1).
In preterm neonates with respiratory support lactate levels correlated significantly negatively with crSO2 and SpO2 5 min after birth (Table 2). In term neonates no correlations were observed.
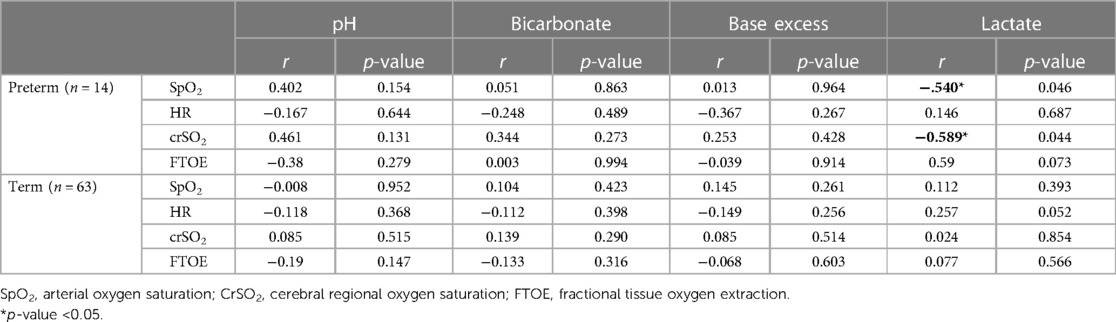
Table 2. Correlation analyses of SpO2, pulse, CrSO2 and FTOE measured 5 min after birth with umbilical artery pH, bicorbonate, base excess and lactate and in preterm neonates with respiratory support and stable term neonates without respiratory support.
4 Discussion and conclusion
In the present study we have demonstrated that crSO2 and SpO2 5 min after birth were associated with lactate levels in compromised preterm neonates with respiratory support, whereas in stable term neonates without respiratory support no associations were observed. In addition, lactate in preterm neonates also tended to be associated with cFTOE. Apart of lactate no further parameters of acid-base status were associated with SpO2, HR, crSO2 or cFTOE, neither in preterm neonates with respiratory support nor in stable term neonates.
SpO2 represents the percentage of hemoglobin saturated with oxygen in peripheral arterial blood (17). Our findings regarding SpO2 values in term neonates 5 min after birth are consistent with those of Rabi et al. (18), who described median SpO2 values of 81% (75–83).
Lactate is produced as a metabolite in anaerobic glycolysis and is elevated in case of impaired cellular oxygenation (19). The correlations in the present study were observed despite the fairly narrow lactate range within the study population with values <2.9 mmol/L. Similar findings with negative correlations between lactate levels obtained from capillary blood and crSO2 and with positive correlations between lactate levels and cFTOE in preterm neonates 15 min after birth were published by Mattersberger et al. (20) as well as by Janaillac et al. (21) in extremely preterm infants in the first 72 h after birth. The observed correlations could be explained by the fact that lactic acidemia induces pulmonary vasoconstriction and decreases cardiac stroke volume as a result of impaired cardiac contractility (22). Subsequently, low cardiac output leads to reduced oxygen supply of peripheral tissue and diminished peripheral oxygen saturation. The association between elevated lactate levels and poor hemodynamics has also been described in previous studies (23, 24). Considering lactate as a parameter of hemodynamics, the observed association between lactate levels and cerebral oxygenation may reflect impaired cerebrovascular autoregulation in preterm neonates. Intact cerebrovascular autoregulation allows constant CBF over a wide range of blood pressure, resulting in CBF independency of CPP. Impaired cerebrovascular autoregulation leads to a pressure-dependent CBF due to its linear correlation with CPP, resulting in deleterious CBF fluctuations in response to CPP variations (12, 25–28). Several studies have already indicated impaired cerebrovascular autoregulation in compromised preterm neonates (25–27, 29–33). Considering that in the present study cFTOE also tends to be associated with lactate in preterm neonates, low crSO2 may not only be a result of diminished oxygen content -in terms of low SpO2-, but also due to increased oxygen extraction in the cerebral microcirculation. In contrast, studies of infants diagnosed prenatally with congenital heart disease (34) and of critically ill neonates and of infants without cerebral damage (35) do not indicate associations between lactate levels and cerebral oxygenation. However, they showed negative correlations between cerebral oxygenation and SpO2. Since these studies included cerebral oxygenation measurements beyond the immediate fetal to neonatal transition and differentiation between preterm and term neonates was not considered, the differences in their findings could be result of a better cerebral autoregulation.
As far as other acid-base and metabolic parameters are concerned, these were slightly more alkaline compared to previous publications, which mostly included neonates born by vaginal delivery (19, 36). This is most probably due to the effect that neonates with laboratory signs of asphyxia were excluded, since we wanted to analyse the effect of blood gases and lactate in non-asphyxiated neonates. Furthermore, differences might be due to differences in the metabolic stress of the fetus caused by repeated uterine contractions during vaginal delivery (19) and Cesarean section.
Measurement of umbilical cord blood pH, HCO3 and BE provides essential information about acid-base metabolism and extrauterine adaption of newborns (13). In accordance to the present study no correlations between crSO2 and pH were found in children during cardiopulmonary bypass surgery (37), in infants diagnosed prenatally with congenital heart disease during the first 72 h (34) and in stable preterm neonates in neonatal intensive care (38). HCO3 is the physiologically most important buffer system in the human body (39). BE is used for quantification of changes in metabolic acid-base status and together with umbilical artery pH it is crucial to estimate the risk for newborn cerebral damage (40, 41). Mattersberger et al. (20) indicated positive correlations between HCO3 measured 15 min postnatally and cFTOE in term neonates and correlations of lower pH and BE also measured 15 min postnatally with lower crSO2 and higher cFTOE in preterm neonates. A point of innovation of the present study compared to Mattersberger et al. is the fact that by using umbilical cord blood the blood sampling is immediately after birth and might help to predict changes in the transition period and especially predict cerebral oxygenation in this vulnerable period (42). Furthermore capillary blood gas measurements do not always predict arterial blood gas values in an accurate way (13). In contrast, neither in stable preterm neonates in neonatal intensive care (38) nor in critically ill neonates and infants without cerebral damage (35) correlations were found between crSO2 and BE. Aldrich et al. (43) showed, that crSO2 is positively correlated with pH and negatively with BE shortly before birth and negative correlations between crSO2 and BE and HCO3, respectively were reported by Nissen et al. (44) in term-born infants with hypertrophic pyloric stenosis. A possible explanation of the differences to our findings are different study populations, comorbidities but also the fact, that in contrast to the present study, blood gas analysis were obtained from capillary blood. Samples obtained from arterial blood or umbilical cord blood tend to have more alkaline pH values than those of capillary blood (45). The present study was a post-hoc-analysis with all its inherent limitations. The small sample size of preterm neonates with respiratory support and possible interactions between the investigated acid-base and metabolic parameters and cerebral oxygenation mark further shortcomings. However, the described correlations in preterm neonates could be interpreted as an impaired cerebral autoregulation and are therefore an important finding.
In conclusion, in non-asphyxiated preterm neonates with respiratory support lactate levels were significantly associated with crSO2 and SpO2, whereas in stable term neonates without respiratory support no associations were observed.
Future studies will be necessary to evaluate causal relationships between acid-base and metabolic parameters and cerebral oxygenation during the period of transition from fetal to neonatal life.
Data availability statement
The raw data supporting the conclusions of this article will be made available by the authors, without undue reservation.
Ethics statement
The studies involving humans were approved by Regional Ethics Committee of the Medical University of Graz. The studies were conducted in accordance with the local legislation and institutional requirements. Written informed consent for participation in this study was provided by the participants’ legal guardians/next of kin.
Author contributions
MD: Writing – review & editing, Writing – original draft, Visualization, Validation, Methodology, Investigation, Formal Analysis, Data curation, Conceptualization. BU: Resources, Writing – review & editing, Validation, Methodology, Formal Analysis, Conceptualization. BS: Investigation, Data curation, Writing – review & editing, Validation, Formal Analysis. N-BS: Writing – review & editing, Validation, Investigation, Formal Analysis, Data curation. CS: Project administration, Writing – review & editing, Investigation, Data curation. CW: Validation, Formal Analysis, Writing – review & editing, Investigation, Data curation. GP: Writing – original draft, Visualization, Supervision, Software, Resources, Project administration, Methodology, Conceptualization, Writing – review & editing, Validation, Investigation, Formal Analysis, Data curation.
Funding
The author(s) declare that no financial support was received for the research, authorship, and/or publication of this article.
Conflict of interest
The authors declare that the research was conducted in the absence of any commercial or financial relationships that could be construed as a potential conflict of interest.
Publisher's note
All claims expressed in this article are solely those of the authors and do not necessarily represent those of their affiliated organizations, or those of the publisher, the editors and the reviewers. Any product that may be evaluated in this article, or claim that may be made by its manufacturer, is not guaranteed or endorsed by the publisher.
References
1. Rudolph AM. Fetal and neonatal pulmonary circulation. Annu Rev Physiol. (1979) 41:383–95. doi: 10.1146/annurev.ph.41.030179.002123
2. Apgar V. A proposal for a new method of evaluation of the newborn infant. Curr Res Anesth Analg. (1953) 32(4):260–7. doi: 10.1213/ANE.0b013e31829bdc5c
3. Pichler G, Schmölzer GM, Urlesberger B. Cerebral tissue oxygenation during immediate neonatal transition and resuscitation. Front Pediatr. (2017) 5:29. doi: 10.3389/fped.2017.00029
4. Wyllie J, Bruinenberg J, Roehr CC, Rüdiger M, Trevisanuto D, Urlesberger B. European resuscitation council guidelines for resuscitation 2015: section 7. Resuscitation and support of transition of babies at birth. Resuscitation. (2015) 95:249–63. doi: 10.1016/j.resuscitation.2015.07.029
5. Wyckoff MH, Aziz K, Escobedo MB, Kapadia VS, Kattwinkel J, Perlman JM. Part 13: neonatal resuscitation: 2015 American heart association guidelines update for cardiopulmonary resuscitation and emergency cardiovascular care. Circulation. (2015) 132(18 Suppl 2):S543–60. doi: 10.1161/CIR.0000000000000252
6. Pichler G, Cheung PY, Aziz K, Urlesberger B, Schmölzer GM. How to monitor the brain during immediate neonatal transition and resuscitation? A systematic qualitative review of the literature. Neonatology. (2014) 105(3):205–10. doi: 10.1159/000357162
7. Naulaers G, Meyns B, Miserez M, Leunens V, Van Huffel S, Casaer P. Use of tissue oxygenation index and fractional tissue oxygen extraction as non-invasive parameters for cerebral oxygenation. A validation study in piglets. Neonatology. (2007) 92(2):120–6. doi: 10.1159/000101063
8. Bruckner M, Pichler G, Urlesberger B. NIRS in the fetal to neonatal transition and immediate postnatal period. Semin Fetal Neonatal Med. (2020) 25(2):101079. doi: 10.1016/j.siny.2020.101079
9. Urlesberger B, Kratky E, Rehak T, Pocivalnik M, Avian A, Czihak J. Regional oxygen saturation of the brain during birth transition of term infants: comparison between elective cesarean and vaginal deliveries. J Pediatr. (2011) 159(3):404–8. doi: 10.1016/j.jpeds.2011.02.030
10. Hansen ML, Pellicer A, Hyttel-Sørensen S, Ergenekon E, Szczapa T, Hagmann C. Cerebral oximetry monitoring in extremely preterm infants. N Engl J Med. (2023) 388(16):1501–11. doi: 10.1056/NEJMoa2207554
11. Pichler G, Baumgartner S, Biermayr M, Dempsey E, Fuchs H, Goos TG. Cerebral regional tissue oxygen saturation to guide oxygen delivery in preterm neonates during immediate transition after birth (COSGOD III): an investigator-initiated, randomized, multi-center, multi-national, clinical trial on additional cerebral tissue oxygen saturation monitoring combined with defined treatment guidelines versus standard monitoring and treatment as usual in premature infants during immediate transition: study protocol for a randomized controlled trial. Trials. (2019) 20(1):178. doi: 10.1186/s13063-019-3258-y
12. Suppan E, Pichler G, Binder-Heschl C, Schwaberger B, Urlesberger B. Three physiological components that influence regional cerebral tissue oxygen saturation. Front Pediatr. (2022) 10:913223. doi: 10.3389/fped.2022.913223
13. Huch A, Huch R, Rooth G. Guidelines for blood sampling and measurement of pH and blood gas values in obstetrics. Eur J Obstet Gynecol Reprod Biol Mai. (1994) 54(3):165–75. doi: 10.1016/0028-2243(94)90277-1
14. Ferreira CS, Melo Â, Fachada AH, Solheiro H, Nogueira Martins N. Umbilical cord blood gas analysis, obstetric performance and perinatal outcome. Rev Bras Ginecol E Obstet Rev Fed Bras Soc Ginecol E Obstet. (2018) 40(12):740–8. doi: 10.1055/s-0038-1675187
15. Baik-Schneditz N, Schwaberger B, Urlesberger B, Wolfsberger CH, Bruckner M, Pichler G. Acid base and blood gas analysis in term neonates immediately after birth with uncomplicated neonatal transition. BMC Pediatr. (2022) 22(1):271. doi: 10.1186/s12887-022-03324-z
16. American College of Obstetricians and Gynecologists, American Academy of Pediatrics, Herausgeber. Neonatal Encephalopathy and Neurologic Outcome. 2nd Edn. Washington, DC: American College of Obstetricians and Gynecologists, Women’s Health Care Physicians, American Adademy of Pediatrics (2014). 236 S.
17. O’Driscoll BR, Howard LS, Earis J, Mak V. BTS guideline for oxygen use in adults in healthcare and emergency settings. Thorax. (2017) 72(Suppl 1):ii1–90. doi: 10.1136/thoraxjnl-2016-209729
18. Rabi Y, Yee W, Chen SY, Singhal N. Oxygen saturation trends immediately after birth. J Pediatr. (2006) 148(5):590–4. doi: 10.1016/j.jpeds.2005.12.047
19. Armstrong L, Stenson BJ. Use of umbilical cord blood gas analysis in the assessment of the newborn. Arch Dis Child Fetal Neonatal Ed. (2007) 92(6):F430–4. doi: 10.1136/adc.2006.099846
20. Mattersberger C, Baik-Schneditz N, Schwaberger B, Schmölzer GM, Mileder L, Urlesberger B. Acid-base and metabolic parameters and cerebral oxygenation during the immediate transition after birth-a two-center observational study. PLoS One. (2023) 18(5):e0283278. doi: 10.1371/journal.pone.0283278
21. Janaillac M, Beausoleil TP, Barrington KJ, Raboisson MJ, Karam O, Dehaes M. Correlations between near-infrared spectroscopy, perfusion index, and cardiac outputs in extremely preterm infants in the first 72 h of life. Eur J Pediatr. (2018) 177(4):541–50. doi: 10.1007/s00431-018-3096-z
22. Teplinsky K, O’Toole M, Olman M, Walley KR, Wood LD. Effect of lactic acidosis on canine hemodynamics and left ventricular function. Am J Physiol. (1990) 258(4 Pt 2):H1193–9. doi: 10.1152/ajpheart.1990.258.4.H1193
23. Phillips LA, Dewhurst CJ, Yoxall CW. The prognostic value of initial blood lactate concentration measurements in very low birthweight infants and their use in development of a new disease severity scoring system. Arch Dis Child Fetal Neonatal Ed. (2011) 96(4):F275–80. doi: 10.1136/adc.2010.185793
24. El-Khuffash A, McNamara PJ. Hemodynamic assessment and monitoring of premature infants. Clin Perinatol. (2017) 44(2):377–93. doi: 10.1016/j.clp.2017.02.001
25. Wolfsberger CH, Bruckner M, Schwaberger B, Mileder LP, Urlesberger B, Pichler G. Impact of carbon dioxide on cerebral oxygenation and vital parameters in stable preterm and term infants immediately after birth. Neonatology. (2022) 119(1):10–7. doi: 10.1159/000519636
26. Baik N, Urlesberger B, Schwaberger B, Avian A, Mileder L, Schmölzer GM. Blood pressure during the immediate neonatal transition: is the mean arterial blood pressure relevant for the cerebral regional oxygenation? Neonatology. (2017) 112(2):97–102. doi: 10.1159/000455965
27. Pfurtscheller D, Wolfsberger CH, Höller N, Schwaberger B, Mileder L, Baik-Schneditz N. Correlation between arterial blood pressures and regional cerebral oxygen saturation in preterm neonates during postnatal transition-an observational study. Front Pediatr. (2022) 10:952703. doi: 10.3389/fped.2022.952703
28. Harper AM. Autoregulation of cerebral blood flow: influence of the arterial blood pressure on the blood flow through the cerebral cortex. J Neurol Neurosurg Psychiatry. (1966) 29(5):398–403. doi: 10.1136/jnnp.29.5.398
29. Wong FY, Leung TS, Austin T, Wilkinson M, Meek JH, Wyatt JS. Impaired autoregulation in preterm infants identified by using spatially resolved spectroscopy. Pediatrics. (2008) 121(3):e604–11. doi: 10.1542/peds.2007-1487
30. Soul JS, Hammer PE, Tsuji M, Saul JP, Bassan H, Limperopoulos C. Fluctuating pressure-passivity is common in the cerebral circulation of sick premature infants. Pediatr Res. (2007) 61(4):467–73. doi: 10.1203/pdr.0b013e31803237f6
31. Seri I, Rudas G, Bors Z, Kanyicska B, Tulassay T. Effects of low-dose dopamine infusion on cardiovascular and renal functions, cerebral blood flow, and plasma catecholamine levels in sick preterm neonates. Pediatr Res. (1993) 34(6):742–9. doi: 10.1203/00006450-199312000-00009
32. Lou HC, Lassen NA, Friis-Hansen B. Impaired autoregulation of cerebral blood flow in the distressed newborn infant. J Pediatr. (1979) 94(1):118–21. doi: 10.1016/S0022-3476(79)80373-X
33. Schwaberger B, Pichler G, Binder C, Avian A, Pocivalnik M, Urlesberger B. Even mild respiratory distress alters tissue oxygenation significantly in preterm infants during neonatal transition. Physiol Meas. (2014) 35(10):2085–99. doi: 10.1088/0967-3334/35/10/2085
34. Mebius MJ, van der Laan ME, Verhagen EA, Roofthooft MT, Bos AF, Kooi EM. Cerebral oxygen saturation during the first 72 h after birth in infants diagnosed prenatally with congenital heart disease. Early Hum Dev. (2016) 103:199–203. doi: 10.1016/j.earlhumdev.2016.10.001
35. Weiss M, Dullenkopf A, Kolarova A, Schulz G, Frey B, Baenziger O. Near-infrared spectroscopic cerebral oxygenation reading in neonates and infants is associated with central venous oxygen saturation. Pediatr Anesth. (2005) 15(2):102–9. doi: 10.1111/j.1460-9592.2005.01404.x
36. Riley RJ, Johnson JW. Collecting and analyzing cord blood gases. Clin Obstet Gynecol. (1993) 36(1):13–23. doi: 10.1097/00003081-199303000-00005
37. Menke J, Möller G. Cerebral near-infrared spectroscopy correlates to vital parameters during cardiopulmonary bypass surgery in children. Pediatr Cardiol. (2014) 35(1):155–63. doi: 10.1007/s00246-013-0754-9
38. Hunter CL, Oei JL, Lui K, Schindler T. Cerebral oxygenation as measured by near-infrared spectroscopy in neonatal intensive care: correlation with arterial oxygenation. Acta Paediatr. (2017) 106(7):1073–8. doi: 10.1111/apa.13848
39. Hamm LL, Nakhoul N, Hering-Smith KS. Acid-base homeostasis. Clin J Am Soc Nephrol. (2015) 10(12):2232–42. doi: 10.2215/CJN.07400715
40. Berend K. Diagnostic use of base excess in acid-base disorders. N Engl J Med. (2018) 378(15):1419–28. doi: 10.1056/NEJMra1711860
41. Ouellet P, Racinet C, Daboval T. Umbilical artery base deficit/excess: sailing blindly in a thick fog. J Matern Fetal Neonatal Med. (2021) 34(23):3990–3. doi: 10.1080/14767058.2019.1685966
42. Aldrich CJ, D’Antona D, Wyatt JS, Spencer JA, Peebles DM, Reynolds EO. Fetal cerebral oxygenation measured by near-infrared spectroscopy shortly before birth and acid-base status at birth. Obstet Gynecol. (1994) 84(5):861–6. doi: 10.1016/0020-7292(95)90022-5
43. Nissen M, Cernaianu G, Thränhardt R, Vahdad MR, Barenberg K, Tröbs RB. Does metabolic alkalosis influence cerebral oxygenation in infantile hypertrophic pyloric stenosis? J Surg Res. (2017) 212:229–37. doi: 10.1016/j.jss.2017.01.019
44. Saili A, Dutta AK, Sarna MS. Reliability of capillary blood gas estimation in neonates. Indian Pediatr. (1992) 29(5):567–70.1500104
Keywords: neonates, acid-base and metabolic parameters, cerebral oxygenation, immediate neonatal transition, near-infrared spectroscopy
Citation: Dusleag M, Urlesberger B, Schwaberger B, Baik-Schneditz N, Schlatzer C, Wolfsberger CH and Pichler G (2024) Acid base and metabolic parameters of the umbilical cord blood and cerebral oxygenation immediately after birth. Front. Pediatr. 12:1385726. doi: 10.3389/fped.2024.1385726
Received: 13 February 2024; Accepted: 13 March 2024;
Published: 28 March 2024.
Edited by:
Karel Allegaert, KU Leuven, BelgiumReviewed by:
Claudio Pellegrino, Agostino Gemelli University Polyclinic (IRCCS), ItalyLuciana Teofili, Fondazione Policlincio Universitario A. Gemelli IRCCS, Italy
© 2024 Dusleag, Urlesberger, Schwaberger, Baik-Schneditz, Schlatzer, Wolfsberger and Pichler. This is an open-access article distributed under the terms of the Creative Commons Attribution License (CC BY). The use, distribution or reproduction in other forums is permitted, provided the original author(s) and the copyright owner(s) are credited and that the original publication in this journal is cited, in accordance with accepted academic practice. No use, distribution or reproduction is permitted which does not comply with these terms.
*Correspondence: Gerhard Pichler gerhard.pichler@medunigraz.at