Photobiology: introduction, overview and challenges
- Plant Molecular Biology (Botany), Faculty of Biology, Ludwig-Maximilians-Universität München, Planegg-Martinsried, Germany
Photobiology is a broad field of research that studies the biological effects of light and has made huge advances in understanding fundamental questions such as how Photosynthesis works and in the area of medicine, which has a direct impact on society. Various specialties of Photobiology have been proposed, and to simplify the overview, six major fields are considered here: Photophysics and Photochemistry, Photosynthesis, Photoreception, Photomedicine, Optogenetics and Optical Bioimaging, and Ecological Photobiology. In this review, an introduction, description and examples are given for each of these major research areas. Current challenges in Photobiology include the understanding of non-visual Photoreception, complex photoreceptive mechanisms and the development of novel therapeutic strategies that may depend on the results of advanced optogenetic approaches. Furthermore, the integration of Photobiology with other fields such as materials science and engineering may lead to artificial photoreceptors and bio-inspired light harvesting and artificial Photosynthesis systems. In an era of global change, understanding the effects of artificial light on organisms and ecosystems is essential to mitigate the ecological disruptions caused by artificial lighting. This non-exhaustive selection of research directions and challenges illustrates the complexity and breadth of Photobiology research.
Introduction
Photobiology is a multidisciplinary and diverse field of research that studies the biological effects of light, bringing together physicists, chemists, biologists, biochemists, medical specialists and many others. The term “light” is generally used to refer to wavelengths that are visible to humans, but invisible infrared and ultraviolet radiation are also included in Photobiology. In general, Photobiology is concerned with understanding the mechanisms by which living organisms interact with light and the biological consequences, whether beneficial or harmful (Smith, 1989).
Because the effects of light span the complexity of biological systems, from the atomic level to that of ecological communities (see Figure 1A), Photobiology encompasses a range of research areas. Smith (1989) lists 13 major areas of Photobiology, including Photophysics, Photochemistry, Photosensitization, UV Radiation Effects, Environmental Photobiology, Photomedicine, Circadian Rhythms, Extraretinal Photoreception, Vision, Photomorphogenesis, Photomovement, Photosynthesis, and Bioluminescence. The American Society for Photobiology (ASP) lists the 7 specialties Photochemistry/Photophysics, Photosensory Biology, Public Health, Phototechnology, Molecular and Cellular Photobiology, Photomedicine and Environmental Photobiology each with 7-8 subspecialties, adding up to 50 subspecialties of Photobiology (ASP News, 2012).
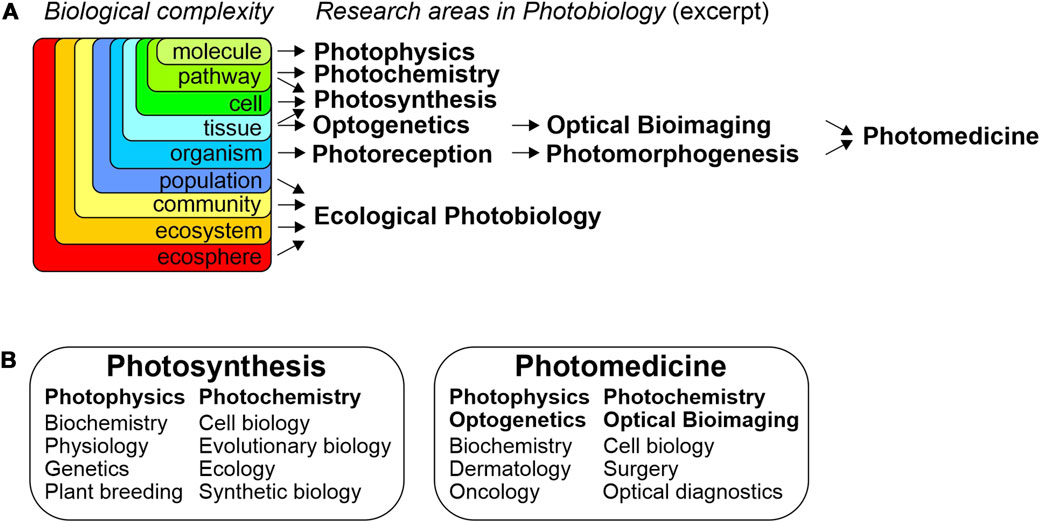
FIGURE 1. Complexity and breadth of Photobiology. (A) Photobiology: Research areas according to biological complexity. The six research areas described in the text relate to different levels of biological complexity. Some research areas, such as Photosynthesis and Ecological Photobiology, span several levels of complexity. (B) Interconnectedness of research areas in Photobiology. For two representative research areas, Photosynthesis and Photomedicine, some of the research disciplines contributing to them are shown to illustrate the complexity and interconnectedness of the six research areas of Photobiology defined in this study.
In this review, I define six broad research areas - Photophysics and Photochemistry, Photosynthesis, Photoreception, Photomedicine, Optogenetics and Optical Biomaging, and Ecological Photobiology - in such a way that they more or less cover most of the specialties defined by Smith and the ASP. Many of these research areas belong to different levels of biological complexity and are therefore interrelated and partly overlapping (see Figures 1A, B; Table 1). In the following, I will highlight the importance of these six research areas of Photobiology and give examples of current research and challenges. Furthermore, a glossary is provided (see Supplementary Table S1), which gives a definition of a number of important terms used in Photobiology.
The six research areas
Photophysics and Photochemistry
Photons do not carry enough energy per quantum to completely remove an electron from an atom or molecule and are therefore considered a form of non-ionising electromagnetic radiation. Therefore, when photons interact with atoms or molecules, they can absorb the photon energy and become excited (photophysical reaction) and react with surrounding molecules (photochemical reaction). Thus, Photophysics and Photochemistry dealing with biomolecules are research areas relevant to Photobiology (Balzani et al., 2014) (Table 1). Photophysical processes include the absorption and emission of light and the subsequent non-radiative transformations of the respective excited states (into each other). Photophysical processes involve changes in electronic states, but not changes in the chemical structure after light absorption, which are photochemical processes. In fact, in photochemical processes, light provides the activation energy for such chemical reactions, and some photochemical reactions are many times faster than thermal reactions. The photon can be absorbed directly by the reactant or indirectly by a Photosensitiver, which absorbs the photon and transfers the energy to the reactant. The opposite process is called Quenching, where a photoexcited state is deactivated by a chemical substance. Photophysics and Photochemistry have been essential in understanding the mechanisms by which light energy is converted into chemical energy during the light reactions of Photosynthesis (see section “Photosynthesis”). In fact, it is widely believed that the reactions of Photosynthesis begin with the absorption of a single photon and elegant photophysical and photochemical experiments have recently been able to support this notion (Li et al., 2023).
A special form of Photochemistry gives rise to Bioluminescence when living organisms generate and emit light (Lee, 2017). This fascinating process involves the interaction of a light-emitting molecule called “luciferin” with a specialised enzyme called “luciferase.” Under certain conditions, the luciferin molecule undergoes oxidation catalysed by the luciferase enzyme, resulting in the release of light. This light production is widespread in organisms and has many ecological functions (see section “Ecological Photobiology”).
Photosynthesis
The Encyclopedia Britannica defines Photosynthesis as “the process by which green plants and certain other organisms convert light energy into chemical energy; during photosynthesis in green plants, light energy is captured and used to convert water, carbon dioxide, and minerals into oxygen and energy-rich organic compounds” (Bassham et al., 2023). However, a broader definition is provided by Robert Blankenship: “Photosynthesis is a process in which light energy is captured and stored by an organism, and the stored energy is used to drive energy-requiring cellular processes” (Blankenship, 2014) (see Table 1). This definition also covers processes that convert light energy but do not produce oxygen as a by-product (non-oxygenic photosynthesis) and it clearly separates Photosynthesis as a metabolic process in which light serves as a substrate from Photoreception in which light conveys information about the environment (see section “Photoreception”). Photosynthesis uses visible light but can also operate at longer wavelengths, particularly in bacteria using bacteriochlorophyll. The biophysical and photochemical processes of Photosynthesis involve multi-protein-pigment complexes that are highly efficient at harvesting light, separating charges and coupling electron transport with proton gradient formation (Stirbet et al., 2020) (see Table 1). In linear electron flow using two Photosystems, electrons are supplied by water, which is oxidised to oxygen. Non-oxygenic Photosynthesis relies on cyclic electron flow around one photosystem, which does not produce oxygen but still generates a proton gradient. The protein gradient is used to produce ATP via the multi-protein complex ATP synthase, while the electrons released from water in the linear electron flow end up in NADPH. The biogenesis of the multi-protein complexes involved in Photosynthesis requires a large number of accessory proteins.
There is great interest in improving Photosynthesis, as it has an overall efficiency of only a few percent and is thought to have been insufficiently optimised in previous breeding approaches (Long et al., 2015; Garcia et al., 2023; Leister, 2023). However, a full understanding of photosynthesis must cover several levels of biological complexity, making approaches to improving the process a formidable challenge (Figures 1A, B).
Photoreception
Light can be detected by a receptor in an organism to monitor the environment. This can provide information about the environment, which is necessary for physiological responses. Photomorphogenesis refers specifically to the light-induced changes in growth and development, particularly in plants, e.g., during seedling development (Von Arnim and Deng, 1996) (see Table 1), whereas the Circadian Clock is a broader internal timing system found in most organisms that regulates many biological processes, including those related to light responses. While the Circadian Clock is an internal timing system that regulates rhythmic processes in organisms (Lane et al., 2023), Photoperiodism is a specific aspect of plant physiology that involves the ability to sense and respond to changes in day length, particularly with respect to flowering and growth patterns. Photomovement allows whole organisms to adjust their position or orientation to optimise their interactions with the environment in terms of maximising their exposure to light or avoiding unfavourable light conditions. Some examples of Photomovement include: phototropism, phototaxis, photokinesis, and photophobic responses. Organelles such as chloroplasts can also exhibit Photomovement (Labuz et al., 2022; Laosuntisuk et al., 2023) (see Table 1).
Vision refers to the sensory ability of an organism to detect and interpret light stimuli for the purpose of forming visual perceptions. It is the process by which organisms, primarily animals, use specialised photoreceptor cells in their eyes to capture and process light information from the environment. Traditionally, Photoreception in mammals has been associated with Vision, but it has become clear that light-sensitive proteins are also present in non-visual tissues and organs (Gehring, 2014). For example, melanopsin is involved in several physiological processes beyond Vision, such as the regulation of circadian rhythms, sleep-wake cycles and mood (Duda et al., 2020; Lucas et al., 2020). In addition, certain organisms such as zebrafish can regenerate damaged photoreceptor cells, prompting strategies to transfer these mechanisms to humans with the aim of treating degenerative retinal diseases (Angueyra and Kindt, 2018). Optogenetics, which uses light-sensitive proteins called opsins can be seen as a spin-off of Photoreception research and has gained significant importance as an approach that allows precise control of neural activity and other cellular processes (see section: “Optogenetics and Optical Bioimaging”).
Photomedicine
The advantage of using light in medicine is that it is less invasive, directional and can penetrate tissue, whereas too much light can be harmful and its adverse effects need to be treated. Photomedicine is an interdisciplinary branch of medicine that involves the study and application of light in relation to health and disease (Regan and Parrish, 1982; Hamblin and Huang, 2013) (see Table 1). Photomedicine can be related to the practice of several medical specialties, including dermatology, surgery, interventional radiology, optical diagnostics, cardiology, circadian rhythm sleep disorders, and oncology (Figure 1B). In fact, melanin pigments protect the body from harmful UV radiation (Ohbayashi and Fukuda, 2020), but too much UV radiation is the main cause of photocarcinogenesis, which contributes to skin cancer (Valejo Coelho et al., 2016; Martens et al., 2018) (see Table 1). Light therapy, also known as phototherapy or bright light therapy, is the use of direct sunlight or artificial light of controlled wavelengths to treat a variety of medical conditions, including seasonal affective disorder, circadian rhythm sleep/wake disorders, cancer, and skin wound infections. Ultraviolet light therapy or ultraviolet phototherapy is a treatment for psoriasis, atopic dermatitis, vitiligo, and other skin conditions using UV-B light. Photodynamic therapy (PDT) uses light and a Photosensitiser (see section “Photophysics and Photochemistry”) in conjunction with molecular oxygen to induce cell death, particularly of cancer cells. In PDT, a photosensitising agent is administered to the patient and allowed to accumulate in the target tissue. The area is then irradiated with light of a specific wavelength, which activates the Photosensitiser and leads to the destruction of the (cancer) cells. PDT has been shown to be effective in treating several types of cancer, including skin, lung and prostate cancer, and it is also used to treat acne, macular degeneration, psoriasis, atherosclerosis and, as an antimicrobial PDT, herpes. Photobiomodulation (PBM), or low-level laser therapy, has been promoted for use in the treatment of several conditions or disorders and has been discussed controversially. Light is also used in medicine as a tool to cut tissue in a targeted way (e.g., laser surgery) or for optical diagnostics (e.g., optical probes, fluorescence imaging, spectroscopy, etc.) (see section: “Optogenetics and Optical Bioimaging”). In addition, optogenetic approaches can be used to restore visual function in blind patients (Sahel et al., 2021) (see sections “Photoreception” and “Optogenetics and Optical Bioimaging”)
Optogenetics and Optical Bioimaging
A recent development in biomedical research is the use of light to control biological systems. This approach, based on Photoreception, is known as Optogenetics and involves the use of genetically modified organisms that can be controlled by light. In Optogenetics, genes encoding light-sensitive proteins are introduced into cells, allowing the cells to be controlled by light. This technique has been used to study a range of biological processes, including neural signalling and gene expression (Fernandez-Ruiz et al., 2022; Lan et al., 2022) (see Table 1). In particular, Optogenetics allows the precise manipulation and study of neuronal function with high temporal and spatial resolution in living tissue by genetically engineering light-sensitive proteins called opsins into target neurons. These opsins, such as channelrhodopsin and halorhodopsin, can be activated or inhibited by specific wavelengths of light. Once the opsins are expressed in the neurons of interest, fibre-optic or other methods are used to deliver light to the target area. By controlling the timing and intensity of the light stimulation, neurons can be activated or inhibited, thereby influencing neural circuitry and allowing to the resulting effects on behaviour or brain activity to be observed.
Bioimaging refers to the visualisation and analysis of biological structures and processes at various scales, from the cellular to the whole organism level (Wang and Jiang, 2022) (see Table 1). It involves the use of various imaging techniques and technologies to capture detailed information about biological systems, enabling the study of their structure, function and dynamics. Bioimaging techniques can be broadly categorised into two main types: in vivo imaging and ex vivo imaging. In vivo imaging involves the imaging of biological processes within a living organism, from (sub)cellular structures, cells to tissues, while ex vivo imaging involves the imaging of biological samples that have been removed from the organism. Various imaging modalities are used in bioimaging, of which those using light or fluorescence (e.g., confocal microscopy, two-photon microscopy, and fluorescence imaging) are related to Photobiology. Photosynthesis can be measured non-invasively and with spatial resolution based on chlorophyll fluorescence (Oxborough, 2004; Schansker et al., 2014) (see Table 1).
Ecological Photobiology
While Photoreception and Photosynthesis are research areas that can be subsumed under the broader term “Environmental Photobiology,” Ecological Photobiology focuses on the ecological interactions between light and living organisms; i.e., the effects of light on the behaviour, physiology and ecology of organisms. In fact, light serves as a crucial signal for organisms, providing information about resource availability, habitat quality, and the presence of potential mates or predators. Ecological Photobiology therefore studies how organisms perceive and respond to specific light cues, and how these responses shape their distribution, abundance, and interactions within ecosystems. This includes studying how light affects predator-prey dynamics, species interactions and the structure of ecological communities.
Two aspects of Photobiology mentioned above, Bioluminescence (see section “Photophysics and Photochemistry”) and human-induced light modification (see section “Photomedicine”), also play a role in Ecological Photobiology. In the marine environment, Bioluminecscence plays a crucial role in communication, defence and predation. Many marine organisms, such as certain species of jellyfish and fish, use Bioluminescence to attract mates, intimidate rivals or deceive predators. In addition, some deep-sea organisms use bioluminescence to create intricate patterns that camouflage their silhouette or confuse predators, effectively blending into the abyssal background. Bioluminescence is also common in other ecosystems. Some fungi, for example, emit a faint glow, often found in decaying wood or forest floors, which can help attract insects to aid the dispersal of spores or possibly deter competing organisms. Terrestrial arthropods, particularly fireflies, are well known for their bioluminescent displays. Fireflies use their light-producing abilities to communicate with potential mates, with each species displaying unique flashing patterns to attract their specific partners. Man-made changes to light, such as Light Pollution and changes to natural light regimes, also have an impact on ecology. Artificial light at night affects the behaviour, physiology and ecological interactions of organisms, including effects on circadian rhythms, navigation, reproduction and species interactions (Walker et al., 2020; Marangoni et al., 2022; Burt et al., 2023). Artificial lighting also has an impact on human health (see section “Photomedicine”). Artificial lighting can disrupt circadian rhythms with negative effects on sleep and metabolism. Studies have linked exposure to artificial light at night to a range of health problems, including sleep disturbance, metabolic disorders and an increased risk of certain cancers.
At the population level, an example of Ecological Photobiology is the diurnal vertical migration (DVM) of marine zooplankton (Enright and Hamner, 1967; Stich and Lampert, 1984) (see Table 1). DVM refers to the daily movement of zooplankton species from deep, dark waters during the day to the surface waters at night. During the day, sunlight penetrates the surface waters and creates a high-intensity light environment near the ocean surface. This intense light is often associated with an increased risk of predation for zooplankton due to the presence of visual predators such as fish. As a result, many zooplankton species have evolved a behavioural strategy to avoid predation by migrating to deeper, darker waters during the day. At night, the intensity of light near the ocean surface decreases, creating a more favourable environment for zooplankton. The reduced light reduces the risk of predation and allows zooplankton to exploit food resources such as phytoplankton, which are abundant near the surface due to their photosynthetic activity during the day.
An example of Ecological Photobiology at the community level is the phenomenon of light competition among plants in a forest canopy (see Table 1). In densely populated forest ecosystems, the availability of sunlight and the spectral composition of light vary according to the structure and density of the canopy (Keuskamp et al., 2010; Ford, 2014). Under-canopy plants, which receive limited direct sunlight due to canopy shading, must compete for available light resources. Different plant species have evolved different strategies to optimise light capture and use, resulting in different patterns of species composition and abundance within the community. One common strategy is shade tolerance, where certain plant species are adapted to low light conditions and can efficiently use the limited light available in the understory. On the other hand, some species are shade intolerant and require higher light levels for growth and reproduction. These species are often found in canopy gaps or areas of higher light penetration, where they can outcompete shade-tolerant plants due to their ability to capture and utilise more intense sunlight.
An example of Ecological Photobiology at the ecosystem level is the role of light availability in coral reef ecosystems (Roth, 2014) (see Table 1). Coral reefs are highly diverse and productive marine ecosystems that rely on the symbiotic relationship between corals and photosynthetic algae. The photosynthetic algae live in the tissues of coral polyps and provide them with essential nutrients through Photosynthesis, while the corals provide shelter and nutrients to the algae. This mutualistic relationship is highly dependent on the availability of light for Photosynthesis, and therefore, the intensity, duration and spectral quality of light directly affect the productivity and growth of both the coral and the algae. However, excessive or prolonged exposure to high-intensity light, as well as elevated temperatures, can stress corals and lead to coral bleaching. Under such stressful conditions, corals expel their algae, resulting in the loss of their main energy source and a bleached appearance (Blackstone and Golladay, 2018). If the stress persists, the corals may die, leading to a decline in the entire ecosystem.
Ecological Photobiology at the ecosphere level refers to the study of how light influences and interacts with the entire Earth’s biosphere, including the interconnectedness of different ecosystems and global biogeochemical cycles (Regnier et al., 2022). Understanding the interactions between solar radiation, primary productivity and carbon cycling at the ecosphere scale is crucial for studying global climate patterns, carbon sequestration, and the overall functioning and resilience of Earth’s ecosystems (Long and Hutchin, 1991).
Discussion and concluding remarks
The above areas of research show that Photobiology is thriving. Current challenges in Photobiology are to understand non-visual photoreception in mammals and complex photoreceptive mechanisms, particularly in the context of comparing mechanisms in different organisms and the interplay between different photoreceptor systems. In Photomedicine, the development of novel therapeutic strategies may depend on the results of advanced Optogenetic approaches with improved safety and efficacy. Furthermore, the integration of Optogenetics with Photomedicine and Photosynthesis with materials science and engineering may lead to artificial photoreceptors and vision, as well as to bio-inspired light harvesting and artificial Photosynthesis systems. Understanding the effects of (artificial) light on organisms and ecosystems is essential to mitigate the ecological disruptions caused by artificial lighting and will contribute to the containment of global change. These are just a few examples of the many other challenges in Photobiology that illustrate the complexity, breadth and timeliness of research in this field. Addressing them requires multidisciplinary collaboration, technological advances and a deeper understanding of the fundamental processes underlying light-organism interactions.
Data availability statement
The original contributions presented in the study are included in the article/Supplementary Material, further inquiries can be directed to the corresponding author.
Author contributions
DL: Conceptualization, Writing–original draft, Writing–review and editing.
Funding
The work in the authors’ laboratory is supported by the ERC (Synergy project Photoredesign project number 854126) and the Deutsche Forschungsgemeinschaft (CRC Transregio 175).
Conflict of interest
The author declares that the research was conducted in the absence of any commercial or financial relationships that could be construed as a potential conflict of interest.
The author(s) DL declared that they were an editorial board member of Frontiers, at the time of submission. This had no impact on the peer review process and the final decision.
Publisher’s note
All claims expressed in this article are solely those of the authors and do not necessarily represent those of their affiliated organizations, or those of the publisher, the editors and the reviewers. Any product that may be evaluated in this article, or claim that may be made by its manufacturer, is not guaranteed or endorsed by the publisher.
Supplementary material
The Supplementary Material for this article can be found online at: https://www.frontiersin.org/articles/10.3389/fphbi.2023.1253330/full#supplementary-material
References
Angueyra, J. M., and Kindt, K. S. (2018). Leveraging zebrafish to study retinal degenerations. Front. Cell Dev. Biol. 6, 110. doi:10.3389/fcell.2018.00110
Balzani, V., Ceroni, P., and Juris, A. (2014). Photochemistry and Photophysics: Concepts, research, applications. Germany: Wiley VCH.
Bassham, J. A., Lambers, H., and Britannica, T. e. o. E. (2023). Photosynthesis. Available: https://www.britannica.com/science/photosynthesis (Accessed June 24, 2023).
Blackstone, N. W., and Golladay, J. M. (2018). Why do corals bleach? Conflict and conflict mediation in a host/symbiont community. Bioessays 40 (8), e1800021. doi:10.1002/bies.201800021
Blankenship, R. E. (2014). Molecular mechanisms of photosynthesis. Second Edition. New Jersey: JohnWiley & Sons, Ltd.
Burt, C. S., Kelly, J. F., Trankina, G. E., Silva, C. L., Khalighifar, A., Jenkins-Smith, H. C., et al. (2023). The effects of light pollution on migratory animal behavior. Trends Ecol. Evol. 38 (4), 355–368. doi:10.1016/j.tree.2022.12.006
Duda, M., Domagalik, A., Orlowska-Feuer, P., Krzysztynska-Kuleta, O., Beldzik, E., Smyk, M. K., et al. (2020). Melanopsin: from a small molecule to brain functions. Neurosci. Biobehav Rev. 113, 190–203. doi:10.1016/j.neubiorev.2020.03.012
Enright, J. T., and Hamner, W. M. (1967). Vertical diurnal migration and endogenous rhythmicity. Science 157 (3791), 937–941. doi:10.1126/science.157.3791.937
Fernandez-Ruiz, A., Oliva, A., and Chang, H. (2022). High-resolution optogenetics in space and time. Trends Neurosci. 45 (11), 854–864. doi:10.1016/j.tins.2022.09.002
Ford, E. D. (2014). The dynamic relationship between plant architecture and competition. Front. Plant Sci. 5, 275. doi:10.3389/fpls.2014.00275
Garcia, A., Gaju, O., Bowerman, A. F., Buck, S. A., Evans, J. R., Furbank, R. T., et al. (2023). Enhancing crop yields through improvements in the efficiency of photosynthesis and respiration. New Phytol. 237 (1), 60–77. doi:10.1111/nph.18545
Gehring, W. J. (2014). The evolution of vision. Wiley Interdiscip. Rev. Dev. Biol. 3 (1), 1–40. doi:10.1002/wdev.96
Keuskamp, D. H., Sasidharan, R., and Pierik, R. (2010). Physiological regulation and functional significance of shade avoidance responses to neighbors. Plant Signal Behav. 5 (6), 655–662. doi:10.4161/psb.5.6.11401
Labuz, J., Sztatelman, O., and Hermanowicz, P. (2022). Molecular insights into the phototropin control of chloroplast movements. J. Exp. Bot. 73 (18), 6034–6051. doi:10.1093/jxb/erac271
Lan, T. H., He, L., Huang, Y., and Zhou, Y. (2022). Optogenetics for transcriptional programming and genetic engineering. Trends Genet. 38 (12), 1253–1270. doi:10.1016/j.tig.2022.05.014
Lane, J. M., Qian, J., Mignot, E., Redline, S., Scheer, F., and Saxena, R. (2023). Genetics of circadian rhythms and sleep in human health and disease. Nat. Rev. Genet. 24 (1), 4–20. doi:10.1038/s41576-022-00519-z
Laosuntisuk, K., Elorriaga, E., and Doherty, C. J. (2023). The game of timing: circadian rhythms intersect with changing environments. Annu. Rev. Plant Biol. 74, 511–538. doi:10.1146/annurev-arplant-070522-065329
Lee, J. (2017). Perspectives on bioluminescence mechanisms. Photochem Photobiol. 93 (2), 389–404. doi:10.1111/php.12650
Leister, D. (2023). Enhancing the light reactions of photosynthesis: strategies, controversies, and perspectives. Mol. Plant 16 (1), 4–22. doi:10.1016/j.molp.2022.08.005
Li, Q., Orcutt, K., Cook, R. L., Sabines-Chesterking, J., Tong, A. L., Schlau-Cohen, G. S., et al. (2023). Single-photon absorption and emission from a natural photosynthetic complex. Nature 619, 300–304. doi:10.1038/s41586-023-06121-5
Long, S. P., and Hutchin, P. R. (1991). Primary production in grasslands and coniferous forests with climate change: an Overview. Ecol. Appl. 1 (2), 139–156. doi:10.2307/1941807
Long, S. P., Marshall-Colon, A., and Zhu, X. G. (2015). Meeting the global food demand of the future by engineering crop photosynthesis and yield potential. Cell 161 (1), 56–66. doi:10.1016/j.cell.2015.03.019
Lucas, R. J., Allen, A. E., Milosavljevic, N., Storchi, R., and Woelders, T. (2020). Can we see with melanopsin? Annu. Rev. Vis. Sci. 6, 453–468. doi:10.1146/annurev-vision-030320-041239
Marangoni, L. F. B., Davies, T., Smyth, T., Rodriguez, A., Hamann, M., Duarte, C., et al. (2022). Impacts of artificial light at night in marine ecosystems-A review. Glob. Chang. Biol. 28 (18), 5346–5367. doi:10.1111/gcb.16264
Martens, M. C., Seebode, C., Lehmann, J., and Emmert, S. (2018). Photocarcinogenesis and skin cancer prevention strategies: an update. Anticancer Res. 38 (2), 1153–1158. doi:10.21873/anticanres.12334
Ohbayashi, N., and Fukuda, M. (2020). Recent advances in understanding the molecular basis of melanogenesis in melanocytes. F1000Res 9, F1000 Faculty Rev-608. doi:10.12688/f1000research.24625.1
Oxborough, K. (2004). Imaging of chlorophyll a fluorescence: theoretical and practical aspects of an emerging technique for the monitoring of photosynthetic performance. J. Exp. Bot. 55 (400), 1195–1205. doi:10.1093/jxb/erh145
Regnier, P., Resplandy, L., Najjar, R. G., and Ciais, P. (2022). The land-to-ocean loops of the global carbon cycle. Nature 603 (7901), 401–410. doi:10.1038/s41586-021-04339-9
Roth, M. S. (2014). The engine of the reef: photobiology of the coral-algal symbiosis. Front. Microbiol. 5, 422. doi:10.3389/fmicb.2014.00422
Sahel, J. A., Boulanger-Scemama, E., Pagot, C., Arleo, A., Galluppi, F., Martel, J. N., et al. (2021). Partial recovery of visual function in a blind patient after optogenetic therapy. Nat. Med. 27 (7), 1223–1229. doi:10.1038/s41591-021-01351-4
Schansker, G., Toth, S. Z., Holzwarth, A. R., and Garab, G. (2014). Chlorophyll a fluorescence: beyond the limits of the QA model. Photosynth Res. 120 (1-2), 43–58. doi:10.1007/s11120-013-9806-5
Stich, H. B., and Lampert, W. (1984). Growth and reproduction of migrating and non-migrating Daphnia species under simulated food and temperature conditions of diurnal vertical migration. Oecologia 61 (2), 192–196. doi:10.1007/BF00396759
Stirbet, A., Lazar, D., Guo, Y., and Govindjee, G. (2020). Photosynthesis: basics, history and modelling. Ann. Bot. 126 (4), 511–537. doi:10.1093/aob/mcz171
Valejo Coelho, M. M., Matos, T. R., and Apetato, M. (2016). The dark side of the light: mechanisms of photocarcinogenesis. Clin. Dermatol 34 (5), 563–570. doi:10.1016/j.clindermatol.2016.05.022
Von Arnim, A., and Deng, X. W. (1996). Light control of seedling development. Annu. Rev. Plant Physiol. Plant Mol. Biol. 47, 215–243. doi:10.1146/annurev.arplant.47.1.215
Walker, W. H., Bumgarner, J. R., Walton, J. C., Liu, J. A., Melendez-Fernandez, O. H., Nelson, R. J., et al. (2020). Light pollution and cancer. Int. J. Mol. Sci. 21 (24), 9360. doi:10.3390/ijms21249360
Keywords: ecological photobiology, optogenetics, photobiology, photochemistry, photomedicine, photophysics, photoreception, optical bioimaging
Citation: Leister D (2023) Photobiology: introduction, overview and challenges. Front. Photobiol. 1:1253330. doi: 10.3389/fphbi.2023.1253330
Received: 05 July 2023; Accepted: 10 August 2023;
Published: 20 September 2023.
Edited by:
Stuart Sullivan, University of Glasgow, United KingdomReviewed by:
Maria Agustina Dominguez-Martin, University of Cordoba, SpainJeong-Il Kim, Chonnam National University, Republic of Korea
Copyright © 2023 Leister. This is an open-access article distributed under the terms of the Creative Commons Attribution License (CC BY). The use, distribution or reproduction in other forums is permitted, provided the original author(s) and the copyright owner(s) are credited and that the original publication in this journal is cited, in accordance with accepted academic practice. No use, distribution or reproduction is permitted which does not comply with these terms.
*Correspondence: Dario Leister, leister@lmu.de