Frontiers in photonics spotlight
- 1Department of Bioengineering, University of California, Riverside, Riverside, CA, United States
- 2Department of Physics, Politecnico di Milano, Milan, Italy
- 3Department of Information Engineering, University of Brescia, Brescia, Italy
- 4Sustainable Energy Research Centre, School of Engineering, Macquarie University, Sydney, NSW, Australia
- 5Bradley Department of Electrical and Computer Engineering, Virginia Tech, Blacksburg, VA, United States
- 6Institute of Microstructure Technology, Karlsruhe Institute of Technology (KIT), Karlsruhe, Germany
- 7Light Technology Institute, Karlsruhe Institute of Technology, Karlsruhe, Germany
- 8Emergent Photonics Research Centre, Department of Physics, Loughborough University, Loughborough, United Kingdom
Highlighting remarkable research output is an important mission of journals and scientists engaged in dissemination. With this spotlight-review we would like to provide visibility to some of the best recent research outputs and stress the pivotal role of their authors in the Photonics field.
1 Introduction
Frontiers in Photonics recognizes that the advancement of the field is the main focus of research, and that progress is made with results that address key scientific questions and important problems.
However, a key challenge in recognizing the excellence of specific research output is finding consensus on what constitutes an outstanding piece of research. While such consensus may develop around specific, well-disseminated outputs, this may take a significant amount of time after publication and may be influenced by the perceived importance of the research topic, the specific publication outlet, and even the reputation of the authors.
Despite the difficulties in constructing an objective view—especially in Photonics, with its very large set of different methodologies and frequent cross-pollination with other domains—it is still pivotal to acknowledge interesting work, promoting and showcasing researchers who are making transformative contributions to their fields. In addition, a spotlight paper can help current decision-makers by reporting key findings and contributing to the shaping of future research, helping to identify common trends and themes, promoting collaboration, and facilitating the exchange of scientific ideas.
In this review, while in biophotonics, Wu et al. demonstrate the use of near-infrared light to stimulate deep-brain neurons developing an optogenetics approach without resorting to brain implants, in plasmonics a team of researchers led by Prof. Andrea Baldi at VU Amsterdam exploited particle swarm optimization to create a plasmonic platform for hydrogen detection. In non-linear optics, McDonnell et al. demonstrated THz emitters based on Pancharatnam-Berry phase non-linear metasurfaces, while in Photovoltaic Materials, D. Kim and colleagues have developed a low-cost solution-processed flexible multi-layer encapsulation technique for perovskite solar cells. In Optical Information Processing and Holography, A. Bulbul et al. have investigated the benefits of a variant of COACH (coded-aperture correlation holography), and lastly, the two spotlighted works from T.P. van Swieten et al. and M. Quintanilla et al. focus on the challenges of using luminescence thermometry in real-world settings.
2 Spotlight in biophotonics
X. Wu, Y. Jiang, N. J. Rommelfanger, F. Yang, Q. Zhou, R. Yin, J. Liu, S. Cai, W. Ren, A. Shin, K. S. Ong, K. Pu, and G. Hong, “Tether-free photothermal deep-brain stimulation in freely behaving mice via wide-field illumination in the near-infrared-II window,” Nat. Biomed. Eng. 6, 754–770 (2022). (Wu et al., 2022).
Biophotonics is the scientific discipline at the interface of light with life and natural sciences. Broadly, it encompasses photons for fundamental studies in biological, physical, and chemical science, and utilizes the knowledge towards development of light-based applications in broad fields ranging from biology, pharmaceutics and medicine, to environmental, agricultural and food sciences. For example, optogenetics has emerged as a sub-area in biophotonics for manipulating neural activities in the brain of live animals with high spatiotemporal precision and neuron-type specificity (Fenno et al., 2011). Conventional optogenetics predominantly uses opsins that respond to short-wavelength visible light, which suffers from limited penetration depth in biological tissues due to the strong scattering and absorption of visible photons therein (Hong et al., 2017; Jiang et al., 2022). As a result, invasive implantation of optical fibers is usually required for deep-brain optogenetics, inevitably leading to permanent tissue damage and chronic gliosis (Salatino et al., 2017; Chen et al., 2021a). Furthermore, the physical tethering of the animal to an external light source via the optical fiber interferes with the subject’s natural behaviors and confounds the results of behavioral experiments (Kim et al., 2013). Despite the emerging neuromodulation techniques to mitigate these issues (Kim et al., 2013; Chen et al., 2015; Wu et al., 2019; Chen et al., 2021b), it remains challenging to achieve remote deep-brain neuromodulation without any brain implants or head tethering via an optical interface.
To address these challenges, Xiang Wu, Guosong Hong and coworkers at Stanford University recently developed a remote and implant-free neuromodulation technique by leveraging tissue-penetrant light in the second near-infrared window (NIR-II, 1,000–1700 nm, Figure 1A) (Wu et al., 2022). Specifically, they first evaluated the penetration depth of light in the optical regime by plotting the effective attenuation coefficient of brain tissue in the 400–1800 nm spectral range. As shown in Figure 1B, 1,064 nm light, a NIR-II wavelength widely available by Nd:YAG lasers, sits near the global minimal of the entire visible-to-NIR spectrum, thus representing the optimal wavelength for deep-brain neuromodulation. However, no opsins have heretofore been reported to respond to widefield 1064-nm illumination, likely due to the low energy of 1064-nm photon at this wavelength. Instead, the researchers sought inspiration from Nature and found that rattlesnakes can sense infrared irradiation (750 nm–1 mm) via their transient receptor potential (TRP) channels (Gracheva et al., 2010). Although TRP channels coupled with 915-nm absorbing gold nanorods have imparted NIR sensitivity to the mouse retina (Nelidova et al., 2020), direct activation of TRP-expressing neurons in the deep brain may lead to undesired heating in the superficial brain, resulting in altered cortical neural activity and even thermal damage. To mitigate this obstacle, the researchers designed polymeric NIR-II nanotransducers coined MINDS (macromolecular infrared nanotransducers for deep-brain stimulation), which strongly absorb 1064-nm light and efficiently convert it into heat (Figures 1C, D). After intracranial delivery, MINDS created a local hot spot up to a depth of 5-mm upon wide-field through-scalp NIR-II illumination, while only minimal heating was observed at the brain surface (Figure 1E). As a result, MINDS successfully sensitized deep-brain TRP-expressing neurons to low intensity NIR-II illumination within the safe exposure limit of 1064-nm light.
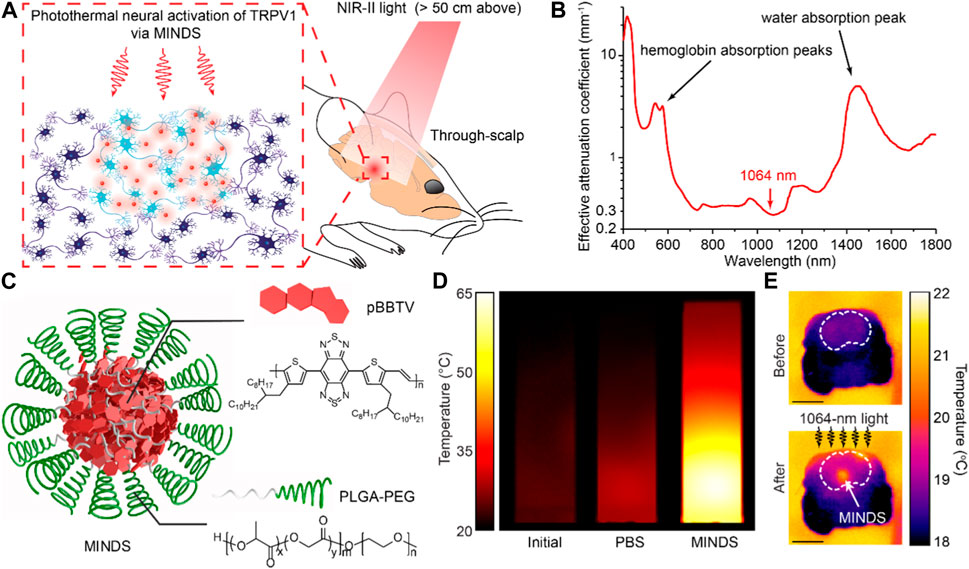
FIGURE 1. (A) Schematic showing the mechanism of NIR-II neuromodulation. (B) Spectrum of the effective attenuation coefficient of brain tissue. (C) Schematic showing the structure of MINDS. (D) Thermal images of MINDS solution before (left) and after (right) NIR-II illumination, as compared with PBS (middle). (E) Cross-sectional thermal images of mouse brain before (top) and after (bottom) NIR-II illumination. The white arrow highlights the MINDS injection site. The scale bars represent 5 mm. Adapted from Wu et al. (2022), with permission from Springer Nature.
To demonstrate the in vivo utility of this NIR-II neuromodulation technique, the researchers used adeno-associated viruses (AAVs) to selectively transduce TRP channels into dopaminergic neurons in the ventral tegmental area (VTA) of mice, an important deep-brain region in the reward circuitry. After MINDS sensation of TRP channels, dopaminergic neurons in freely behaving mice respond to remotely applied wide-field NIR-II illumination without any brain implant or head tethering (Figure 2A). To evaluate the effectiveness of NIR-II neuromodulation, the researchers performed a conditioned place preference test on freely-behaving mice inside a Y-maze (Figure 2B). While the mouse was allowed to freely explore the entire arena, NIR-II illumination was only present in one of the three arm terminals, all of which are decorated with distinct grating patterns on the wall. After three consecutive days of training, mice learned to associate the specific wall pattern at the arm terminal with NIR-II illumination therein, thus exhibiting a strong place preference for this arm terminal (Figure 2C). All control mice lacking either TRP-expression or MINDS sensitization, however, did not show the similar place preference behavior (Wu et al., 2022). Due to its tether-free and implant-free optical interface, this NIR-II neuromodulation technique enables the study of animal behaviors in the most naturalistic state and is particularly suitable for modulating neural activities in social interaction experiments involving multiple objects.
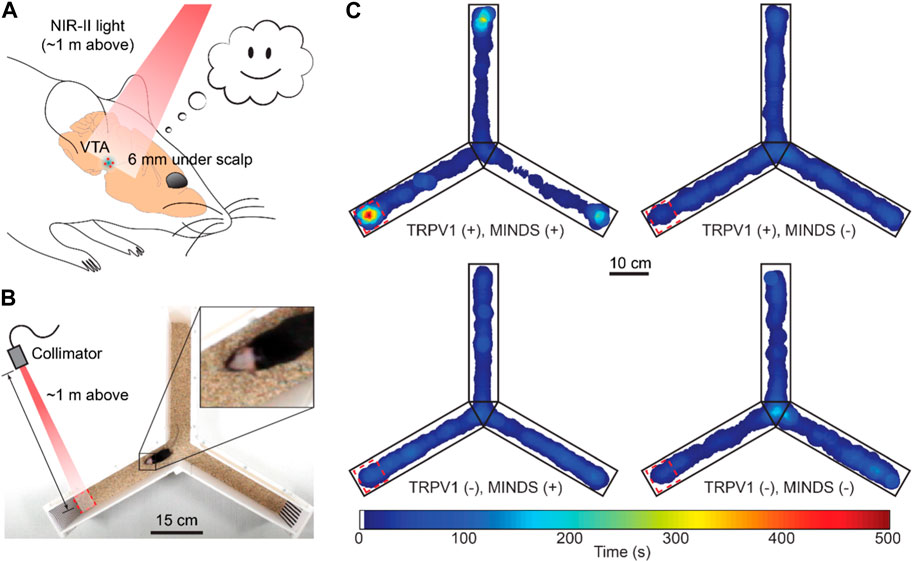
FIGURE 2. (A) Schematic of NIR-II neuromodulation in the VTA. (B) Photo showing a mouse in the Y-maze during the conditioned place preference test. (C) Heatmaps showing the time of travel of mice under different experimental conditions. Red dashed rectangles highlight the region with NIR-II illumination. Adapted from Wu et al. (2022), with permission from Springer Nature.
The Hong lab has been devoted to addressing the challenge of limited penetration of light in biological tissue. As the next step, they are currently working on new material systems to sensitise neurons to radio-frequency waves with much longer wavelengths and deeper tissue-penetration. Furthermore. they have previously developed a technique termed “Sono-optogenetics,” which utilized deep-penetrating focused ultrasound to “gate” the emission of light in deep tissues from circulation-delivered mechanoluminescent nano transducers (Wu et al., 2019; Yang et al., 2022a; Yang et al., 2022b). These materials-based methods of delivering light deep inside the tissue will offer new opportunities for many biophotonic applications.
3 Spotlight in plasmonics
F. A. A. Nugroho, P. Bai, I. Darmadi, G. W. Castellanos, J. Fritzsche, C. Langhammer, J. Gómez Rivas, and A. Baldi, “Inverse designed plasmonic metasurface with parts per billion optical hydrogen detection,” Nat Commun 13(1), 5737 (2022). (Nugroho et al., 2022).
An inverse design to enhance hydrogen detection in plasmonic sensors . Molecular and gas sensing is among the most promising field of application in plasmonics. The rapid evolution of label-free biosensing involves the development of various approaches, such as interferometry, that are even more sensitive than plasmonic platforms. Nevertheless, the latter still remains a “golden” standard in the field. According to Prof. Laura M. Lechuga, one of the most prominent scientists working in the field of biosensing, “If you can use plasmonics for biosensing, do it without hesitation. It is still the easiest and more reliable technique at hand”. Devices based on Surface Plasmon Resonance (SPR) are already routinely employed for static and kinetic analyte detection. In these devices, the analyte is detected by monitoring the shift induced in the wavelength of the SPR propagating on flat gold substrates, which grants unprecedented sensitivity and ease of use (Homola, 2008). Yet, the exploitation of metal nanostructures provides even higher sensitivities, thanks to the higher field confinements and enhancements associated to the Localized SPR (LSPR) in these nanoscale systems (Sepúlveda et al., 2009; Saha et al., 2012). In addition, properly-engineered periodical arrays of nanoparticles may also feature extremely sharp spectral features associated with surface lattice resonances (SLRs) [Bin-Alam2021], which can be exploited to further boost the sensitivity.
One of the most appealing applications of plasmonic nanoparticle-based platforms is in the field of hydrogen (H2) detection (Liu et al., 2011; Wadell et al., 2014) and (photo)catalytic activation (Sytwu et al., 2021; Ezendam et al., 2022) and hydrogen detection. Hybrid plasmonic systems based on palladium (Pd) nanostructures, which strongly react with H2, coupled to gold (Au) nanoparticles are among the most promising platforms suggested thus far for these tasks. This realisation allows to combine the strong reactivity of Pd nanoparticles with the strong field enhancements Au nanostructures, hence circumventing the inherent significant losses and the consequent low sensitivity of Pd at optical wavelengths.
In a recent interdisciplinary paper that appeared in Nature Communication, a team of physicists, chemists, and materials scientists led by Prof. Andrea Baldi from VU Amsterdam broke the paradigm for hybrid plasmonic sensors in hydrogen detectors (Figure 3). By exploiting an inverse design based on particle swarm optimization, they realized a plasmonic platform based on a periodic arrangement of Pd nanostructures, which is capable of unprecedented sensitivity in hydrogen detection (Nugroho et al., 2022). The particle swarm.
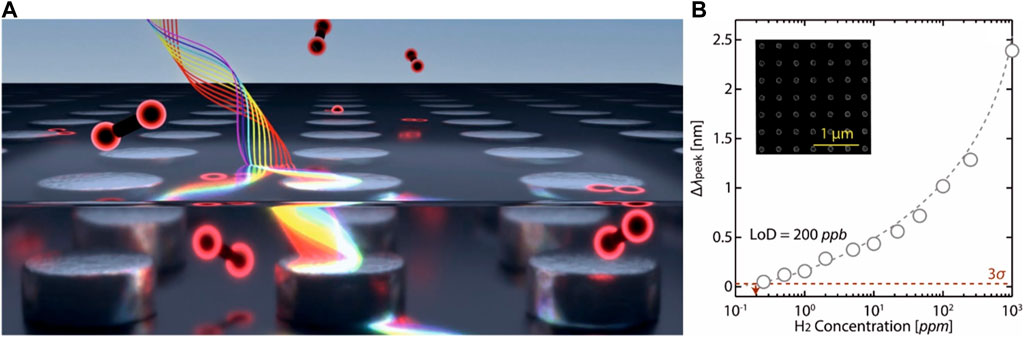
FIGURE 3. (A) Artistic view of the Pd nanoparticle array sensor. Image courtesy of Ananta Rizki Fareza. (B) Measured Δλpeak as a function of H2 concentration [see (Nugroho et al., 2022) for details]. Gray dashed line is a guide to the eye and extrapolates the sensor response to the 3σ value (0.03 nm, red dashed line), indicating a LoD ∼200 ppb (red dashed arrow). Inset: SEM image of the fabricated sensor. Panel b reproduced under CC-BY-4.0. from Nugroho et al. (2022).
Optimization employed by the researchers is an artificial intelligence (AI) approach, which allows to optimize the sensitivity of the device by properly tuning the LSPR associated with the individual nanostructures with respect to the narrower spectral feature associated with the SLRs (Bin-Alam et al., 2021) in the periodic array. This allowed circumventing the above-mentioned limit in sensitivity posed by the high losses in Pd nanoparticles and obtaining a record sensitivity of about 250 parts per billion (ppb). This result makes this device a state-of-the-art platform for sub-ppm detection of H2, which is almost one order of magnitude lower than state-of-the-art detection limits. The authors also claim that their approach “can be extended to arrays of surface-functionalized nanoparticles with resonances that are sensitive to the adsorption of specific gasses via refractive index effects or chemical interface damping, with the potential to address a wider range of societal needs, from home safety to urban air pollution monitoring.”
4 Spotlight in non-linear optics
C. McDonnell, J. Deng, S. Sideris, T. Ellenbogen, and G. Li, “Functional THz emitters based on Pancharatnam-Berry phase non-linear metasurfaces,” Nat. Commun. 12, 30 (2021). (McDonnell et al., 2021).
An interesting emerging direction within the field of non-linear metasurfaces is their ability to generate tailored THz waves. It was shown that the excitation of plasmonic metasurfaces by femtosecond lasers leads to broadband single-cycle bursts of THz radiation (Polyushkin et al., 2011; Luo, Chatzakis, Wang, Niesler, Wegener, Koschny, Soukoulis, 2014). The possibility of engineering the effective non-linear tensor locally on the metasurface opens new directions for the creation of functional non-linear metasurface-based THz emitters (Keren-Zur et al., 2019). In particular, McDonnell et al. (2021) have recently demonstrated functional THz emitters based on Pancharatnam-Berry (PB) phase non-linear metasurfaces. They used a non-linear metasurface made of gold meta-atoms with C3 rotational symmetry and calculated the selection rules for the optical to THz conversion (Figures 4A,B). They showed that excitation of the metasurface with linearly polarized femtosecond laser generates THz photons with ± 3θ PB phase imposed on opposite circular polarization states, where θ is the angle between the direction of linear polarization and the director axis of the meta-atoms. The PB phase was used by them to demonstrate the continuous rotation of the polarization state of the generated broadband THz wavepacket (Figures 4C,D). In addition they demonstrated experimentally the generation of broadband THz radiation with pure circular polarization states diffracting to opposite diffraction orders of a phase gradient metasurface. This enabled demonstration of a THz circular dichroism spectroscopic measurement of alpha lactose powder. Furthermore, the combination with the direct space-to-time mapping concept was leveraged to generate 5-cycle THz pulses with abrupt temporal polarization dispersion within the pulse. The disruptive development of functional metasurface THz emitters may be used for the demonstration of many more functionalities and for new applications. For example, it was also recently used for generation of toroidal single-cycle pulses of light that possess intriguing electromagnetic characteristics (Zdagkas et al., 2022). In addition to the demonstration of new functionalities efforts in the field are now focused on studies of all-dielectric THz metasurface emitters (Hale et al., 2022; Leon et al., 2022), that may be promising in enhancing the generation efficiency and adding new tools to control the THz generation.
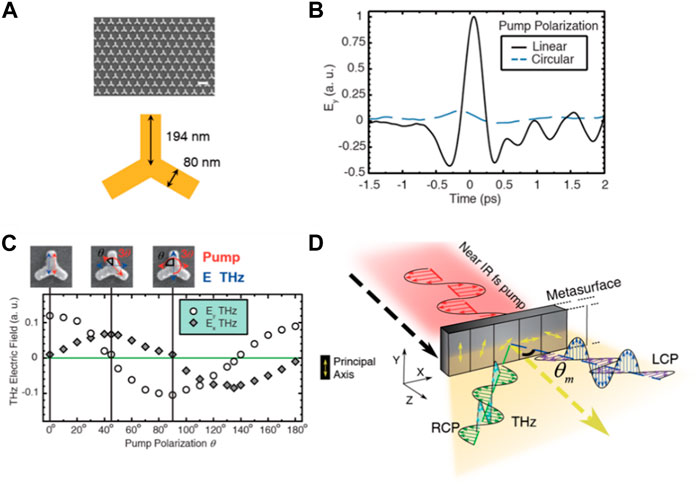
FIGURE 4. (A) Gold metasurface with C3 meta-atoms for THz generation. (B) Experimental confirmation of selection rules for the THz generation that allow/forbid generation by linearly/circularly polarized excitation. (C) Experimental demonstration of rotation of linear polarization of THz photons by
5 Spotlight in photovoltaic materials
D. Kim, G. G. Jeon, J. H. Kim, J. Kim, and N. Park, “Design of a Flexible Thin-Film Encapsulant with Sandwich Structures of Perhydropolysilazane Layers,” ACS Appl. Mater. Interfaces 14(30), 34678–34685 (2022). (Kim et al., 2022).
Metal halide perovskite solar cells have emerged as a promising thin film photovoltaic (PV) technology owing to their inherently excellent optical and electronic properties such as large optical absorption coefficient, high carrier mobility, long carrier lifetime and low mid-gap state density (Kim et al., 2019). The worldwide research effort has driven enormous efficiency improvement from 3.8% in 2009% to 25.7% in 2021, comparable to the record efficiency of c-Si (NREL Best, 2022). Apart from its excellent efficiency, perovskite PV also has the advantages of being lightweight and flexible, therefore, can find broader applications in niche markets of structure-integrated PV, including buildings, vehicles and space crafts. However, the pressing material instability is the key issue hindering the commercialization of perovskite PV. In the last few years, research effort has shifted towards the development of stable perovskite materials and encapsulation techniques preventing moisture ingress, a critical environmental agent causing the decomposition of perovskite light absorber.
Compared to the conventional glass cover encapsulation technique, thin film encapsulation possesses distinguished advantages of lightweight and flexibility, and can target market like portable devices, building-integrated photovoltaics and even spacecrafts. Multilayered flexible barrier films have demonstrated excellent water permeation properties as characterized by water vapor transmission rate (WVTR). However, fabrication of these barrier films often relies on physical or chemical vapour deposition methods, hence expensive and time-consuming.
Recently, Dr. Nochang Park and colleagues at Korea Electronics Technology Institute developed a low-cost solution-processed flexible multi-layer encapsulation method and demonstrated excellent WVTR as well as PV cell lifetime when applied to encapsulate perovskite solar cells (Kim et al., 2022). They used perhydropolysilazane (PHPS), which consists of −SiH2−NH− backbone, as a precursor solution to form SiOx - SiONx barrier layer on a flexible poly (ethylene terephthalate) (PET) film. After deep UV light treatment, the spin-coated PHPS converted to a dense and pin-hole free inorganic thin film as shown in Figure 5A. They also design a multi-layer structure to further enhance the water permeation property by sandwiching a PET film between two PHPS layers. The sandwiched PHPS/PET/PHPS film has demonstrated improved water permeation performance with a WVTR of 9.2 × 10−4 gm−2 d−1, while the WVTR of a single PHPS/PET film was 7.77 × 10−3 gm−2 d−1 (Figure 5B). They also evaluated the mechanical property of the sandwiched barrier layer. WVTR values remained stable even after 1,000 bending cycles, indicating that the PHPS/PET/PHPS encapsulation layer maintained its excellent moisture barrier quality under flexible conditions.
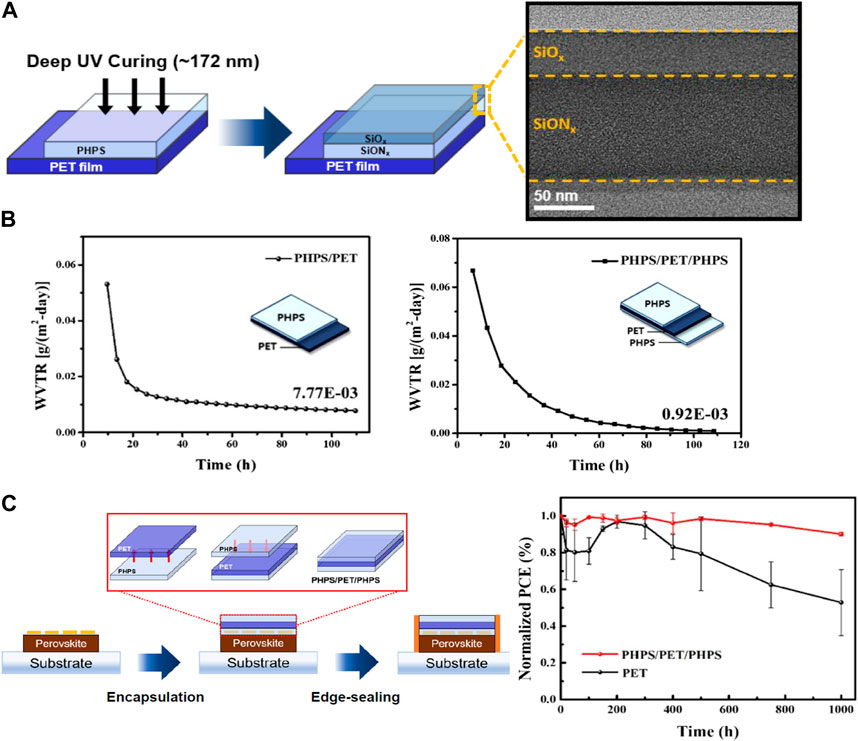
FIGURE 5. (A) A diagram of the formation and SEM image of inorganic barrier films. (B) WVTR values of a single layer PHPS coated on a PET substrate and double PHPS sandwiched PET. (C) A diagram of the encapsulation process for a perovskite solar cell and the stability test results. Adapted with permission from Kim et al., 2022. Copyright © 2022, American Chemical Society.
They then used this high-performing sandwiched PHPS/PET/PHPS film to encapsulate perovskite solar cells and tested the device performance after storing it in water reservoir. They found that the solar cell encapsulated by PHPS/PET/PHPS film retained 90% of its initial efficiency after 1,000 h of operation in the reservoir test, while the efficiency of the solar cells encapsulated by a PET film decreased ∼50% as shown in Figure 5C. This work demonstrated a low-cost and robust encapsulation technique for both flexible and rigid PV devices to stabilize the emerging next-generation perovskite solar cells.
6 Spotlight in optical information processing and holography
A. Bulbul, N. Hai, and J. Rosen, “Coded aperture correlation holography (COACH) with a superior lateral resolution of FINCH and axial resolution of conventional direct imaging systems,” Opt. Express 29(25), 42106 (2021). (Bulbul et al., 2021).
In the area of incoherent optical processing, when compared with their coherent counterparts, incoherent systems have a much better signal-to-noise ratio (SNR). In incoherent holography, the intensity distribution of a 3D object is captured holographically (Zhang and Poon, 2023). Incoherent digital holography (IDH) opens the door to extend the practical applications of digital holography. Indeed, any light source, including self-luminous or fluorescent light, can be used to record holograms. One of the most important applications of IDH is the invention of holographic fluorescence microscopes for biomedical 3D imaging.
A research topic in the area of incoherent digital holography is spotlighted as a recent advance in self-interference incoherent digital holography is reported. Historically, scanning-based methods were first developed for IDH (Zhang and Poon, 2023). Non-scanning approaches called Fresnel incoherent correlation holography (FINCH) and coded-aperture correlation holography (COACH) based on the principle of self-inter-reference were subsequently developed. FINCH has an enhanced lateral resolution and therefore comes at a price of low axial resolution according to the 3D imaging principle (direct imaging), which states that the higher the lateral resolution is, the lower the axial resolution will be. In comparison to FINCH, COACH, on the other hand, preserves a higher axial resolution but a lower lateral resolution. In the spotlight paper, the advantages of FINCH and COACH are investigated and maximized through a proposed variant of COACH called coded aperture with FINCH responses (CAFIR). Figure 6 shows the point spread function distributions along the axial direction for direct imaging, FINCH and CAFIR. We note that FINCH has a lower axial resolution, while CAFIR and direct imaging have a higher axial resolution, and at the same time CAFIR has FINCH-like enhanced lateral resolution.
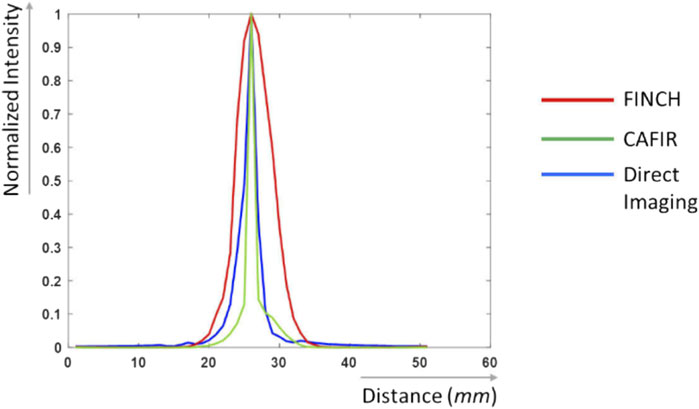
FIGURE 6. Point spread functions along the axial direction (adapted with permission from Bulbul et al. (2021), under the terms of the Optica Open Access Publishing Agreement).
The work could potentially impact 3D imaging in biomedical applications where enhanced lateral resolution and high axial resolution are needed simultaneously.
7 Spotlight in light sources and luminescent materials
T. P. van Swieten, A. Meijerink, and F. T. Rabouw, “Impact of Noise and Background on Measurement Uncertainties in Luminescence Thermometry,” ACS Photonics (94), 1,366–1,374 (2022). (van Swieten et al., 2022).
M. Quintanilla, M. Henriksen-Lacey, C. Renero-Lecuna, and L. M. Liz-Marzán, “Challenges for optical nanothermometry in biological environments,” Chem. Soc. Rev. 51(11), 4223–4242 (2022). (Quintanilla et al., 2022).
Temperature-dependent luminescence is exhibited by a wide range of materials, ranging from bulk phosphors (Gao et al., 2021) to nanomaterials (Brites et al., 2012). The pursuit of nanothermometers, which can enable contactless temperature measurement on the microscopic scale, has huge potential applications in biological sciences (Ximendes et al., 2016), electronic and electrical components (van Swieten et al., 2021), and catalysis (Hartman et al., 2020). In nanothermometry, the temperature is determined based on either the i) emission spectrum or ii) luminescence lifetime, as outlined in Figure 7. The most common approach is to measure the intensity ratio between two temperature-dependent emission bands, as this method is not affected by variation in the excitation intensity, changes in alignment, and any scattering of the luminescence.
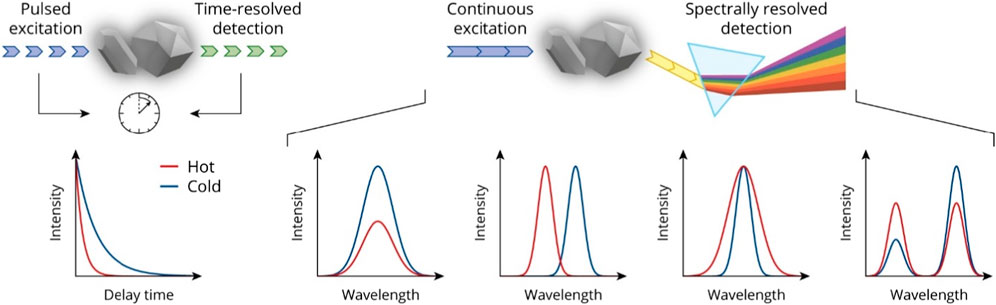
FIGURE 7. Overview of different approaches for using luminescence signals for extracting temperature information, primarily divided into the analysis of the luminescence lifetime (left-hand side) or the emission spectra (remainder of figure). Reproduced with permission from Van Swieten (2022), with permission from T.P. Van Swieten.
However, several real-world aspects still hinder the adoption of the technology and hinder the interpretation of results, and two papers from 2022 that focus on such issues feature as spotlights: i) the article by van Swieten, Meijerink and Rabouw from the University of Utrecht (van Swieten et al., 2022); and ii) the tutorial review published by a Spanish collaboration of Quintanilla et al. (2022). The scientific progress presented in these two works will be described in detail below.
In the first work, van Swieten et al. point out that the majority of research articles focus on relative sensitivity Sr (units: %/K), i.e., to what degree the intensity ratio changes with temperature (van Swieten et al., 2022). However, the reliability of temperature readout depends not only on Sr, but also on the signal-to-noise ratio of the measurement, such that the temperature uncertainty σT can be determined. Since the value σT–unlike Sr–is not an intrinsic property of a nanothermometery material, this has resulted in reported uncertainties varying by several orders of magnitude, even for the same thermometer under similar environmental conditions (van Swieten et al., 2022).
In the article by van Swieten et al. (2022), the authors investigate how photon detection statistics can be used to evaluate the effect that both noise and background signals have on the uncertainty of a luminescence nanothermometry experiment. Background-free luminescence is achieved by using two green upconversion bands (516–534 nm and 538–545 nm) of NaYF4:Er3+(2%),Yb3+(18%) nanocrystals, excited at 980 nm. The authors then proceed to measure all sources of noise associated with photon detection and subsequently present statistical models to quantitatively predict σT over a wide temperature range and under different experimental conditions. It is pointed out that increasing the gain in the CCD-based detector can significantly reduce the uncertainty down to a level where the readout noise is overcome. The authors also investigate the effect that background emission–from the surroundings of the nanothermometer–contribute to σT, for example, the effect that an observed spectrum (that is distorted by such affects) can have on σT even after background subtraction. At the end, the authors present a guideline as to how to compare different nanothermometers, eliminating the effects of the sample being investigated and/or the spectroscopic equipment employed.
In the second work, Quintanilla et al. (2022) review the challenges involved in applying nanothermometers in medical diagnosis. In particular, the challenge is that both excitation light and the emitted luminescence have to travel through tissue. This is an example of the optical properties of the sample affecting not only the brightness of the emission but also the shape of the emission spectra being altered and, therefore, potentially misinterpreted.
Focusing on the one hand, the effects of a biological environment on the excitation, the authors demonstrate how optimising the width of the excitation beam can be utilised to enable scattering effects to reach deeper tissue. Furthermore, even when operating in the near infrared, it is already known that some components, such as melanin, exhibit luminescence. Thus, this autofluorescence presents a background signal problem. Now switching focus to the other hand, Quintanilla et al. examine the interaction of the emission with tissue. As mentioned above, the main challenge here is tissue absorption altering the shape of the luminescence spectrum, thus becoming a source of error when the measurement of an absolute temperature is desired. For this reason, many in vivo experiments instead rely on reporting relative temperature values instead, which given the sensitivity of living beings to slight changes in temperature is not ideal. A further challenge is the low brightness of lanthanide-doped materials–which is mostly limited by their poor absorption cross-section–that becomes a major limiting factor when considering penetration depth and thermal resolution. While optical antennas have been demonstrated to be able to channel light towards a lanthanide ion, these have not yet been applied in a biomedical environment. Thus, the challenge of engineering more highly absorbing and brighter (higher quantum yield) lanthanide-based emitters remains.
Overall, the development of techniques for optical measurement of temperature at the micro-scale could play a valuable role in applications such as biological imaging and process control.
Author contributions
All the authors contributed to the general setting of the review and the identification of the spotlighted papers. In particular, BA, MC, CA, SH, T-CP, and BR managed the drafting of the Spotlights on Biophotonics, Plasmonics, Non-linear Optics, Photovoltaic Materials, Optical Information Processing and Holography, and Light Sources and Luminescent Materials, respectively. MP supervised the overall organization, drafting, and finalization of the paper.
Acknowledgments
The authors are indebted to all the authors of the spot-lighted papers for providing feedback on their outputs and to Springer Nature, the Optica Publishing Group and the American Chemical Society for providing the necessary reprint permission, where required.
Conflict of interest
The authors declare that the research was conducted in the absence of any commercial or financial relationships that could be construed as a potential conflict of interest.
Publisher’s note
All claims expressed in this article are solely those of the authors and do not necessarily represent those of their affiliated organizations, or those of the publisher, the editors and the reviewers. Any product that may be evaluated in this article, or claim that may be made by its manufacturer, is not guaranteed or endorsed by the publisher.
References
Bin-Alam, M. S., Reshef, O., Mamchur, Y., Alam, M. Z., Carlow, G., Upham, J., et al. (2021). Ultra-high-Q resonances in plasmonic metasurfaces. Nat. Commun. 12, 974. doi:10.1038/s41467-021-21196-2
Brites, C. D. S., Lima, P. P., Silva, N. J. O., Millán, A., Amaral, V. S., Palacio, F., et al. (2012). Thermometry at the nanoscale. Nanoscale 4, 4799. doi:10.1039/c2nr30663h
Bulbul, A., Hai, N., and Rosen, J. (2021). Coded aperture correlation holography (COACH) with a superior lateral resolution of FINCH and axial resolution of conventional direct imaging systems. Opt. Express 29, 42106. doi:10.1364/OE.446945
Chen, R., Gore, F., Nguyen, Q-A., Ramakrishnan, C., Patel, S., Kim, S. H., et al. (2021). Deep brain optogenetics without intracranial surgery. Nat. Biotechnol. 39, 161–164. doi:10.1038/s41587-020-0679-9
Chen, R., Romero, G., Christiansen, M. G., Mohr, A., and Anikeeva, P. (2015). Wireless magnetothermal deep brain stimulation. Science 347, 1477–1480. doi:10.1126/science.1261821
Chen, Y., Rommelfanger, N. J., Mahdi, A. I., Wu, X., Keene, S. T., Obaid, A., et al. (2021). How is flexible electronics advancing neuroscience research? Biomaterials 268, 120559. doi:10.1016/j.biomaterials.2020.120559
Ezendam, S., Herran, M., Nan, L., Gruber, C., Kang, Y., Gröbmeyer, F., et al. (2022). Hybrid plasmonic nanomaterials for hydrogen generation and carbon dioxide reduction. ACS Energy Lett. 7, 778–815. doi:10.1021/acsenergylett.1c02241
Fenno, L., Yizhar, O., and Deisseroth, K. (2011). The development and application of optogenetics. Annu. Rev. Neurosci. 34, 389–412. doi:10.1146/annurev-neuro-061010-113817
Gao, G., Busko, D., Katumo, N., Joseph, R., Madirov, E., Turshatov, A., et al. (2021). Ratiometric luminescent thermometry with excellent sensitivity over a broad temperature range utilizing thermally-assisted and multiphoton upconversion in triply-doped La 2 O 3:Yb 3+/Er 3+/Nd 3+. Adv. Opt. Mater 9, 2001901. doi:10.1002/adom.202001901
Gracheva, E. O., Ingolia, N. T., Kelly, Y. M., Cordero-Morales, J. F., Hollopeter, G., Chesler, A. T., et al. (2010). Molecular basis of infrared detection by snakes. Nature 464, 1006–1011. doi:10.1038/nature08943
Hale, L. L., Jung, H., Gennaro, S. D., Briscoe, J., Harris, C. T., Luk, T. S., et al. (2022). Terahertz pulse generation from GaAs metasurfaces. ACS Photonics 9, 1136–1142. doi:10.1021/acsphotonics.1c01908
Hartman, T., Geitenbeek, R. G., Wondergem, C. S., van der Stam, W., and Weckhuysen, B. M. (2020). Operando nanoscale sensors in catalysis: All eyes on catalyst particles. ACS Nano 14, 3725–3735. doi:10.1021/acsnano.9b09834
Homola, J. (2008). Surface Plasmon resonance sensors for detection of chemical and biological species. Chem. Rev. 108, 462–493. doi:10.1021/cr068107d
Hong, G., Antaris, A. L., and Dai, H. (2017). Near-infrared fluorophores for biomedical imaging. Nat. Biomed. Eng. 1, 0010. doi:10.1038/s41551-016-0010
Jiang, S., Wu, X., Rommelfanger, N. J., Ou, Z., and Hong, G. (2022). Shedding light on neurons: Optical approaches for neuromodulation. Natl. Sci. Rev. 9, nwac007. doi:10.1093/nsr/nwac007
Keren-Zur, S., Tal, M., Fleischer, S., Mittleman, D. M., and Ellenbogen, T. (2019). Generation of spatiotemporally tailored terahertz wavepackets by nonlinear metasurfaces. Nat. Commun. 10, 1778. doi:10.1038/s41467-019-09811-9
Kim, D., Jeon, G. G., Kim, J. H., Kim, J., and Park, N. (2022). Design of a flexible thin-film encapsulant with Sandwich structures of perhydropolysilazane layers. ACS Appl. Mater Interfaces 14, 34678–34685. doi:10.1021/acsami.2c06699
Kim, J., Ho-Baillie, A., and Huang, S. (2019). Review of novel passivation techniques for efficient and stable perovskite solar cells. Sol. RRL 3, 1800302. doi:10.1002/solr.201800302
Kim, T., McCall, J. G., Jung, Y. H., Huang, X., Siuda, E. R., Li, Y., et al. (2013). Injectable, cellular-scale optoelectronics with applications for wireless optogenetics. Science 340, 211–216. doi:10.1126/science.1232437
Leon, U. A., Rocco, D., Carletti, L., Peccianti, M., Maci, S., Della Valle, G., et al. (2022). THz-photonics transceivers by all-dielectric phonon-polariton nonlinear nanoantennas. Sci. Rep. 12, 4590. doi:10.1038/s41598-022-08695-y
Liu, N., Tang, M. L., Hentschel, M., Giessen, H., and Alivisatos, A. P. (2011). Nanoantenna-enhanced gas sensing in a single tailored nanofocus. Nat. Mater 10, 631–636. doi:10.1038/nmat3029
Luo, L., Chatzakis, I., Wang, J., Niesler, F. B. P., Wegener, M., Koschny, T., et al. (2014). Broadband terahertz generation from metamaterials. Nat. Commun. 5, 3055. doi:10.1038/ncomms4055
McDonnell, C., Deng, J., Sideris, S., Ellenbogen, T., and Li, G. (2021). Functional THz emitters based on Pancharatnam-Berry phase nonlinear metasurfaces. Nat. Commun. 12, 30. doi:10.1038/s41467-020-20283-0
Nelidova, D., Morikawa, R. K., Cowan, C. S., Raics, Z., Goldblum, D., Scholl, H. P. N., et al. (2020). Restoring light sensitivity using tunable near-infrared sensors. Science 368, 1108–1113. doi:10.1126/science.aaz5887
NREL Best (2022). Research-cell efficiency chart. Available at: https://www.nrel.gov/pv/assets/pdfs/best-research-cell-efficiencies-rev220630.pdf.
Nugroho, F. A. A., Bai, P., Darmadi, I., Castellanos, G. W., Fritzsche, J., Langhammer, C., et al. (2022). Inverse designed plasmonic metasurface with parts per billion optical hydrogen detection. Nat. Commun. 13, 5737. doi:10.1038/s41467-022-33466-8
Polyushkin, D. K., Hendry, E., Stone, E. K., and Barnes, W. L. (2011). THz generation from plasmonic nanoparticle arrays. Nano Lett. 11, 4718–4724. doi:10.1021/nl202428g
Quintanilla, M., Henriksen-Lacey, M., Renero-Lecuna, C., and Liz-Marzán, L. M. (2022). Challenges for optical nanothermometry in biological environments. Chem. Soc. Rev. 51, 4223–4242. doi:10.1039/D2CS00069E
Saha, K., Agasti, S. S., Kim, C., Li, X., and Rotello, V. M. (2012). Gold nanoparticles in chemical and biological sensing. Chem. Rev. 112, 2739–2779. doi:10.1021/cr2001178
Salatino, J. W., Ludwig, K. A., Kozai, T. D. Y., and Purcell, E. K. (2017). Glial responses to implanted electrodes in the brain. Nat. Biomed. Eng. 1, 862–877. doi:10.1038/s41551-017-0154-1
Sepúlveda, B., Angelomé, P. C., Lechuga, L. M., and Liz-Marzán, L. M. (2009). LSPR-based nanobiosensors. Nano Today 4, 244–251. doi:10.1016/j.nantod.2009.04.001
Sytwu, K., Vadai, M., Hayee, F., Angell, D. K., Dai, A., Dixon, J., et al. (2021). Driving energetically unfavorable dehydrogenation dynamics with plasmonics. Science 371, 280–283. doi:10.1126/science.abd2847
van Swieten, T. P., Meijerink, A., and Rabouw, F. T. (2022). Impact of noise and background on measurement uncertainties in luminescence thermometry. ACS Photonics 9, 1366–1374. doi:10.1021/acsphotonics.2c00039
Van Swieten, T. P. (2022). The design and application of luminescent thermometers: A closer look at biases and uncertainties- PhD thesis. Utrecht, Netherlands: University of Utrecht. (In Press).
van Swieten, T. P., van Omme, T., van den Heuvel, D. J., Vonk, S. J. W., Spruit, R. G., Meirer, F., et al. (2021). Mapping elevated temperatures with a micrometer resolution using the luminescence of chemically stable upconversion nanoparticles. ACS Appl. Nano Mater 4, 4208–4215. doi:10.1021/acsanm.1c00657
Wadell, C., Syrenova, S., and Langhammer, C. (2014). Plasmonic hydrogen sensing with nanostructured metal hydrides. ACS Nano 8, 11925–11940. doi:10.1021/nn505804f
Wu, X., Jiang, Y., Rommelfanger, N. J., Yang, F., Zhou, Q., Yin, R., et al. (2022). Tether-free photothermal deep-brain stimulation in freely behaving mice via wide-field illumination in the near-infrared-II window. Nat. Biomed. Eng. 6, 754–770. doi:10.1038/s41551-022-00862-w
Wu, X., Zhu, X., Chong, P., Liu, J., Andre, L. N., Ong, K. S., et al. (2019). Sono-optogenetics facilitated by a circulation-delivered rechargeable light source for minimally invasive optogenetics. Proc. Natl. Acad. Sci. 116, 26332–26342. doi:10.1073/pnas.1914387116
Ximendes, E. C., Santos, W. Q., Rocha, U., Kagola, U. K., Sanz-Rodríguez, F., Fernández, N., et al. (2016). Unveiling in vivo subcutaneous thermal dynamics by infrared luminescent nanothermometers. Nano Lett. 16, 1695–1703. doi:10.1021/acs.nanolett.5b04611
Yang, F., Wu, X., Cui, H., Jiang, S., Ou, Z., Cai, S., et al. (2022). Palette of rechargeable mechanoluminescent fluids produced by a biomineral-inspired suppressed dissolution approach. J. Am. Chem. Soc. 144, 18406–18418. doi:10.1021/jacs.2c06724
Yang, F., Wu, X., Cui, H., Ou, Z., Jiang, S., Cai, S., et al. (2022). A biomineral-inspired approach of synthesizing colloidal persistent phosphors as a multicolor, intravital light source. Sci. Adv. 8, eabo6743. doi:10.1126/sciadv.abo6743
Zdagkas, A., McDonnell, C., Deng, J., Shen, Y., Li, G., Ellenbogen, T., et al. (2022). Observation of toroidal pulses of light. Nat. Photonics 16, 523–528. doi:10.1038/s41566-022-01028-5
Keywords: photonics highlights, biophotonics, plasmonics, non-linear optics, photovoltaic materials, optical information processing and holography and light sources, luminescent materials
Citation: Anvari B, Celebrano M, De Angelis C, Huang S, Poon T-C, Richards BS and Peccianti M (2023) Frontiers in photonics spotlight. Front. Photonics 4:1131853. doi: 10.3389/fphot.2023.1131853
Received: 26 December 2022; Accepted: 27 February 2023;
Published: 12 April 2023.
Edited by:
Murugan Senthil Mani Rajan, Anna University, IndiaReviewed by:
Renzhe Bi, Institute of Bioengineering and Nanotechnology (A∗STAR), SingaporeXian Chen, Shenzhen University, China
Copyright © 2023 Anvari, Celebrano, De Angelis, Huang, Poon, Richards and Peccianti. This is an open-access article distributed under the terms of the Creative Commons Attribution License (CC BY). The use, distribution or reproduction in other forums is permitted, provided the original author(s) and the copyright owner(s) are credited and that the original publication in this journal is cited, in accordance with accepted academic practice. No use, distribution or reproduction is permitted which does not comply with these terms.
*Correspondence: Marco Peccianti, m.peccianti@lboro.ac.uk