A review of the low-energy K−-nucleus/nuclei interactions with light nuclei AMADEUS investigations
- 1Marian Smoluchowski Institute of Physics, Jagiellonian University, Kraków, Poland
- 2Center for Theranostics, Jagiellonian University, Kraków, Poland
- 3Stefan-Meyer-Institut für Subatomare Physik, Vienna, Austria
- 4Physik Department E62, Technische Universität München, Garching, Germany
- 5Instituto Nazionale di Fisica Nucleare, Laboratori Nazionali di Frascati, Frascati, Italy
- 6Excellence Cluster “Origin and Structure of the Universe”, Garching, Germany
- 7Centro Ricerche Enrico Fermi—Museo Storico della Fisica e Centro Studi e Ricerche “Enrico Fermi”, Roma, Italy
- 8Horia Hulubei National Institute of Physics and Nuclear Engineering, Magurele, Romania
- 9Department of Theoretical Physics, National Centre for Nuclear Research, Warsaw, Poland
- 10INFN Sezione di Roma Tre, Roma, Italy
- 11Department of Physics, China University of Geosciences, Wuhan, China
- 12Dipartimento di Scienze Matematiche e Informatiche, Scienze Fisiche e Scienze della Terra dell’Università di Messina, Messina, Italy
- 13INFN Sezione di Catania, Catania, Italy
- 14Dipartimento di Scienze e Tecnologie Applicate, Università “Guglielmo Marconi”, Roma, Italy
The AMADEUS Collaboration conducts research aimed to experimentally investigate the low-energy K− hadronic interactions with light nuclei like hydrogen, helium, and carbon, in order to provide new constraints to the antikaon-nucleon strong interaction studies in the non-perturbative quantum chromodynamics regime. K− nuclear absorption, both at-rest and in-flight, are explored using the unique low-momentum and monochromatic kaon beam from the DAΦNE collider interacting with the KLOE detector components, a detector characterized by high acceptance and excellent position and momentum resolutions. This paper presents an overview of the AMADEUS results.
1 Introduction
The AMADEUS (Anti-kaonic Matter At DAΦNE: An Experiment with Unraveling Spectroscopy) Collaboration performed research of the low-energy K−-nucleon/nuclei interactions in light nuclear targets for over a decade [1–3]. The primary objective of these studies is an investigation of the poorly known Λ(1405) resonance and a deeper understanding of the K− single- and multi-nucleon absorption processes, both at-rest and in-flight, including the possible formation of kaonic bound states.
The investigation of the in-medium modification of the
Two main theoretical approaches have been developed to overcome these difficulties, namely, phenomenological potential models based on the
Recently the ALICE Collaboration confirmed the couple-channel character of the
Investigation of K− multi-nucleon absorption contributions plays a very important role in the determination of the K−-nucleus optical potential. Existing K− single-nucleon optical potentials, combined with phenomenologically determined K− multi-nucleon absorption term (based on global absorption bubble chamber data) do not reproduce the kaonic atoms data along the periodic table of the elements [28, 29]. Therefore, it is crucial to improve the theoretical model by providing complete characteristics of the absorption processes, by extracting the two-, three-, and four-nucleon absorptions (2NA, 3NA, and 4NA). The first comprehensive measurement of K− multi-nucleon absorptions, including a contribution of the possible K−pp bound state, has been completed [30].
The purpose of the article is to provide an overview of the current status of the research performed by AMADEUS collaboration. It begins with an introduction of the experimental facility, namely, the DAΦNE accelerator and the KLOE detector. Thereupon, K− single- and multi-nucleon absorption studies and their impact on the field are discussed, which is followed by Conclusions.
2 Experimental facility
The AMADEUS studies are based on an experimental data sample, corresponding to 1.74 fb−1 integrated luminosity, collected with the KLOE detection system [31, 32], installed at the double electron-positron ring of the DAΦNE collider [33] located at the National Laboratory in Frascati of INFN (Italy).
The DAΦNE facility (so-called ϕ meson factory) was designed to work at the center of mass energy of the ϕ meson. The acceleration complex deliver low-momentum (∼127 MeV/c) monochromatic charged kaon beam, characterized by a very small hadronic background, originating from the ϕ-meson decays (BR(K+K−) = (48.9 ± 0.5)%) which, in turn, is produced in e+e− collisions (beam energies of 0.51 GeV). The back-to-back topology of the kaons pair production allows to extrapolate non-identified charged kaon tracks.
The KLOE detector system has a 4π geometry and surrounds the DAΦNE interaction region (geometrical acceptance of 98%). The detection setup consists of two basic components: a large cylindrical Drift Chamber (DC) [31, 34] and an electromagnetic calorimeter (EMC) consisting of groved lead with scintillating fibers [31, 35]. The detection system was immersed in a 0.52 T magnetic field along the beam axis, provided by a superconducting solenoid.
The DC, designed for tracking and identification of charged particles, containing a total of about 52,000 wire, was filled with a mixture of helium (90%) and isobutane (10%) C4H10. Its inner radius, outer radius, and length were equal to 0.25, 2, and 3.3 m, respectively. The DC entrance wall was built of 750 μm layer of low-density carbon fiber and 150 μm layer of aluminum. The momenta of charged particles were determined with excellent relative accuracy of
The EMC composed of a cylindrical barrel with an inner radius of 2 m and two end-caps was dedicated to neutral particles detection. It also provided Time-of-Flight (TOF) information for the charged particles. The volume ratio of lead-scintillating fibers (lead/fibers/glue = 42:48:10) was optimized to achieve high light yield and high efficiency for photons in the 20–300 MeV/c energy range. The cluster position resolution along the fibers was
3 Single- and multi-nucleon K− absorption studies
Since the Λ(1405)’s resonance line shape is expected to depend on both, the production mechanism and the observed decay channel [26], experimental investigation of its properties is challenging. Additionally, extraction of the shape of the Λ(1405) invariant mass in reactions induced by negatively charged kaons (K−) is complicated by two biases. The first bias arises from the threshold of the Σπ invariant mass, which is limited by the last nucleon binding energy. This threshold is approximately 1,412 MeV/c2 for K− capture at rest on 4He and around 1,416 MeV/c2 on 12C. Therefore, to verify the existence of the predicted high mass pole of the Λ(1405), which is expected to be located at approximately 1,420 MeV/c2, it is necessary to explore the K− absorption in flight. As shown in [36], the experimental Σ0π0 invariant mass threshold for K− captures in 12C in flight (pK ∼ 100 MeV/c) is shifted upwards by about 10 MeV with respect to the capture at-rest, thus opening the access to the energy range of interests. The Σ0π0 is the so-called “golden decay channel” since it provides a clear Λ(1405) signature in the I = 0 isospin.
Another crucial bias impacting the (Σπ)0 invariant mass spectrum is associated with the non-resonant contribution, which needs to be subtracted in order to extract the shape and investigate the characteristics of the Λ(1405) resonance. Chiral SU(3) meson-baryon coupled channels interaction models (Barcelona (BCN) [37], Prague (P) [38], Kyoto-Munich (KM) [39], Murcia (M1,M2) [40], Bonn (B2,B4) [41]) provide the K−n → Λπ/Σπ scattering amplitudes, which, however, strongly differ in the
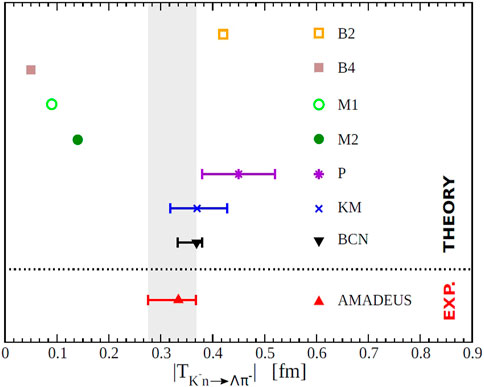
FIGURE 1. Modulus of the measured non resonant K−n → Λπ− transition amplitude (with combined statistical and systematic errors) compared with theoretical calculations, see details in the text. Figure is adapted from [43].
The obtained result enables to test the chiral predictions in the subthreshold region which allow constraining the corresponding non-resonant background for I = 0 channel (Σπ)0 and hence to determine the Λ(1405) properties.
Apart from single-nucleon capture studies, AMADEUS conducted research specifically focused on K− absorptions on two or more nucleons. The investigation is highly significant for the determination of K−-nucleus/nucleon optical potential which has a strong impact on various sectors of physics, like nuclear and particle physics as well as astrophysics [44–46]. A detailed characterization of the K− two-, three- and four-nucleon absorption processes (2NA, 3NA, and 4NA) in K− capture on 12C nuclei was obtained by investigating Λ(Σ0)p decay channels [30, 47]. A comprehensive study was performed [30] based on the phenomenological model for the K− captures at-rest and in-flight on light nuclei [42, 48]. The simultaneous fit of the experimental Λp invariant mass, Λp angular correlation, Λ and proton momentum spectra with the corresponding simulated distributions of the contributing processes (including the Σ0 productions followed by Σ0 → Λγ decay and for the 2NA: 1) the Quasi-Free (QF) processes, 2) elastic Final State Interaction (FSI) processes and 3) inelastic FSI processes due to conversion (ΣN →ΛN′)), allowed to extract the K− 2NA, 3NA and 4NA branching ratios (BRs) and cross sections for low-momentum kaons in Λp and Σ0p final states. The obtained BRs and cross sections are summarized in Table 1.
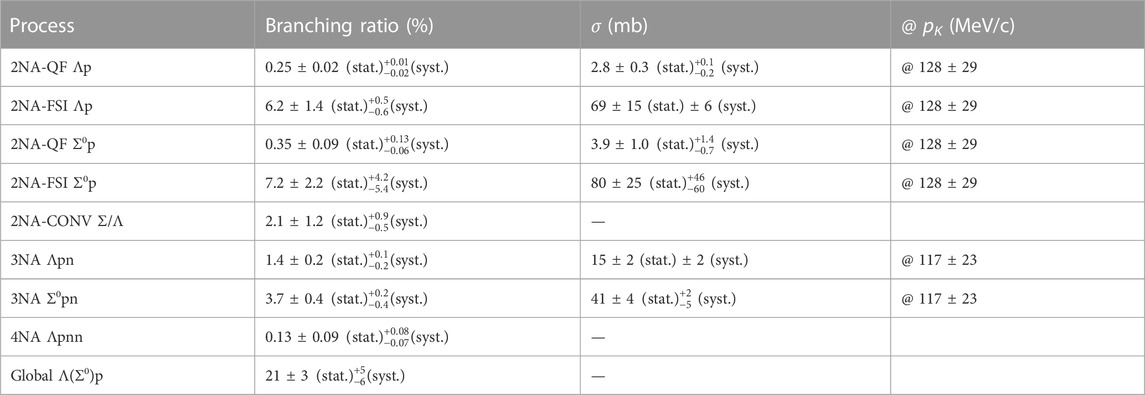
TABLE 1. Branching ratios (for the K− captured at-rest) and cross sections (for the K− captured in-flight) of the K− multi-nucleon absorption processes. The K− momentum is evaluated in the centre of mass reference frame of the absorbing nucleons, thus it differs for the 2NA and 3NA processes. The statistical and systematic errors are also given. The Table is adapted from [30].
The determined global BR (sum of 2NA, 3NA, and 4NA BRs) (21 ± 3
The performed studies show that the experimental BR of the Λp QF production in K− 2NA interaction is lower than that of Σ0p QF production:
The potential contribution of the K−pp bound system to the Λp spectra was explored revealing the entire overlap of the signal associated with the formation of the K−pp cluster in K−-induced reactions on carbon with the K− 2NA-QF process [30]. Repeating the analysis in the FINUDA-like measurement [23] conditions (selection of back-to-back Λp events (cosθΛp < −0.8)) yielded the same results (BRs are in agreement with those obtained from entire data sample), indicating that if the bound system exists, it cannot be distinguished from the two-nucleon capture process within this type of analysis.
4 Conclusion
In this paper, the results obtained by the AMADEUS collaboration in studying the low-energy K− interactions with light nuclei inducing single- and multi-nucleon absorptions, were reviewed. The investigation of K−-nucleons/nuclei interactions is fundamental for a better understanding of the non-perturbative quantum chromodynamics QCD in the strangeness sector.
By conducting studies on the K−n single nucleon absorption in 4He, it was possible to provide the first characterization of the non-resonant K−N → Yπ production below the
The AMADEUS Collaboration is currently completing studies of K− 4NA in the Λt golden channel and analyses related to K−p → Σ0 π0(Λ π0) cross section determination for kaon momentum below 100 MeV/c [53] which will provide additional new experimental constraints to the
Author contributions
The manuscript was initially drafted by MAS, and later edited and contributed to by CC and KP. AS, KP, RG, OV, and MIS carried out simulations and data analysis. The results were discussed and analyzed by all authors. All authors contributed to the article and approved the submitted version.
Funding
We acknowledge the Centro Ricerche Enrico Fermi—Museo Storico della Fisica e Centro Studi e Ricerche “Enrico Fermi,” for the Project PAMQ. Part of this work was supported by the Austrian Science Fund (FWF): [P24756-N20]; Austrian Federal Ministry of Science and Research BMBWK 650962/0001 VI/2/2009; the Croatian Science Foundation, Under Project 8570; Polish National Science Center through Grant No. UMO-2016/21/D/ST2/01155; the SciMat and qLife Priority Research Areas budget under the program Excellence Initiative–Research University at the Jagiellonian University; EU Horizon 2020 STRONG2020-No. 824093 Project.
Acknowledgments
We acknowledge the KLOE/KLOE-2 Collaboration for their support and for having provided us the data and the tools to perform the analysis presented in this paper.
Conflict of interest
The authors declare that the research was conducted in the absence of any commercial or financial relationships that could be construed as a potential conflict of interest.
Publisher’s note
All claims expressed in this article are solely those of the authors and do not necessarily represent those of their affiliated organizations, or those of the publisher, the editors and the reviewers. Any product that may be evaluated in this article, or claim that may be made by its manufacturer, is not guaranteed or endorsed by the publisher.
References
1. Skurzok M, Bazzi M, Bragadireanu M, Bosnar D, Cargnelli M, Clozza A, et al. Investigation of the low-energy K− hadronic interactions with light nuclei by AMADEUS. Int J Mod Phys E (2022) 31:08. doi:10.1142/S0218301322400018
2. Curceanu C, Piscicchia K, Bazzi M, Berucci C, Bosnar D, Bragadireanu A, et al. Unprecedented studies of the low-energy negatively charged kaons interactions in nuclear matter by AMADEUS. Acta Phys Polon B (2015) 46:203. doi:10.5506/APhysPolB.46.203
3. Del Grande R, Piscicchia K, Cargnelli M, Fabbietti L, Marton J, Moskal P, et al. On the K− absorptions in light nuclei by AMADEUS. Few-body Syst (2021) 62:1. doi:10.1007/s00601-020-01589-7
4. Bernard V, Kaiser N, Meisner UG. Aspects of chiral pion-nucleon physics. Nucl Phys A (1997) 615:483–500. doi:10.1016/S0375-9474(97)00021-3
5. Scherer S, Schindler MR. A primer for chiral perturbation theory. Lect Notes Phys (2012) 830:1–338. doi:10.1007/978-3-642-19254-8
6. Bazzi M, Beer G, Bombelli L, Bragadireanu A, Cargnelli M, Corradi G, et al. A new measurement of kaonic hydrogen X-rays. Phys Lett B (2011) 704:113–7. doi:10.1016/j.physletb.2011.09.011
7. Akaishi Y, Yamazaki T. Nuclear K− bound states in light nuclei. Phys Rev C (2002) 65:044005. doi:10.1103/PhysRevC.65.044005
8. Ikeda Y, Sato T. Strange dibaryon resonance in the K−NN - πYN system. Phys Rev C (2007) 76:035203. doi:10.1103/PhysRevC.76.035203
9. Wycech S, Green AM. Variational calculations for K−-few-nucleon systems. Phys Rev C (2009) 79:014001. doi:10.1103/PhysRevC.79.014001
10. Shevchenko NV, Gal A, Mares J. Faddeev calculation of a K−pp quasi-bound state. Phys Rev Lett (2007) 98:082301. doi:10.1103/PhysRevLett.98.082301
11. Révai J, Shevchenko NV. Faddeev calculations of the K¯NN system with chirally-motivated K¯N interaction. II. The K−pp quasibound state. Phys Rev C (2014) 90:034004. doi:10.1103/PhysRevC.90.034004
12. Maeda S, Akaishi Y, Yamazaki T. Strong binding and shrinkage of single and double an external file that holds a picture, illustration, etc. Object name is pjab-89-418-i001.jpg nuclear systems (K−pp, K−ppn, K−K−p and K−K−pp) predicted by Faddeev-Yakubovsky calculations. Proc Jpn Acad B (2013) 89:418–37. doi:10.2183/pjab.89.418
13. Akaishi Y, Yamazaki T. (K−, π−) production of nuclear K− bound states in proton-rich systems via Λ* doorways. Phys Lett B (2002) 535:70–6. doi:10.1016/S0370-2693(02)01738-0
14. Dote A, Hyodo T, Weise W. Variational calculation of the pp K− system based on chiral SU(3) dynamics. Phys Rev C (2009) 79:014003. doi:10.1103/PhysRevC.79.014003
15. Dote A, Inoue T, Myo T. Fully coupled-channel study of K−pp resonance in a chiral SU(3)-based potential. Phys Lett B (2018) 784:405–10. doi:10.1016/j.physletb.2018.08.029
16. Barnea N, Gal A, Liverts EZ. Realistic calculations of K¯NN, K¯NNN, and K¯K¯NN quasibound states. Phys Lett B (2012) 712:132–7. doi:10.1016/j.physletb.2012.04.055
17. Ikeda Y, Kamano H, Sato T. Energy dependence of FormulaN interactions and resonance Pole of strange dibaryons K¯N interactions and resonance Pole of strange dibaryons. Prog Theor Phys (2010) 124:533–9. doi:10.1143/PTP.124.533
18. Bicudo P. Large degeneracy of excited hadrons and quark models. Phys Rev D (2007) 76:094005. doi:10.1103/PhysRevD.76.094005
19. Bayar M, Oset E. The K¯NN system revisited including absorption. Nucl Phys A (2013) 914:349–53. doi:10.1016/j.nuclphysa.2013.02.005
20. Sekihara T, Oset E, Ramos A. On the structure observed in the in-flight 3He(K−, Λp)n reaction at J-PARC. Prog Theor Exp Phys (2016) 2016:123D03. doi:10.1093/ptep/ptw166
21. Workman RL, Burkert VD, Crede V, Klempt E, Thoma U, Tiator L, et al. Review of particle physics. Prog Theor Exp Phys (2022) 2022. doi:10.1093/ptep/ptac097
22. Maggiora M, Kienle P, Suzuki K, Yamazaki T, Alexeev M, Amoroso A, et al. DISTO data on K−pp. Nucl Phys A (2010) 835:43–50. doi:10.1016/j.nuclphysa.2010.01.173
23. Agnello M, Beer G, Benussi L, Bertani M, Bianco S, Botta E, et al. Evidence for a kaon-bound state K−pp produced in K− absorption reactions at rest. Phys Rev Lett (2005) 94:212303. doi:10.1103/PhysRevLett.94.212303
24. Suzuki T, Iio M, Itahashi K, Iwasaki M, Matsuda Y, Ohnishi H, et al. YN correlations from the stopped K− reaction on 4He. Mod Phys Lett (2008) A23:2520–3. doi:10.1142/S021773230802971X
25. Ajimura S, Asano H, Beer G, Berucci C, Bhang H, Bragadireanu M, et al. “K−pp”, a K‾ meson nuclear bound state, observed in 3He(K−,p)n reactions. Progr Theor Exp Phys (2018) 789:620–5. doi:10.1016/j.physletb.2018.12.058
26. Acharya S, Adamová D, Adhya S, Adler A, Adolfsson J, Aggarwal M, et al. Scattering studies with low-energy kaon-proton femtoscopy in proton-proton collisions at the LHC. Phys Rev Lett (2020) 124:092301. doi:10.1103/PhysRevLett.124.092301
27. Piscicchia K, Wycech S, Fabbietti L, Cargnelli M, Curceanu C, Del Grande R, et al. First measurement of the K−n → Λπ− non-resonant transition amplitude below threshold. Phys Lett B (2018) 782:339–45. doi:10.1016/j.physletb.2018.05.025
28. Hrtánková J, Mares J. K− nuclear states: Binding energies and widths. Phys Rev C (2017) 96:015205. doi:10.1103/PhysRevC.96.015205
29. Friedman E, Gal A. K−N amplitudes below threshold constrained by multi-nucleon absorption. Nucl Phys A (2017) 959:66–82. doi:10.1016/j.nuclphysa.2016.12.009
30. Del Grande R, Piscicchia K, Vazquez Doce O, Cargnelli M, Curceanu C, Fabbietti L, et al. K− multi-nucleon absorption cross sections and branching ratios in Lambda p and Sigma0 p final states. Eur Phys J C (2019) 79:190. doi:10.1140/epjc/s10052-019-6694-7
32. Bossi F, De Lucia E, Lee-Franzini J, Miscetti S, Palutan M, Collaboration K. Precision kaon and hadron physics with KLOE. Rivista Del Nuovo Cimento (2008) 31:531–623. doi:10.1393/ncr/i2008-10037-9
33. Gallo A, Alesini D, Biagini ME, Biscari C, Boni R, Boscolo M, et al. DAFNE status report. Conf Proc (2006) C060626:604–6.
34. Adinolfi M, Ambrosino F, Andryakov A, Antonelli A, Antonelli M, Bacci C, et al. The tracking detector of the KLOE experiment. Nucl Instrum Meth (2002) A488:51–73. doi:10.1016/S0168-9002(02)00514-4
35. Adinolfi M, Ambrosino F, Antonelli A, Antonelli M, Anulli F, Barbiellini G, et al. The KLOE electromagnetic calorimeter. Nucl Instrum Meth (2002) A482:364–86. doi:10.1016/S0168-9002(01)01502-9
36. Piscicchia K, Cargnelli M, Curceanu C, Del Grande R, Fabbietti L, Guaraldo C, et al. Low energy antikaon-nucleon/nuclei interaction studies by AMADEUS. Acta Phys Polon B Proc Suppl (2018) 11:609. doi:10.5506/aphyspolbsupp.11.609
37. Feijoo A, Magas V, Ramos A. S=1 meson-baryon interaction and the role of isospin filtering processes. Phys Rev C (2019) 99:035211. doi:10.1103/PhysRevC.99.035211
38. Cieply A, Smejkal J. Chirally motivated K¯N amplitudes for in-medium applications. Nucl Phys A (2012) 881:115–26. doi:10.1016/j.nuclphysa.2012.01.028
39. Ikeda Y, Hyodo T, Weise W. Chiral SU(3) theory of antikaon-nucleon interactions with improved threshold constraints. Nucl Phys A (2012) 881:98–114. doi:10.1016/j.nuclphysa.2012.01.029
40. Guo ZH, Oller JA. Meson-baryon reactions with strangeness -1 within a chiral framework. Phys Rev C (2013) 87:035202. doi:10.1103/PhysRevC.87.035202
41. Mai M, Meißner UG. Constraints on the chiral unitary
42. Piscicchia K, Wycech S, Curceanu C. On the K−4He → Λπ−3He resonant and non-resonant processes. Nucl Phys A (2016) 954:75–93. doi:10.1016/j.nuclphysa.2016.05.007
43. Feijoo A, Magas VK, Ramos A. The constraining effect of isospin filtering processes in low energy meson-baryon interactions. AIP Conf Proc (2019) 2130:040013. doi:10.1063/1.5118410
44. Fuchs C. Strangeness production in heavy ion reactions at intermediate energies. Prog Part Nucl Phys (2004) 53:113–24. doi:10.1016/j.ppnp.2004.02.023
45. Weise W. Topics in low-energy QCD with strange quarks. Hyperfine Interact (2015) 233:131–40. doi:10.1007/s10751-015-1129-9
46. Tolos L, Fabbietti L. Strangeness in nuclei and neutron stars. Progr Part Nucl Phys (2020) 112:103770. doi:10.1016/j.ppnp.2020.103770
47. Vázquez Doce O, Fabbietti L, Cargnelli M, Curceanu C, Marton J, Piscicchia K, et al. K− absorption on two nucleons and ppK− bound state search in the Σ0p final state. Phys Lett B (2016) 758:134–9. doi:10.1016/j.physletb.2016.05.001
48. Del Grande R, Piscicchia K, Wycech S. Formation of Σπ pairs in nuclear captures of K− mesons. Acta Phys Polon B (2017) 48:1881. doi:10.5506/APhysPolB.48.1881
49. Vander Velde-Wilquet C, Sacton J, Wickens JH, Tovee DN, Davis DH. Determination of the branching fractions for K− -meson absorptions at rest in carbon nuclei. II Nuovo Cimento A (1977) 39:538–47. doi:10.1007/bf02771028
50. Del Grande R, Piscicchia K, Cargnelli M, Curceanu C, Fabbietti L, Marton J, et al. Total branching ratio of the k− two-nucleon absorption in 12c. Phys Scripta (2020) 95:084012. doi:10.1088/1402-4896/ab9ed3
51. Katz P, Bunnell K, Derrick M, Fields T, Hyman LG, Keyes G. Reactions of K− stopping in helium. Phys Rev D (1970) 1(5):1267–76. doi:10.1103/physrevd.1.1267
52. Hrtánková J, Ramos A. Single- and two-nucleon antikaon absorption in nuclear matter with chiral meson-baryon interactions. Phys Rev C (2020) 101(3):035204. doi:10.1103/PhysRevC.101.035204
Keywords: strangeness, kaon absorption, antikaon interactions in nuclear matter, strong interaction, cross section
Citation: Skurzok M, Cargnelli M, del Grande R, Fabbietti L, Guaraldo C, Marton J, Moskal P, Piscicchia K, Scordo A, Silarski M, Sirghi DL, Vazquez Doce O, Zmeskal J, Wycech S, Branchini P, Czerwiński E, Kang X, Mandaglio G, Martini M, Selce A and Curceanu C (2023) A review of the low-energy K−-nucleus/nuclei interactions with light nuclei AMADEUS investigations. Front. Phys. 11:1237644. doi: 10.3389/fphy.2023.1237644
Received: 09 June 2023; Accepted: 29 June 2023;
Published: 31 July 2023.
Edited by:
Kanchan Khemchandani, Federal University of São Paulo, BrazilReviewed by:
Zilong Chang, Indiana University, United StatesCopyright © 2023 Skurzok, Cargnelli, del Grande, Fabbietti, Guaraldo, Marton, Moskal, Piscicchia, Scordo, Silarski, Sirghi, Vazquez Doce, Zmeskal, Wycech, Branchini, Czerwiński, Kang, Mandaglio, Martini, Selce and Curceanu. This is an open-access article distributed under the terms of the Creative Commons Attribution License (CC BY). The use, distribution or reproduction in other forums is permitted, provided the original author(s) and the copyright owner(s) are credited and that the original publication in this journal is cited, in accordance with accepted academic practice. No use, distribution or reproduction is permitted which does not comply with these terms.
*Correspondence: Magdalena Skurzok, magdalena.skurzok@uj.edu.pl