Development of fullerene acceptors and the application of non-fullerene acceptors in organic solar cells
- 1Center for Advanced Laser Technology, Hebei University of Technology, Tianjin, China
- 2Hebei Key Laboratory of Advanced Laser Technology and Equipment, Tianjin, China
Organic solar cells (OSCs) enjoy a huge market and public attention as an emerging renewable and clean energy source due to their accessibility, sustainability, translucency, good flexibility, non-toxicity, and low preparation cost. However, the choice of an acceptor material is now the pivotal factor restricting the development of OSCs. To continuously improve stability and increase power conversion efficiency (PCE) for better performance, high-performance acceptor materials are an important part of enabling OSCs. From fullerenes and their derivatives and non-fullerenes, we sum up the latest research progress on high-performance acceptor materials for OSCs and then introduce the synthesis methods of non-fullerenes. The latest strategies to enhance the performance of organic solar cells and the wide range of applications of non-fullerene acceptors (NFAs) on different OSCs are also discussed. In addition, challenges faced by OSCs in improving their performance and the prospects for future development are revealed, providing new ideas for designing the next generation of high-performance OSCs.
1 Introduction
Solar energy has gradually become a widely used sustainable, renewable, and easily accessible clean energy for resolving the issue of energy shortage shared by the whole world [1]. Solar cells for generating electricity from solar energy have also been around for decades, gradually deriving from the first generation into various forms, including dye-sensitized solar cells, thin-film solar cells, and OSCs [2]. OSCs may turn into the most inexpensive electric power form as the power conversion efficiency (PCE) of them continues to rise [3]. The characteristics of OSCs include semitransparency, non-toxicity, good flexibility, and low preparation cost, fit for roll-to-roll massive processing, so they have received worldwide attention [4]. Based on these advantages, OSCs are also used in many applications, including sensors, architecture, the Internet of Things (IoT), and some wearable electronics, with considerable promise [5].
However, OSCs also have a number of issues and challenges that seriously affect the commercialization and wider application of them, which include the complexity of the synthesized materials, poor stability, low PCE, poor performance, great sensitivity to processing conditions, and overall performance that can significantly be degraded by changes in thickness [6]. Moreover, the progress of appropriate electron acceptors is highly concerned with improving PCE (B. [7,8]). Therefore, to boost the performance of OSCs, the selection of acceptor materials is crucial, and then, it can be said that the key to solving the OSC performance problem lies in the selection of high-performance acceptor materials [9]. Additionally, machine learning can play an auxiliary role in identifying high-performance materials [10]. It is able to predict the parameters that affect the performance of OSCs, so as to screen high-performance small molecule acceptor materials [11]. In addition, the choice of solvent can also affect the performance, and machine learning can predict the solubility to select a more suitable solvent [12].
High-performance acceptor materials can significantly advance the stability and PCE of OSCs, which in turn improves the overall performance and enables OSCs to be developed in the longer term [13]. Therefore, research on high-performance acceptor materials for OSCs is essential. We introduce the development and the primary materials of OSCs and emphasize the progress of high-performance acceptor materials for OSCs from the perspectives of fullerenes and their derivatives and non-fullerenes in this mini-review. In addition, we discuss in depth the typical applications of high-performance acceptor materials in OSCs. Ultimately, we look ahead to the foreground and challenges of high-performance acceptor materials for OSCs.
2 Development of OSCs
OSCs (Figure 1A) have grown by leaps and bounds, with PCEs of single-junction OSCs achieving almost 20%, and the important reasons for this progress are device engineering and novel materials since the 21st century [14]. In terms of novel materials, recent research in organic solar energy includes single-component OSCs, water- or alcohol-soluble interface materials, non-fused and fused ring acceptors, and polymer acceptors [15]. The novel materials include interlayers and active layer materials (Figure 2B), where active layer materials include electron acceptor and electron donor materials [16]. The donor materials mainly include symmetric D–A–D (or A–D–A structure) and an asymmetric electron push–pull molecule [17]. Acceptor materials mainly include fullerene derivative acceptors and small molecule non-fullerene acceptors (NFAs) (Figure 2A) [4]. Additionally, a variety of OSCs can also be constituted by the combination of acceptor and donor materials, including polymer–small molecule, polymer–fullerene, and all-polymer–all-small molecule [18]. The efficient photovoltaic performance of OSCs depends on the active layer morphology control, device engineering, and compatibility between acceptor and donor materials [13].
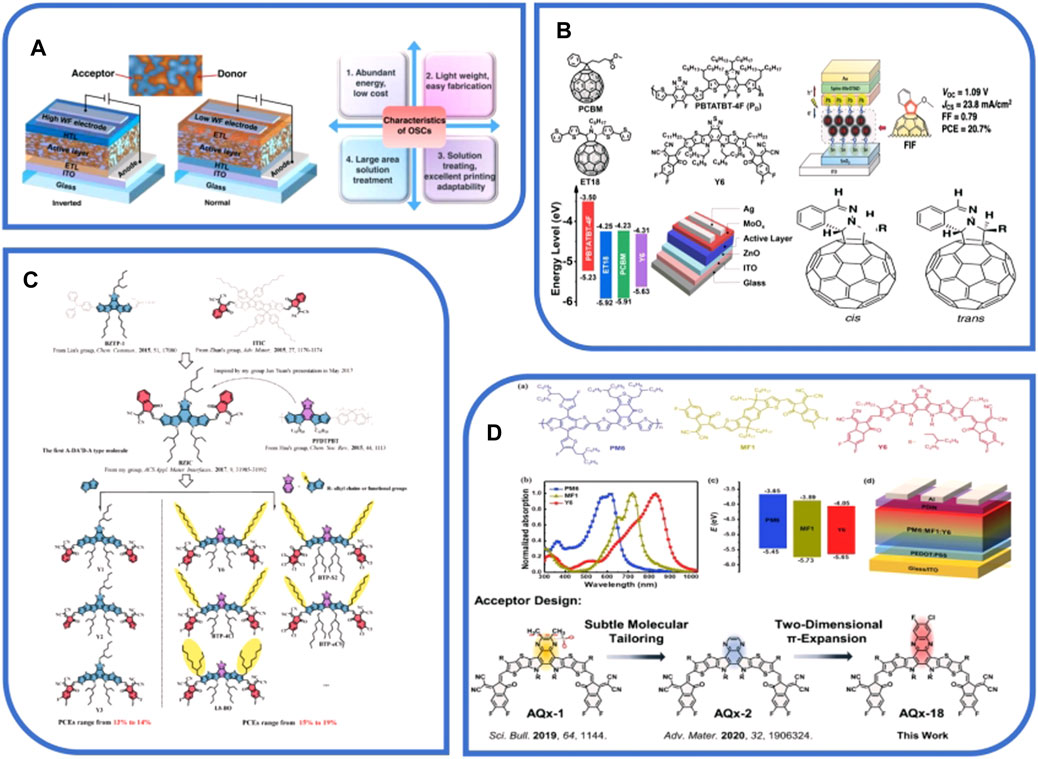
FIGURE 1. (A) Development, working principle, structure, and characteristics of organic solar cells, reproduced with permission from [28]. (B) Structure and research of fullerenes and their derivatives, reproduced with permission from [20,21,25]. (C) Progress of A-DA'D-A-type non-fullerene small molecule acceptors, reproduced with permission from [36]. (D) Solar cells based on non-fullerene acceptors and methods for improving performance, reproduced with permission from [9,47].
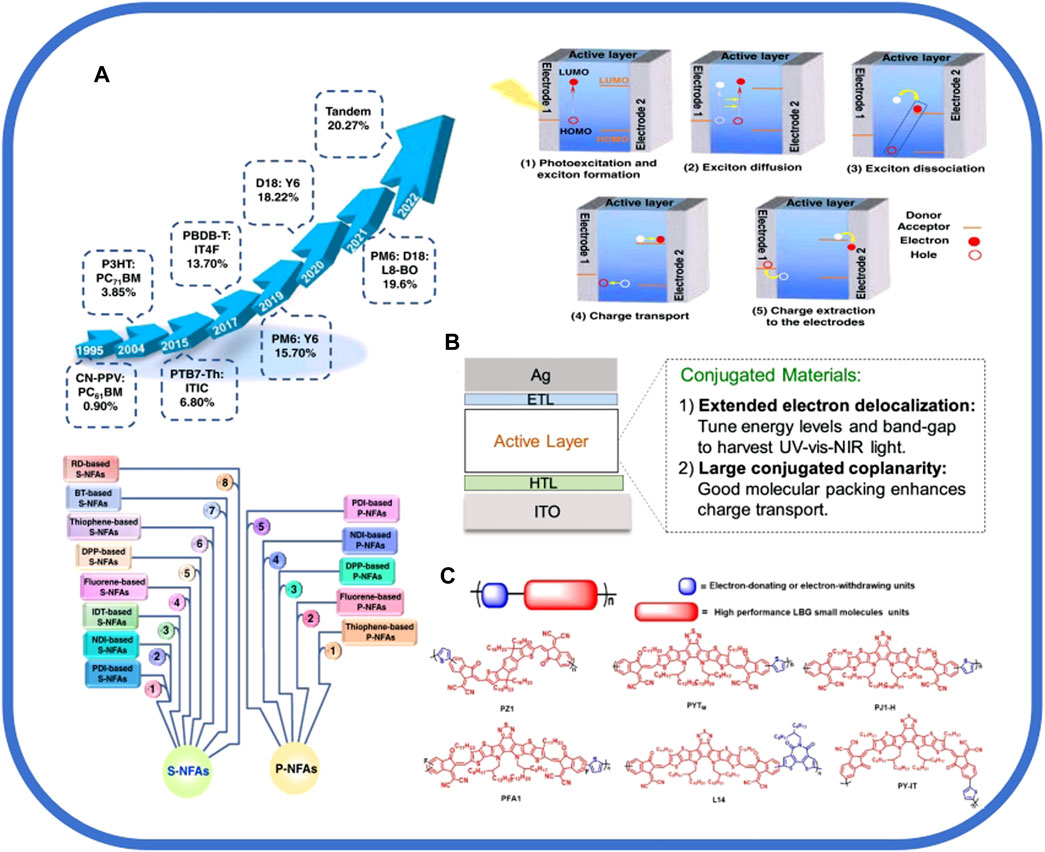
FIGURE 2. (A) Classification of NFAs and the development and working principle of OSCs, reproduced with permission from [28]. (B)Device structure of OSCs and merits of conjugated materials used for the active layer, reproduced with permission from [7]. (C) Structures of polymer NFAs containing SMA segments, reproduced with permission from [19].
3 High-performance acceptor materials for OSCs
3.1 Fullerenes and derivatives
The era of fullerenes and derivatives (Figure 1B) in OPV applications officially began in 1995; in 10 years, fullerene derivatives were developed in fields such as molecular electronics and perovskite solar cells, which are now in an urgent need for fullerene derivatives with new properties; therefore, it is necessary to carry out and study the brand new device engineering of fullerene derivatives and design strategies (J [19]). Lithium-ion inner surface fullerenes, doped with organic semi-conducting molecules and carbon nanotubes (CNTs), can enhance stability and PCE [20]. Mumyatov et al. synthesized two new types of soluble bis(pyrrolidino)[60]fullerenes, which have reduced electron affinity compared to normal fullerenes. Therefore, they can increase the open-circuit voltage by nearly 200 mV and have the ability to suppress the photooxidation of conjugated polymers. These properties can be useful for the research of time-proof and high-efficiency organic photovoltaic devices [21]. In the same year, the team also discovered that pyrrolidino [2,1-a]phthalazino[60]fullerenes, which have tunable optoelectronic properties and are easy to prepare, are very promising organic electronics and photovoltaics, which can have a power conversion efficiency of more than 14% if used as electron-transport materials, and the reactions involved provide a completely new idea for the subsequent preparation of a wider variety of fullerene derivatives [22].
In addition to the use in improving the efficacy of optical devices, fullerenes and derivatives play a huge role in ternary OSCs. Ternary OSCs are made from donor polymers blended with different mixtures of fullerene acceptors, which have the best light-absorbing and electron acceptor properties and, thus, can often be used as materials for solar cells and photodetectors [23]. Fullerene-based acceptors in ternary blends with specially designed structures can enhance the photostability of NFAs and also conjugated polymers [24]. The stability of the cells can be improved in large measures if two fullerene acceptors, namely, PCBM and ET18, are used as the third component of OSCs, which are based on the P-D:Y6 blends [25]. Fullerene derivatives, as the third component of OSCs, can improve the power conversion efficiency and tune the blend morphology, which is an ideal material for efficient OSCs that can be realized by finely tuning the morphology. The morphology of the active layer can be tuned more accurately and the photovoltaic performance can be improved as well, if IC60BA is brought in the PTZ1:IDIC hybrid system as a kind of fullerene derivative [26].
3.2 Non-fullerene
NFAs are generally composed of electron-deficient and electron-rich units. The effect between these units can modulate the energy levels of acceptor materials, adjust the morphology of films, and broaden the absorption of light. Meanwhile, the performance of OSCs which are based on NFAs is continuously increasing [27]. As they can improve the stability and the PCE of OSCs and broaden the absorption range, NFAs have been widely considered for use by the public [28]. Among them, Y6, as a non-fullerene acceptor molecule, has gradually appeared in the public view and has already played a great role in various aspects of OSCs, enabling single-junction OSCs to achieve efficiencies of more than 15% (X [29]). However, Y-series non-fullerenes still have many problems; for example, their relative dissolvability in non-halogenated green solvents is poor. This problem can be addressed by using non-chlorinated solvents to dissolve large-heterojunction photoreactive layers which are based on Y-series NFAs, as well as realizing environment-friendly manufacturing [30].
There are many ways to synthesize NFAs, among which side-chain engineering is a very promising method, which can be used to improve the crystallinity and solubility of NFAs and then increase the PCE of OSCs. Using side-chain engineering, a brand new acceptor, IT-BnC6-4F, was developed, which has a higher crystallinity and a broader absorption spectrum, and it has a PCE of 13.36% [31]; Tian et al. investigated the application of PSMA and SMA (Figure 2C) as Y-series NFAs in non-fullerene OSCs and pointed out the challenges it may encounter in side chain regulation for Y-series NFAs with possible strategies to deal with them [32]. In addition to side-chain engineering, there is another synthetic method capable of achieving highly efficient performance of non-fullerene acceptors, which is replacing the beta position of the thiophene unit on the Y6-based dithienothiophen[3,2-b]-pyrrolobothiadiazole core by a branched alkyl chain. The whole method is capable of radically changing the behavior of the molecule stacking in the non-fullerene acceptors, and then, it improves the charge transport and structural order of the films [33]. High-performance synthesis strategies also include the construction of NFAs in an asymmetric configuration with different heteroatoms added to the bay position, and the asymmetric perylene diimide (PDI)-based acceptor PDI2Se–FT, which is introduced with both Se and S atoms, is able to exhibit excellent performance. Additionally, its maximum PCE can reach 6.96% [34].
There are also many competitive non-fullerene acceptor materials, among which the NFA PDI has high stability and low material cost, and in these years, the performance of OSC devices has taken a remarkable step forward, which are based on monomeric PDI acceptors, with PCEs up to more than 12%, and current research targeting monomeric PDI acceptors is working toward addressing the barriers of fill-factor values and moderate–short current density (J(sc)) [35]. There is also a non-fullerene A-DA'D-A-type acceptor (Figure 1C), with an absorption spectrum complementary to the donor and good transport properties, mainly due to its fusion backbone centered on a trapezoidal electron-deficient nucleus [36]. In addition, NFAs sealed by a strong electron-deficient group and based on indacenodithiophene (IDT) are also advantageous in many ways. The advantages are strong absorbency and good energy level arrangement in visible regions and near-infrared (NIR) regions and so on [37]. UF-Qx-2Cl and UF-Qx-2F are two unfused non-fullerene acceptors, which are centered on the electron-deficient quinoxaline (Qx) unit. The synthesis of the unfused NFA is also of great importance in advancing the stability of OSCs, reducing preparation costs, and realizing their performance, and at the same time, this has also broadened the prospect in the commercial application of OSCs [38].
4 Applications
High-performance acceptor materials, especially NFAs, have developed rapidly and are widely used in different forms in different OSCs, which have become a major research hotspot. Among them, small molecule NFAs are gradually incorporated into the relevant research field, which can improve the optoelectronic properties of polymer molecules and, thus, bring OSCs into full commercialization [9]. NFAs NTIC-4Cl and NTIC-4F for OSCs have the advantage of low preparation cost because they contain naphtho[1,2-c:5,6-c ']bis([1,2,5]thiadiazole) moieties. The chlorinated and fluorinated dicyanomethylidene-indan-1-one units are also used as the flanking end-groups [39]. In addition to individual non-fullerene acceptors, multiple acceptors can be integrated, such as the integration of NFAs L8BO and Y6 and the wide-bandgap electron-donating polymer PTzBI-dF, and the OSC can achieve 18.26% efficiency, based on its structure [40]. Non-fullerene acceptors also play a very important role in the photocurrent of dilute donor organic solar cells (DDOSCs). The photocurrent of DDOSCs based on non-fullerene acceptors is more affected compared to the photocurrent of DDOSCs based on fullerene acceptors, mainly because non-fullerene acceptors are more capable of modifying charge transport and generation, which in turn affects the Jsc of DDOSCs [41].
With the rapid development of high-performance NFAs, non-fullerene-based OSCs are no longer satisfied with small areas on a laboratory scale, and increasing attention is gradually shifted to large-area OSCs, which are based on NFAs. Xue et al. also proposed a feasible design scheme in terms of related equipment development, device component processing strategies, and manufacturing technologies [42]. However, this process will inevitably have the risk of loss of efficiency, so for the purpose of enhancing the efficiency of OSCs and better utilizing the high-efficiency photovoltaic performance of OSCs, it is necessary to apply some new design methods and strategies (Figure 1D). The appearance of cathode interface engineering can improve the charge collection and transfer within OSCs and, thus, boost its PCE (H [43]). The bimolecular recombination rates and charge transfer of high-performance OSCs based on NFAs can also be investigated by the drift diffusion simulations of voltage–current peculiarities in light and steady-state dark injection measurements, concluding that the inhibition of bimolecular recombination has a strong impact on the performance and high filling coefficient of OSCs [44]. In addition, the structure of non-fullerene receptors can also be analyzed and modified. The performance–structure relationship of high-performance Y6-based non-fullerene acceptors from both phase separation and crystal structure perspectives can be constructed to morphologically optimize OSCs which are based on Y6 NFAs to promote higher power conversion efficiencies [45]. Nowadays, there are many difficulties in the development of highly efficient low-bandgap NFAs for high-performance OSCs; in order to solve this problem, it has been attempted to modify the end caps of the novel acceptor molecule (IDT-ED-4F), and the modified molecule can effectively facilitate the application of high-performance OSCs; finally, a new acceptor material JA1-JA4 can be obtained [46]. In addition, 2D p-expansion strategies can be used to fine-tune the crystalline behaviors and electronic structures of NFAs to construct binary OSCs based on AQx-18, which can improve J(sc), filling factor, and V-oc and enable OSCs to perform better, with PCE reaching 18.2% [47].
5 Conclusion and outlook
The PCE of OSCs has continued to progress and develop in the history of decades, and it can basically reach 18%. This has been achieved due to high-performance acceptor materials for OSCs, in which fullerenes and their derivatives have been used in many optical devices, especially in ternary OSCs. Fullerenes and their derivatives acting as a third component can increase their stability and performance. The non-fullerene acceptor materials, represented by the Y6 acceptor molecule in the Y series, are gradually taking the place of fullerenes and their derivatives due to better properties. Side-chain engineering is a very typical method for synthesizing NFAs, and it can influence the degree of acceptor crystallinity and the PCE of OSCs. OSCs that are based on NFAs are also developing rapidly, and the performance can be improved by just changing the internal mechanism of the cell or modifying the structure of the non-fullerene acceptor. For a gradual breakthrough in PCE, improved performance of OSCs, and design reference for next-generation high-performance OSCs, more in-depth research is still needed to create new types of acceptors with higher performance in terms of materials or structures, and more profound research is needed to study the internal operation mechanism of OSCs.
Author contributions
W-SD: writing–original draft, investigation, and resources. GW: resources, supervision, and writing–review and editing. Y-FL: funding acquisition, supervision, and writing–review and editing. YY: funding acquisition, resources, and writing–review and editing.
Funding
The authors declare that financial support was received for the research, authorship, and/or publication of this article. This work was supported by the 173 Project Technical Fund [grant number JSLY-16-B16006]; the Central Government Guides Local Funds for Scientific and Technological Development [grant number 236Z1813G]; the Natural Science Research Foundation of Hebei University of Technology [grant number JBKYXX2203]; the Funding Projects for the Introduction of Overseas Staff of Hebei Province [grant number C20210334]; the National Natural Science Foundation of China [grant numbers 62075056]; the Natural Science Foundation of Hebei Province [grant numbers F2022202035].
Conflict of interest
The authors declare that the research was conducted in the absence of any commercial or financial relationships that could be construed as a potential conflict of interest.
Publisher’s note
All claims expressed in this article are solely those of the authors and do not necessarily represent those of their affiliated organizations, or those of the publisher, the editors, and the reviewers. Any product that may be evaluated in this article, or claim that may be made by its manufacturer, is not guaranteed or endorsed by the publisher.
References
1. Lye Y-E, Chan K-Y, Ng Z-N. A review on the progress, challenges, and performances of tin-based perovskite solar cells. Nanomaterials (2023) 13(3):585. doi:10.3390/nano13030585
2. Soonmin H, Hardani P, Nandi P, Mwankemwa BS, Malevu TD, Malik MI. Overview on different types of solar cells: an update. Appl Sciences-Basel (2023) 13(4):2051. doi:10.3390/app13042051
3. Shoaee S, Sanna AL, Sforazzini G. Elucidating charge generation in green-solvent processed organic solar cells. Molecules (2021) 26(24):7439. doi:10.3390/molecules26247439
4. Li Y, Huang W, Zhao D, Wang L, Jiao Z, Huang Q, et al. Recent progress in organic solar cells: a review on materials from acceptor to donor. Molecules (2022) 27(6):1800. doi:10.3390/molecules27061800
5. Ghorab M, Fattah A, Joodaki M. Fundamentals of organic solar cells: a review on mobility issues and measurement methods. Optik (2022) 267:169730. doi:10.1016/j.ijleo.2022.169730
6. Ansari MA, Ciampi G, Sibilio S. Tackling efficiency challenges and exploring greenhouse-integrated organic photovoltaics. Energies (2023) 16(16):6076. doi:10.3390/en16166076
7. Fan B, Gao H, Jen AKY. Biaxially conjugated materials for organic solar cells. Acs Nano (2023) 18(1):136–54. doi:10.1021/acsnano.3c11193
8. Jaemin K, Sang-Yong N. Electron acceptors in organic solar cells. Appl Chem Eng (2022) 33(2):119–25. doi:10.14478/ace.2021.1110
9. Mola GT, Ahmed AYA, Ike JN, Liu M, Hamed MSG, Zhang Y. Engineering non-fullerene acceptors as a mechanism to ControlFilm morphology and energy loss in organic solar cells. Energy and Fuels (2022) 36(9):4691–707. doi:10.1021/acs.energyfuels.2c00462
10. Mahmood A, Irfan A, Wang JL. Machine learning and molecular dynamics simulation-assisted evolutionary design and discovery pipeline to screen efficient small molecule acceptors for PTB7-Th-based organic solar cells with over 15% efficiency. J J Mater Chem A (2022) 10(No.8):4170–80. doi:10.1039/d1ta09762h
11. Mahmood A, Irfan A, Wang J. Machine learning for organic photovoltaic polymers: a minireview. J Chin J Polym Sci (English Edition) (2022) 40(No.8):870–6. doi:10.1007/s10118-022-2782-5
12. Asif Mahmood YS, Wang J-L, Wang JL. Easy and fast prediction of green solvents for small molecule donor-based organic solar cells through machine learning. J Phys Chem Chem Phys : PCCP (2023) 25(No.15):10417–26. doi:10.1039/d3cp00177f
13. Liu J, Qiu L, Shao S. Emerging electronic applications of fullerene derivatives: an era beyond OPV. J Mater Chem C (2021) 9(45):16143–63. doi:10.1039/d1tc04038c
14. Liu Y, Li B, Ma C-Q, Huang F, Feng G, Chen H, et al. Recent progress in organic solar cells (Part I material science). Sci China-Chemistry (2022) 65(2):224–68. doi:10.1007/s11426-021-1180-6
15. Liu Y, Liu B, Ma C-Q, Huang F, Feng G, Chen H, et al. Recent progress in organic solar cells (Part II device engineering). Sci China-Chemistry (2022) 65(8):1457–97. doi:10.1007/s11426-022-1256-8
16. Ouyang H, Han L, Liang Y, Zhou D. Research progress on nonfullerene acceptor material for OSCs. New Chem Mater (2022) 50(8):48–56. doi:10.19817/j.cnki.issn1006-3536.2022.08.010
17. Fan H, Altan B, Ning J, Tana , Tegusi . Research progress of solution-processable symmetric A-D-A small molecule donor materials for organic solar cells. Mater Rev (2022) 36(5B). doi:10.11896/cldb.20070075
18. Yi J, Zhang G, Yu H, Yan H. Advantages, challenges and molecular design of different material types used in organic solar cells. Nat Rev Mater (2024) 9(1):46–62. doi:10.1038/s41578-023-00618-1
19. Liu W, Xu X, Yuan J, Leclerc M, Zou Y, Li Y. Low-bandgap non-fullerene acceptors enabling high-performance organic solar cells. Acs Energ Lett (2021) 6(2):598–608. doi:10.1021/acsenergylett.0c02384
20. Matsuo Y. Creation of highly efficient and durable organic and perovskite solar cells using nanocarbon materials. Bull Chem Soc Jpn (2021) 94(3):1080–9. doi:10.1246/bcsj.20200404
21. Mumyatov AV, Chernyak AV, Elnaggar MM, Kuznetsov PM, Shestakov AF, Troshin PA. Pyrrolidino 2,1-a phthalazino 60 fullerenes: a new family of fullerene derivatives for photovoltaic applications. Physica Status Solidi-Rapid Res Lett (2021) 15(8). doi:10.1002/pssr.202100181
22. Mumyatov AV, Prudnov FA, Sagdullina DK, Martynov IV, Inasaridze LN, Chernyak AV, et al. Bis(pyrrolidino) 60 fullerenes: promising photostable fullerene-based acceptors suppressing light-induced absorber degradation pathways. Synth Met (2021) 271:116632. doi:10.1016/j.synthmet.2020.116632
23. Sperlich A, Auth M, Dyakonov V. Charge transfer in ternary solar cells employing two fullerene derivatives: where do electrons go? Isr J Chem (2022) 62(7-8). doi:10.1002/ijch.202100064
24. Mumyatov AV, Troshin PA. A review on fullerene derivatives with reduced electron affinity as acceptor materials for organic solar cells. Energies (2023) 16(4):1924. doi:10.3390/en16041924
25. Trippodo E, Campisciano V, Feng L-W, Chen Y, Huang W, Alzola JM, et al. Air-stable ternary organic solar cells achieved by using fullerene additives in non-fullerene acceptor-polymer donor blends. J Mater Chem C (2023) 11(24):8074–83. doi:10.1039/d2tc04971f
26. Guo B, Li W, Guo X, Li G, Meng X, Ma W, et al. Fine-tuned active layer morphology for bulk heterojunction organic solar cells with indene-C60 bisadduct as a third component. ACS Appl Mater Inter (2023) 15:58693–9. doi:10.1021/acsami.3c13823
27. Lu B, Zhang Z, Wang J, Cai G, Wang J, Yuan X, et al. Nonfullerene electron acceptors with electron-deficient units containing cyano groups for organic solar cells. Mater Chem Front (2021) 5(15):5549–72. doi:10.1039/d1qm00558h
28. Zhou D, Wang J, Xu Z, Xu H, Quan J, Deng J, et al. Recent advances of nonfullerene acceptors in organic solar cells. Nano Energy (2022) 103:107802. doi:10.1016/j.nanoen.2022.107802
29. Xu X, Li K, Wei Q, Yuan J, Zou Y. Organic solar cells based on Non.Fullerene small molecular acceptor Y6. Prog Chem (2021) 33(2):165–78. doi:10.7536/pc201016
30. Liu K, Jiang Y, Liu F, Ran G, Huang F, Wang W, et al. Organic solar cells with over 19% efficiency enabled by a 2D-conjugated non-fullerene acceptor featuring favorable electronic and aggregation structures. Adv Mater (2023) 35(32). doi:10.1002/adma.202300363
31. Man Y, Ye L, Cai Y, Sun X, Sun Y. Benzyl side-chain engineering of non-fullerene acceptors for efficient organic solar cells. Dyes Pigm (2021) 195:109706. doi:10.1016/j.dyepig.2021.109706
32. Tian L, Liu C, Huang F. Recent progress in side chain engineering of Y-series non-fullerene molecule and polymer acceptors. Sci China-Chemistry (2023). doi:10.1007/s11426-023-1774-6
33. Li C, Zhou J, Song J, Xu J, Zhang H, Zhang X, et al. Non-fullerene acceptors with branched side chains and improved molecular packing to exceed 18% efficiency in organic solar cells. Nat Energ (2021) 6(6):605–13. doi:10.1038/s41560-021-00820-x
34. Wang L, Shen H, You X, Wu D, Xia J. The synthesis of asymmetric perylene diimide acceptors and their optoelectronic properties studies. Eur J Org Chem (2022) 2022(3). doi:10.1002/ejoc.202101554
35. Zhang B, Bonner JC, Xu W, Piper RT, Murthy LNS, Hsu JWP. Contributions of charge generation versus transport to photocurrent in dilute-donor organic solar cells with non-fullerene acceptors. J Phys Chem C (2022) 126(49):20793–9. doi:10.1021/acs.jpcc.2c07821
36. Yuan J, Zou Y. The history and development of Y6. Org Elect (2022) 102:106436. doi:10.1016/j.orgel.2022.106436
37. Khlaifia D, Ettaghzouti T, Chemek M, Alimi K. Indacenodithiophene (IDT) and indacenodithienothiophene (IDTT)-based acceptors for non-fullerene organic solar cells. Synth Met (2021) 274:116736. doi:10.1016/j.synthmet.2021.116736
38. Chang M, Zhang Y, Lu B-S, Sui D, Wang F, Wang J, et al. The design of quinoxaline based unfused non-fullerene acceptors for high performance and stable organic solar cells. Chem Eng J (2022) 427:131473. doi:10.1016/j.cej.2021.131473
39. Li Y, Zhang D, Huang Z, Zhang T, Zheng N, Peng F, et al. Design and synthesis of non-fused non-fullerene acceptors containing naphthobisthiadiazole for organic solar cells. J Mater Chem C (2023) 11(44):15426–34. doi:10.1039/d3tc01742g
40. An K, Zhong W, Peng F, Deng W, Shang Y, Quan H, et al. Mastering morphology of non-fullerene acceptors towards long-term stable organic solar cells. Nat Commun (2023) 14(1):2688. doi:10.1038/s41467-023-38306-x
41. Zhang L, Chen Z, Sun F, Wang Y, Bao H, Gao X, et al. Progress of monomeric perylene diimide derivatives as non-fullerene acceptors for organic solar cells. J Electron Mater (2022) 51(8):4224–37. doi:10.1007/s11664-022-09728-y
42. Xue P, Cheng P, Han RPS, Zhan X. Printing fabrication of large-area non-fullerene organic solar cells. Mater Horizons (2022) 9(1):194–219. doi:10.1039/d1mh01317c
43. Xu H, Zhang Z, Li J. Progress in cathode interface engineering of organic solar cells based on non-fullerene acceptor. Electron Components Mater (2022) 41(12):1259–71. doi:10.14106/j.cnki.1001-2028.2022.0388
44. Wu Y, Li Y, van der Zee B, Liu W, Markina A, Fan H, et al. Reduced bimolecular charge recombination in efficient organic solar cells comprising non-fullerene acceptors. Scientific Rep (2023) 13(1):4717. doi:10.1038/s41598-023-31929-6
45. Zhu L, Zhang M, Zhong W, Leng S, Zhou G, Zou Y, et al. Progress and prospects of the morphology of non-fullerene acceptor based high-efficiency organic solar cells. Energ Environ Sci (2021) 14(8):4341–57. doi:10.1039/d1ee01220g
46. Zeshan MB, Sultana N, Tariq MI, Jamil S, Janjua MRSA. Physicochemical insights and in silico designing of new fullerene-free acceptor molecules for highly efficient and stable organic solar cells. J Phys Chem Sol (2022) 169:110842. doi:10.1016/j.jpcs.2022.110842
Keywords: organic solar cells, fullerenes and derivatives, non-fullerene acceptors, power conversion efficiency, high performance
Citation: Du W-S, Wang G, Li Y-F and Yu Y (2024) Development of fullerene acceptors and the application of non-fullerene acceptors in organic solar cells. Front. Phys. 12:1378909. doi: 10.3389/fphy.2024.1378909
Received: 30 January 2024; Accepted: 12 February 2024;
Published: 26 February 2024.
Edited by:
Teng Zhang, Beijing Institute of Technology, ChinaReviewed by:
Asif Mahmood, Beijing Institute of Technology, ChinaCopyright © 2024 Du, Wang, Li and Yu. This is an open-access article distributed under the terms of the Creative Commons Attribution License (CC BY). The use, distribution or reproduction in other forums is permitted, provided the original author(s) and the copyright owner(s) are credited and that the original publication in this journal is cited, in accordance with accepted academic practice. No use, distribution or reproduction is permitted which does not comply with these terms.
*Correspondence: Gong Wang, wanggong@hebut.edu.cn; Yun-Fei Li, yfli@hebut.edu.cn