Robotic locomotor training for spasticity, pain, and quality of life in individuals with chronic SCI: A pilot randomized controlled trial
- 1Department of Human Biology, Physical Activity, Lifestyle and Sport Research Centre (HPALS), University of Cape Town, Cape Town, South Africa
- 2Department of Sport Management, Cape Peninsula University of Technology, Cape Town, Western cape, South Africa
- 3Institute of Sport and Exercise Medicine, Faculty of Medicine and Health Sciences, Stellenbosch University, Tygerberg Campus, Cape Town, Western cape, South Africa
- 4International Olympic Committee Research Center, IOC Research Center, Cape Town, South Africa
Objective: The prevention and treatment of secondary complications is a key priority for people with spinal cord injury and a fundamental goal of rehabilitation. Activity-based Training (ABT) and Robotic Locomotor Training (RLT) demonstrate promising results for reducing secondary complications associated with SCI. However, there is a need for increased evidence through randomized controlled trials. Therefore, we aimed to investigate the effect of RLT and ABT interventions on pain, spasticity, and quality of life in individuals with spinal cord injuries.
Methods: Participants with chronic motor incomplete tetraplegia (n = 16) were recruited. Each intervention involved 60-minute sessions, 3× per week, over 24-weeks. RLT involved walking in an Ekso GT exoskeleton. ABT involved a combination of resistance, cardiovascular and weight-bearing exercise. Outcomes of interest included the Modified Ashworth Scale, the International SCI Pain Basic Data Set Version 2, and the International SCI Quality of Life Basic Data Set.
Results: Neither intervention altered symptoms of spasticity. Pain intensity increased from pre-post intervention for both groups, with a mean increase of 1.55 [−0.82, 3.92] (p = 0.03) and 1.56 [−0.43, 3.55] (p = 0.02) points for the RLT and ABT group, respectively. The ABT group had an increase in pain interference scores of 100%, 50%, and 109% for the daily activity, mood, and sleep domain, respectively. The RLT group had an increase in pain interference scores of 86% and 69% for the daily activity and mood domain respectively, but no change in the sleep domain. The RLT group had increased perceptions of quality of life with changes of 2.37 [0.32, 4.41], 2.00 [0.43, 3.56] and 0.25 [−1.63, 2.13] points, p = 0.03, for the general, physical, and psychological domains, respectively. The ABT group had increased perceptions of general, physical and psychological quality of life with changes of 0.75 [−1.38, 2.88], 0.62 [−1.83, 3.07] and 0.63 [−1.87, 3.13] points, respectively.
Conclusions: Despite increased pain ratings and no change in symptoms of spasticity, there was an increase in perceived quality of life for both groups over 24-weeks. This dichotomy warrants additional investigation in future large-scale randomized controlled trials.
1. Introduction
The complex and widespread physiological consequences of a spinal cord injury (SCI) and the associated physical inactivity can lead to increased risk of secondary health complications (1–3). Both spasticity and chronic pain are common secondary complications, affecting approximately 80% of individuals with SCI (1, 4–8). Spasticity and pain are rated as some of the most debilitating secondary consequences of SCI, causing significant physical disability, restricting independence and activities of daily living (ADLs), and reducing quality of life (QoL) (1, 4, 8–10). Additionally, these secondary complications are a frequent cause of morbidity and mortality for people with SCI and can lead to increased rates of rehospitalization, increased medical costs, loss of employability and social engagement with resultant decreased psychological well-being (1, 2, 11).
Therefore, the prevention and treatment of secondary complications is a key priority for people with SCI and a fundamental goal of SCI rehabilitation (1, 12). Regular engagement in physical activity can attenuate the risk of developing secondary complications in the SCI population (8, 10). Activity-based Training (ABT) utilises regular standing, aerobic, and resistance exercises to aid in the prevention and management of secondary complications (13–15). There is preliminary evidence that an alternative exercise therapy, Robotic Locomotor Training (RLT), also has beneficial effects on spasticity and pain in this population (16, 17). Although these results are promising, continued experimental research is required to study the extent to which RLT mitigates comorbidity risk, so that exercise guidelines can be developed to prevent these conditions and improve overall QoL (3). Thus, this study aimed to determine the effects of RLT compared to conventional ABT on spasticity, pain and QoL experienced by individuals with SCI during a pilot randomized controlled trial.
2. Materials and methods
2.1. Participants
This study is a secondary analysis from a pilot RCT, for which information on recruitment, adherence, methods and sample size determination have previously been reported and published (18, 19). A total of 17 participants with chronic [>1 year] traumatic, motor incomplete tetraplegia were recruited and assigned via random number generation to the RLT or ABT intervention groups (Figure 1). Detailed inclusion and exclusion criteria are contained in Supplementary Table S1. Each participant provided written informed consent prior to the study. The study was approved by the XX Human Research Ethics Committee (Ref: XX) and has been registered on the XX Clinical Trials Registry (XX). A post-trial care period of three months was implemented after participants finished the intervention, with continued access to rehabilitation equipment and medical professionals provided.
2.2. Rehabilitation interventions
An overview of the testing timeline, procedures and intervention has been previously described (19). Both interventions consisted of three sessions per week, 60-minutes each, for 24-weeks and were overseen by trained exercise therapists. RLT involved solely walking in an Ekso® GT exoskeleton [Ekso Bionics, Richmond, CA, US]. Intensity levels were determined by the attending therapist and ranged from standing and walking time of 10 to 50 min and between 50 and 1,800 steps taken.
The ABT intervention was adapted from the Beyond Therapy model used by the Shepherd Centre (15). ABT consisted of a combination of resistance, cardiovascular, and flexibility training in various positions as well as gait retraining, without a treadmill or robotic assistance. Upper and lower body resistance training was performed using bodyweight exercises and various apparatus, including bands, wrist weights, dumbbells, and cables. The approximate standardised time allocation for each ABT session was as follows: warm-up and mobility [5 min], resistance training [20–30 min], and cardiovascular training [20–30 min]. Five minutes were allocated for transfers and the setting up of various apparatus. Participants' physical activity levels were monitored using the PARA-SCI (20) tool and were advised not to change their physical activity habits outside of the trial.
2.3. Testing procedures
All 16 participants underwent evaluations of spasticity and completed the pain and QoL questionnaires at baseline, 6, 12, and 24 weeks. This study is a secondary analysis of a larger pilot trial that assessed functional capacity and cardiovascular outcomes (18, 19). Specific methods pertaining to the secondary complication assessments are provided below:
2.3.1. Spasticity evaluation: Modified Ashworth Scale
Spasticity was measured in all participants using the Modified Ashworth Scale (MAS) developed by Bohannon and Smith in 1986 (21). The MAS has been shown to be a reliable tool in SCI populations (21) and it is the most widely used assessment tool to measure resistance to limb movement in a clinical setting (22). Assessment techniques were standardized, including the test positions, right–left test order, speed of assessment and adequate training within a single rater.
2.3.2. Pain questionnaire: International SCI pain basic data set version 2
Due to the subjective nature of pain, an individual's perception of his/her pain is essential for a comprehensive evaluation of pain after SCI (23). The validated International SCI Pain Basic Data Set Version 2 (24) was used to determine the intensity and location of pain that participants experienced, and the subsequent impact of that pain interference on three domains: (A) Daily activities; (B) Mood; (C) Sleep.
2.3.3. Quality of life questionnaire: International SCI quality of life basic data set
Self-report measures are widely used in the SCI literature to assess a participant's mental and emotional state (25). The validated International SCI Quality of Life Basic Data Set (26) was used to assess average QoL over the last month across three domains: (A) life as a whole (general life); (B) physical health; (C) psychological well-being. Scores were rated on a scale ranging from 0 (completely dissatisfied) to 10 (completely satisfied). A higher score indicates greater perceptions of QoL.
2.4. Statistical analysis
All data were analysed using statistical software (R, R Core Team, Auckland, New Zealand and Prism 8, GraphPad Software Inc, California, USA). Normality was assessed using histograms and plots to validate the models. Linear mixed effect models assessed continuous responses which were measured at four time points [0, 6, 12 and 24-weeks]. These mixed effects models formally compared the effect of the group (ABT vs. RLT) interventions across the total 24-week period and tested for any changes over time (pre vs. post). Due to small sample size, it was not possible to fit nonlinear time trends; hence only a linear time effect over the entire 24-week period was considered. To account for the within-subject association between repeated measures, subject specific random effects were included (modelled coefficient p-values and 95% CIs). Response profiles were illustrated using plots of means and half-width 95% confidence intervals (CI) for observed data. Significance was accepted at a p < 0.05. Magnitude-based inferences of change (effect size) were calculated according to Cohen's d (27) to show estimates for observed significant differences. A Cohen's d of zero denotes no effect, whereas ranges from 0.2–0.5, 0.5–0.8 and >0.8 represent small, medium and large effects, respectively (27).
3. Results
3.1. Participant characteristics
A total of 16 participants, aged 19–60 (mean ± SD: 38.4 ± 14.3 years), completed the trial (Table 1). The RLT and ABT groups were matched at baseline for age and time since injury. Motor vehicle accidents accounted for 63% of injury aetiology, whilst stabbing, gunshot, rugby, motorcycle, mountain bicycle and diving accounted for 12.5% each. One participant discontinued the intervention after being enrolled in the RLT group for three weeks. Persistent right leg weakness necessitated a magnetic resonance imaging study (MRI) which provided images consistent with the diagnosis of a tibial stress fracture. Only baseline measures had been recorded for the participant which may have been confounded by an existing stress fracture. Thus, the participant was excluded from all analyses and received treatment for the fracture outside of the trial protocol. No other adverse events or negative side effects were experienced.
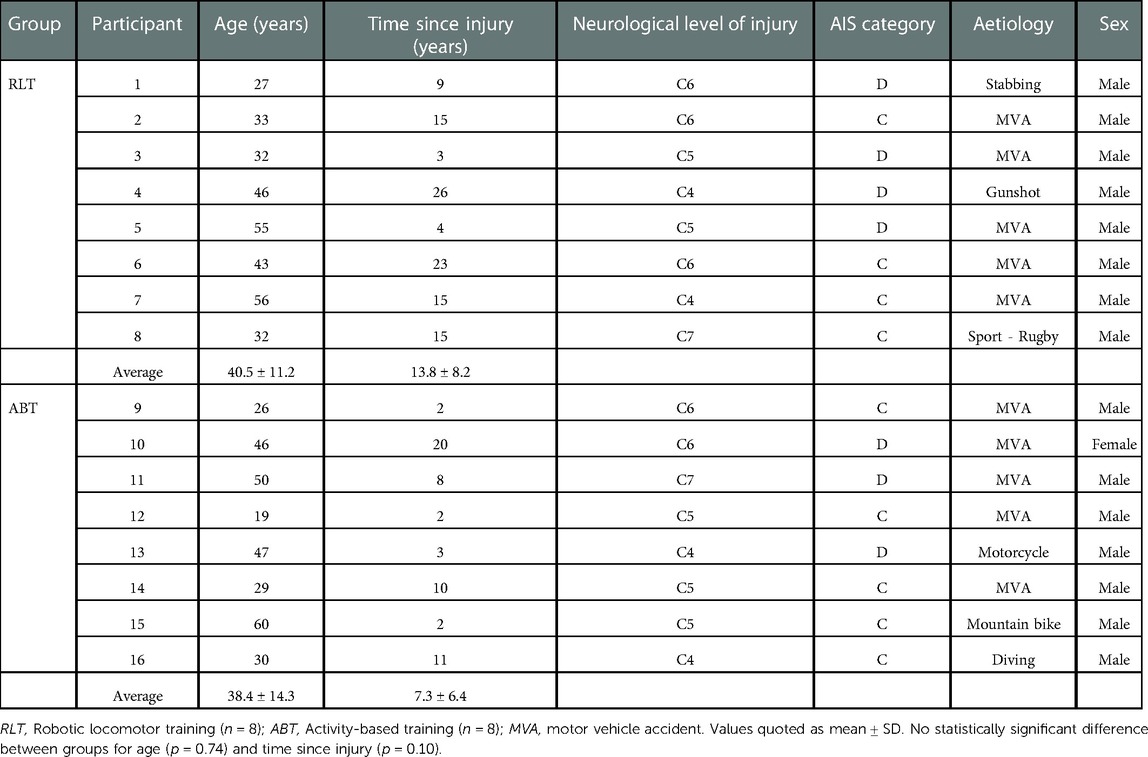
Table 1. Participant characteristics of the Robotic Locomotor Training and Activity-based Training groups.
3.2. Spasticity
Whole body spasticity scores were calculated from the individual scores of the 22 measured body areas. Figure 2 shows that the modelled difference in total spasticity at baseline between the groups was 9.45 units, a non-significant difference (p = 0.09). There was no significant difference in total spasticity scores between the RLT and ABT groups over time (p = 0.25; ES = 0.57) (Table 2).
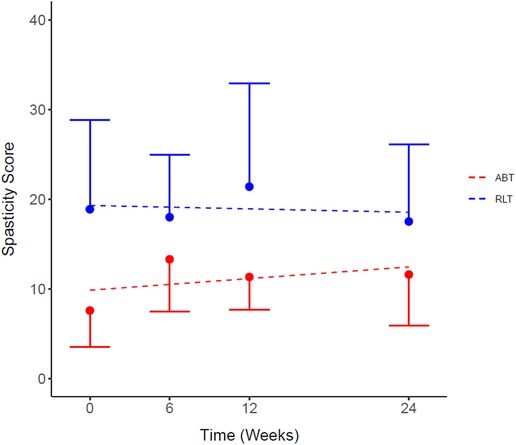
Figure 2. Total spasticity scores for the Robotic Locomotor Training and Activity-based Training groups over time. RLT: Robotic Locomotor Training (n = 8); ABT, Activity-based Training (n = 8); Spasticity score, sum of scores for 22 tested body areas (combined right and left side) using Modified Ashworth Scale. Data presented as observed mean ± half-width 95% CI. Modelled linear estimates shown as superimposed lines (predicted mean). *No significant differences in spasticity scores at baseline (p = 0.09).
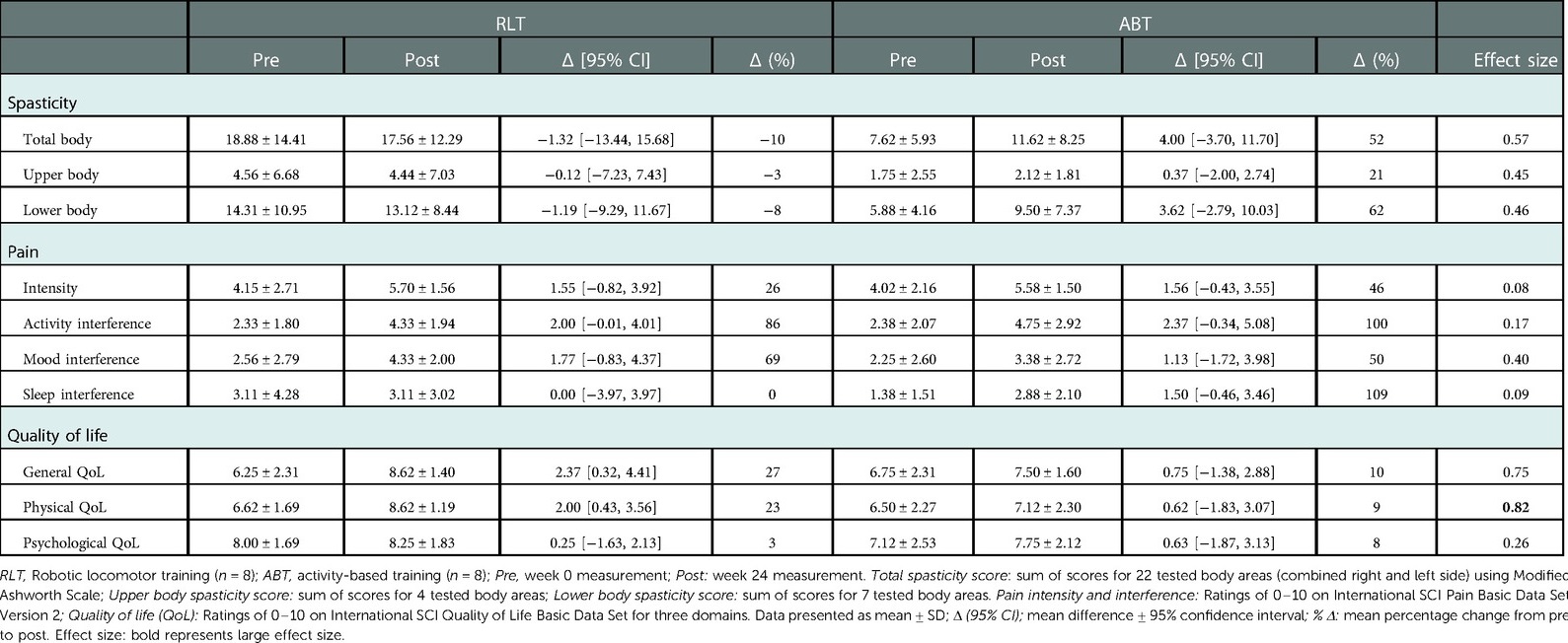
Table 2. Secondary complications for the Robotic Locomotor Training and Activity-based Training groups at baseline and week 24.
3.3. Pain
The responses to the pain questionnaire demonstrated 81% (n = 13) of the participants experienced pain at baseline, whereas by week 24, 100% (n = 16) of the participants experienced pain. There was no significant difference between the RLT and ABT groups for pain intensity (p = 0.67; ES = 0.08) (Figure 3). However, there was a significant increase in pain intensity from pre to post intervention for both groups, with a mean increase of 1.55 [−0.82, 3.92] (37%) (p = 0.03) and 1.56 [−0.43, 3.55] (39%) (p = 0.02) for the RLT and ABT group, respectively (Table 2).
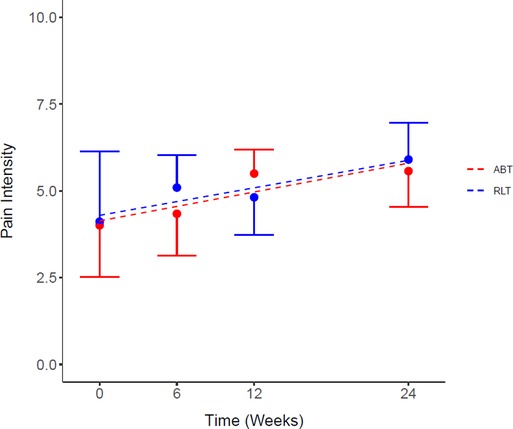
Figure 3. Average pain intensity score for the Robotic Locomotor Training and Activity-based Training groups over time. RLT, Robotic Locomotor Training (n = 8); ABT, Activity-based Training (n = 8); Pain intensity score (0–10), averaged over number of pain locations reported in the International SCI Pain Basic Data Set Version 2. Data presented as observed mean ± half-width 95% CI. Modelled linear estimates shown as superimposed lines (predicted mean).
There was no significant difference between the RLT and ABT groups over time for pain interference ratings (p = 0.61; ES < 0.80). However, both groups reported pain to interfere in an increasing manner with the three measured domains over time (Figures 4A–C). There was a significant time effect for pain interference for the daily activity domain (Figure 3A) (p = 0.05) with an increase in pain interference ratings of 2.00 [−0.01, 4.01] and 2.37 [−0.34, 5.08] for the RLT and ABT group, respectively. The ABT group had an increase in pain interference scores of 100%, 50%, and 109% for the daily activity, mood, and sleep domain, respectively. The RLT group had an increase in pain interference scores of 86% and 69% for the daily activity and mood domain respectively, but no change in the sleep domain (0% change) (Table 2).
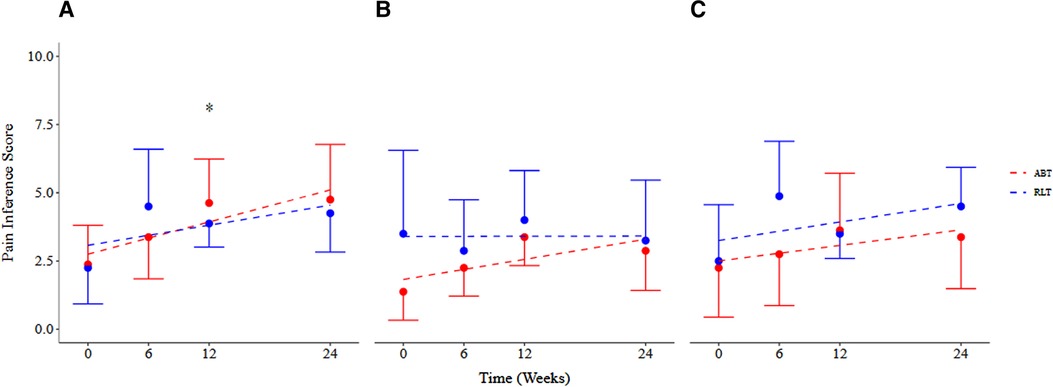
Figure 4. Pain interference scores across (A) daily activity, (B) sleep, and (C) mood domains for the Robotic Locomotor Training and Activity-based Training groups over time. RLT, Robotic Locomotor Training (n = 8); ABT, Activity-based Training (n = 8); Pain interference score (0–10), International SCI Pain Basic Data Set Version 2; Pain intensity score (0–10), averaged over number of pain locations reported in the International SCI Pain Basic Data Set Version 2. Data presented as observed mean and half-width of 95% CI. Modelled linear estimates shown as superimposed lines (predicted mean). *Significant increase in pain experienced in the daily activity domain over time (p = 0.05).
The highest overall pain was experienced in the shoulder for the ABT group and the lower back for the RLT group (Table 3). The ABT group experienced greater reported pain in various areas, with the neck and shoulder areas dominating. The RLT group experienced noticeable variability in the reported pain areas over time, with only the lower back and upper arm being reported as pain areas at both week 0 and week 24.
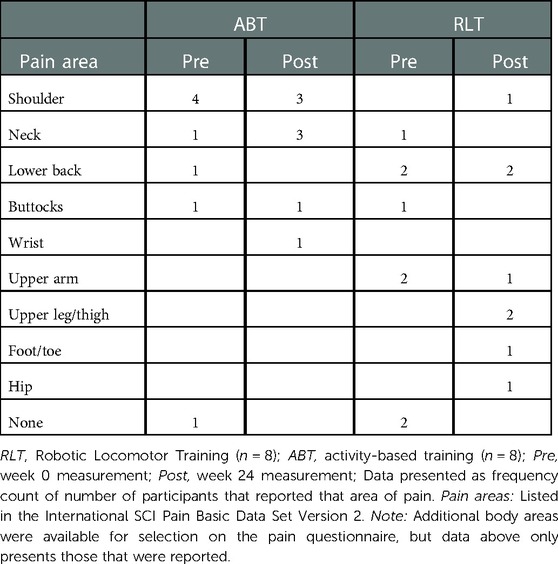
Table 3. Primary pain complaint area reported for the Robotic Locomotor Training and Activity-based Training groups at baseline and week 24.
3.4. Quality of life
There was no significant difference for perceptions of general QoL (p = 0.16; ES = 0.75) and psychological QoL (p = 0.26; ES = 0.62) between the groups by week-24. However, the large effect size of ES = 0.82 (p = 0.12) indicated a group difference in perceptions of physical QoL after 24 weeks of training, with a change of 2.00 [0.43, 3.56] for the RLT group and 0.62 [−1.83, 3.07] for the ABT group post training. Both the ABT and RLT groups showed increasing perceptions of QoL over time, across all three domains: general life, physical health, and psychological well-being (Figure 5). The RLT group had increased (p = 0.03) perceptions of general, physical and psychological QoL with changes of 27%, 23% and 3% from pre to post intervention, respectively. The ABT group had non-significant increases of 10%, 9% and 8% for the three domains, respectively (Table 2).
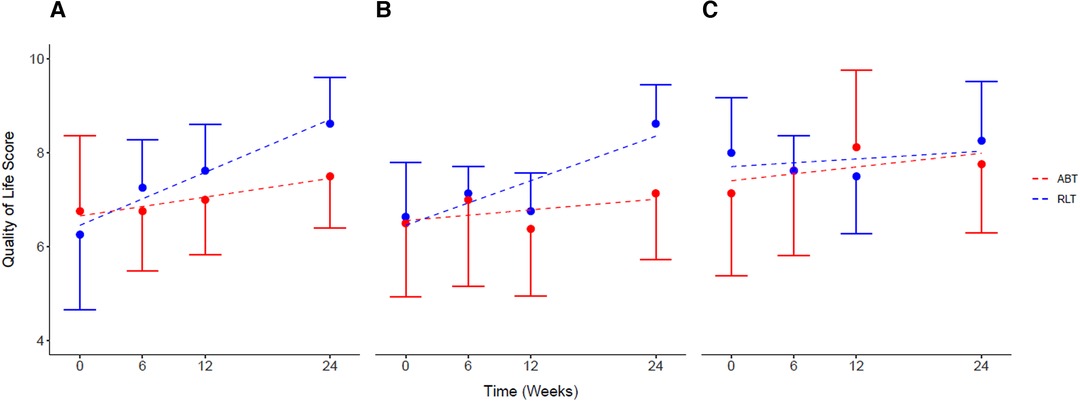
Figure 5. Self-reported quality of life for (A) general life, (B) physical health, and (C) psychological health, for the Robotic Locomotor Training and Activity-based Training groups over time. RLT, Robotic Locomotor Training (n = 8); ABT, Activity-based Training (n = 8); Quality of life score (0–10), International SCI Quality of life (QOL) Basic Data Set; (A): life as a whole; (B): physical health; (C): psychological health. Data presented as observed mean ± half-width 95% CI. Modelled linear estimates shown as superimposed lines (predicted mean).
4. Discussion
This study aimed to describe the effects of RLT compared to ABT on secondary complications, specifically spasticity, pain and QoL. This is a pilot study that needs to be followed up with a high powered, large-scale RCT.
The first important finding is increased pain ratings for both groups over time. Chronic pain after SCI has been found to be prevalent in approximately four out of five individuals with SCI (1). Thus, it is not surprising that most of the participants in this study experienced pain at baseline and throughout the study. The high prevalence and increasing intensity of pain observed in this study is consistent with previous results documented in a SCI population (23, 28). The increased perceptions of pain within this study could possibly be due to the high intensity and frequency of exercise sessions within the groups. It is well documented that this type of training can cause increased fatigue and chronic muscle or joint pain due to an increase in exercise load over time (29, 30). Another plausible explanation for the increasing perceptions of pain was due to an increase in musculoskeletal pain caused by muscle stiffness above the level of injury (31, 32). This delayed-onset-muscle-soreness (DOMS) is a common occurrence following bouts of unaccustomed strenuous physical activity and can result in muscle tenderness and debilitating pain symptoms (31). Thus, the training of new skills, and utilising often unused muscle groups, might have led to stressed muscles during training which was experienced by the participants as an increase in pain levels (32, 33). Several studies have reported on the relationship between the presence of pain and poor mood, reduced health and the ability of pain to interfere significantly with daily functioning (34, 35). This was also evident in the current study which showed an increase in the perception of pain interference with life domains over time, particularly for daily activities.
The shoulder has been reported to be the joint most commonly associated with pain above the level of injury in individuals with SCI (32, 36, 37). Due to the shoulder's complex functional anatomy and limited muscle mass, it is especially at risk for overuse injuries (32). Therefore, it is expected that the ABT group in our study rated the shoulder as the most painful area, due to the high levels of upper body utilization within this training modality. RLT participants may have also encountered upper body pain attributed to the performance of a new exercise modality that uses the upper extremities for support in the Ekso GT exoskeleton (38). However, in the current study, the RLT group rated the lower back as the site of greatest pain. Lower back pain is common within the SCI population with a prevalence of between 50% and 70% (36, 38) and may have been caused by the strain involved in maintaining upright posture and reaching the weights shifts required in the exoskeleton.
The second relevant finding of this pilot trial was that no changes in total spasticity were observed over the intervention period or between the groups. The beneficial effects that physical therapy modalities, such as ABT, have on spasticity in people with SCI is well-established (8, 17, 33, 39–42). Improvements in spasticity with the use of RLT have been speculated based on benefits previously described with body-weight-supported-treadmill training (BWSTT) (43). Regular standing and active exercises have both shown improvements in passive range of movement, posture, muscle strengthening and reduced stress and fatigue, which in turn aid in reducing spasticity symptoms (40). Both static and active standing may increase inhibition of the stretch reflex, reduce motor neuron excitability and subsequently reduce spasticity in individuals with SCI (40–42). Despite the proposed benefits of physical activity on spasticity, the current study showed no statistical changes in total spasticity scores between the RLT and ABT group or over time. However, there are no clear guidelines in the literature regarding the correct dosage and timing of exercise interventions required for these observed effects (22). Additionally, spasticity depends on the type, site, and duration of injury as well as other influencing factors that can trigger or aggravate spasticity symptoms, such as caffeine, lack of sleep, heat changes and pharmacological options (21, 22). These various contributing factors could be potential co-founding variables adding to the diverse spasticity responses found within this study.
The last notable finding in the current study is the increased perceptions of QoL for both the RLT and ABT group over time (Figure 4). These preliminary results add to previous findings that link engagement in physical activity with improved health and QoL (3). Standing and ambulation in particular, have been linked to improved psychological well-being and QoL in people with SCI (11, 44). Interestingly, despite the increasing levels of pain and minimal changes in spasticity reported in this study, QoL improved over time. Chronic pain is the most frequently reported reason for decreased QoL after SCI (45). However, this study indicates a dichotomous relationship between pain, spasticity and QoL among the participants. This may be due to the nature of the pain experienced by the participants, in that the pain was related to the demanding physical training and not as a complication of their SCI. Furthermore, although pain may have increased during the trial, the psychological benefits of partaking in the intervention, including standing and being active may have outweighed the effects of pain on QoL. There are many psychological and social benefits to standing, including improved self-image, eye-to-eye interpersonal contact and increased independence, all of which contribute to enhancing QoL (16). Wheelchair users may enjoy and value the normalizing experience of seeing themselves upright and participating in the walking motion (46). Thus, the increased QoL reported in this study could be attributed to the improved psychological benefit of standing and even walking again (47). For individuals with SCI, many ADLs are physical in nature (e.g., transferring, wheeling, eating). As a result, individuals who lack the physical capacity to perform basic ADLs may judge these tasks as stressful because of feelings of helplessness due to an inability to cope with the demands of daily living (35). Thus, the achievement of physical goals over the 24-week interventions may have also have led to increased perceptions of physical QoL, greater satisfaction with physical abilities and improved self-image and self-efficacy (48–50).
In addition, quality relationships and providing or receiving social and emotional support, can improve psychological well-being in people with SCI (48, 49, 51). Within the South African context, most individuals with SCI do not receive out-patient rehabilitation, let al.one the opportunity to exercise in a large multi-disciplinary training setting (52). Due to the lack of neurological specific rehabilitation centres in South Africa, most of the participants in this trial were unfamiliar with training in groups or alongside others with similar conditions. Consequently, the effect of regular social participation and interpersonal support, provided by the rehabilitation setting, may have led to improved psychological QoL for the participants in this study (53).
4.1. Limitations
As pain was a secondary analysis of the RCT, a limitation to the interpretation of the results is that analgesic/non-steroidal anti-inflammatory use was not documented. In addition, this study did not have a true experimental control in which no exercise was performed. Although an equivalent control group may be the experimental ideal, it is not feasible when conducting exercise interventions within the SCI population due to health and ethical implications. Although the small sample size was restrictive for statistical power and limits the generalisability of these findings to the larger SCI population, it may still provide important preliminary information for researchers to expand upon, as statistically insignificant changes could be of substantial clinical significance for people with SCI. An RCT with a larger sample size is warranted to further examine these findings.
4.2. Conclusion
In conclusion, it appears that neither intervention had an effect on reducing spasticity symptoms over 24-weeks. Pain ratings increased significantly for both interventions, though QoL perceptions were improved for both groups across the intervention period. This finding represents an interesting dichotomous relationship that requires additional investigation. Furthermore, the improved perceptions of QoL highlight the potential effectiveness of exercise interventions to support the well-being of people with SCI. Despite the small sample size, the adherence rate was extremely high and the cohort was homogenous, highlighting the strengths of this study and its contribution to the body of evidence on secondary health complications following SCI. A strong evidence base for the prevention and effective management of secondary complications will be essential for future breakthroughs in SCI health and well-being. Therefore, continued experimental research and rigorous studies are warranted to investigate these results further.
Data availability statement
The raw data supporting the conclusions of this article will be made available by the authors, without undue reservation.
Ethics statement
The studies involving human participants were reviewed and approved by University of Cape Town, Faculty of Health Sciences, Human Research Ethics Committee (HREC 718/2017). The patients/participants provided their written informed consent to participate in this study.
Author contributions
CS, RE, SW, WD and YA contributed to the conception and design of the study. CS organized the database and performed the statsical analysis. CS wrote the first draft of the manuscript. All authors contributed to the article and approved the submitted version.
Funding
This research was funded by the National Research Foundation of South Africa Career Grant, University of Cape Town Development Grant [grant number 91421] and the Oppenheimer Memorial Trust [grant number 20523].
Acknowledgments
Tristin Naidoo from UCT for his assistance with the statistical analysis. Jason Bantjes, Leslie Swartz and Philippa Skowno for monitoring the psychological well-being of the participants involved in this trial. Ed Baalbergen for the health screening of the participants involved in the trial.
Conflict of interest
The authors declare that the research was conducted in the absence of any commercial or financial relationships that could be construed as a potential conflict of interest.
Publisher's note
All claims expressed in this article are solely those of the authors and do not necessarily represent those of their affiliated organizations, or those of the publisher, the editors and the reviewers. Any product that may be evaluated in this article, or claim that may be made by its manufacturer, is not guaranteed or endorsed by the publisher.
Supplementary material
The Supplementary Material for this article can be found online at: https://www.frontiersin.org/articles/10.3389/fresc.2023.1003360/full#supplementary-material.
References
1. Sezer N, Akkuş S, Uğurlu FG. Chronic complications of spinal cord injury. World J Orthop. (2015) 6(1):24–33. doi: 10.5312/wjo.v6.i1.24
2. Crane DA, Hoffman JM, Reyes MR. Benefits of an exercise wellness program after spinal cord injury. J Spinal Cord Med. (2017) 40(2):154–8. doi: 10.1179/2045772315Y.0000000038
3. Ginis K, Jörgensen S, Stapleton J. Exercise and sport for persons with spinal cord injury. PM R. (2012) 4(11):894–900. doidoi: 10.1016/j.pmrj.2012.08.006
4. Burchiel KJ, Hsu FPK. Pain and spasticity after spinal cord injury: mechanisms and treatment. Spine (Phila Pa 1976). (2001) 26(24):146–60. doi: 10.1097/00007632-200112151-00024
5. Ginis KAM, Latimer AE, Mckechnie K, Ditor DS, Mccartney N, Hicks AL, et al. Using exercise to enhance subjective well-being among people with spinal cord injury: the mediating influences of stress and pain. Rehabil Psychol. (2003) 48(3):157–64. doi: 10.1037/0090-5550.48.3.157
6. Hulsebosch CE, Hains BC, Crown ED, Carlton SM. Mechanisms of chronic central neuropathic pain after spinal cord injury. Brain Res Rev. (2008) 60(1):202–13. doi: 10.1016/j.brainresrev.2008.12.010
7. Adams MM, Hicks AL. Spasticity after spinal cord injury. Spinal Cord. (2005) 43:577–86. doi: 10.1038/sj.sc.3101757
8. Nas K, Yazmalar L, Şah V, Aydın A, Öneş K. Rehabilitation of spinal cord injuries. World J Orthop. (2015) 6(1):8–16. doi: 10.5312/wjo.v6.i1.8
9. Hubscher CH, Herrity AN, Williams CS, Montgomery R, Willhite AM, Angeli CA, et al. Improvements in bladder, bowel and sexual outcomes following task-specific locomotor training in human spinal cord injury. PLoS One. (2018) 13:1–26. doi: 10.1371/journal.pone.0190998
10. Simpson LA, Eng JJ, Hsieh JTC, Wolfe DL. The health and life priorities of individuals with spinal cord injury: a systematic review. J Neurorestoratology. (2013) 29(8):1548–55. doi: 10.1089/neu.2011.2226
11. Dryden DM, Saunders LD, Rowe BH, May LA, Yiannakoulias N, Svenson LW, et al. Utilization of health services following spinal cord injury: a 6-year follow-up study. Spinal Cord. (2004) 42(9):513–25. doi: 10.1038/sj.sc.3101629
12. Anderson KD. Targeting recovery: priorities of the spinal cord-injured population. J Neurotrauma. (2004) 21(10):1371–83. doi: 10.1089/neu.2004.21.1371
13. Dolbow DR, Gorgey AS, Recio AC, Stiens SA, Curry AC, Sadowsky L, et al. Activity-based restorative therapies after spinal cord injury: inter-institutional conceptions and perceptions. Aging Dis. (2015) 6(4):254–61. doi: 10.14336/AD.2014.1105
14. Behrman AL, Ardolino EM, Harkema SJ. Activity-based therapy: from basic science to clinical application for recovery after spinal cord injury. J Neurol Phys Ther. (2017) 41(July):1–14. doi: 10.1097/NPT.0000000000000184
15. Jones ML, Evans N, Tefertiller C, Backus D, Sweatman M, Tansey K, et al. Activity-based therapy for recovery of walking in individuals with chronic spinal cord injury: results from a randomized clinical trial. Arch Phys Med Rehabil. (2014) 95(12):2239–2246.e2. doi: 10.1016/j.apmr.2014.07.400
16. Kressler J, Thomas CK, Field-Fote EC, Sanchez J, Widerström-Noga E, Cilien DC, et al. Understanding therapeutic benefits of overground bionic ambulation: exploratory case series in persons with chronic, complete spinal cord injury. Arch Phys Med Rehabil. (2014) 95(10):1878–1887.e4. doi: 10.1016/j.apmr.2014.04.026
17. Shackleton C, Evans R, Shamley D, West S, Albertus Y. Effectiveness of over-ground robotic locomotor training in improving walking performance, cardiovascular demands, secondary complications and user-satisfaction in individuals with spinal cord injuries: a systematic review. J Rehabil Med. (2019) 51(10):723–33. doi: 10.2340/16501977-2601
18. Shackleton C, Evans R, West S, Derman W, Albertus Y. Robotic walking for recovery of functional capacity in individuals with incomplete spinal cord injury: a randomized pilot trial. MedRxiv. (2021) Preprint:1–28. doi: 10.1097/phm.0000000000001937
19. Evans RW, Shackleton CL, West S, Derman W, Laurie Rauch HG, Baalbergen E, et al. Robotic locomotor training leads to cardiovascular changes in individuals with incomplete spinal cord injury over a 24-week rehabilitation period: a randomized controlled pilot study. Arch Phys Med Rehabil. (2021) 102(8):1447–56. doi: 10.1016/j.apmr.2021.03.018
20. Ginis KAM, Latimer AE, Hicks AL, Craven BC. Development and evaluation of an activity measure for people with spinal cord injury. Med Sci Sports Exerc. (2005) 37(7):1099–111. doi: 10.1249/01.mss.0000170127.54394.eb
21. Mishra C, Ganesh GS. Inter-rater reliability of modified modified ashworth scale in the assessment of plantar flexor muscle spasticity in patients with spinal cord injury. Physiother Res Int. (2014) 19(4):231–7. doi: 10.1002/pri.1588
22. Thompson AJ, Jarrett L, Lockley L, Marsden J, Stevenson VL. Clinical management of spasticity. J Neurol Neurosurg Psychiatry. (2005) 76:463–5. doi: 10.1136/jnnp.2004.035998
23. Widerström-Noga EG, Felipe-Cuervo E, Yezierski RP. Relationships among clinical characteristics of chronic pain after spinal cord injury. Arch Phys Med Rehabil. (2001) 82(9):1191–7. doi: 10.1053/apmr.2001.25077
24. Widerström-Noga E, Biering-Sørensen F, Bryce TN, Cardenas DD, Finnerup NB, Jensen MP, et al. The international spinal cord injury pain basic data set (version 2.0). Spinal Cord. (2014 Apr) 52(4):282–6. doi: 10.1038/sc.2014.4
25. Pollard C, Kennedy P. A longitudinal analysis of emotional impact, coping strategies and post-traumatic psychological growth following spinal cord injury: a 10-year review. Br J Health Psychol. (2007) 12(Pt 3):347–62. doi: 10.1348/135910707X197046
26. Charlifue S, Post MW, Biering-Sørensen F, Catz A, Dijkers M, Geyh S, et al. International spinal cord injury quality of life basic data set. Spinal Cord. (2012) 50(9):672–5. doi: 10.1038/sc.2012.27
28. Warms CA, Turner JA, Marshall HM, Cardenas DD. Treatments for chronic pain associated with spinal cord injuries : many are tried, few are helpful. Clin J Pain. (2002) 18:154–63. doi: 10.1097/00002508-200205000-00004
29. Brenner JS, Small EW, Bernhardt DT, Congeni JA, Gomez JE, Gregory AJM, et al. Overuse injuries, overtraining, and burnout in child and adolescent athletes. Pediatrics. (2007) 119(6):1242–5. doi: 10.1542/peds.2007-0887
30. Brooks K, Carter J. Overtraining, exercise, and adrenal insufficiency. J Nov Physiother. (2013) 3(125):1–10. doi: 10.4172/2165-7025.1000125
31. Cheung K, Hume PA, Maxwell L. Delayed onset muscle soreness: treatment strategies and performance factors. Sport Med. (2003) 33(2):145–64. doi: 10.2165/00007256-200333020-00005
32. Drongelen SV, Groot SD, Veeger HEJ, Angenot ELD, Dallmeijer AJ, Post MWM. Upper extremity musculoskeletal pain during and after rehabilitation in wheelchair-using persons with a spinal cord injury. Spinal Cord. (2006) 44:152–9. doi: 10.1038/sj.sc.3101826
33. Bhimani R, Anderson L. Clinical understanding of spasticity: implications for practice. Rehabil Res Pract. (2014) 2014:1–10. doi: 10.1155/2014/279175
34. Siddall PJ, Mcclelland JM, Rutkowski SB, Cousins MJ. A longitudinal study of the prevalence and characteristics of pain in the first 5 years following spinal cord injury. Pain. (2003) 103:249–57. doi: 10.1016/S0304-3959(02)00452-9
35. Latimer AE, Ginis KAM, Hicks AL, Mccartney N. An examination of the mechanisms of exercise-induced change in psychological well-being among people with spinal cord injury. J Rehabil Res Dev. (2004) 41(5):643–52. doi: 10.1682/JRRD.2003.04.0043
36. Ullrich PM, Jensen MP, Loeser JD, Cardenas DD. Pain intensity, pain interference and characteristics of spinal cord injury. Spinal CordCord. (2008) 46:451–5. doi: 10.1038/sc.2008.5
37. Alm M, Saraste H, Norrbrink C. Shoulder pain in persons with thoracic spinal cord injury: prevalence and characteristics. J Rehabil Med. (2008) 40:277–83. doi: 10.2340/16501977-0173
38. Kolakowsky-Hayner SA, Crew J, Moran S, Shah A. Safety and feasibility of using the EksoTM bionic exoskeleton to aid ambulation after spinal cord injury. J Spine. (2013) 4:1–8. doi: 10.1080/10790268.2019.1671076
39. Nash MS. Exercise as a health-promoting activity following spinal cord injury. J Neurol Phys Ther. (2005) 29(2):87–106. doi: 10.1097/01.NPT.0000282514.94093.c6
40. Walter JS, Sacks J, Othman R, Rankin AZ, Nemchausky B, Chintam R, et al. A database of self-reported secondary medical problems among VA spinal cord injury patients : its role in clinical care and management. J Rehabil Med. (2002) 39(1):53–61. PMID: 11926327
41. Sadeghi M, Mclvor J, Finlayson H, Sawatzky B. Static standing, dynamic standing and spasticity in individuals with spinal cord injury. Spinal Cord. (2016) 54(5):376–82. doi: 10.1038/sc.2015.160
42. Eng JJ, Levins SM, Townson AF, Mah-jones D, Bremner J, Huston G. Use of prolonged standing for individuals with spinal cord injuries. Phys Ther. (2001) 81(8):1392–9. doi: 10.1093/ptj/81.8.1392
43. Adams MM, Hicks AL. Comparison of the effects of body-weight-supported treadmill training and tilt-table standing on spasticity in individuals with chronic spinal cord injury. J Spinal Cord Med. (2011) 34(5):488–94. doi: 10.1179/2045772311Y.0000000028
44. Paleg G, Livingstone R. Systematic review and clinical recommendations for dosage of supported home-based standing programs for adults with stroke, spinal cord injury and other neurological conditions. BMC Musculoskelet Disord. (2015) 16(1):358. doi: 10.1186/s12891-015-0813-x
45. Widerström-Noga EG, Felipe-Cuervo E, Broton JG, Duncan RC, Yezierski RP. Perceived difficulty in dealing with consequences of spinal cord injury. Arch Phys Med Rehabil. (1999) 80(5):580–6. doi: 10.1016/S0003-9993(99)90203-4
46. Hicks AL, Martin Ginis KA. Treadmill training after spinal cord injury: it's not just about the walking. J Rehabil Res Dev. (2008) 45(2):241–8. doi: 10.1682/JRRD.2007.02.0022
47. Stampacchia G, Rustici A, Bigazzi S, Gerini A, Tombini T, Mazzoleni S. Walking with a powered robotic exoskeleton: subjective experience, spasticity and pain in spinal cord injured persons. NeuroRehabilitation. (2016) 39(2):277–83. doi: 10.3233/NRE-161358
48. Mashola MK, Mothabeng DJ. Associations between health behaviour, secondary health conditions and quality of life in people with spinal cord injury. African J Disabil. (2019) 8:1–9. doi: 10.4102/ajod.v8i0.463
49. DeRoon-Cassini T, Valvano A, Aubin E, Hastings J, Horn P. Psychological well-being after spinal cord injury : perception of loss and meaning making. Rehabil Psychol. (2009) 54(3):306–14. doi: 10.1037/a0016545
50. Anneken V, Hirschfeld S, Scheuer T, Thietje R. Influence of physical exercise on quality of life in individuals with spinal cord injury. Spinal Cord. (2010) 48:393–9. doi: 10.1038/sc.2009.137
51. Beedie A, Kennedy P. Quality of social support predicts hopelessness and depression post spinal cord injury. J Clin Psychol Med Settings. (2002) 9(3):227–34. doi: 10.1023/A:1016003428370
52. Joseph C, Scriba E, Wilson V, Mothabeng J, Theron F. People with spinal cord injury in republic of South Africa. Am J Phys Med Rehabil. (2017) 96(2):S109–11. doi: 10.1097/PHM.0000000000000594
53. Evans RW, Bantjes J, Shackleton CL, West S, Derman W, Albertus Y, et al. “I was like intoxicated with this positivity”: the politics of hope amongst participants in a trial of a novel spinal cord injury rehabilitation technology in South Africa. Disabil Rehabil Assist Technol. (2020):1–7. doi: 10.1080/17483107.2020.1815086
Keywords: spinal cord injuries, exoskeleton device, exercise therapy, pain, muscle spasticity, quality of life
Citation: Shackleton C, Evans R, West S, Derman W and Albertus Y (2023) Robotic locomotor training for spasticity, pain, and quality of life in individuals with chronic SCI: A pilot randomized controlled trial. Front. Rehabil. Sci. 4:1003360. doi: 10.3389/fresc.2023.1003360
Received: 26 July 2022; Accepted: 5 January 2023;
Published: 30 January 2023.
Edited by:
Cristina L. Sadowsky, Kennedy Krieger Institute, United StatesReviewed by:
Zhuoying Qiu, China Rehabilitation Research Center/WHO Collaborating Center for Family International Classifications, ChinaNicola Theis, University of Gloucestershire, United Kingdom
© 2023 Shackleton, Evans, West, Derman and Albertus. This is an open-access article distributed under the terms of the Creative Commons Attribution License (CC BY). The use, distribution or reproduction in other forums is permitted, provided the original author(s) and the copyright owner(s) are credited and that the original publication in this journal is cited, in accordance with accepted academic practice. No use, distribution or reproduction is permitted which does not comply with these terms.
*Correspondence: Yumna Albertus yumna.albertus@uct.ac.za
Specialty Section: This article was submitted to Disability, Rehabilitation, and Inclusion, a section of the journal Frontiers in Rehabilitation Sciences