A perspective on cellulose dissolution with deep eutectic solvents
- Department of Chemical Engineering, Izmir Institute of Technology, Izmir, Türkiye
Currently, membrane manufacturing relies heavily on fossil-based solvents and polymers, resulting in significant negative impacts on human health and the environment. Thus, there is an urgent need for eco-friendly, low-toxicity, and sustainable solvents and polymers to comply with the United Nations’ sustainable development goals. Cellulose, as a green, natural, and abundant polymer, offers a sustainable source for membrane manufacturing. However, a significant challenge exists in dissolving cellulose due to strong intermolecular and intramolecular hydrogen bonds within cellulose molecules. Deep eutectic solvents (DESs), which contain both hydrogen bond donor and acceptor groups, have received significant attention as alternative solvents for cellulose dissolution owing to their low cost, low toxicity, environmentally friendly nature, ease of synthesis, and versatility. This review examines experimental studies, and theoretical approaches, highlighting key findings and factors influencing cellulose dissolution in deep eutectic solvents.
1 Introduction
Membrane technology has been considered a green technology. However, the green membrane manufacturing process has just recently become a concern. Membrane production requires a large amount of fossil-based solvents and polymers. Polyethylene, polypropylene, polysulfone, poly (vinylidenefluoride), polyimide), and poly (benzimidazole) are commonly used petroleum derived polymers to fabricate membranes. Conventional solvents used for dissolving these polymers, such as N-methyl pyrrolidone (NMP), N, N-dimethylformamide (DMF), and dimethylacetamide (DMAc) (Heinze and Koschella, 2005), are also derived from fossil raw materials causing negative impact on the environment and human health. Bio-solvents have been alternatively produced from bio-based feedstock such as carbohydrates, carbohydrate polymers, proteins, alkaloids, plant oils, and animal fats. However, purification of these solvents, usually with extraction, requires toxic chemicals and high-energy input and generates a waste stream (Jin et al., 2017). Therefore, the adoption of greener membrane fabrication methods is currently being considered to align with the United Nations Sustainable Development Goals. In this regard, transitioning from fossil-based polymers to natural polymers is crucial, with cellulose emerging as a significant and abundant renewable biopolymer among them. Composed of repeating D-glucose monomers, each with three hydroxyl groups, cellulose offers a favorable structure for chemical modification. However, its strong intermolecular hydrogen bonds make this semicrystalline polymer insoluble in most organic solvents (Chen et al., 2019), thus, presenting a challenge for cellulose membrane fabrication using conventional methods like phase inversion. Traditional solvents like N-methylmorpholine-N-oxide (Rosenau et al., 2002), lithium chloride/N,N-dimethylacetamide (DMAc) (Zhang et al., 2014), alkali/urea, or alkali/thiourea aqueous solutions (Xiong et al., 2014), used for dissolving cellulose, are not considered environmentally friendly due to their negative impact on the environment. High-temperature pretreatment or solvent exchange is necessary before dissolving cellulose in DMAc (Raus et al., 2012), while the alkali-urea aqueous system can only dissolve low molecular weight cellulose below −12°C (Egal et al., 2008). Newer, more popular ionic liquids (ILs) have been suggested as sustainable alternatives for cellulose dissolution (Swatloski et al., 2002; Zhao et al., 2009; Velioglu et al., 2014; Zhang et al., 2017). The low vapor pressure of ILs is an appealing feature for reducing air pollution. However, their relatively high solubilities in water and poor biodegradability (Jordana and Gathergood, 2015) cause toxicity in water (Flieger and Flieger, 2020), and their dissolution requires high energy consumption (Chundawat et al., 2020). Additionally, their synthesis is not environmentally friendly.
Deep eutectic solvents (DESs) are also recognized as green solvents and can be easily prepared by combining hydrogen bond donors (HBD) and acceptors (HBA) in a stoichiometric ratio (Cardellini et al., 2015). Similar to ILs, DESs exhibit low vapor pressure but with lower toxicity profiles, particularly when derived from natural compounds such as amino acids, sugars, and carboxylic acids (Hayyan et al., 2016). Furthermore, their synthesis does not require any solvent or extensive purification, making it a more cost-effective option. Like ILs, DESs have the potential to dissolve cellulose due to the hydrogen bonding interaction of hydrogen bond donor (HBD) and hydrogen bond acceptor (HBA). DESs are considered “designer” solvents of a new generation; changing the type and molar ratio of HBA and HBDs can finely adjust their physicochemical properties. This adaptability presents a challenge in selecting the appropriate DES for cellulose dissolution. The multitude of HBA and HBD combinations in various molar ratios increase the potential number of DES candidates for exploration. Consequently, developing predictive tools for designing DESs to enhance cellulose solubility is crucial for greener membrane fabrication. This mini-review aims to offer insights into cellulose dissolution in DESs and investigate the synergies between experimental and theoretical approaches to advance our current understanding of cellulose dissolution in designer DESs.
2 Recent progress in cellulose dissolution using deep eutectic solvents
The research on cellulose dissolution with DESs started in 2012 by testing 26 different DESs to evaluate their capability to dissolve lignin, starch, and cellulose (Francisco et al., 2012). Even though the tested DESs dissolved up to 14.9 wt% of lignin, they demonstrated a limited dissolution capacity for cellulose. The highest cellulose solubility (0.78 wt%) was observed with the DES composed of malic acid and proline in a 1:3 ratio, while the DES consisting of malic acid (HBD) and alanine (HBA) in a 1:1 ratio showed the lowest solubility (0.11 wt%.). Sharma et al. (2013) tried different cellulose dissolution methods, including heating at 100°C for 10 h, ultrasonication followed by heating at 80°C for 1 h, and microwave irradiation at 80°C for 2 h. They achieved an 8 wt% cellulose solubility using the choline chloride and urea (1:2 ratio) combination by keeping the dissolution temperature at 100°C. Surprisingly, microwave irradiation did not significantly enhance cellulose solubility.
Pan et al. (2017) observed that while choline chloride/urea effectively dissolved amorphous cellulose in rice straw, it was not efficient for the solubility of α-cellulose. Tenhunen et al. (2017) similarly reported that choline chloride/urea (molar ratio 1:2) failed to modify cellulose fibers. Hertel et al. (2012) noted the importance of temperature on cellulose dissolution. They found that microcrystalline cellulose (MCC) did not dissolve in DESs containing urea/choline chloride (2:1), oxalic acid/choline chloride, malonic acid/choline chloride (1:1), and formamide/ammonium formate (2:1) at 45°C. Increasing the temperature to 80°C slightly improved the dissolution in malonic acid/choline chloride and formamide/ammonium formate. While the acidic environment for urea/choline chloride and oxalic acid/choline chloride did not enhance cellulose dissolution, a basic medium (pH 14) did enhance it. Ren et al. (2016a) first activated the cellulose using ultrasound-assisted saturated calcium chloride solution. Their data demonstrated that cellulose solubility increased from 1.43 wt% in a choline chloride and urea mixture to 2.48 wt% when imidazole replaced urea. Malaeke et al. (2018) reported a maximum solubility of 6.1 wt% in choline chloride and resorcinol through the use of ultrasound irradiation. Zhong et al. (2022) claimed that in contrast to the data reported in the literature, most choline chloride-based DESs cannot effectively dissolve cellulose. They introduced a novel DES by combining urea (Ur) with choline hydroxide (ChOH), which dissolved 9.5 wt% cellulose with a degree of polymerization (DP) of 926 (from cotton litter pulp). The ChOH/Ur system contained approximately 11 wt% water, and complete dissolution was achieved within 30 min at 70°C. The highest reported cellulose solubility in deep eutectic solvents (DESs) thus far is 15%, as documented by Sirvio and Heiskanen (2020), Sharma et al. (2021), and Tong et al. (2021; 2022). Sirvio and Heiskanen (2020) formulated DESs using tetraethylammonium hydroxide (TEAOH) combined with urea, N-methylurea, N-ethylurea, and 1,3-dimethylurea, achieving the best dissolution performance (15%) with the TEAOH:Water:Urea DES in a ratio of 1:15:2. Sharma and colleagues synthesized 22 zwitterion-based DESs by mixing four types of zwitterions with four saccharides at varying ratios. Two DES formulations, involving zwitterion C15 and 5 wt% sucrose and fructose, respectively, dissolved 15% and 10% cellulose at 120°C. Tong et al. (2021) combined zinc chloride (ZnCl2), water, and phosphoric acid, achieving 15 wt% cellulose dissolution at room temperature in this DES with a molar ratio of 1:3:0.6. In a subsequent study, Tong et al. (2022) developed DESs using ZnCl2, water, and formic acid combinations, achieving the highest solubility of 15% for microcrystalline cellulose in a molar ratio of 1:1:4. The dissolution process occurred under relatively mild conditions with minimal heat energy usage, effectively dissolving various cellulose types, including cotton with high crystallinity and degree of polymerization (DP). Table 1 provides a summary of cellulose solubility in different DESs as reported in the literature.
3 Exploration of key factors for cellulose dissolution in deep eutectic solvents
Literature studies indicate that the hydrogen bond acceptor strength (β) of a deep eutectic solvent (DES) is the primary factor influencing its ability to dissolve cellulose. Ren et al (2016a) reported the highest cellulose solubility of 2.48% for the DES, choline chloride/imidazole, with the highest basicity (β = 0.864). The order of solubility of DES correlated with the order of basicity values: Choline chloride/imidazole (2.48 wt%, β = 0.864) >choline chloride/urease (1.45 wt%, β = 0.821) >choline chloride/ammonium thiocyanate (0.83 wt%, β = 0.81). Similarly, the data reported by Zhong et al. (2022) show that the higher cellulose solubility in choline hydroxide/urea (β = 1.88) than in choline chloride/urea (β = 0.49) is directly related to the basicities of the DESs.
Viscosity of the DES is another important parameter controlling its dissolution power. The solvents with lower viscosity penetrate the polymer matrix more readily, enhancing polymer chain mobility, leading to faster dissolution rates. Ren et al. (2016b) noted that the DES composed of allyl triethyl ammonium chloride ([ATEAm]Cl) and oxalic acid (Oxa) demonstrated greater cellulose dissolution compared to choline chloride (ChCl) and Oxa. This difference was attributed to its lower viscosity, as illustrated in Figure 1. A lower activation energy for [ATEAm]Cl-Oxa (44.56 kJ mol−1), compared to ChCl-Oxa (57.82 kJ mol−1) suggests weaker hydrogen bonding between chloride and Oxa in [ATEAm]Cl-Oxa, leading to increased interaction with cellulose molecules and a higher cellulose dissolution capacity. In another study, the same research group reported the highest cellulose solubility at 2.48% in choline chloride/imidazole, which exhibited the lowest viscosity among the investigated DESs (Ren et al., 2016a). Fan et al. (2021) found that the viscosity of the natural deep eutectic solvents (NADES) correlates with the hydrogen-bond number and lifetime. Among five terpene-based natural deep eutectic solvents (NADES) including camphor/formic acid, menthol/acetic acid, menthol/β-citronellol, menthol/lactic acid, and thymol/β-citronellol, terpene-acid-based NADES with single sites exhibited the lowest viscosity due to their weak and unstable hydrogen bonding. Conversely, NADES based on multi-site terpene acids had comparatively higher viscosity. Based on their results, the DES with the lowest binding energy also demonstrated the lowest viscosity. Ghaedi et al. (2017) reported that the higher molecular weight of the DES resulted in the higher viscosity and surface tension.
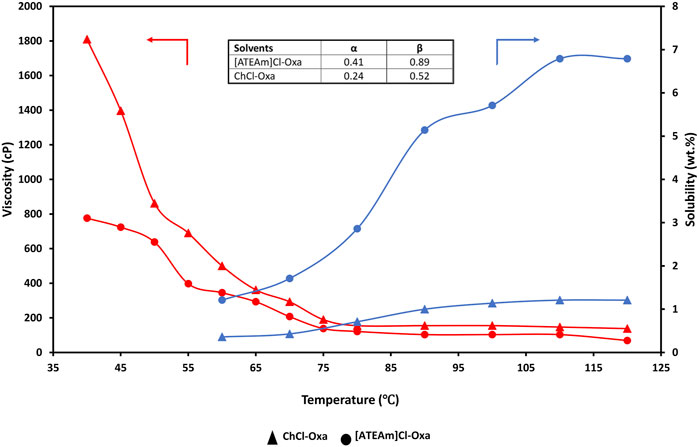
Figure 1. The variation in viscosity and solubility of the DESs, ChCl-Oxa and [ATEAm] Cl-Oxa, with temperature. Data were taken from Ren et al. (2016b).
The intrinsic viscosity (ηsp) of a DES/cellulose solution serves as another reference standard for solvation capacity of the DESs and can be calculated by Eqs 1, 2.
where KH represents the Huggins constant, and solutes are considered dissolved in solvents when KH is less than 0.5 (Kulicke and Kniewsker, 1984). Tong et al. (2022) reported that the KH values, and consequently the viscosity of the cellulose solution increased with the degree of polymerization of cellulose, leading to a lower level of cellulose dissolution.
Theoretically, cellulose dissolution takes place when Gibbs free energy change (
The presence of excessive water molecules is another factor affecting the dissolution power of DES. An increased water content in the DES formulation resulted in a reduction of the dissolved cellulose amount. (Tong et al., 2022; Zhong et al., 2022). Furthermore, the addition of the hydrophilic compound PEG in the DES formulation played a crucial role in disrupting the strong intermolecular hydrogen bonds between cellulose molecules. Ren et al. (2016a) reported improved cellulose solubility in choline chloride/imidazole-coupled PEG.
4 Computational tools for predicting the cellulose solubility in deep eutectic solvents
Generally, the solubility parameter (or Hildebrand parameter) is the most commonly used criterion in choosing a suitable solvent for dissolving a polymer based on the principle of “like dissolves like” (Hildebrand et al., 1962; Hansen et al., 2007). The small value of Flory-Huggins interaction parameter, (χ < 0.5), which is proportional to polymer (
Recent experimental studies have shown that the HBA strength, β values, can be a valuable indicator for evaluating the DES’s cellulose dissolving power. β values and the other 2 KamletTaft (KAT) polarity parameters can be experimentally measured using solvatochromic dyes (Zhong et al., 2022). However, considering the large number of HBA and HBD combinations for DES formulation, experimental screening of all formulations is expensive and impractical. A quantum chemical (QC) based conductor-like screening model real solvent (COSMO-RS) approach is a practical tool for predicting the KAT parameters of the DESs. In this method, the COSMO file is generated through independent optimization of the geometries of the HBAs and HBDs by QC calculation. Next, hydrogen bonding energy (EHB), misfit energy (EMF), van der Waals energy, and non-bonded interactions of DES are estimated using the COSMO-RS model. The H-bond acidity and basicity values are then calculated using the three specific interactions by Eqs 3, 4.
The constants Aα, Bα, Cα, and Dα, as well as Aβ, Bβ, Cβ, and Dβ, are determined by fitting the interaction energies of a specific subclass of DES to the experimentally determined KAT parameters. The subclasses are constructed based on the hydrophilicity/hydrophobicity of the DESs. Within each hydrophilic or hydrophobic group, the subgroups are then generated based on HBA and HBD types. Kundu et al. (2020) analyzed experimental data on 88 types of DESs. Among these, 26 fall into the hydrophilic class while the remaining belong to the hydrophobic class. The hydrophilic class comprises 19 ChCl-based DESs, 3 other choline cation-based DESs, 3 betaine DESs, and 1 ethyl (2-hydroxyethyl) dimethylammonium DES. The hydrophobic class is further divided into 34 tetraalkylammonium salt-based DESs (including chloride and bromide anions), 11 menthol-based DESs, and 17 thymol-based DESs. They predicted α and β parameters for these 88 DESs, yielding deviations of less than 2.25% and 3.14%, respectively. Subsequently, these models were applied to predict α and β values for novel hydrophilic and hydrophobic DESs formulated with new HBA and HBD. Based on their predictions, tetraalkylammonium-based hydrophobic deep eutectic solvents (DESs) containing tetraheptylammonium chloride octanoic acid ([N7777]Cl/OctA) and tetraoctylammonium chloride octanoic acid ([N8888]Cl/OctA) show potential for high cellulose dissolution capacity, attributed to their relatively high β values. These estimations align with the experimental data reported by Sirvio and Heiskanen (2020) for tetraethylammonium hydroxide/water, which dissolved 15% cellulose at room temperature.
The KAT parameters were also predicted with the molecular dynamics (MD) and Monte Carlo (MC) approaches based on interactions with solvatochromic dye. However, developing reliable force fields for the DESs is still challenging. Consequently, the accuracy of predicted datasets remains questionable when employing the MD approach. Moreover, both approaches are computationally expensive, and given the ever-growing number of DES, constructing a comprehensive database using these approaches would be time consuming. Therefore, these methods are not suitable for a fast priori prediction.
5 Future directions and conclusion
Knowing the hydrogen bond acceptor strength (β) and viscosity of the DESs can provide insight into their capacity for dissolving cellulose. However, the experimental measurement of these quantities is a significant challenge and practically unfeasible task due to numerous alternative HBA and HBD couples that can be used in DES formulation. At this stage, computational tools can serve as a valuable resource for fast screening alternative DES formulations (Figure 2).
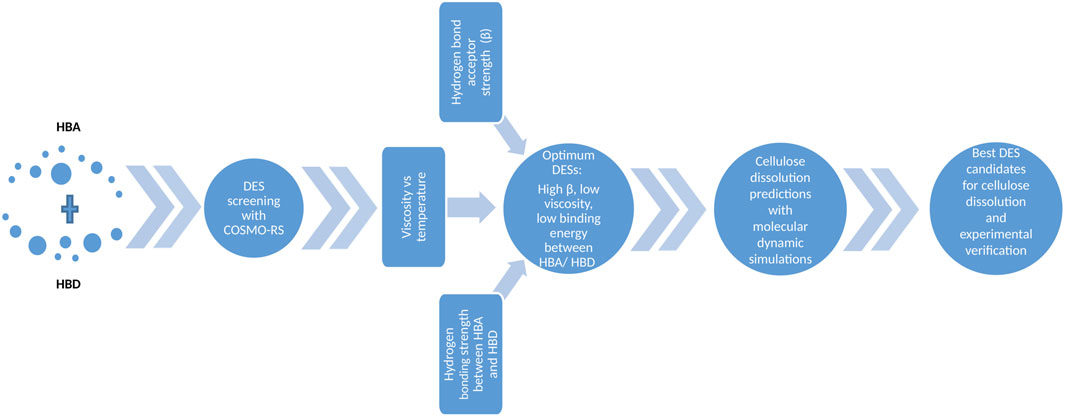
Figure 2. COSMO-RS and molecular dynamics simulation aided design of deep eutectic solvents for cellulose dissolution.
The COSMO-RS-aided design proves helpful in attaining this objective, as it can predict KAT parameters, viscosity, and hydrogen bonding strength between HBA and HBD groups in DES. Screening numerous DES formulations to identify those with high hydrogen bond basicity (β), low viscosity, low activation energy, and weak hydrogen-bonding strength saves time and resources. As a result, this approach enhances the chances of discovering promising DESs. The subsequent step involves implementing molecular dynamic simulations to predict cellulose dissolution with optimal DES formulations, followed by experimental verification of the predictions. Utilizing computational power and experimental tools is believed to advance our current understanding of cellulose dissolution in DESs. The accuracy of COSMO-RS predictions is significantly dependent on the precise theoretical representation of interaction energies within the framework. As a result, further improvement of the predicted and extended scale database should be achieved by incorporating various interaction parameters available in the literature. Moreover, the emergence of artificial intelligence (AI)-based methodologies provides a novel avenue for establishing correlations between structure and material properties.
Cellulose dissolution occurs only when the dissolved state has lower energy compared to the solid state, indicating a balance between the enthalpy of mixing and the entropy term. From this perspective, designing DESs with excellent interaction with cellulose, thereby overcoming the low entropy gain, and providing an exothermic heat of mixing will enhance cellulose dissolution. Given that cellulose has both hydrophilic (equatorial OH-groups) and hydrophobic (axial CH groups) features, DES formulations should incorporate both a hydrogen bonding component and a hydrophobic segment to achieve high dissolution capacity for cellulose. Additionally, the experimental findings suggest that the HBA and HBD couples for DES design should feature short chains and single sites for binding. This design choice leads to DESs with lower molecular weights, resulting in reduced viscosity and weaker hydrogen bonding.
The exploration of industrial applications for DESs is still in its early stages of development. Future research in this area should focus on developing tailored DESs with low viscosity, high thermal stability, long lifetime, and the ability to provide efficient dissolution at room temperature within a short timeframe.
Author contributions
SA: Conceptualization, Data curation, Formal Analysis, Investigation, Writing–original draft, Writing–review and editing.
Funding
The author(s) declare that no financial support was received for the research, authorship, and/or publication of this article.
Conflict of interest
The author declares that the research was conducted in the absence of any commercial or financial relationships that could be construed as a potential conflict of interest.
The author(s) declared that they were an editorial board member of Frontiers, at the time of submission. This had no impact on the peer review process and the final decision.
Publisher’s note
All claims expressed in this article are solely those of the authors and do not necessarily represent those of their affiliated organizations, or those of the publisher, the editors and the reviewers. Any product that may be evaluated in this article, or claim that may be made by its manufacturer, is not guaranteed or endorsed by the publisher.
References
Cardellini, F., Germani, R., Cardinali, G., Corte, L., Roscini, L., Spreti, N., et al. (2015). Room temperature deep eutectic solvents of (1S)-(+)-10-camphorsulfonic acid and sulfobetaines: hydrogen bond-based mixtures with low ionicity and structure-dependent toxicity. RSC Adv. 5, 31772–31786. doi:10.1039/c5ra03932k
Chen, Y.-L., Zhang, X., You, T.-T., and Xu, F. (2019). Deep eutectic solvents (DESs) for cellulose dissolution: a mini-review. Cellulose 26, 205–213. doi:10.1007/s10570-018-2130-7
Chundawat, S. P. S., Sousa, L. D., Roy, S., Yang, Z., Gupta, S., Pal, R., et al. (2020). Ammonia-salt solvent promotes cellulosic biomass deconstruction under ambient pretreatment conditions to enable rapid soluble sugar production at ultra-low enzyme loadings. Green Chem. 22, 204–218. doi:10.1039/c9gc03524a
Egal, M., Budtova, T., and Navard, P. (2008). The dissolution of microcrystalline cellulose in sodium hydroxide-urea aqueous solutions. Cellulose 15, 361–370. doi:10.1007/s10570-007-9185-1
Fan, C., Liu, Y., Sebbah, T., and Cao, X. L. (2021). A theoretical study on terpene-based natural deep eutectic solvent: relationship between viscosity and hydrogen-bonding interactions. Glob. Challenges 5, 2000103. doi:10.1002/gch2.202000103
Flieger, J., and Flieger, M. (2020). Ionic liquids toxicity—benefits and threats. Int. J. Mol. Sci. 21, 6267. doi:10.3390/ijms21176267
Francisco, M., van den Bruinhorst, A., and Kroon, M. C. (2012). New natural and renewable low transition temperature mixtures (LTTMs): screening as solvents for lignocellulosic biomass processing. Green Chem. 14, 2153–2157. doi:10.1039/c2gc35660k
Ghaedi, H., Ayoub, M., Sufian, S., Shariff, A. M., and Lal, B. (2017). The study on temperature dependence of viscosity and surface tension of several Phosphonium-based deep eutectic solvents. J. Mol. Liq. 241, 500–510. doi:10.1016/j.molliq.2017.06.024
Hakkinen, R., and Abbott, A. (2019). Solvation of carbohydrates in five choline chloride-based deep eutectic solvents and the implication for cellulose solubility. Green Chem. 21, 4673–4682. doi:10.1039/c9gc00559e
Hayyan, M., Mbous, Y. P., Looi, C. Y., Wong, W. F., Hayyan, A., Salleh, Z., et al. (2016). Natural deep eutectic solvents: cytotoxic profile. SpringerPlus 5, 913. doi:10.1186/s40064-016-2575-9
Heinze, T., and Koschella, A. (2005). Solvents applied in the field of cellulose chemistry: a mini review. Polı´meros 15, 84–90. doi:10.1590/s0104-14282005000200005
Hertel, M., Bommarius, A., Realff, M., and Kang, Y. (2012). Deep eutectic solvent systems and methods. Patent. WO2012/145522.
Hildebrand, J. H., and Scott, R. L. (1962). Regular solutions. Prentice Hall. Englewood Cliffs, N. J.
Ismail, N., Pan, J., Rahmati, M., Wang, Q., Bouyer, D., Khayet, M., et al. (2022). Non-ionic deep eutectic solvents for membrane formation. J. Membr. Sci. 646, 120238. doi:10.1016/j.memsci.2021.120238
Jin, S., Byrne, F., McElroy, C. R., Sherwood, J., Clark, J. H., and Hun, A. J. (2017). Challenges in the development of bio-based solvents: a case study on methyl(2,2-dimethyl-1,3-dioxolan-4- yl)methyl carbonate as an alternative aprotic solvent. Faraday Discuss. 202, 157–173. doi:10.1039/c7fd00049a
Jordana, A., and Gathergood, N. (2015). Biodegradation of ionic liquids – a critical review. Chem. Soc. Rev. 44, 8200–8237. doi:10.1039/c5cs00444f
Kulicke, M., and Kniewsker, R. (1984). The shear viscosity dependence on concentration, molecular weight, and shear rate of polystyrene solutions. Rheol. Acta 23, 75–83. doi:10.1007/bf01333878
Kundu, D., Rao, P. S., and Banerjee, T. (2020). First-principles prediction of Kamlet−Taft solvatochromic parameters of deep eutectic solvent using the COSMO-RS model. Industrial Eng. Chem. Res. 59, 11329–11339. doi:10.1021/acs.iecr.0c00574
Lynam, J. G., Kumar, N., and Wong, M. J. (2017). Deep eutectic solvents’ ability to solubilize lignin, cellulose, and hemicellulose; thermal stability; and density. Bioresour. Technol. 238, 684–689. doi:10.1016/j.biortech.2017.04.079
Malaeke, H., Housaindokht, M. R., Monhemi, H., and Izadyar, M. (2018). Deep eutectic solvent as an efficient molecular liquid for lignin solubilization and wood delignification. J. Mol. Liq. 263, 193–199. doi:10.1016/j.molliq.2018.05.001
Miller-Chou, B. A., and Koenig, J. L. (2003). A review of polymer dissolution. Prog. Polym. Sci. 28, 1223–1270. doi:10.1016/s0079-6700(03)00045-5
Pan, M., Zhao, G., Ding, C., Wu, B., Lian, Z., and Lian, H. (2017). Physicochemical transformation of rice straw after pretreatment with a deep eutectic solvent of choline chloride/urea. Carbohydr. Polym. 176, 307–314. doi:10.1016/j.carbpol.2017.08.088
Raus, V., Sturcova, A., Dybal, J., Slouf, M., Vackova, T., Salek, P., et al. (2012). Activation of cellulose by 1,4-dioxane for dissolution in N,N-dimethylacetamide/LiCl. Cellulose 19, 1893–1906. doi:10.1007/s10570-012-9779-0
Ren, H., Chen, C., Guo, S., Zhao, D., and Wang, Q. (2016b). Synthesis of a novel allyl-functionalized deep eutectic solvent to promote dissolution of cellulose. BioResources 11 (4), 8457–8469. doi:10.15376/biores.11.4.8457-8469
Ren, H. W., Chen, C. M., Wang, Q. H., Zhao, D. S., and Guo, S. H. (2016a). The properties of choline chloride-based deep eutectic solvents and their performance in the dissolution of cellulose. BioResources 11, 5435–5451. doi:10.15376/biores.11.2.5435-5451
Rosenau, T., Potthast, A., Adorjan, I., Hofinger, A., Sixta, H., Firgo, H., et al. (2002). Cellulose solutions in N-methylmorpholine-N-oxide (NMMO)–degradation processes and stabilizers. Cellulose 9, 283–291. doi:10.1023/a:1021127423041
Sharma, G., Takahashi, K., and Kuroda, K. (2021). Polar zwitterion/saccharide-based deep eutectic solvents for cellulose processing. Carbohydr. Polym. 267, 118171. doi:10.1016/j.carbpol.2021.118171
Sharma, M., Mukesh, C., Mondal, D., and Prasad, K. (2013). Dissolution of α-chitin in deep eutectic solvents. RSC Adv. 3, 18149–18155. doi:10.1039/c3ra43404d
Sirvio, J. A., and Heiskanen, J. P. (2020). Room-temperature dissolution and chemical modification of cellulose in aqueous tetraethylammonium hydroxide–carbamide solutions. Cellulose 27, 1933–1950. doi:10.1007/s10570-019-02907-x
Swatloski, R. P., Spear, S. K., Holbrey, J. D., and Rogers, R. D. (2002). Dissolution of cellulose [correction of cellose] with ionic liquids. J. Am. Chem. Soc. 124 (18), 4974–4975. doi:10.1021/ja025790m
Tenhunen, T.-M., Lewandowska, A. E., Orelma, H., Johansson, L.-S., Virtanen, T., Harlin, A., et al. (2017). Understanding the interactions of cellulose fibres and deep eutectic solvent of choline chloride and urea. Cellulose 25, 137–150. doi:10.1007/s10570-017-1587-0
Tong, Z., Meng, J., Liu, S., Liu, Y., Zeng, S., Wang, L., et al. (2021). Room temperature dissolving cellulose with a metal salt hydrate-based deep eutectic solvent. Carbohydr. Polym. 272, 118473. doi:10.1016/j.carbpol.2021.118473
Tong, Z., Wang, W., Zeng, S., Sun, Y., Meng, J., Liu, Y., et al. (2022). Hydrogen bond reconstruction strategy for eutectic solvents that realizes room-temperature dissolution of cellulose. Green Chem. 24, 8760–8769. doi:10.1039/d2gc03372k
Ueki, T., and Watanabe, M. (2012). Polymers in ionic liquids: dawn of neoteric solvents and innovative materials. Bull. Chem. Soc. Jpn. 85, 33–50. doi:10.1246/bcsj.20110225
Velioglu, S., Yao, X., Devémy, J., Ahunbay, M. G., Tantekin-Ersolmaz, S. B., Dequidt, A., et al. (2014). Solvation of a cellulose microfibril in imidazolium acetate ionic liquids: effect of a cosolvent. J. Phys. Chem. B 118, 14860–14869. doi:10.1021/jp508113a
Wang, J., Wang, Y., Ma, Z., and Yan, L. (2020). Dissolution of highly molecular weight cellulose isolated from wheat straw in deep eutectic solvent of Choline/L-Lysine hydrochloride. Green Energy & Environ. 5, 232–239. doi:10.1016/j.gee.2020.03.010
Winterton, N. (2006). Solubilization of polymers by ionic liquids. J. Mater. Chem. 16, 4281–4293. doi:10.1039/b610143g
Xiong, P., Zhao, K., Hu, L., Zhang, L., and Cheng, G. (2014). Dissolution of cellulose in aqueous NaOH/urea solution: role of urea. Cellulose 21, 1183–1192. doi:10.1007/s10570-014-0221-7
Zhang, C., Liu, R., Xiang, J., Kang, H., Liu, Z., and Huang, Y. (2014). Dissolution mechanism of cellulose in N,N-dimethylacetamide/lithium chloride: revisiting through molecular interactions. J. Phys. Chem. B 118, 9507–9514. doi:10.1021/jp506013c
Zhang, H., Lang, J., Lan, P., Yang, H., Lu, J., and Wang, Z. (2020). Study on the dissolution mechanism of cellulose by ChCl-based deep eutectic solvents. Materials 13, 278. doi:10.3390/ma13020278
Zhang, J., Wu, J., Yu, J., Zhang, X., He, J., and Zhang, J. (2017). Application of ionic liquids for dissolving cellulose and fabricating cellulose-based materials: state of the art and future trends. Mater. Chem. Front. 1, 1273–1290. doi:10.1039/c6qm00348f
Zhang, L., Yu, H., Liu, S., Wang, Y., Mu, T., and Xue, Z. (2023). Kamlet−Taft parameters of deep eutectic solvents and their relationship with dissolution of main lignocellulosic components. Ind. Eng. Chem. Res. 62, 11723–11734. doi:10.1021/acs.iecr.3c01309
Zhao, H., Jones, C. L., Baker, G. A., Xia, S., Olubajo, O., and Person, V. N. (2009). Regenerating cellulose from ionic liquids for an accelerated enzymatic hydrolysis. J. Biotechnol. 139, 47–54. doi:10.1016/j.jbiotec.2008.08.009
Keywords: deep eutectic solvents, cellulose, polymer membranes, COSMO-RS aided deep eutectic solvent design, green solvent, basic deep eutectic solvent
Citation: Alsoy Altinkaya S (2024) A perspective on cellulose dissolution with deep eutectic solvents. Front. Membr. Sci. Technol. 3:1382054. doi: 10.3389/frmst.2024.1382054
Received: 04 February 2024; Accepted: 15 March 2024;
Published: 09 April 2024.
Edited by:
Nalan Kabay, Ege University, TürkiyeReviewed by:
Isabel Coelhoso, NOVA University of Lisbon, PortugalMarek Bryjak, Wrocław University of Technology, Poland
Copyright © 2024 Alsoy Altinkaya. This is an open-access article distributed under the terms of the Creative Commons Attribution License (CC BY). The use, distribution or reproduction in other forums is permitted, provided the original author(s) and the copyright owner(s) are credited and that the original publication in this journal is cited, in accordance with accepted academic practice. No use, distribution or reproduction is permitted which does not comply with these terms.
*Correspondence: Sacide Alsoy Altinkaya, sacidealsoy@iyte.edu.tr