Effects of row spacing on soil nitrogen availability, wheat morpho-physiological traits and radiation use efficiency
- 1College of Agronomy, Henan Agricultural University/Key Laboratory of Regulating and Controlling Crop Growth and Development, Ministry of Education, Zhengzhou, China
- 2College of Life Science, Henan Agricultural University, Zhengzhou, China
Optimizing row spacing is an important measure to exploit the full yield potential, achieve a continuous increase in wheat yield and green development without increasing input. The objective of this study was to compare the effects of wide–narrow row spacing pattern (12 cm-12 cm-12 cm-24 cm, R1) and conventional uniform row spacing mode (20 cm, CK; 12 cm. R2) on nitrogen (N) availability in soil, canopy structure, photosynthetic characteristics, radiation use efficiency (RUE) and yield. The results showed that R1 increased the relative abundance of ammonia-oxidizing bacteria and ammonia-oxidizing archaea in the rhizosphere compared to CK. Nitrate in soils treated R1 at post-anthesis and nitrogen use efficiency of the plant were 59.92% and 27.01% higher than those treated with CK, respectively. Above-ground growth of wheat showed that R1 increased leaf area index by 27.42%, specific leaf weight by 22.67% and leaf photosynthetic rate by 8.86%, respectively compared to CK. R2 had similar availability of N and plant growth as CK. Moreover, the ability of the plant to allocate more nitrogen to grains was enhanced at post-anthesis with R1 than it with CK and R2, which greatly improved the RUE by 10.13% and 2.27%. As the result, the yield for R1 increased by 27.54% and 21.46%, respectively, compared to CK and R2. Therefore, using wide–narrow row spacing pattern (R1) is a practically and environmentally feasible approach for wheat production without extra input in the straw-returning wheat-corn rotation system.
1 Introduction
Wheat is an essential food crop around the globe, and its high and stable yield has become global food security issue. China is the largest wheat producer, accounting for 17% of world wheat production (1), and approximately 50% of production comes from the North China plain (2). Constructing a reasonable canopy structure is helpful to realize the full potential of crop production. The canopy structure was not only different among varieties but also affected by water/fertilizer management and field arrangements (3–5). However, faced with limited resources and the objective of green production, yield seems impossible to achieve larger gains by increasing the cultivated area or investments of water and fertilizer (6, 7). Future yield gains must rely on the improvements in resource use efficiency. In this context, biomass production depends on the capacity to use solar radiation, which is known as radiation use efficiency (RUE). Thus, adjusting agronomic practices such as manipulation of row arrangements are presumably important for increasing radiation use efficiency and yield.
The structural arrangement of wheat was able to regulate through adjusting row spacing or density (8). Increasing the spacing at a constant density will increase the spatial heterogeneity of the crop population, producing a stronger impact than increasing density and effectively reducing the amount of seeding (9). So, optimizing row spacing was a more effective measure to increase yield without increasing seeding rate. Increasing row spacing favors the allocation of dry matter to grain (10), which can be explained by reduced competition between plants (11), while too wide row spacing would reduce the mean leaf angle, leaf area index and canopy light interception, and the resources might not be used efficiently. Decreasing row spacing was beneficial in reducing light loss and increasing leaf area index and yield (12), while too narrow row spacing might have negative effects on plant growth and development because of significant competition. To solve these problems, wide-narrow row spacing might be a good solution to reduce plant competition and increase RUE and yield. Many research showed a strong effect of wide-narrow row spacing mode on crops such as maize, rape and cotton (13, 14). As for wheat, the study found that wide-narrow row spacing mode under straw return and reduced tillage conditions have positive effects on yield and tillage efficiency of wheat (15). Additionally, adjusting row spacing mode will affect the spatiotemporal distribution of plant roots and the formation of soil aggregates, which are important for the crop to obtain soil nutrients (16). It’s essential to seek rational row spacing and understand its mechanism of increasing yield for exploiting wheat yield potential with existing inputs, which need to focus on nutrient availability and plant growth.
Plant photosynthesis and dry matter accumulation are closely related to soil nutrient supply capacity. An adequate supply of nitrogen and improvement of N use efficiency maintained a high photosynthetic rate and provided carbohydrates for yield formation (17). The final N accumulation in grain is mainly due to N uptake from soil and N retransfer from vegetative organs during the filling stage (18). The improvement of soil nutrient status and plant N utilization by optimizing row spacing pattern has been proved in maize (19). However, whether a similar response exists in wheat was unclear. Inorganic fertilizer in the soil is transformed into nutrients that can be directly absorbed by crop roots through the metabolic activities of soil microorganisms (20). For example, ammonium is quickly transformed to nitrate in the soil by nitrification, and nitrate is the primary source of inorganic nitrogen uptake by plant roots in aerobic soil (21). The ammonia oxidation process involving ammonia-oxidizing bacteria (AOB) and ammonia oxidizing archaea (AOA) is a key rate-limiting step in soil nitrification, which affects the nitrate content in soil (22, 23). Heterogeneous distribution of microbial in the soil is well-known. The rhizosphere microorganisms have an important influence on the growth and development of plants. Not only does it affect the soil N availability (24), but also is the driving factor for the secretion of organic acids and other substances by the roots (25). Most of N absorbed from soil is transported to the leaves for assimilation, and leaf photosynthate (C) is deposited in the grain and root via phloem tissue. In turn, the C allocated to roots could regulate the absorption of N (26), which have the potential to modify the rhizosphere environment, and affect microbial abundance (19).
In the current study, we conducted a field experiment over two consecutive years. Wheat was grown with three different row spacing modes. We explore the effects of row spacing modes on soil N availability, wheat canopy morphology, leaf photosynthetic characteristics and yield, with the aim to find an optimal row spacing mode. The results provide useful information for appropriate wheat cultivation mode recommendations and enhancing grain yield.
2 Materials and methods
2.1 Site description and experimental design
The experiment was conducted at the experimental station of Henan Agricultural University in Xuchang, Henan Province, China (34°08’6.69”N, 113°48’7.87”E). Weather conditions during the wheat growth period for two seasons are given in Figure S1. The soil type was loam, which contained 16.53 g kg−1 organic matter, 1.22 g kg−1 total N, 53.38 mg kg−1 available N, 10.14 mg kg−1 available phosphate, and 208.75 mg kg−1 available K.
The wheat cultivar Zhoumai27 were grown at three row spacing modes under same density (270 plant/m2): a wide-narrow row spacing termed R1 (spacing = 12 cm-12 cm-12 cm-24 cm) (Figure 1, R1), a narrow uniform row spacing termed R2 (spacing = 12 cm) (Figure 1, R2), and the typical farmer’s uniform row spacing termed CK (spacing = 20 cm) (Figure 1, CK). Three replicates of each treatment were laid out in a completely randomized experimental design. The planting direction was north-south, and the area of each plot was 28 m2 (4 m × 7 m). Wheat was sown on October 20, 2015 and October 20, 2016. Harvest dates were June 10, 2016 and June 5, 2017. The nitrogen application to wheat was 225 kg hm−2, of which 60% was applied before sowing and 40% was applied at the jointing stage (z32). The amount of phosphate fertilizer (P2O5) and potassium fertilizer (KCl) applied to wheat was 120 kg hm−2, and both were applied before wheat sowing. Other cultivation-management measures were the same as those performed according to high-yield fields.
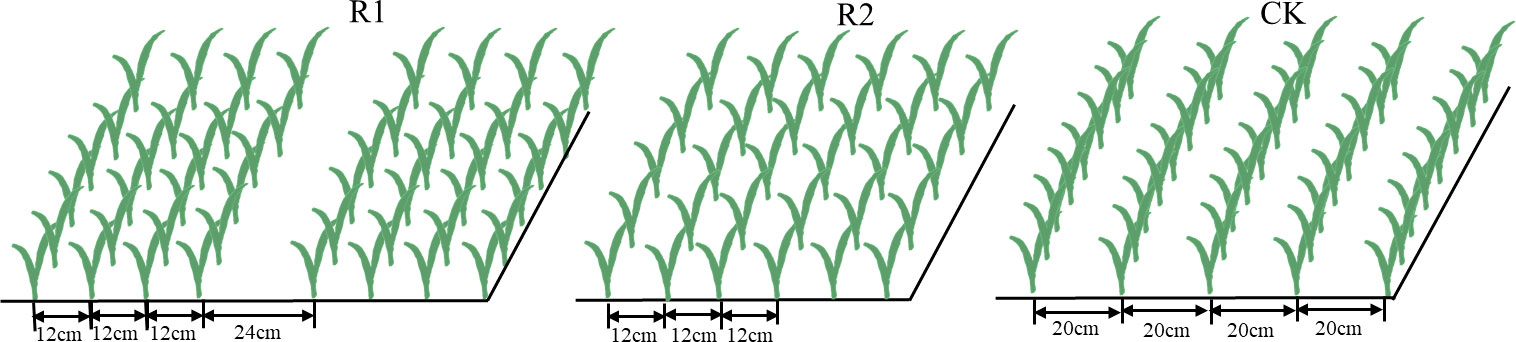
Figure 1 Illustration of different row spacings. CK, uniform planting (20 cm); R1, wide-narrow row planting (12 cm-12 cm-12 cm-24 cm); R2, narrow uniform planting (12 cm). Three row spacings used at the same population density.
2.2 Data acquisition
2.2.1 Wheat canopy structure and plant biomass
Mean leaf angle (MLA) and diffuse non-interceptance (DIFN) were measured near the center of each plot with LAI 2200C Canopy Analyzer (LI-COR, Lincoln, NE, USA) from 8 a.m. to 10 a.m. at anthesis (z65). The sensor was placed 5 cm above ground when measuring; the measures were performed in wide and narrow rows under R1 because of asymmetrical row spacing and the average of the results was used as the final data. Each measurement was repeated six times.
At anthesis (z65), 20 days after anthesis (z85), and maturity (z91), ten wheat plants were selected randomly near the center of each plot. Five plants were selected randomly from wide and narrow rows, respectively, under R1. Leaf area was measured using a laser area meter (CI-203; CID Bio-Science, USA), and samples were placed in a constant temperature drying box at 105°C for 20 minutes, followed by drying to constant weight at 80°C to determine leaf weight and plant biomass. Finally, biomass accumulations were calculated during z65–z85 and z85–z91. The following formulas were used to estimate leaf area index (LAI) and specific leaf weight (SLW, g m−2):
where LA is the leaf area per plant (m2), GA is the ground area per plant (m2), and LW is the leaf dry weight (g).
2.2.2 Light interception in the wheat population
An AccuPAR canopy analyzer (LP-80, METER, USA) was used to measure photosynthetically active radiation (PAR) at the top (20 cm above the canopy) and bottom of the canopy at anthesis (z65), 20 days after anthesis (z85), and maturity (z91). The sensor was placed at the top (20 cm above the canopy) and bottom of canopy in wide and narrow rows to determine the total PAR interception under R1. The light extinction coefficient of the canopy (K), intercepted photosynthetically active radiation (PAR) accumulation (IPAR; MJ m−2) (27, 28), PAR interception efficiency (In), and RUE were estimated with the following formulas:
where TPAR is the surface of the PAR; PAR is the radiation flux density at the top of the canopy; Qa is the total PAR accumulation between sampling dates; LAIm and Km are averaged between sampling dates; W is the biomass accumulation per unit area between sampling dates calculated in 2.2.1.
2.2.3 Wheat photosynthetic parameters
At anthesis (z65), three flag leaves were selected randomly near the center of each plot (To make the data more representative, three samples were selected respectively within wide and narrow rows under R1 to measure, and the average of the results was used as the final data point.) to determine the photosynthetic rate (Pn), stomatal conductance (Gs), and intercellular carbon dioxide concentration (Ci) using LI-6400XT portable photosynthesis system (LI-COR, Lincoln, NE, USA) with a red-blue light emitting diode (LED) light source and a light intensity of 1000 μmol m−2 s−1.
2.2.4 Wheat plant nitrogen content
Samples were crushed after the biomass measurements in 2.2.1, and total nitrogen content was measured with an AA3 autoanalyzer (SEAL Analytical, Germany) after H2SO4–H2O2 heating digestion (29).
2.2.5 Soil microbial abundance and nutrient content
Plants were selected randomly near the center of each plot, and excavated from the soil at the wheat jointing stage (z32) and the completion of anthesis (z69). Plants were selected randomly from wide and narrow rows, respectively, under R1, and put together to further analysis. Soil that remained closely adhered to the root system was used as the rhizosphere soil sample to determine relevant microbial parameters. Real-time PCR was used to measure the abundance of ammonia-oxidizing bacteria (AOB) and ammonia-oxidizing archaea (AOA). Gene copies of the ammonia monooxygenase genes amoA and arch-amoA were used to estimate the abundance of AOB and AOA, respectively, and each sample was repeated three times. Primers and amplification conditions are described in Table S1 (30, 31). The 20 μl real-time PCR reaction system included 2 μl DNA template, 10 μl SYBR, 0.4 μl each of upstream and downstream primers, and 7.2 μl ddH2O.
Soil samples were placed in ice boxes, transferred to the laboratory and stored in a 4°C refrigerator for further analysis. A portion was used for measurement of soil microbial biomass carbon and nitrogen by potassium dichromate titration and an AA3 autoanalyzer (SEAL Analytical, Germany) after chloroform fumigation–K2SO4 extraction. Another portion was used for the measurement of nitrate and ammonium content with an AA3 autoanalyzer (SEAL Analytical, Germany) after KCl leaching filtration.
2.2.6 Wheat yield and nitrogen use efficiency
When the wheat had matured, two complete 1 m2 regions of wheat in each plot were selected and harvested manually to determine yield and 1000-grain weight. Two rows of 1 m were used to count the number of ears per unit area in the field, and 30 ears of wheat were randomly selected from each plot to calculate the average number of kernels per ear. All measurements were replicated three times. Nitrogen uptake efficiency (NUpE), nitrogen utilization efficiency (NUtE) and nitrogen use efficiency (NUE) were calculated using the equation:
where QtyN is total N content in the aboveground tillers, including grains at harvest/maturity time point; NP is the total amount of N applied; YG is grain yield.
2.3 Data analysis
Data are presented as mean ± SD (standard deviation) of biological repetition, and were analyzed by one-way ANOVA using SPSS statistical software (IBM, USA). Multiple comparisons among the treatments were performed with the least significant difference (LSD) test, and the significance level at the 0.05 probability level (P < 0.05).
3 Results
3.1 Effect of row spacing modes on wheat yield
Row spacing modes significantly affected the yields of wheat during two growing seasons. Compared to CK and R2, the wheat yield of R1 was 9.83% and 4.57% higher, respectively. In terms of yield components, the kernels per ear and 1000-kernel weight were significantly higher in R1. The number of ears per unit area was also higher in R1, and the differences between R1 and other treatments in 2016–2017 reached significant level. Additionally, the nitrogen accumulation of single plants differed with row spacing and was 8.9% and 5.7% higher in R1 than in CK and R2, respectively (Table 1). In addition, Zhoumai27 used in this research is a widely used cultivar in China, and its plant type is loose. To verify whether the wide-narrow row spacing used in this study is also applicable to other cultivar types, we conducted verification tests with other cultivars. R1 also improved yield by 10.05%-13.91% in other cultivars (Table S2) compared with CK.
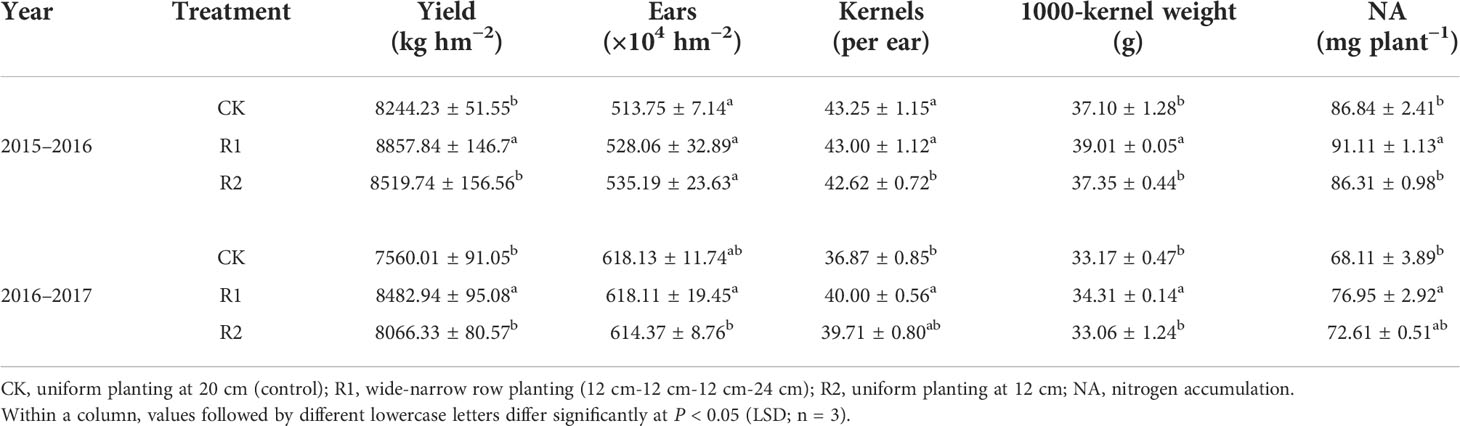
Table 1 Effects of row spacing on wheat yield, yield components, and nitrogen accumulation during two years.
3.2 Effect of row spacing on wheat canopy morphology and photosynthetic parameters
As can be seen from Table 2, there were significant differences in wheat canopy morphology among the row spacing modes. In both years, MLA was significantly higher in the narrow row spacing (R2) and wide-narrow row spacing (R1) treatments than in the CK treatment, but the difference between R1 and R2 failed to reach the significant level. DIFN showed the opposite trend and was significantly lower in R2 and R1 compared with CK. Among the treatments, LAI and SLW were the greatest in R1, LAI of R1 was 14.48% and 27.42% higher than R2 and CK, and SLW of R1 was 12.67% and 22.67% higher than R2 and CK, respectively. These results suggest that the mode of wide-narrow row spacing produces beneficial changes in canopy structure and leaf morphology and provides improved conditions for light interception.
Different row spacing modes affected light interception (In) and leaf photosynthetic efficiency of wheat. Table 3 shows that In and IPAR in R1 and R2 were significantly higher than those in CK (9.41% and 11.61% higher for In and 5.32% and 8.11% higher for IPAR) from anthesis to 20 days after anthesis (z65–z85), and neither parameter differed significantly between R1 and R2. From 20 days after anthesis to maturity (z85–z91), In showed the same pattern as before, but there were no significant differences in IPAR among the treatments.
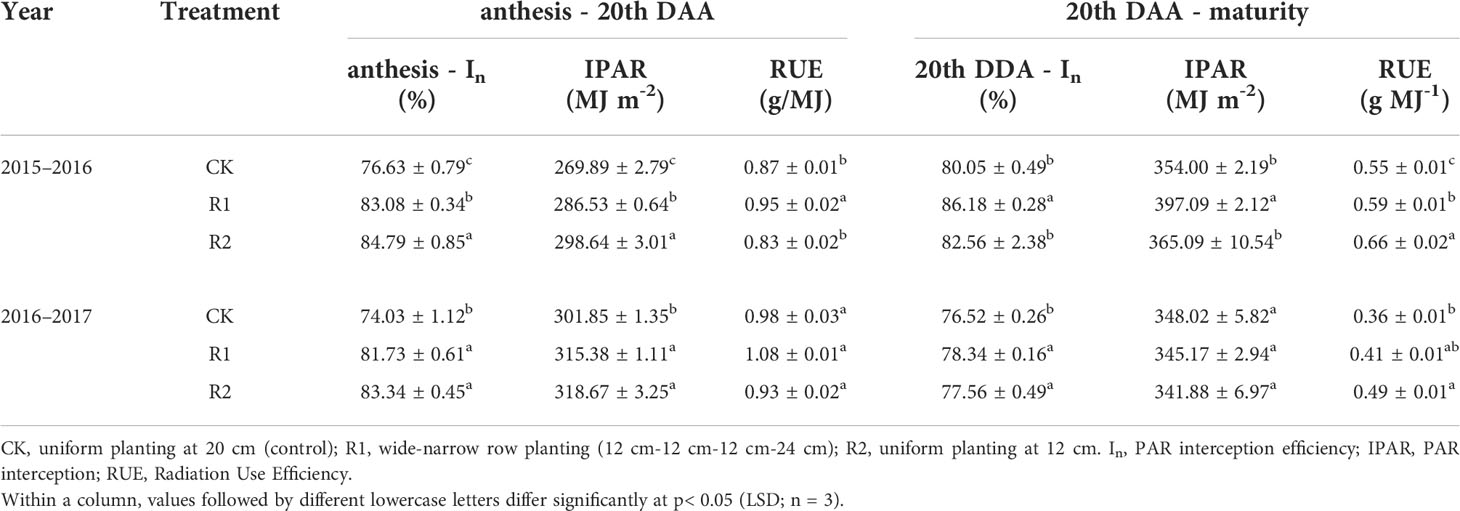
Table 3 PAR interception (IPAR), PAR interception efficiency (In) and Radiation Use Efficiency (RUE).
Differences in canopy light accumulation affected the leaf photosynthetic rate. Table 4 shows that net photosynthetic rate (Pn) of the flag leaf under R1 was significantly higher than that under R2 and CK. Specifically, Pn in R1 was 6.95% and 8.86% higher compared with R2 and CK. Leaf stomatal conductance (Gs) also followed the same pattern, and intercellular carbon dioxide concentration (Ci) was lowest in CK (Table 4).
The radiation use efficiency (RUE) of the treatments also differed between the two periods. RUE in R1 was the highest from anthesis to 20 days after anthesis (z65–z85) and was 9.7% and 15.29% higher than CK and R2, respectively. From 20 days after anthesis to maturity (z85–z91), RUE of the treatments was ranked R2> R1> CK, and RUE of R2 was 28.06% and 15.69% higher than that of CK and R1, respectively (Table 3). The increase in RUE during the filling stage was beneficial to grain yield formation.
3.3 Effect of wheat row spacing on soil microbiota and soil nutrient status
Row spacing had a significant effect on the abundance of ammonia-oxidizing microorganisms in the rhizosphere soil (Figure 2). At the jointing stage (z32), the abundance of AOB was greater than that of AOA, and the AOA/AOB ratio was less than one in all treatments. AOB abundance in all treatments was ranked R1> CK >R2, and AOB abundance in R1 was 33.5% and 3.13% higher than that in CK and R2, respectively. At anthesis completion (z69), the soil AOA abundance increased sharply and that of AOB decreased, and the AOA/AOB ratio rose to greater than one in all treatments. The abundance of both microorganisms was significantly higher in R1 than in other treatments, and the AOA abundance in R1 was 8.79% and 20.04% higher than that in CK and R2, respectively.
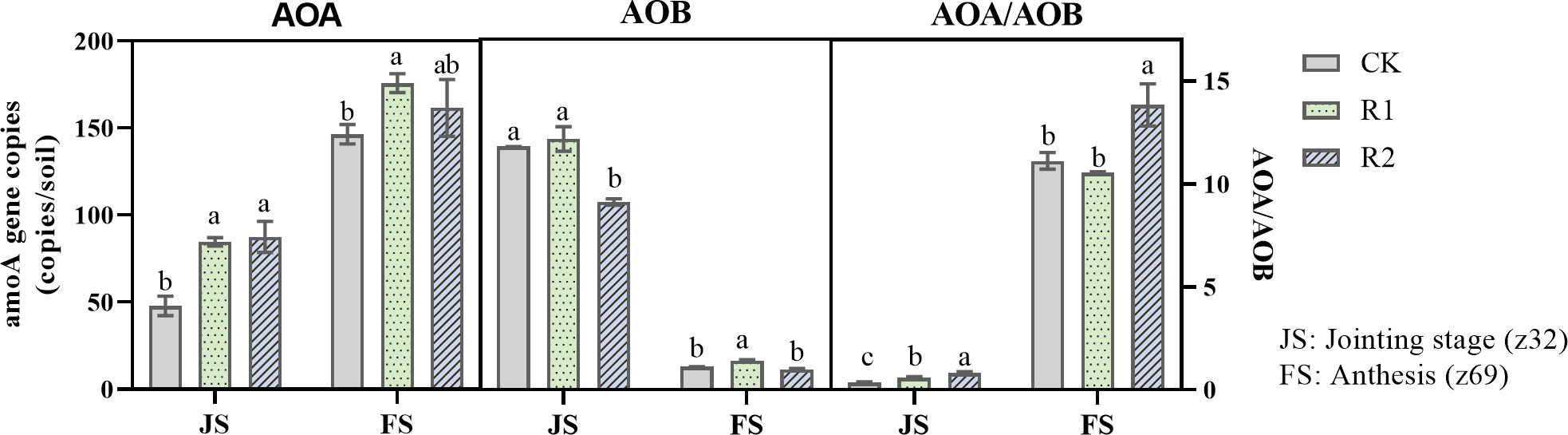
Figure 2 Abundances of ammonia-oxidizing bacteria and ammonia-oxidizing archaea in soil under different treatments in 2016–2017. AOA, ammonia-oxidizing archaea; AOB, ammonia-oxidizing bacteria; AOA/AOB, the ratio of ammonia-oxidizing archaea and ammonia-oxidizing bacteria; CK, uniform planting (20 cm); R1, wide-narrow row planting (12 cm-12 cm-12 cm-24 cm); R2, narrow uniform planting (12 cm). Different letters indicate significant differences among row spacing mode at the P < 0.05 level.
At the jointing stage (z32), microbial biomass carbon and nitrogen in R1 were significantly higher than those in R2 and CK. Specifically, microbial biomass carbon was 18.06% and 13.53% higher in R1 than in R2 and CK, and microbial biomass nitrogen was 9.17% and 11.22% higher in R1 than in R2 and CK. The nitrate-N content was ranked R1> R2> CK, and R1 nitrate content was 28.28% and 11.36% higher than that of CK and R2. At anthesis completion (z69), ammonium content was stable, but soil microbial biomass carbon and nitrogen had decreased markedly. At the same time, nitrate content was higher than at the jointing stage and was ranked R1> R2> CK. The nitrate content of R1 was 59.92% and 9.18% higher than that of CK and R2 (Table 5).
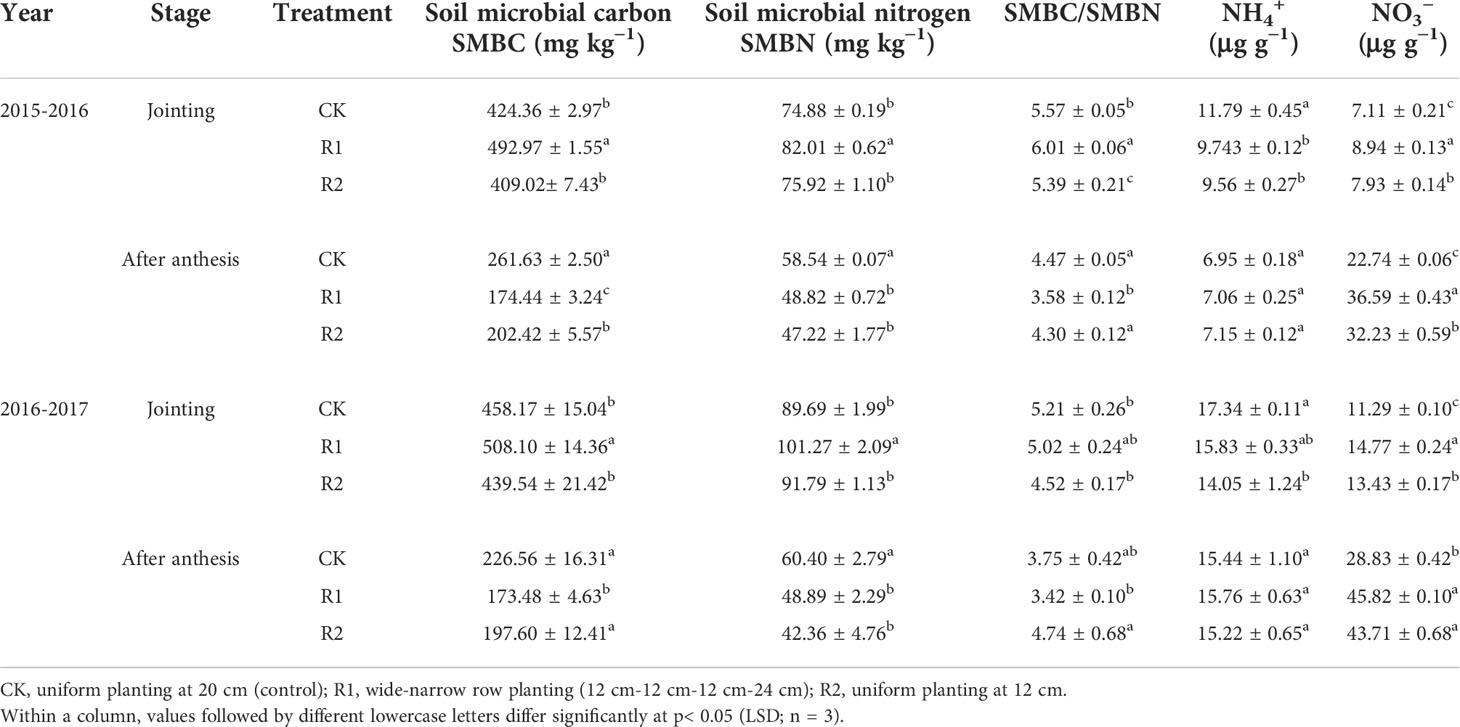
Table 5 Microbial biomass and inorganic nitrogen content in soil under different row spacing treatments in two years.
3.4 Effect of row spacing on nitrogen retransfer from nutrient organs to grain after anthesis, grain nitrogen accumulation and nitrogen use efficiency of wheat
Adjusting row spacings differently altered the wheat nitrogen uptake and transport after anthesis. The nitrogen content of wheat at anthesis (z65) in CK was significantly higher than that in other treatments, although this difference disappeared when the wheat had matured (z91). Table 6 shows that R2 was at a disadvantage in the process of nitrogen accumulation after anthesis. The nitrogen transport performance before anthesis was ranked CK> R1> R2, and the contribution to the grains showed the same pattern. After anthesis, nitrogen accumulation was the highest in R1, and the contribution of remobilized N to grain nitrogen accumulation was ranked R1> R2> CK.
Analysis of plant NUE showed significant improvements under R1 compared to other treatments during two years (Figure 3). In addition, NUpE was significantly 8.99% and 5.69% higher under R1 versus CK and R2, respectively, while there was no significant difference in NUtE among the treatments. Therefore, the increase in NUE of R1 was mainly achieved by increasing the NUpE.
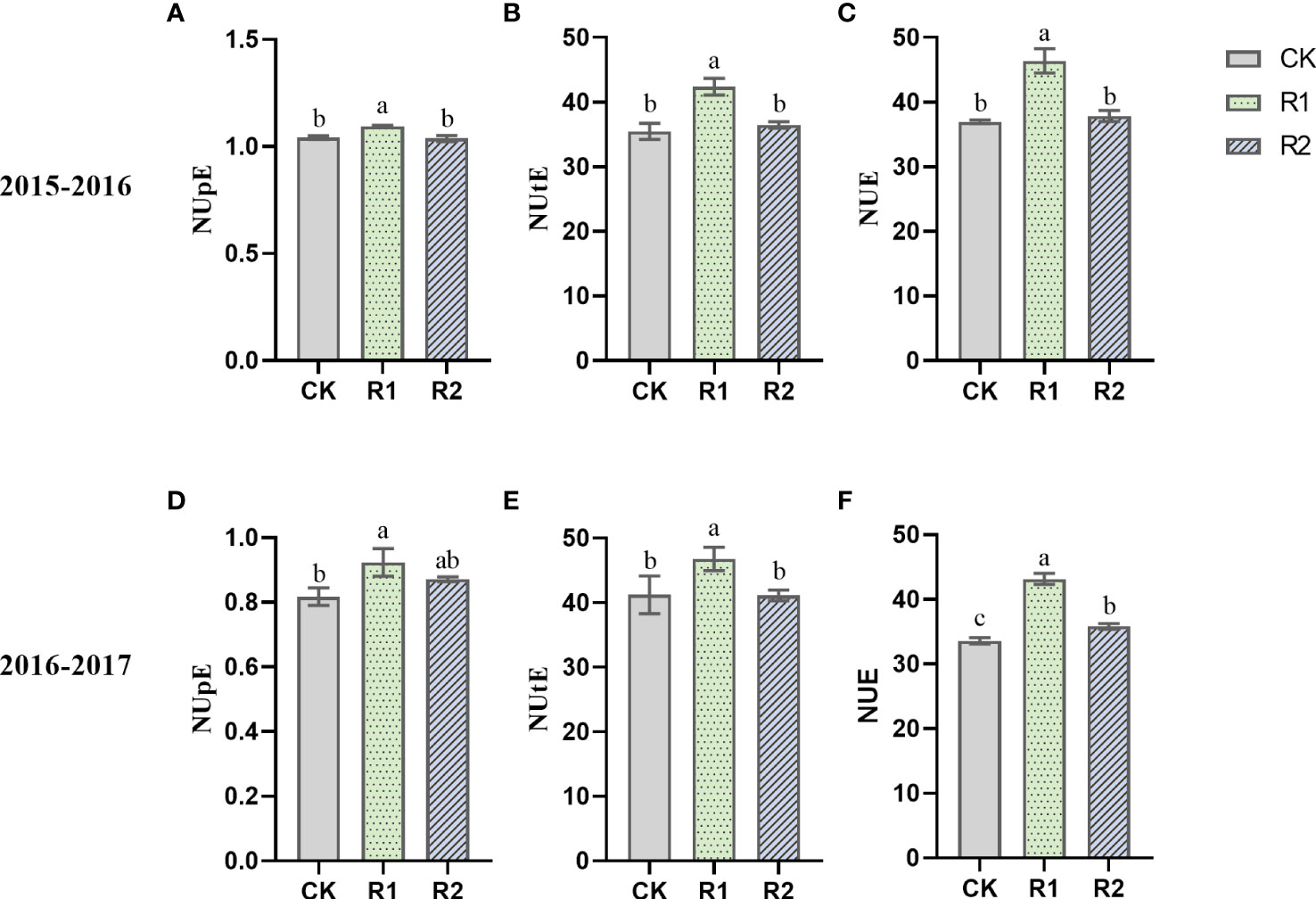
Figure 3 Nitrogen uptake efficiency (NUpE) (A, D), Nitrogen utilization efficiency (NUtE) (B, E) and nitrogen use efficiency (NUE) (C, F) under different treatments in 2016–2017. CK, uniform planting (20 cm); R1, wide-narrow row planting (12 cm-12 cm-12 cm-24 cm); R2, narrow uniform planting (12 cm). Different letters indicate significant differences among row spacing mode in the specific year at the P < 0.05 level.
4 Discussion
Row spacing greatly affected the canopy structure of wheat, consistent with previous research (32, 33). Verhagen has suggested that the optimal canopy structure obtains the maximum effective LAI by continuously changing the distribution of MLA (34). Compared with traditional uniform row spacing (20 cm), R1 had significantly higher LAI and SLW, indicating that the overall light capture of the crop had increased (35–37), as confirmed by IPAR after anthesis. A strong capacity for light capture not only laid the foundation for wheat dry matter accumulation but also improved RUE (Table 3), enabling the R1 to achieve higher RUE than other treatments from anthesis to 20 days after anthesis (z65–z85). Although the wheat canopy structure under R2 had been optimized, the narrow row spacing nonetheless resulted in poor ventilation and light transmission within the crop. The stomatal conductance of the leaves decreased, which had a negative impact on photosynthesis (38). Therefore, the accumulated light within the wheat population increased significantly compared with CK, but the dry matter accumulation from anthesis to 20 days after anthesis (z65-z85) was lower, resulting in low RUE during this period and ultimately affecting both yield and grain nitrogen accumulation. From 20 days after anthesis to maturity (z85–z91), there were almost no differences in light interception and accumulation among all treatments. The narrow row spacing (R2) had a greater dry matter accumulation and RUE, while the dry matter weight per area was still less than that of R1, and the process of transferring the matter to the grain was inferior to that of the other treatments (Table 6), and the yield was not ideal. Wide-narrow row spacing optimizes the wheat crop structure, and the edge effect of the wide row improves the overall light utilization of the crop, offsetting the negative effect brought about by the narrow row (5). As the result, the yield of R1 increased by 3.97%-12.21% compared to uniform row spacing mode.
Plant canopy structure, photosynthesis and other physiological processes were affected by N (Duan et al., 2019). In the case of without extra N fertilizer input, soil N availability and N utilization capacity of plant were important factors determining plant N content and allocation. Studies have shown that the N deficiency will have the consequences of reducing plant leaf index and light interception, affecting the allocation of dry matter to reproductive organs, and reducing yield (39). In our study, the adjustment of row spacing altered the soil N availability. Compared with uniform row spacing mode, wide-narrow row spacing mode significantly increased the nitrate content in the soil, especially at post-anthesis, which was closely related to the activities of soil microorganisms. As the main driving factor for soil nitrogen conversion (40), a richer abundance of ammonia-oxidizing microorganisms has significant positive impacts on soil nitrification (41), and inorganic fertilizer in the soil converted to nitrate more rapidly, which increased the soil N availability. In our study, Narrowing the row spacing had a positive influence on soil AOA abundance and a negative influence on AOB abundance. However, the wide and narrow row spacing created an edge effect, and the overall growth spacing was appropriate, so the abundance of both AOA and AOB increased, and the ratio (AOA/AOB) fluctuation was small (Figure 2). This meant that the soil could be activated effectively (42, 43), and the content of microbial biomass carbon and nitrogen was also improved. The value of AOA/AOB under each treatment was less than 1 at the jointing stage (z32), indicating that the number of AOB was dominant. Some studies have shown that N application to increase ammonium concentration provides favourable conditions for AOB survival (44, 45), so the higher abundance of AOB at the jointing stage (z32) may be related to N fertilizer application. The N forms and concentration in the soil changed as the growth stage advanced, and the abundance of AOB decreased. The specific contribution of AOA and AOB to soil N availability requires further studies on community composition and activity of ammonia oxidizers. Meanwhile, nitrate stimulates root elongation and lateral root growth (19). The change of plant spatial arrangement might trigger the root recognition response to the competitive environment (46), and thus improve the N use efficiency of plant (Figure 3). It provided abundant N for leaf expansion during the critical period of wheat growth, which in turn improved the leaf area index, SWL and photosynthetic capacity. Then the transport of N to grains during filling stage was promoted, which laid a foundation for the increase of RUE and yield.
In that respect, the improvement of soil nitrogen availability and N use efficiency may be the important factors for optimizing the canopy structure and photosynthetic capacity. Compared with the typical farmer’s cropping system, R1 has a positive effect on soil N availability and N uptake of wheat, ultimately affecting plant growth and yield (Figure 4). As the result, the number of ears per unit area was the highest in the R1 treatment. Kernels and 1000-kernel weight were also significantly increased, but there was no significant difference between each processing harvest index. Contrary to expectations, there was no significant increase in the number of ears for R1 in 2015–2016, which could be attributed to climate differences between the two years (Figure S1). The results in other cultivars have also demonstrated the wide applicability of the wide-narrow row spacing mode.
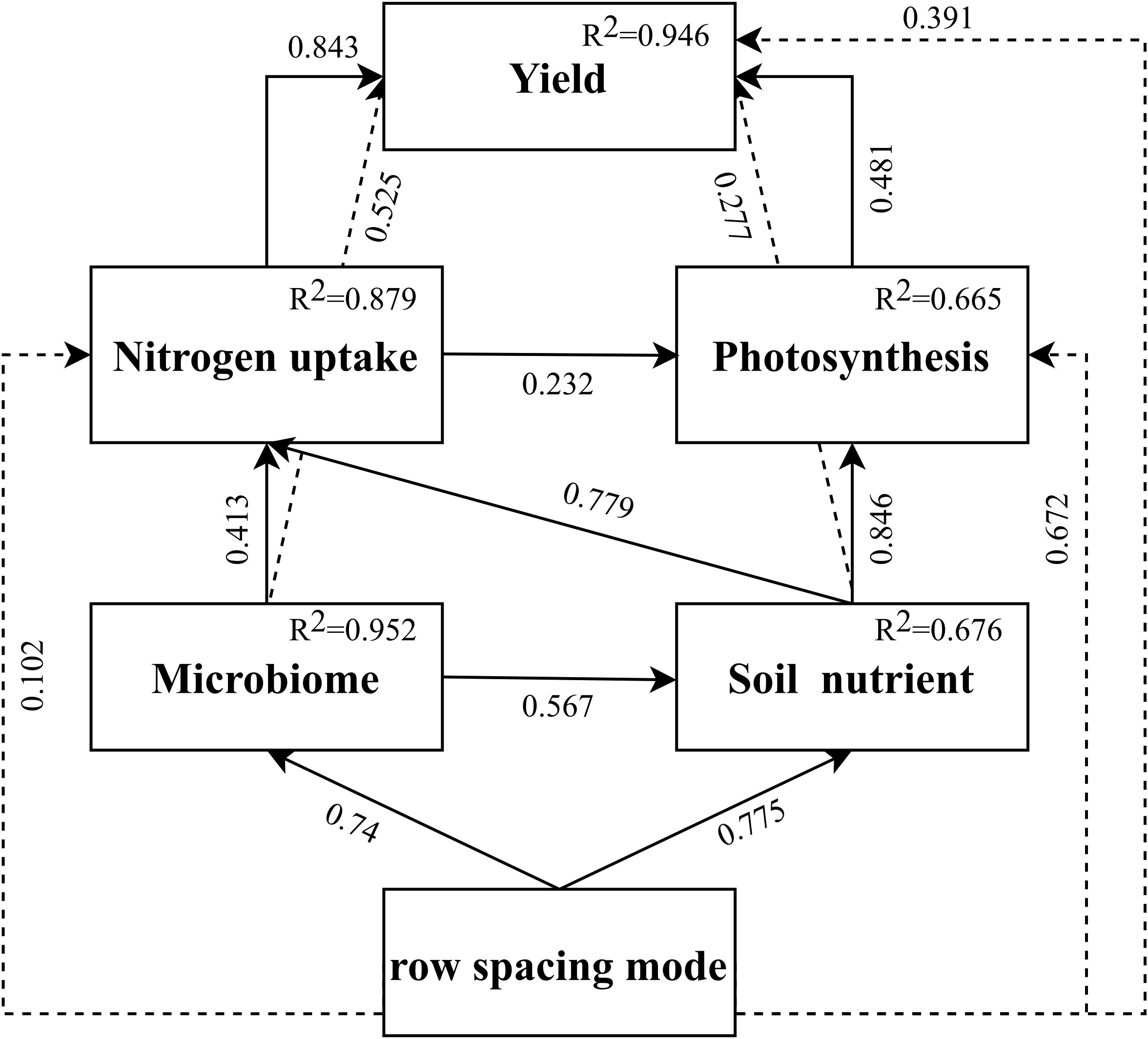
Figure 4 The path analysis correlation coefficient among of row spacing mode, microbiome, soil nutrient, nitrogen uptake, photosynthesis and yield. The solid and dotted lines are direct and indirect effects. Numbers next the arrow line indicate correlation. The ratio of interpretation variance (R2) appears in the upper right of each indicator in the model.
After the wheat was harvested, we sowed maize in the wide rows of R1, effectively avoiding the effects of residual wheat straw and stubble and reducing the difficulty of mechanized planting (Figure S1). In this treatment, the maize row spacing was 60 cm, which is the commonly used row spacing in the North China plain. Therefore, this system is easy to promote and implement, encouraging the mechanization transition from simple production to responsible production and environmental protection. In addition, a system like R1 greatly increases the number of emergences at the same density and with no tillage, providing a foundation for high maize yield (Table S3). In addition, the increase in maize emergence allowed R1 treatment to use a greater amount of straw for returning to field than CK in the next wheat season. The products released from straw decomposition are beneficial to the formation of organic matter such as soil humus. Studies have shown that an increase in the amount of straw in the corn season combined with reasonable nitrogen application can improve soil microbial activity and bacterial growth, which in turn improves soil structure and promotes crop root growth and wheat yield (47).
5 Conclusion
Wide-narrow row spacing mode increased the abundance of ammonia-oxidizing microorganisms in the soil, which facilitated the acceleration of the nitrification process and improved the soil nitrogen availability, thus increasing the nitrogen uptake from soil and nitrogen use efficiency of wheat. Moreover, the measurable improvement of leaf area and canopy structure led to, more favorable to light interception and high net photosynthetic rate of wheat and ultimately promoted dry matter accumulation. Wide-narrow row spacing mode increased wheat yields by 3.97%-12.21% compared to other treatments. Therefore, wide-narrow row spacing mode can achieve higher wheat yields without increasing input, which is more consistent with the goal of sustainable agriculture.
Data availability statement
The original contributions presented in the study are included in the article/Supplementary Material, further inquiries can be directed to the corresponding authors.
Author contributions
XM conceived the project. XW and SX helped with the experimental design. JZ, RC and ZZ participated in the field experiments and analyzed the data. JZ and XM wrote the manuscript. All authors contributed to the article and approved the submitted version.
Funding
This study was supported by the National Key Research and Development Program of China (2016YFD0300205, 2016YFD0300609), the Global Environment Fund (P144531), and the Major science and technology project of Henan Province (221100110800).
Conflict of interest
The authors declare that the research was conducted in the absence of any commercial or financial relationships that could be construed as a potential conflict of interest.
Publisher’s note
All claims expressed in this article are solely those of the authors and do not necessarily represent those of their affiliated organizations, or those of the publisher, the editors and the reviewers. Any product that may be evaluated in this article, or claim that may be made by its manufacturer, is not guaranteed or endorsed by the publisher.
Supplementary material
The Supplementary Material for this article can be found online at: https://www.frontiersin.org/articles/10.3389/fsoil.2022.981263/full#supplementary-material
References
1. Duan J, Wu Y, Zhou Y, Ren X, Shao Y, Feng W, et al. Approach to higher wheat yield in the Huang-huai plain: improving post-anthesis productivity to increase harvest index. Front Plant Sci (2018) 9:1457. doi: 10.3389/fpls.2018.01457
2. Sun X, Long Z, Song G, Chen C. Effects of climate change on cropping pattern and yield of summer maize-winter wheat in Huang-Huai-Hai plain. Scientia Agricultura Sinica (2017) 50(13):2476–87. doi: 10.3864/j.issn.05781752.2017.13.007
3. Liu T, Wang Z, Cai T. Canopy apparent photosynthetic characteristics and yield of two spike-type wheat cultivars in response to row spacing under high plant density. PloS One (2016) 11(2):e0148582. doi: 10.1371/journal.pone.0148582
4. Wu L, Ouyang Z. Effects of row spacing and seeding rate on radiation use efficiency and grain yield of wheat. Chin J Eco-Agriculture (2014) 22(1):31–6. doi: 10.3724/SP.J.1011.2014.30568
5. Wang Z, Zhao X, Wu P, Gao Y, Yang Q, Shen Y. Border row effects on light interception in wheat/maize strip intercropping systems. Field Crops Res (2017) 214:1–13. doi: 10.1016/j.fcr.2017.08.017
6. Ittersum MKV. Crop yields and global food security. will yield increase continue to feed the world? Eur Rev Agric Economics (2016) 97(1):191–2. doi: 10.1093/erae/jbv034
7. Furbank RT, Jimenez-Berni JA, George-Jaeggli B, Potgieter AB, Deery DM. Field crop phenomics: enabling breeding for radiation use efficiency and biomass in cereal crops. New Phytologist (2019) 223(4):1714–27. doi: 10.1111/nph.15817
8. Fischer RA, Ramos OHM, Monasterio IO, Sayre KD. Yield response to plant density, row spacing and raised beds in low latitude spring wheat with ample soil resources: An update. Field Crops Res (2019) 232:95–105. doi: 10.1016/j.fcr.2018.12.011
9. Hiltbrunner J, Liedgens M, Stamp P, Streit B. Effects of row spacing and liquid manure on directly drilled winter wheat in organic farming. Eur J Agronomy (2005) 22(4):441–7. doi: 10.1016/j.eja.2004.06.003
10. Salem AKM. Effect of nitrogen levels, plant spacing and time of farmyard manure application on the productivity of rice. J Appl Sci Res (2006) 2(11):980–7.
11. Marshall GC, Ohm HW. Yield responses of 16 winter wheat cultivars to row spacing and seeding rate. Agron J (1987) 79:1027–30. doi: 10.2134/agronj1987.00021962007900060015x
12. Farooq S, Hussain M, Lee D, Farooq M, Mahmood Z, Khan MB. Narrow row spacing ensures higher productivity of low tillering wheat cultivars. Int J Agric Biol (2012) 14(3):413–8.
13. Liu S, Jian S, Li X, Wang Y. Wide–narrow row planting pattern increases root lodging resistance by adjusting root architecture and root physiological activity in maize (Zea mays l.) in northeast China. Agriculture (2021) 11(6):517. doi: 10.3390/agriculture11060517
14. Wang R, Cheng T, Hu L. Effect of wide–narrow row arrangement and plant density on yield and radiation use efficiency of mechanized direct-seeded canola in central China. Field Crops Res (2015) 172:42–52. doi: 10.1016/j.fcr.2014.12.005
15. Hu H, Lu C, Wang Q, Li H, He J, Xu D, et al. Influences of wide-narrow seeding on soil properties and winter wheat yields under conservation tillage in north China plain. Int J Agric Biol Engineering (2018) 11(4):74–80. doi: 10.25165/j.ijabe.20181104.3399
16. Li Q, Chen Y, Liu M, Liu M, Zhou X, Yu S, et al. Effects of irrigation and planting patterns on radiation use efficiency and yield of winter wheat in north China. Agric Water Management (2008) 95(4):469–76. doi: 10.1016/j.agwat.2007.11.010
17. Li G, Zhang ZS, Gao HY, Liu P, Dong ST, Zhang JW, et al. Effects of nitrogen on photosynthetic characteristics of leaves from two different stay-green corn (Zea mays l.) varieties at the grain-filling stage. Can J Plant Sci (2012) 92:671–80. doi: 10.4141/cjps2012-039
18. Kong L, Xie Y, Hu L, Feng B, Li S. Remobilization of vegetative nitrogen to developing grain in wheat (Triticum aestivum l.). Field Crops Res (2016) 196:134–44. doi: 10.1016/j.fcr.2016.06.015
19. Gao J, Lei M, Yang L, Wang P, Tao H, Huang S. Reduced row spacing improved yield by optimizing root distribution in maize. Eur J Agronomy (2021) 127:126291. doi: 10.1016/j.eja.2021.126291
20. Oburgen E, Gruber B, Wamek W, Watzinger A, Stanetty C, Schindlegger Y, et al. Microbial decomposition of 13C- labeled phyto siderophores in the rhizosphere of wheat: Mineralization dynamics and key microbial groups involved. Soil Biol Biochem (2016) 98:196–207. doi: 10.1016/j.soilbio.2016.04.014
21. Xuan W, Beeckman T, Xu G. Plant nitrogen nutrition: sensing and signaling. Curr Opin Plant Biol (2017) 39:57–65. doi: 10.1016/j.pbi.2017.05.010
22. Fujii C, Nakagawa T, Onodear Y, Matsutani N, Sasada K, Takahashi R, et al. Succession and community composition of ammonia-oxidizing archaea and bacteria in bulk soil of a Japanese paddy field. Soil Sci Plant Nutr (2010) 56(2):212–9. doi: 10.1111/j.1747-0765.2010.00449.x
23. Chen X-P, Zhu Y-G, Xia Y, Shen J-P, He J-Z. Ammonia-oxidizing archaea: important players in paddy rhizosphere soil? Environ Microbiol (2008) 10:1978–87. doi: 10.1111/j.1462-2920.2008.01613.x
24. Ai C, Liang G, Sun J, Wang X, He P, Zhou W. Different roles of rhizosphere effect and long-term fertilization in the activity and community structure of ammonia oxidizers in a calcareous fluvo-aquic soil. Soil Biol Biochem (2013) 57:30–42. doi: 10.1016/j.soilbio.2012.08.003
25. Chen S, Waghmode TR, Sun R, Kuramae EE, Hu C, Liu B. Root-associated microbiomes of wheat under the combined effect of plant development and nitrogen fertilization. Microbiome (2019) 7:136. doi: 10.1186/s40168-019-0750-2
26. Rajjcan I, Tollenaar M. Source: sink ratio and leaf senescence in maize: I. dry matter accumulation and partitioning during grain filling. Field Crops Res (1999) 60:245–53. doi: 10.1016/S0378-4290(98)00142-7
27. Piao L, Qi H, Li C, Zhao M. Optimized tillage practices and row spacing to improve grain yield and matter transport efficiency in intensive spring maize. Field Crops Res (2016) 198:258–68. doi: 10.1016/j.fcr.2016.08.012
28. Dermody O, Long SP, Mcconnaughay K, Delucia EH. How do elevated CO2 and O3 affect the interception and utilization of radiation by a soybean canopy? Global Change Biol (2008) 14:556–64. doi: 10.1111/j.1365-2486.2007.01502.x
29. Guo JB, Zhang J, Xiong SP, Zhang ZY, Wei QQ, Zhang W, et al. Hyperspectral assessment of leaf nitrogen accumulation for winter wheat using different regression modeling. Precis Agriculture (2021) 22:1634–58. doi: 10.1007/s11119-021-09804-z
30. Rotthauwe J, Witzel K, Liesack W. The ammonia monooxygenase structural gene amoA as a functional marker: molecular fine-scale analysis of natural ammonia-oxidizing populations. Appl Environ Microbiol (1997) 63(12):4704–12. doi: 10.1128/aem.63.12.4704-4712.1997
31. Pester M, Schleper C, Wagner M. The thaumarchaeota: an emerging view of their phylogeny and ecophysiology. Curr Opin Microbiol (2011) 14(3):300–6. doi: 10.1016/j.mib.2011.04.007
32. Eberbach P, Pala M. Crop row spacing and its influence on the partitioning of evapotranspiration by winter-grown wheat in northern Syria. Plant Soil (2005) 268(1):195–208. doi: 10.1007/s11104-004-0271-y
33. Liu T, Song F, Liu S, Zhu X. Light interception and radiation use efficiency response to narrow-wide row planting patterns in maize. Aust J Crop Sci (2012) 6(3):506–13. doi: 10.1016/S2095-3119(17)61715-5
34. Verhagen AMW, Wilson JH, Britten EJ. Plant production in relation to foliage illumination. Ann Botany (1963) 27(108):627–40. doi: 10.1093/oxfordjournals.aob.a083875
35. Freschet GT, Violle C, Bourget MY, Scherer-Lorenzen M, Fort F. Allocation, morphology, physiology, architecture: the multiple facets of plant above- and below-ground responses to resource stress. New Phyto (2018) 219:1338–52. doi: 10.1111/nph.15225
36. Aerts R, Chapin FS. The mineral nutrition of wild plants revisited: A re-evaluation of processes and patterns. Adv Ecol Res (2000) 30:1–67. doi: 10.1016/S0065-2504(08)60016-1
37. Freschet GT, Swart EM, Cornelissen JH. Integrated plant phenotypic responses to contrasting above- and below-ground resources: key roles of specific leaf area and root mass fraction. New Phyto (2015) 206(4):1247–60. doi: 10.1111/nph.13352
38. Kleemann S, Gill G. Influence of row spacing on water use and yield of rain-fed wheat (Triticum aestivum l.) in a no-till system with stubble retention. Crop Pasture Sci (2010) 61(11):892–8. doi: 10.1071/CP10124
39. Long SP, Zhu XG, Naidu SL, Ort DR. Can improvement in photosynthesis increase crop yields? Plant Cell Environ (2006) 29(3):315–30. doi: 10.1111/j.1365-3040.2005.01493.x
40. Song Y. Succession of abundance and community structure of ammonia-oxidizing archaea in paddy soil during flooding. J Agro-Environ Sci (2014) 33(5):999–1006. doi: 10.1007/s00374-011-0542-8
41. Chen J, Li J, Yan J, Li H, Zhou X. Abundance and community composition of ammonia-oxidizing bacteria and archaea under different regeneration scenarios in Chinese loess plateau. Soil Sci (2014) 179(8):369–75. doi: 10.1097/SS.0000000000000080
42. Polyanskaya LM, Sukhanova NI, Chakmazyan KV, Zvyyagintsev DG. Changes in the structure of soil microbial biomass under fallow. Eurasian Soil Sci (2012) 45(7):710–6. doi: 10.1134/S1064229312030088
43. González-Quiñones V, Stockdale EA, Banning NC, Hoyle FC, Sawada Y, Wherrett A, et al. Soil microbial biomass-interpretation and consideration for soil monitoring. Soil Res (2011) 49:287–304. doi: 10.1071/SR10203
44. Zou W, Lang M, Zhang L, Liu B, Chen X. Ammonia-oxidizing bacteria rather than ammonia-oxidizing archaea dominate nitrification in a nitrogen-fertilized calcareous soil. Sci Total Environ (2022) 811:151402. doi: 10.1016/j.scitotenv.2021.151402
45. Zhang G, Huang J, Jia M, Liu F, Yang Y, Wang Z, et al. Ammonia-oxidizing bacteria and archaea: response to simulated climate warming and nitrogen supplementation. Soil Sci Am J (2019) 83(6):1683–95. doi: 10.2136/sssaj2019.05.0134
46. Crepy MA, Casal JJ. Photoreceptor-mediated kin recognition in plants. New Phyto (2015) 205(1):329–38. doi: 10.1111/nph.13040
Keywords: row spacing mode, soil nitrogen availability, canopy structure, radiation use efficiency, nitrogen use efficiency
Citation: Zhang J, Cao R, Zhang Z, Wang X, Ma X and Xiong S (2022) Effects of row spacing on soil nitrogen availability, wheat morpho-physiological traits and radiation use efficiency. Front. Soil Sci. 2:981263. doi: 10.3389/fsoil.2022.981263
Received: 29 June 2022; Accepted: 25 August 2022;
Published: 13 September 2022.
Edited by:
Xin Zhao, China Agricultural University, ChinaReviewed by:
Tangyuan Ning, Shandong Agricultural University, ChinaJian-Fu Xue, Shanxi Agricultural University, China
Copyright © 2022 Zhang, Cao, Zhang, Wang, Ma and Xiong. This is an open-access article distributed under the terms of the Creative Commons Attribution License (CC BY). The use, distribution or reproduction in other forums is permitted, provided the original author(s) and the copyright owner(s) are credited and that the original publication in this journal is cited, in accordance with accepted academic practice. No use, distribution or reproduction is permitted which does not comply with these terms.
*Correspondence: Shuping Xiong, shupxiong@henau.edu.cn; Xinming Ma, maxinming@henau.edu.cn