Effects of recurrent summer droughts on arbuscular mycorrhizal and total fungal communities in experimental grasslands differing in plant diversity and community composition
- 1Swammerdam Institute of Life Sciences, University of Amsterdam, Amsterdam, Netherlands
- 2Department Soil Ecology, Helmholtz Centre for Environmental Research – UFZ, Halle (Saale), Germany
- 3German Centre for Integrative Biodiversity Research (iDiv), Halle-Jena-Leipzig, Leipzig, Germany
- 4Institute of Biology, Leipzig University, Leipzig, Germany
- 5Department of Geography, Remote Sensing Laboratories, University of Zürich, Zürich, Switzerland
- 6Fredericton Research and Development Centre, Agriculture and Agri-Food Canada, Fredericton, NB, Canada
Introduction: Biodiversity loss and climate change have been determined as major global drivers affecting ecosystems and their functioning. In this context, drought was shown to have negative effects on ecosystems by disrupting ecological processes, which could be buffered in more biodiverse systems. Many studies, however, focus on effects on aboveground communities of single drought events, while dynamics of soil-borne communities are still widely unclear, despite their important roles in ecosystem functioning.
Methods: To elucidate the effect of recurrent summer drought periods on fungal communities in a long-term grassland biodiversity experiment, roof shelters were installed on grassland plots ranging in plant species richness from 1 to 16 species and plant functional group richness (1-4 groups) and composition. After 9 years of summer droughts, bulk soil was sampled and used for Illumina sequencing of the ITS2 and SSU genes to characterize the total fungal and arbuscular mycorrhizal fungal (AMF) communities, respectively.
Results: We found shifts of AMF and total fungi community structures caused by recurrent drought and plant species richness, but no buffering of drought effects by plant diversity. Alpha-diversity (VT or ASV richness) of both AMF and total fungi increased with plant species richness but was not significantly affected by drought. Even though drought overall had minimal long-lasting effects, we found Diversispora and Paraglomus among the AMF and Penicillium among total fungal communities to be more abundant after the drought treatment. AMF communities were affected by the presence of individual plant functional groups, reacting stronger to presence of legumes under drought, while total fungal interaction with plant communities were similar under drought as control. AMF α-diversity differed between plant functional groups in control conditions but was independent of plant community composition under drought. In contrast, total fungi α-diversity was increased by presence of herbs and legumes only under drought.
Discussion: From our results, we conclude that recurring moderate summer droughts do not strongly affect soil fungal communities. All shifts can be explained by indirect effects through the plant community and its top-down effect on soils altered by drought. Further, AMF are not less affected than total fungal communities, but rather respond differently by interacting more strongly with legumes in response to drought. Consequently, not plant species richness, but plant functional composition, dominates in shaping fungal communities under recurrent droughts.
1 Introduction
Plant diversity has been linked to increased productivity of ecosystems (1), but also to their stability in the face of climate extremes (2–5). For instance, in grasslands that are hot spots of biodiversity, rich in specialized plant, animal and microbe species, drought causes loss of both productivity and of biodiversity (6). Drought manipulation has been widely used to test the diversity-dependent response of grassland ecosystems’ productivity and functioning (3, 4). While drought sensitivity, subsequent loss in productivity, and recovery are dependent on precipitation patterns (7), more diverse ecosystems may be more resistant to (3, 8) and recover faster after drought (9). The diversity-stability relationship has been connected to belowground processes through quality and quantity of root exudates, litter, and root traits (10, 11). Consequently, plant diversity has been shown to positively affect soil microorganisms (12, 13) even under unfavourable conditions (14, 15).
The establishment of symbioses between plants and arbuscular mycorrhizal fungi (AMF) is considered to be one of the underlying mechanisms of the increased productivity and stability in diverse plant communities (16, 17). Mutualistic fungal communities in grasslands are dominated by arbuscular mycorrhiza, as most plant species depend on AMF for their health and growth (18, 19). The effect of drought on AMF is of special interest, because of their role in plant productivity: AMF have been shown to enhance plants’ resistance to drought across a wide range of plant species and environmental conditions (20–24). Despite their positive effects on plants, AMF themselves might be affected by global change factors, e.g. altered mycelial growth in warmed drier soils (25). In general, low soil moisture reduces microbial respiration and biomass (26). On the other hand, drought triggers an enhanced root exudation and thereby indirectly an increase of soil microbial respiration (27). Overall, low soil moisture was found to be an even stronger factor controlling soil carbon dynamics than elevated CO2 and temperature (28, 29), although nutrient fluxes in fungi seem to be less affected by drought than in bacteria (30).
Interactions between AMF and plants are also affected by drought: Temperature-related alterations in spore germination and hyphal growth of AMF (25, 31) might reduce root colonisation and consequently negatively impact plant communities in the future, as root colonising dynamics change (32, 33). Even though it has been shown that root exudation is maintained during drought (34), plant-fungal interactions may only recover slowly afterwards (35). For long-term effects of drought events, de Vries, Griffiths et al. (36) hypothesize that despite recovery of plant productivity, plant-microbe interactions remain altered through altered root exudation profiles, which also modify microbial assembly and trigger microbial activity. In grasslands, AMF identity and species richness affect plant productivity with a positive correlation between AMF and plant species richness (37, 38). Vice versa, plant identity and species richness shape AMF communities. AMF species richness can increase with plant species richness (38) and AMF community composition is shaped by plant responses to the environment (39). The community composition of AMF further shifts throughout a growing season in response to plant growth, fluctuations of root exudation, and other environmental factors (40, 41). Such short-term AMF diversity studies have shown that AMF communities and plants contribute equally to shifts in their interactions and switching between their multiple potential partners (42, 43). Hence, the soil-fungi-plant system should be looked at as a mutually shifting entity, responding to environmental and seasonal changes (44), including drought, to understand mechanisms of resistance and resilience of such systems. Most studies on effects of drought in grasslands, however, focus on aboveground effects or only consider general belowground proxies such as microbial biomass or microbial respiration (4, 6, 15, 45–47). More specifically, it is debated whether the diversity-stability relationship forwards a buffering effect towards belowground communities against drought. Findings vary from no buffering to increased plant diversity enhancing below-ground productivity under drought (48–50).
Ecosystem functioning relationships do not solely rely on plant species richness, but also plant functional identities are drivers of diversity effects (51, 52). Considering functional traits of plants may help with a more mechanistic understanding of BEF relationships. Distinct traits relate to functions e.g., rooting depth to acquisition of soil water and nutrients (53), and these traits are therefore determinants of diversity-productivity relationships creating a trait-axis from legumes to grasses (53). This translates to belowground communities where plant species identity and especially root traits determine the AMF community composition (54). For example, AMF respond to root diameter and consequently interact more with legumes than grasses on the root-trait axis (55), leading to legumes-associated AMF communities being distinct from those associated with grasses and herbs (56).
Our study focuses on the long-term effects of drought simulated by reducing summer precipitation to generate recurrent drought periods repeatedly for 9 years. This precipitation modification was nested in the Jena Experiment, which manipulates both plant species richness and functional group composition in a grassland ecosystem. Our investigations put a special emphasis on AMF and their interaction with plant diversity. Earlier research within the same setting showed that in the short-term, the positive relations between plant species richness and plant aboveground productivity (46) as well as soil microbial biomass (50) are maintained under drought conditions. However, this maintained higher productivity of species-rich plant communities relies on increased spring productivity to recover from growth reduction during the summer drought of the preceding year (8). As introduced above, long-term effects of prolonged drought may differ in above-ground vs. below-ground systems. While plant diversity promoted stability in aboveground productivity, it could not directly buffer summer drought effects on belowground processes such as reduced litter loss rate and microbial basal respiration and biomass, which occurred independently of plant diversity (50). We therefore aimed to expand previous findings by uncovering lasting effects of drought through analysing fungal and plant interactions one year after the last drought treatment.
Combining the potential effects of recurrent summer drought and plant diversity and functional composition on AMF and total fungal communities together, we tested the following hypotheses: i) different AMF are recruited by plants under drought, reflected also by a shift in AMF community composition and increase of AMF alpha-diversity in soil under repeated drought; ii) importantly, because plant species richness has been shown to be an important factor for ecosystem resistance and resilience under drought, more diverse plant communities should better buffer effects of drought on belowground community compositions; alternatively, the presence or richness of plant functional groups alters drought effects; iii) AMF community responses to drought are weaker than those of other fungal taxa, and this may be due to stronger effects of specific plant species shaping AMF communities, which can be due to interactions in water or nutrient uptake.
2 Materials and methods
2.1 Experimental setup
This study was performed at the Jena Experiment field site (51) on an Eutric Fluvisol (FAO-Unesco 1997) developed from up to 2 m thick loamy fluvial sediments alongside the Saale river in Jena, Thuringia, Germany (50°55’N, 11°35’E, 130 a.s.l.). The mean annual precipitation is 610 mm and mean annual temperature 9.9°C (57). The site was used as arable field for 40 years, before a conversion to grassland in 1960s, and was highly fertilized prior to the experiment setup (sowing of grassland communities) in 2002. The field site is split into 80 large plots of 20 x 20 m containing different plant community compositions varying in both species richness (1, 2, 4, 8, 16, and 60 plant species) and number of functional groups (1-4 groups: grasses, small herbs, tall herbs - summarized here as ‘herbs’ - and legumes) as described in Roscher, Schumacher et al. (51). The plots are distributed among four blocks to account for spatial edaphic variations (including soil texture and water holding capacity) which is related to the distance of the plots to the river. Each block contains the same number of plots at each plant species richness level, covering the range of functional groups (51). Overall, there are four replicates per species richness x functional group richness combination, with plant species in different communities chosen randomly out of a pool of 60 mesophilic grassland species. The sown grassland communities are maintained by three weeding campaigns per year in March, June, and October. There are two mowing events per year, in June and September.
The drought experiment was established in 2008 as a sub-experiment nested in the pre-existing plots. Prior to the second mowing in September, transparent rainout shelters (wood and PVC sheets, 2.6 x 3 m) were installed for 6 weeks every year to induce a prolonged summer drought period (50) over a span of 9 years (2008–2016). Of the two sheltered subplots – hereafter referred to as treatments, one received no water after installation (‘drought’) and one received collected rain water after precipitation events (‘control’), thereby controlling for non-drought roofing effects such as altered light and temperature (58). The roof shelters excluded 39.5 mm precipitation in 2009 and reduced summer precipitation by an average of 42% in 2008-2014 (8, 46). In July 2013, the field site was completely flooded during a natural flood event (occurring once in 200 years), and the drought treatments were continued thereafter.
2.2 Sampling
To gain information on the lasting effect of 9-year history of drought vs non-drought on fungal communities, bulk soil samples were taken one year after the last summer drought simulation in August 2017 from all plots except the 60-species mixes. Around 50 g of bulk soil were taken by pooling 3-5 soil cores of 0-15 cm depths per subplot. The soil was sieved at 2 mm for homogenization and to remove any plant material. Soil samples were stored at -80°C until processing for next generation sequencing. As bulk soil was sampled one year after the last summer drought treatment, two 1 g subsamples were used for measuring gravimetrically the soil water content to ensure extant drought effect. For this, 2x 1g soil were dried at 104°C with Mettler Toledo and Kern DBS moisture analyzers.
To determine drought legacy effects on plant communities, plant aboveground biomass was determined by harvesting a 0.1 m2 subplot at the end of August/begin of September 2017 by cutting plants with scissors at 3 cm above soil surface. The material was then sorted into target species, non-target weed, unidentifiable, and dead plant material and dried at 70°C for at least 48 h and weighed at weight constancy. This same procedure was also carried out throughout the 9 consecutive years of drought treatment, sampling aboveground biomass every year in May and August. Changes in aboveground plant biomass over the years were tested with ANOVA model:
2.3 Library preparation and sequencing
Genomic DNA was extracted from bulk soil samples using DNeasy PowerSoil Kit (Qiagen). DNA quantity and purity was assessed using a NanoDrop 8000 spectrophotometer (Thermo Fisher Scientific). The SSU rRNA region of AMF was amplified in triplicates with a nested PCR using primer pairs Glomer1536 and WT0 [AATARTTGCAATGCTCTATCCCA/CGAGDWTCATTCAAATTTCTGCCC, Wubet, Weiß et al. (59), Morgan and Egerton-Warburton (60)] for the first and NS31 and AML2 [TTGGAGGGCAAGTCTGGTGCC/GAACCCAAACACTTTGGTTTCC, Simon, Lalonde et al. (61), Lee, Lee et al. (62) for the second PCR step following the protocol of Wahdan, Reitz et al. (63). To amplify the fungal ITS2 region, DNA was diluted to concentrations of 20 ng/µl and amplified in triplicates with primer pair ITS4 [TCCTCCGCTTATTGATATGC, Ihrmark, Bodeker et al. (64)] and fITS7 [GTGARTCATCGAATCTTTG, Gardes and Bruns et al. (65)] as described in Prada-Salcedo, Goldmann et al. (66).
Illumina sequencing libraries were prepared by purifying amplicons, barcoding and quality checking as described in Wahdan, Reitz et al. (63) and Prada-Salcedo, Goldmann et al. (66), respectively. Paired-end sequencing of 2 x 300 bp was performed at the Illumina MiSeq platform at the Department of Soil Ecology at Helmholtz Centre for Environmental Research – UFZ, Halle (Saale), Germany.
2.4 Bioinformatics
Raw SSU and ITS2 sequence data were filtered, trimmed, aggregated to amplicon sequence variants (ASV), and annotated with help of the dadasnake pipeline (67), which is based on DADA2 (68) using amongst other softwares cutadapt (69) and mothur (70). Within the dadasnake pipeline, AMF sequences were classified against SILVA 138 (71) and ITS2 sequences were classified against the eukaryote UNITE (version 8.2) database (72) using mothur’s implementation of the naïve Bayes taxonomic classifier (70). The final ASV tables were filtered for target taxa Glomeromycota and Fungi, respectively. This resulted in a total of 2,422,141 reads with on average 16,040 (SD +- 6,642) per sample of AMF. And for total fungi in 7,000,330 reads in total with an average of 46,360 (SD +- 6,202) per sample.
The taxonomic annotation of the AMF ASVs was not used further than to filter for Glomeromycota. Instead, AMF ASVs were aligned to virtual taxa (VTX) via MaarjAM’s BLASTn (73, 74). The read counts of ASVs assigned to the same VTX were summed up, condensing 4,865 ASVs to 114 VTX. To avoid uneven taxonomic resolution but include ASVs that are not yet represented in MaarjAM, ASVs that could not be assigned to a virtual taxon were extracted to construct a maximum likelihood phylogenetic tree based on a general time-reversible, discrete gamma (GTR+G) model using MAFFT (75) and raxML (76). Custom virtual taxa (VTC) with cophenetic distances below 0.03 were assigned. Their read counts were merged, resulting in a total of 211 virtual taxa (VTX plus VTC).
Obtained VT/ASV abundance and taxonomy results were further analysed in R version 4.0.4 (77). Abundances were sum-normalised and VTs/ASVs that were only present in up to 3 samples and with only one read were removed.
2.5 Statistical analysis of fungal communities
Data was rarefied to the lowest number of reads per sample (7,350 for AMF/27,000 for ITS) to calculate observed VT/ASV richness with phyloseq v 1.34.0 (78). Relative abundances were generated with transform_sample_counts calculating sample-wise fractional abundances in phyloseq. Jensen-Shannon divergence (JSD) which is effective at capturing compositional changes and commonly used in microbial ecology (79, 80), was calculated with vegan v 2.5-7 (81) and visualized by NMDS. Environmental factors were fitted onto the ordination with envfit and factors with significant correlations plotted as vectors onto the ordinations. Vegan was also used for PERMANOVA models (adonis2) and analysis of multivariate group dispersion (betadisper). Graphics were created with ggplot2 v 3.3.3 (82).
We used multiple models to test for different interactions of fungal communities with their environment. Multivariate effects of experimental factors on JSD matrices were assessed with the following three models:
1. To analyse whether the experimental factors drought and plant diversity have an interactive effect on fungal communities (stratified by plot):
2. To test whether natural variation of soil water content across the field site adds to the drought effects (stratified by plot):
and 3. to gain a better understanding of the differences in plant-fungal-interactions between drought and control conditions, a model of effects of plant community composition within the drought/control treatment plots was tested for each treatment separately (stratified by block):
Similar models were used for ANOVA for α-diversity, soil moisture and plant biomass, with the exception of plot/block being added as random terms.
We calculated matrix dissimilarities of Drought and Roofed Control VT/ASV abundances from similarity matrices (1 – JSD matrix) based on the modified RV coefficient using package iTOP (83, 84). We analysed differential abundances of ASV/VT in drought vs control subplots with the function ANCOM (85).
3 Results
3.1 Long-term effects of the drought treatment on soil moisture and plant biomass
To test whether the impact of the repeated summer drought treatment was extant in the drought manipulated plots, we analysed soil water content of the bulk soil samples used for DNA extractions. Soil water content was significantly lower (t = -3.16, p< 0.05) in drought-treatment plots than in roofed controls. Soil water content was affected by the repeated drought treatments, but also by the plot position on the field site and the thereby existing variation in soil properties (Figure S1). Separately analyzing drought from control plots, we found significant effects of plant species richness and block on soil water content in both drought and control plots (Table S2). The effect size of these diversity and spatial gradients was reduced under drought.
Aboveground biomass of target plant species was determined for 2008-2016 every year in May and August. Tested against the sampling date, drought treatment and plant diversity we found plant biomass differed with sampling date (F = 17.61, p< 0.001) and plant diversity (F = 373.94, p < 0.001). However, testing biomass data of May and August separately erased the significance of the sampling date, while plant diversity effects remained, showing that the sampling date caused seasonal fluctuations instead of changes over the years. In May, plant biomass over the years was additionally affected by the interaction of drought treatment and plant diversity (F = 4.23, p = 0.04). Plant biomass significantly increased from August 2016 (last drought treatment) to August 2017 (F = 155.95, p< 0.001) influenced by plant diversity (F = 40.79, p< 0.001). This increase in biomass after the last drought treatment was consistent for all plant functional groups: legumes (F = 8.59, p > 0.01), grasses (F = 10.27, p< 0.01) and herbs (F = 10.57, p< 0.01), however, biomass of the plant functional groups individually was not affected by the drought treatment or plant diversity (Figure S5D).
At the time of soil sampling in August 2017, total plant biomass per plot was slightly lower in former drought-exposed than in control plots, but not significantly (t = -0.64, p = 0.52). The ANOVA model for total plant biomass showed no effect of the drought treatment (F = 0.41, p = 0.53), but instead of the specific soil water content per plot (F = 20.72, p< 0.001; Table S2). Considering plant community composition as a factor for plant biomass, we found plant species richness to positively affect total plant biomass in both drought and control (F = 8.19, p< 0.01), with the effect size increasing under drought (F = 19.41, p< 0.001). Along the plant diversity gradient, drought did not significantly change plant biomass (neither in species-poor nor species-rich communities). The absence of legumes significantly lowered plant biomass, overall (t = -4.93, p< 0.001), and the effect size of legumes on total plant biomass increased under drought (drought: F = 6.12, p = 0.02, control; F = 5.98, p = 0.02). The biomass of legumes also slightly increased under drought, as opposed to grasses and herbs that declined in biomass. The differences in plant biomass were however not significant (Table S1).
3.2 Community compositions
To test whether the drought and plant species and functional richness treatments have lasting effects on the composition of total fungal and AMF communities in the grassland plots, we calculated distance matrices based on Jensen-Shannon divergence and conducted PERMANOVAs. The first model for drought and plant diversity interaction showed significant effects with low effect size for both the drought treatment (R2 = 0.01, p< 0.001, Figure 1C) and plant diversity (R2 = 0.07, p< 0.001) on AMF communities and no interaction of drought and plant diversity (R2 = 0.01, p = 0.06). The variation of soil water content within the drought treatment had no significant effect on AMF communities For the AMF community composition, the overall model for effects of drought revealed evidence of a small treatment effect (R2 = 0.01, p< 0.001) and no significance of soil water content (R2 = 0.06, p = 0.09, Figure 1E). To assess correlation of abundance matrices of drought and control treatments, we calculated the correlation coefficient for similarity matrices (modified RV). AMF drought and control matrices showed a significant correlation coefficient (RV = 0.43, p< 0.001). Within the drought treatment plots, AMF community composition was affected by plant species richness and presence of grasses and legumes, which explained a total of 17% of variation in the beta-diversity (Figure 1G). Presence of herbs and number of functional groups present were not significant determining factors of AMF communities under drought. In control plots however, all plant composition factors except presence of herbs played a role in AMF community structuring: plant species richness, presence of grasses and legumes plus the number of functional groups present amounted to 21% explained variation. The low effect size of all these factors is apparent in the non-metric multidimensional scaling of the JSD matrix, as neither treatment nor plant community cause strict clustering of communities (Figure 1A). A test for homogeneity of dispersion within AMF communities was not significant for treatments (F = 1.29, p = 0.25) nor for plant diversity (F = 2.27, p = 0.06) suggesting homogeneous dispersion of groups.
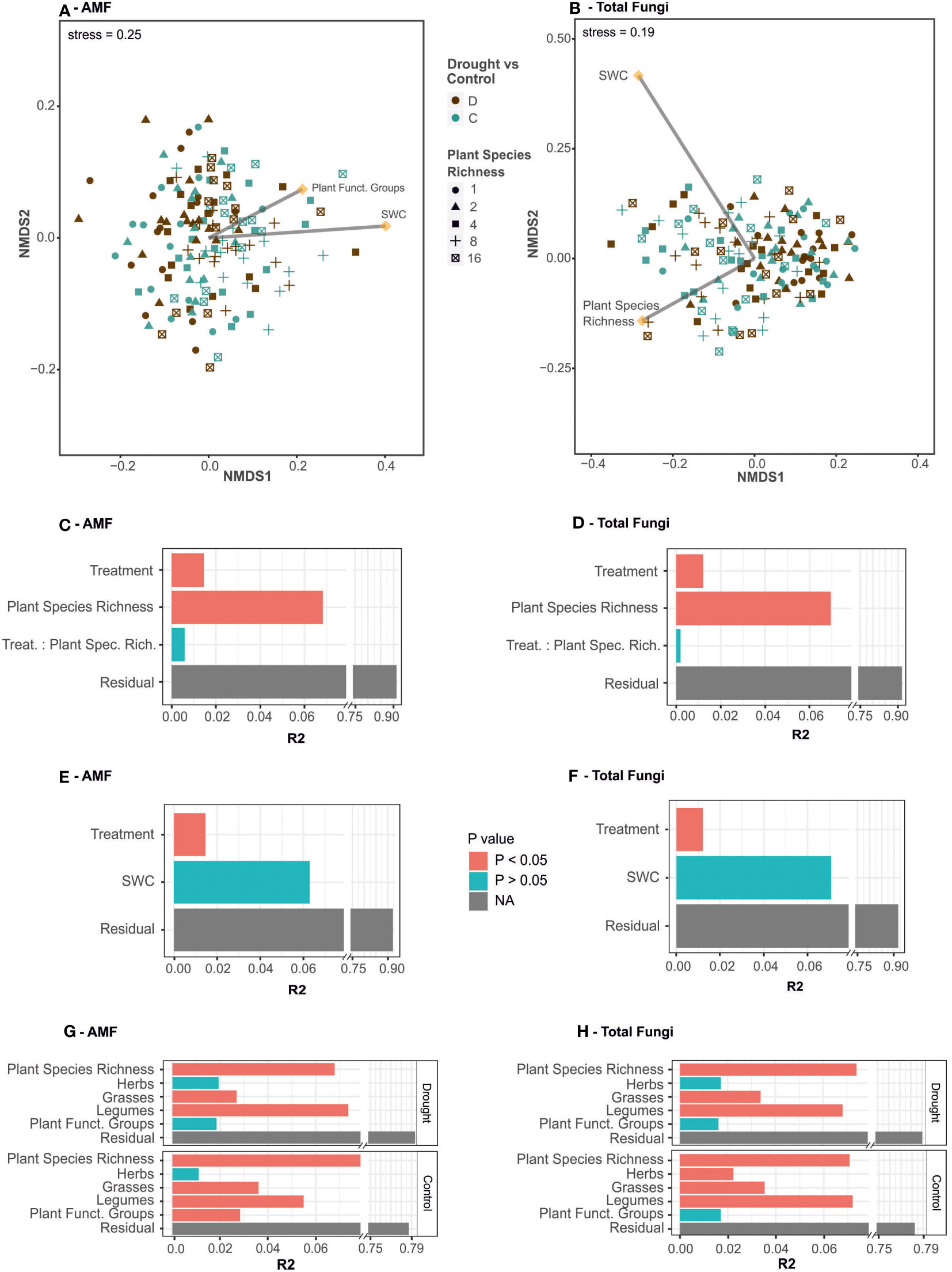
Figure 1 Effects of drought treatment, soil water content, and plant community composition on AMF and total fungal community composition. Non-metric multidimensional scaling of Jensen-Shannon dissimilarities for (A) AMF and (B) total fungal communities. Drought treatment is displayed by color (drought – brown, control – teal) and plant species richness by shape (1 – circle, 2 – triangle, 4- square, 8 – cross and 16 species – crossed square). Environmental factors fitted with envfit are indicated with arrows. Distance matrices were tested with PERMANOVA against effects of drought treatment and plant sown species richness and their interaction (C, D), and effects of drought treatment and SWC (E, F) and plant community composition (G, H). PERMANOVA results are displayed as R2 value and p value (red – significant, blue – non-significant).
For the beta-diversity of the total fungal communities (Figure 1B), the first model on drought and plant diversity interactions revealed significant effects with low effects size for the drought treatment (R2 = 0.01, p< 0.001, Figure 1D) and plant diversity (R2 = 0.07, p< 0.001), however these two factors did not interact (R2 = 0.00, p = 0.63). The variation in soil water content tested with PERMANOVA model 2 does not add additional variation to total fungal communities (R2 = 0.07, p = 0.20, Figure 1F). The coefficient of correlation between drought and control similarity matrices was higher than in the case of AMF with RV = 0.74 (p< 0.001). Within the drought exposed plots, plant species richness, presence of grasses and legumes, but not herbs, shaped total fungal communities with a total explanatory value of 17.6%. Total fungal communities under drought were not affected by the number of functional groups present (R2 = 0.07, p = 0.11). The influence of these plant community factors was similar in significance and effect size in control plots (Figure 1H), however in control plots herbs also determined fungal community structures (R2 = 0.02, p = 0.02). Tests for homogeneity of dispersion within ITS communities were not significant for the drought treatment (F = 0.22, p = 0.64), but significant for plant species richness (F = 3.34, p = 0.01). An adhoc TukeyHSD further revealed different dispersion between groups of 4 and 1 plant species (padj = 0.05) and 16 and 1 plant species (padj = 0.05), suggesting that parts of the observed differences were due to differences in dispersion rather than between the treatments.
Differential abundances were analysed with ANCOM (Figure 2). Among the AMF were only the two taxa VTX00354 (Diversispora Torrecillas 12b) and VTC00015 (Paraglomus sp) significantly differentially abundant – both higher abundant under drought with log fold changes of 1.15 (VTC00015) and 1.21 (VTX00354). Within the total fungal communities, we found three taxa that were significantly higher abundant under drought conditions: Penicillium jenensii with a log fold change of 1.85, an unidentified Penicillium sp. (log fold change = 0.33) and an unidentified Ascomycot sp. (log fold change = 1.02).
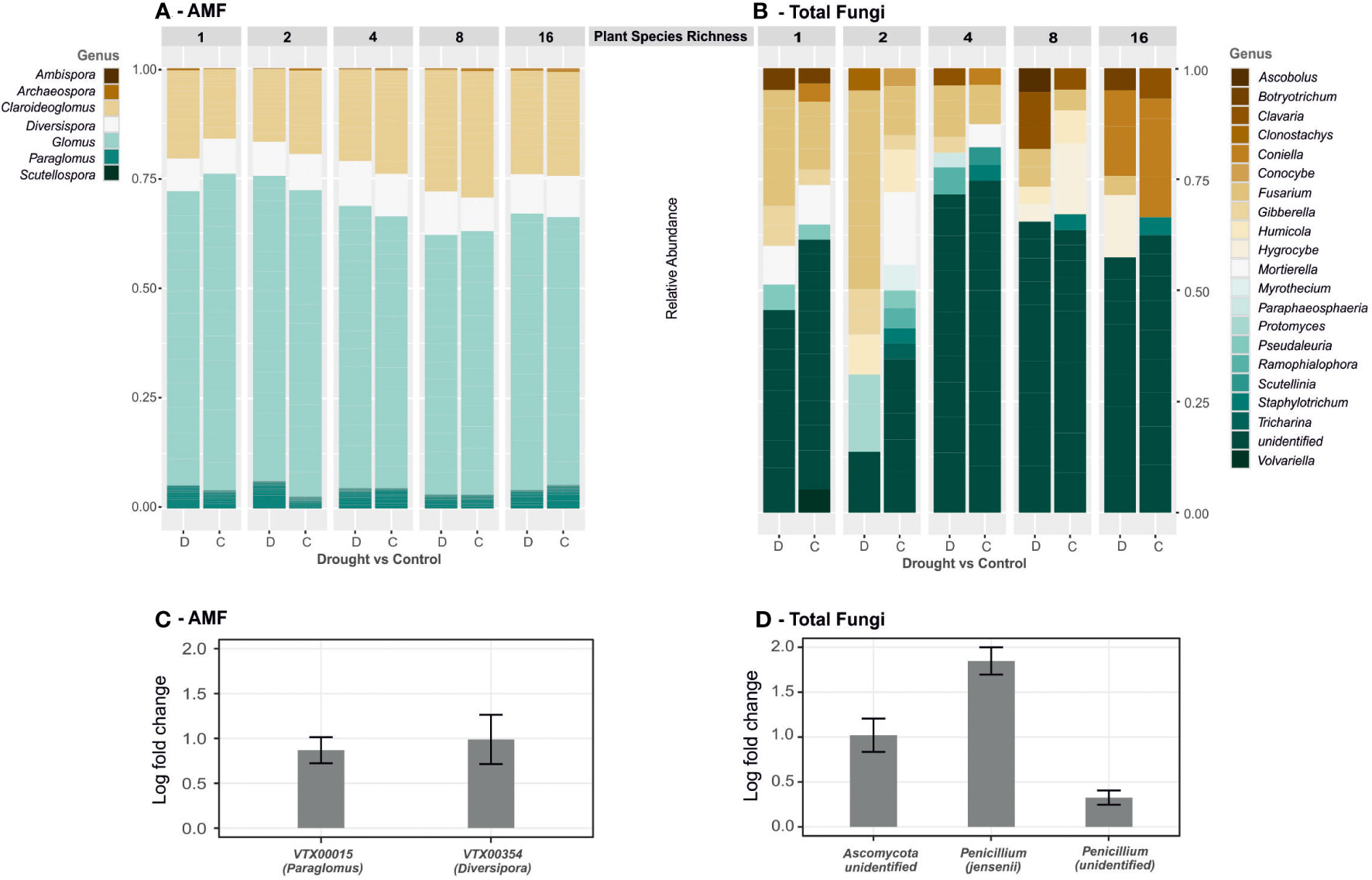
Figure 2 Genus level composition and changes of AMF and total fungal communities. Relative abundances of (A) VT of AMF and (B) ASV of total fungal communities > 5%, subdivided by the plant species richness levels (1 to 16) in drought (D) vs control (C) plots. Changes in significantly differential abundant taxa (C – AMF, D – total fungi), presented as log fold change of abundance after drought compared to control plots with standard deviations bar.
In brief, AMF and total fungal communities were shaped by plant community composition and the drought treatment caused lasting shifts in community structures. However, drought and plant community explained only small proportions of the AMF and total fungi community structures.
3.3 Drought and plant diversity effects on α-diversity of AMF and total fungal communities
Given the observed effect on community composition, we also tested whether the α-diversity of AMF and total fungi was affected by the repeated drought treatment. Paired t-tests of VT/ASV richness in drought and control subplots showed no significant differences for neither AMF (t = 0.39, p-value = 0.69) nor total fungal (t = 1.70, p = 0.09) communities. Testing α-diversities against model 1, AMF VT richness was neither significantly altered by drought (F = 0.13, p = 0.72) nor plant diversity (F = 0.12, p =0.73) nor their interaction (F = 2.23, p = 0.14). Total Fungi ASV richness was not altered by drought (F = 1.29, p = 0.26) or an interaction of drought and plant diversity (F= 0.02, p = 0.88), but showed marginal significance for a plant diversity effect (F = 4.08, p = 0.05).
When applying ANOVA with the drought treatment model to α-diversity, neither drought treatment as a factor nor specific SWC were influencing α-diversity of AMF or total fungi (Figure 3 and Table S3). Regarding the influence of plant richness and presence of functional groups on fungal α-diversity, plant species richness (R2 = 0.13, p = 0.03) and presence of herbs (R2 = 0.05, p = 0.03) increased α-diversity of AMF in control plots, but no plant functional group was significant for AMF α-diversity under drought (Table S3). In contrast, the presence of herbs (R2 = 0.06, p = 0.03) and legumes (R2 = 0.05, p = 0.04) significantly increased total fungal communities under drought, but not in control plots. The fungal α-diversities in more diverse plant communities were not affected by the drought treatment.
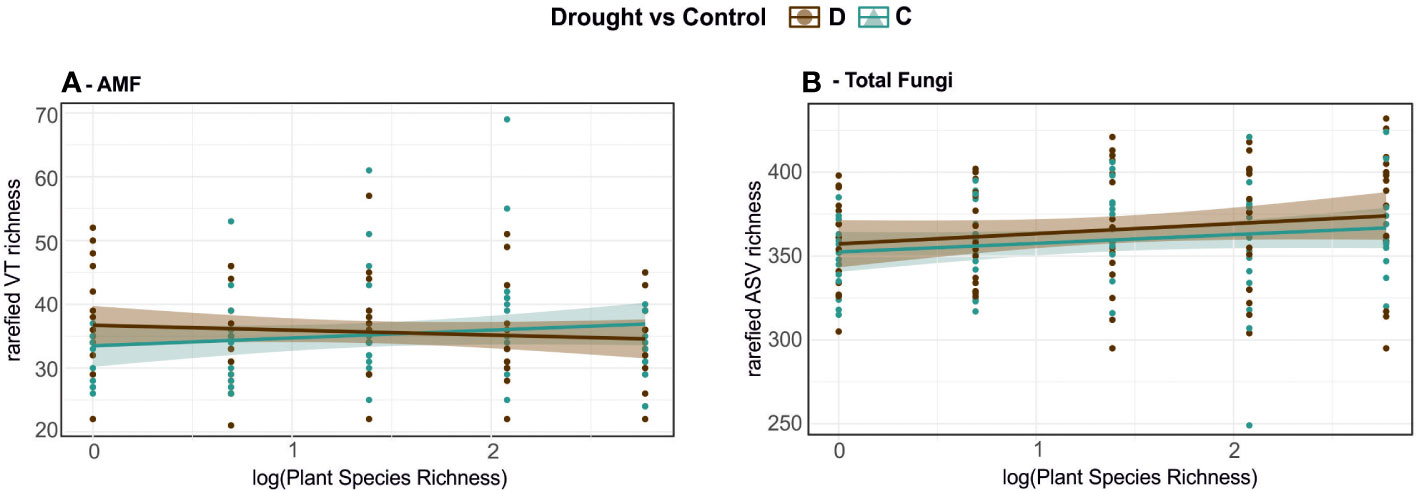
Figure 3 Alpha-diversity of AMF and total fungal communities according to plant species levels and treatments. (A) Observed AMF VT richness and (B) total fungal ASV richness after rarefaction versus log-transformed plant species richness (1 to 16). Treatments: brown – drought; teal – control subplots.
In short, drought had no major impact on fungal α-diversity. Plant functional groups played a role under drought for total fungal communities, but not for AMF. AMF α-diversity however was affected by plant communities in control plots.
4 Discussion
4.1 Repeated summer drought has lasting effects on soil water content but not on AMF α-diversity
The 9-year history of drought treatment effectively changed the soil water content, which appeared still significantly lower in plots that were exposed to repeated drought even one year after the last treatment. Previously, Vogel, Fester et al. (58) found soil moisture to not differ significantly between the treatments in the same experiment after one year, but found a response of soil moisture to the drought treatment over time, as the difference was significant in the third year of drought treatment. This is congruent with the soil water content being still divergent one year after the end of treatment, despite the field site flooding in 2013 (86). The reduction amounted to only about 2% less soil water in the drought treatment compared to control plots. SWC additionally varied across the field site, coinciding with edaphic variation of the field site (87) which was accounted for by always including the field block as error term. Overall this shows that even with a moderate, but repeated, drought treatment, changes in ecosystems become visible, and we need even more experimental approaches of realistic climate scenarios as opposed to climate extremes (88) to understand consequences on multiple ecosystems levels.
Arbuscular mycorrhiza have repeatedly been shown to enhance drought tolerance of their plant partners (89). Previous studies have shown that plants rely on mycorrhizal symbiosis under drought stress, but that AMF colonisation decreases under drought (90, 91). Soil moisture regulates AMF community assembly, with drought causing shifts in relative abundances including the absence of certain AMF, which results in a reduced α-diversity (92). Therefore, we expected to see changes in the α-diversity of AMF in response to repeated drought. Our treatment with nine years of prolonged summer droughts, however, had no significant impact on AMF α-diversity (VT richness). Neither the repeated drought treatment nor the resultant SWC had significant effects on AMF α-diversity, therefore drought in our experiment did not cause a recruitment of more or less species. Analyses of the root and rhizosphere mycorrhiza may yield stronger α-diversity effects because they represent the place of AMF-plant interaction. In this regard, we also have to take into account that the flooding of the field mid-experiment might have been a mode of transport for propagules.
Despite the α-diversity of AMF remaining similar under drought across the diversity treatments, the community composition did change. This effect was apparent regardless of the high variation of SWC along the field and across the plant diversity gradient. However, the variation explained by drought was limited, which is in line with AMF having been shown able to endure drought (36). Two AMF VT were found to have a higher relative abundance under drought. The first one was identified as Diversispora, whose mycorrhizal infection is inhibited by drought (93). But Diversispora were found to be present predominantly outside rather than inside plant roots (94, 95) exhibiting low infection rates of roots but proofing effective for plant productivity (96). These edaphophilic AMF show high biomass allocation to extraradical hyphae and increase plant nutrient uptake, but are usually characterized as sensitive to drought (97). With moderate drought as we had in our experiment, this drought sensitivity of Diversispora might however have been overruled by their interaction with plants. The second AMF genus with increased abundance under drought was Paraglomus. This genus is rhizophilic with high abundance in grass roots (97) and was previously reported as having a positive influence on drought resistance of plants (98, 99). A higher relative abundance of these two genera under drought suggests distinct responses of AMF species in relation to their host preference and their drought response. However, this is in terms of relative not quantitative abundance and might also be results of these genera being persistent while others decrease.
Summing up the effects of repeated summer drought treatment on AMF communities, we found minimal drought-induced changes on AMF α- and β-diversity, but the differential abundances of an edaphophilic and rhizophilic AMF indicate shifts in the interaction of AMF with plant partners. A reason for the relatively small drought effects may be the sampling of bulk instead of rhizosphere soil. Even though bulk soil in grasslands is still in vicinity of and highly shaped by plant roots (100), we cannot be sure that our results would be identical in the rhizo- or endosphere. Rhizosphere soil is subject to stronger changes through plant nutrients and biomass and other microbes like bacteria (101) and even in the confines of pot-experiments has been reported to respond stronger to drought stress than bulk soil (102). Nevertheless, resistance of bulk soil communities is important for potential feedback on plant communities because they represent the general pool of available interaction partners.
4.2 More diverse plant communities do not buffer effects of drought on belowground communities
Recurring drought events affect ecosystems negatively, however plant species richness proved to be an important factor of resistance and alleviation (3, 4, 8, 46, 103). As Wagg, O’Brien et al. (8) reported, the plots with high plant diversity maintained long-term productivity under drought in our experiment. Similarly, in the year after the last drought treatment, we found aboveground plant biomass to be lower after repeated drought with the reduction of productivity tending to be higher in less diverse plant communities. The influence of plant diversity as well as presence of legumes on biomass increased under drought. Noteworthy, legumes were the only plant functional group with an increase in biomass after drought exposure.
Throughout the repeated drought treatments plant biomass was overall not greatly reduced by drought. The recurring drought however induced seasonal shifts: productivity in May was more susceptible to drought and drought-diversity interaction as already shown for the first 5 years of recurring drought by Wagg, O’Brien (8). It seems, especially highly diverse plant communities had stumped productivity, however, all drought exposed plant communities could recover quickly after the last drought exposure. This is in line with findings of Chen, Vogel (104) who collected seeds from this same experiment, and exposed the plants of drought or ambient legacy once again to drought and found drought-legacy plants to recover much quicker after droughts than ambient-legacy plants.
By sampling a year after the last drought treatment, we tested for a drought legacy effect rather than a drought response. Plants have been shown to adapt to drought stress and even establish a drought memory with physiological and morphological alterations (105). Changes in precipitation patterns can reduce net primary production and affect e.g. soil respiration and nitrogen mineralization (106). Microbial processes respond quickly to changes in soil moisture, but can revert back just as quickly (105). Contingently, the shifts in microbial communities we found are a result of the plants’ drought memory.
With plants shaping belowground processes, we expected to see a positive effect of plant species richness on AMF community compositions in both control and drought plots and a buffering effect against the drought effect by higher plant diversity. Correlations of the distances between community compositions as well as the PERMANOVA models showed that total fungi and AMF were affected by plant species richness and community composition, independent of drought events. Likewise, our results suggest that a higher plant diversity does not buffer negative drought effects on soil fungi diversity. This lack of effect might reflect that the drought effect on soil moisture was weak across the plant diversity gradient. In addition, while soil under richer plant communities had a higher water content in control plots, this relationship decreased under drought, indicating that the long-term buffering effect of diverse plant communities on the soil water content was also weak. Finally, plant species richness was an important factor for β-diversity as well in controls as under drought stress. Our results are in accordance with data from the beginning of the experiment (50) showing that after one year of drought, higher plant diversity did not buffer against belowground drought effects, such as lower litter decomposition rates and microbial respiration. These findings suggest that plants in high diversity settings maintain their aboveground rather than belowground productivity under short-term adverse conditions. In our study, plant biomass was not significantly reduced under drought at any plant diversity level. However, increasing effect size of plant species richness on biomass under drought indicate stronger differences between the diversity levels. The described maintenance of plant productivity could be explained by short-term variation in biomass production, which increased in more diverse communities due to compensatory recovery from drought (8). Considering this drought-induced short-term variation, buffering effects of diversity might become more visible with seasonal variations, and Wagg, O’Brien et al. (8) found spring growth to compensate for summer drought induced productivity losses but only at high plant diversity. Our data comes from just a single time point in the driest month of the year, which may have caused us to miss temporary changes. Thus, we would expect those short-term changes to occur predominantly in the rhizo- or endosphere, while the bulk soil microbiome reacts less to droughts (102, 107).
Correlations of AMF abundance and plant species richness have been previously shown, for example, AMF abundance decreasing in the presence of certain grass species and increasing in the presence of legumes (55). Legumes have been proven to facilitate carbon allocation to fungi under moderate drought stress (108). Our results showed that the number of different functional groups is only relevant for α-diversity of AMF in drought but not control plots, while it shaped β-diversity only in controls. However, presence of grasses and legumes promote AMF communities in both control and drought with the influence of legumes even increasing under drought. The reduced effect of grasses under drought on AMF communities might stem from grasses raising the soil water content in the short term (50), an effect which did not persist to the end of the experiment according to our soil water content measurement. Kivlin, Mann et al. (54) found plant identity to be a major factor in shaping fungal communities despite environmental gradients, but diverse climate changes can interrupt plant-microbe interactions (109). Drought in particular affects water uptake and nutrient concentration, such as C:N ratio, leading to increased plant-plant competition (110). Some functional plant groups benefit more from AMF, as Chagnon, Bradley et al. (111) suggest: limitation of carbon allocation to root exudates caused by stress negatively affects plant-soil interactions, but slow growing plants invest more in AMF and receive more long-term benefits. Additionally, AMF have varying effects on productivity of different plant functional groups. Legumes seem to benefit in terms of whole plant growth while mycorrhization for herbs increases reproductive growth (112). This may explain the stronger effect of the presence of legumes on AMF communities. Legumes use more water, but have a higher water use efficiency than e.g. grasses (113) and together with their facilitated carbon allocation towards fungi under drought become a more desirable interaction partner for fungi. Microbes vice versa intensifying plant-plant competition under drought (110) could further explain the increase in legume biomass and legume-fungal interaction confirming long-term benefits of AMF symbiosis. AMF have host preferences (43) which might increase under stress conditions in relation to available transferable nutrients. Interestingly, AMF α-diversity was higher in monocultures even though AMF host-preferences would suggest higher AMF diversity with increasing plant diversity (114, 115). However, monocultures seem to benefit from AMF colonization even under drought, while plant mixtures might encounter disadvantages from demanding AMF species (116).
4.3 AMF response to drought is similar to that of other fungi
To test whether AMF as biotrophic interaction partners of plants are specific in their response to drought, we compared their response to that of other fungi. Glomeromycota made up 3.5% of abundance in control plots within the ITS2 dataset and increased to 4.1% of abundance under drought. This increase was expected: Even though fungal communities in general are rather resistant to drought (30), they have been shown to shift towards a lowered abundance in Agaricomycetes and other fungal groups and a higher abundance in Glomeromycota (117). However, the low proportion of Glomeromycota is due to the choice of the general fungal ITS2 primers, which bias amplification against the Glomeromycota (118, 119). In addition, most ITS2-reads of Glomeromycota could not be identified at the genus level. Therefore, the results discussed in the previous sections stem from AMF-specific amplifications and the rest of the community was assessed independently.
The α-diversity of total fungal communities did not change in response to drought, as for the AMF. But the explanatory value of legumes for general fungi increased under drought, indicating that the increasing biomass of legumes under drought plays a role for general fungal diversity and maybe a shift towards more AMF species. Sweeney, de Vries et al. (55) showed not only that AMF have a preference for legumes driven by root traits, but also that higher abundance of AMF in roots leads to a reduced abundance of fungal pathotrophs.
Both AMF and the total fungi β-diversities did minimally change under repeated drought, but they differed in their response to plant communities under adverse conditions. In the control plots we found AMF to be more responsive to plant species richness than the total fungi communities. The effect of legumes and herbs was similar for both fungal communities, but total fungi are additionally shaped by grasses, while for AMF communities the plant functional diversity plays a role. Total fungi communities under ambient and stress conditions were consistently affected by plant diversity and functional composition. This is in line with several studies showing that the fungal community is driven by plant functional identity and root traits with e.g. saprotrophic fungi being less abundant in grass roots (55) and plant functional diversity being a major driver for fungal richness and community structures even under stress conditions like drought (120, 121).
While we found two significantly higher abundant AMF VT under drought, the total fungi community did not detect any differential AMF at genus level. Here we found Penicillium to be more abundant under drought and no genera significantly decreasing under drought stress. One of the two Penicillium, P. jensenii, is often found in locations with higher moisture (122), but as saprophytic and phosphate-solubilizing fungi they might thrive under drought as more dead plant matter becomes available (123).
Comparing the responses of AMF and total fungi in our experiment, AMF communities displayed stronger shifts under drought than the total fungal communities. The drought-induced shifts were towards a slightly higher richness and abundance of AMF. Juxtaposing AMF communities and total fungi communities emphasizes even more the importance of plant functional identity as driver of fungal soil communities and plant-fungal interactions.
4.4 Conclusion
In summary, the effect of nine consecutive years of prolonged summer drought events on fungal communities was minimal across the plant diversity gradient. This means soil-borne fungi, including AMF, were similarly resilient to drought at lower and higher plant species richness. Fungal α-diversity was not negatively affected by drought, and β-diversity of AMF and total fungi communities only slightly shifted. The fungal communities therefore did not seem to be protected from drought by plant diversity, in contrast to plant productivity, which was more strongly affected by plant species richness under drought. All these findings suggest that plant diversity itself does not shape fungal community structures under drought stress. We also found that the influence of plant functional diversity on AMF communities decreased under drought, while it increased for general fungi. However, the effect of the presence of legumes increased under drought. While overall plant biomass productivity did not change greatly in response to repeated yet moderate drought exposure, shifts we do find in fungal communities indicate indirect drought effects caused by changes in plant-fungal interactions.
Data availability statement
The datasets presented in this study can be found in online repositories. The names of the repository/repositories and accession number(s) can be found below: https://www.ncbi.nlm.nih.gov/, PRJNA914200.
Author contributions
AV, CW and NE conceived and carried out the field experiment. CA conducted sample preparations and statistical analyses. CA, AH-B and FB wrote the manuscript with input from all authors. All authors contributed to the article and approved the submitted version.
Funding
AH-B was funded by the German Centre for Integrative Biodiversity Research (iDiv) Halle–Jena–Leipzig (DFG FZT 118, 202548816). CA was funded by the Deutsche Forschungsgemeinschaft (DFG, FOR 5000). The Jena Experiment is funded by the Deutsche Forschungsgemeinschaft (DFG, FOR 5000), with additional support by the Friedrich Schiller University Jena, the Max Planck Institute for Biogeochemistry, and the German Centre for Integrative Biodiversity Research (iDiv) Halle–Jena–Leipzig (DFG FZT 118, 202548816).
Acknowledgments
We thank the technical staff Steffen Eismann, Ute Köber, Gerlinde Kratzsch, Katja Kunze and Heike Scheffler for their work in maintaining the field site and also many student helpers for weeding of the experimental plots. Further, we thank the central coordination team Anne Ebeling and Annette Jesch, and the data management team. We further thank Alexandra Weigelt and Michael Scherer-Lorenzen who were involved in the design of the experiment and Anne Ebeling and Holger Schielzeth for assisting with statistics and the writing process. The sequencing data were processed at the High-Performance Computing (HPC) Cluster EVE, a joint effort of both the Helmholtz Centre for Environmental Research - UFZ and the German Centre for Integrative Biodiversity Research (iDiv) Halle-Jena-Leipzig, whose administrators we thank for excellent support.
Conflict of interest
The authors declare that the research was conducted in the absence of any commercial or financial relationships that could be construed as a potential conflict of interest.
Publisher’s note
All claims expressed in this article are solely those of the authors and do not necessarily represent those of their affiliated organizations, or those of the publisher, the editors and the reviewers. Any product that may be evaluated in this article, or claim that may be made by its manufacturer, is not guaranteed or endorsed by the publisher.
Supplementary material
The Supplementary Material for this article can be found online at: https://www.frontiersin.org/articles/10.3389/fsoil.2023.1129845/full#supplementary-material
References
1. Tilman D, Isbell F, Cowles JM. Biodiversity and ecosystem functioning. Annu Rev Ecol Evol Sys (2014) 45(1):471–93. doi: 10.1146/annurev-ecolsys-120213-091917
2. Tilman D, Reich PB, Knops JMH. Biodiversity and ecosystem stability in a decade-long grassland experiment. Nature (2006) 441(7093):629–32. doi: 10.1038/nature04742
3. Isbell F, Craven D, Connolly J, Loreau M, Schmid B, Beierkuhnlein C, et al. Biodiversity increases the resistance of ecosystem productivity to climate extremes. Nature (2015) 526(7574):574–7. doi: 10.1038/nature15374
4. Craven D, Isbell F, Manning P, Connolly J, Bruelheide H, Ebeling A, et al. Plant diversity effects on grassland productivity are robust to both nutrient enrichment and drought. Philos Trans R Soc B: Biol Sci (2016) 371(1694):20150277. doi: 10.1098/rstb.2015.0277
5. De Boeck HJ, Lemmens CMHM, Zavalloni C, Gielen B, Malchair S, Carnol M, et al. Biomass production in experimental grasslands of different species richness during three years of climate warming. Biogeosciences (2008) 5(2):585–94. doi: 10.5194/bg-5-585-2008
6. Tilman D, El Haddi A. Drought and biodiversity in grasslands. Oecologia (1992) 89(2):257–64. doi: 10.1007/BF00317226
7. Cherwin K, Knapp A. Unexpected patterns of sensitivity to drought in three semi-arid grasslands. Oecologia (2012) 169(3):845–52. doi: 10.1007/s00442-011-2235-2
8. Wagg C, O’Brien MJ, Vogel A, Scherer-Lorenzen M, Eisenhauer N, Schmid B, et al. Plant diversity maintains long-term ecosystem productivity under frequent drought by increasing short-term variation. Ecology (2017) 98(11):2952–61. doi: 10.1002/ecy.2003
9. Van Ruijven J, Berendse F. Diversity enhances community recovery, but not resistance, after drought. J Ecol (2010) 98(1):81–6. doi: 10.1111/j.1365-2745.2009.01603.x
10. Orwin KH, Wardle DA. Plant species composition effects on belowground properties and the resistance and resilience of the soil microflora to a drying disturbance. Plant Soil (2005) 278(1):205–21. doi: 10.1007/s11104-005-8424-1
11. Schmid MW, Hahl T, van Moorsel SJ, Wagg C, De Deyn GB, Schmid B. Feedbacks of plant identity and diversity on the diversity and community composition of rhizosphere microbiomes from a long-term biodiversity experiment. Mol Ecol (2019) 28(4):863–78. doi: 10.1111/mec.14987
12. Eisenhauer N, Beßler H, Engels C, Gleixner G, Habekost M, Milcu A, et al. Plant diversity effects on soil microorganisms support the singular hypothesis. Ecology (2010) 91(2):485–96. doi: 10.1890/08-2338.1
13. Scherber C, Eisenhauer N, Weisser WW, Schmid B, Voigt W, Fischer M, et al. Bottom-up effects of plant diversity on multitrophic interactions in a biodiversity experiment. Nature (2010) 468(7323):553–6. doi: 10.1038/nature09492
14. Chung H, Zak DR, Reich PB, Ellsworth DS. Plant species richness, elevated Co2, and atmospheric nitrogen deposition alter soil microbial community composition and function. Global Change Biol (2007) 13(5):980–9. doi: 10.1111/j.1365-2486.2007.01313.x
15. Thakur MP, Milcu A, Manning P, Niklaus PA, Roscher C, Power S, et al. Plant diversity drives soil microbial biomass carbon in grasslands irrespective of global environmental change factors. Global Change Biol (2015) 21(11):4076–85. doi: 10.1111/gcb.13011
16. van der Heijden MGA, Klironomos JN, Ursic M, Moutoglis P, Streitwolf-Engel R, Boller T, et al. Mycorrhizal fungal diversity determines plant biodiversity, ecosystem variability and productivity. Nature (1998) 396(6706):69–72. doi: 10.1038/23932
17. Wagg C, Jansa J, Schmid B, van der Heijden MGA. Belowground biodiversity effects of plant symbionts support aboveground productivity. Ecol Lett (2011) 14(10):1001–9. doi: 10.1111/j.1461-0248.2011.01666.x
18. Hall IR. Effects of endomycorrhizas on the competitive ability of white clover. New Z J Agric Res (1978) 21(3):509–15. doi: 10.1080/00288233.1978.10427441
19. Lambers H, Teste FP. Interactions between arbuscular mycorrhizal and non-mycorrhizal plants: Do non-mycorrhizal species at both extremes of nutrient availability play the same game. Plant Cell Environ (2013) 36(11):1911–5. doi: 10.1111/pce.12117
20. Gholamhoseini M, Ghalavand A, Dolatabadian A, Jamshidi E, Khodaei-Joghan A. Effects of arbuscular mycorrhizal inoculation on growth, yield, nutrient uptake and irrigation water productivity of sunflowers grown under drought stress. Agric Water Manage (2013) 117:106–14. doi: 10.1016/j.agwat.2012.11.007
21. Amiri R, Nikbakht A, Etemadi N. Alleviation of drought stress on rose geranium [Pelargonium graveolens (L.) herit.] in terms of antioxidant activity and secondary metabolites by mycorrhizal inoculation. Sci Hortic (2015) 197:373–80. doi: 10.1016/j.scienta.2015.09.062
22. Meddich A, Jaiti F, Bourzik W, Asli AE, Hafidi M. Use of mycorrhizal fungi as a strategy for improving the drought tolerance in date palm (Phoenix dactylifera). Sci Hortic (2015) 192:468–74. doi: 10.1016/j.scienta.2015.06.024
23. Begum N, Qin C, Ahanger MA, Raza S, Khan MI, Ashraf M, et al. Role of arbuscular mycorrhizal fungi in plant growth regulation: implications in abiotic stress tolerance. Front Plant Sci (2019) 10:1068. doi: 10.3389/fpls.2019.01068
24. Basyal B, Emery SM. An arbuscular mycorrhizal fungus alters switchgrass growth, root architecture, and cell wall chemistry across a soil moisture gradient. Mycorrhiza (2021) 31(2):251–8. doi: 10.1007/s00572-020-00992-6
25. Tommerup I. Temperature relations of spore germination and hyphal growth of vesicular-arbuscular mycorrhizal fungi in soil. Trans Br Mycological Soc (1983) 81(2):381–7. doi: 10.1016/S0007-1536(83)80090-4
26. Liu W, Zhang Z, Wan S. Predominant role of water in regulating soil and microbial respiration and their responses to climate change in a semiarid grassland. Global Change Biol (2009) 15(1):184–95. doi: 10.1111/j.1365-2486.2008.01728.x
27. de Vries FT, Williams A, Stringer F, Willcocks R, McEwing R, Langridge H, et al. Changes in root-Exudate-Induced respiration reveal a novel mechanism through which drought affects ecosystem carbon cycling. New Phytol (2019) 224(1):132–45. doi: 10.1111/nph.16001
28. Hawkes CV, Shinada M, Kivlin SN. Historical climate legacies on soil respiration persist despite extreme changes in rainfall. Soil Biol Biochem (2020) 143:107752. doi: 10.1016/j.soilbio.2020.107752
29. Patel KF, Myers-Pigg A, Bond-Lamberty B, Fansler SJ, Norris CG, McKever SA, et al. Soil carbon dynamics during drying vs. rewetting: Importance of antecedent moisture conditions. Soil Biol Biochem (2021) 156:108165. doi: 10.1016/j.soilbio.2021.108165
30. de Vries FT, Griffiths RI, Bailey M, Craig H, Girlanda M, Gweon HS, et al. Soil bacterial networks are less stable under drought than fungal networks. Nat Commun (2018) 9(1):3033. doi: 10.1038/s41467-018-05516-7
31. Schenck N, Graham S, Green N. Temperature and light effect on contamination and spore germination of vesicular-arbuscular mycorrhizal fungi. Mycologia (1975) 67(6):1189–92. doi: 10.1080/00275514.1975.12019864
32. Rillig MC. Arbuscular mycorrhizae and terrestrial ecosystem processes. Ecol Lett (2004) 7(8):740–54. doi: 10.1111/j.1461-0248.2004.00620.x
33. Hoeppner SS, Dukes JS. Interactive responses of old-field plant growth and composition to warming and precipitation. Global Change Biol (2012) 18(5):1754–68. doi: 10.1111/j.1365-2486.2011.02626.x
34. Karlowsky S, Augusti A, Ingrisch J, Akanda MKU, Bahn M, Gleixner G. Drought-induced accumulation of root exudates supports post-drought recovery of microbes in mountain grassland. Front Plant Sci (2018) 9:1593. doi: 10.3389/fpls.2018.01593
35. Karlowsky S, Augusti A, Ingrisch J, Hasibeder R, Lange M, Lavorel S, et al. Land use in mountain grasslands alters drought response and recovery of carbon allocation and plant-microbial interactions. J Ecol (2018) 106(3):1230–43. doi: 10.1111/1365-2745.12910
36. de Vries FT, Griffiths RI, Knight CG, Nicolitch O, Williams A. Harnessing rhizosphere microbiomes for drought-resilient crop production. Science (2020) 368(6488):270–4. doi: 10.1126/science.aaz5192
37. Vogelsang KM, Reynolds HL, Bever JD. Mycorrhizal fungal identity and richness determine the diversity and productivity of a tallgrass prairie system. New Phytol (2006) 172(3):554–62. doi: 10.1111/j.1469-8137.2006.01854.x
38. Hiiesalu I, Pärtel M, Davison J, Gerhold P, Metsis M, Moora M, et al. Species richness of arbuscular mycorrhizal fungi: associations with grassland plant richness and biomass. New Phytol (2014) 203(1):233–44. doi: 10.1111/nph.12765
39. Fu W, Chen B, Rillig MC, Jansa J, Ma W, Xu C, et al. Community response of arbuscular mycorrhizal fungi to extreme drought in a cold-temperate grassland. New Phytol (2021) 234:2003–17. doi: 10.1111/nph.17692
40. Bueno de Mesquita CP, Martinez del Río CM, Suding KN, Schmidt SK. Rapid temporal changes in root colonization by arbuscular mycorrhizal fungi and fine root endophytes, not dark septate endophytes, track plant activity and environment in an alpine ecosystem. Mycorrhiza (2018) 28(8):717–26. doi: 10.1007/s00572-018-0863-7
41. Goldmann K, Boeddinghaus RS, Klemmer S, Regan KM, Heintz-Buschart A, Fischer M, et al. Unraveling spatiotemporal variability of arbuscular mycorrhizal fungi in a temperate grassland plot. Environ Microbiol (2020) 22(3):873–88. doi: 10.1111/1462-2920.14653
42. Argüello A, O’Brien MJ, van der Heijden MGA, Wiemken A, Schmid B, Niklaus PA. Options of partners improve carbon for phosphorus trade in the arbuscular mycorrhizal mutualism. Ecol Lett (2016) 19(6):648–56. doi: 10.1111/ele.12601
43. Kiers ET, Duhamel M, Beesetty Y, Mensah JA, Franken O, Verbruggen E, et al. Reciprocal rewards stabilize cooperation in the mycorrhizal symbiosis. Science (2011) 333(6044):880–2. doi: 10.1126/science.1208473
44. Shaver GR, Canadell J, Chapin FS, Gurevitch J, Harte J, Henry G, et al. Global warming and terrestrial ecosystems: a conceptual framework for analysis: Ecosystem responses to global warming will be complex and varied. ecosystem warming experiments hold great potential for providing insights on ways terrestrial ecosystems will respond to upcoming decades of climate change. documentation of initial conditions provides the context for understanding and predicting ecosystem responses. BioScience (2000) 50(10):871–82. doi: 10.1641/0006-3568(2000)050[0871:Gwatea]2.0.Co;2
45. Van Peer L, Nijs I, Reheul D, De Cauwer B. Species richness and susceptibility to heat and drought extremes in synthesized grassland ecosystems: compositional vs physiological effects. Funct Ecol (2004) 18(6):769–78. doi: 10.1111/j.0269-8463.2004.00901.x
46. Vogel A, Scherer-Lorenzen M, Weigelt A. Grassland resistance and resilience after drought depends on management intensity and species richness. PloS One (2012) 7(5):e36992–e. doi: 10.1371/journal.pone.0036992
47. Craine JM, Ocheltree TW, Nippert JB, Towne EG, Skibbe AM, Kembel SW, et al. Global diversity of drought tolerance and grassland climate-change resilience. Nat Climate Change (2013) 3(1):63–7. doi: 10.1038/nclimate1634
48. Kahmen A, Perner J, Buchmann N. Diversity-dependent productivity in semi-natural grasslands following climate perturbations. Funct Ecol (2005) 19(4):594–601. doi: 10.1111/j.1365-2435.2005.01001.x
49. Kreyling J, Beierkuhnlein C, Elmer M, Pritsch K, Radovski M, Schloter M, et al. Soil biotic processes remain remarkably stable after 100-year extreme weather events in experimental grassland and heath. Plant Soil (2008) 308(1):175. doi: 10.1007/s11104-008-9617-1
50. Vogel A, Eisenhauer N, Weigelt A, Scherer-Lorenzen M. Plant diversity does not buffer drought effects on early-stage litter mass loss rates and microbial properties. Global Change Biol (2013) 19(9):2795–803. doi: 10.1111/gcb.12225
51. Roscher C, Schumacher J, Baade J, Wilcke W, Gleixner G, Weisser W, et al. The role of biodiversity for element cycling and trophic interactions: an experimental approach in a grassland community. Basic Appl Ecol (2004) 5:107–21. doi: 10.1078/1439-1791-00216
52. Ebeling A, Pompe S, Baade J, Eisenhauer N, Hillebrand H, Proulx R, et al. A trait-based experimental approach to understand the mechanisms underlying biodiversity–ecosystem functioning relationships. Basic Appl Ecol (2014) 15(3):229–40. doi: 10.1016/j.baae.2014.02.003
53. Roscher C, Schumacher J, Gubsch M, Lipowsky A, Weigelt A, Buchmann N, et al. Using plant functional traits to explain diversity–productivity relationships. PloS One (2012) 7(5):e36760. doi: 10.1371/journal.pone.0036760
54. Kivlin SN, Mann MA, Lynn JS, Kazenel MR, Taylor DL, Rudgers JA. Grass species identity shapes communities of root and leaf fungi more than elevation. ISME Commun (2022) 2(1):25. doi: 10.1038/s43705-022-00107-6
55. Sweeney CJ, de Vries FT, van Dongen BE, Bardgett RD. Root traits explain rhizosphere fungal community composition among temperate grassland plant species. New Phytol (2021) 229(3):1492–507. doi: 10.1111/nph.16976
56. Dassen S, Cortois R, Martens H, de Hollander M, Kowalchuk GA, van der Putten WH, et al. Differential responses of soil bacteria, fungi, archaea and protists to plant species richness and plant functional group identity. Mol Ecol (2017) 26(15):4085–98. doi: 10.1111/mec.14175
57. Hoffmann K, Bivour W, Früh B, Koßmann M, Voß P-H. Klimauntersuchungen in Jena für die Anpassung and Klimawandel und seine erwarteten Folgen: ein Ergebnisbericht. Berichte des Deutschen Wetterdienstes (2014) 243.
58. Vogel A, Fester T, Eisenhauer N, Scherer-Lorenzen M, Schmid B, Weisser WW, et al. Separating drought effects from roof artifacts on ecosystem processes in a grassland drought experiment. PloS One (2013) 8(8):e70997. doi: 10.1371/journal.pone.0070997
59. Wubet T, Weiß M, Kottke I, Oberwinkler F. Two threatened coexisting indigenous conifer species in the dry afromontane forests of Ethiopia are associated with distinct arbuscular mycorrhizal fungal communities. Can J Bot (2006) 84(10):1617–27. doi: 10.1139/b06-121
60. Morgan BST, Egerton-Warburton LM. Barcoded Ns31/Aml2 primers for sequencing of arbuscular mycorrhizal communities in environmental samples. Appl Plant Sci (2017) 5(8):1700017. doi: 10.3732/apps.1700017
61. Simon L, Lalonde M, Bruns TD. Specific amplification of 18s fungal ribosomal genes from vesicular-arbuscular endomycorrhizal fungi colonizing roots. Appl Environ Microbiol (1992) 58(1):291–5. doi: 10.1128/AEM.58.1.291-295.1992
62. Lee J, Lee S, Young JPW. Improved pcr primers for the detection and identification of arbuscular mycorrhizal fungi. FEMS Microbiol Ecol (2008) 65(2):339–49. doi: 10.1111/j.1574-6941.2008.00531.x
63. Wahdan S, Reitz T, Heintz-Buschart A, Schädler M, Roscher C, Breitkreuz C, et al. Organic agricultural practice enhances arbuscular mycorrhizal symbiosis in correspondence to soil warming and altered precipitation patterns. Environ Microbiol (2021) 23:6163–76. doi: 10.1111/1462-2920.15492
64. Ihrmark K, Bodeker IT, Cruz-Martinez K, Friberg H, Kubartova A, Schenck J, et al. New primers to amplify the fungal Its2 region–evaluation by 454-sequencing of artificial and natural communities. FEMS Microbiol Ecol (2012) 82(3):666–77. doi: 10.1111/j.1574-6941.2012.01437.x
65. Gardes M, Bruns TD. ITS primers with enhanced specificity for basidiomycetes–application to the identification of mycorrhizae and rusts. Mol Ecol (1993) 2(2):113–8. doi: 10.1111/j.1365-294X.1993.tb00005.x
66. Prada-Salcedo LD, Goldmann K, Heintz-Buschart A, Reitz T, Wambsganss J, Bauhus J, et al. Fungal guilds and soil functionality respond to tree community traits rather than to tree diversity in European forests. Mol Ecol (2021) 30(2):572–91. doi: 10.1111/mec.15749
67. Weißbecker C, Schnabel B, Heintz-Buschart A. Dadasnake, a snakemake implementation of Dada2 to process amplicon sequencing data for microbial ecology. GigaScience (2020) 9(12):giaa135. doi: 10.1093/gigascience/giaa135
68. Callahan BJ, McMurdie PJ, Rosen MJ, Han AW, Johnson AJA, Holmes SP. Dada2: High-resolution sample inference from illumina amplicon data. Nat Methods (2016) 13(7):581–3. doi: 10.1038/nmeth.3869
69. Martin M. Cutadapt removes adapter sequences from high-throughput sequencing reads. EMBnetjournal (2011) 17(1):10–2. doi: 10.14806/ej.17.1.200
70. Schloss PD, Westcott SL, Ryabin T, Hall JR, Hartmann M, Hollister EB, et al. Introducing mothur: open-source, platform-independent, community-supported software for describing and comparing microbial communities. Appl Environ Microbiol (2009) 75(23):7537–41. doi: 10.1128/AEM.01541-09
71. Quast C, Pruesse E, Yilmaz P, Gerken J, Schweer T, Yarza P, et al. The Silva ribosomal rna gene database project: improved data processing and web-based tools. Nucleic Acids Res (2012) 41(D1):D590–D6. doi: 10.1093/nar/gks1219
72. Nilsson RH, Larsson K-H, Taylor AFS, Bengtsson-Palme J, Jeppesen TS, Schigel D, et al. The unite database for molecular identification of fungi: handling dark taxa and parallel taxonomic classifications. Nucleic Acids Res (2018) 47(D1):D259–D64. doi: 10.1093/nar/gky1022
73. Öpik M, Davison J, Moora M, Zobel M. DNA-Based detection and identification of glomeromycota: the virtual taxonomy of environmental sequences. Botany (2014) 92(2):135–47. doi: 10.1139/cjb-2013-0110
74. Öpik M, Vanatoa A, Vanatoa E, Moora M, Davison J, Kalwij JM, et al. The online database maarjam reveals global and ecosystemic distribution patterns in arbuscular mycorrhizal fungi (Glomeromycota). New Phytol (2010) 188(1):223–41. doi: 10.1111/j.1469-8137.2010.03334.x
75. Katoh K, Kuma K-i, Toh H, Miyata T. Mafft version 5: improvement in accuracy of multiple sequence alignment. Nucleic Acids Res (2005) 33(2):511–8. doi: 10.1093/nar/gki198
76. Stamatakis A. Raxml version 8: A tool for phylogenetic analysis and post-analysis of Large phylogenies. Bioinformatics (2014) 30(9):1312–3. doi: 10.1093/bioinformatics/btu033
78. McMurdie PJ, Holmes S. Phyloseq: An r package for reproducible interactive analysis and graphics of microbiome census data. PloS One (2013) 8(4):e61217. doi: 10.1371/journal.pone.0061217
79. Chen B, He X, Pan B, Zou X, You N. Comparison of beta diversity measures in clustering the high-dimensional microbial data. PloS One (2021) 16(2):e0246893. doi: 10.1371/journal.pone.0246893
80. Arumugam M, Raes J, Pelletier E, Le Paslier D, Yamada T, Mende DR, et al. Enterotypes of the human gut microbiome. Nature (2011) 473(7346):174–80. doi: 10.1038/nature09944
83. Aben N, Westerhuis JA, Song Y, Kiers HA, Michaut M, Smilde AK, et al. Itop: Inferring the topology of omics data. Bioinformatics (2018) 34(17):i988–i96. doi: 10.1093/bioinformatics/bty636
84. Smilde AK, Kiers HAL, Bijlsma S, Rubingh CM, van Erk MJ. Matrix correlations for high-dimensional data: The modified rv-coefficient. Bioinformatics (2008) 25(3):401–5. doi: 10.1093/bioinformatics/btn634
85. Lin H, Peddada SD. Analysis of compositions of microbiomes with bias correction. Nat Commun (2020) 11(1):3514. doi: 10.1038/s41467-020-17041-7
86. Wright AJ, Ebeling A, de Kroon H, Roscher C, Weigelt A, Buchmann N, et al. Flooding disturbances increase resource availability and productivity but reduce stability in diverse plant communities. Nat Commun (2015) 6(1):6092. doi: 10.1038/ncomms7092
87. Roscher C, Gerighausen U, Schmid B, Schulze E-D. Plant diversity and community history shift colonization success from early- to mid-successional species. J Plant Ecol (2014) 8(3):231–41. doi: 10.1093/jpe/rtu011
88. Korell L, Auge H, Chase JM, Harpole S, Knight TM. We need more realistic climate change experiments for understanding ecosystems of the future. Global Change Biol (2020) 26(2):325–7. doi: 10.1111/gcb.14797
89. Abbaspour H, Saeidi-Sar S, Afshari H, Abdel-Wahhab MA. Tolerance of mycorrhiza infected pistachio (Pistacia Vera l.) seedling to drought stress under glasshouse conditions. J Plant Physiol (2012) 169(7):704–9. doi: 10.1016/j.jplph.2012.01.014
90. Ryan M, Ash J. Colonisation of wheat in southern new south Wales by vesicular-arbuscular mycorrhizal fungi is significantly reduced by drought. Aust J Exp Agric (1996) 36(5):563–9. doi: 10.1071/EA9960563
91. Al-Karaki GN, Al-Raddad A. Effects of arbuscular mycorrhizal fungi and drought stress on growth and nutrient uptake of two wheat genotypes differing in drought resistance. Mycorrhiza (1997) 7(2):83–8. doi: 10.1007/s005720050166
92. Deepika S, Kothamasi D. Soil moisture–a regulator of arbuscular mycorrhizal fungal community assembly and symbiotic phosphorus uptake. Mycorrhiza (2015) 25(1):67–75. doi: 10.1007/s00572-014-0596-1
93. Zhang F, Ni Q, Zou Y, Wu Q, Huang Y. Preliminary study on the mechanism of AMF in enhancing the drought tolerance of plants. J Fungal Res (2017) 15(1):8–13. doi: 10.13341/j.jfr.2014.1702
94. Varela-Cervero S, Vasar M, Davison J, Barea JM, Öpik M, Azcón-Aguilar C. The composition of arbuscular mycorrhizal fungal communities differs among the roots, spores and extraradical mycelia associated with five Mediterranean plant species. Environ Microbiol (2015) 17(8):2882–95. doi: 10.1111/1462-2920.12810
95. Phillips M, Weber S, Andrews L, Aronson E, Allen M, Allen E. Fungal community assembly in soils and roots under plant invasion and nitrogen deposition. Fungal Ecol (2019) 40:107–17. doi: 10.1016/j.funeco.2019.01.002
96. Wagg C, Jansa J, Stadler M, Schmid B, van der Heijden MGA. Mycorrhizal fungal identity and diversity relaxes plant–plant competition. Ecology (2011) 92(6):1303–13. doi: 10.1890/10-1915.1
97. Weber SE, Diez JM, Andrews LV, Goulden ML, Aronson EL, Allen MF. Responses of arbuscular mycorrhizal fungi to multiple coinciding global change drivers. Fungal Ecol (2019) 40:62–71. doi: 10.1016/j.funeco.2018.11.008
98. Tian Y-h, Lei Y-b, Zheng Y-l, Cai Z-q. Synergistic effect of colonization with arbuscular mycorrhizal fungi improves growth and drought tolerance of plukenetia volubilis seedlings. Acta Physiol Plant (2013) 35(3):687–96. doi: 10.1007/s11738-012-1109-5
99. Zhang F, Jia-Dong H, Qiu-Dan N, Qiang-Sheng W, Ying-Ning Z. Enhancement of drought tolerance in trifoliate orange by mycorrhiza: changes in root sucrose and proline metabolisms. Notulae Botanicae Horti Agrobotanici Cluj-Napoca (2018) 46(1):270–6. doi: 10.15835/nbha46110983
100. Hallett PD, Feeney DS, Bengough AG, Rillig MC, Scrimgeour CM, Young IM. Disentangling the impact of am fungi versus roots on soil structure and water transport. Plant Soil (2009) 314(1):183–96. doi: 10.1007/s11104-008-9717-y
101. Armada E, Leite MFA, Medina A, Azcón R, Kuramae EE. Native bacteria promote plant growth under drought stress condition without impacting the rhizomicrobiome. FEMS Microbiol Ecol (2018) 94(7). doi: 10.1093/femsec/fiy092
102. Breitkreuz C, Herzig L, Buscot F, Reitz T, Tarkka M. Interactions between soil properties, agricultural management and cultivar type drive structural and functional adaptations of the wheat rhizosphere microbiome to drought. Environ Microbiol (2021) 23(10):5866–82. doi: 10.1111/1462-2920.15607
103. Klaus VH, Hölzel N, Prati D, Schmitt B, Schöning I, Schrumpf M, et al. Plant diversity moderates drought stress in grasslands: implications from a Large real-world study on 13c natural abundances. Sci Total Environ (2016) 566-567:215–22. doi: 10.1016/j.scitotenv.2016.05.008
104. Chen Y, Vogel A, Wagg C, Xu T, Iturrate-Garcia M, Scherer-Lorenzen M, et al. Drought-exposure history increases complementarity between plant species in response to a subsequent drought. Nat Commun (2022) 13(1):3217. doi: 10.1038/s41467-022-30954-9
105. Walter J, Jentsch A, Beierkuhnlein C, Kreyling J. Ecological stress memory and cross stress tolerance in plants in the face of climate extremes. Environ Exp Bot (2013) 94:3–8. doi: 10.1016/j.envexpbot.2012.02.009
106. Knapp AK, Beier C, Briske DD, Classen AT, Luo Y, Reichstein M, et al. Consequences of more extreme precipitation regimes for terrestrial ecosystems. BioScience (2008) 58(9):811–21. doi: 10.1641/b580908
107. Santos-Medellín C, Edwards J, Liechty Z, Nguyen B, Sundaresan V. Drought stress results in a compartment-specific restructuring of the rice root-associated microbiomes. mBio (2017) 8(4):e00764–17. doi: 10.1128/mBio.00764-17
108. Jongen M, Albadran B, Beyschlag W, Unger S. Can arbuscular mycorrhizal fungi mitigate drought stress in annual pasture legumes? Plant Soil (2022) 472(1):295–310. doi: 10.1007/s11104-021-05233-z
109. Rudgers JA, Afkhami ME, Bell-Dereske L, Chung YA, Crawford KM, Kivlin SN, et al. Climate disruption of plant-microbe interactions. Annu Rev ecol evol sys (2020) 51(1):561–86. doi: 10.1146/annurev-ecolsys-011720-090819
110. Hawkins AP, Crawford KM. Interactions between plants and soil microbes may alter the relative importance of intraspecific and interspecific plant competition in a changing climate. AoB Plants (2018) 10(4). doi: 10.1093/aobpla/ply039
111. Chagnon P-L, Bradley RL, Maherali H, Klironomos JN. A trait-based framework to understand life history of mycorrhizal fungi. Trends Plant Sci (2013) 18(9):484–91. doi: 10.1016/j.tplants.2013.05.001
112. Jayne B, Quigley M. Influence of arbuscular mycorrhiza on growth and reproductive response of plants under water deficit: a meta-analysis. Mycorrhiza (2014) 24(2):109–19. doi: 10.1007/s00572-013-0515-x
113. Badaruddin M, Meyer DW. Water use by legumes and its effect on soil water status. Crop Sci (1989) 29(5):cropsci1989.0011183X002900050025x. doi: 10.2135/cropsci1989.0011183X002900050025x
114. Helgason T, Merryweather JW, Denison J, Wilson P, Young JPW, Fitter AH. Selectivity and functional diversity in arbuscular mycorrhizas of Co-occurring fungi and plants from a temperate deciduous woodland. J Ecol (2002) 90(2):371–84. doi: 10.1046/j.1365-2745.2001.00674.x
115. Johnson D, Vandenkoornhuyse PJ, Leake JR, Gilbert L, Booth RE, Grime JP, et al. Plant communities affect arbuscular mycorrhizal fungal diversity and community composition in grassland microcosms. New Phytol (2004) 161(2):503–15. doi: 10.1046/j.1469-8137.2003.00938.x
116. Sendek A, Karakoç C, Wagg C, Domínguez-Begines J, do Couto GM, van der Heijden MGA, et al. Drought modulates interactions between arbuscular mycorrhizal fungal diversity and barley genotype diversity. Sci Rep (2019) 9(1):9650. doi: 10.1038/s41598-019-45702-1
117. Bei Q, Moser G, Wu X, Müller C, Liesack W. Metatranscriptomics reveals climate change effects on the rhizosphere microbiomes in European grassland. Soil Biol Biochem (2019) 138:107604. doi: 10.1016/j.soilbio.2019.107604
118. Stockinger H, Krüger M, Schüßler A. DNA Barcoding of arbuscular mycorrhizal fungi. New Phytol (2010) 187:461–74. doi: 10.1111/j.1469-8137.2010.03262.x
119. Lekberg Y, Vasar M, Bullington LS, Sepp S-K, Antunes PM, Bunn R, et al. More bang for the buck? can arbuscular mycorrhizal fungal communities be characterized adequately alongside other fungi using general fungal primers? New Phytol (2018) 220(4):971–6. doi: 10.1111/nph.15035
120. Francioli D, van Rijssel SQ, van Ruijven J, Termorshuizen AJ, Cotton TEA, Dumbrell AJ, et al. Plant functional group drives the community structure of saprophytic fungi in a grassland biodiversity experiment. Plant Soil (2020) 461:91–105. doi: 10.1007/s11104-020-04454-y
121. Francioli D, Van Ruijven J, Bakker L, Mommer L. Drivers of total and pathogenic soil-borne fungal communities in grassland plant species. Fungal Ecol (2020) 48:100987. doi: 10.1016/j.funeco.2020.100987
122. Maggi O. Diversity and variability in soil fungi from a disturbed tropical rain forest. Mycologia (1998) 90(2):206–14. doi: 10.1080/00275514.1998.12026900
Keywords: biodiversity-ecosystem functioning relationships, experimental drought, plant-fungi interaction, AMF, Jena Experiment
Citation: Albracht C, Eisenhauer N, Vogel A, Wagg C, Buscot F and Heintz-Buschart A (2023) Effects of recurrent summer droughts on arbuscular mycorrhizal and total fungal communities in experimental grasslands differing in plant diversity and community composition. Front. Soil Sci. 3:1129845. doi: 10.3389/fsoil.2023.1129845
Received: 22 December 2022; Accepted: 28 March 2023;
Published: 21 April 2023.
Edited by:
César Marín, Santo Tomás University, ChileReviewed by:
Pierre-Luc Chagnon, Montreal University, CanadaCarlos Aguilar-Trigueros, Freie Universität Berlin, Germany
Copyright © 2023 Albracht, Eisenhauer, Vogel, Wagg, Buscot and Heintz-Buschart. This is an open-access article distributed under the terms of the Creative Commons Attribution License (CC BY). The use, distribution or reproduction in other forums is permitted, provided the original author(s) and the copyright owner(s) are credited and that the original publication in this journal is cited, in accordance with accepted academic practice. No use, distribution or reproduction is permitted which does not comply with these terms.
*Correspondence: Anna Heintz-Buschart, a.u.s.heintzbuschart@uva.nl