Enhancing Climate Resilience of Rain-Fed Potato Through Legume Intercropping and Silicon Application
- 1The Consultative Group for International Agricultural Research (CGIAR), Research Program on Climate Change, Agriculture and Food Security (CCAFS), Nairobi, Kenya
- 2International Potato Center, Nairobi, Kenya
- 3Department of Land Resource Management and Agricultural Technology, College of Agriculture and Veterinary Sciences, University of Nairobi, Nairobi, Kenya
- 4The CGIAR Research Program on Roots, Tubers and Bananas (RTB), Nairobi, Kenya
- 5Department of Agricultural Sciences and Technology, School of Agriculture and Enterprise Development, Kenyatta University, Nairobi, Kenya
- 6Bingen Technical University of Applied Sciences, Bingen am Rhein, Germany
A large portion of sub-Saharan Africa is situated in belts of uncertain rainfall and are characterized by low soil fertility with limited capacity to adapt to and mitigate the impacts of climate change. A field study was conducted in the semi-humid potato-growing belt of Kenya to test the effect of legume intercropping and water soluble silicon (orthocilicic acid) on soil erosion, and use efficiency of light and water. Potato (Solanum tuberosum L.) was grown singly and intercropped with dolichos (Lablab purpureus L.) or hairy vetch (Vicia sativa L.). Each cropping system was subjected to granular water-soluble silicon (Si) amendment at two rates [2.5 kg Si ha−1 (+Si) vs. 0 kg Si ha−1 (–Si)]. Plants receiving Si maintained significantly higher (p < 0.05) percent relative leaf water content (62–89% vs. 52–72% in controls) and exhibited higher concentrations of proline (1.99–2.91 vs. 1–1.19 umol g−1), soluble carbohydrates (28–59 vs. 10–28 umol g−1) and electrolyte conductance (1,409–3,903 vs. 746–2,307 mS cm−1). Legume intercropping enhanced groundcover establishment and reduced soil and nutrient losses by 45–80% compared with sole potato. Crop yields were 2–3-fold greater in intercropping relative to sole potato and were significantly greater in treatments subjected to Si application. Land equivalent ratios were above unity in intercropping but less than unity in sole potato, and were 8–20% increased by Si application. Use efficiency of water (5.99–9.09 Kg ha−1 m−3) and light (1.98–2.98 g MJ−1) were significantly greater under legume intercropping compared with sole potato (1.13–3.23 Kg ha−1 m−3 and 0.77–0.98 g MJ−1, respectively) and increased with Si application. Integrative use of Si and legume intercropping presents the smallholder farmers an opportunity to increase productivity of potato while enhancing resource use efficiency and soil fertility in the semi-humid tropics.
Introduction
Declining soil productivity and potato yield in sub-Saharan Africa (SSA) presents the need to develop more sustainable production systems. The average potato yield in this region is only 13 t ha−1 against potential yield of 40 t ha−1 (FAOSTAT, 2017; Gitari et al., 2018a). This situation is expected to deteriorate as climatic change is projected to lead to warmer and drier conditions with more variable and extreme weather events (Meehl et al., 2007). Declining rainfall and rising temperatures, which are associated with this change will have a direct negative effect on vegetation cover, which in turn will contribute to soil degradation because of the exposure of the soil surface to wind and water erosion. Consequently, SSA is projected to lose about 14% of cultivable land and about 20% of its pasture production potential by 2080 (Shah et al., 2008).
Potato is vulnerable to water deficit because of its fibrous root systems, which are concentrated in the upper (0–0.3 m) soil profile (Gitari et al., 2018a,b; Nyawade et al., 2018; Gitari et al., 2020b). Appreciable decrease in tuber yield occurs if a balance between soil temperature, soil moisture content, and crop nutrient uptake is not attained (Ferreira and Gonçalves, 2007; Nyawade et al., 2019b, 2020). High temperatures within the ridges at tuber initiation cause fluctuations in soil moisture, resulting in tuber malformation and tuber growth cracks (Polgar et al., 2017). Below soil water content of 20%, the matric potential of the ridges and furrows decline rapidly, slowing the redistribution of water within the soil profile (Nyawade et al., 2018). This impairs the nutrient uptake potential of potato leading to irregular plant growth.
There is therefore a growing need to provide farmers with practical and sustainable solutions to maintain potato yields under more challenging conditions while using water, nutrients, and soil resources more efficiently. Legume intercropping is one of such climate-smart cropping practices suitable to smallholder farmers. This is due to its potential to achieve multiple benefits that relate to climate mitigation and adaptation and general risk aversion via diversification (Gitari et al., 2019a,b; Nyawade and Gitari, 2020). Intercropping that includes forage legumes improves quality of forage while improving soil structure (Nyawade et al., 2018). This in turn increases water infiltration and air circulation, thus improving soil water-holding capacity.
The effectiveness of intercropping technology may be enhanced by use of silicon (Crusciol et al., 2009; Farhad et al., 2011; Pilon et al., 2014). Silicon is absorbed by plants mainly as orthosilicic acid (H4SiO4) also known as monosilicic or silicic acid (Mitani and Ma, 2005). At pH > 9, orthosilicic acid occurs in ionized form (H3SiO4/H2SiO4) (Knight and Kinrade, 2001). Most soils with pH < 9 thus contain Si as undissociated silicic acid over concentrations range of 0.1–0.6 mM and is in equilibrium with soil SiO2 at pH 3 (Tubaña and Heckman, 2015). At low soil pH, Si may reduce soil phosphorus sorption by increasing the soil pH or by competing for Mn, Fe, and Al ions for the active sites thereby enhancing P availability for plant uptake (Pilon et al., 2014).
Nevertheless, little research has been conducted to assess the effect of Si application on nutrient availability and crop water productivity. This information is needed for identification of climate-smart management practices that would sustainably increase the productivity of soil in the current and future climatic scenario. Therefore, the present work was designed to test the hypothesis that potato-legume intercropping interacts with silicon application to increase light and water productivity, and minimize the high soil erosion rates in the semi-humid sloppy terrains of Kenya.
Materials and Methods
Study Area
This study was conducted at Upper Kabete Research Farm of University of Nairobi, Kenya during the 5 rainy seasons commencing in 2016 long rains and running to 2018 short rains. The farm is located at latitude 1°14′45.00″S and longitude 36°44′19.51″E (Figure 1). The region is characterized by semi-humid climatic conditions and lies in agro-ecological region II, which receives an average rainfall amount of 1,000 mm per annum. These rains come in two seasons with the wet season occurring between March and August, and dry season between September and December. The area has an annual mean temperature of 20.4°C and is dominated by semi-intensive farming system where potato production accounts for more than half of the total cropped area (Jaetzold et al., 2006; Kibunja et al., 2018). The soils in Kabete are dark red friable clay classified as Humic Nitisol (Jaetzold et al., 2006). Details of the measured soil properties (0–0.3 m depth) before and after the experiment are provided in Table 1.
Experimental Design and Crop Husbandry
A split-plot randomized complete block study design was implemented in runoff plots measuring 5.8 m long by 2.4 m wide and were separated from each other by 1 m path with four replicates (blocks). Cropping system was considered a whole-plot factor and Si application as subplot factor. Each cropping system was subjected to granular water-soluble silicon (Si) amendment at rates of 2.5 kg ha−1 (+Si) and 0 kg ha−1 Si (–Si, the controls). The water-soluble Si was in the form of orthocilicic acid (OSA) with 0.4% Si and 5% fulvic acid (Privi Nutrifight-Privi Life Sciences PVT.LTD).
The cropping system consisted of potato (Solanum tuberosum L.) grown singly and intercropped with hairy vetch (Vicia sativa L.) or dolichos (Lablab purpureus L.), and the respective single crop of the legumes. The “Unica” (CIP 392797.22) potato cultivar used in this study is locally used for chips, crisp and boiling. This cultivar is heat and water stress tolerant making it well-adapted to wide range of agro-ecological conditions [National Potato Council of Kenya (NPCK), 2017; Rukundo et al., 2019]. The Rongai cultivar of dolichos (KT 003) used has a high biomass production with high crude protein content. This cultivar is used both as forage and as pulse and is a popular choice in the midlands and lowlands as a cover crop on infertile, acidic soils, and because of its high tolerance to drought. Vetch is not commonly grown by the farmers in this study area, but has great capacity to supplement the pasture and conserve the soil against erosion.
Sole potato rows were spaced 0.9 m with an interplant spacing of 0.3 m giving a plant population of 37,037 per ha. Sole legumes were spaced 0.5 m between rows and 0.3 m within rows giving plant population of 66,667 per ha. Intercropping was done in the inter-rows of potato spaced at 0.9 m, at ~0.45 m from potato to the legume with interplant spacing of 0.3 m (Figure 2). This gave a plant population of 101,074 plants per ha. All the potato treatments received basal fertilization at a rate of 50 kg N ha−1, 90 kg ha−1 of P2O5, 100 kg ha−1 of K2O and topdress of 40 kg N ha−1. Legumes received only basal phosphorus (46% triple super phosphate) applications at a rate of 20 kg ha−1. Weeding and hilling were performed at 14–21 days after crop emergence by hand hoeing. The legumes were sprayed with Duduthrin 1.7 EC (Lambda-cyhalothrin 17.5 g L−1) alternating with Bestox 100 EC (Alpha-cypermethrin 50 g L−1) to control aphids while potatoes were sprayed alternately with Ridomil Gold MZ 68WG (Mefenoxam 40 g kg−1 + Mancozeb 640 g kg−1) and Agriphite-600 (phosphonate) to control potato blight disease. Potatoes were harvested at maturity by digging out the tubers using hand hoes while dolichos were harvested by plucking out the pods and retaining the residues, which together with hairy vetch biomass were incorporated back into the soil.
Soil Sampling and Soil Physico-Chemical Analyses
Soil samples were taken from each plot at 0–0.3 m depths just before planting and at the end of each season using soil auger. For each plot, the soil samples were drawn from the inner rows and bulked to give one composite sample. The soils were passed through 2 mm sieve, analyzed for gravimetric moisture content, and stored at 4°C. Soil pH was measured in 1:2.5 soil to water suspensions, soil texture by the hydrometer method (Gee and Bauder, 1986), total N by modified Kjeldahl method (Bremner and Mulvaney, 1982) and organic carbon by modified Walkley and Black method (Nelson and Sommers, 1996). Extraction of soil samples for analysis of available P and extractable K was done using Mehlich 1 procedures (Mylavarapu et al., 2002) and determined using UV–vis spectrophotometer (Murphy et al., 1998) and flame photometry (Jackson, 1967) methods, respectively.
Climatic Data
Rainfall amount was recorded after every rainfall event using an onsite rain gauge. Air temperature were obtained from the onsite HOBO temperature sensors. Micro-lysimeters installed to a depth of 0.3 m were used to estimate the soil evaporation. The cumulative seasonal rainfall amounts received during the potato-growing period of 2016 long rains, 2016 short rains, 2017 long rains, 2017 short rains and 2018 long rains were 338, 194, 269, 372, and 388 mm, respectively (Figure 3). These rains occurred mainly at potato sprout development and at tuber initiation regardless of the season. The corresponding mean maximum air temperatures were 26.3, 21.2, 22.9, 22.3, and 21.2°C, respectively.
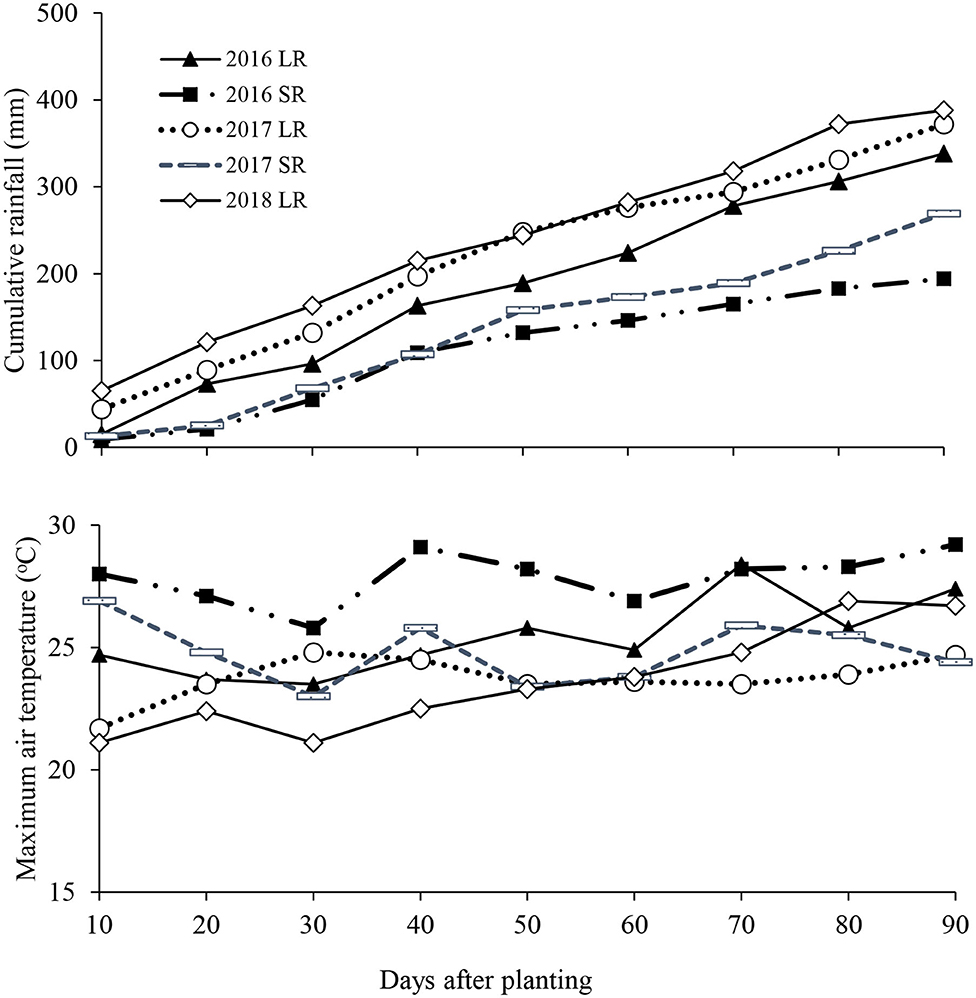
Figure 3. Rainfall and temperature for the period between potato planting and harvesting. LR and SR denote long and short rains, respectively.
Soil Temperature, Soil Water Content, and Soil Water Retention
Tensiometers (0–100 kPa) installed at 0.3 m were used to measure the soil water contents and soil water potential. The tensiometers located at radial distances of 0.15 and 0.3 m represented ridge and furrow positions in the potato plots. Soil temperature were recorded by automatic Onset HOBO USeries, UX120-006 M data-logging equipment at 1 h common step.
Estimation of Root Length Density
Root samples were taken separately at 0–0.3 and 0.3–0.9 m soil depths to establish the spatial complementarity exerted by legume intercropping. The roots were extracted using 0.15 m × 0.1 m × 0.1 m metal cores directly driven under the plant rhizosphere (Bohm, 1979; Nyawade et al., 2018). Soil cores containing the roots were placed in a bucket of water and gently agitated to break down larger soil particles, and to remove the debris and dead roots. This mixture was sieved through 2 mm mesh placed in a shallow tub of water to wash away fine soil particles attached to the roots. The roots were floated on shallow water placed in a tray and scanned using Epson Expression 1680 Scanner (Seiko Epson Corp., Tokyo, Japan). Root length density analysis was done using WinRHIZO Root Analyzer System (Regent Instruments Inc., Quebec, Canada) (Equation 1).
Assessment of Leaf Water Content
Four plants (potato and legumes) were harvested at 40 days after emergence for determination of leaf water content (LWC). Five leaves sampled from the fifth fully expanded leaf (from the plant apex) of the four plants were used (Barr and Weatherley, 1962). Sampling was done from the center of the leaves avoiding the veins and immediately weighed to prevent water loss, and to obtain the fresh weight (FW). The leaves were placed in petri dishes and soaked in deionized water for 12 h in the dark to attain saturation. The leaf samples were wiped with paper towels to remove the excess water and weighed again to get the turgid weight (TW). The samples were oven dried at 65°C for 24 h and placed in a desiccator for 30 min to reach ambient temperature. The leaves were weighed to obtain dry weight (DW) and the LWC computed using Equation (2).
Physiological and Biochemical Processes
Canopy temperature and stomatal conductance were measured by a portable photosynthesis system (LI-6400, LI-COR, Lincoln, NE, USA) at vegetative phase of potato growth. Nine plants from each experimental plot were randomly selected and the measurements were conducted on the fourth petiole and averaged. All the measurements were done on a sunny day.
Computations of proline contents was done at 40 days after emergence (beginning of tuber bulking stage) (Pilon et al., 2014). The leaf samples used were obtained from the fourth leaf of the plant apex, which were wrapped in aluminum foil, immersed in liquid N and stored in a freezer (−80°C) for subsequent biochemical analysis. The leaf samples (1 g) were extracted with 3% sulphosalicylic acid and 2 mL of the extract added to 1 mL acid ninhydrin and 1 mL glacial acetic acid (Bates et al., 1973). The mixture was held in a water bath for 1 h before a 2 mL toluene was added. The proline concentrations were measured with a spectrophotometer (Shimadzu UVmini-1240, Shimadzu Corp., Kyoto, Japan) at a wavelength of 520 nm and calculated against a standard proline (Pilon et al., 2014).
For total soluble carbohydrates, leaves from 4 randomly sampled plants (both potato and the legumes) were placed in oven with forced air circulation at 70°C for 72 h. Upon drying, the leaves were ground to powdery and the powder stored in a glass container in the dark at 15°C until biochemical analyses. About 20 mg of the leaf powder was incubated with 2.0 μL of 80% ethanol at 95°C for 20 min and centrifuged for 5 min at 20°C. A 100 μL supernatant sample was carefully decanted and added to a solution containing 1.25 μL of 100% H2SO4, 70 μL of 15% phenol, and 580 μL H2O before the soluble carbohydrate contents were measured at 490 nm using glucose as a standard (Dubois et al., 1956).
Leaf electrolyte conductance was measured at stolon and tuber formation stage of potato growth using procedure adapted from Hirashima et al. (2009). The uppermost fully expanded leaf trifoliate, both for potato and legumes was selected. The three leaflets, excluding the petioles were cut, placed in 50 ml eppendorf plastic tubes and covered to minimize water loss. About 20 mL of deionized water was added to 50 ml eppendorf plastic tubes, covered with lids and shaken for 2 h on an orbital shaker at 144 revolutions per minute (rpm). Initial conductance was measured using a conductivity meter (Model WTWCond3110 fitted with a tetracon 325 electrode; WTW 82362 Wellheim, Germany). This measurement indicated free leaf electrolytes outside the leaf cell membrane (apoplast). The leaf segments were boiled by placing the tubes on a 100°C water bath for 15 min followed by centrifuging at 144 rpm for another 2 h. The conductance of the boiled leaf tissues was measured after cooling down to a room temperature. This conductance indicated electrolyte status of electrolytes in the apoplast and electrolytes, which were previously bound within the leaf cell membrane (symplast) before being released because of aggravated membrane damage arising from exposure of the leaf segments to the hot water bath. To allow for direct comparison of apoplast, symplast and total leaf electrolyte status of the potato and legume species evaluated, the leaf segments were oven-dried to a constant weight at 85°C for 24 h.
Chlorophyll Content, Light Interception, Crop Yield and Radiation Use Efficiency
At 60 days after potato emergence (middle period of the tuber bulking stage), two leaf samples from the third leaf were collected for determination of chlorophyll content (Moran and Porath, 1980). The leaf samples were placed in 1.5 mL of dimethylformamide for 48 h and then incubated. The chlorophyll readings were taken using a spectrophotometer at wavelengths of 480, 646.8, and 663.8 nm.
Radiation interception of photosynthetically active radiation (PAR) and leaf area index (LAI) were measured from 14 days after potato emergence and progressively at 14–16 days interval until physiological maturity using a Sunfleck Ceptometer-LP-80 (Decagon Devices, Pullman, WA, USA). Measurements were taken only under blue-sky conditions with no or minimum clouds between 1130 and 0130 h (local time), and during a period of constant incident solar radiation. For each measurement, nine above and below canopy readings were taken perpendicularly to the crop rows to ensure that more leaf area was exposed to the light sensors. Corresponding LAI values were read upon averaging the above and below canopy readings. Plot values were computed from the average of four successive middle row readings. The PAR intercepted was calculated using Equations (3–5) (Koocheki et al., 2016).
Where PAR = photosynthetically active radiation (400–700 nm); LAI = leaf area index, λ = light extinction coefficient; PARo = PAR incident equal to half the daily global radiation (Monteith and Unsworth, 1990). Daily global radiation was estimated from the daily sunny hours recorded from the adjacent meteorological station. The light extinction coefficient (λ) was determined from the slope of the linear regression between the natural logarithm of radiation transmission and leaf area index (Monteith, 1965).
The tuber and legume yields were estimated from the central 1.2 m2 area of each plot. About 500 g of tuber harvested from each plot were sliced and dried in an oven at 65°C for 72 h and reweighed to determine the tuber dry weight. For the legumes, the shoot biomass estimations was done by cutting the plants at the soil line using machetes. The dry mass was determined by oven-drying about 500 g samples at 65°C to a constant mass. The yields were converted into potato equivalent (PEY) using Equation (6) (Gitari et al., 2018a, 2020a). For dolichos, the estimations considered grain and shoot biomass separately as this legume is used as both pulse and forage.
Where PEY = potato equivalent yield, PY = potato yield, LY = legume yield, PP = market price of potato (0.38 US$ kg−1) and LP = market price of the legume (0.51, 0.28, and 1.15 US$ kg−1 for vetch forage, dolichos forage, and dolichos grain, respectively).
Radiation use efficiency (RUE) (g MJ−1) was estimated by fitting a linear regression (least square) to the cumulative amount of radiation absorption (MJ m−2) and dry matter accumulation from successive harvests (g m−2) (Monteith, 1994). The slope of each regression was taken as the RUE for each treatment.
Estimation of Soil Water Balance and Crop Water Productivity
The topsoil (0–0.3 m) water balance was estimated at the end of each season according to procedures outlined by Allen et al. (1998) (Equation 7).
where Dr, i and Dr, i−1 are the cumulative depth of evaporation (root-zone moisture depletion) following complete wetting from the exposed and wetted fraction of the topsoil at the end of time i and time i-1, respectively, P is precipitation, RO is runoff from the soil surface, I is net irrigation depth that infiltrates into the soil, CR is capillary rise from the groundwater table, ET is the actual crop evapotranspiration, and Dp is deep percolation beyond the root-zone, ΔSW = change in soil water storage in root zone between planting and harvesting (ΔSW). All terms are expressed in mm relative to time i, that is, start of season and harvest. Capillary rise was assumed negligible because the groundwater table was more than 25 m below the soil surface (Karuku et al., 2014). The total amount of surface runoff was quantified from the runoff plots, soil evaporation by micro-lysimeters installed in each plot. Deep percolation (Dp) was estimated as residual term of potato root-zone water balance (Bethune et al., 2008). No irrigation water was applied throughout the growing period, similar to what the farmers in this area do.
Crop water productivity (CWP) was computed using Equation (8) (Pereira et al., 2012).
Where PEY = potato equivalent yield; P = precipitation; CR = capillary rise of water; ΔSW = change in soil water storage in root zone between planting and harvesting period (ΔSW) and I = irrigation (I); R = runoff.
Quantification of Soil Loss and Runoff
Eroded sediment was quantified following procedures described by Wendelaar and Purkins (1979). The runoff-sludge mixture was thoroughly stirred, allowing the resultant suspension to settle for 30 min. The runoff water overlying the settled sludge was decanted and measured using a graduated bucket. A 100 ml suspension sample was oven-dried at 105°C for 48 h and expressed as dry soil mass in grams per liter. Total soil loss was computed using Equation (9).
Runoff from each plot was converted into mm depth using Equation (10).
Nutrient loss was expressed as enrichment ratio, the ratio of nutrient element in the eroded sediment to that in the source soil (Polyakov and Lal, 2008).
Assessment of Intercrop Productivity
The land equivalent ratio (LER) (Mead and Willey, 1980) was used to evaluate the productivity of intercrops over sole cropping (Equation 11) while the system productivity index (SPI) (Odo, 1991) was used to assess the stability of the intercropping systems (Equation 12).
Where Yil and Yip are intercrop yields of legume and potato, respectively, while Ysl and Ysp are the sole yields of legume and potato. LER > 1 indicates a production advantage; LER = 1 indicates no production advantage; and LER < 1 indicates a production disadvantage of the crop system.
Where Ypi and Yli depict the economic yield of potato and legume under intercropping, respectively, whereas Yps and Yls represents the respective yields under pure stands.
Data Analysis
The statistical analyses were performed in R software, version 3.4.2 (R Core Team, 2017) using linear mixed model analysis of variance (ANOVA). The datasets explored were soil temperature, soil moisture, canopy temperature, leaf water content, stomatal conductance, proline contents, total soluble carbohydrates, leaf electrolyte conductance, root length density, chlorophyll content, light interception, crop yield, radiation use efficiency, crop water productivity, soil loss, and runoff. The mixed-models were defined with the R-package lme4 (Bates et al., 2015) using likelihood based inference (Demidenko, 2013). Treatments and their interactions were considered as fixed factors while season and blocks (replications) were regarded as random factors because the study objective was to compute the variability of treatment differences across different seasons. Significant interactions between fixed factors were detected using likelihood ratio tests for generalized linear mixed models. Whenever treatment effects were significant, the response variable was subjected to Tukey's Honest Significant Difference (HSD) test (p ≤ 0.05) for multiple mean comparisons with the agricolae package. Differences between treatments are indicated by different lower-case letters in figures and tables. Mean values are reported with standard errors, except for soil loss, runoff, and enrichment ratios where estimates are reported with 95% confidence intervals. Whenever necessary, data were either log transformed or an unequal variance model used to meet assumptions of normality and equal variance. Substitutive design was used to hold the total density of the intercrop constant and vary the ratio among the intercrop species.
Results
Changes in Soil Moisture and Soil Temperature
There were significant differences (p ≤ 0.05) in the mean volumetric soil water contents (SWCs) measured between the different cropping systems and Si applications (Table 2). Compared with the sole potato stands, which recorded the lowest SWC (0.08–0.15 cm3 cm−3), the highest SWC in the surface soil layer (0–0.3 m) was measured under potato-dolichos intercropping (0.17–0.24 cm3 cm−3) irrespective of Si application. The soil moisture content were influenced by Si application irrespective of the cropping system. The corresponding matric potential values varied among the treatments and were significantly higher (p ≤ 0.05) in intercrops (−44–59 cmH2O) relative to sole potato (−85–99 cmH2O) and increased with Si application. Mean soil temperatures (23.1–27.1°C) were higher in sole potato plots than in potato-legume intercropping (20.9–23.1°C). Similarly, soil evaporation was significantly greater under sole potato (92.2–101.3 mm) than under intercrops (61.1–81.1 mm) and were significantly influenced by Si application (p ≤ 0.01). The soil water balance was greatest under sole legumes (6.5–14.2 mm) followed by intercrops (5.2–11.1 mm) and lowest in sole potato (3.3–5.1 mm). Dolichos recorded higher soil water balance compared with vetch. Seasons, and interaction of Si and cropping system had significant effect on soil water balance (p ≤ 0.05).
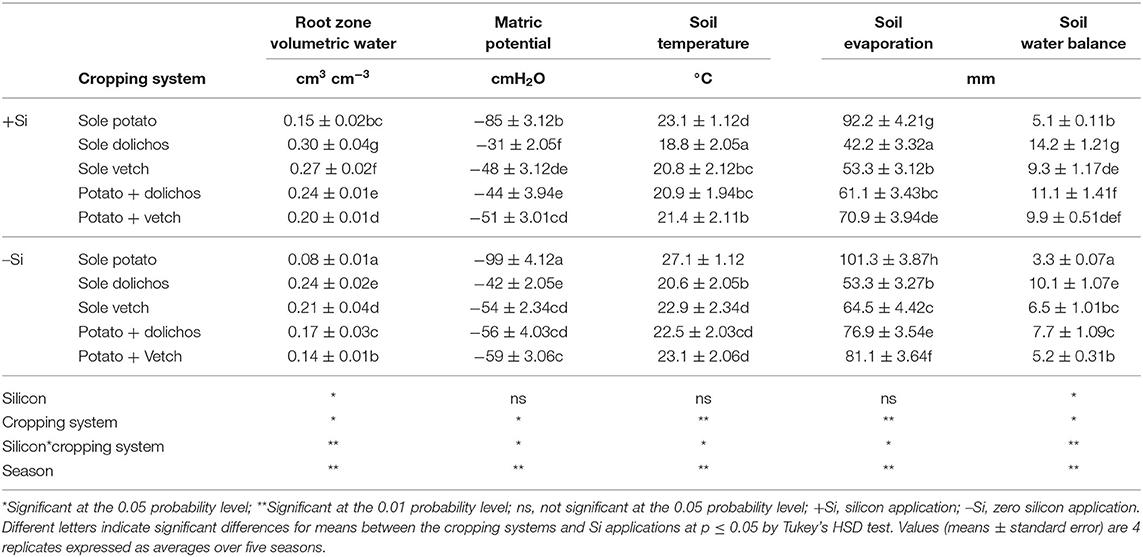
Table 2. Effect of silicon application and legume intercropping on soil, soil temperature, soil evaporation, and soil water balance.
Intercropping and Silicon Application Effects on Crop Growth
Intercropping and Si interaction exhibited significant effect on crop growth and leaf nitrogen content (Table 3; Figure 4). Leaf area index was significantly greater in intercrops (3.3–4.5) followed by sole stands of legumes (3.3–4.1) and was lowest in pure potato stands (2.1–2.8). Leaf area index increased by 18–33% with Si application. Intercropping significantly (p ≤ 0.05) lowered the leaf extinction coefficient of both legumes and potato with mean value of 0.56 in sole potato compared with 0.41 in intercrops. Specific leaf nitrogen ranged from 1.13–1.63 g N m−2 leaf in sole potato compared with 1.88–2.84 g N m−2 leaf in intercrops and were up to 51% increased by Si application. Stomatal conductance ranged from 0.21–0.36 molm2 s−2 in sole potato compared with 0.48–0.55 molm2 s−2 in intercropping, and significantly increased with Si application. Chlorophyll contents were similarly higher (p < 0.01) in intercrops (5.52–8.30 ug m−2) relative to sole potato treatments (3.12–4.93 ug m−2) and increased with Si application. The LAI, chlorophyll content, stomatal conductance, and specific leaf nitrogen content responded significantly to Si application and to Si by cropping system interaction (p ≤ 0.05). Season had significant effect on LAI, leaf extinction coefficient, and stomatal conductance.
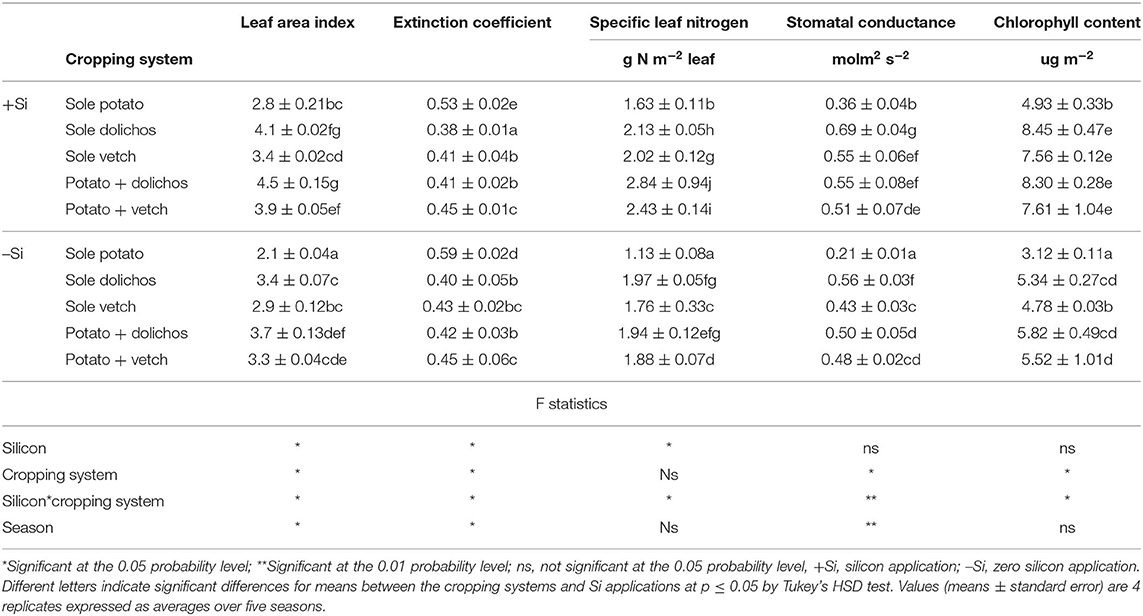
Table 3. Effect of silicon application and legume intercropping on leaf are index, leaf orientation, leaf nitrogen, stomatal conductance, and chlorophyll content.
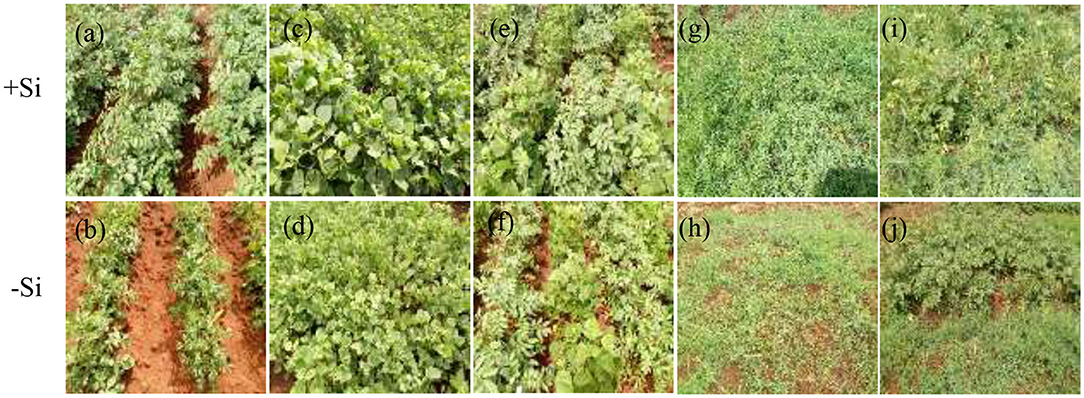
Figure 4. Effect of Si application and legume intercropping on crop growth. (a,b) sole potato, (c,d) sole dolichos, (e,f) potato-dolichos intercrop, (g,h) sole vetch, (i,j) potato-vetch intercrop, with Si (+Si) and without Si (–Si) applications, respectively.
Root length density (RLD) increased from 4.13–5.29 cm cm−3 in sole potato to 5.52–6.32 cm cm−3 in intercrops. The potato plants subjected to Si application exhibited significantly higher RLD both in the 0–0.3 and 0.3–0.9 m depths (Figure 5). In the 0.3–0.9 m depth, sole legumes exhibited significantly higher RLD (8.94–10.40 cm cm−3) followed by intercrops (5.96–7.90 cm cm−3) and lowest in sole potato (0.28–1.01 cm cm−3). Dolichos generally showed significant higher RLD density (p ≤ 0.05) in relation to vetch irrespective of cropping system and Si application.
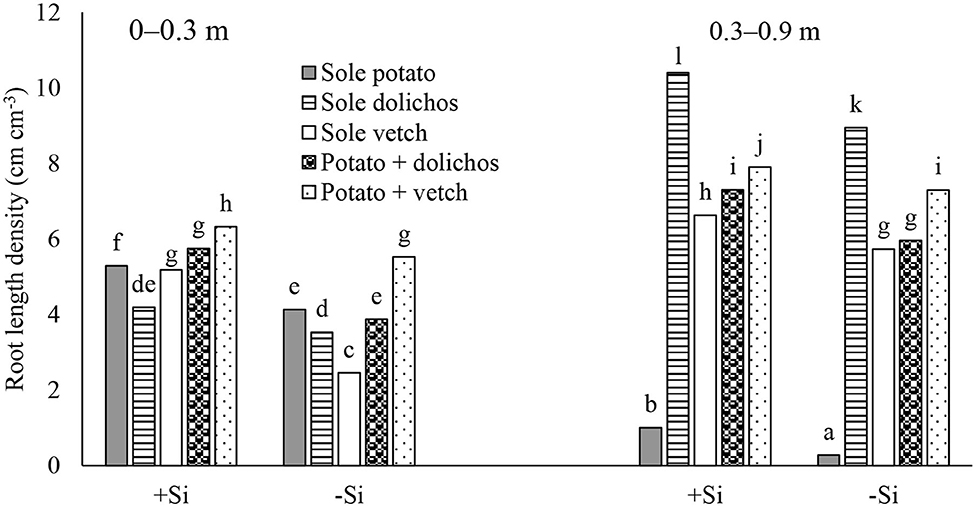
Figure 5. Root length density as affected by intercropping and silicon application. +Si, silicon application; –Si, zero silicon application. Different letters indicate significant differences for means between the cropping systems and Si applications at p ≤ 0.05 by Tukey's HSD test.
Canopy Temperature and Biochemical Processes
Intercropping significantly (p ≤ 0.05) lowered the canopy temperature from 25.6 to 27.8°C in sole potato to 18.7–21.8°C, the effect of which increased with Si application (Table 4). The percent leaf water content was significantly higher (p ≤ 0.05) in the plants subjected to combined application of Si and intercropping (75.2–78.5%) compared with the –Si treatments (62.9–63.3%). Leaf electrolyte conductance ranged from 746–1,409 mS cm−1 in sole potato compared with 1,423–3,107 mS cm−1 in intercropping and were increased by 36–111% with Si application. Silicon application increased the contents of total soluble carbohydrates from 28 to 59 umol g−1 (110%). Similarly, Si application increased the contents of proline from 1.0 to 2.9 umol g−1 in sole potato (191%), 1.12–2.3 umol g−1 (104%) in potato-dolichos intercropping and 1.08 to 2.32 umol g−1 (115%) in potato-vetch intercropping. A significant effect of Si cropping system, season and Si by cropping system interaction was found on canopy temperature, leaf water content, proline content, total soluble carbohydrates and leaf electrolyte conductance.
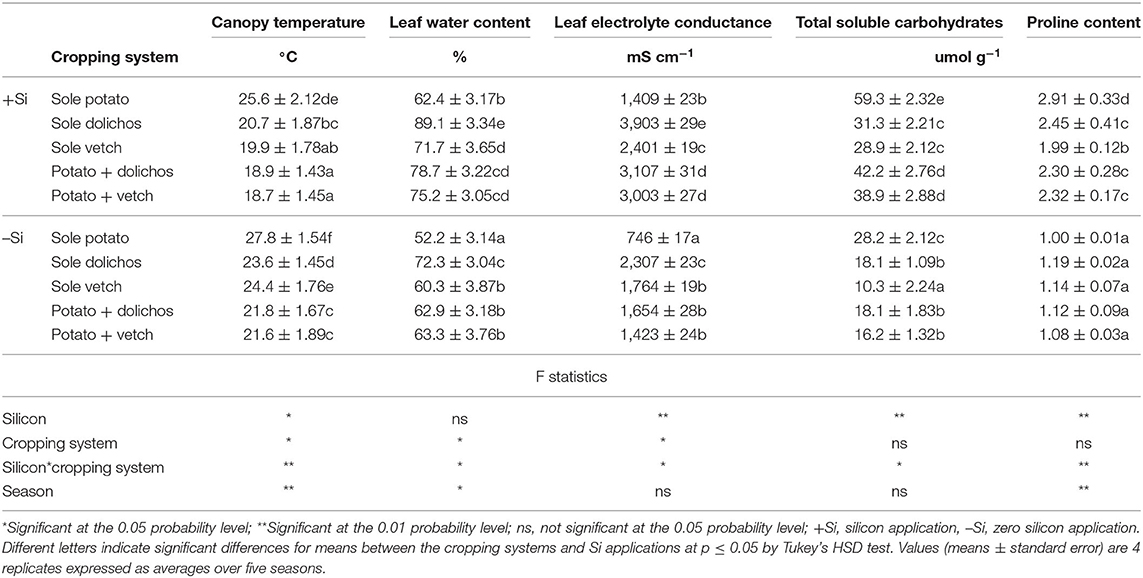
Table 4. Silicon and intercropping effects on canopy temperature, leaf water content, and crop biochemical processes.
Soil Erosion and Nutrient Loss
Cumulative soil loss and runoff differed significantly among the treatments (p ≤ 0.05) and were consistently highest in sole potato plots (198–221 t ha−1 and 88–108 mm) and lowest in potato-dolichos intercrop (79–99 t ha−1 and 42–52 mm) plots, respectively (Table 5). Enrichment ratios of the measured parameters were above unity irrespective of treatments and differed significantly among the treatments (p ≤ 0.05) except for the sand fractions. The highest values of enrichment ratios for NPK were recorded in sole potato plots (1.87–3.09) and lowest in sole dolichos plots (1.21–1.39). Even though an observable reduction in the enrichment ratio was observed due to Si application, these values were not significantly different from the controls (–Si treatments).
Intercropping Productivity
Cumulative biomass was significantly greater in intercrops (8.8–15.8 t ha−1) than in sole potato stands (1.9–2.1 t ha−1) and increased significantly with Si application (Table 6). Highest potato equivalent yields were recorded by intercrops relative to sole crop of potato, vetch, or dolichos. Potato equivalent yields for potato-dolichos system subjected to Si application was 14.5 t ha−1 greater than that of sole dolichos, and 23.3 t ha−1 than that of sole potato. Yield increase due to vetch intercropping was 11.6 and 16 t ha−1 greater than that of sole vetch and sole potato respectively. Irrespective of the Si application, crop water productivity was 3–8-fold greater in intercrop relative to sole potato. Fractions of light intercepted were consistently greatest in intercrops (0.67–0.87) than in sole legumes (0.54–0.76) and lowest in the sole potato stand (0.31–0.43) (p ≤ 0.05). Intercrop of potato and dolichos subjected to Si application recorded the highest significant RUE, which was 3-fold greater than that of sole potato.
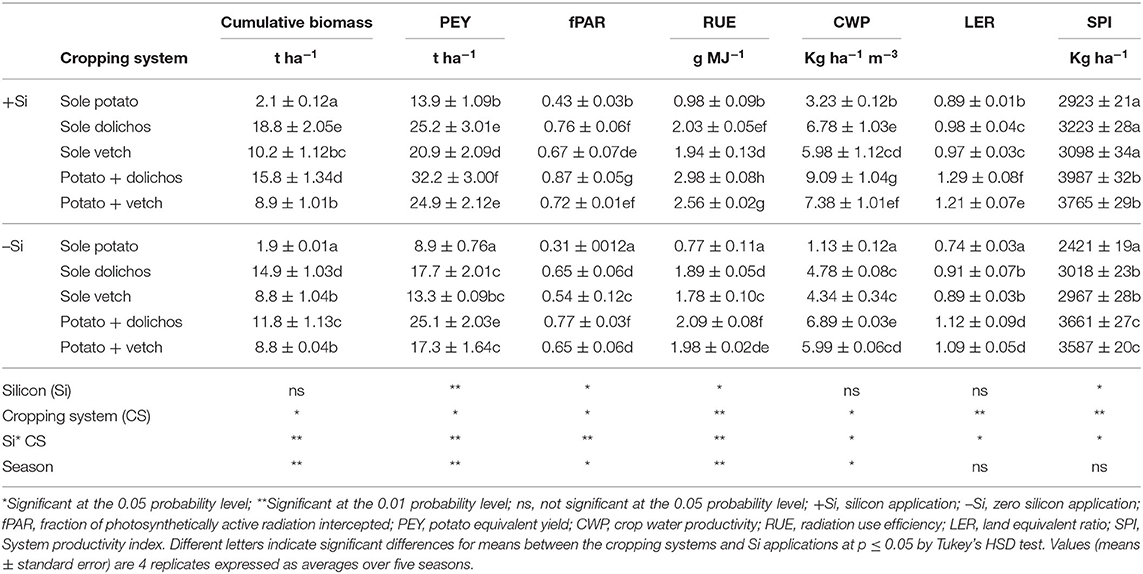
Table 6. Silicon and legume intercropping effect on biomass yield, potato yield, and resource use efficiency.
Land equivalent ratios were greater than unity in intercrops compared with sole cropping system of potato, which recorded LER values below unity. Regardless of the cropping system, application of Si significantly increased the crop water productivity, radiation use efficiency, and light interception (PAR). Silicon and intercropping interaction had significant effect on land productivity as measured by LER. System productivity index (SPI) was significantly (p ≤ 0.05) influenced by potato-legume intercropping systems. The index increased with Si application.
Discussions
Intercropping and Silicon Effect on Soil Moisture and Soil Temperature
The significantly higher soil water content within the potato root-zone in intercropped plots indicated long-term moisture accumulation. The greater rooting system by potato grown in intercropping increased the capacity of crops to extract water by increasing the size of the water reservoir. While potato crop was characterized by roots that rarely exceeded a vertical depth of 0.3 m, dolichos and vetch roots colonized the 0.3–0.9 m depth. Legumes could therefore access soil water to deeper subsurface layers than the potato crop, allowing potato to draw the soil moisture in the 0–0.3 m depth. Giller (2015) highlighted that legumes have prolonged water uptake from deep soil layers. This helps the plants to improve their leaf water status and to maintain transpiration rates and dry matter production. As legume intercropping enhanced canopy formation and overlap, this meant a high groundcover that considerably reduced soil evaporation and increased soil water storage.
Soil temperatures were lower in intercropping due to the shade created by the canopy overlap between the intercrop components. The higher soil water content under intercropping further enhanced the soil cooling. Generally, a dry soil has lower heat capacity than a wet soil (Li et al., 2017). For the sole potato plots, heat from the topsoil was easily lost to the atmosphere through the intervening bare soil. Silicon application enhanced soil cooling due to its indirect effect on soil temperature as this amendment facilitated potato and legume growth thus enhancing canopy formation and subsequent shading of the soil (see the photo on Figure 4).
Effect of Silicon Application on Potato Growth, Physiology and Biochemical Composition
The greater LAI due to intercropping could be explained by the differences that existed in the vertical foliage arrangement and canopy architecture of the different crop varieties. Potato canopy was generally characterized by bare surfaces between the crop rows. While vetch provided low-density canopy, which closed the bare spaces between potato rows, the effective canopy overlap by dolichos bridged the inter-row spacing thus enhancing the LAI development. Nyawade et al. (2019a) observed that the larger and nearly vertical leaves exhibited by dolichos provided complementarity to the slender and more bent leaves of potato thus increasing the LAI. Even though the full canopy closure of potato occurred only after 40–45 days followed by rapid decline after physiological maturity, the legume components conferred a complimentary canopy that kept LAI relatively high during these periods. The higher leaf extinction coefficient (above 0.5) observed in pure potato stand was an indication of sagged leaves with increased propensity to converge solar radiation.
Plant growth and photosynthesis capacity were influenced by Si application. Silicon strengthened the plant tissues, an observation reflected in the relatively lower leaf extinction coefficient. This observation was supported by the measured high leaf water content in plants receiving Si relative to the controls. These plants were thus able to provide more leaf area toward light, thereby enhancing light interception. In addition, silicon increased the specific leaf nitrogen content, which is a major component of plant chlorophyll. Similar results have been found in a related study (Albiski et al., 2012). The increase in root length density due to Si application implies that Si promoted maintenance of root growth at the expense of shoot growth, a factor which has been shown to contribute to drought tolerance (Jefferies, 1993).
Both Si and legume intercropping significantly lowered the canopy temperatures and thus conferred an effective cooling mechanism to potato plants. This improved the plant tolerance to water deficit and to the high heat stress. Meunier et al. (2017) reported that Si stimulates the formation of plant-silicified structures, which offset leaf heat-load in plants. As was reflected in its additive effect on proline accumulations, Si altered the biochemical composition of potato tissues making the plant more resistant to heat stress under water deficit conditions. This is in agreement with the previous work, which demonstrated the role of proline in offsetting the adverse effects of water deficit thereby conferring stress tolerance (Sapre and Vakharia, 2016). Other studies have verified that proline concentrations in potato leaves increase with increase in Si concentrations under water-deficit conditions, which indicates that silicon may be associated with plant osmotic adjustment (Crusciol et al., 2009; Farhad et al., 2011; Pilon et al., 2014). This argument is supported by the fact that proline represents a water loss regulatory mechanism that reduces cell water potential (Fumis and Pedras, 2002), and is a biochemical marker of metabolic alterations generated by different types of stress (Lima et al., 2004). High proline levels enable potato plants to maintain cell turgidity, a fact attributed to the more negative osmotic potential of the cells and consequently alleviating the effects of water deficit (Moussa and Abdel-Aziz, 2008).
The increase in total leaf electrolyte conductance by treatments subjected to Si was possibly a consequence of Si-induced osmotic adjustment process that according to previous studies triggers accumulation of compatible solutes including sugars, ions, and amino acids in response to water deficit and heat stress (Crusciol et al., 2009; Farhad et al., 2011; Pilon et al., 2014). Accordingly, these plants had significantly higher total soluble carbohydrates content compared with the plants not subjected to Si application. The increased accumulation of solutes was a strategy meant to lower leaf osmotic potential and allow movement of water into the leaf cells, thereby maintaining turgor potential (Midega et al., 2017). This consequently increased plant tissue tolerance to low soil water conditions. According to Chaves et al. (2003), the accumulated solutes sequester water molecules and protect the cell membranes and protein complexes, thus allowing continued cell metabolic functioning. Leaf electrolyte conductance and total carbohydrate content of potato grown in intercrop without Si application were similar to those of sole potato receiving Si. This suggests that intercropping caused the potato plants to maintain their leaf cell membrane stability, which not only enhanced retention of water but also enabled accumulation of electrolytes within the symplast during exposure to heat and water deficit stress. This observation was attributed to the enhanced soil water content caused by decreased soil evaporation under higher ground cover contributed by legume intercrops.
Intercropping Effect on Soil Erosion
Compared with pure potato plots, soil loss was significantly reduced by intercropping relative to sole potato cropping. These losses were generally higher in the wet seasons, at emergence and after crop senesces when canopy cover was below 40%. This time constituted 60% of the cumulative soil loss in pure potato plots indicating that vegetal cover had effect on soil detachment. Seasonal effect was due mainly to the rainfall amounts, which were higher in the wet seasons. Potato took about 2 weeks to sprout and up to 45 days to close the canopy while dolichos emerged after 7 days and closed the canopy in only 21 days. The canopy closure with vetch occurred at 28 days after planting and was characterized by low dense cover with slender leaves and high propensity to control soil erosion. The canopy closure with dolichos was extended up to 1 month after potato harvest thus minimizing the offseason soil losses. The higher kinetic energy of rainfall that occurred early in the season sagged the weak leaf petioles, increasing the amount of bare soil between the potato rows and weakening the dissipating effect of the canopy on energy. The canopy heterogeneity under intercropping contributed by differences in plant heights, generally intercepted and dispersed the raindrops at different levels. The first raindrops that hit the canopy were intercepted and dispersed by the intercrops, thus gradually weakening their erosion potential. Ma et al. (2015) noted that the degree of leaf bending under splash effect differs with the type of plants with some leguminous crops being more prone to bending under high kinetic energy of rainfall due to their wider and softer leaves.
The highest values of enrichment ratio for total nitrogen, available phosphorus, extractable potassium, magnesium, and calcium were recorded in pure potato plots pointing to the high nutrient losses under pure potato stands. Potato delayed to establish protective cover and left the soil highly exposed to erosion, thus causing a substantial loss to the applied fertilizer. These losses occurred mainly in the first few weeks after planting when heavy rainstorms caused substantial soil erosion. Compared with vetch, growth of dolichos was more rapid and provided protective soil cover, which significantly minimized the nutrient losses. The enrichment ratio was particularly high for phosphorus because this element is usually adsorbed and fixed as iron phosphates in acidic soils (Quinton et al., 2001) and is therefore mobilized with the eroded sediment. The result thus implies that a slight soil loss through erosion may lead to a greater loss of phosphorus. The enrichment ratio for exchangeable potassium was lower than that of total nitrogen and phosphorus because phosphorus is uniformly distributed within the soil profile (Nyawade, 2015).
Across the treatments, the enrichment ratio of total soil organic carbon was above unity indicating that most of the eroded sediment was enriched in soil organic matter. This suggests the preferential transport of soil organic matter in sediments probably due to its low density. Rainfall splash effect may have peeled the soil aggregates exposing their outer layers, which have higher SOC concentration compared with the inner core resulting into SOC ratios above unity (Ghadiri and Rose, 1991). The soil pH enrichment ratio was above unity suggesting that the eroded soil material was enriched in bases relative to the source soil and may lead to Ca, Mg, K, and Na deficiency. This was asserted by the enrichment ratio of CEC, which was above unity in all the plots indicating that the eroded soil materials had higher positive charges relative to the source soil material. The enrichment ratio for clay and silt were greater than one, but that for sand was less than unity, indicating that the erosion process was selective, carrying with it the lighter material (clay and silt) and leaving the heavier material in the plots. This is because the energy required to entrain and transport silt and clay particles is comparatively lower than that of the coarser sand-sized aggregates (Boix-Fayos et al., 2009).
Intercropping and Silicon Effect on Crop Yield and Resource Productivity
Intercropping increased biomass yield, an observation that was associated with either increase in numbers of tubers per hill or increase in individual tuber weight. The yields were greater in the wet seasons due to the greater rainfall amounts. The intercropping effect on soil temperature was of great importance in explaining the yield variability between the treatments. In a study conducted by Radeni and Caesar (1986), heating the soil to 28°C reduced flow of assimilates to tubers. Similarly, Krauss and Marschner (1984) observed cessation of starch accumulation when developing tubers were subjected to soil temperature of 30°C. It could also be possible that allocation of assimilated carbon into non-structural and structural carbon was altered by the high soil temperature (Arai-Sanoh et al., 2010). For the legumes, the effects of high soil temperature were mediated in part by the deep roots coupled with their good adaptations to high temperatures.
Synergistic interaction between silicon application and intercropping on crop biomass accumulation under water deficit conditions was evident. Silicon enhanced the production of photoassimilates as was shown by the greater accumulation of total soluble carbohydrates. Further, the Si-mediated increase of water uptake under water deficit conditions enabled the potato plants to tolerate short periods of water- stress, minimizing its detrimental effects on tuber yield. For the sole potato plots not applied with Si, water stress not only restrained the potato foliage and plant development but also limited the number of leaves. Potato plants with few leaves are unable to produce large number of tubers and therefore exhibit lower yields (Obidiegwu et al., 2015).
The potato plants supplied with Si in intercropping recorded higher LAI and number of leaves, which enhanced light interception and biomass accumulation. In addition, potato intercrop exhibited greater root length density, which enhanced the crop's capacity to absorb soil water. The adverse effect of water shortage altered chlorophyll contents of the potato plants, decreased the leaf stomatal conductance and inhibited their net photosynthesis. These plants had limited capacity to mobilize the photosynthates to tubers leading to less dry matter formed per amount of water used.
The increased solar radiation interception and use efficiency by intercropping relative to monocropping was asserted to the increased canopy size and duration. The intercrops generally attained maximum canopy above 3, a value that corresponds to full groundcover by a typical potato cropping system (Burke, 2017). This was partly attributed to the increased number of leaves forming on lateral branches of potato grown in intercrops. Plants in intercropping systems were thus able to occupy all the empty niches thus contributing strongly to canopy size and radiation interception. Vetch put short, dense canopy with many slender leaves relative to dolichos, which established tall, broad dense crown with fewer interior leaves, which allowed more light to pass directly through the canopy. Unlike legumes, which indicated progressive growth with little response to prevailing heat stress, potato crop responded by developing leaves showing downward curvatures. This mechanism greatly reduced leaf area exposure to solar radiation and thus reduced radiation interception. When the crops suffered longer heat stress, potato leaves drooped followed by wilting that started from the lower strata leaves. Only leaves that exhibited some level of greenness recovered turgor and finalized their production cycle. These leaves however had limited capacity to absorb solar radiation, an observation affirmed by the proportional decrease of LAI with increasing soil and ambient temperatures.
The land equivalent ratios recorded under intercropping systems were greater than one, an indication that integration of legumes into potato-based cropping systems favored growth and yield of the companion crops grown in mixtures. The system productivity index of intercrops were greater than that of the sole potato, which was an indication that interspecific facilitation was greater than interspecific competition (Gitari et al., 2020a). This implies that intercropping resulted in greater land-use efficiency and resource productivity (Wahla et al., 2009; Machiani et al., 2018). This ability was enhanced by Si application as was indicated by the higher LER and SPI values. Seasonal effects on LER and SPI were due to fluctuating rainfall amounts, which were generally greater in the wet seasons.
Conclusion
This study demonstrated the beneficial effect of legume intercropping and silicon application on resource productivity, potato yield, and risk aversion in the semi-humid tropics. For silicon to be fully integrated into potato cropping, the element should be considered essential for higher plants and should form part of soil amendment. Silicate fertilizer should be processed from the complex silicate minerals dominating in the tropics and be availed in the local markets. As this study did not integrate an open participatory learning approach, it lacked the farmer-researcher interactions necessary for enhanced adoption. To bridge this gap, close connections with the farming sector, including the farmers, extension services, and fertilizer blending companies is necessary during scaling up. Where possible, crowdsourcing applications can be adopted to provide inputs that meet the researchers' needs and help in closing the knowledge dissemination loop between researchers and practitioners and foster farmer-to-farmer interactions.
Data Availability Statement
The raw data supporting the conclusions of this article will be made available by the authors, without undue reservation.
Author Contributions
SN conceptualized the idea, took part in the design of study, and drafted and proofread the manuscript. HG, NK, CG, KS, ES-G, and MP took part in synthesis of idea, design of the manuscript, and manuscript review and proofreading. All authors contributed to the article and approved the submitted version.
Conflict of Interest
The authors declare that the research was conducted in the absence of any commercial or financial relationships that could be construed as a potential conflict of interest.
Acknowledgments
This work was implemented as part of the CGIAR Research Program on Climate Change, Agriculture and Food Security (CCAFS), which is carried out with support from CGIAR Fund Donors and through bilateral funding agreements. For details, please visit https://ccafs.cgiar.org/donors. The authors greatly acknowledge Privi Life Sciences Private Limited for providing the orthocilicic acid amendment. The views expressed in this document cannot be taken to reflect the official opinions of these organizations.
References
Albiski, F., Najla, S., Sanoubar, R., Alkabani, N., and Murshed, R. (2012). In vitro screening of potato lines for drought tolerance. Physiol. Mol. Bio. Plants 18, 315–321. doi: 10.1007/s12298-012-0127-5
Allen, R. G., Pereira, L. S., Raes, D., and Smith, M. (1998). “Crop evapotranspiration. Guidelines for computing crop water requirements,” in FAO Irrigation and Drain Paper 56 (FAO, Rome), 300.
Arai-Sanoh, Y., Tsutomu, I., Akihiro, O., and Motohiko, K. (2010). Effects of soil temperature on growth and root function in rice. Plant Prod. Sci. 13, 235–242. doi: 10.1626/pps.13.235
Barr, H. D., and Weatherley, P. E. (1962). A re-examination of relative turgidity technique for estimating water deficit in leaves. Aust. J. Biol. Sci. 15, 413–428. doi: 10.1071/BI9620413
Bates, D., Machler, M., Bolker, B. M., and Walker, S. C. (2015). Fitting linear mixed-effects models using lme4. J. Stat. Softw. 67, 1–48. doi: 10.18637/jss.v067.i01
Bates, L. S., Waldren, R. P., and Teare, I. D. (1973). Rapid determination of free proline for water-stress studies. Plant Soil 39, 205–207. doi: 10.1007/BF00018060
Bethune, M. G., Selle, B., and Wang, Q. J. (2008). Understanding and predicting deep percolation under surface irrigation. Water Resour. Res. 44:W12430. doi: 10.1029/2007WR006380
Boix-Fayos, C., Vente, J., Albaladejo, J., and Martinez-Mena, M. (2009). Soil carbon erosion and stock as affected by land use changes at the catchment scale in Mediterranean ecosystems. Agr. Ecosys. Environ. 133, 75–85. doi: 10.1016/j.agee.2009.05.013
Bremner, J. M., and Mulvaney, C. S. (1982). “Nitrogen total,” in Methods of Soil Analysis, Part 2, Chemical and Microbiological Properties, 2nd Edn., Vol. 9. Agron. eds A. L. Page, R. H. Miller, and D. R. Keeney (Madison: ASA; SSSA), 595–624.
Chaves, M. M., Maroco, J. P., and Pereira, J. S. (2003). Understanding plant responses to drought: from genes to the whole plant. Funct. Plant Biol. 30, 239–264. doi: 10.1071/FP02076
Crusciol, A. C., Adriano, L., Pulz, L. B., Lemos, R. P., Soratto, R., and Giuseppina, P. P. (2009). Effects of silicon and drought stress on tuber yield and leaf biochemical characteristics in potato. Crop Sci. 49, 949–954. doi: 10.2135/cropsci2008.04.0233
Dubois, M., Gilles, K. A., Hamilton, J. K., Rebers, P. A., and Smith, F. (1956). Colorimetric method for determination of sugars and related substances. Anal. Chem. 28, 350–356. doi: 10.1021/ac60111a017
FAOSTAT (2017). Available online at: http://www.fao.org/faostat/en/#data/QC (accessed June 26, 2020).
Farhad, M. S., Babak, A. M., Reza, Z. M., Hassan, R. S. M., and Afshin, T. (2011). Response of proline, soluble sugars, photosynthetic pigments and antioxidant enzymes in potato (Solanum tuberosum L.) to different irrigation regimes in greenhouse condition. Aust. J. Crop Sci. 5, 55–60. Available online at: http://www.cropj.com/masoudi_5_1_2011_55_60.pdf
Ferreira, T. C., and Gonçalves, D. A. (2007). Crop-yield/water-use production functions of potatoes (Solanum tuberosum L.) grown under differential nitrogen and irrigation treatments in a hot dry climate. Agric. Water Manage. 90, 45–55. doi: 10.1016/j.agwat.2007.02.012
Fumis, T. F., and Pedras, J. F. (2002). Variation in levels of proline, diamine and polyamines in wheat cultivars under drought stress. (in Portuguese, with English abstract). Pesqi. Agropecu. Bras. 37, 449–459. doi: 10.1590/S0100-204X2002000400004
Gee, G. W., and Bauder, J. W. (1986). “Particle-size analysis,” in Methods of Soil Analysis, Part 1. Vol. 9. Agron, 2nd Edn., ed A. Klute (Madison: ASA), 383–411.
Ghadiri, H., and Rose, C. W. (1991). Sorbed chemical-transport in overland-flow: enrichment ratio variation with erosion processes. J. Environ. Qual. 20, 634–641. doi: 10.2134/jeq1991.00472425002000030021x
Giller, K. E. (2015). Nitrogen Fixation in Tropical Cropping Systems, 2nd Edn. Wallingford, CT: CABI, 423.
Gitari, H. I., Gachene, C. K. K., Karanja, N. N., Kamau, S., Nyawade, S., and Schulte-Geldermann, E. (2019b). Potato-legume intercropping on a sloping terrain and its effects on soil physico-chemical properties. Plant Soil 438, 447–460. doi: 10.1007/s11104-019-04036-7
Gitari, H. I., Gachene, C. K. K., Karanja, N. N., Kamau, S., Nyawade, S., Sharma, K., et al. (2018a). Optimizing yield and economic returns of rain-fed potato (Solanum tuberosum L.) through water conservation under potato-legume intercropping systems. Agric. Water Manag. 208, 59–66. doi: 10.1016/j.agwat.2018.06.005
Gitari, H. I., Karanja, N. N., Gachene, C. K. K., Kamau, S., Sharma, K., and Schulte-Geldermann, E. (2018b). Nitrogen and phosphorous uptake by potato (Solanum tuberosum L.) and their use efficiency under potato-legume intercropping systems. Field Crops Res. 222, 78–84. doi: 10.1016/j.fcr.2018.03.019
Gitari, H. I., Nyawade, S., Kamau, S., Raza, M. A., Karanja, N. N., Gachene, C. K. K., et al. (2020a). Revisiting intercropping indices with respect to potato-legume intercropping systems. Field Crops Res. 258:107957. doi: 10.1016/j.fcr.2020.107957
Gitari, H. I., Nyawade, S. O., Kamau, S., Gachene, C. K. K., Karanja, N. N., and Schulte-Geldermann, E. (2019a). Increasing potato equivalent yield increases returns to investment under potato-legume intercropping systems. Open Agric. 4, 623–629. doi: 10.1515/opag-2019-0062
Gitari, H. I., Shadrack, N., Kamau, S., Gachene, C. K. K., Karanja, N. N., and Schulte-Geldermann, E. (2020b). Agronomic assessment of phosphorus efficacy for potato (Solanum tuberosum L) under legume intercrops. J. Plant Nutr. 43, 864–878. doi: 10.1080/01904167.2019.1702202
Hirashima, M., Tanaka, R., and Tanaka, A. (2009). Light-independent cell death induced by accumulation of pheophorbide a in Arabidopsis thaliana. Plant Cell Physiol. 50, 719–729. doi: 10.1093/pcp/pcp035
Jaetzold, R., Schmidt, H., Hornetz, B., and Shisanya, C. A. (2006). Farm Management Handbook of Kenya. Natural Conditions and Farm Information Volume II, Part 2/B2, 2nd Edn. Nairobi: Ministry of Agriculture; GTZ.
Jefferies, R. A. (1993). Responses of potato genotypes to drought. I. Expansion of individual leaves and osmotic adjustment. Ann. Appl. Biol. 122, 93–104. doi: 10.1111/j.1744-7348.1993.tb04017.x
Karuku, G. N., Gachene, C. K. K., Karanja, N., Cornelisb, W., and Verplacke, H. (2014). Effect of different cover crop residue management practices on soil moisture content under a tomato crop (Lycopersicon esculentum). Trop. Subtrop. Agroecosyst. 17, 509–523. Available online at: https://www.redalyc.org/pdf/939/93935728005.pdf
Kibunja, C., Keziah, W., Ndungu-Magiroi, D. K., Wamae, T. J., Mwangi, J., Leonard, N., et al. (2018). Optimizing Fertilizer Use within the Context of Integrated Soil Fertility Management in Kenya. Available online at: https://agronomy.unl.edu/research/OFRA/OFRABookChapters/OFRA%20Chapter%207_Kenya_HR.pdf (accessed August 24, 2020).
Knight, C. T. G., and Kinrade, S. D. (2001). Chapter 4 A primer on the aqueous chemistry of silicon. Plant Sci. 57–84. doi: 10.1016/S0928-3420(01)80008-2
Koocheki, A., Mahallati, M. N., Solouki, H., and Karbor, S. (2016). Evaluation of radiation absorption and use efficiency in substitution intercropping of sesame (Sesamum indicum L.) and mung bean (Vigna Radiata L.). Adv. Plants Agric. Res. 3, 100–109. doi: 10.15406/apar.2016.03.00109
Krauss, A., and Marschner, H. (1984). Growth rate and carbohydrate metabolism of potato tubers exposed to high temperatures. Potato Res. 27, 297–303. doi: 10.1007/BF02357638
Li, X., Jiri, S., Haibin, S., Jianwen, Y., Zunyuan, P., and Xuewen, G. (2017). Spatial distribution of soil water, soil temperature, and plant roots in adrip-irrigated intercropping field with plastic mulch. Europ. J. Agron. 83, 47–56. doi: 10.1016/j.eja.2016.10.015
Lima, M. G. S., Lopes, N. F., Bacarin, M. A., and Mendes, C. R. (2004). Effect of salt stress on pigments and proline concentrations in leaves of rice. Bragantia 63, 63335–63340. doi: 10.1590/S0006-87052004000300003
Ma, B., Liu, Y., Liu, X., Ma, F., Wu, F., and Li, Z. (2015). Soil splash detachment and its spatial distribution under corn and soybean cover. Catena 127, 142–151. doi: 10.1016/j.catena.2014.11.009
Machiani, M. A., Javanmarda, A., Morshedloo, M. A., and Maggi, F. (2018). Evaluation of competition, essential oil quality and quantity of peppermint intercropped with soybean. Ind. Crops Prod. 111, 743–754. doi: 10.1016/j.indcrop.2017.11.052
Mead, R., and Willey, R. W. (1980). The concept of a land equivalent ratio and advantages in yields for intercropping. Exp. Agric. 16, 217–228. doi: 10.1017/S0014479700010978
Meehl, G. A., Stocker, T. F., Collins, W. D., Friedlingstein, P., Gaye, A. T., and Noda, A. (2007). “Global climate projections,” in Climate Change 2007: The Physical Science Basis, eds S. D. Solomon, M. Qin, Z. Manning, M. Chen, K. B. Marquis, M. Averyt, et al. (Cambridge: Cambridge University Press), 747–846.
Meunier, J. D., Doris, B., Muhammad, A., Clement, L., and Perrine, C. (2017). Effect of phytoliths for mitigating water stress in durum wheat. New Phytol. 215, 229–239. doi: 10.1111/nph.14554
Midega, C. A. O., Charles, J. W., Antony, M. H., John, A. P., and Zeyaur, R. K. (2017). Drought-tolerant desmodium species effectively suppress parasitic striga weed and improve cereal grain yields in western Kenya. J. Crop Prot. 98, 94–101. doi: 10.1016/j.cropro.2017.03.018
Mitani, N., and Ma, J. F. (2005). Uptake system of silicon in different plant species. J. Exp. Bot. 56, 1255–1261. doi: 10.1093/jxb/eri121
Monteith, J. L. (1994). Validity of the correlation between intercepted radiation and biomass. Agr. Forest Meteorol. 68, 213–220. doi: 10.1016/0168-1923(94)90037-X
Monteith, J. L., and Unsworth, M. (1990). Principles of Environmental Physics 2nd Edn. London: Edward. Arnold.
Moran, R., and Porath, D. (1980). Chlorophyll determination in intact tissues using N,N-Dimethylformamide. Plant Physiol. 65, 478–479. doi: 10.1104/pp.65.3.478
Moussa, H. R., and Abdel-Aziz, S. M. (2008). Comparative response of drought tolerant and drought sensitive maize genotypes to water stress. Aust. J. Crop Sci. 1, 31–36. Available online at: http://www.cropj.com/Helal%20Final%20version3_5_.pdf
Murphy, D., Sparling, G. P., and Fillery, I. R. P. (1998). Stratification of microbial biomass C and N and gross N mineralization with soil depth in two contrasting Western Australian Agricultural soils. Aust. J. Soil Res. 36, 45–56. doi: 10.1071/S97045
Mylavarapu, R. S., Sanchez, J. F., Nguyen, J. H., and Bartos, J. M. (2002). Evaluation of mehlich-1 and mehlich-3 extraction procedures for plant nutrients in acid mineral soils of Florida. Commun. Soil Sci. Plant 33, 807–820. doi: 10.1081/CSS-120003067
National Potato Council of Kenya (NPCK) (2017). Potato Variety Catalogue. Available online at: https://npck.org. (accessed August 19, 2020).
Nelson, D. W., and Sommers, L. E. (1996). “Carbon and organic matter,” in Methods of Soil Analysis, Part 3, Chemical Analysis, eds D. L. Sparks, A. L. Page, P. A. Helmke, R. H. Loeppert, P. N. Soltanpour, M. A. Tabatabai, et al. (Madison: SSSA; ASA), 961–1010.
Nyawade, O. S. (2015). Effect of potato (Solanum tuberosum L.) cropping systems on soil and nutrient losses through run-off in a Humic Nitisol (MSc. Thesis). University of Nairobi, Nairobi, Kenya.
Nyawade, S., and Gitari, H. (2020). “Unlocking legume intercropping technology in smallholder potato farming systems for climate change adaptation. Book of Abstracts,” in GEA International (Geo Eco-Eco Agro) Conference (Podgorica).
Nyawade, S. O., Gachene, C. K. K., Karanja, N. N., Gitari, H. I., Schulte-Geldermann, E., and Parker, M. (2019a). Controlling soil erosion in smallholder potato farming systems using legume intercrops. Geoderma Region. 17:e00225. doi: 10.1016/j.geodrs.2019.e00225
Nyawade, S. O., Karanja, N. N., Gachene, C. K. K., Gitari, H. I., Schulte-Geldermann, E., and Parker, M. (2019b). Intercropping optimizes soil temperature and increases crop water productivity and radiation use efficiency of rainfed potato. Am. J. Potato Res. 96, 457–471. doi: 10.1007/s12230-019-09737-4
Nyawade, S. O., Karanja, N. N., Gachene, C. K. K., Gitari, H. I., Schulte-Geldermann, E., and Parker, M. (2020). Optimizing soil nitrogen balance in a potato cropping system through legume intercropping. Nut. Cycling Agroecosys. 117, 43–59. doi: 10.1007/s10705-020-10054-0
Nyawade, S. O., Karanja, N. N., Gachene, C. K. K., Schulte-Geldermann, E., and Parker, M. (2018). Effect of potato hilling on soil temperature, soil moisture distribution and sediment yield on a sloping terrain. Soil Till. Res. 184, 24–36. doi: 10.1016/j.still.2018.06.008
Obidiegwu, J. E., Bryan, G. J., Jones, H. G., and Ankush, P. (2015). Coping with drought: stress and adaptive responses in potato and perspectives for improvement. Front. Plant Sci. 6:542. doi: 10.3389/fpls.2015.00542
Odo, P. E. (1991). Evaluation of short and tall sorghum varieties in mixture with cowpea in the Sudan savanna of Nigeria: land equivalent ratio, grain yield and system productivity index. Exp. Agric. 27, 435–441. doi: 10.1017/S0014479700019426
Pereira, L. S., Cordery, I., and Iacovides, I. (2012). Improved indicators of water use performance and productivity for sustainable water conservation and saving. Agric. Water Manage. 108, 39–51. doi: 10.1016/j.agwat.2011.08.022
Pilon, C., Rogério, P., Soratto, F. B., and Adalton, M. F. (2014). Foliar or soil applications of silicon alleviate water-deficit stress of potato plants. Agron. J. 106, 2325–2334. doi: 10.2134/agronj14.0176
Polgar, Z., Laszlo, G., Zsolt, V., and Istvan, W. (2017). “Manifestation of the abiotic stress tolerance of the new Hungarian potato varieties,” in Twentieth European Association for Potato Research (EAPR). Triennial Conference (Versailles).
Polyakov, V. O., and Lal, R. (2008). Soil organic matter and CO2 emission as affected by water erosion on field runoff plots. Geoderma 143, 216–222. doi: 10.1016/j.geoderma.2007.11.005
Quinton, J. N., Catt, J. A., and Hess, T. M. (2001). Selective removal of phosphorus from soil: is event size important? J. Environ. Qual. 30, 538–545. doi: 10.2134/jeq2001.302538x
R Core Team (2017). R: A Language and Environment for Statistical Computing. R Foundation for Statistical Computing, Vienna.
Radeni, G., and Caesar, K. (1986). Effects of soil temperature on the carbohydrate status in the potato plant. J. Agron. Crop Sci. 156, 217–224. doi: 10.1111/j.1439-037X.1986.tb00030.x
Rukundo, P., Ndacyayisenga, T., Ntizo, S., Kirimi, S., and Nshimiyima, J. C. (2019). Yield and yield components of CIP advanced potato clones under Rwandan agro-ecologies. J. Appl. Biosci. 136, 13909–13920. doi: 10.4314/jab.v136i1.7
Sapre, S. S., and Vakharia, D. N. (2016). Role of silicon under water deficit stress in wheat: (biochemical perspective): a review. Agric. Rev. 37, 109–116. doi: 10.18805/ar.v37i2.10736
Shah, M. M., Fischer, G., and van, V. H. (2008). Food Security and Sustainable Agriculture: The Challenges of Climate Change in sub-Saharan Africa. Commission on Sustainable Development (CSD), CSD-16 Review Session 5–16 May 2008 (United Nations, New York, NY).
Tubaña, B. S., and Heckman, J. R. (2015). “Silicon in soils and plants,” in Silicon and Plant Diseases, eds F. A. Rodrigues and L. E. Datnoff (Switzerland: Springer International Publishing), 7–51.
Wahla, I. H., Ahmad, R., Ehsanullah, A. A., and Jabbar, A. (2009). Competitive functions of components crops in some barley based intercropping systems. Int. J. Agric. Biol. 11, 69–72. Available online at: https://agris.fao.org/agris-search/search.do?recordID=PK2009000847
Keywords: climate change adaptation, crop water productivity, legume intercropping, silicon, smallholder potato farmers, soil conservation
Citation: Nyawade S, Gitari HI, Karanja NN, Gachene CKK, Schulte-Geldermann E, Sharma K and Parker ML (2020) Enhancing Climate Resilience of Rain-Fed Potato Through Legume Intercropping and Silicon Application. Front. Sustain. Food Syst. 4:566345. doi: 10.3389/fsufs.2020.566345
Received: 27 May 2020; Accepted: 28 September 2020;
Published: 06 November 2020.
Edited by:
Paulo Mazzafera, Campinas State University, BrazilReviewed by:
Amos Ngwira, Ministry of Agriculture, and Food Security, MalawiLeonard Rusinamhodzi, International Institute of Tropical Agriculture (IITA), Nigeria
Copyright © 2020 Nyawade, Gitari, Karanja, Gachene, Schulte-Geldermann, Sharma and Parker. This is an open-access article distributed under the terms of the Creative Commons Attribution License (CC BY). The use, distribution or reproduction in other forums is permitted, provided the original author(s) and the copyright owner(s) are credited and that the original publication in this journal is cited, in accordance with accepted academic practice. No use, distribution or reproduction is permitted which does not comply with these terms.
*Correspondence: Shadrack Nyawade, shadnyawade@gmail.com