Utilization of Food Processing By-products in Extrusion Processing: A Review
- 1Food Processing Laboratory, School of Food Science, College of Agricultural, Human, and Natural Resource Sciences, Washington State University, Pullman, WA, United States
- 2Faculty of Chemical and Food Engineering, Institute of Technology of Cambodia, Phnom Penh, Cambodia
The processing of agricultural products into value-added food products yields numerous by-products or waste streams such as pomace (fruit and vegetable processing), hull/bran (grain milling), meal/cake (oil extraction), bagasse (sugar processing), brewer's spent grain (brewing), cottonseed meal (cotton processing), among others. In the past, significant work in exploring the possibility of the utilization of these by-products has been performed. Most by-products are highly nutritious and can be excellent low-cost sources of dietary fiber, proteins, and bioactive compounds such as polyphenols, antioxidants, and vitamins. The amount of energy utilized for the disposal of these materials is far less than the energy required for the purification of these materials for valorization. Thus, in many cases, these materials go to waste or landfill. Studies have been conducted to incorporate the by-products into different foods in order to promote their utilization and tackle their environmental impacts. Extrusion processing can be an excellent avenue for the utilization of these by-products in foods. Extrusion is a widely used thermo-mechanical process due to its versatility, flexibility, high production rate, low cost, and energy efficiency. Extruded products such as direct-expanded products, breakfast cereals, and pasta have been developed by researchers using agricultural by-products. The different by-products have a wide range of characteristics in terms of chemical composition and functional properties, affecting the final products in extrusion processing. For the practical applications of these by-products in extrusion, it is crucial to understand their impacts on the qualities of raw material blends and extruded products. This review summarizes the general differences in the properties of food by-products from different sources (proximate compositions, physicochemical properties, and functional properties) and how these properties and the extrusion processing conditions influence the product characteristics. The discussion of the by-product properties and their impacts on the extrudates and their nutritional profile can be useful for food manufacturers and researchers to expand their applications. The gaps in the literature have been highlighted for further research and better utilization of by-products with extrusion processing.
Introduction
In a world with an increasing population, the sensible and resource-saving use of raw materials is becoming critical. The rising demand for food involves the production of more waste and by-products and thus raises the question to address this concern (King et al., 2017; Krishnan et al., 2020). The agricultural and food processing sector generates a large quantity of waste annually (Ravindran and Jaiswal, 2016). The accumulation of these products from the industries creates a negative impact on the environment due to the issues associated with waste disposal and dumping. The waste produced by the food industries is collected over the different phases throughout the supply chain, including the stages of harvesting, grading, sorting, and processing (Raak et al., 2017; Marić et al., 2018; Teigiserova et al., 2019). There is a need to valorize these agricultural wastes into food products as they serve as a cheap source of dietary fiber, protein, and bioactive compounds such as phenolic compounds, antioxidants, minerals, and vitamins (Peschel et al., 2006; Santana-Méridas et al., 2012; Ravindran and Jaiswal, 2016; Masli et al., 2018b; Rivera-González et al., 2019; Wang et al., 2019). The utilization of by-products could lower the negative environmental impacts and improve the nutritional profile of products for human consumption (Lai et al., 2017; Majerska et al., 2019). However, most often, agricultural by-products are considered low-value assets, and in the post-harvest stage, about 14% of food loss and waste have occurred worldwide (FAO, 2019).
By-products are versatile and arise from a variety of products and processes. For instance, a great amount of pomace is generated during juice processing; bran, hull, husk, or pods are obtained during grain milling; and a variety of oil cakes is produced in the oil industry (Norajit et al., 2011; Santana-Méridas et al., 2012; Sharma et al., 2015; Marić et al., 2018; Majerska et al., 2019). Besides their origin, the by-products greatly vary in composition and functional properties and therefore play a significant role in the determination of the characteristics of fortified products (Ačkar et al., 2018; Marić et al., 2018; Wang et al., 2019; Xie et al., 2021). Significant research has been reported on strategies to convert the food waste into more value-added products, including incorporating them into different foods, fractionation into various components, and extraction of compounds from the waste (Ravindran and Jaiswal, 2016; Lai et al., 2017; Majerska et al., 2019; Teigiserova et al., 2019).
Agricultural wastes can be incorporated into value-added products manufactured using extrusion processing as extrusion is a versatile technique that can handle a variety of raw materials and is an energy-efficient process (Ačkar et al., 2018; Gu et al., 2019). This is because extrusion combines the simultaneous application of unit operations, such as mixing, kneading, cooking, and forming under high temperature, high shear, and short-time processing technique (Stojceska et al., 2008a; Singkhornart et al., 2013; Gu et al., 2020). Cereal flours are the most common raw materials used for extrusion processing (Faraj et al., 2004). An advantage of extrusion processing is the minimization of nutritional losses as compared to conventional heat processing techniques due to the short processing times (Sevatson and Huber, 2000). Additionally, the high-temperature processing of extrusion destroys the antinutritional compounds such as phytates and trypsin inhibitors and enzymes such as lipases and lipoxidases (Rathod and Annapure, 2016; Gu et al., 2020; Liu et al., 2020).
This review aims to discuss the status of the utilization of agricultural by-products from different sources in extrusion processing. An attempt has been made to compare the chemical composition of food by-products and their functionality as it helps to predict their performance in the extrusion processing. The effects of by-product inclusions and extrusion conditions on physicochemical properties and nutritional profile of final products (direct-expanded products, third-generation products, and pasta) are reviewed thoroughly. Finally, research gaps are highlighted for the better valorization of agricultural by-products utilizing extrusion processing.
Brief Overview of Extrusion Processing
Extrusion is a process in which material is continuously moved through a barrel encasing a single or a set of screws and forced through a shaped opening (die) by combining diverse operations in a short time (Bordoloi and Ganguly, 2014; Kaisangsri et al., 2016; Masli et al., 2018a). The number of extruded food applications, including pasta, snacks, texturized vegetable protein, and others, has increased tremendously over the years. The versatility of extrusion processing makes it an ideal candidate for the utilization of the by-products.
During extrusion, the raw material is subjected to shear forces, high temperatures, and a high-pressure condition (Kazemzadeh, 2012; Gu et al., 2017). As a result, the solid material undergoes physicochemical changes into a melt state (Alam et al., 2016; Kaisangsri et al., 2016; Gu et al., 2017). As this viscous material leaves the system and equilibrates with the atmosphere, it further experiences changes in its physicochemical properties before it arrives at the final stable state.
Extrusion processing has been explained as multiple input and multiple output system (MIMO) (Eerikäinen et al., 1994), where the parameters are divided into extrusion processing parameters (input), extrusion system parameters (dependent), and product parameters (output) (Gu et al., 2017). The extrusion input parameters include feed moisture, feed rate, screw speed, temperature, and die dimensions, and the system parameters include back pressure, torque, and specific mechanical energy (SME). Expansion index, solubility properties, and density of the extrudates are considered as output parameters. Figure 1 shows the dependencies of these parameters.
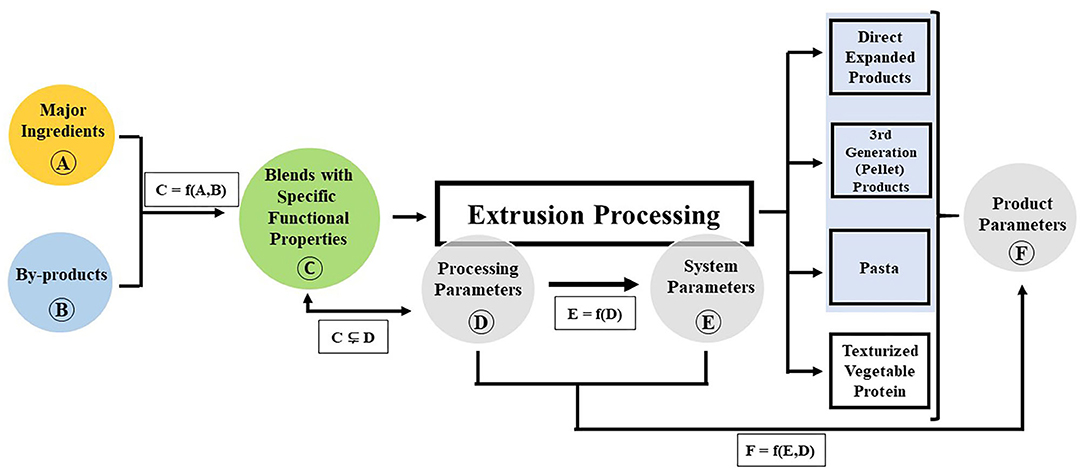
Figure 1. Dependences of factors during extrusion processing. C = f(A,B): C is a function of A and B; C ⊊ D: C is a subset of D; E = f(D): E is a function of D; F = f(E,D): F is a function of E and D. The paper reviews the effect of by-products on direct expanded products, 3rd generation products, and pasta products; texturized vegetable protein products are not covered but may offer a great opportunity to do so in the future.
The versatility of the extrusion process comes from the enormous flexibility that the extruder provides in terms of the options in the processing parameters. The setup of the equipment, including the screw configuration as well as the die design, and the selection of the raw material, screw speed, temperature, among others, have an impact on the final quality of the extrudate produced. The screw profile can be modified to impart different degrees of shear to manufacture different products (Ek and Ganjyal, 2020). Products such as direct-expanded snacks and texturized vegetable proteins need high shear and are typically manufactured using a twin-screw extrusion system. In comparison, pasta manufacturing requires lower shear rates and typically utilize a single-screw system. System parameters can be modified indirectly by altering the extrusion processing variables (Gu et al., 2017). They significantly affect the molecular transformation of the material and therefore, impact the final texture of the extrudates (Filli et al., 2012; Singha et al., 2017). The SME determines the energy required to extrude materials; it influences the degree of breakdown/transformation of starch during extrusion and is calculated using the screw speed, torque, and flow rate values (Godavarti and Karwe, 1997; Filli et al., 2012; Fang et al., 2014; Kowalski et al., 2018). Torque values are correlated with the viscosity of the melt (Chevanan et al., 2010; Masli et al., 2018a; Mazlan et al., 2019). Backpressure (BP) is the pressure opposing the flow of the material toward the die. BP is also positively correlated with the viscosity of the melt and further shows a negative correlation with the screw speed due to the shear-thinning behavior of the melt (Kaisangsri et al., 2016; Wang S. et al., 2017).
The overall interactions between the extrusion processing parameters and system parameters determine the final product characteristics of extrudates. Therefore, a thorough understanding of the input variables, their correlations, and their impact on extrudate properties is essential for designing extruded products with consistent quality. Additionally, it becomes important to understand the physicochemical characteristics of the by-products and their components, and the various changes that the individual components undergo during extrusion, to effectively incorporate by-products into the extruded products. Along with this, it is also critical to understand how the ingredient components interact with each other as they are subjected to extrusion conditions.
Starch is the main component of numerous extruded products. Under the extrusion conditions of high shear and high-temperature, starch is transformed into a viscoelastic material by the combination of mechanical breakdown, gelatinization, and melting (Lai and Kokini, 1991; Ye et al., 2018). The ability of starch to expand when exiting the system through the die is important for many extrudates (Kowalski et al., 2015; Aluwi et al., 2016). The pressure drop enables the vaporization of the moisture in the extrudate resulting in an expanded cell structure (Gu et al., 2017). On the contrary, proteins, and lipids generally hinder the full expansion of the starch. Proteins lose their native structure, denature, interact with starch, and affect the water distribution in the material (Moraru and Kokini, 2003; Allen et al., 2007; Day and Swanson, 2013; Offiah et al., 2019). Lipids act as lubricants by reducing the mechanical energy input and the friction between the material and the screws as well as between the material particles (Guy, 2001; Moraru and Kokini, 2003; Fasina et al., 2006). Due to this lubricating effect, the temperature in the barrel decreases and lowers the degree of starch gelatinization. Besides that, lipid concentrations above a critical level result in the coating of starch granules and hinder starch from absorbing water for gelatinization (Moraru and Kokini, 2003; Ilo et al., 2008).
One of the major components of agricultural by-products are dietary fibers, which can be categorized into soluble and insoluble fibers. Shear forces cause the fiber to degrade into compounds with reduced molecular weight, and thus, the fraction of insoluble fibers often decreases in favor of the soluble fraction (Alam et al., 2016). At low concentrations, insoluble dietary fibers (IDF) generally distribute evenly throughout the starch matrix, strengthen the starch matrix, and may result in an increased expansion (Ganjyal and Hanna, 2004; Kaisangsri et al., 2016; Masli et al., 2018a; Wang et al., 2019). However, at higher fiber levels, uniform distribution is not achieved and fiber particles may disrupt cell walls causing a decrease in expansion. In addition, IDF may compete with starch for water, hindering the starch to fully gelatinize, increasing the melt viscosity, and raising the resistance against cell formation (Yanniotis et al., 2007; Robin et al., 2012; Masli et al., 2018a). Soluble dietary fibers (SDF), on the other hand, do not have a negative effect on the expansion; in fact, they may lead to a slight increase in the expansion (Brennan et al., 2008; Robin et al., 2012; Wang et al., 2019).
Sources and General Characteristics of By-Products
The characteristics of by-products are not only dependent on the source of raw materials but also on their processing. Knowing the chemical composition of by-products is essential to understand how they may behave together with other ingredients in the extrusion process. This understanding is useful for setting the extrusion process parameters for different mixtures.
Table 1 provides the chemical composition of selected by-products from the literature reports. It is important to note that they cannot be compared due to the differences in the way the materials are processed, along with the differences in the raw materials. In general, fruit and vegetable by-products contain a high amount of dietary fiber, total sugars, and are low in fat and protein. On the contrary, by-products from grain milling contain a high amount of dietary fiber, protein, and in some cases, a high amount of fat. Oil cake, the by-products from oil processing, should be defatted and contain a high amount of protein, except olive pomace. The following subsections describe briefly different by-products and their chemical compositions.
Fruit and Vegetable Processing
Fruit and vegetable processing generate by-products in the form of pomace, seed, and pulp, peel, or stem. Fruit pomace is the by-product of juice processing, and it may contain a high sugar content. Fruit pomace and peel from various fruits, including apple, citrus, grape, berries, mango, pineapple, and others, have been studied in extrusion applications. Vegetable by-products include carrot pomace, tomato, cauliflower trimming, asparagus residues, and others.
Fruit and vegetable by-products generally contain high total fiber content and bioactive compounds, including polyphenols, antioxidant compounds, and vitamins. They are a low-cost source of bioactive compounds and vitamins and could be incorporated into food products to offer nutraceutical functionality. For example, carrot and tomato pomace contained a high content of fiber and carotenes (Altan et al., 2008a; Kaisangsri et al., 2016). In terms of dietary fiber, fruit and vegetable by-products may have different contents of insoluble and soluble fiber, which can affect their degree of inclusion in the direct-expanded products (Brennan et al., 2008; Wang et al., 2019). For instance, the total dietary fiber, insoluble fiber, and soluble fiber contents of citrus pomace are 62.6–68.3%, 54.0–62.0%, and 4.6–10.3%, respectively (Figuerola et al., 2005). Wang et al. (2019) reported that the total dietary fiber of pomace from berries (blueberry, cranberry, and grape) ranged from 45.9 to 59.3%, of which 2.4–3.4% was soluble fiber; meanwhile, apple pomace had 6.1% of soluble fiber and 53.1% of total dietary fiber.
Grain Milling
The by-products of grain milling include bran, hull, husk, or pods. The most used components in the extrusion process are bran and hulls (from wheat, corn oat, rice, rye, and others). As shown in Table 1, bran contains high levels of insoluble fiber, a significant amount of protein, and in some cases, a high amount of fat (Grigelmo-Miguel and Martin-Belloso, 1999; Zhang et al., 2011; Wang P. et al., 2017; Aktas-Akyildiz et al., 2020). In addition to high dietary fiber, β-glucan, and antioxidant compounds are of interest from the wheat bran (Fleischman et al., 2016). On the other hand, rice bran, which is also a good source of dietary fiber and micronutrients, contains a high content of fat that needs to be defatted, and it also contains lipase enzyme, which needs to be stabilized by heat treatment before further utilization (Wang P. et al., 2017). The rye bran is mainly utilized for its arabinoxylan and β-glucan content (Nikinmaa et al., 2017).
Oil Processing
The major by-product of oil processing is oil cake or pomace such as olive pomace, soybean meal, hemp cake, sesame oil cake, and others. Due to the high oil content, most of these oil cakes or pomace should be defatted before using in food applications. As shown in Table 1, defatted soybean meal, defatted hemp cake, semi-defatted sesame oil cake, and cottonseed meal have a high protein content (34.5–55.3 %) (Nascimento et al., 2012; Reyes-Jáquez et al., 2012; Korkerd et al., 2015; Antun et al., 2017). Meanwhile, olive pomace has 13.4% protein and 42.7% fiber (Ying et al., 2017).
Others
Other potential by-products for the inclusion in extruded products include brewer's spent grain from the brewing industry, sugarcane bagasse from sugar processing, and whey protein from cheese processing. Stojceska et al. (2008b) reported that brewer's spent grain contained 16% of total dietary fiber, 4.8% of protein, and 2.1% of fat. Sugarcane bagasse contains approximately 90% of total dietary fiber (Masli et al., 2018b).
The by-products can vary significantly in their chemical composition. It is also important to realize that some of these by-products require special treatments and processing for their further utilization due to their chemical composition. The chemical composition and the structure of these by-products have a tremendous influence on their functional characteristics, and then their applications in extrusion processing. In addition, by-products inherently have different colors. Therefore, high inclusion levels of these by-products may result in color changes in the extrudates, which can be desirable or undesirable by consumers.
Functional Properties of Agricultural By-Products
The functional characteristics of food ingredients typically include hydration properties (water absorption and solubility), rheological properties (under different conditions of temperature and shear), emulsification properties, melting properties, and others (Ying et al., 2017; Masli et al., 2018b; Wang et al., 2019; Demuth et al., 2020). These characteristics significantly influence how each ingredient interacts with the processes and other ingredients in a food product (Table 2). Both the composition and the functional properties dictate the transformation of ingredients during extrusion processing and the final characteristics of the extruded products.
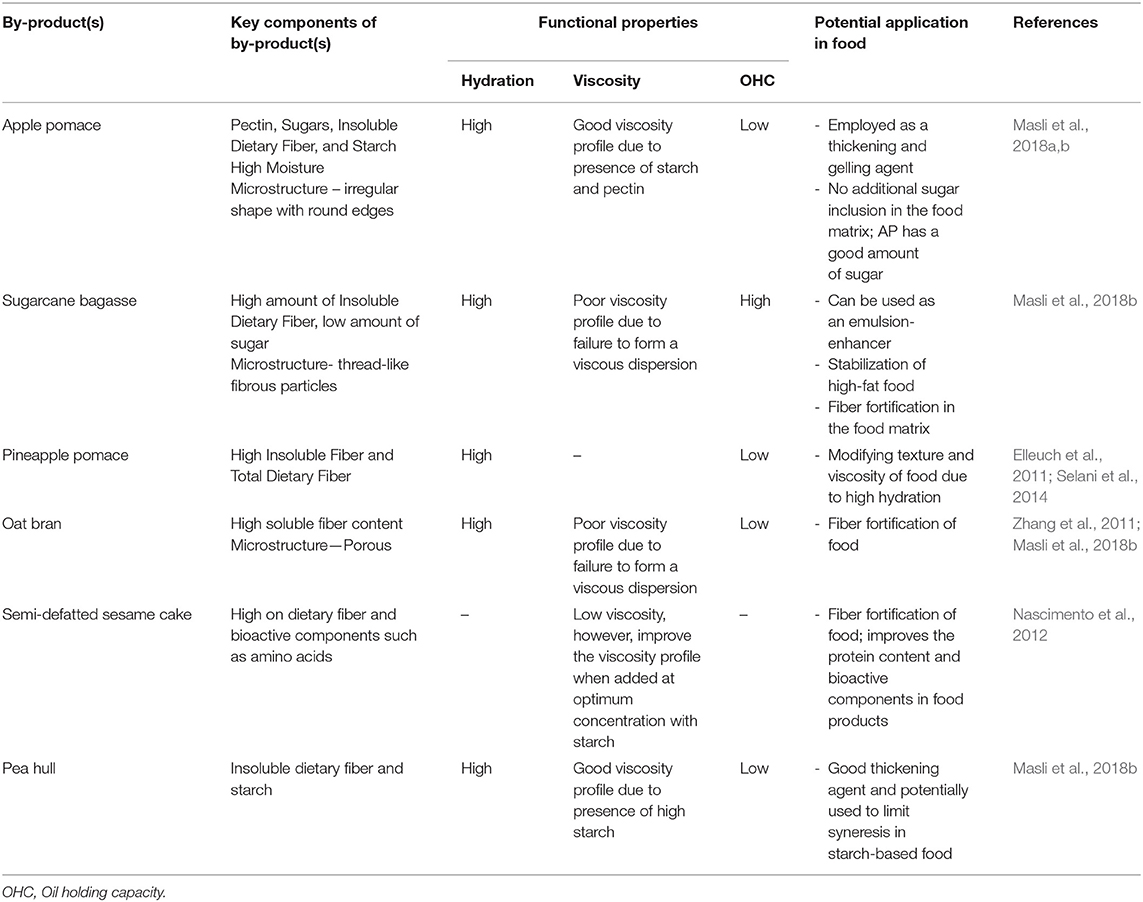
Table 2. Functional properties and potential applications of selected by-products in food processing.
Hydration Properties
It is essential to understand the hydration properties of the by-products since they determine the final quality and stability of extruded products. The hydration properties of by-products are associated with the chemical and structural composition of the fiber (Robertson and Eastwood, 1981). Most by-products are known to have good hydration properties; hence, they tend to compete with starch for absorption of water. The interference thereby affects the viscosity of the starch melt and impacts the final product characteristics.
The water holding capacity of by-products depends on the source of by-products due to the variability in the chemical and structural composition of the by-products (Elleuch et al., 2011). The soluble fractions of fiber, such as pectin and gum, participate in increasing the viscosity; hence, they act as thickeners and texture improving agents in food processing (Masli et al., 2018b). Insoluble fractions of fiber, such as cellulose, hemicellulose, and lignin, have a high tendency to increase the water and oil binding properties. Hence, these materials can be used in high-fat foods as stabilizers and offer additional functionality by improving the shelf life of food (Elleuch et al., 2011; Masli et al., 2018b).
Substantial work has been done in the past on analyzing and defining the hydration properties of pomaces from various sources (Lopez et al., 1996; Elleuch et al., 2011; Selani et al., 2014; Kurek et al., 2015; Wang S. et al., 2017; Masli et al., 2018a,b). The hydration properties are influenced by the chemical composition and structure of the by-products. The amorphous nature of fiber is responsible for the high-water binding capacity and behaves as a hydrocolloid. Water retention capacity (WRC) of cranberry pomace is reported to be 5.60 ± 0.08 g/g due to the high amount of lignin of 31.3 ± 2.2 g/100 g (Wang et al., 2019); this might be due to the amorphous nature of lignin and its tendency to absorb water. Similarly, apple pomace had a WRC and swelling capacity (SC) of 5.60 ± 0.08 g/g and 5.66 ± 0.53 ml/g, respectively, and this is attributed to the high soluble fiber content and particle size of apple pomace. Selani et al. (2014) reported that pineapple pomace had approximately 45.22 ± 3.62 % insoluble fiber component, which is responsible for the maximum water absorption capacity of 5.32 ± 0.67 g water/g sample in comparison to lemon peel (1.74–1.85 g water/g sample) and apple pomace (1.62–1.87 g water/g sample). These results suggest the potential application of pineapple pomace in products to improve viscosity (Elleuch et al., 2011).
In a study on wheat bran extrusion, the water-binding capacity (WBC) for the non-extruded sample was 361.9%. The WBC decreased with an increase in die temperature. Due to the modification of soluble fiber during extrusion, the amount of soluble dietary fiber was observed to increase from 2.3% (d.b.) to 3.8% (d.b.) during extrusion at 100 rpm and 105°C. In the case of wheat bran, it might have some amount of starch, which could contribute to water absorption, thus affecting the WBC values (Masatcioglu et al., 2014).
The SC was higher for extruded oat bran compared to the untreated oat bran due to the higher SDF content of extruded oat bran, as the solubility of fiber is reported to increase during extrusion (Zhang et al., 2011). The solvent retention capacity was lower for unextruded oat bran; however, the extruded oat bran had a higher solvent retention capacity. With the increase in the concentration of hemp, the WAI and WSI increased (Norajit et al., 2011), as the fiber content in the formulation increased.
The SC and WBC are the two properties determined by the amount of soluble fiber fractions in the food matrix. Therefore, it is essential to understand the chemical composition and physical characteristics such as particle size (Alam et al., 2014; Kallu et al., 2017; Wang S. et al., 2017), density (Altan et al., 2008a; Wang et al., 2019), and porosity (Altan et al., 2008a; O'Shea et al., 2014), as these attributes define the behavior of by-products during hydration.
Oil Holding Capacity
The OHC of by-products is an essential functional property to understand the emulsifying properties and flavor retention in food products such as meat and baked products (Cadavid et al., 2014). Additionally, the information on OHC is useful in post-extrusion processing steps, especially during seasoning the extruded products with oil.
The OHC was high for sugarcane bagasse and potato due to the high insoluble fiber content as well as the microstructure of the pomace, which was fibrous and porous. The insoluble fiber content of sugarcane was 98.97 ± 0.97% and 54.97 ± 1.10% for potato peels (Masli et al., 2018b). The OHC for pineapple pomace was 2.01 ± 0.23 g oil/g sample (Selani et al., 2014), which is higher than peach (1.02–1.11 g oil/g sample) and lower than pomegranate (5.9 g oil/g sample) as reported in an earlier study by Viuda-Martos et al. (2012). The comparatively higher OHC for pineapple could be attributed to the high insoluble fiber component of 44.44 ± 3.60% d.b.
A study on jaboticaba fruit and its by-products-peels and pomace reported the OHC to be in the range of 2.79–2.98 g oil/g sample. However, the values for OHC were not significantly different between the whole fruit, peels, and pomace as the insoluble fiber content was similar in the material, and additionally, there was not a major difference in the particle sizes of the sample. Additionally, the authors also mentioned that jaboticaba pomace has an anthocyanin content of 5.73 ± 0.71 mg C3G/g d.w, and it could be incorporated in snacks.
OHC of by-products depends on the source, particle size, composition, and microstructure of the by-product. The OHC of by-products is a function of porosity rather than the tendency to soak oil (Nelson, 2001). Therefore, the by-products with porous structures have a higher tendency to bind oil (Petravić-Tominac et al., 2011).
Viscosity
The pasting properties help to understand the functionality of the by-products in terms of thickening and gelling characteristics and texture-improving properties. Viscosity increases with the increase in the level of dietary fiber and decreases with the increase in temperature (Elleuch et al., 2011). The viscosity profile of the by-products depends upon the level of by-product inclusion, particle size, structure, chemical composition, functional properties, and extrusion processing parameters. The insoluble fraction of fiber, such as cellulose and hemicellulose, show high hydration capacities; however, they fail to form viscous solutions and therefore, cannot be used as a thickening or gelling agent in food processing (Lecumberri et al., 2007; O'Shea et al., 2015).
Masli et al. (2018b) reported that apple and potato by-products showed high viscosity due to the high starch and pectin content. Pea hulls had a peak viscosity of 723 ± 0.9 mPa.s due to high starch content in the range of 15.99–19.86%. In the case of bran, the viscosity was poor due to the low starch content and the high insoluble fiber content of 91.04 ± 0.64 % d.b. Another study by O'Shea et al. (2015) reported that apple pomace had a higher viscosity compared to orange pomace. Though orange pomace has a higher pectin content than apple pomace, the presence of low degree methylated pectin led to a lower viscosity.
The inclusion of by-products at high levels in the food matrix dilutes the amount of starch due to the competition between by-products and starch for water absorption. This interaction leads to poor viscosity of the mixture. For instance, the inclusion of olive oil pomace, when incorporated at higher levels, interferes with the swelling and transformation of starch and disrupts the starch matrix (Ying et al., 2017). This leads to lower viscosity of the final product. Therefore, olive oil pomace cannot alone impart the functionality of improving textures in food products.
Zhang et al. (2011) reported that extrusion improved the functionality of soluble dietary fiber of oat bran. The apparent viscosity was lower for the untreated oat bran. The viscosity of oat bran improved due to molecular changes under the conditions studied. Demuth et al. (2020) reported that there were significant changes in the structure and functionality of Arabinoxylan (AX), depending on the parameters of extrusion processing.
Antun et al. (2017) used pressed hemp cake at different concentrations (0, 5, and 10%) with two different moisture contents, 20 and 25%, and extruded at four different temperatures of 150, 160, 165, and 180°C. The peak viscosity of unextruded samples decreased with an increase in hemp concentration; however, upon extrusion, the peak viscosity (PV) values further decreased. This reduction is due to the damage of starch and gelatinization of starch during extrusion. In the case of corn grits, the PV was 129 BU at 150°C, and 10% hemp extrudates had PV of 129 BU at 180°C. This signifies the role of proteins and fiber in hemp that protects starch from damage due to the formation of starch-protein-fiber complexes. Additionally, the protective function of hemp decreases the cold paste viscosity of starch, indicating better starch stability in suspension. The cold paste viscosity was observed to decrease after extrusion, as the cold paste viscosity is an indicator of the viscosity at the end of the cooling phase.
Nascimento et al. (2012) reported the application of semi-defatted sesame cake (SDSC) in the extrusion of corn grits. The viscosity profile of SDSC had a viscosity drop during the cooling stage, then increased at 25°C, and showed a further reduction in viscosity. The change in the viscosity profile of SDSC can be attributed to the denaturation of native proteins (35 ± 0.3 g/100 g), resulting in molecular rearrangement and thereby increasing the free volume of polypeptide chains. The dietary fiber content of SDSC was 22.7 ± 0 g/100 g, and for corn grits, it was 0.7 ± 0.0 g/100 g. Due to the high dietary fiber content, SDSC could additionally impart functionality in the form of swelling capacity and hydration properties; however, the authors did not assess these functional properties of the raw SDSC.
Based on the source and chemical composition of the by-products, their functionalities differ. Therefore, an approach to categorize the by-products based on their functionalities can be a valuable tool for the food processors to incorporate these materials into the food matrices.
Effect of Extrusion Processing on Direct-Expanded Products
Direct-expanded products, including cereals and snacks, usually have a crispy texture. The major ingredient used in direct-expanded products is usually cereal flour or starch that contains (>70%) of starch (Moraru and Kokini, 2003). The moisture content used for direct expanded products is usually in the range of 14–20% (wet basis), the melt temperature at the die usually ranges from 120 to 160°C (Chinnaswamy, 1993; Ek et al., 2020).
A significant amount of work has been reported to incorporate different by-products into the direct-expanded products such as apple pomace, carrot pomace (Kaisangsri et al., 2016), pomaces from berries (Wang et al., 2019), pineapple pomace (Selani et al., 2014), mango peel (Mazlan et al., 2019), passion fruit shell (da Silva Alves et al., 2018), wheat bran (Robin et al., 2011a; Fleischman et al., 2016), rye bran (Alam et al., 2016), oat fiber (Sayanjali et al., 2017), rice bran (Wang P. et al., 2017), olive pomace (Ying et al., 2017), and brewer's spent grain (Stojceska et al., 2008b). The following sections discuss how by-product inclusions affect the properties of final extruded products. Table 3 provides the general effects of by-products on the final products.
Expansion and Density
The most common way to express expansion is the expansion ratio (ER), which is the ratio of the diameter of an extrudate and the diameter of the die. Most of the studies on by-products in extrusion processing showed that as the by-product content increased, the expansion ratio decreased, and the density of the extrudates increased (Selani et al., 2014; Kaisangsri et al., 2016; da Silva Alves et al., 2018). The porosity and cell size of the extrudate cross-section reduce with increasing by-product content, especially for the by-products containing high amount of insoluble fiber (Yanniotis et al., 2007; Robin et al., 2011a; Kaisangsri et al., 2016; Masli et al., 2018a; Mazlan et al., 2019; Wang et al., 2019). Depending on the source and particle size of by-products, the inclusion levels of 5–10% can either maintain or increase the expansion of extrudates with more uniform and smaller cell sizes. A study on carrot pomace inclusions in cornstarch extrudates found that the addition of 5 g/100 g carrot pomace had a higher expansion ratio (3.80) compared to the extrudate without the pomace (3.62) at the moisture content of 15%. The expansion decreased significantly at 10 and 15 g/100 g of carrot pomace. In another study, the expansion ratio of extrudates with 10.5% of pineapple pomace was not significantly different from the control at the same extrusion conditions (Selani et al., 2014). This result supported other findings that by-products with high fiber content can increase or maintain the expansion ratio up to 10% inclusions at specific extrusion conditions (Kaisangsri et al., 2016; Kallu et al., 2017; Wang S. et al., 2017; Masli et al., 2018a).
Small particle size (<125 μm) of the by-products aids in the better expansion of extrudates compared to larger particle sizes at the same level of inclusions (Alam et al., 2016; Kallu et al., 2017; Wang et al., 2019). A smaller particle size (~28 μm) of rye bran increased the expansion and crispiness of rye-flour extrudates compared to the larger particle size bran (Alam et al., 2016). With smaller particle size, extrudates with the levels of inclusion of 15 and 30% had no significant differences in expansion compared to control (Alam et al., 2016). This retention of expansion at a high inclusion level of bran could be attributed to the high starch content (38.4–44.8%) in rye bran.
Fleischman et al. (2016) showed that the expansion of extrudates with purple wheat bran was the highest, followed by white and red wheat bran. The purple wheat bran contained the highest starch content and lower fiber content relative to others. Wang et al. (2019) reported that the apple pomace exhibited the highest expansion, followed by cranberry, blueberry, and grape, respectively. The authors explained that the chemical composition of these pomace, including total dietary fiber (TDF), soluble dietary fiber (SDF), insoluble dietary fiber (IDF), and sugar content, affected the expansion significantly at studied conditions. High SDF, high sugar content, and low crystallinity index could be the reason for the highest expansion for apple pomace, among others. Another study with grape pomace also explained that high sugar content in grape pomace could be the reason for the increase in expansion ratio of extrudate with 5% of grape pomace, compared to the control (Bibi et al., 2017). In another study on apple pomace addition to regular and waxy cornstarch showed that extrudates with 15% inclusion of apple pomace had similar or even higher ER than the control in some conditions (Masli et al., 2018a). This was attributed to the characteristics of apple pomace containing high content of SDF (12%), high sugar content (35%), and irregular round shape microstructure. In contrast, the extrudates with sugarcane bagasse had significantly lower ER compared to the control extrudate, which was attributed to the high IDF content and fibrous-like microstructure of sugar cane bagasse (Masli et al., 2018a). SDF could interact with water and change the viscosity profile of starch melt, which could still maintain the continuous phase of starch melt and be favorable for the expansion of extrudates (Brennan et al., 2008; Xie et al., 2021). Unlike SDF, IDF could disrupt the continuous starch melt so that the melt could not be strong enough to hold the cells during expansion and thus decrease expansion (Robin et al., 2011b; Masli et al., 2018a). In addition, a low level of sugar could support the expansion because sugar can lower the glass transition temperature of the starch melt and thus change the viscoelastic properties of starch melt (Carvalho and Mitchell, 2000). The interactions of sugar, SDF, and IDF of the by-products and their synergic effects on the expansion of direct-expanded products need further investigation.
The inclusion of broccoli in corn-based extrudates exhibited higher expansion compared to the inclusion of olive paste (Bisharat et al., 2013). The presence of high lipids in olive paste was concluded as the reason for this effect. Insoluble fibers in the by-products are one of the main reasons for reduced expansion. Meanwhile, the presence of lipids in some by-products could hinder the expansion further.
In addition, the extrusion process conditions could help to improve the expansion of extrudates with by-product inclusion, even though in most cases, the improvement is not significant compared to control. Mazlan et al. (2019) reported that there were combined effects of mango peel level, feed moisture content, temperature, and screw speed on the expansion of corn-grit extrudates. The expansion of extrudates with 8% inclusion of mango peel increased from 1.76 to 2.70 when the temperature and screw speed increased from 100 to 150°C, and 76–100 rpm, respectively (Mazlan et al., 2019). Barley-tomato pomace extrudates had a decrease in sectional expansion as the tomato pomace and die temperature increased; however, screw speed did not affect the expansion of extrudates significantly (Altan et al., 2008a).
Another way to improve the performance of by-products in the direct-expanded products is pre-treatments, including fermentation, or surface modifications of by-products. Rye bran treated with fermentation maintained the ER of rye flour-based extrudates up to 40% of the inclusion level (Nikinmaa et al., 2017). The authors explained that the increase in soluble fiber and dextran could be the reason for maintaining the ER. Brennan et al. (2008) reported that extrudates with soluble fibers had higher expansion than insoluble fiber.
Water Absorption Index and Water Solubility Index
Water Absorption Index (WAI) and Water Solubility Index (WSI) are the important qualities related to the bowl-life of breakfast cereals and the mouthfeel of direct-expanded snacks. Generally, long bowl-life or being crispy longer in milk is preferred for breakfast cereals, so low WAI and low WSI are desirable for these products (Kowalski et al., 2015). For baby food or snacks, high WSI is preferable because the products can easily melt in the mouth (Kowalski et al., 2015). WSI generally increases with the increase in expansion ratio (Kowalski et al., 2015). On the contrary, both WAI and WSI values may depend on the expansion ratio and the initial water absorption characteristics of raw materials, especially when by-products with high fiber contents are added.
The increased inclusion level of by-products generally yields extrudates with increased WAI and decreased WSI. However, these two properties are significantly influenced by the combined effects of raw materials, the expansion of the extrudates, and the processing conditions. WAI values were reported to increase with the increase of grape pomace (Bibi et al., 2017), carrot pomace (Kaisangsri et al., 2016), and cauliflower trimming (Stojceska et al., 2008a). The authors attributed these results to the water absorption capability of fiber present in the by-products. In the case of the passion fruit shell, WAI was reduced when 10% of passion fruit shell was added, but WAI was higher than the control in some conditions at 15% of passion fruit shell (da Silva Alves et al., 2018). On the contrary, WAI of barley-tomato pomace extrudates decreased with the increased tomato pomace (Altan et al., 2008a). Interestingly, the WAI of extrudates enriched by pineapple was not significantly affected by the pineapple content (Selani et al., 2014). At feed moisture of 15 g/100 g, WAI of carrot pomace enriched extrudates increased with the carrot pomace levels. At high feed moisture (30 g/100 g), the carrot pomace inclusion did not significantly impact the WAI of the corn starch extrudates (Kaisangsri et al., 2016).
The overall WSI values of extrudates decrease with increasing by-product inclusions (Selani et al., 2014; Kaisangsri et al., 2016). However, the WSI of grape-pomace enriched extrudates increased with grape pomace at the low moisture content (16%), while WSI values decreased with pomace at the high moisture content (24%) (Bibi et al., 2017). It could be attributed to the degradation of starch and the breakdown of grape pomace into the more soluble fiber at a high energy input of extrusion due to the low moisture content in this case. Meanwhile, the WSI of extrudates increased with increasing screw speed, increasing tomato pomace levels, and decreasing temperature (Altan et al., 2008a).
Due to the combined effects of by-products and processing conditions, using specific mechanical energy input to anchor the effects of by-products inclusion on WSI could be useful. A study of wheat bran incorporated into wheat flour showed that WSI of extrudates positively correlated to SME for wheat flour and low wheat bran inclusion (12.6%), while WSI had a weak positive correlation to SME for high wheat bran inclusion (22.4%) (Robin et al., 2011a). It could be attributed to the more rigid nature of the wheat bran or fiber and the decrease of starch content.
Hardness
The extrudate hardness has been observed to increase with the decrease of the expansion ratio. The increased level of by-products generally increases the hardness of the extrudates because their expansion decreases. For example, the hardness of corn-mango peel extrudates increased as the mango peel content exceeded 8% (Mazlan et al., 2019). Similar observations were reported in the studies of grape pomace, tomato pomace, and broccoli trimming (Altan et al., 2008a,b; Bisharat et al., 2013; Bibi et al., 2017). On the contrary, the amount of pineapple pomace added did not affect the hardness of extrudates (Selani et al., 2014).
Nutritional Properties
Adding by-products into raw materials obviously increases the nutritional content of the blends. However, the fate of different compounds in the final product depends upon the interactions of all the ingredients in the mixtures and the processing conditions. The compounds of interest are also different depending on the by-products, for instance, dietary fiber and β-carotene for carrot pomace, dietary fiber and lycopene for tomato peel, and total phenolic compounds and dietary fiber for fruit pomaces.
A study on the effect of extrusion processing on the antioxidant activity of apple pomace showed that the polyphenols of extruded products retained 80–97% compared to the raw mixtures (Leyva-Corral et al., 2016). The high retention was attributed to the combined effect of feed moisture content, shear stress, and temperature, which degrade the compounds or cause their transformation to easily extractable forms (Leyva-Corral et al., 2016). Total phenolic compounds of grape-pomace enriched extrudates retained ~75 at 5% inclusion, and there was no significant loss at 10% inclusion of grape pomace before and after extrusion (Bibi et al., 2017). The total antioxidant activity of extrudates was retained to approximately 90% compared to raw mixtures. This retention was higher compared to the baking of grape seed flour, and it could be attributed to the short residence time (cooking time) in extrusion processing (Bibi et al., 2017). Similarly, another study found that extrusion cooking almost doubled the total phenolic compounds of the extrudates enriched with cauliflower inclusion of up to 20% (Stojceska et al., 2008a). Total antioxidant capacity also increased after extrusion for up to 10% of cauliflower inclusion (Stojceska et al., 2008a). The increase could be the consequence of high temperature, water stress, which could induce the production of phenolic compounds.
Interestingly, Kaisangsri et al. (2016) reported that at a low level (5 g/100 g) of carrot pomace, the retention of β-carotene was higher (74%) compared to the 30 g/100 g of carrot pomace (35%), after the extrusion processing. At a low level of pomace, the β-carotene may be protected by the starch matrix, which makes β-carotene less susceptible to degradation.
The fiber content of the extrudates decreased because of the conversion of insoluble fiber to soluble fiber (Rzedzicki et al., 2004; Sayanjali et al., 2017; Demuth et al., 2020). Extrusion processing conditions such as feed moisture content and temperature affected the TDF, IDF, and SDF fraction. Increasing moisture content from 14 to 23% increased the TDF and IDF because low moisture content led to more intensive change or degradation of TDF and IDF while resistant starch was formed (Rzedzicki et al., 2004).
Effects of By-Products on Physicochemical Properties of 3RD Generation Extruded (Pellet) Products
The third-generation extruded products (TGE) or pellets are processed using extrusion under conditions of high moisture (30–35%), low shear, and low temperatures of <100°C in the forming zone of the extruder (Aguilar-Palazuelos et al., 2006). After extrusion, the pellets are cooled and dried to 10–11% moisture, and the TGE becomes stable and reaches the glassy state (Osman et al., 2000; Sevatson and Huber, 2000; Aguilar-Palazuelos et al., 2006). TGE does not expand on extrusion and is termed as a non-expanded product. Generally, the starch content for TGE is >60% (Sevatson and Huber, 2000) in contrast to direct-expanded products that utilize starch above 80% concentration (Gu et al., 2017). The TGE produced by extrusion is highly dense and is firm compared to direct-expanded products and is processed further using a microwave, baking, or frying to form expanded products before consumption (Sevatson and Huber, 2000). On the industrial scale, TGE is expanded by hot oil, hot air puffing, or microwaving. They are further seasoned and flavored and sold as ready-to-eat snacks that are shelf-stable.
The functional properties of starches impart the final texture characteristics to the pellets, such as firmness, expansion, and crunchiness. Additionally, proteins, dietary fiber, vegetable oil, shortenings, flavorings, and colorants may be added to improve the nutritional and textural attributes of the products (Aguilar-Palazuelos et al., 2006). The inclusion of vegetable oil or shortenings is employed as processing aids as they help to reduce the stickiness and control expansion of the products (Sevatson and Huber, 2000).
The physicochemical properties of TGE include the expansion index (EI), bulk density, and the penetration force to break the extrudates (Table 3). The production of pellets with the inclusion of by-products from the agricultural industries is limited. However, there are a few reports on pomace inclusion in pellets in the literature, which are briefly discussed below.
Expansion Index (EI)
The critical factors responsible for the TGE expansion include time, moisture content, and temperature of cooking. The high EI of extrudates is obtained by partial gelatinization of starch, which allows entrapment of water in the extrudates, which can be utilized during microwave expansion (Tovar-Jiménez et al., 2015).
Lower EI values can be attributed to the starch dextrinization due to the shear and temperature during extrusion. The EI is reported to increase under conditions of high temperature and low moisture, as lower moisture imparts higher shear rates and improves viscoelastic properties of the melt by the degradation of starch. Ruiz-Armenta et al. (2018) reported maximum EI of whole-grain yellow corn and Naranjita fruit bagasse pellets at a barrel temperature of >128°C and moisture content of <24%. These pellets were expanded in a microwave oven for 20 s post extrusion. It should also be noted that an optimal gelatinization percentage of about 50% is ideal for higher EI. Lower gelatinization of starch would lead to reduced absorption of water by starch, which would result in less expanded structures. However, in this study, the higher level of Naranjita bagasse led to a decrease in the EI, as fiber interferes during the formation of the air cells and collapses the microstructure of the extrudates.
Tovar-Jiménez et al. (2015) reported on the inclusion of orange vesicle flour in starch extrusion used in the microwave for the expansion of the pellets. The optimum microwave time to obtain pellets with maximum EI was 28 s, and extrusion processing conditions such as low moisture (22%) and high temperatures (143°C) are responsible for high EI. High shear and high temperature may lead to starch dextrinization (Sarifudin and Assiry, 2014), which is also responsible for lowering of EI. Generally, the inclusion of pomace reduces the EI of pellets due to the interference of pomaces in the cooking of starch during extrusion.
Penetration Force (PF)
PF is the maximum force required for initial breakage of the extrudates, and it measures the resistance of the extrudates to breakage (Yagci and Gögüş, 2009; Tovar-Jiménez et al., 2015; Navarro-Cortez et al., 2018). PF is negatively correlated to EI and positively correlated to bulk density (BD) (Pérez et al., 2008; Ruiz-Armenta et al., 2018). High PF values can be associated with high BD of extrudates, wherein the extrudates with high PF and BD result in hard texture. Tovar-Jiménez et al. (2015) reported that as the moisture content decreased, the penetration force decreased in their study on orange vesicle flour. In the same study, high PF values were attributed to high temperature and high moisture conditions, as higher moisture content is linked to the hardness of the extrudates.
Additionally, the increase in fiber content in the formulation with lower barrel temperature is also responsible for higher PF values. As with higher fiber, the starch content is diluted, thereby leading to the collapse of the air bubbles, which further reduces the EI and increases the PF (Navarro-Cortez et al., 2018). The increase in fiber inclusion is also reported to increase in hardness, as it interferes with the formation of nucleation and formation of air bubbles (Stojceska et al., 2008a). The increase in hardness is another factor for the increase in PF and BD of the extrudates (Navarro-Cortez et al., 2018).
Inclusion of By-Products In Pasta Products
Pasta is usually produced by a cold-extrusion process using a single screw extruder (New Food, 2014; Tiwari and Jha, 2017). The screw typically exhibits a constant diameter with deep flights and a uniform pitch (New Food, 2014). The temperature typically remains below 50°C, and the moisture content ranges depending on the pasta shape, in most cases, from 28 to 32% (Wójtowicz and Mościcki, 2011). As a result, extrusion primarily serves as a compounding tool rather than a cooking tool. Precooked pasta, on the contrary, is produced at higher temperatures ranging from 70 to 100°C with moisture content up to 36% (Wójtowicz and Mościcki, 2011). The raw material most used to produce pasta is durum wheat semolina (Wójtowicz and Mościcki, 2011; Alam et al., 2016). However, other flours, such as those from different wheat varieties, rice, maize, or corn, can also be used. Eggs, vegetables, natural emulsifiers, protein, and enriching supplements, among others, may be added as supplements. Common parameters that help to determine the quality of pasta are optimal cooking time, cooking loss, hardness or firmness, swelling index, and water absorption. High water absorption, low cooking loss, and high firmness/hardness are indicators of high cooking quality (Bruneel et al., 2010).
Many studies have been conducted to incorporate agricultural by-products into pasta products. The inclusion of by-products has a significant effect on the gluten and starch matrix. Most components in the by-products, especially fiber, disrupt the matrix and thus enhance the penetration of water into the pasta product (Kaur et al., 2012; Lončarić et al., 2014; Padalino et al., 2018; Simonato et al., 2019). In addition, the by-product components compete with the major components, mainly starch, for water, and hence, inhibit the formation of a strong starch and gluten network (Ajila et al., 2010; Lončarić et al., 2014). High protein concentrations, on the other hand, result in the formation of a strong protein network and prevent water penetration (Sozer and Kaya, 2003; Nilusha et al., 2019). The strength of the gluten and starch matrix highly affects the parameters that are used for the determination of the quality of pasta. The following sections will discuss how the inclusion of agricultural by-products influences the optimal cooking time, cooking loss, firmness or hardness, and water absorption as well as swelling index. An overview of the general effect by-products has on pasta is provided in Table 3.
Optimal Cooking Time
The optimal cooking time (OCT) is defined as the time that is required for the white core inside the pasta to disappear (Lončarić et al., 2014; Sant'Anna et al., 2014; Piwińska et al., 2015; Simonato et al., 2019). Due to the disruption of the gluten matrix and the resulting enhanced penetration of water inside the pasta, the inclusion of by-products, in general, leads to a decrease in OCT. The reported OCTs for durum wheat semolina pasta without any incorporation of by-product vary greatly throughout the literature. For instance, Zarzycki et al. (2020) reported an OCT of 5 min for ribbon pasta, whereas Simonato et al. (2019) indicated that the OCT for spaghetti was > 13 min. The wide range for the control pasta may be a result of diverse shapes as well as different preparation conditions during extrusion processing; however, exact conditions are rarely reported. Therefore, it is difficult to compare the changes of OCTs, that are induced by the incorporation of diverse by-products from different studies, and to draw conclusions about the reasons for the different behaviors of the various by-products.
Sant'Anna et al. (2014) and Crizel et al. (2015) reported that the incorporation of grape marc powder and orange dietary fiber into wheat flour fettuccine up to a level of 7.5% did not change the OCT significantly. These results may be explained by the low concentrations of by-products that do not disrupt the gluten matrix continuously. A study by Lončarić et al. (2014), who studied the effect of apple (Granny Smith) peel powder on durum semolina pasta, strengthens this assumption. Fettuccine that was enriched with 10% apple by-product had an OCT of 7.3 min, like the control. However, an increase above 10% level led to an OCT decrease. The OCT for the pasta with 15% of the by-product was more than 1 min shorter (6.2 min). Studies on olive pomace, on the contrary, showed that even concentrations up to 5% of by-products could result in an OCT drop. Simonato et al. (2019) reported that 5% olive pomace in durum wheat semolina spaghetti lowered the OCT from 13.3 to 12.3 min.
The OCT drop because of the incorporation of different cereal brans or oat fiber compared to the studies on apple and olive pomace is comparatively low when considering the relatively high inclusion levels. The fortification of durum semolina pasta with 25% of wheat, rice, oat, and barley bran resulted in a decrease in OCT by 14 s, 16 s, 21 s, and 16 s, respectively, relative to the control (Kaur et al., 2012). This lesser effect on the OCT is most likely a result of the different compositions of the by-products. Wheat, rice, barley, and oat bran contained a lower amount of total dietary fiber compared to olive pomace (Kaur et al., 2012; Simonato et al., 2019). Additionally, the different brans showed relatively high carbohydrate contents that may have partly supported the gluten matrix formation rather than its disruption. However, Piwińska et al. (2015) studied the impact of oat fiber on fusilli pasta. Although this by-product contained a great amount of fiber, a relatively small decline in OCT of 24 s was reported for an inclusion level of 20%. These results suggest that other factors, such as particle size, in addition to the fiber content in the by-products, also play a role in the disruption of the gluten matrix.
Although most studies state a lower OCT for pasta that is fortified with any agricultural by-product, exceptions can be seen. Zarzycki et al. (2020) reported a significant increase of the OCT between the control and pasta that contained 5% flaxseed cake. A further increase in flaxseed concentration up to 23%, however, did not change the OCT significantly. This may be due to the continuous disruption of the starch and gluten matrix at an incorporation level of 5% by-product.
The above-mentioned studies have shown that the incorporation of by-products up to a certain fortification level generally does not affect the OCT significantly. Increasing the by-product concentration, however, leads with a few exceptions to an unfavorable decrease in the OCT. The level at which a significant decrease in the OCT can be seen varies greatly throughout the different by-products and is likely dependent on the by-product composition.
Cooking Loss
During cooking, pasta loses solid matter into the cooking water. The amount of solid matter in the cooking water, reported as the percentage of the weight of the starting material, is defined as the cooking loss (CL) (Ajila et al., 2010; Lončarić et al., 2014; Gull et al., 2015; Padalino et al., 2018; Simonato et al., 2019). Good-quality pasta should not exceed a CL of 12% (Sant'Anna et al., 2014). The weaker matrix of fortified pasta, in general, leads to an increase of this parameter as the starch retention is diminished and hence leaches into the cooking water more easily. Besides that, substances from the by-products itself may also dissolve in water and hence increase the CL (Kaur et al., 2012; Simonato et al., 2019).
Studies on mango peel powder (Ajila et al., 2010), grape marc powder (Sant'Anna et al., 2014), carrot pomace (Gull et al., 2015), and orange dietary fiber (Crizel et al., 2015) revealed that concentrations of 2.0 to 2.5% of such by-products do not change the CL significantly. These findings suggest that such levels may not weaken the starch and gluten matrix to a great extent. However, by-product inclusions of 4 or 5% showed a significant increase in CL not only compared to the control sample but also compared to the treatments with the lower incorporation level. The substitution of 5% durum semolina with mango peel powder or orange dietary fiber led to a rise of CL by about 40% compared to the control pasta (Ajila et al., 2010; Crizel et al., 2015). The inclusion of 4% carrot pomace increased the solid loss by more than 60% (Gull et al., 2015). Simonato et al. (2019) showed an even greater rise in CL when including 5% olive pomace into spaghetti; the CL increased by more than 95%, from 4.13% (control) to 8.07% (5% by-product). Again, the composition of the by-products may be one reason for the different behaviors. For instance, according to Gull et al. (2015), carrot pomace contained significantly less fiber (11.66 ± 0.57 g/100 g) than what was reported for olive pomace (48 ± 1.7 g/100 g DM) by Simonato et al. (2019). However, the high concentration of total dietary fiber in mango peel powder (51.2 ± 1.08 %) reveals that the amount of fiber is not the only factor that affects the CL. When raising the level of grape marc powder, carrot pomace, orange dietary fiber, and olive pomace to 7.5% or 10%, no further significant change in CL was observed (Sant'Anna et al., 2014; Crizel et al., 2015; Gull et al., 2015; Simonato et al., 2019). Lončarić et al. (2014), on the contrary, reported a higher CL change (9.9% vs. 11.5%) when the apple peel powder level in fettuccine was increased from 10% to 15% compared to the increase of by-product from 0% to 10% for which the CL increased by only 0.5%.
A completely different pattern was observed when flaxseed cake or oat fiber was added to semolina flour. Zarzycki et al. (2020) stated that the CL dropped with an increase in flaxseed cake from 5.67% for the control pasta to 4.44% when 23% of by-product was added. Similarly, the inclusion of oat fiber, as reported by Piwińska et al. (2015), led to a lower CL in fusilli. The control showed a CL of 5.15% that declined to 3.45% when 16% of durum semolina was substituted by oat by-product. However, raising the level of oat fiber to 20% resulted in a significant increase to 4.31%. The decreases of CL may be a result of suspended insoluble fiber fractions in the gelatinized starch and the interactions between these two components and gluten that prevent the starch from leaching into the cooking water (Piwińska et al., 2015).
The selected studies have shown that the fortification of pasta with different by-products may have a significant impact on the CL. In most cases, the CL increases when by-products are incorporated into pasta. Similar to the OCT, low concentrations of by-products usually do not affect the CL extensively.
Firmness or Hardness
Many studies report values for firmness or hardness of pasta. However, the definitions of the terms are not used consistently. Some surveys define firmness as the (maximum) force that is required to cut the pasta (Ajila et al., 2010; Wójtowicz and Mościcki, 2011; Zarzycki et al., 2020), whereas other studies use both terms to describe the mean maximum force during compression to a predetermined degree (Lončarić et al., 2014; Gull et al., 2015; Padalino et al., 2018; Simonato et al., 2019). It is furthermore important to note that the conditions at which the different analyses were undertaken vary greatly. Besides that, the extrusion conditions, which are, as mentioned earlier, rarely given, may also influence the firmness/hardness as reported by Wójtowicz and Mościcki (2011). They showed that the hardness of pasta increased with screw speed. The comparison of different studies reveals that the incorporation of by-products can lead to an increase of firmness/hardness as well as to a decrease, and thus a generalized statement about a positive or negative effect of by-products on the firmness/hardness of pasta cannot be made.
Inclusion of pomaces such as carrot pomace, apple peel powder, and flaxseed cake reduce the firmness/hardness of pasta (Lončarić et al., 2014; Gull et al., 2018; Zarzycki et al., 2020). This lower firmness/hardness can be attributed to by-product components that prevent the development of a strong gluten network. The fortification of durum semolina Ziti-cut pasta with 2% carrot pomace decreased the firmness significantly from 5.94 N to 2.88 N (Gull et al., 2015). Raising the level of by-product in steps of 2% up to 10%, however, did not result in a further significant change. The incorporation of apple peel powder by Lončarić et al. (2014) was conducted at by-product levels of 10% and 15%. A significant change in hardness was observed between the control pasta (1,328 ± 201 g) and the pasta that contained 10% apple peel powder (1,109 ± 125 g). No further significant change was seen when 15% of by-product was added. Due to the lack of data at lower inclusion levels, it is not apparent which amount of apple by-product was required to lead to a significant change of firmness and if firmness increases with the inclusion level up to 10% or if it stagnates at a lower level such as for carrot pomace. Zarzycki et al. (2020), who used the pasta cutting force as an indirect indicator for hardness, recorded the first significant decrease of hardness between 9 and 13% of flaxseed cake incorporation. However, in this study, the further increase of by-product level up to 23% resulted in a continuous decrease of hardness. Due to a lack of information on the by-product characteristics, such as composition or particle size, an assumption on the reasons for the different behaviors cannot be made. Padalino et al. (2018) studied the fortification of pasta with tomato and olive by-product, suggested smaller by-product particles, or the additional inclusion of transglutaminase for a beneficial influence of the pasta's characteristics by minimizing the decrease of hardness.
Completely different results regarding firmness/hardness of pasta when fortified with by-products were reported by Ajila et al. (2010) and Simonato et al. (2019). They analyzed the effect of mango peel powder and olive pomace, respectively, and reported an increase in firmness when such by-products were added. The gradual addition of mango peel powder, from 2.5% up to 7.5%, caused a constant increase of force required to shear the macaroni by about 10 gf for each inclusion level from 44.0 ± 1.70 gf for the control to 73.46 ± 1.22 gf for the pasta that contained 7.5% by-product (Ajila et al., 2010). The firmness reported by Simonato et al. (2019) increased significantly from 132 ± 0.69 N for the control spaghetti to 150.53 ± 0.57 N when 5% olive pomace was incorporated and 163.18 ± 0.18 N when 10% of durum semolina was substituted by the by-product. These results suggest that by-products that are high in fiber may also play a beneficial role in the firmness of pasta products due to the resistance of the dietary fiber particles (Ajila et al., 2010; Simonato et al., 2019).
The firmness/hardness of by-product fortified pasta generally differs from the control pasta. However, it is not possible to give a general correlation between the inclusion level and the change in firmness/hardness as some studies showed a greater difference in firmness/hardness at lower inclusion levels, whereas others reported that higher levels led to bigger changes.
Water Absorption and Swelling Index
Water absorption describes the amount of water that is held by the pasta after cooking, while the swelling index (SI) is an indicator of absorbed water that is utilized for the gelatinization of starch and hydration of proteins (Gull et al., 2018; Padalino et al., 2018). In a disrupted gluten matrix, water is absorbed more easily. A strong protein network, however, prevents water penetration and reduces starch swelling (Sozer and Kaya, 2003; Nilusha et al., 2019). Furthermore, hydrophilic components may compete with starch for water and preferentially absorb available water (Fuad and Prabhasankar, 2010). Keeping the above factors under consideration, it becomes comprehensible that different by-products have contrasting effects on the characteristics of pasta. However, both parameters, the water absorption, as well as SI, mostly increase with an increase in by-product incorporation levels.
Lončarić et al. (2014), Gull et al. (2015), Piwińska et al. (2015), and Simonato et al. (2019) reported an increase in water absorption when pasta was fortified with apple peel powder, different cereal brans (wheat, rice, barley, and oat), high-fiber oat powder, and olive pomace, respectively. Apple peel powder increased the mass of water that was absorbed by 100 g cooked pasta from 128.7 g for the control to 131.1 g and 132.3 g when 10 or 15% apple peel powder was incorporated (Lončarić et al., 2014). The substitution of durum semolina with different cereal brans resulted in greater changes in water absorption for the diverse incorporation levels. The control showed a water absorption of about 118%, which increased depending on the bran origin continuously to about 123–126% for the samples with 10% by-product, and to about 128–130% for the samples with 25% by-product (Kaur et al., 2012). The enrichment of pasta with high-fiber oat powder by Piwińska et al. (2015) showed similar results up to a level of 12% by-product. Raising the level further to 16 and 20% led to a slight, but not significant, decrease in water absorption when pasta was dried conventionally; however, vacuum dried pasta that contained 16 or 20% oat powder took up much less water. These declines may be attributed to the shorter OCT at such by-product level inclusions. An even larger rise in water absorption was reported by Simonato et al. (2019), who studied the effect of olive pomace in spaghetti. The water absorption increased from 178.00% for the control to 199.43% for spaghetti, which contained 10% by-product. The authors further described a significant rise in SI for the mentioned samples. The SI increased from 1.65 ± 0.02 g water per g dry pasta (control) to 1.92 ± 0.09 g water per g dry pasta (10% olive pomace). Both parameters, the water absorption, as well as the SI, are contradictory to what was reported by Padalino et al. (2018), who worked on the incorporation of olive paste flour into pasta. A slight, but not significant, decline in water absorption could be seen between the control (141%) and the spaghetti that was enriched with 10% olive paste flour (138%). However, the SI decreased from 1.86 for the control to 1.75 g water per g of dry pasta for 10% olive by-product fortified pasta. These conflicting outcomes may be explained by different processing conditions but could also be a result of different compositions of the two olive by-products.
Most of the studies have shown that fortified pasta holds more water than the corresponding control. However, the different by-products affect the water absorption as well as the SI dependent on their composition and other physical characteristics.
Conclusions and Prospects
Many efforts have been undertaken to incorporate food by-products into extruded food products. The diverse agricultural by-products and their functionalities play a significant role in the effect they have on the final extruded product. Generally, at the by-product inclusion levels of 5–10%, the extruded products retain good textural properties. Products enriched with by-products provide more nutritional value, including an increase in dietary and bioactive compounds; however, their color and texture are modified.
Despite the extensive research reported so far, there are still gaps in understanding the role of by-products and their functionality to improve the texture and sensory attributes of the extruded products, especially in pasta, 3rd generation (or pellet type) products, and texturized protein products.
The functionality of by-products is a critical factor that influences the final properties of extruded products. A categorization of by-products based on functionality could be a valuable resource for the food processors to manufacture products that are nutritious with better texture and palatability.
The lack of understanding of the molecular interactions between different food components during extrusion makes it hard to evaluate the impact of by-products that have different compositions thoroughly. As the by-products constitute a variety of components (dietary fiber, antioxidants, minerals, vitamins, and acid), a detailed understanding of each component and their interactions with major food ingredients (carbohydrate, protein, and lipid) is important to produce snacks with desirable flavors and texture. Fourier Transformed Infrared (FTIR), Nuclear Magnetic Resonance (NMR), and X-ray diffraction (XRD) could explain these molecular interactions. The understanding of the interactions of the components could be informative to alter the final properties of extrudates.
For the pellet and pasta products, the reports on system parameters of the extrusion processing are scarce, and they should be addressed. Uncovering the impact of parameters on their properties would be informative to increase the usage of by-products. Moreover, the incorporation of by-products in other extruded products could open new avenues for processors in the future. For instance, the by-product inclusion in texturized vegetable protein (TVP) would allow them to make these products rich in fiber and bioactive compounds. The by-products depending on their functionality can also find applications in the high moisture meat analog (HMMA) products.
There is limited information on the sensory analysis of the by-product inclusion in extruded products. The sensory evaluation of these products should be addressed because consumer acceptability is a drive for the commercialization of these value-added products. In addition, studies with a holistic approach concerning the costs and benefits of using a specific by-product should be evaluated if the benefits outweigh the costs. Commercial, environmental, and nutritional aspects should also be addressed for their effective utilization.
Author Contributions
DD, JR, PE, and B-JG prepared the outline of the paper, conducted the literature review, drafted the manuscript, and prepared figures and tables. GG conceptualized the study and edited the manuscript. All authors reviewed and approved the final version of the manuscript.
Funding
This work was supported by the USDA National Institute of Food and Agriculture, AFRI project proposal number 2017-07669, awarded to GG. This work was also supported in part by the USDA National Institute of Food and Agriculture, Hatch project Accession number 1016366.
Conflict of Interest
The authors declare that the research was conducted in the absence of any commercial or financial relationships that could be construed as a potential conflict of interest.
References
Ačkar, A., Jozinović, A., Babić, J., Miličević, B., Panak Balentić, J., and Šubarić, D. (2018). Resolving the problem of poor expansion in corn extrudates enriched with food industry by-products. Innov. Food Sci. Emerg. Technol. 47, 517–524. doi: 10.1016/j.ifset.2018.05.004
Aguilar-Palazuelos, E., Zazueta-Morales, J. D. J., and Martinez-Bustos, F. (2006). Preparation of high-quality protein-based extruded pellets expanded by microwave oven. Cereal Chem. 83, 363–369. doi: 10.1094/CC-83-0363
Ajila, C. M., Aalami, M., Leelavathi, K., and Rao, U. J. S. P. (2010). Mango peel powder : a potential source of antioxidant and dietary fiber in macaroni preparations. Innov. Food Sci. Emerg. Technol. 11, 219–224. doi: 10.1016/j.ifset.2009.10.004
Aktas-Akyildiz, E., Masatcioglu, M. T., and Köksel, H. (2020). Effect of extrusion treatment on enzymatic hydrolysis of wheat bran. J. Cereal Sci. 93:102941. doi: 10.1016/j.jcs.2020.102941
Alam, M. S., Kaur, J., Khaira, H., and Gupta, K. (2016). Extrusion and extruded products: changes in quality attributes as affected by extrusion process parameters: a review. Crit. Rev. Food Sci. Nutr. 56, 445–473. doi: 10.1080/10408398.2013.779568
Alam, S. A., Järvinen, J., Kirjoranta, S., Jouppila, K., Poutanen, K., and Sozer, N. (2014). Influence of particle size reduction on structural and mechanical properties of extruded rye bran. Food Bioprocess Technol. 7, 2121–2133. doi: 10.1007/s11947-013-1225-2
Allen, K. E., Carpenter, C. E., and Walsh, M. K. (2007). Influence of protein level and starch type on an extrusion-expanded whey product. Int. J. Food Sci. Technol. 42, 953–960. doi: 10.1111/j.1365-2621.2006.01316.x
Altan, A., McCarthy, K. L., and Maskan, M. (2008a). Evaluation of snack foods from barley-tomato pomace blends by extrusion processing. J. Food Eng. 84, 231–242. doi: 10.1016/j.jfoodeng.2007.05.014
Altan, A., McCarthy, K. L., and Maskan, M. (2008b). Twin-screw extrusion of barley-grape pomace blends: extrudate characteristics and determination of optimum processing conditions. J. Food Eng. 89, 24–32. doi: 10.1016/j.jfoodeng.2008.03.025
Aluwi, N. A., Gu, B-.J., Dhumal, G. S., Medina-Meza, I. G., Murphy, K. M., and Ganjyal, G. M. (2016). Impacts of scarification and degermination on the expansion characteristics of select quinoa varieties during extrusion processing. J. Food Sci. 81, E2939–E2949. doi: 10.1111/1750-3841.13512
Antun, J., Urdica, A., Stela, J., Jurislav, B., Jelena Panak, B., Marija, B., et al. (2017). Optimisation of extrusion variables for the production of corn snack products enriched with defatted hemp cake. Czech J. Food Sci. 35, 507–516. doi: 10.17221/83/2017-CJFS
Bibi, S., Kowalski, J., Ganjyal, M., and Zhu, J. (2017). Stability and functionality of grape pomace used as a nutritive additive. J. Food Process. Technol. 8:6. doi: 10.4172/2157-7110.1000680
Bisharat, G. I., Oikonomopoulou, V. P., Panagiotou, N. M., Krokida, M. K., and Maroulis, Z. B. (2013). Effect of extrusion conditions on the structural properties of corn extrudates enriched with dehydrated vegetables. Food Res. Int. 53, 1–14. doi: 10.1016/j.foodres.2013.03.043
Bordoloi, R., and Ganguly, S. (2014). Extrusion technique in food processing and a review on its various technological parameters. Indian J. Sci. Res. Technol. 2, 1–3.
Brennan, M. A., Monro, J. A., and Brennan, C. S. (2008). Effect of inclusion of soluble and insoluble fibres into extruded breakfast cereal products made with reverse screw configuration. Int. J. Food Sci. Technol. 43, 2278–2288. doi: 10.1111/j.1365-2621.2008.01867.x
Bruneel, C., Pareyt, B., Brijs, K., and Delcour, J. A. (2010). The impact of the protein network on the pasting and cooking properties of dry pasta products. Food Chem. 120, 371–378. doi: 10.1016/j.foodchem.2009.09.069
Cadavid, E. L. A., Molina, D. A. R., and Valenzuela, J. R. C. (2014). Chemical, physicochemical and functional characteristics of dietary fiber obtained from asparagus by-products (asparagus officinalis l.) Rev. Fac. Nac. Agron. Medellin. 68, 7533–7544. doi: 10.15446/rfnam.v68n1.47842
Carvalho, C. W. P., and Mitchell, J. R. (2000). Effect of sugar on the extrusion of maize grits and wheat flour. Int. J. Food Sci. Technol. 35, 569–576. doi: 10.1111/j.1365-2621.2000.00454.x
Chevanan, N., Kurt, R., and Kasiviswanathan, M. (2010). Effects of processing conditions on single screw extrusion of feed ingredients containing DDGS. Food Bioprocess. Technol. 3, 111–120. doi: 10.1007/s11947-008-0065-y
Chinnaswamy, R. (1993). Basis of cereal starch expansion. Carbohydr. Polym. 21, 157–167. doi: 10.1016/0144-8617(93)90012-S
Crizel, T. D. M., Rios, A. D. O., Cruz, R., Thys, S., and Flôres, S. H. (2015). Effects of orange by-product fiber incorporation on the functional and technological properties of pasta. Food Sci. Technol. 35, 546–551. doi: 10.1590/1678-457X.6719
da Silva Alves, P. L., Berrios, J. D. J., Pan, J., and Ascheri, J. L. R. (2018). Passion fruit shell flour and rice blends processed into fiber-rich expanded extrudates. CyTA J. Food 16, 901–908. doi: 10.1080/19476337.2018.1503618
Day, L., and Swanson, B. G. (2013). Functionality of protein-fortified extrudates. Compr. Rev. Food Sci. Food Saf. 12, 546–564. doi: 10.1111/1541-4337.12023
Demuth, T., Betschart, J., and Nyström, L. (2020). Structural modifications to water-soluble wheat bran arabinoxylan through milling and extrusion. Carbohydr. Polym. 240:116328. doi: 10.1016/j.carbpol.2020.116328
Eerikäinen, T., Zhu, Y.-H., and Linko, P. (1994). Neural networks in extrusion process identification and control. Food Control 5, 111–119. doi: 10.1016/0956-7135(94)90096-5
Ek, P., and Ganjyal, G. M. (2020). “Chapter 1: Basics of extrusion processing,” in Extrusion Cooking: Cereals and Grains Processing, ed G. M. Ganjyal (Cambridge, MA: Woodhead Publishing), 1–28. doi: 10.1016/B978-0-12-815360-4.00001-8
Ek, P., Kowalski, R. J., and Ganjyal, G. M. (2020). “Chapter 4: Raw material behaviors in extrusion processing I (Carbohydrates),” in Extrusion Cooking: Cereals and Grains Processing, ed G. M. Ganjyal (Cambridge, MA: Woodhead Publishing), 119–152. doi: 10.1016/B978-0-12-815360-4.00004-3
Elleuch, M., Bedigian, D., Roiseux, O., Besbes, S., Blecker, C., and Attia, H. (2011). Dietary fibre and fibre-rich by-products of food processing: characterisation, technological functionality and commercial applications: a review. Food Chem. 124, 411–421. doi: 10.1016/j.foodchem.2010.06.077
Fang, Y., Zhang, B., and Wei, Y. (2014). Effects of the specific mechanical energy on the physicochemical properties of texturized soy protein during high-moisture extrusion cooking. J. Food Eng. 121, 32–38. doi: 10.1016/j.jfoodeng.2013.08.002
FAO (2019). The State of Food and Agriculture 2019. Moving Forward on Food Loss and Waste Reduction. Rome. License: CC BY-NC-SA 3.0 IGO.
Faraj, A., Vasanthan, T., and Hoover, R. (2004). The effect of extrusion cooking on resistant starch formation in waxy and regular barley flours. Food Res. Int. 37, 517–525. doi: 10.1016/j.foodres.2003.09.015
Fasina, O. O., Hallman, H., Craig-Schmidt, M., and Clements, C. (2006). Predicting temperature-dependence viscosity of vegetable oils from fatty acid composition. J. Am. Oil Chem. Soc. 83, 899–903. doi: 10.1007/s11746-006-5044-8
Figuerola, F., Hurtado, M. L., Estévez, A. M., Chiffelle, I., and Asenjo, F. (2005). Fibre concentrates from apple pomace and citrus peel as potential fibre sources for food enrichment. Food Chem. 91, 395–401. doi: 10.1016/j.foodchem.2004.04.036
Filli, K. B, Nkama I., Jideani, V. A., and Ibok, U. I. (2012). System parameters and product properties responses during extrusion of fura from millet-soybean mixtures. Niger. Food J. 30, 82–100. doi: 10.1016/S0189-7241(15)30017-5
Fleischman, E. F., Kowalski, R. J., Morris, C. F., Nguyen, T., Li, C., Ganjyal, G., et al. (2016). Physical, textural, and antioxidant properties of extruded waxy wheat flour snack supplemented with several varieties of bran. J. Food Sci. 81, E2726–E2733. doi: 10.1111/1750-3841.13511
Fuad, T., and Prabhasankar, P. (2010). Role of ingredients in pasta product quality : a review on recent developments. Crit. Rev. Food Sci. Nutri. 50, 787–798. doi: 10.1080/10408390903001693
Ganjyal, G. M., and Hanna, M. A. (2004). Effects of extruder die nozzle dimensions on expansion and micrographic characterization during extrusion of acetylated starch. Starch - Stärke 56, 108–117. doi: 10.1002/star.200300200
Godavarti, S., and Karwe, M. V. (1997). Determination of specific mechanical energy distribution on a twin-screw extruder. J. Agric. Eng. Res. 67, 277–287. doi: 10.1006/jaer.1997.0172
Grigelmo-Miguel, N., and Martin-Belloso, O. (1999). Comparison of dietary fibre from by-products of processing fruits and greens and from cereals. Food Sci. Technol. 32, 503–508. doi: 10.1006/fstl.1999.0587
Gu, B.-J., Kowalski, R. J., and Ganjyal, G. (2017). Food Extrusion Processing: an Overview. Pullman, WA: Washington State University Extension. 1–8.
Gu, B.-J., Masli, M. D. P., and Ganjyal, G. M. (2019). Whole faba bean flour exhibits unique expansion characteristics relative to the whole flours of lima, pinto, and red kidney beans during extrusion. J. Food Sci. 85, 404–413. doi: 10.1111/1750-3841.14951
Gu, B.-J., Wolcott, M. P., and Ganjyal, G. M. (2020). Optimized screw profile design proved to inhibit re-agglomeration that occurs during extrusion of fine-milled forest residuals for producing fermentable sugars. Ind. Crops Prod. 154:112730. doi: 10.1016/j.indcrop.2020.112730
Gull, A., Prasad, K., and Kumar, P. (2015). Effect of millet flours and carrot pomace on cooking qualities, color and texture of developed pasta. LWT 63, 470–474. doi: 10.1016/j.lwt.2015.03.008
Gull, A., Prasad, K., and Kumar, P. (2018). Nutritional, antioxidant, microstructural, and pasting properties of functional pasta. J. Saudi Soc. Agric. Sci. 17, 147–153. doi: 10.1016/j.jssas.2016.03.002
Guy, R. (ed.). (2001). “Chapter 1: Introduction” in Extrusion Cooking Technologies and Applications (England: Woodhead Publishing), 1–2. doi: 10.1016/B978-1-85573-731-0.50001-3
Ilo, S., Schoenlechner, R., and Berghofe, E. (2008). Role of lipids in the extrusion cooking processes. Grasas Aceites 51, 97–110. doi: 10.3989/gya.2000.v51.i1-2.410
Kaisangsri, N., Kowalski, R. J., Wijesekara, I., Kerdchoechuen, O., Laohakunjit, N., and Ganjyal, G. M. (2016). Carrot pomace enhances the expansion and nutritional quality of corn starch extrudates. LWT 68, 391–399. doi: 10.1016/j.lwt.2015.12.016
Kallu, S., Kowalski, R. J., and Ganjyal, G. M. (2017). Impacts of cellulose fiber particle size and starch type on expansion during extrusion processing. J. Food Sci. 82, 1647–1656. doi: 10.1111/1750-3841.13756
Kaur, G., Sharma, S., Nagi, H. P., and Dar, B. N. (2012). Functional properties of pasta enriched with variable cereal brans. J. Food Sci. Technol. 49, 467–474. doi: 10.1007/s13197-011-0294-3
Kazemzadeh, M. (2012). “Chapter 1: introduction to extrusion technology” in Advances in Food Extrusion Technology. Contemporary Food Engineering Series, Vol. 13, eds M. Maskan and A. Altan (CRC Press), 1–28. doi: 10.1017/CBO9781107415324.004
King, T., Cole, M., Farber, J. M., Eisenbrand, G., Zabaras, D., Fox, E. M., et al. (2017). Food safety for food security: relationship between global megatrends and developments in food safety. Trends Food Sci. Technol. 68, 160–175. doi: 10.1016/j.tifs.2017.08.014
Korkerd, S., Wanlapa, S., Puttanlek, C., Uttapap, D., and Rungsardthong, V. (2015). Expansion and functional properties of extruded snacks enriched with nutrition sources from food processing by-products. J. Food Sci. Technol. 53, 561–570. doi: 10.1007/s13197-015-2039-1
Kowalski, R. J., Hause, J. P., Joyner, H., and Ganjyal, G. M. (2018). Waxy flour degradation-Impact of screw geometry and specific mechanical energy in a co-rotating twin screw extruder. Food Chem. 239, 688–696. doi: 10.1016/j.foodchem.2017.06.120
Kowalski, R. J., Morris, C. F., and Ganjyal, G. M. (2015). Waxy soft white wheat: extrusion characteristics and thermal and rheological properties. Cereal Chem. 92, 145–153. doi: 10.1094/CCHEM-03-14-0039-R
Krishnan, R., Agarwal, R., Bajada, C., and Arshinder, K. (2020). Redesigning a food supply chain for environmental sustainability - an analysis of resource use and recovery. J. Cleaner Prod. 242:118374. doi: 10.1016/j.jclepro.2019.118374
Kurek, M. A., Piwińska, M., Wyrwisz, J., and Wierzbicka, A. (2015). Automated static image analysis as a novel tool in describing the physical properties of dietary fiber. Food Sci. Technol. 35. doi: 10.1590/1678-457X.6720
Lai, L. S., and Kokini, J. L. (1991). Physicochemical changes and rheological properties of starch during extrusion (a review). Biotechnol. Prog. 7, 251–266. doi: 10.1021/bp00009a009
Lai, W. T., Khong, N. M. H., Lim, S. S., Hee, Y. Y., Sim, B. I., Lau, K. Y., et al. (2017). A review: modified agricultural by-products for the development and fortification of food products and nutraceuticals. Trends Food Sci. Technol. 59, 148–160. doi: 10.1016/j.tifs.2016.11.014
Lecumberri, E., Mateos, R., Izquierdo-Pulido, M., Rupérez, P., Goya, L., and Bravo, L. (2007). Dietary fibre composition, antioxidant capacity and physico-chemical properties of a fibre-rich product from cocoa (Theobroma cacao L.). Food Chem. 104, 948–954. doi: 10.1016/j.foodchem.2006.12.054
Leyva-Corral, J., Quintero-ramos, A., Camacho-d, A., Ruiz-anchondo, T. D. J., and Mel, C. O. (2016). Polyphenolic compound stability and antioxidant capacity of apple pomace in an extruded cereal. LWT 65, 228–236. doi: 10.1016/j.lwt.2015.07.073
Liu, J., Jin, S., Song, H., Huang, K., Li, S., Guan, X., et al. (2020). Effect of extrusion pretreatment on extraction, quality and antioxidant capacity of oat (Avena Sativa L.) bran oil. J. Cereal Sci. 95:102972. doi: 10.1016/j.jcs.2020.102972
Lončarić, A., Kosović, I., Jukić, M., Ugarčić, Ž., and PiliŽota, V. (2014). Effect of apple by-product as a supplement on antioxidant activity and quality parameters of pasta. Croatian J. Food Sci. Technol. 6, 97–103. doi: 10.17508/CJFST.2014.6.2.05
Lopez, G., Ros, G., Rincon, F., Periago, M., Martinez, M. C., and Ortuno, J. (1996). Relationship between physical and hydration properties of soluble and insoluble fiber of artichoke. J. Agric. Food Chem. 44, 2773–2778. doi: 10.1021/jf9507699
Majerska, J., Michalska, A., and Figiel, A. (2019). A review of new directions in managing fruit and vegetable processing by-products. Trends Food Sci. Technol. 88, 207–219. doi: 10.1016/j.tifs.2019.03.021
Marić, M., Grassino, A. N., Zhu, Z., Barba, F. J., Brnčić, M., and Rimac Brnčić, S. (2018). An overview of the traditional and innovative approaches for pectin extraction from plant food wastes and by-products: ultrasound, microwaves, and enzyme-assisted extraction. Trends Food Sci. Technol. 76, 28–37. doi: 10.1016/j.tifs.2018.03.022
Masatcioglu, M., Yalcin, E., Hwan, P. J., Ryu, G., Celik, S., and Koksel, H. (2014). Hull-less barley flour supplemented corn extrudates produced by conventional extrusion and CO2 injection process. Innov. Food Sci. Emerg. Technol. 26, 302–309. doi: 10.1016/j.ifset.2014.06.003
Masli, M. D. P., Gu, B.-J., Rasco, B. A., and Ganjyal, G. M. (2018a). Fiber-rich food processing by-products enhance the expansion of cornstarch extrudates. J. Food Sci. 83, 2500–2510. doi: 10.1111/1750-3841.14290
Masli, M. D. P., Rasco, B. A., and Ganjyal, G. M. (2018b). Composition and physicochemical characterization of fiber-rich food processing by-products. J. Food Sci. 83, 956–965. doi: 10.1111/1750-3841.14081
Mazlan, M. M., Talib, R. A., Mail, N. F., Taip, S., Chin, N. L., Sulaiman, R., et al. (2019). Effects of extrusion variables on corn-mango peel extrudates properties, torque and moisture loss. Int. J. Food Prop. 22, 54–70. doi: 10.1080/10942912.2019.1568458
Moraru, C. I., and Kokini, J. L. (2003). Nucleation and expansion during extrusion and microwave heating of cereal foods. Compr. Rev. Food Sci. Food Saf. 2, 147–165. doi: 10.1111/j.1541-4337.2003.tb00020.x
Nascimento, E. M. D. G. C., Carvalho, C. W. P., Takeiti, C. Y., Freitas, D. D. G. C., and Ascheri, J. L. R. (2012). Use of sesame oil cake (Sesamum indicum L.) on corn expanded extrudates. Food Res. Int. 45, 434–443. doi: 10.1016/j.foodres.2011.11.009
Navarro-Cortez, R. O., Aguilar-Palazuelos, E., Castro-Rosas, J., Cortés, R. N. F., Ramírez, A. C., Delgado-Licon, E., et al. (2018). Physicochemical and sensory characterization of an extruded product from blue maize meal and orange bagasse using the response surface methodology. CyTA J. Food 16, 498–505. doi: 10.1080/19476337.2017.1416674
Nelson, A. L. (2001). Properties of high-fiber ingredients. Cereal Foods World 46, 93–97. doi: 10.1094/1891127233
New Food (2014). Pasta Extrusion: Conversion of Semolina into Pasta. Available online at: https://www.newfoodmagazine.com/article/15045/pasta-extrusion/ (accessed May, 2020).
Nikinmaa, M., Alam, S. A., Raulio, M., Katina, K., Kajala, I., Nordlund, E., et al. (2017). Bioprocessing of bran with exopolysaccharide producing microorganisms as a tool to improve expansion and textural properties of extruded cereal foams with high dietary fibre content. LWT 77, 170–177. doi: 10.1016/j.lwt.2016.11.041
Nilusha, R. A. T., Jayasinghe, J. M. J. K., Perera, O. D. A. N., and Perera, P. I. P. (2019). Development of pasta products with nonconventional ingredients and their effect on selected quality characteristics : a brief overview. Int. J. Food Sci. 2019:6750726. doi: 10.1155/2019/6750726
Norajit, K., Gu, B.-J., and Ryu, G.-H. (2011). Effects of the addition of hemp powder on the physicochemical properties and energy bar qualities of extruded rice. Food Chem. 129, 1919–1925. doi: 10.1016/j.foodchem.2011.06.002
Offiah, V., Kontogiorgos, V., and Falade, K. O. (2019). Extrusion processing of raw food materials and by-products: a review. Crit. Rev. Food Sci. Nutr. 59, 2979–2998. doi: 10.1080/10408398.2018.1480007
O'Shea, N., Arendt, E., and Gallagher, E. (2014). Enhancing an extruded puffed snack by optimising die head temperature, screw speed and apple pomace inclusion. Food Bioprocess Technol. 7, 1767–1782. doi: 10.1007/s11947-013-1181-x
O'Shea, N., Ktenioudaki, A., Smyth, T. P., Mcloughlin, P., Doran, L., Auty, M. A. E., et al. (2015). Physicochemical assessment of two fruit by-products as functional ingredients : apple and orange pomace. J. Food Eng. 153, 89–95. doi: 10.1016/j.jfoodeng.2014.12.014
Osman, M. G., Sahai, D., and Jackson, D. S. (2000). Oil absorption characteristics of a multigrain extrudate during frying: effect of extrusion temperature and screw speed. Cereal Chem. 77, 101–104. doi: 10.1094/CCHEM.2000.77.2.101
Padalino, L., D'Antuono, I., Durante, M., Conte, A., Cardinali, A., Linsalata, V., et al. (2018). Use of olive oil industrial by-product for pasta enrichment. Antioxidants 7, 1–15. doi: 10.3390/antiox7040059
Pérez, A. A., Drago, S. R., Carrara, C. R., De Greef, D. M., Torres, R. L., and González, R. J. (2008). Extrusion cooking of a maize/soybean mixture: factors affecting expanded product characteristics and flour dispersion viscosity. J. Food Eng. 87, 333–340. doi: 10.1016/j.jfoodeng.2007.12.008
Peschel, W., Sánchez-Rabaneda, F., Diekmann, W., Plescher, A., Gartzía, I., Jiménez, D., et al. (2006). An industrial approach in the search of natural antioxidants from vegetable and fruit wastes. Food Chem. 97, 137–150. doi: 10.1016/j.foodchem.2005.03.033
Petravić-Tominac, V., Zechner-Krpan, V., Berković, K., Galović, P., Herceg, Z., Srečec, S., et al. (2011). Rheological properties, water-holding and oil-binding capacities of particulate β-glucans isolated from spent brewer's yeast by three different procedures. Food Technol. Biotechnol. 49, 56–64.
Piwińska, M., Wyrwisz, J., Kurek, M., and Wierzbicka, A. (2015). Hydration and physical properties of vacuum-dried durum wheat semolina pasta with high-fiber oat powder. LWT 63, 647–653. doi: 10.1016/j.lwt.2015.03.022
Raak, N., Symmank, C., Zahn, S., Aschemann-Witzel, J., and Rohm, H. (2017). Processing and product related causes for food waste and implications for the food supply chain. Waste Manage. 61, 461–472. doi: 10.1016/j.wasman.2016.12.027
Rathod, R. P., and Annapure, U. S. (2016). Effect of extrusion process on antinutritional factors and protein and starch digestibility of lentil splits. LWT 66, 114–123. doi: 10.1016/j.lwt.2015.10.028
Ravindran, R., and Jaiswal, A. K. (2016). Exploitation of food industry waste for high-value products. Trends Biotechnol. 34, 58–69. doi: 10.1016/j.tibtech.2015.10.008
Reyes-Jáquez, D., Casillas, F., Flores, N., Andrade-González, I., Solís-Soto, A., Medrano-Roldán, H., et al. (2012). The effect of glandless cottonseed meal content and process parameters on the functional properties of snacks during extrusion cooking. Food Nutr. Sci. 3, 1716–1725. doi: 10.4236/fns.2012.312225
Rivera-González, G., Amaya-Guerra, C. A., and de la Rosa-Millán, J. (2019). Physicochemical characterisation and in vitro starch digestion of avocado seed flour (Persea americana V. Hass) and its starch and fibrous fractions. Int. J. Food Sci. Technol. 54, 2447–2457. doi: 10.1111/ijfs.14160
Robertson, J. A., and Eastwood, M. A. (1981). An examination of factors which may affect the water holding capacity of dietary fibre. Br. J. Nutr. 45, 83–88. doi: 10.1079/BJN19810079
Robin, F., Dubois, C., Pineau, N., Schuchmann, H. P., and Palzer, S. (2011a). Expansion mechanism of extruded foams supplemented with wheat bran. J. Food Eng. 107, 80–89. doi: 10.1016/j.jfoodeng.2011.05.041
Robin, F., Schuchmann, H. P., and Palzer, S. (2012). Dietary fiber in extruded cereals: limitations and opportunities. Trends Food Sci. Technol. 28, 23–32. doi: 10.1016/j.tifs.2012.06.008
Robin, F., Théoduloz, C., Gianfrancesco, A., Pineau, N., Schuchmann, H. P., and Palzer, S. (2011b). Starch transformation in bran-enriched extruded wheat flour. Carbohydr. Polym. 85, 65–74. doi: 10.1016/j.carbpol.2011.01.051
Ruiz-Armenta, X. A., Zazueta-Morales, J. D. J., Delgado-Nieblas, C. I., López-Diaz, A., Camacho-Hernández, I. L., Gutiérrez-Dorado, R., et al. (2018). Effect of extrusion on the carotenoid content, physical and sensory properties of snacks added with bagasse of naranjita fruit : optimization process. CyTA J. Food 16, 172–180. doi: 10.1080/19476337.2017.1368717
Rzedzicki, Z., Kozlowska, H., and Troszynska, A. (2004). Application of pea hulls for extrudate production. Pol. J. Food Nutr. Sci. 13, 363–368.
Santana-Méridas, O., González-Coloma, A., and Sánchez-Vioque, R. (2012). Agricultural residues as a source of bioactive natural products. Phytochem. Rev. 11, 447–466. doi: 10.1007/s11101-012-9266-0
Sant'Anna, V., Christiano, F. D. P., Marczak, L. D. F., Tessaro, I. C., and Thys, R. C. S. (2014). The effect of the incorporation of grape marc powder in fettuccini pasta properties. LWT 58, 497–501. doi: 10.1016/j.lwt.2014.04.008
Sarifudin, A., and Assiry, A. M. (2014). Some physicochemical properties of dextrin produced by extrusion process. J. Saudi Soc. Agric. Sci. 13, 100–106. doi: 10.1016/j.jssas.2013.02.001
Sayanjali, S., Ying, D., Sanguansri, L., Buckow, R., Augustin, M. A., and Gras, S. L. (2017). The effect of extrusion on the functional properties of oat fibre. LWT 84, 106–113. doi: 10.1016/j.lwt.2017.05.025
Selani, M. M., Brazaca, S. G. C., dos Santos Dias, C. T., Ratnayake, W. S., Flores, R. A., and Bianchini, A. (2014). Characterisation and potential application of pineapple pomace in an extruded product for fibre enhancement. Food Chem. 163, 23–30. doi: 10.1016/j.foodchem.2014.04.076
Sevatson, E., and Huber, G. R. (2000). “Chapter 9: Extruders in food applications,” in Extruders in Food Applications, ed M. N. Riaz (Boca Raton, FL: CRC Press), 167–204.
Sharma, S. K., Bansal, S., Mangal, M., Dixit, A. K., Gupta, R. K., and Mangal, A. K. (2015). Utilization of food processing by-products as dietary, functional, and novel fiber: a review. Crit. Rev. Food Sci. Nutr. 56, 1647–1661. doi: 10.1080/10408398.2013.794327
Simonato, B., Trevisan, S., Tolve, R., Favati, F., and Pasini, G. (2019). Pasta fortification with olive oil pomace: effects on the technological characteristics and nutritional properties. LWT 114:108368. doi: 10.1016/j.lwt.2019.108368
Singha, P., Kasiviswanathan, M., and Padmanaban, K. (2017). Influence of processing conditions on apparent viscosity and system parameters during extrusion of distiller's dried grains-based snacks. Food Sci. Nutr. 6, 101–110. doi: 10.1002/fsn3.534
Singkhornart, S., Gu, B.-J., and Ryu, G. H. (2013). Physicochemical properties of extruded germinated wheat and barley as modified by CO2 injection and difference extrusion conditions. Int. J. Food Sci. Technol. 48, 290–299. doi: 10.1111/j.1365-2621.2012.03186.x
Sozer, N., and Kaya, A. (2003). Changes in cooking and textural properties of spaghetti cooked with different levels of salt in the cooking water. J. Texture Stud. 34, 381–390. doi: 10.1111/j.1745-4603.2003.tb01070.x
Stojceska, V., Ainsworth, P., Plunkett, A., Ibanoglu, E., and Ibanoglu, S. (2008a). Cauliflower by-products as a new source of dietary fibre, antioxidants and proteins in cereal based ready-to-eat expanded snacks. J. Food Eng. 87, 554–563. doi: 10.1016/j.jfoodeng.2008.01.009
Stojceska, V., Ainsworth, P., Plunkett, A., and Senol, I. (2008b). The recycling of brewer's processing by-product into ready-to-eat snacks using extrusion technology. J. Cereal Sci. 47, 469–479. doi: 10.1016/j.jcs.2007.05.016
Teigiserova, D. A., Hamelin, L., and Thomsen, M. (2019). Review of high-value food waste and food residues biorefineries with focus on unavoidable wastes from processing. Resour. Conserv. Recycl. 149, 413–426. doi: 10.1016/j.resconrec.2019.05.003
Tiwari, A., and Jha, S. K. (2017). Extrusion cooking technology: principal mechanism and effect on direct expanded snacks - an overview. Int. J. Food Stud. 6, 113–128. doi: 10.7455/ijfs/6.1.2017.a10
Tovar-Jiménez, X., Caro-Corrales, J., Limón-Valenzuela, V., Castro-Rosas, J., Hernández-Ávila, J., and Aguilar-Palazuelos, E. (2015). Third generation snacks manufactured from orange by-products : physicochemical and nutritional characterization. J. Food Sci. Technol. 52, 6607–6614. doi: 10.1007/s13197-015-1726-2
Viuda-Martos, M., Ruiz-Navajas, Y., Martin-Sánchez, A., Sánchez-Zapata, E., Fernández-López, J., Sendra, E., et al. (2012). Chemical, physico-chemical and functional properties of pomegranate (Punica granatum L.) bagasses powder co-product. J. Food Eng. 110, 220–224. doi: 10.1016/j.jfoodeng.2011.05.029
Wang, P., Fu, Y., Wang, L., Saleh, A. S. M., Cao, H., and Xiao, Z. (2017). Effect of enrichment with stabilized rice bran and extrusion process on gelatinization and retrogradation properties of rice starch. Starch Stärke 69:1600201. doi: 10.1002/star.201600201
Wang, S., Gu, B.-J., and Ganjyal, G. M. (2019). Impacts of the inclusion of various fruit pomace types on the expansion of corn starch extrudates. LWT 110, 223–230. doi: 10.1016/j.lwt.2019.03.094
Wang, S., Kowalski, R. J., Kang, Y., Kiszonas, A. M., Zhu, M. J., and Ganjyal, G. M. (2017). Impacts of the particle sizes and levels of inclusions of cherry pomace on the physical and structural properties of direct expanded corn starch. Food Bioprocess Technol. 10, 394–406. doi: 10.1007/s11947-016-1824-9
Wójtowicz, A., and Mościcki, L. (2011). Effect of wheat bran addition and screw speed on microstructure and textural characteristics. Polish J. Food Nutr. Sci. 61, 101–107. doi: 10.2478/v10222-011-0010-z
Xie, F., Gu, B.-J., Saunders, S. R., and Ganjyal, G. M. (2021). High methoxyl pectin enhances the expansion characteristics of the cornstarch relative to the low methoxyl pectin. Food Hydrocoll. 110:106131. doi: 10.1016/j.foodhyd.2020.106131
Yagci, S., and Gögüş, F. (2009). Development of extruded snack from food by-products: a response surface analysis. J. Food Process Eng. 32, 565–586. doi: 10.1111/j.1745-4530.2007.00232.x
Yanniotis, S., Petraki, A., and Soumpasi, E. (2007). Effect of pectin and wheat fibers on quality attributes of extruded cornstarch. J. Food Eng. 80, 594–599. doi: 10.1016/j.jfoodeng.2006.06.018
Ye, J., Hu, X., Luo, S., Liu, W., Chen, J., Zeng, Z., et al. (2018). Properties of starch after extrusion: a review. Starch/Staerke 70, 1–8. doi: 10.1002/star.201700110
Ying, D., Hlaing, M. M., Lerisson, J., Pitts, K., Cheng, L., Sanguansri, L., et al. (2017). Physical properties and FTIR analysis of rice-oat flour and maize-oat flour based extruded food products containing olive pomace. Food Res. Int. 100, 665–673. doi: 10.1016/j.foodres.2017.07.062
Zarzycki, P., Sykut-Domanska, E., Sobota, A., Teterycz, D., Kraawecka, A., Blicharz-Kania, A., et al. (2020). Flaxseed enriched pasta-chemical composition and cooking quality. Foods 9:404. doi: 10.3390/foods9040404
Keywords: by-product, extrusion, functional properties, direct expansion, pellets, pasta
Citation: Dey D, Richter JK, Ek P, Gu B-J and Ganjyal GM (2021) Utilization of Food Processing By-products in Extrusion Processing: A Review. Front. Sustain. Food Syst. 4:603751. doi: 10.3389/fsufs.2020.603751
Received: 07 September 2020; Accepted: 18 December 2020;
Published: 26 January 2021.
Edited by:
Carlos Martin-Rios, University of Applied Sciences and Arts of Western Switzerland, SwitzerlandReviewed by:
Juncai Tu, Lincoln University, New ZealandJose Luis Ramirez Ascheri, Embrapa Food Technology, Brazil
Copyright © 2021 Dey, Richter, Ek, Gu and Ganjyal. This is an open-access article distributed under the terms of the Creative Commons Attribution License (CC BY). The use, distribution or reproduction in other forums is permitted, provided the original author(s) and the copyright owner(s) are credited and that the original publication in this journal is cited, in accordance with accepted academic practice. No use, distribution or reproduction is permitted which does not comply with these terms.
*Correspondence: Girish M. Ganjyal, girish.ganjyal@wsu.edu