Physical and culinary analysis of long gluten-free extruded pasta based on high protein quinoa flour
- Departamento de Agroindustria, Facultad de Ciencias Agrarias, Universidad del Cauca, Popayán, Colombia
The consumption of foods with high protein content from pseudocereals is of great industrial interest. Pasta has a high gluten content; consequently, obtaining these gluten-free products is a technological challenge. The products obtained from quinoa show excellent results in protein and fiber with low glycemic index. This work focused on studying the effect of quinoa fat on the production of long pasta by extrusion in different mixtures of hyperprotein quinoa (HHP). It was observed that formulations with high percentages of starch showed a higher expansion rate, due to a higher fat content. Likewise, extruded pastes showed higher values of brightness than those containing lower percentages of starch and crude fat. The fracturability results were associated with the resistance of the paste to the pressure exerted for its deformation, which does not exceed 3.73 mm. The formulations with lower fat content presented high values in fracture stress due to the low diffusion of water and lipids. It was shown that fat has an indirect influence with a strong correlation with the expansion index and fracture stress and a moderate correlation (p > 0.05) with Young's modulus, indicating that increasing the added fat content increases the percentage of mass loss by baking. The melting and cohesiveness of all components improved during extrusion due to the pregelatinization of cassava starch, the addition of defatted HHP and the availability of quinoa starch granules.
Introduction
There is now a trend to consume foods high in non-animal protein for their health benefits, environmental sustainability, and ethical merit (Faber et al., 2021). Non-animal protein sources had been mainly extracted and utilized from cereals, vegetables, legumes, algae, fungi and bacteria, with high acceptability in food products (Boukid et al., 2021). Wheat is one of the raw materials used in the generation of vegan products, because of its varied protein source with gluten being its most important compound (Galanakis, 2019). However, intolerance of this protein in some people had been detect worldwide, and this autoimmune system disorder is known as celiac disease triggered by gluten in the superior part of the intestine, resulting in malnutrition, diarrhea, growth retardation, anemia and fatigue (Yu et al., 2021).
A product with the highest gluten content is pasta (Dziki, 2021), the production of gluten-free pasta products is a technological achievement, as the viscosity and elasticity of the initial dough differs significantly from those made from wheat (Lorenzo et al., 2018). To improve the initial working mass, water binding agents and thickeners, structure and flavor forming agents, surfactants and especially higher amounts of fat and sugars have been added to mask both the color of the final product and its flavor (Gao et al., 2018), which represent a higher cost than those made with wheat and without additives.
The development of pasta-type products has been studied employing alternative raw materials such as rice flour, maize, sorghum, amaranth, quinoa, among others (Brites et al., 2018; Gao et al., 2018), being mainly low in protein percentage and majority in carbohydrate content, attributing to products with a high glycemic index than those containing gluten (Culetu et al., 2021; di Pede et al., 2021).
Some authors have reported the existence of more than 16,000 varieties of quinoa accessions around the world, showing the structural attributes and antioxidant capacities of six quinoa cultivars with high productive potential in the central regions of Colombia (García-Parra et al., 2020, 2021). On the other hand, edaphoclimatic conditions have a differential effect on quinoa cultivars, which can alter quinoa production, modifying the compositional characteristics of its seeds (García-Parra et al., 2022). Consequently, several publications have been made in relation to the physicochemical properties and behavior of the components of quinoa, including the quantification of moisture, lipids, and protein of raw materials for the validation of high protein flour (Muñoz-Pabon et al., 2022).
Food products produced from quinoa have shown great results in terms of higher protein and fiber content, as well as low glycemic index (Montemurro et al., 2019; Ramos-Diaz et al., 2020). However, it may not be used in large quantities because of its intense flavor, as it lowers the quality of the final product. In recent years, there has been great interest in pseudocereals for its high nutritional value. One of them is quinoa, which is high in protein and essential amino acids, but its excellence and importance lies not only in the quantity of protein but also in its quality. The protein content of these species has been reported to be around 10–20%. The biological and techno-functional properties of protein isolates (PI) and their protein-protein, protein-polysaccharide and protein-lipid interactions have been studied given the characteristics of reserve proteins in cereals and pseudocereals (Ortiz-Gómez et al., 2022a).
Abrasive milling results have been reported, which allows obtaining flours with different composition rich in fiber and proteins. This flour is fine and ideal for addition in formulations requiring high solubility with high soluble protein content. Important properties in the elaboration of functional foods with high protein content (Roa-Acosta et al., 2020; Ortiz-Gómez et al., 2022a). One of the solutions is the use of these high-protein flours, some of which reach a protein content of around 34% (Roa-Acosta et al., 2020), and therefore not only reduces the addition of quinoa flour but also increases the protein percentage of the pasta type products, which commercially do not exceed 12% protein.
Rheological studies have been reported on dispersions of defatted (HHPD) and non-fatted high protein quinoa flours (HHP), obtaining a dilatant behavior. The application of heat treatments produces differences in the consistency index and the fluidity index of the dispersions depending on the concentration and/or type of high protein flour. Mechanical defatting produces high protein flours which produce dispersions with higher viscosity profiles compared to chemically defatted or non-fatted flours. These differences are the result of the composition and structural changes of the proteins and starches present in the mixture.
To produce pasta-type products, its fat content could influence the quality of the finished product and the desired rheological properties, its interaction with the proteins, starches and carbohydrates presents in the formulation, which are necessary to guarantee a suitable long pasta, being unknown and little evaluated. The protein content is higher than 20% of these pastas. Some examples are: the red lentil pasta (25 g protein), the chickapea pasta (24 g protein), the red lentil and quinoa pasta (25 g protein).
For example, it is still uncertain how proteins and starch influence each other's behavior during the cooking of pasta. Studying the mechanism of the effect of temperature on proteins and starch during the heating of these flours is an important step in predicting and controlling the processing and functional properties of more complex cereal food systems. This work evaluates the effect of quinoa fat in the production of long extruded pasta type product in different mixtures of hyper-protein quinoa (HHP) and defatted hyper-protein quinoa in a base formulation of rice, corn, and cassava starch for the generation of long extruded pasta with a high percentage of non-animal protein (21%), evaluating its physical and culinary properties. The protein content was established according to the requirement of the company (SEGALCO SAS), who are interested in the vegetarian pasta products market.
The novelty of this research is focused on the use of flours of high nutritional value obtained from pseudocereals. The behavior of the physicochemical, structural and rheological properties of these processed flours has not yet been sufficiently studied. Consequently, being able to generate new developments from these flours has a great impact on the sustainability of the farming community that lives from this crop.
Materials and methods
Raw materials
Maize flour (8.0% moisture, 9.4% protein, 2.2% crude fat, 6.9% crude fiber), rice flour (10.7% moisture, 7.0% protein, 0.8% crude fat, 7.2% crude fiber), cassava starch modified with natural maize starch (14% moisture, 0.1% protein, 0.0% crude fat, 0.0% crude fiber, 86% total carbohydrates), high protein quinoa flour (4.23% moisture, 30.1% protein, 18.8% crude fat, 9.4% crude fiber) supplied by SEGALCO S.A.S. (Popayán, Colombia) with its respective composition technical data sheet (% w/w).
Hyperprotein defatted quinoa flour (HHPD) was obtain using an Oil Press Machine Automatic Oil (Presser Cold/Hot Press equipment) (Cgoldenwall K28, China) at 120°C. HHPD was subjected to proximate analysis for moisture (2.8%), protein (40.3%), crude fat (9.7%) and crude fiber (15.2%), following AOAC methodologies 925.10, 955.04, 963.15 and 962.09, respectively (AOAC, 2000).
Experimental design—pasta formulation
The pasta formulations were made with a mixture of maize flour, rice, cassava starch, HHP and HHPD, always guaranteeing around 21% protein (w/w). A minimum (~4.7) and maximum (~15.7) percentage of modified cassava starch (previous trials) was fixing in the mass balances, varying with and without HHP and HHPD. In addition, controls were performing without modified cassava starch (formulations 9 and 10). Table 1 shows the formulations used to produce gluten-free and vegetable protein extruded pasta, and Table 2 shows each of the formulations with their respective percentages of protein and lipids calculated theoretically by mass balance from the proximate composition of each of the flours used.
Extruded paste production
The formulations were each brought to 30% (w/w) moisture prior to extrusion in a 4.8 L mixer (KitchenAid Model Artisan KSM 1520, St. Joseph, MI, USA). Extrusion was performed in a extruder (Haake Rheomex OS, Thermo Scientific, U.S.A.), equipped with twin screws at a temperature profile of 80.9°C (75, 81, 90, 80, 95, 70, 75), 160 rpm screw speed and 2.5 mm extruder outlet nozzle diameter. The pastes were dried at room temperature for three days in Popayán, Colombia, under controlled conditions that guaranteed the safety of the product (20 ± 1°C, 78 ± 1% relative humidity (RH), until reaching a final water activity (awf) of 0.51).
Physical properties
The percentage moisture content of the dried pastes was evaluated on a moisture meter (XM 10 SE, Precisa Instruments AG, Switzerland). Water activity (aw) was evaluated using a water activity meter (AquaLab, Pawkit, USA) (Table 3). Equation calculated the expansion index (IE) (Eq. 1), where: D is the diameter of dry pulp obtained and DB is the diameter of the nozzle used in extrusion, each measurement was performed 10 times. All measurements were performed in triplicate.
The CIELab values of the samples were determined in triplicate in transmission mode, using 10 mm polystyrene cuvettes and the color change was measured at 25°C with the help of spectrophotometer color analyzer (CM-5, Konica Minolta Sensing Inc., Tokyo, Japan), with D65 illuminant and an observation angle of 10°. To determine the components, 5 mm long paste samples were taken and placed in 20 mm Petri dishes, between 4 and 5 g each (Roa-Acosta et al., 2020). All samples were measured in four replicates.
Textural characterization
The rupture test obtained the characteristics of the mechanical properties in the pastes, using a universal testing machine (EZ Test, Shimadzu, Japan) employing the three-point bending technique (Brandolini et al., 2018). A 12-centimeter strip of paste was taken and placed in the three-point device, which had a length of 60 mm, with a blade moving at 1 mm/min. The measurements were replicated 10 times per sample. The firmness of the paste was measured, to measure the maximum breaking strength (F) and fracturability (distance traveled to obtain F). The fracture toughness (σfrac) was calculated using Equation (2), considering the moment of inertia for a uniform bar of circular cross-section (Bruns and Bourne, 1975). The elastic modulus (Young's modulus) was calculated by the slope between fracture stress and apparent strain (ε) (Equation 3), as a function of time.
Where L is the length between support points (60 mm), r is the radius of the dry paste, d is the diameter of the sample and h is the distance traveled to break it (fracturability).
Culinary properties
The increase in weight of the pasta during cooking (water absorption) was evaluated by weighing the pasta before and after cooking (Lorusso et al., 2017). The results were expressed according to equation (4).
Where W1 is the weight of the cooked pasta and W0 is the weight of the raw samples.
The mass loss in cooking (expressed as grams of loss/100 g of pulp) was determined gravimetrically by weighing the suspended solids, following the method described by Curiel et al. (2014) with some modifications. Approximately 3 g of paste were weighed, then placed in a beaker with 50 ml of boiling water for 5 min, after which time the paste was removed, 5 ml of the cooking water was taken and placed in Petri dishes (previously dried at 90°C for 12 h and weighed). Finally, the dry material was weighed to quantify the amount of solids lost by cooking (SW). The calculation of % mass loss by cooking was calculated by using equation (5).
For the determination of the cooking time of the gluten-free dough, the method described by Ramos-Diaz et al. (2020) with some modifications, was used. Twelve 1 cm long strands of pasta were cut, placed in a beaker with 50 ml of water and brought to boiling point (time zero). Subsequently, every minute, by breaking the sample in half, it was determined whether the white line in the center (cooking control) of the dough disappears as a function of time by complete cooking of the pasta.
Statistical analysis
Treatments were run using a randomized complete block design and one-way analysis of variance (ANOVA) at the 95% confidence level was used to determine significant differences between properties and samples. Tukey's test was used to determine which mean scores differed significantly from the others. Pearson's correlation coefficient analysis was used to observe the effect of the addition of HHPD and the correlations between the different techniques. Statistical analysis of the results was performed using STATGRAPHICS Centurion XV software.
Results and discussion
Physical characterization
The pastes were dried at room temperature to a moisture content of 3%. Table 3 shows the physical characterization of the extruded pastes, in all pastes a similar aw between 0.65 and 0.72 was observed, showing that they will be susceptible products for the growth of fungi and yeasts under storage conditions. A crucial factor in the extrusion processes for the determination of the expansion rate are high percentages of both starch and protein, evaluated for all protein extruded pasta (Tables 2, 5) formulations according to Rolandelli et al. (2020). It was observed that formulations 6, 7 and 8 with high percentages of starch showed higher expansion index (Table 3), but with a significant difference between them (p < 0.05), due to higher fat content. This behavior could be attributed to the fact that the lower the fat content, the higher the expansion rate (Saeleaw and Schleining, 2010; Cueto et al., 2015; Tiga et al., 2021; Chen et al., 2022). However, in formulations 4 and 8 showed high expansion indexes (17.24 ± 1.29 and 35.40 ± 2.90, respectively), the same behavior was observed, but it could also be explained from the point of view of the synergistic interaction between the crude fat and starch content in the formulation, which shows that there is a close dependence in the relationship between these two components that affect the increase or decrease in the expansion index, showing a trend depending on this relationship.
A commercial characteristic of gluten-free products is the color of the final product, as shown in Table 4. The coordinates L*, a* and b* provide color properties that can vary with the chemical composition of the blending materials that are part of the formulation. The lightness (L*) of the pastes obtained were dark, which is attributed to the presence of quinoa flour in the formulations (Ramos-Diaz et al., 2020). Extruded pastes were found to have higher brightness values than those containing lower percentages of starch and crude fat (formulations 4, 5 and 8). However, as has been found in other studies (Torres et al., 2021), with the same trend of a* and b* values in the samples, report higher values of yellowness (b*), a behavior characteristic of quinoa-based products.
Textural characterization
A parameter to evaluate the quality of long dough is its behavior to fracture by the bending technique. Table 5 shows the results of the textural behavior of the dry pasta based on high protein flour. It shows that there are differences in texture between the different samples, and when compared with other studies, significant low differences were observed (Sosa et al., 2018). The reason for this could be that the formulations were made with a high percentage of protein, which could influence the cross-linking of the components (protein, carbohydrates, water) present in the dough mixture. Therefore, it is necessary to use gums as an agent of intermolecular interaction with water and thickener, to improve the techno-functional characteristics of the initial dough in terms of viscosity and elasticity that attribute similar physicochemical properties to wheat (Tiga et al., 2021).
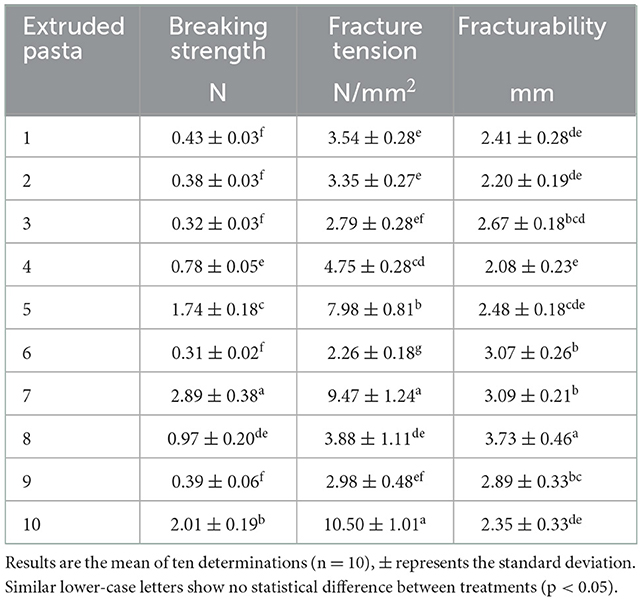
Table 5. Results of textural characterization by three-point test on long pasta based on high protein quinoa.
The results of texture (Table 5) show that the formulations have a higher fiber content (Table 2) in their structure, presenting a higher breaking strength. This behavior of the paste is due to materials with high fiber content. The behavior is different in formulations 7 and 10, however, and is because of a major interaction between the components of the paste mixture, improving its structure (Aribas et al., 2020). Formulations 4, 5, 7, 8 and 10 were the samples with the lowest fat content and the highest fracture toughness values, which is due to the low diffusion of water and lipid molecules, which does not allow a fast separation in the matrix, and therefore, high amounts of micro-cracks do not occur during the extrusion and drying processes of the pastes. This could be explained from the point of view of the formation of intermolecular hydrogen bonds due to cross-linking by protein-protein interactions. Therefore, these interactions could be favored by the reduced amount of lipids and by the temperature applied during the drying cycles (Table 2). In fact, proteins are well known to be susceptible to heat treatment and protein polymerization at 60°C due to oxidation of SH groups, i.e., the intermolecular interactions involved in protein polymerization during heating are mainly free SH, desulphated and non-covalent bonds. In this case, polysaccharides may exhibit stretching of the O-H bond between hydrogen and stretching of the methylene (C-H) bond due to an increase in temperature, which enhances the hydration of the mixture (Mills et al., 2003; Vonhoff et al., 2010; Hu et al., 2011; He et al., 2014; Yin et al., 2019; Zhang et al., 2019). During thermal processing, stretching of the hydroxyl of the biopolymer may occur, leading to increased intermolecular interaction during heating, possibly due to the formation of the starch-lipid complex. The intermolecular content of α-helix and β-sheets increases with increasing temperature, while the content of β-sheets may decrease as a function of temperature. In addition, it has been reported that the β-antiparallel and β-turn sheet content fluctuates during heating. The intermolecular content of the α-helix and β-sheets changes with increasing temperature. One possible explanation may be that when heated between 60 and 100°C, the α-helix increases its intermolecular content, as well as the β-sheet content, which increases as the temperature rises from 60 to 100°C.
The fracturability results (Table 5) could be associated with the resistance of the paste to the pressure exerted for its deformation. The results obtained do not exceed 3.73 mm, with a minimum of 2.08. Figure 1 shows the Young's modulus values calculated for each extruded pulp, showing a correlation with the fracture stress values, corroborating that the micro-cracks possibly arising from the extrusion and drying processes of the pulps depend on the fat and fiber content of the pulps.
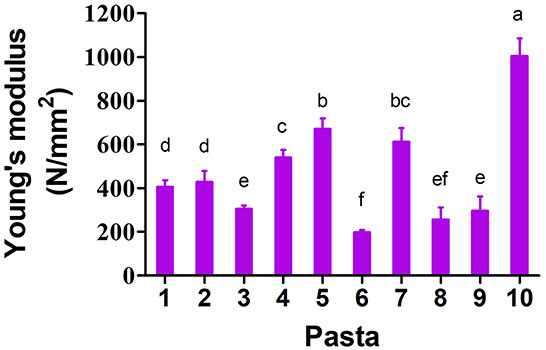
Figure 1. Elastic modulus values for extruded pasta based on hyper-protein quinoa. Results are the mean of ten determinations (n = 10), ± represents the standard deviation. Similar lower-case letters show no statistical difference between treatments (p < 0.05).
Culinary properties
Figure 2 shows the culinary properties of the extruded dough, evaluated by weight increase and mass loss by cooking processes (Table 6), expressed in percentage, with a significant difference of p < 0.05. The analysis of the behavior of these properties was divided according to corn and rice flour content, starch content, HHP content and HHPD content. According to Table 1, all formulations contain similar corn and rice flour content, except for 2 and 3, which do not contain rice and corn, respectively. The content of these flours apparently had no significant effect on the behavior of weight increase and mass loss by cooking in the extruded pasta (Figure 2). However, it appears that weight gain and mass loss in formulations 2 and 3 are dependent on the inclusion of these flours, observing that the non-inclusion of corn flour produces less weight loss by cooking (formulation 3), while the non-inclusion of rice flour (formulation 2) produces an increase in weight loss by cooking. Consequently, in formulations 1 to 4, these contain less starch content, presenting an increase in cooking weight greater than 35% with respect to the other formulations.
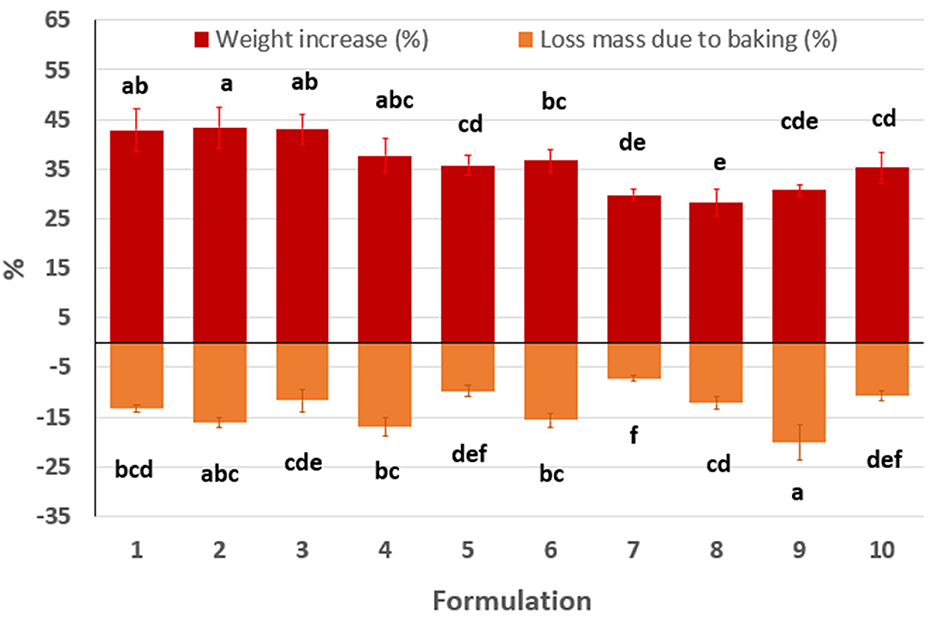
Figure 2. Percentage increase in weight and loss of dough by cooking pasta based on high protein flour. Similar lower-case letters indicate no statistical difference between treatments (p < 0.05).
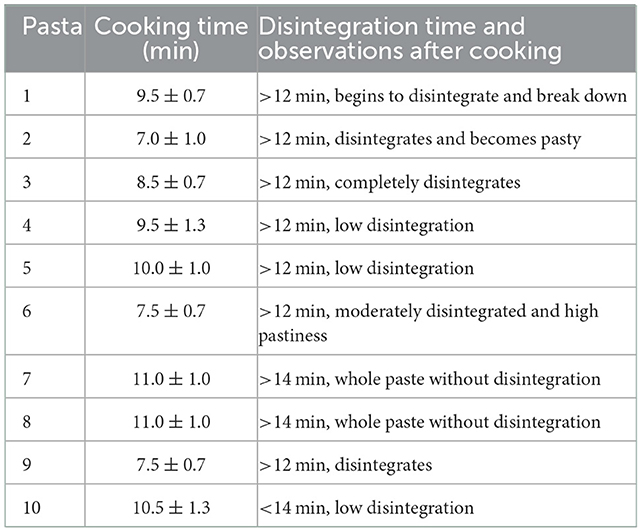
Table 6. Cooking time determined and visual observations after cooking in hyper-protein quinoa-based pasta.
Likewise, it was observed that the behavior in mass loss by cooking, in these formulations, was less than 15%, except formulation 4, which presented a mass loss around 16%, which could be due to the inclusion of HHPD and HHP in a lower percentage than in the other formulations, i.e., the addition of these two flours with higher protein richness and availability probably tends to generate thermodynamic competitivity in the phase of the biphasic system formed by these two macromolecular components, protein and available starch (see Table 2). This happens because the folding of these biopolymers in the mass of a dilute solution or in single-molecule conditions are governed by cooperativity in conformational changes due to the presence of large-scale intermolecular interactions that form multiple structures (Mao and Zhang, 2012; Uyeda et al., 2018).
If the system is formed by a protein-polysaccharide mixture, adsorption is more complex, since there may be competitive adsorption (Damodaran, 2003) and thermodynamic incompatibility (Tolstoguzov, 2003a) of these biopolymers in the blend or interface, i.e., the polysaccharide tends to improve the functionality of the adsorbed proteins (Sengupta and Damodaran, 2000, 2001; Tolstoguzov, 2000, 2003a; Mackie et al., 2001; Damodaran, 2003, 2004). Although polysaccharides are mostly hydrophilic, they promote the stability of protein dispersions, acting as a thickening (viscosity) or gelling agent (Dickinson, 1992). Therefore, the addition of polysaccharides causes an increase in the amount of protein adsorbed at the interface, thus contributing to the formation and stabilization of the dispersions, in this particular case, the interactions necessary to stabilize the pasta (spaghetti) (Yang et al., 2020; Cao et al., 2022; Ning et al., 2022).
Consequently, pH, salt concentration (ionic strength), temperature and molecular weight also affect the thermodynamic compatibility of protein-polysaccharide-water systems, which vary depending on the type of polysaccharide present (Rubio-Ríos et al., 2019; Solanilla Duque et al., 2022). Therefore, as the pH approaches the isoelectric point of the protein, thermodynamic compatibility decreases due to intermolecular interactions between proteins and carboxylic and neutral polysaccharides (Tolstoguzov, 2003a). In all cases, thermodynamic compatibility between proteins and anionic or neutral polysaccharides decreases with increasing polysaccharide molecular weight, salt concentration and temperature. However, when sulfated polysaccharides are present, thermodynamic compatibility is higher when the protein is close to its isoelectric point. Analyzing from the point of view of the starch composition present, we can say that the variation of amylopectin crystallization predicts the staling of the product. Thus, an increase in concentration of dough favors bread staling (Tolstoguzov, 2003b; Ribotta and Rosell, 2010). This behavior may be due to an increase in mass concentration (Ang et al., 2022).
Another explanation for this phenomenon, especially in pasta products after high-temperature drying, is when the volume excluded in the amylopectin recrystallization its effect is observed in the higher content of resistant starch (Sarker et al., 2013; Ogawa and Adachi, 2017; Cimini et al., 2019). In the case of the formulations that do not contain starch (9 and 10), these present differences in the percentage of mass loss, observing a greater increase in formulation 9 with respect to formulation 10. This behavior may be due to the fact that the latter formulation (10) contains HHPD, which contributes to a lower mass loss and a greater increase in cooking weight.
Consequently, the absence of HHP [which contributes lipids in the formulation (Table 2)] and the lack of starch in this formulation (compensated by the higher content of corn and rice flours), make formulation 10 perform better than formulation 9. The mass loss by cooking was more evident in formulation 9, which contained only HHP and 0% added cassava starch (control 1), with a significant difference with control 2 (HHPD and 0% added cassava starch). As for control 1, this could be since cassava starch modified with natural maize starch helps to improve cohesiveness between the different components, in addition to the possible pre-gelatinization of all the starch sources added (maize, rice, quinoa). However, as for control 2, the release of quinoa starch by the defatting process is vital to improve pregelatinization processes and to improve cohesiveness and compactness between mixed raw materials, as observed in the formulations containing only HHPD where they do not exceed 15% mass loss by cooking.
Formulations 1 to 3, including 6 and 9, have a higher HHP content, but do not contain HHPD, while formulations 5, 7 and 10 do not contain HHP but have a higher HHPD content. It should be noted that formulation 5 is low in starch. In relation to these formulations, it was observed that formulations 1 to 3 show better behavior both in weight increase and mass loss by cooking. This behavior may be due to the synergistic interaction between the non-defatted protein (HHP) and the low starch content present due to a greater thermodynamic compatibility between both biopolymers, which is reflected in a greater increase in dough weight by cooking (Cimini et al., 2019). However, it was observed that a higher inclusion of starch in formulation 6 compensates for the increase in weight by cooking, but when comparing this value with formulation 9, it is evident that the lack of starch produces a lower increase in weight and a higher mass loss. As explained above, this behavior is due to the absence of HHP and the lack of starch in this formulation, evidencing that the presence and synergy between both biopolymers (intermolecular interactions) is necessary to improve these culinary properties, as evidenced above. The protein denaturation contributes to gel formation, where the protein-protein interaction allows a better-structured network due to the formation of protein aggregates as protein (Ortiz-Gómez et al., 2022b).
Similarly, the absence or low starch content in the formulation is independent of the synergistic behavior among the blend components, as observed in formulations 5 and 10. In these, it is evident that the increase in cooking weight is possibly compensated by the higher content of corn and rice flours, which would be contributing starch and protein to the blend. Additionally, the absence or low starch content and the presence of defatted protein (HHPD) probably cause a greater thermodynamic compatibility between the macromolecular components of the blend in the two-phase system when these are at lower concentrations (Damodaran, 2003). Contrary case was observed in the behavior of formulation 7, which, in spite of having a greater starch content, presents a lower increase in weight by cooking. Therefore, it is possible that this phenomenon can be attributed to a thermodynamic incompatibility between the HHPD and the starch present, and to a lesser extent, to the lower contribution of macromolecular components of the corn and rice flours contained in the formulation. This could be explained from the blend point of view of the macromolecular components (proteins and polysaccharides) present in the formulation, because eventually there is a limited thermodynamic compatibility between these two biomolecules, probably due to the fact that these two macromolecular components are more or less in the same concentrated single phase of the two-phase system (Appelqvist and Debet, 1997). Another phenomenon that could be happening there is that, being a two-phase system in which the two macromolecular components are apparently in the same concentrated phase, it is probable that an insoluble electrostatic complex of proteins and polysaccharides has partially formed (Warnakulasuriya and Nickerson, 2018; Gałkowska et al., 2021).
It should also be noted that formulation 6, as well as formulations 7 and 8, contain cassava starch higher than 15.6%. The latter two have similar culinary properties, while formulation 6 shows better performance, probably due to a higher HHP content. Formulations 4 and 8 have lower HHP content and similar HHPD content. These last three observations indicate that the adsorption behavior of adequately distributed biopolymers is considered as multiple blends, in which all the individual macromolecular components compete for adsorption on the surface, that, under thermodynamic equilibrium conditions, high affinity compounds are preferentially adsorbed over low affinity compounds (de Gennes, 1980; Stuart et al., 1980; Tolstoguzov, 2003b; Zhou et al., 2021; Ang et al., 2022). However, at concentrations well below surface saturation, molecules find an available adsorption site on the surface, i.e., as the two-phase system becomes saturated, molecules with high affinity adsorb more extensively, while molecules with low affinity adsorb less efficiently and move toward the more liquid phase. Consequently, the excluded volume interactions of the adsorbed molecules directly impact the free energy of the system. When the surface is saturated, the concentration of the components with high affinity to the phase increases establishing a dynamic equilibrium between the adsorbed and dissolved species, adsorbing to give access to another molecule of identical chemical nature at the interface at minimum free energy.
Finally, the presence of multiple components in blends can affect starch or protein behavior during processing through the potential for complex formations. The presence of multiple components in flour is assumed to affect the functionality of protein and starch in blends. For example, the presence of proteins and other components in cereal or pseudocereal-based food systems may affect the dynamic behavior of starch or protein during thermal processing, due to the likelihood of complex formation between these macromolecular components. Consequently, the study of the behavior of protein or starch that is isolated from the flour system cannot fully reflect the intermolecular change and interaction between the blended components during processing as a function of temperature (Tables 6, 7). In future works these formulations will be studied the effect of gums in the stability and mass loss by baking processes.
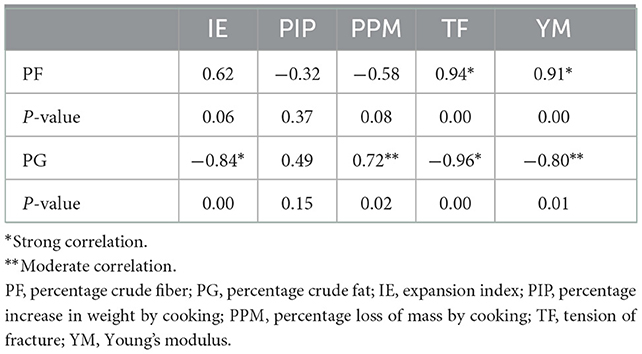
Table 7. Correlation coefficient of fat and fiber composition with physical and culinary properties of pasta based on hyperprotein quinoa.
An important parameter in the culinary properties of the pastes is the final complete cooking time. Table 6 shows the results determined for each sample, as well as incorporating detailed results of the visual studies that were found after finding the cooking time. It was found that in most of the extruded pastes the complete cooking time was between 8 and 10 min, but what could be observed is that in several formulations there is an initial and complete disintegration of the product, leaving only formulations 7 and 8 without any type of disintegration. This could be due to the pre-gelatinization of the high percentage of modified cassava starch added and the use of defatted hyperprotein quinoa flour, which has a lower fat content and greater availability of quinoa starch granules, which improves the fusion and cohesiveness of all the components during extrusion, aided by the pre-gelatinization as mentioned previously.
Pearson's correlation coefficient
The results found in this study have shown how the results vary according to the ether and fiber (Table 2) composition of the formulations in the different techniques used. Table 7 shows the Pearson correlation coefficient analysis, which shows that the percentage of fiber in its composition only has a direct and strong effect (p < 0.05) with the textural characterization (fracture toughness and Young's modulus). With respect to the percentage of fat, it is evident that it has a strong (p < 0.05) indirect influence on the expansion index (Table 3) and fracture stress, with a moderate correlation (p > 0.05) and also indirect with the calculated Young's modulus, which indicates that, at high percentages of fat in the pasta formulations, these evaluated parameters would decrease, while the percentage of mass loss by cooking would increase directly (p > 0.05) when increasing the % of crude fat added. These observations are in agreement with the culinary properties reported above. Where the textural behavior of the pastes, are dependent on the different blends made in the formulations.
Conclusion
In general, formulations 6, 7 and 8, with the highest fat and starch contents, showed a high expansion index. In addition, due to these components, it showed better brightness values. The texture of the extruded pastes improved significantly with the addition of gums and was temperature dependent. Furthermore, it was observed that its behavior was influenced by the relationship between protein availability and fat and starch content. According to these results, the incorporation of HHPD improves the techno-functional properties of the dough, making it an excellent alternative to generate foods with high protein value. The drying process should be optimized because it directly influences the textural properties and shelf life of the product. Therefore, in the drying process the aw should be around 0.6 to ensure product safety. Consequently, the conclusion is that the sorption and desorption curves should be evaluated to establish the relationship between water content and aw. These variables will be the subject of future studies to determine the behavior of these thermophysical properties of the paste as a function of temperature, moisture content and salt concentration (NaCl).
Data availability statement
The raw data supporting the conclusions of this article will be made available by the authors, without undue reservation.
Author contributions
All authors listed have made a substantial, direct, and intellectual contribution to the work and approved it for publication.
Funding
Project Quinoa financed by the Sistema General de Regalías – SGR, Departamento Nacional de Planeación (DNP) Colombia, with BPIN number 2020000100052.
Acknowledgments
The authors acknowledge support from Project Quinoa (SGR) BPIN 2020000100052 and ID code 5637, Universidad del Cauca.
Conflict of interest
The authors declare that the research was conducted in the absence of any commercial or financial relationships that could be construed as a potential conflict of interest.
Publisher's note
All claims expressed in this article are solely those of the authors and do not necessarily represent those of their affiliated organizations, or those of the publisher, the editors and the reviewers. Any product that may be evaluated in this article, or claim that may be made by its manufacturer, is not guaranteed or endorsed by the publisher.
References
Ang, C. L., Goh, K. K. T., Lim, K., and Matia-Merino, L. (2022). High-protein foods for dysphagia: manipulation of mechanical and microstructural properties of whey protein gels using de-structured starch and salts. Gels 8, 399. doi: 10.3390/gels8070399
AOAC (2000). Official Methods of Analysis (M. Gaithersburg, ed.; 17th Edn.). Association of Official Analytical Chemists.
Appelqvist, I. A. M., and Debet, M. R. M. (1997). Starch-biopolymer interactions—a review, Food Rev. Int. 13, 163–224. doi: 10.1080/87559129709541105
Aribas, M., Kahraman, K., and Koksel, H. (2020). In vitro glycemic index, bile acid binding capacity and mineral bioavailability of spaghetti supplemented with resistant starch type 4 and wheat bran. J. Func. Foods 65, 103778. doi: 10.1016/j.jff.2020.103778
Boukid, F., Rosell, C. M., Rosene, S., Bover-Cid, S., and Castellari, M. (2021). Non-animal proteins as cutting-edge ingredients to reformulate animal-free foodstuffs: present status and future perspectives. Crit. Rev. Food. Sci. Nutr. 62:23, 6390–6420, doi: 10.1080/10408398.2021.1901649
Brandolini, A., Lucisano, M., Mariotti, M., and Hidalgo, A. (2018). A study on the quality of einkorn (Triticum monococcum L. ssp. monococcum) pasta. J. Cereal Sci. Monococcum Pasta 82, 57–64. doi: 10.1016/j.jcs.2018.05.010
Brites, L. T. G. F., Schmiele, M., and Steel, C. J. (2018). Gluten-free bakery and pasta products. Alter. Replace. Foods 17, 385–410. doi: 10.1016/B978-0-12-811446-9.00013-7
Bruns, A. J., and Bourne, M. C. (1975). Effects of sample dimensions on the snapping force of crisp foods. J. Texture Stud. 6, 445–458. doi: 10.1111/j.1745-4603.1975.tb01420.x
Cao, G., Chen, X., Wang, N., Tian, J., Song, S., Wu, X., et al. (2022). Effect of konjac glucomannan with different viscosities on the quality of surimi-wheat dough and noodles. Int. J. Biol. Macromol. 221, 1228–1237. doi: 10.1016/j.ijbiomac.2022.09.024
Chen, J., Yang, S., Zhang, M., Shan, C., and Chen, Z. (2022). Effects of potato starch on the characteristics, microstructures and quality attributes of indica rice flour and instant rice noodles. Int. J. Food Sci. Technol. 57, 2285–2297. doi: 10.1111/ijfs.15580
Cimini, A., Cibelli, M., and Moresi, M. (2019). Reducing the cooking water-to-dried pasta ratio and environmental impact of pasta cooking. J. Sci. Food Agric. 99, 1258–1266. doi: 10.1002/jsfa.9299
Cueto, M., Porras-Saavedra, J., Farroni, A., Alamilla-Beltrán, L., Schöenlechner, R., Schleining, G., et al. (2015). Physical and mechanical properties of maize extrudates as affected by the addition of chia and quinoa seeds and antioxidants. J. Food Eng. 167, 139–146. doi: 10.1016/j.jfoodeng.2015.07.027
Culetu, A., Duta, D. E., Papageorgiou, M., Varzakas, T., and Rosenberg, M. (2021). The Role of hydrocolloids in gluten-free bread and pasta; rheology, characteristics, staling and glycemic index. Foods 10, 3121. doi: 10.3390/foods10123121
Curiel, J. A., Coda, R., Limitone, A., Katina, K., Raulio, M., Giuliani, G., et al. (2014). Manufacture and characterization of pasta made with wheat flour rendered gluten-free using fungal proteases and selected sourdough lactic acid bacteria. J. Cereal Sci. 59, 79–87. doi: 10.1016/j.jcs.2013.09.011
Damodaran, S. (2003). Competitive adsorption and thermodynamic incompatibility of mixing of β-casein and gum arabic at the air–water interface. Food Hydrocolloids 17, 355–363. doi: 10.1016/S0268-005X(02)00098-X
Damodaran, S. (2004). Adsorbed layers formed from mixtures of proteins. Curr. Opin. Colloid Interface Sci. 9, 328–339. doi: 10.1016/j.cocis.2004.09.008
de Gennes, P. G. (1980). Conformations of polymers attached to an interface. Macromolecules 13, 1069–1075. doi: 10.1021/ma60077a009
di Pede, G., Dodi, R., Scarpa, C., Brighenti, F., Dall'asta, M., and Scazzina, F. (2021). Glycemic index values of pasta products: an overview. Foods 10, 2541. doi: 10.3390/foods10112541
Dziki, D. (2021). Current trends in enrichment of wheat pasta: quality, nutritional value and antioxidant properties. Processes 9, 1280. doi: 10.3390/pr9081280
Faber, I., Henn, K., Brugarolas, M. M. B., and Perez-Cueto, F. J. A. (2021). Relevant characteristics of food products based on alternative proteins according to European consumers. J. Sci. Food Agric. 102, 5034–5043. doi: 10.1002/jsfa.11178
Galanakis, C. M. (2019). Lipids and Edible Oils: Properties, Processing and Applications. London: Academic Press.
Gałkowska, D., Dudycz, A., and Juszczak, L. (2021). Effect of potato protein on thermal and rheological characteristics of maize starches with different amylose contents. Starch-Stärke 73, 2000216. doi: 10.1002/star.202000216
Gao, Y., Janes, M. E., Chaiya, B., Brennan, M. A., Brennan, C. S., Prinyawiwatkul, W., et al. (2018). Gluten-free bakery and pasta products: prevalence and quality improvement. Int. J. Food Sci. Technol. 53, 19–32. doi: 10.1111/ijfs.13505
García-Parra, M., Roa-Acosta, D., and Bravo-Gómez, J. E. (2022). Effect of the altitude gradient on the physiological performance of quinoa in the Central Region of Colombia. Agronomy 12, 2112. doi: 10.3390/agronomy12092112
García-Parra, M., Roa-Acosta, D., García-Londoño, V., Moreno-Medina, B., and Bravo-Gomez, J. (2021). Structural characterization and antioxidant capacity of quinoa cultivars using techniques of FT-MIR and UHPLC/ESI-Orbitrap MS spectroscopy. Plants 10, 2159. doi: 10.3390/plants10102159
García-Parra, M., Zurita-Silva, A., Stechauner-Rohringer, R., Roa-Acosta, D., and Jacobsen, S. E. (2020). Quinoa (Chenopodium quinoa Willd.). and its relationship with agroclimatic characteristics: a Colombian perspective. Chilean J. Agric. Res. 80, 290–302. doi: 10.4067/S0718-58392020000200290
He, R., He, H. Y., Chao, D., Ju, X., and Aluko, R. (2014). Effects of high pressure and heat treatments on physicochemical and gelation properties of rapeseed protein isolate. Food Bioprocess Technol. 7, 1344–1353. doi: 10.1007/s11947-013-1139-z
Hu, X., Shmelev, K., Sun, L., Gil, E. S., Park, S. H., Cebe, P., et al. (2011). Regulation of silk material structure by temperature-controlled water vapor annealing. Biomacromolecules 12, 1686–1696. doi: 10.1021/bm200062a
Lorenzo, G., Sosa, M., and Califano, A. (2018). Alternative proteins and pseudocereals in the development of gluten-free pasta. Altern. Replace. Foods 17, 433–458. doi: 10.1016/B978-0-12-811446-9.00015-0
Lorusso, A., Verni, M., Montemurro, M., Coda, R., Gobbetti, M., Rizzello, C. G., et al. (2017). Use of fermented quinoa flour for pasta making and evaluation of the technological and nutritional features. LWT 78, 215–221. doi: 10.1016/j.lwt.2016.12.046
Mackie, A. R., Gunning, A. P., Ridout, M. J., Wilde, P. J., and Morris, V. J. (2001). Orogenic displacement in mixed β-Lactoglobulin/β-Casein films at the air/water interface. Langmuir 17, 6593–6598. doi: 10.1021/la010687g
Mao, Y., and Zhang, J. (2012). Understanding thermodynamic competitivity between biopolymer folding and misfolding under large-scale intermolecular interactions. J. Am. Chem. Soc. 134, 631–639. doi: 10.1021/ja209534c
Mills, E. N. C., Marigheto, N. A., Wellner, N., Fairhurst, S. A., Jenkins, J. A., Mann, R., et al. (2003). Thermally induced structural changes in glycinin, the 11S globulin of soya bean (Glycine max)—an in situ spectroscopic study. Biochimica et Biophysica Acta (BBA)-Proteins and Proteomics 1648, 105–114. doi: 10.1016/S1570-9639(03)00114-6
Montemurro, M., Pontonio, E., and Rizzello, C. G. (2019). “Quinoa flour as an ingredient to enhance the nutritional and functional features of cereal-based foods,” in Flour and Breads and Their Fortification in Health and Disease Prevention, eds. V. Watson, R. Preedy, and R. Ross (New York, NY: Academic Press; Elsevier), 453–464. Available online at: https://linkinghub.elsevier.com/retrieve/pii/B9780128146392000368
Muñoz-Pabon, K. S., Parra-Polanco, A. S., Roa-Acosta, D. F., Hoyos-Concha, J. L., and Bravo-Gomez, J. E. (2022). Physical and paste properties comparison of four snacks produced by high protein quinoa flour extrusion cooking. Front. Sustain. Food Sys. 6, 1–10. doi: 10.3389/fsufs.2022.852224
Ning, X., Zhou, Y., Wang, Z., Zheng, X., Pan, X., Chen, Z., et al. (2022). Evaluation of passion fruit mesocarp flour on the paste, dough, and quality characteristics of dried noodles. Food Sci. Nutr. 10, 1657–1666. doi: 10.1002/fsn3.2788
Ogawa, T., and Adachi, S. (2017). Drying and rehydration of pasta. Drying Technol. 35, 1919–1949. doi: 10.1080/07373937.2017.1307220
Ortiz-Gómez, V., Fernández-Quintero, A., Roa-Acosta, D. F., Bravo-Gómez, J. E., and Solanilla-Duque, J. F. (2022a). Physicochemical characterization of quinoa (chenopodium quinoa cv. nariño) co-products obtained by wet milling. Front. Sustain. Food Syst. 6, 851433. doi: 10.3389/fsufs.2022.851433
Ortiz-Gómez, V., Nieto-Calvache, J. E., Roa-Acosta, D. F., Solanilla-Duque, J. F., and Bravo-Gómez, J. E. (2022b). Preliminary characterization of structural and rheological behavior of the quinoa hyperprotein-defatted flour. Front. Sustain. Food Syst. 6, 852332. doi: 10.3389/fsufs.2022.85233
Ramos-Diaz, J. M., Kince, T., Sabovics, M., Gürbüz, G., Rauma, A., Lampi, A. M., et al. (2020). Relationship of compositional, mechanical, and textural properties of gluten-free pasta using different quinoa (Chenopodium quinoa) varieties. Foods 9, 1–16. doi: 10.3390/foods9121849
Ribotta, P. D., and Rosell, C. M. (2010). Effects of enzymatic modification of soybean protein on the pasting and rheological profile of starch-protein systems. Starch-Stärke 62, 373–383. doi: 10.1002/star.200900259
Roa-Acosta, D. F., Bravo-Gómez, J. E., García-Parra, M. A., Rodríguez-Herrera, R., and Solanilla-Duque, J. F. (2020). Hyper-protein quinoa flour (Chenopodium Quinoa Wild): monitoring and study of structural and rheological properties. LWT 121, 108952. doi: 10.1016/j.lwt.2019.108952
Rolandelli, G., García-Navarro, Y. T., García-Pinilla, S., Farroni, A. E., Gutiérrez-López, G. F., Buera, M., et al. (2020). Microstructural characteristics and physical properties of corn-based extrudates affected by the addition of millet, sorghum, quinoa and canary seed flour. Food Struct. 25, 100140. doi: 10.1016/j.foostr.2020.100140
Rubio-Ríos, A., Rosales-Marines, L., Solanilla-Duque, J. F., Reyes-Acosta, Y. K., Salazar-Sánchez, M. D. R., Rodríguez-Herrera, R., et al. (2019). “Biobased nanoemulsions: concept, formulation, and applications,” in Nanobiotechnology in Bioformulations. First., eds. P. Ram, et al. (USA: Springer), pp. 1–31. doi: 10.1007/978-3-030-17061-5_1
Saeleaw, M., and Schleining, G. (2010). Effect of blending cassava starch, rice, waxy rice and wheat flour on physico-chemical properties of flour mixtures and mechanical and sound emission properties of cassava crackers. J. Food Eng. 100, 12–24. doi: 10.1016/j.jfoodeng.2010.03.020
Sarker, M. Z. I., Elgadir, M. A., Ferdosh, S., Akanda, M. J. H., Aditiawati, P., and Noda, T. (2013). Rheological behavior of starch-based biopolymer mixtures in selected processed foods. Starch-Stärke 65, 73–81. doi: 10.1002/star.201200072
Sengupta, T., and Damodaran, S. (2000). Incompatibility and phase separation in a bovine serum albumin/β-Casein/water ternary film at the air–water interface. J. Colloid Interface Sci. 229, 21–28. doi: 10.1006/jcis.2000.6992
Sengupta, T., and Damodaran, S. (2001). Lateral phase separation in adsorbed binary protein films at the air–water interface. J. Agric. Food Chem. 49, 3087–3091. doi: 10.1021/jf001111k
Solanilla Duque, J. F., Carrera, C., and Patino, J. M. R. (2022). Effect of pH on the interfacial and foaming properties of Maillard reaction-modified proteins. Biophys. Chem. 291, 106906. doi: 10.1016/j.bpc.2022.106906
Sosa, M., Califano, A., and Lorenzo, G. (2018). Influence of quinoa and zein content on the structural, rheological, and textural properties of gluten-free pasta. Eur. Food Res. Technol. 245, 343–353. doi: 10.1007/s00217-018-3166-5
Stuart, M. A. C., Scheutjens, J. M. H. M., and Fleer, G. J. (1980). Polydispersity effects and the interpretation of polymer adsorption isotherms. J. Polymer Sci.: Polymer Phys. Edn. 18, 559–573. doi: 10.1002/pol.1980.180180315
Tiga, B. H., Kumcuoglu, S., Vatansever, M., and Tavman, S. (2021). Thermal and pasting properties of quinoa—wheat flour blends and their effects on production of extruded instant noodles. J. Cereal Sci. 97, 103120. doi: 10.1016/j.jcs.2020.103120
Tolstoguzov, V. (2000). Phase behaviour of macromolecular components in biological and food systems. Nahrung/Food 44, 299–308. doi: 10.1002/1521-3803(20001001)44:5<299::AID-FOOD299>3.0.CO;2-9
Tolstoguzov, V. (2003a). Some thermodynamic considerations in food formulation. Food Hydrocolloids 17, 1–23. doi: 10.1016/S0268-005X(01)00111-4
Tolstoguzov, V. (2003b). Thermodynamic considerations of starch functionality in foods. Carbohydrate Polymers 51, 99–111. doi: 10.1016/S0144-8617(02)00171-6
Torres, O. L., Lema, M., and Galeano, Y. V. (2021). Effect of using quinoa flour (Chenopodium quinoa Willd.) on the physicochemical characteristics of an extruded pasta. Int. J. Food Sci. 2021, 8813354. doi: 10.1155/2021/8813354
Uyeda, T. Q., Ngo, K. X., Kodera, N., and Tokuraku, K. (2018). “Uni-directional propagation of structural changes in actin filaments,” in The Role of Water in ATP Hydrolysis Energy Transduction by Protein Machinery. First., ed. M. Suzuki (Singapore: Springer Singapore), 157–177. doi: 10.1007/978-981-10-8459-1_11
Vonhoff, S., Condliffe, J., and Schiffter, H. (2010). Implementation of an FTIR calibration curve for fast and objective determination of changes in protein secondary structure during formulation development. J. Pharmaceut. Biomed. Anal. 51, 39–45. doi: 10.1016/j.jpba.2009.07.031
Warnakulasuriya, S. N., and Nickerson, M. T. (2018). Review on plant protein-polysaccharide complex coacervation, and the functionality and applicability of formed complexes. J. Sci. Food Agric. 98, 5559–5571. doi: 10.1002/jsfa.9228
Yang, X., Li, A., Li, X., Sun, L., and Guo, Y. (2020). An overview of classifications, properties of food polysaccharides and their links to applications in improving food textures. Trends Food Sci. Technol. 102, 1–15. doi: 10.1016/j.tifs.2020.05.020
Yin, H., Jia, F., Huang, J., Zhang, Y., Zheng, X., and Zhang, X. (2019). Effect of extrusion on the structure and antigenicity of soybean β-conglycinin. Grain Oil Sci. Technol. 2, 67–72. doi: 10.1016/j.gaost.2019.09.003
Yu, X., Vargas, J., Green, P. H. R., and Bhagat, G. (2021). Innate lymphoid cells and celiac disease: current perspective. Cell. Mol. Gastroenterol. Hepatol. 11, 803–814. doi: 10.1016/j.jcmgh.2020.12.002
Zhang, J., Liu, L., Liu, H., Yoon, A., Rizvi, S. S. H., Wang, Q., et al. (2019). Changes in conformation and quality of vegetable protein during texturization process by extrusion. Crit. Rev. Food Sci. Nutr. 59, 3267–3280. doi: 10.1080/10408398.2018.1487383
Keywords: hyperprotein, starch/fat, quinoa, gluten-free, texture, paste
Citation: Córdoba-Cerón DM, Carranza-Saavedra D, Roa-Acosta DF, Hoyos-Concha JL and Solanilla-Duque JF (2022) Physical and culinary analysis of long gluten-free extruded pasta based on high protein quinoa flour. Front. Sustain. Food Syst. 6:1017324. doi: 10.3389/fsufs.2022.1017324
Received: 11 August 2022; Accepted: 30 November 2022;
Published: 22 December 2022.
Edited by:
Didier Bazile, Centre de Coopération Internationale en Recherche Agronomique pour le Développement (CIRAD), FranceReviewed by:
Lena Gálvez Ranilla, Universidad Católica de Santa María, PeruChanthima Phungamngoen, Khon Kaen University, Thailand
Copyright © 2022 Córdoba-Cerón, Carranza-Saavedra, Roa-Acosta, Hoyos-Concha and Solanilla-Duque. This is an open-access article distributed under the terms of the Creative Commons Attribution License (CC BY). The use, distribution or reproduction in other forums is permitted, provided the original author(s) and the copyright owner(s) are credited and that the original publication in this journal is cited, in accordance with accepted academic practice. No use, distribution or reproduction is permitted which does not comply with these terms.
*Correspondence: Jose Fernando Solanilla-Duque, jsolanilla@unicauca.edu.co