Soil Microbes Determine Outcomes of Pathogenic Interactions Between Radopholus similis and Fusarium oxysporum V5w2 in Tissue Culture Banana Rhizospheres Starved of Nitrogen, Phosphorus, and Potassium
- Laboratory of Entomology, Wageningen University & Research, Wageningen, Netherlands
The contributions of soil biota toward outcomes of pathogenic interactions between Radopholus similis and Fusarium oxysporum V5w2 in tissue culture banana plants starved of nitrogen (N), phosphorus (P), and potassium (K) were investigated. The study was based on three screenhouse factorial experiments (2 × 2 × 2) comprising of potted banana plants with or without R. similis, with or without F. oxysporum V5w2, and either grown in sterile or non-sterile soil. All plants in each of the three experiments received nutrient solutions that were deficient in N, P, or K, respectively. In all the three nutritional regimes, plants inoculated with R. similis were heavily colonized by the nematode with high percentage dead roots and necrosis, while their root biomasses were low. N-starved plants co-inoculated with R. similis and F. oxysporum V5w2 had lower percentage dead roots and tended to have numerically lower nematode density compared to those treated with R. similis only, especially in non-sterile soil. N-starved plants inoculated with R. similis had higher shoot dry weight, were taller with more leaves that were larger, compared to those not inoculated with the nematode. Plants grown in non-sterile soil had lower percentage dead roots, necrosis and R. similis density than those from sterile soil, regardless of the nutrient regime. N-starved plants from non-sterile soil were shorter with smaller leaves having decreased chlorophyll content and lower biomass, compared to those from sterile soil. By contrast, P and K starved plants from non-sterile soil were taller with larger leaves and more biomass, compared to those from sterile soil. Roots inoculated with R. similis had higher endophytic colonization by Fusarium spp., especially when co-inoculated with F. oxysporum V5w2 and grown in sterile soil among the N and K-starved plants. In conclusion, pathogenic interactions between R. similis and F. oxysporum V5w2 are predominantly suppressed by a complex of soil microbes that exert plant growth promoting effects in tissue culture banana plants through N, P, and K dependent processes. Nitrogen is the most important limiting factor in rhizosphere interactions between banana roots, beneficial microbes and the pathogens. Soil sterilization and the stringent aseptic tissue culture techniques still require the development of alternative innovative ways of conserving microbial services for sustainable agriculture.
Introduction
Soil-borne microbial and invertebrate pests and diseases are a major problem to crop production around the world. Of particular importance are disease complexes involving plant-parasitic nematodes and numerous fungal pathogen strains that cause root rots and wilt diseases (Back et al., 2002; Zhang et al., 2020). In banana production, Radopholus similis is among a complex of plant-parasitic nematodes that interact with the Fusarium of Banana Complex (FOBC) in causing root rots and wilt diseases (Speijer and Sikora, 1993; Ochieno, 2020; Rocha et al., 2020). Control of such disease complexes has focused on developing pest and disease resistant banana varieties (Tripathi et al., 2017; Siamak and Zheng, 2018), and multiplying them as clean planting material through aseptic cell and tissue culture technologies (Almekinders et al., 2019; Jacobsen et al., 2019).
Aseptic tissue culture protocols target the elimination of complexes of non-beneficial endophytic and soil-derived biota that are associated with plant growth suppression (Cassells, 2012; Kumar et al., 2021). Endophytic and rhizosphere plant growth promoting microbes are re-introduced to restore their beneficial functions lost through aseptic tissue culture and soil sterilization techniques (Niere et al., 1999; Sikora and Pocasangre, 2006; Sikora et al., 2008; Soumare et al., 2021). For instance, Fusarium oxysporum strain V5w2 is among endophytic microbes that have successfully been re-introduced into tissue culture banana plants and transferred to farmers in East Africa (Dubois et al., 2006, 2011; zum Felde, 2011). Induced systemic resistance is among the key mechanisms said to be involved in pest suppression by F. oxysporum V5w2 among other microbial biological control agents (Vu et al., 2006; Paparu et al., 2007, 2009a,b, 2010; Bubici et al., 2019; Damodaran et al., 2020). However, future research is still necessary to elucidate the mechanisms underpinning nematode suppression and increased banana yield observed after treatment with F. oxysporum V5w2 (Kisaakye et al., 2022). This is partly because F. oxysporum V5w2 has been reported to be a non-beneficial endophyte that interacts with R. similis in a wilt disease complex of banana (Ochieno, 2020, 2021).
Assessments of the improved banana varieties for resistance to soil-borne pests and diseases, as well as the function of plant growth promoting microbes, have been primarily performed through controlled screenhouse experiments utilizing potted plants and sterilized soil substrates (Dubois et al., 2004; Paparu et al., 2009b; Waweru et al., 2013). This way, integrated pest management (IPM) strategies for banana are developed.
Experiments with potted plants have often produced varied results. For instance, infections by strains of Fusarium oxysporum have been associated with effects ranging from being positive, neutral, or negative toward the host banana plants and nematode pests (Athman et al., 2007; Paparu et al., 2009b; Ochieno, 2010, 2020). Studies that only feature potted banana plants and nematode pests in sterile soil fall short of information on the contribution of fungal pathogens toward parasitism (Hasan, 1993; Ochieno, 2010, 2020). This is also the case for studies that assess potted banana plants and fungal pathogens in sterile soil without plant-parasitic nematodes (Ochieno, 2010, 2020; Khan and Sharma, 2020). These two previous scenarios fail to address the natural interactions between plant-parasitic nematodes and fungal pathogens in root disease complexes. Moreover, possible roles of rhizosphere biota that exist in agroecosystems cannot be addressed through experiments that only feature sterilized soil (Ochieno, 2010, 2020; Kuijken et al., 2015; Jayaraman et al., 2021). Sterile soil may still be recolonized by different microbes (Li et al., 2019), while the axenic explants may still contain endophytic microbes or get re-infected (Ochieno, 2010, 2020; Thomas and Sekhar, 2017; Kithaku et al., 2019).
Abiotic factors especially nutrient compounds have been included into experiments to clarify their effects on interactions between plants, antagonistic and beneficial soil biota. This includes approaches that involve nutrient omission experimental treatments (Kolawole et al., 2018; Mugo et al., 2021). Where nutrient uptake by potted plants is not addressed, depletion of such resources could lead to variations in results. Comparison of plant-microbe-nematode interactions under different nutrient regimes produces varied results with different interpretations (Ochieno, 2010). Although differences in nutrient content and concentration are usually assumed, variations in other physico-chemical properties of the formulations including pH, salinity, conductivity, and toxicity may also affect the outcomes. Therefore, there is dilemma on how results from experiments utilizing nutrient formulations varying in multiple physico-chemical attributes should be interpreted (Ochieno, 2010). This is even further confounded by changes in soil physico-chemical composition that result from sterilization (De Deyn et al., 2004; Gundale et al., 2019; Dietrich et al., 2020). Experiments on plant-microbe-nematode interactions would therefore be better interpreted when conducted under a standard nutrient regime (Lancien et al., 1999; Shin et al., 2005; Ochieno, 2010), while addressing the contribution of rhizosphere microbes (De Rooij-van der Goes, 1995; Troelstra et al., 2001; Ochieno, 2010, 2020).
The present work is part of studies that are aimed at providing information on interactions between plant-parasitic nematodes and fungal pathogens, with specific focus on R. similis and F. oxysporum V5w2 in tissue culture banana (Ochieno, 2020). The studies explore how the two root parasites interact with soil biota under nitrogen (N), phosphorus (P), and potassium (K) deficient conditions. It is hypothesized that, pathogenic interactions between R. similis and F. oxysporum V5w2 under N, P, and K deficient conditions are enhanced by a complex of non-beneficial soil microbes that exert plant growth suppressive effects in tissue culture banana plants.
Materials and Methods
Experimental Design
Three experiments were conducted that comprised full factorial treatments (2 × 2 × 2) derived from banana plants either inoculated with F. oxysporum V5w2 or not, inoculated with R. similis or not, and either grown in sterile or non-sterile soil, hence resulting in eight treatment combinations (Ochieno, 2010). Each of the eight treatment combinations had 15 plants repeated twice over time as the replicates. The banana plants were completely randomized in the screen house. All plants in the three experiments were either supplied with nitrogen-deficient, phosphorus-deficient, or potassium-deficient nutrient solution, respectively.
Banana Plants
Tissue culture banana plants (genomic group AAA-EA, cv. Kibuzi; Onguso et al., 2004; Akankwasa et al., 2021), were obtained from the Tissue Culture Laboratory at the Sendusu Field Station of the International Institute of Tropical Agriculture in Uganda (IITA-Uganda). The plants had been micro-propagated by the shoot-tip culture technique in which plants are produced by multiplication of the meristematic tissue of banana corms (Vuylsteke, 1998). The banana plants had been maintained for 6 weeks in nutrient solution (1 g/L, Polyfeed™, Haifa Chemicals, Israel), after which they were graded into four sizes and equally distributed to the eight treatment groups of 15 plants. Four groups were marked for inoculation with F. oxysporum V5w2 and the other four were controls.
Pre-planting initial plant growth parameters were recorded through a slight modification of the method described by Akello et al. (2007) and Paparu et al. (2009b). Initial plant height (the distance from the base of the plant to the youngest leaf axil), initial number of open leaves, and initial width (widest part of the lamina) and initial length (the distance from the leaf apex to the leaf stalk) of the largest open leaf were recorded using a measuring tape. Initial fresh weight of each plant was measured using a toploading laboratory balance (Mettler Toledo™, Columbus, Ohio, United States), and the number of healthy-looking functional roots recorded.
Pre-planting initial plant growth-related parameters (mean ± SE) in the three experiments did not vary between the treatments. In the experiment with N-starved banana plants, the initial measurements were fresh weight (8.5 ± 0.24 g), plant height (5.3 ± 0.15 cm), number of leaves (3.3 ± 0.05), leaf length (12.4 ± 0.19 cm), leaf width (4.8 ± 0.08 cm), and number of functional roots (3.6 ± 0.07) (n = 340, F-test, p > 0.05). In the experiment with P-starved banana plants, the initial measurements were fresh weight (6.9 ± 0.20 g), plant height (5.5 ± 0.10 cm), number of leaves (3.7 ± 0.04), leaf length (11.2 ± 0.20 cm), leaf width (4.1 ± 0.10 cm), and number of functional roots (3.6 ± 0.08) (n = 350, F-test, p > 0.05). In the experiment with K-starved banana plants, the initial measurements were fresh weight (8.1 ± 0.20 g), plant height (5.6 ± 0.12 cm), number of leaves (3.3 ± 0.03), leaf length (12.7 ± 0.17 cm), leaf width (4.1 ± 0.10 cm), and number of functional roots (4.9 ± 0.08) (n = 376, F-test, p > 0.05).
Fungal Inoculum, Nematodes, and Soil
The study utilized F. oxysporum V5w2 (nit 3), a fungal strain proposed as a biological control agent that suppresses banana pests through induced systemic resistance (Dubois et al., 2011; Kabuye et al., 2011; zum Felde, 2011; Paparu et al., 2013), but has also been found to express mixed pathogenicity in banana plants (Ochieno, 2010, 2020). This fungal strain was originally obtained from a symptomless banana plant in Uganda by Schuster et al. (1995). The fungal inoculum had been maintained on sterile soil culture in the Laboratory of Microbiology at the Sendusu Field Station (IITA-Uganda). Inoculum was prepared by sprinkling the soil culture onto 90 mm diameter Petri dishes containing half-strength PDA (19 g/L, Sigma-Aldrich, Germany). Ten days later, the fungal spores and mycelia were scraped from the media into a 500 mL beaker containing sterile water. The spore mixture was sieved and homogenized, and then adjusted to 1.5 × 106 spores/mL.
Radopholus similis were originally obtained from banana fields at Sendusu Field Station (IITA-Uganda) and the culture was maintained aseptically on carrot discs (Speijer and De Waele, 1997). Nematodes for the experiment were supplied in sterile water suspension containing mixed stages of ~250 R. similis/mL.
Loamy soil was collected within a depth of 10 cm from a 5-year-old unmulched banana field at Sendusu Field Station (IITA-Uganda). The soil was sieved (5 mm aperture) before being thoroughly mixed. Soil samples were analyzed for chemical properties at the National Agricultural Research Laboratories (NARL) in Kawanda, Uganda. Available P and exchangeable K were extracted using the Mehlich-3 method (Mehlich, 1984). Phosphorus (P) in the extract was determined using the molybdenum blue colorimetric method and K using a flame photometer (Okalebo et al., 2002). Total N was analyzed by Kjeldahl oxidation and semi-micro Kjeldahl distillation (Bremner, 1960). Organic matter (OM) was determined using the Walkley-Black method (Walkley, 1947). Soil pH was analyzed using deionized water with a soil to water ratio of 1:2.5. The soil contained N (0.15 %), P (3.6 ppm), K (149 ppm i.e., 0.38 cmolc/kg), OM (2.4 %), and pH 5.1. Part of the loamy soil was steam-sterilized at 100°C for 1 h using an electrode steam conditioner (Marshall-Fowler, South Africa). Analysis of soil chemical properties was not done after sterilization.
Inoculation and Planting
Banana plants were inoculated with F. oxysporum V5w2 by the root-dipping technique (Paparu et al., 2006, 2009a), but without prior breaking of the root tips (Ochieno, 2010). The roots were immersed into a 1.5 L spore suspension contained in tubs (30 × 25 × 15 cm, LWH) for 4 h. Control plants were immersed in distilled water. The plants were grown in 2.5 L buckets with 6 holes at the base containing either sterile or non-sterile soil under screen house conditions (25 ± 3°C, 70–75% RH, 12L: 12D photoperiod). Initial plant heights were recorded. Each plant was supplied with rain water (100 mL) daily, and the respective N-deficient, P-deficient, and K-deficient nutrient solutions (100 mL) once every week. The N, P, and K-deficient nutrient solutions were formulated by omitting the respective elements from the chemical ingredients in the solution prepared by Murashige and Skoog (1962) (Table 1). Twenty (20) days after planting, three holes (5 cm deep) were made into the soil around the base of each plant using a disinfected stick. Each plant was inoculated with ~500 female R. similis in 2 mL of the nematode suspension, distributed across the three holes before covering them with soil.
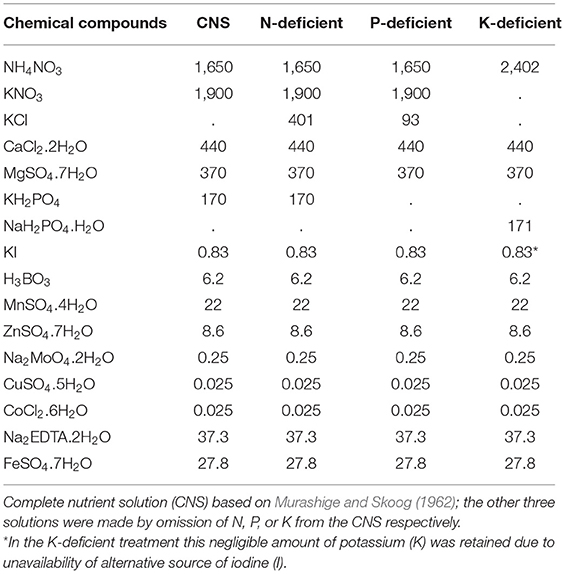
Table 1. Chemical contents (milligrams) of complete nutrient solution (CNS) and those deficient in nitrogen (N), phosphorus (P) and potassium (K).
Plant Growth-Related Parameters
Sixty days after inoculation with R. similis chlorophyll content in the youngest open leaf of every banana plant was measured in SPAD units using a Chlorophyll Meter (SPAD 502, Spectrum Technologies, Illinois, USA; Reeves et al., 1993; Bakaze et al., 2021). Chlorophyll content was only measured in N-starved plants. At 100 days after nematode inoculation, plant height, number of leaves, length and width of youngest open leaf were recorded. Plants were harvested and the number of dead and healthy roots were recorded and used to calculate the percentage dead roots. The shoots and roots were detached and their fresh weights recorded. Root and shoot biomasses were combined for total plant biomass. The shoots were oven-dried at 70°C for a discontinuous period of 14 days to determine their dry weight. The roots were preserved at 4°C awaiting the extraction of nematodes and root-invading microbes. Five roots per plant were randomly selected for assessment of necrosis caused by nematodes, and for estimation of nematode densities. Three roots were randomly selected per plant to estimate the level of endophytic colonization by F. oxysporum V5w2.
Root Necrosis Assessment and Nematode Densities
Root necrosis assessment and quantification of nematode densities were undertaken based on the methods of Speijer and De Waele (1997) and Brooks (2004). Each of the five roots selected per plant for necrosis assessment was cut into 10 cm length. The pieces were then split longitudinally and one half selected from each of them. The cumulative length of necrotic lesions of cortical tissue on each 10 cm side of the stele was recorded as a fraction of the total 20 cm length per root. The sum length of the necrotic tissue for five roots per plant was then expressed as percentage necrosis. These roots were chopped into small pieces and 5 g sub-samples were mixed with 50 mL of water, and macerated at medium speed for 20 s using a Waring® laboratory blender. Nematodes were extracted from the suspension using a Baermann tray for 24 h. The extracts were transferred into 100 mL glass bottles and stored at 4°C for 24 h to allow the nematodes to sediment. The water volume was standardized to 25 mL by gentle siphoning from the surface. The suspension was homogenized by stirring, and nematodes characterized based on identification keys (Fortuner, 1988; Luc et al., 2005), while being quantified on a counting dish at ×10 magnifications from triplicate 2.5 mL pipette sub-samples. Densities of R. similis were expressed as counts per 100 g of root material, while other identified nematode species were not quantified.
Endophytic Colonization by F. oxysporum V5w2
Each of the three roots selected for estimation of endophytic colonization by F. oxysporum V5w2 was surface-sterilized by dipping it into 96% ethanol followed by flaming. This ensured that only microbes within the tissues remained alive. Each root was then cut into six pieces. The root pieces were plated on 60 mm glass Petri dishes containing PDA enriched with KClO3 for 7 days. Growth of Fusarium strains including V5w2 on PDA is characterized by whitish mycelia with pink pigmentation (Leslie and Summerell, 2008). The number of root pieces with growth of Fusarium species among six assessed pieces was recorded. Root piece colonization was the percentage of all root pieces yielding Fusarium among all assessed pieces within a treatment. Other root-invading microbes growing from the root pieces were sub-cultured and morphologically identified through the TSBF-CIAT project on Below-Ground Biodiversity (BGBD) funded by GEF-UNEP at the Soil Microbiology Laboratory, Makerere University, Uganda (Nakintu et al., 2008; Ochieno, 2010).
In vitro growth inhibition tests between the microbes and F. oxysporum V5w2 were conducted. Fusarium oxysporum V5w2 was inoculated in the center of a glass Petri dish containing PDA using a sterile needle. The microbes were inoculated on four spots surrounding the fungal pathogen. The fungal pathogen or the test microbe was considered inhibitive if its mycelia grew into the colonies of the other marked with a boundary between them.
Optical Density of Root Extracts
In the experiment with N-starved plants, it was realized that roots that were stored at 4°C exhibited differences in color after 3 days. Therefore, visual and colorimetric tests on roots and their extracts was conducted to assess possible effects of the experimental treatments on root color. Roots from all plants per treatment were mixed. This was followed by random selection of up to 30 roots from every treatment; the color of the external root surface was visually recorded as either brown or light-brown by comparing with two roots that had been pre-selected as standards for the two colors. For colorimetric assessments, five roots from three plants per treatment were chopped together into a composite sample. This was conducted in triplicate. Ten grams (10 g) of each composite sample were mixed with 100 mL distilled water, and macerated using a Waring® Laboratory Blender (Christison Scientific, Gateshead, UK). The root extracts were diluted (10 ×) and their optical density (absorbance) measured at 320 nm using a spectrophotometer (Genesys 10 UV, Thermo Fisher Scientific, USA).
Statistical Analysis
Data were analyzed using SAS 9.1 software (SAS, 2004). Means were generated by Proc Means. Diagnostic check for normality was conducted using Proc Univariate. Proc Transreg was used to find appropriate Box-Cox transformations, which included generation of suitable powers for data that required transformation, with particular reference to root biomass, total biomass, shoot fresh and dry weight, plant height, number and size leaves. Percentage dead roots and percentage necrosis (x) were arcsine√(x/100) transformed, while untransformed data were used for nematode densities, chlorophyll content and optical density of root extracts. Proc GLM was used for three-way factorial analyses of variance (ANOVA) among the treatments. Mean separation was done by t-tests (LSD) when ANOVA was significant. Root piece colonization by Fusarium was analyzed by Proc Genmod (binomial distribution); Bonferroni-adjusted p-values were obtained by Proc Multtest from raw p-values in Proc Genmod, and used for pairwise comparisons between treatments. Root coloration data were analyzed by Proc Genmod and pairwise comparisons using contrasts.
Results
Experiment With N-Starved Tissue Culture Banana Plants
All plants expressed symptoms that may be linked to N-starvation in the form of being stunted with yellow leaves that had pinkish veins. The leaves dried from the margins toward the midribs, and wilted from the lower older ones, toward the upper young ones. The mean chlorophyll (nitrogen) content of all plants was 29 SPAD units. Plants that were inoculated with R. similis or F. oxysporum V5w2 visually appeared to have greener leaves than those without the nematode, with this tendency being weakly reflected in leaf chlorophyll contents (Figure 1). Plants grown in sterile soil had higher chlorophyll (nitrogen) content than those from non-sterile soil, scoring 31 and 28 SPAD units, respectively (Figure 1). Roots of the banana plants generally appeared light colored, were thin and considerably elongated to densely coil inside the pots, while the soil substrate became sticky and depleted.
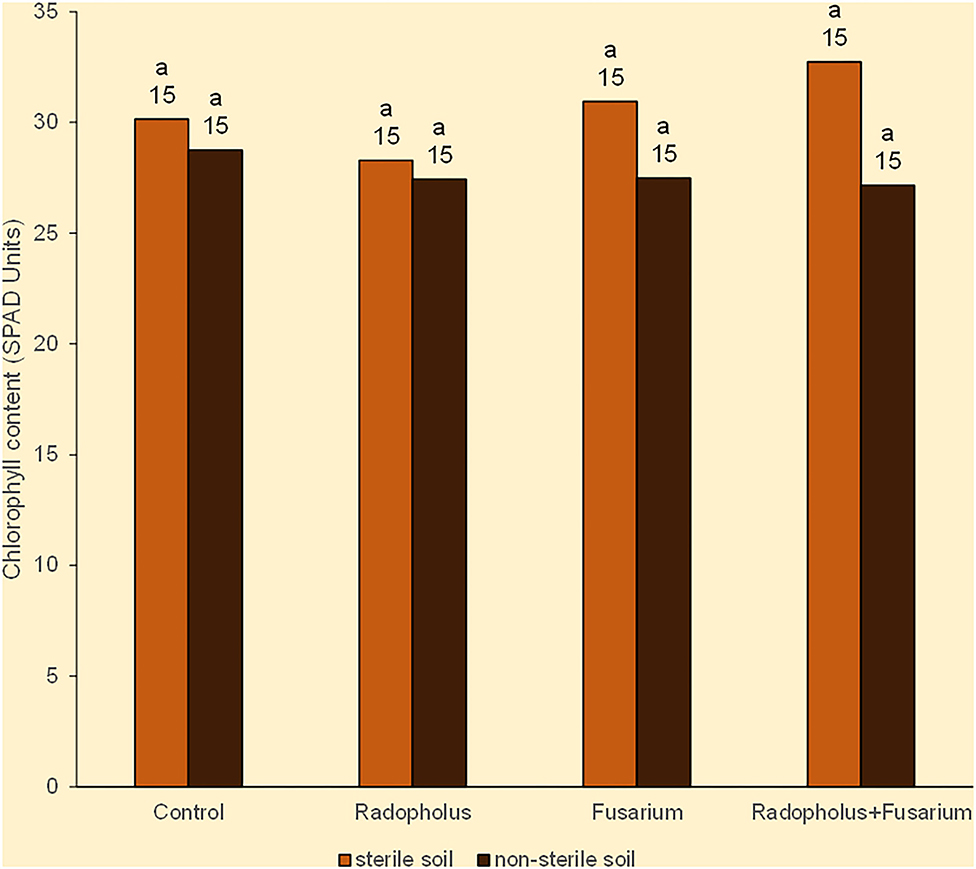
Figure 1. Chlorophyll content in Nitrogen-starved tissue culture banana plants subjected to independent or combined inoculation with Radopholus similis and the Fusarium oxysporum V5w2 and grown in sterile or non-sterile soil. Bars with the same letter represent values that are not statistically different, with the sample sizes indicated below the letters (χ2-test, p < 0.05).
Dead roots, necrosis and R. similis were mainly found in plants that had been inoculated with the nematode. Banana plants inoculated with F. oxysporum V5w2 had lower density of R. similis than those not inoculated with the fungal endophyte (Table 2). R. similis-inoculated plants grown in sterile soil had higher percentage dead roots than those from non-sterile soil (Table 2). Among R. similis-inoculated plants grown in non-sterile soil, those inoculated with F. oxysporum had a lower percentage dead roots than those not inoculated with the fungal endophyte. In contrast, among R. similis-inoculated plants grown in sterile soil, there was no difference in percentage dead roots between F. oxysporum-inoculated plants and those not inoculated with the fungal endophyte. Radopholus similis-inoculated plants grown in sterile soil had a higher percentage root necrosis and higher density of R. similis than those from non-sterile soil (Table 2). Thirteen other nematode species, identified mainly in non-sterile soil among the soil samples, included; the plant parasitic Helicotylenchus multicintus, Meloidogyne spp., Pratylenchus goodeyi, Xiphinema spp., Trophorus spp., Scutellonema spp., Rotylenchus spp., Tylenchus spp., Hemicycliophora spp., Paratrichodorus spp., and Ditylenchus spp. Saprophytic genera included Aphelenchus spp. and Rhabditis spp. among numerous others that could not be identified. The first three nematode species were frequently recorded in root samples from non-sterile soil.
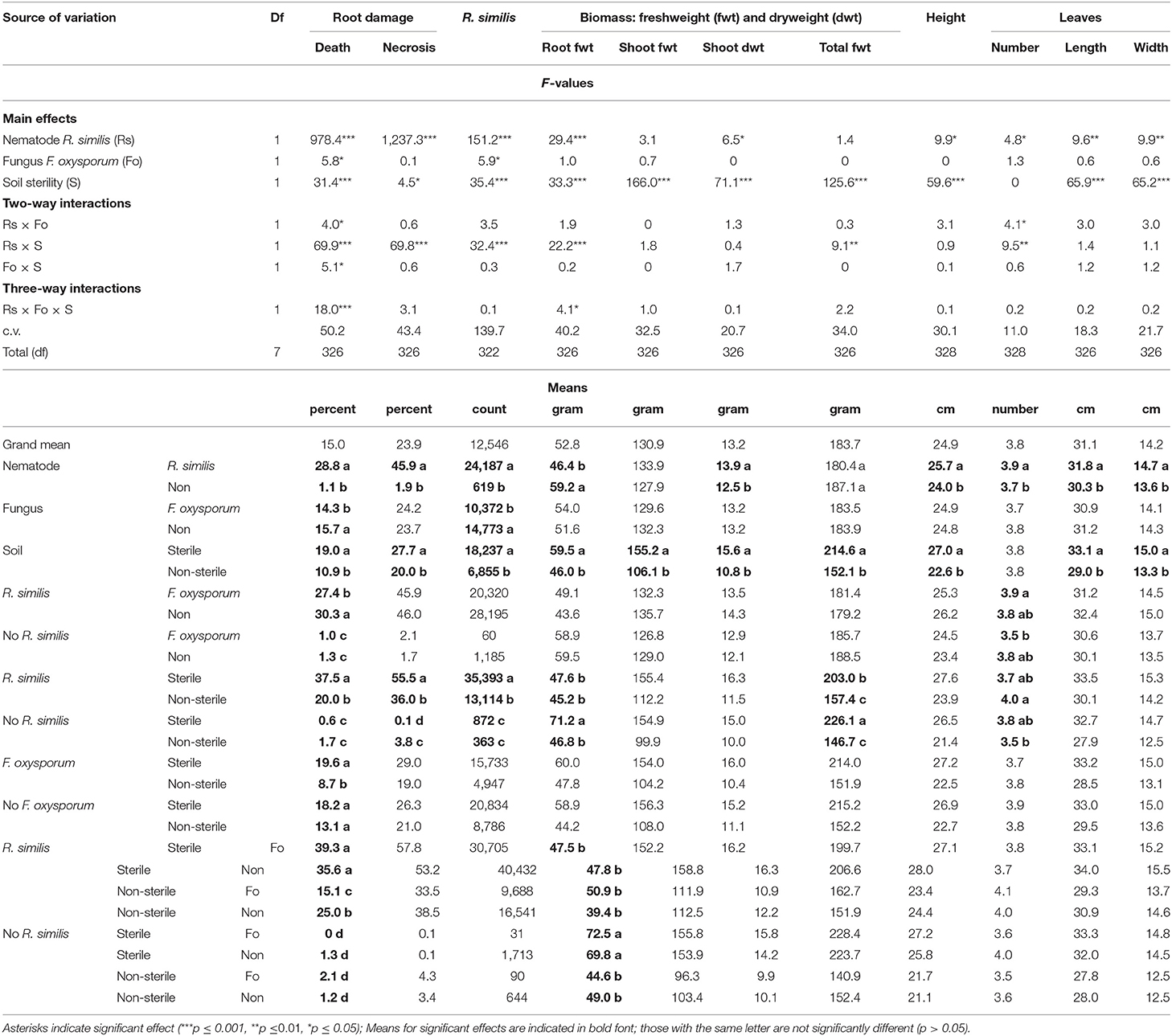
Table 2. Effect of soil sterility on infection symptoms of Radopholus similis and Fusarium oxysporum V5w2 in tissue culture banana plants grown under nitrogen deficient conditions.
Banana plants grown in sterile soil without R. similis inoculation had a higher root biomass compared to those from the remaining treatments, which had similar root biomass (Table 2). Plants from sterile soil had higher total biomass than those from non-sterile soil. In sterile soil, R. similis-inoculated plants had lower total biomass than those without nematodes. However, in non-sterile soil, total plant biomass did not vary between R. similis-inoculated and those not inoculated with the nematode.
Shoot dry weight, plant height and size of leaves had higher values in R. similis-inoculated plants than in those not inoculated with the nematode (Table 2). F. oxysporum-inoculated plants that received R. similis inoculum had more leaves than those that were not inoculated with the nematode. In non-sterile soil, plants that were inoculated with R. similis had more leaves than those not inoculated with the nematode. Shoot fresh and dry weights, plant height, length, and width of leaves were greater in plants from sterile than those from non-sterile soil (Table 2).
Percentage root piece colonization by Fusarium spp. was higher in banana plants that received independent and combined inoculations with F. oxysporum V5w2 and R. similis compared to the non-inoculated controls (Figure 2A). Those inoculated with R. similis had higher root piece colonization by Fusarium spp., especially when co-inoculated with F. oxysporum V5w2 and grown in sterile soil. Twenty-five other endophytic fungi were isolated from the banana root pieces with 11 genera among them identified and included; Fusarium spp., Rhizoctonia spp., Trichoderma spp., Sclerotium spp., Bipolaris spp., Curvularia spp., Mucor spp., Rhizopus spp., Papulaspora spp., Pleurophragmium spp., and Trichocladium spp. In vitro growth inhibition tests revealed the presence of Fusarium spp. and other microbes that interacted with F. oxysporum V5w2 with both antagonistic and neutral effects (Ochieno, 2010).
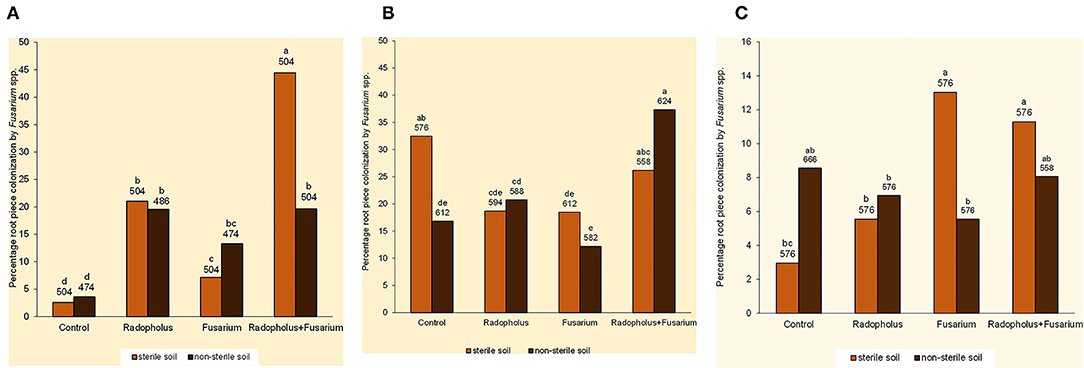
Figure 2. Root colonization by endophytic Fusarium spp. in (A) nitrogen-starved, (B) phosphorus-starved, and (C) potassium-starved tissue culture banana plants subjected to independent or combined inoculation with Radopholus similis and the Fusarium oxysporum V5w2 and grown in sterile or non-sterile soil. Bars with the same letter represent values that are not statistically different, with the sample sizes indicated below the letters (χ2-test, p < 0.05).
Darkened roots were associated with plants that were inoculated with R. similis and those that were grown in non-sterile soil (Figure 3). Optical density of root extracts exhibited small ranges of indiscernible variations that averaged 0.51 absorbance.
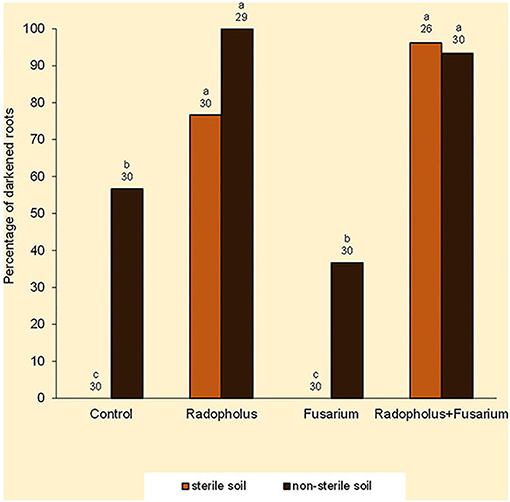
Figure 3. Percentage of dark-colored roots in Nitrogen-starved tissue culture banana plants subjected to independent or combined inoculation with Radopholus similis and Fusarium oxysporum V5w2 and grown in sterile or non-sterile soil. Bars with the same letter represent values that are not statistically different, with the sample sizes indicated below the letters (χ2-test, p < 0.05).
Experiment With P-Starved Tissue Culture Banana Plants
Most plants exhibited visual symptoms that could be linked to P-starvation, which included dark-green leaves with slightly yellowing margins, while the older leaves curled downwards and dried. Dead roots were mainly found in R. similis-inoculated plants, and their percentage was higher in sterile than in non-sterile soil. Necrotic lesions were mainly found in plants that were inoculated with R. similis, and the percentage root necrosis was higher in non-sterile than in sterile soil (Table 3).
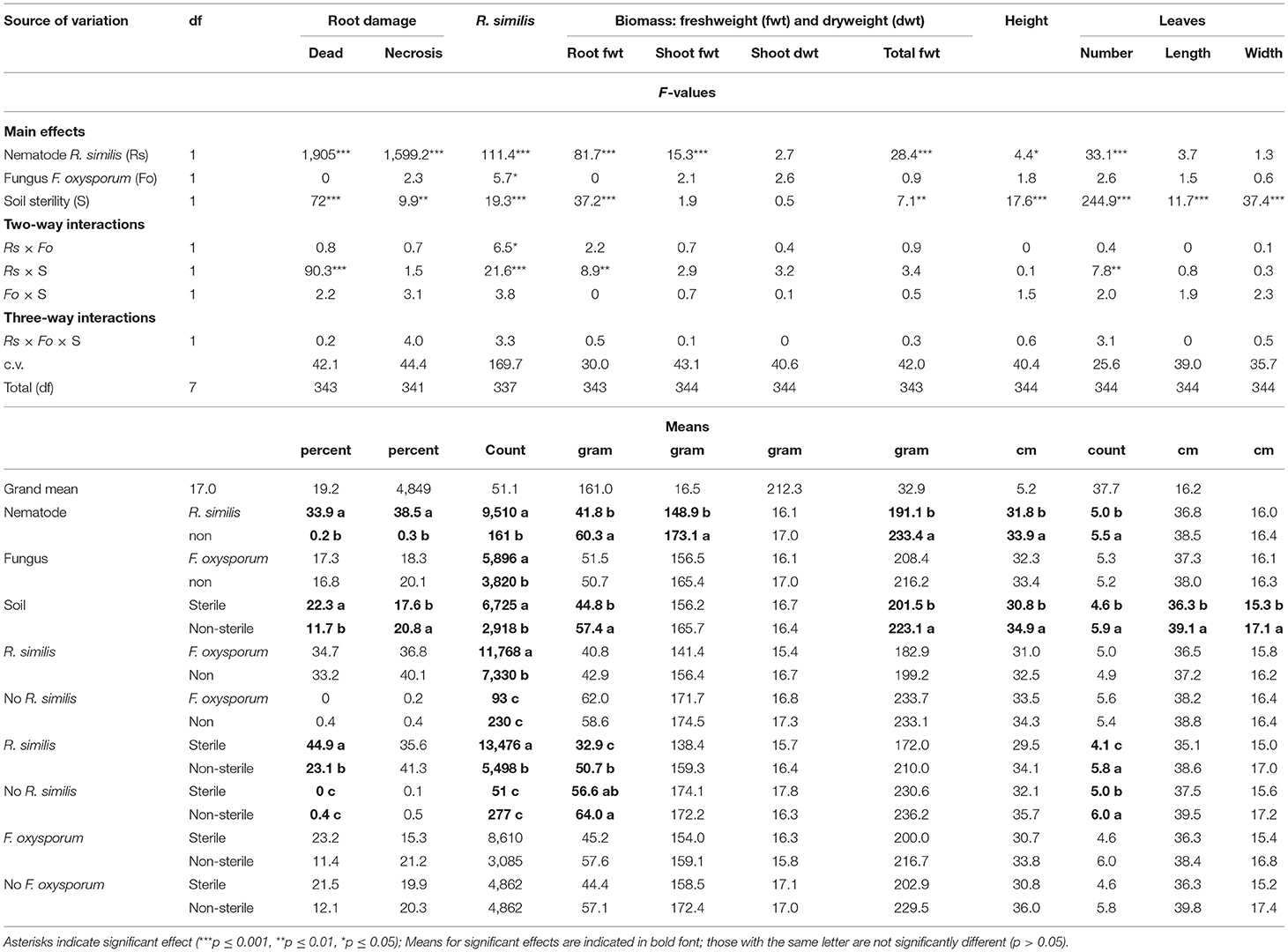
Table 3. Effect of soil sterility on infection symptoms of Radopholus similis and Fusarium oxysporum V5w2 in tissue culture banana plants grown under phosphorus deficient conditions.
Roots of plants co-inoculated with R. similis and F. oxysporum V5w2 had a higher density of the nematode than those that were not inoculated with the fungal pathogen (Table 3). Roots of nematode-inoculated plants from sterile soil had a higher density of R. similis than those from non-sterile soil (Table 3).
R. similis-inoculated plants in non-sterile soil had greater root biomass than those from sterile soil. R. similis-inoculated plants were shorter, had lower shoot fresh weight and total biomass than those without the nematode (Table 3). Plants in non-sterile soil were taller and had more total biomass than those from sterile soil. However, there was no difference in shoot dry weight between treatments (Table 3).
Plants that were grown in non-sterile soil produced more leaves than those from sterile soil (Table 3). Within sterile soil, R. similis-inoculated plants had fewer leaves than those without the nematode. However, in non-sterile soil, the number of leaves did not vary with R. similis treatment (Table 3). Leaves of plants that were grown in non-sterile soil were longer and wider than those from sterile soil. Plants inoculated with F. oxysporum V5w2 were generally smaller than those not inoculated with the fungus, although these differences were only of numerical significance (Table 3).
Fusarium spp. that were not visually different from the inoculated F. oxysporum V5w2 were observed in roots of all treatments and controls. Mycelia of Fusarium spp. that emerged from these roots appeared very sparse with scarcity of spores. In non-sterile soil, percentage root piece colonization by Fusarium spp. was highest in plants co-inoculated with F. oxysporum V5w2 and R. similis (Figure 2B). Trends in percentage root piece colonization by Fusarium spp. in sterile soil did not clearly reflect those in non-sterile soil (Figure 2B). Root pieces from the P-starved plants appeared more heavily contaminated with a variety of other microbes that were also isolated, especially from samples arising from non-sterile.
Experiment With K-Starved Tissue Culture Banana Plants
A symptom that could be linked to K-starvation, which was characterized by development of bright-orange coloration on leaf laminae, was evident in the K-starved plants. R. similis-inoculated plants in sterile soil had a higher percentage dead roots and higher density of the nematode than those from non-sterile soil (Table 4). Percentage root necrosis was higher in plants that were inoculated with R. similis than those without the nematode (Table 4).
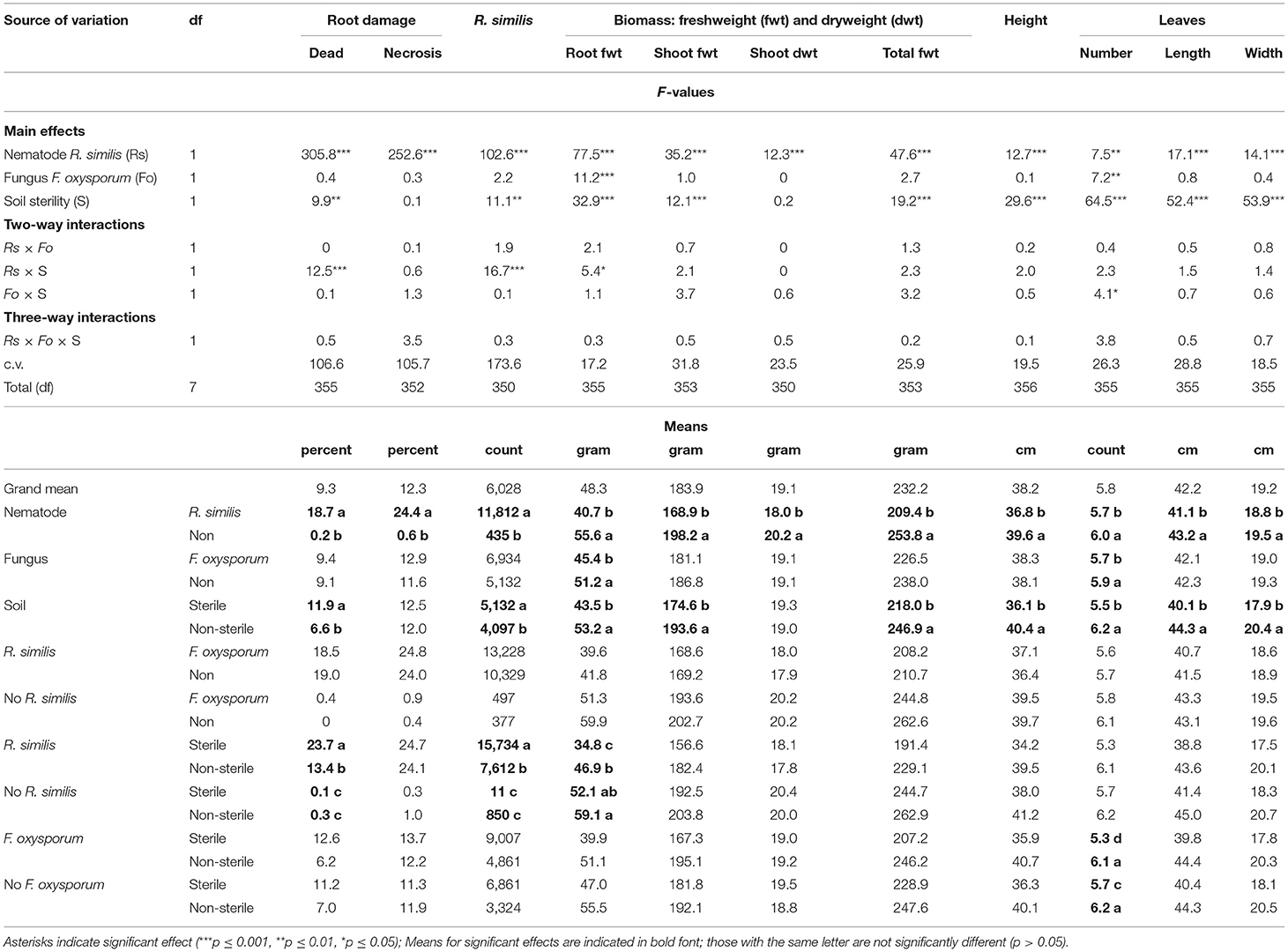
Table 4. Effect of soil sterility on infection symptoms of Radopholus similis and Fusarium oxysporum V5w2 in tissue culture banana plants grown under potassium deficient conditions.
Root biomass for F. oxysporum V5w2-inoculated plants was lower than for plants that were not inoculated with the fungal pathogen (Table 4). Root biomass for R. similis-inoculated plants that were grown in sterile soil was lower than for plants from non-sterile soil (Table 4). Shoot fresh weight, total biomass, plant height, leaf length, and width, were lower in plants that were inoculated with R. similis than in non-inoculated ones, and higher in non-sterile than in sterile soil (Table 4). Shoot dry weight was lower in R. similis-inoculated plants than in the non-inoculated ones (Table 4).
Nematode-treated plants had fewer leaves than those without nematodes (Table 4). Plants that were inoculated with F. oxysporum V5w2 and grown in sterile soil had fewer leaves than those that were not inoculated (Table 4). Plants that were grown in non-sterile soil had more leaves than those from sterile soil (Table 4).
Percentage root piece colonization by Fusarium spp. was high in plants inoculated with F. oxysporum V5w2 and grown in sterile soil (Figure 2C). Percentage root piece colonization by Fusarium spp. was lower in non-sterile soil than in sterile soil among plants that received the fungal inoculum (Figure 2C).
Discussion
The present three experiments were conducted to find out whether, pathogenic interactions between R. similis and F. oxysporum V5w2 under nitrogen (N), phosphorus (P), and potassium (K) limited conditions, are enhanced by a complex of non-beneficial soil microbes that exert plant growth suppressive effects in tissue culture banana (Kumar et al., 2021). Plant parasitic activities of R. similis on banana plants were evident in the form of increased density of the nematode that resulted in root damage through necrosis, death and reduced plant biomass. This root burrowing nematode is known to cause such root damage that inhibits water and nutrient uptake from the rhizosphere resulting in poor growth and toppling of banana trees (Devi et al., 2009; Haegeman et al., 2010; Seenivasan, 2017). Root damage by R. similis also allows co-infections by root-invading organisms such as microbial pathogens and other plant-parasitic nematodes involved in plant disease complexes (Khan and Sharma, 2020). Such root-invading organisms may have included the 25 endophytic fungi that were isolated from the banana root pieces among them Fusarium spp., Rhizoctonia spp., Trichoderma spp., Sclerotium spp., Bipolaris spp., Curvularia spp., Mucor spp., Rhizopus spp., Papulaspora spp., Pleurophragmium spp., and Trichocladium spp.; as well as plant-parasitic nematodes that included Helicotylenchus multicintus, Meloidogyne spp., Pratylenchus goodeyi, Xiphinema spp., Trophorus spp., Scutellonema spp., Rotylenchus spp., Tylenchus spp., Hemicycliophora spp., Paratrichodorus spp., and Ditylenchus spp.
Contrary to expectations, N-starved banana plants inoculated with R. similis exhibited better growth in terms of shoot biomass, height, number, and size of leaves. The N-starved banana plants inoculated with R. similis even tended to have higher chlorophyll content that made them appear less yellow or otherwise greener. Probably, N in the form of ammonium excreted by R. similis feeding on the roots is preferentially directed toward chlorophyll synthesis under N-deficient conditions. This is because the nematode-excreted ammonium (; Neher, 2001; Zinov'eva et al., 2004), is the most readily available form of nitrogen for assimilation by plants (Mur et al., 2017; Ochieno et al., 2021). Also, root-feeding activities of R. similis may have enhanced the rhizodeposition of carbon-rich compounds including enzymes that favor the acquisition of scarce N from soil organic matter (SOM; Gebremikael et al., 2016). Rhizodeposition is part of physiological and morphological changes that include elongation of roots which enable N-starved plants to survive the adverse conditions (Bowsher et al., 2018).
Root infection by R. similis appeared to be beneficial to Fusarium spp. through creation of wounds and necrotic tissues for saprophytic growth of the fungus (Back et al., 2002; Khan and Sharma, 2020). This manifested in the form of enhanced root piece colonization under the three nutrient deficient regimes (Ochieno, 2010, 2020). F. oxysporum V5w2 was expected to cause wilt symptoms (Ochieno, 2020), which are related to pathogenicity of F. oxysporum races in susceptible banana cultivars (Dita et al., 2018; Maryani et al., 2019; Segura-Mena et al., 2021). However, there was no basis for linking F. oxysporum V5w2 with wilt-related pathogenicity in the N-starved banana plants. This is because the N-starved banana plants were already expressing nutrient stress symptoms including yellowing of leaves and stunted growth (Ochieno, 2010), which also occur in plants infected with pathogenic F. oxysporum races (Warman and Aitken, 2018). Possible pathogenic invasion of the N-deficient xylem may not have been nutritively beneficial to endophytic F. oxysporum (MacHardy and Beckman, 1981; Pegg et al., 2019), as necrotrophic and saprophytic colonization may have been a more viable nutritional strategy (Orr and Nelson, 2018; Pegg et al., 2019; Ochieno, 2020).
Inoculation of banana plants with F. oxysporum V5w2 is expected to suppress R. similis multiplication and the associated root damage in banana plants (Kumar and Dara, 2021; Kisaakye et al., 2022). This was the case in the study that utilized N-starved banana plants. Inoculation with F. oxysporum V5w2 under conditions of N-starvation was associated with reduced densities of R. similis and root damage by the nematode especially in the microbe-rich non-sterile soil. However, endophytic and rhizosphere colonization by F. oxysporum V5w2 may have intensified competition for the already limited N and hence inhibiting the multiplication of R. similis (Ochieno, 2020). Reduction in optical density of root extracts of banana plants infected with F. oxysporum V5w2 and R. similis may indicate competition for nutrient resources (Ochieno, 2010, 2020). Therefore, competition for N under its extreme deficiency (Ochieno, 2020), is an alternative to explanations that are based on the concept of induced systemic resistance (Vu et al., 2006; Paparu et al., 2007, 2010, 2013; Sikora et al., 2008; Bubici et al., 2019; Damodaran et al., 2020).
In all the three nutrient starvation regimes, banana plants grown in non-sterile soil had reduced densities of R. similis in roots, while root damage caused by the nematode was lower than in sterile soil. The synergistic effect of R. similis on root colonization by F. oxysporum V5w2 was suppressed in non-sterile soil in the presence of P within the N- and K-deficient nutrient solutions. These were indications of the presence of pest-suppressive microbes in non-sterile soil (Shen et al., 2015; Giné et al., 2016; Jayaraman et al., 2021). For instance, among the microbial genera isolated from banana roots in the present study, some that belong to Fusarium, Trichoderma, and Sclerotium have been associated with nematostatic activities (Akinsanmi and Adekunle, 2003; Poveda et al., 2020; Kumar and Dara, 2021). Furthermore, R. similis may have encountered antagonistic interactions with other nematode genera identified, especially through competition with plant-parasitic nematodes such as Helicotylenchus multicintus, Meloidogyne spp., and Pratylenchus goodeyi (Freckman and Caswell, 1985; Ochieno, 2010). Inclusion of F. oxysporum V5w2 in non-sterile soil even caused further reduction in root damage and R. similis densities among N-starved banana plants. Elimination of such antagonistic microbes through soil sterilization allowed R. similis to multiply and cause root damage. However, suppression of R. similis multiplication and the associated root damage in non-sterile soil did not improve the growth of N-starved banana plants. Instead, the N-starved banana plants grown in non-sterile soil were even smaller in terms of biomass and size. Increased demand and hence competition for N by soil microbes including F. oxysporum V5w2 in non-sterile soil (Jones et al., 2018), may have limited the growth of the N-starved banana plants and pest-related activities of R. similis. Such competition for N was manifested in the form of reduced chlorophyll content in leaves of banana plants grown in non-sterile soil. Inclusion of N in non-sterile soil reversed such trends toward suppression of root damaging activities of R. similis while improving banana plant growth, as was evident in the root biomass of plants that received P- and K-deficient solutions that contained adequate N. Therefore, microbial services cannot be well attained under conditions of poor soil fertility and plant nutrition, especially when N is limited. Beneficial soil microbes require proper nutrition to prevent them turning into competitors.
Plants inoculated with F. oxysporum V5w2 were smaller in size in terms of leaf development under K-starvation, with most plant growth related parameters inclining toward such trend under P-starvation. This is a pointer to the fact that the fungal pathogen F. oxysporum V5w2 may have initiated its plant growth suppressing effects within the short period (Ochieno, 2010, 2020). Under P-starvation, co-infection of banana roots with R. similis and F. oxysporum V5w2 resulted in increased density of the nematode and higher root colonization by Fusarium spp. Such trends in R. similis and Fusarium spp. densities appeared to manifest in roots of banana plants grown under K starvation. Densities of R. similis, Meloidogyne sp., and Pratylenchus sp. have also been found to increase in roots of F. oxysporum-inoculated field-grown banana plants (Ochieno, 2010, 2020, 2021). Such trends in R. similis and Fusarium spp. densities are indications of nematode-fungi disease complexes (Back et al., 2002; Khan and Sharma, 2020; Ochieno, 2020), which may be favored in soils that are deficient in P and K among other unfavorable ecological conditions (Almeida et al., 2018; Olivares et al., 2021).
Various speculations can been put forward to explain the positive outcomes of interactions between R. similis and F. oxysporum V5w2 under the P-limited conditions. First, it is worth noting that P is an important nutrient in root development (Crombez et al., 2019; Siedliska et al., 2021). Phosphorus is part of the phospholipids that form the plant cell membranes (Wang et al., 2017, 2021). P-deficiency is associated with weakened cell membranes (Gniazdowska et al., 1999; Pfaff et al., 2020), which may easily be penetrated by R. similis and F. oxysporum V5w2 resulting in increased colonization by the two pathogens. Phosphorus is a chemical component of adenosine triphosphate (ATP; Wang et al., 2021), which provides energy for biosynthesis of plant defensive compounds (Dhakshinamoorthy et al., 2014; Crombez et al., 2019; Desmedt et al., 2020). P-deficiency may limit the biosynthesis of such defensive compounds and render banana plants less resistant to R. similis and F. oxysporum V5w2. Under P-deficiency, F. oxysporum V5w2 may have released phosphatases to enhance acquisition of the limited nutrient from organic sources (Tarafdar et al., 2001; Nunez-Rodriguez et al., 2020). Such enzymes may have further degraded the weakened and damaged roots to facilitate penetration and colonization by R. similis and F. oxysporum V5w2. P-deficiency may also have triggered increased root exudation of organic carbon in the form of sugars into the rhizosphere (Louw-Gaume et al., 2010, 2017; Canarini et al., 2019). The organic carbon may have intensified rhizosphere colonization by the saprophytic F. oxysporum V5w2 (Ochieno, 2020; Clocchiatti et al., 2021), making root invasion, colonization and degradation even more severe for easier penetration and multiplication of R. similis. Potassium on the other hand has been considered to have multiple roles including plant defense against pests and diseases (Amtmann et al., 2008; He et al., 2020). K-starvation may have interfered with mechanisms related to plant disease resistance to F. oxysporum V5w2 resulting in poor growth.
Soil sterilization exerted various effects on nematode-fungus-banana root interactions that are not only related to the removal of beneficial and pathogenic microbes (Li et al., 2019), but also to other physico-chemical changes that may have been instigated by the high sterilization temperatures (De Deyn et al., 2004; Lees et al., 2018). In the presence of N, banana plants grown in non-sterile soil had low densities of R. similis that caused less death of roots, resulting in better growth in terms of biomass and size. Poor plant growth in sterilized soil may indicate the elimination of beneficial microbes in the endospheres and rhizospheres (Ochieno, 2010; Kaushal et al., 2020), including nematode-suppressive microbes, arbuscular mycorrhizal fungi (AMF), phosphate solubilizing microbes among other beneficial soil biota (Paliwoda and Mikiciuk, 2020; Van der Veken et al., 2021). However, the poor growth of banana plants in the steam-sterilized soil may also have alternative explanations. For instance, it is possible that soil sterilization led to decreased bioavailability of P through heat-driven sorption (Shaw et al., 1999; Sinegani and Sedri, 2011; Bai et al., 2017), or even made the fixed P more water-soluble and hence prone to loss through leaching (Shaw et al., 1999; Sinegani and Sedri, 2011; Bai et al., 2017), with the condition being made more extreme through supply of the P-deficient solution. Despite the explained presence of beneficial microbes, root necrosis was higher in P-starved banana plants grown in non-sterile soil. This raises questions as to why root necrosis did not relate directly with R. similis densities and root death that instead appeared to have been suppressed. Evidence from the data presented in the present study shows that R. similis and soil sterility exerted independent activities toward root necrosis under the P-deficient conditions. It is likely that other root parasitic nematodes and root-rot microbes in non-sterile soil contributed to the development of the necrotic lesions under the P-limited conditions (Ochieno, 2010; Finkel et al., 2019). This may have involved the secretion of microbial phosphatases for the acquisition of P from disintegrating root tissues (Tarafdar et al., 2001; Cabugao et al., 2017, 2021).
Despite all these speculations having considerably valid bases, consensus toward the outcomes of interactions between F. oxysporum V5w2 and other fungal phytopathogens with plant-parasitic nematodes in the banana rhizosphere context (Ochieno, 2020), are complex matters that still exist within a conundrum of diverse views in plant sciences (Schulz and Boyle, 2006; Ochieno, 2010, 2020; Avedi et al., 2014; Taylor, 2014; Schouten, 2016; Brader et al., 2017; Maryani et al., 2019; Geiser et al., 2020; Ochieno et al., 2021). This requires more investment toward research on the diversity and function of root and rhizosphere microbiomes under different ecological conditions (Li et al., 2019; Berg et al., 2020; Kaushal et al., 2020). Proper identification of F. oxysporum V5w2 among other strains that have been proposed for biological control requires whole genome sequence (WGS) analysis to differentiate them from pathogenic ones within the Fusarium of Banana Complex (FOBC; Kurtz, 2010; Schouten, 2016; Maryani et al., 2019; Bedoya et al., 2021). Characterization of interacting endosphere and rhizosphere microbes and invertebrates could be strengthened with better methods such as those based on metagenomic DNA sequence analyses (Sharpton, 2014; Nesme et al., 2016; Zakeel and Safeena, 2020). Metabolomic analyses of root exudates based on gas chromatography-mass spectrometry (GC-MS), liquid chromatography (LC) platforms or nuclear magnetic resonance (H-NMR) would have provided further details on changes instigated due to banana root interactions with rhizosphere biota and nutrients among other factors (van Dam and Bouwmeester, 2016; Vives-Peris et al., 2020). Changes in rhizosphere microbe populations in the form of colony forming units (CFU) could be monitored through plate dilution method of plant and soil samples (Vieira and Nahas, 2005), or by other multiple culture-independent measurements including adenosine tri-phosphate (ATP), flow cytometry (FCM), quantitative real-time PCR (qPCR), phospholipid fatty acids (PLFA), and microbial biomass carbon (MBC) (Li et al., 2008; Zhang et al., 2017). Follow-up studies may as well explore the possible mechanisms of R. similis suppression in the non-sterile rhizosphere, which may include direct parasitism or predation on the nematode or its eggs (Sikora et al., 2008; Li et al., 2015), antibiosis through production of toxic metabolites (Mwaura et al., 2010; Keswani et al., 2020), competition for nutrients and other resources (Kerry, 2000), as well as induced systemic resistance (Sikora et al., 2008; Walters, 2009; Paparu et al., 2013; Poveda et al., 2020). Such studies may be complemented with those assessing microorganisms that assist the survival and parasitism of plant-parasitic nematodes by protecting them against antagonistic microbes (Topalović and Vestergård, 2021). It should also be considered that microbes such as arbuscular mycorrhizal (AM) fungi known to be beneficial to host plants may also suppress plant growth while increasing infections by plant-parasitic nematodes (Frew et al., 2018). In all these, it remains an open question on whether development and evaluation of resistant banana cultivars should focus on independent pest species (Tripathi et al., 2015; Seenivasan, 2017; Rebouças et al., 2018), or whether a broader approach that tries to simultaneously tackle disease complexes and abiotic stresses would be achievable in providing better banana cultivars (Lorenzen et al., 2010; Almeida et al., 2018; Batte et al., 2019; Tripathi et al., 2019, 2020; Rocha et al., 2020).
In summary, results from the present three experiments have shown that soil microbes provide ecosystem services through pest and disease suppression while promoting banana plant growth. Soil sterilization and tissue culture eliminates such beneficial microbes to enhance root infection and damage by R. similis and F. oxysporum V5w2. Plant growth promoting benefits of the soil microbes are negated by nutrient stresses, especially N deficiency that shifts their activities toward being competitors that suppress banana plant growth. However, under such extreme N-deficient conditions, pathogenic F. oxysporum V5w2 and R. similis may create some yet to be clearly understood impression of banana plant growth promoting services. Competition for N under extreme starvation of this very important nutrient explains the suppression of R. similis by F. oxysporum V5w2, but not the widely claimed induced systemic resistance mechanisms in banana plants. On the other hand, P deficiency appears to provide physiological conditions that favor synergistic interactions between F. oxysporum V5w2 and R. similis, whereby the densities of both pathogens in banana root tissues are increased. Potassium deficiency appears to provide physiological conditions that favor pathogenicity of F. oxysporum V5w2 by constraining plant growth, especially in terms of leaf development. These are part of issues that could inform the application of microbial services in Sustainable Food Systems (Timmis et al., 2017), particularly in the pending decision on registration of F. oxysporum V5w2 as a commercial biopesticide (Dubois et al., 2006, 2011; zum Felde, 2011; Ochieno, 2020, 2021; Kisaakye et al., 2022), in line with International Standards for Phytosanitary Measures (ISPM) among other related policies (Wabule et al., 2003; Avedi et al., 2014; KEPHIS, 2014; FAO-ISPM, 2021).
In conclusion, pathogenic interactions between R. similis and F. oxysporum V5w2 are predominantly suppressed by a complex of soil microbes that exert plant growth promoting effects in tissue culture banana plants through N, P, and K dependent processes. Nitrogen is the most important limiting factor in rhizosphere interactions between banana roots, beneficial microbes and the pathogens. Soil sterilization and the stringent aseptic tissue culture techniques still require the development of alternative innovative ways of conserving microbial services for sustainable agriculture.
Data Availability Statement
The datasets presented in this article are not readily available because the conflict of interest as declared only makes it possible to refer to data that was jointly published in Ochieno (2010). Requests to access the datasets should be directed to dwochieno@gmail.com.
Author Contributions
The author confirms being the sole contributor of this work and has approved it for publication.
Funding
This work was part of my PhD studies that were funded by Wageningen University and Research Center (WUR) (Project number PE&RC 06034) and the International Institute of Tropical Agriculture (IITA-Uganda) through finances from the German Federal Ministry for Economic Cooperation and Development (BMZ) (GTZ Project number 03.7860.4-001.00). Masinde Muliro University of Science and Technology (MMUST) under the leadership of the Vice Chancellor among other staff provided funds for presentation of this research at the X International Symposium on Banana ISHS-ProMusa Symposium, 10–14 October 2016, Montpellier, France.
Conflict of Interest
This work has been disassociated from studies that propose Fusarium oxysporum V5w2 as an Endophytic Biological Control Agent and the matter has already been openly declared (Ochieno, 2020). Prof. Marcel Dicke, Prof. Arnold van Huis, Dr. Thomas Dubois, Dr. Danny Coyne and Dr. Piet J.A. van Asten have renounced their co-authorship due to difference of opinion about the interpretation of the data and the conclusions of this study. My current employer who is Masinde Muliro University of Science and Technology (MMUST) with its Centre for African Medicinal Flora and Fauna (CAMNFF) was not involved in the research described in this article.
Publisher's Note
All claims expressed in this article are solely those of the authors and do not necessarily represent those of their affiliated organizations, or those of the publisher, the editors and the reviewers. Any product that may be evaluated in this article, or claim that may be made by its manufacturer, is not guaranteed or endorsed by the publisher.
Acknowledgments
The study was originally co-authored as Chapter 6 and 7 (Ochieno, 2010), with Thomas Dubois, Danny Coyne, Piet J. A. van Asten (IITA-Uganda), and Arnold van Huis and Marcel Dicke (Laboratory of Entomology, WUR). Richard A. Sikora (University of Bonn), Thomas Kuijper, Jos Raaijmakers (WUR), and MNEJ Smit (Private IPM Consultant) reviewed the original manuscripts. Thanks to Joop C. van Lenteren (Laboratory of Entomology-WUR), Clifford S. Gold (IITA-Uganda), Ellie Osir (International Center of Insect Physiology and Ecology-ICIPE), Micheni Ntiba, and Lucy W. Irungu (School of Biological Sciences, University of Nairobi) for facilitating my recruitment into the great Wageningen Sandwich PhD program. Thanks to Stephen Mutoro, the Secretary General of the Consumers Federation of Kenya (COFEK) and Member of the University Council (MMUST) for fostering public-participation by representing farmers and other stakeholders interests in the registration of Fusarium oxysporum V5w2 as a biopesticide through the Legislative Process in Kenya.
References
Akankwasa, K., Marimo, P., Tumuhimbise, R., Asasira, M., Khakasa, E., Mpirirwe, I., et al. (2021). The East African highland cooking bananas “Matooke” preferences of farmers and traders: implications for variety development. Int. J. Food Sci. Technol. 56, 1124–1134. doi: 10.1111/ijfs.14813
Akello, J., Dubois, T., Gold, C. S., Coyne, D., Nakavuma, J., and Paparu, P. (2007). Beauveria bassiana (Balsamo) Vuillemin as an endophyte in tissue culture banana (Musa spp.). J. Invertebr. Pathol. 96, 34–42. doi: 10.1016/j.jip.2007.02.004
Akinsanmi, O. A., and Adekunle, O. K. (2003). Effect of Fusarium oxysporum f. sp. glycines and Sclerotium rolfsii on the pathogenicity of Meloidogyne incognita race 2 to soybean. Plant Soil 253, 429–435. doi: 10.1023/A:1024880115651
Almeida, N. O., Teixeira, R. A., Carneiro, F. A., de Oliveira, C. M., Ribeiro, V. A., Lobo Júnior, M., et al. (2018). Occurrence and correlations of nematodes, Fusarium oxysporum and edaphic factors on banana plantations. J. Phytopathol. 166, 265–272. doi: 10.1111/jph.12683
Almekinders, C. J. M., Walsh, S., Jacobsen, K. S., Andrad-Piedra, J. L., McEwan, M. A., de Haan, S., et al. (2019). Why interventions in the seed systems of roots, tubers and bananas crops do not reach their full potential. Food Sec. 11, 23–42. doi: 10.1007/s12571-018-0874-4
Amtmann, A., Troufflard, S., and Armengaud, P. (2008). The effect of potassium nutrition on pest and disease resistance in plants. Physiol. Plant. 133, 682–691. doi: 10.1111/j.1399-3054.2008.01075.x
Athman, S. Y., Dubois, T., Coyne, D., Gold, C. S., Labuschagne, N., and Viljoen, A. (2007). Effect of endophytic Fusarium oxysporum on root penetration and reproduction of Radopholus similis in tissue culture-derived banana (Musa spp.) plants. Nematology 9, 599–607. doi: 10.1163/156854107782024839
Avedi, E. K., Ochieno, D. M. W., Ajanga, S., Wanyama, C., Wainwright, H., Elzein, A., et al. (2014). Fusarium oxysporum f. sp. strigae strain Foxy 2 did not achieve biological control of Striga hermonthica parasitizing maize in Western Kenya. Biol. Control 77, 7–14. doi: 10.1016/j.biocontrol.2014.05.012
Back, M. A., Haydock, P. P. J., and Jenkinson, P. (2002). Disease complexes involving plant parasitic nematodes and soilborne pathogens. Plant Pathol. 51, 683–697. doi: 10.1046/j.1365-3059.2002.00785.x
Bai, J., Ye, X., Jia, J., Zhang, G., Zhao, Q., Cui, B., et al. (2017). Phosphorus sorption-desorption and effects of temperature, pH and salinity on phosphorus sorption in marsh soils from coastal wetlands with different flooding conditions. Chemosphere 188, 677–688. doi: 10.1016/j.chemosphere.2017.08.117
Bakaze, E., Dzomeku, B. M., and Wünsche, J. N. (2021). Banana defence responses to Cosmopolites sordidus feeding and methyl jasmonate application. Ann. Appl. Biol. 178, 98–108. doi: 10.1111/aab.12638
Batte, M., Swennen, R., Uwimana, B., Akech, V., Brown, A., Tumuhimbise, R., et al. (2019). Crossbreeding East African Highland Bananas: lessons learnt relevant to the botany of the crop after 21 years of genetic enhancement. Front. Plant Sci. 10:81. doi: 10.3389/fpls.2019.00081
Bedoya, E. T., Bebber, D. P., and Studholme, D. J. (2021). Taxonomic revision of the Banana Fusarium wilt TR4 pathogen is premature. Phytopathology 111:2141–2145. doi: 10.1094/PHYTO-03-21-0089-LE
Berg, G., Rybakova, D., Fischer, D., Cernava, T., Champomier Vergès, M. C., Charles, T., et al. (2020). Microbiome definition re-visited: old concepts and new challenges. Microbiome 8:103. doi: 10.1186/s40168-020-00875-0
Bowsher, A. W., Evans, S., Tiemann, L. K., et al. (2018). Effects of soil nitrogen availability on rhizodeposition in plants: a review. Plant Soil 423, 59–85. doi: 10.1007/s11104-017-3497-1
Brader, G., Compant, S., Vescio, K., Mitter, B., Trognitz, F., Ma, L. J., et al. (2017). Ecology and genomic insights into plant-pathogenic and plant-nonpathogenic endophytes. Annu. Rev. Phytopathol. 55, 61–83. doi: 10.1146/annurev-phyto-080516-035641
Bremner, J. M (1960). Determination of nitrogen in soil by the Kjeldahl method. J. Agric. Sci. 55, 11–33. doi: 10.1017/S0021859600021572
Brooks, F. E (2004). Plant-parasitic nematodes of banana in American Samoa. Nematropica 34, 65–72. Available online at: https://journals.flvc.org/nematropica/article/view/69688 (accessed February 14, 2022).
Bubici, G., Kaushal, M., Prigigallo, M. I., Gómez-Lama Cabanás, C., and Mercado-Blanco, J. (2019). Biological control agents against Fusarium wilt of Banana. Front. Microbiol. 10:616. doi: 10.3389/fmicb.2019.00616
Cabugao, K. G., Timm, C. M., Carrell, A. A., Childs, J., Lu, T.-Y. S., Pelletier, D. A., et al. (2017). Root and rhizosphere bacterial phosphatase activity varies with tree species and soil phosphorus availability in Puerto Rico Tropical Forest. Front. Plant Sci. 8:1834. doi: 10.3389/fpls.2017.01834
Cabugao, K. G., Yaffar, D., Stenson, N., Childs, J., Phillips, J., Mayes, M. A., et al. (2021). Bringing function to structure: root–soil interactions shaping phosphatase activity throughout a soil profile in Puerto Rico. Ecol. Evol. 11, 1150–1164. doi: 10.1002/ece3.7036
Canarini, A., Kaiser, C., Merchant, A., Richter, A., and Wanek, W. (2019). Root exudation of primary metabolites: mechanisms and their roles in plant responses to environmental stimuli. Front. Plant Sci. 10:157. doi: 10.3389/fpls.2019.00157
Cassells, A. C (2012). Pathogen and biological contamination management in plant tissue culture: phytopathogens, in vitro pathogens, and in vitro pests. Methods Mol. Biol. 877, 57–80. doi: 10.1007/978-1-61779-818-4_6
Clocchiatti, A., Hannula, S. E., van den Berg, M., Hundscheid, M. P. J., and de Boer, W. (2021). Evaluation of phenolic root exudates as stimulants of saptrophic fungi in the rhizosphere. Front. Microbiol. 12:644046. doi: 10.3389/fmicb.2021.644046
Crombez, H., Motte, H., and Beeckman, T. (2019). Tackling plant phosphate starvation by the roots. Dev. Cell 48, 599–615. doi: 10.1016/j.devcel.2019.01.002
Damodaran, T., Rajan, S., Manoharan Muthukumar, R. G., Yadav, K., Kumar, S., Ahmad, I., et al. (2020). Biological management of Banana Fusarium wilt caused by Fusarium oxysporum f. sp. cubense tropical race 4 using antagonistic fungal isolate CSR-T-3 (Trichoderma reesei). Front. Microbiol. 11:595845. doi: 10.3389/fmicb.2020.595845
De Deyn, G. B., Raaijmakers, C. E., and Van der Putten, W. H. (2004). Plant community development is affected by nutrients and soil biota. J. Ecol. 92, 824–834. doi: 10.1111/j.0022-0477.2004.00924.x
De Rooij-van der Goes, P. C. E. M (1995). The role of plant-parasitic nematodes and soil-borne fungi in the decline of Ammophila arenaria (L.) link. New Phytol. 129, 661–669. doi: 10.1111/j.1469-8137.1995.tb03035.x
Desmedt, W., Mangelinckx, S., Kyndt, T., and Vanholme, B. (2020). A phytochemical perspective on plant defense against nematodes. Front. Plant Sci. 11:602079. doi: 10.3389/fpls.2020.602079
Devi, N. A., Ponnuswami, V., Sundararaju, P., Van den Bergh, I., and Kavino, M. (2009). Histopathological changes in banana roots caused by Pratylenchus coffeae, Meloidogyne incognita and Radopholus similis, and identification of RAPD markers associated with P. coffeae resistance. Acta Hortic. 828, 283–290. doi: 10.17660/ActaHortic.2009.828.28
Dhakshinamoorthy, S., Mariama, K., Elsen, A., and De Waele, D. (2014). Phenols and lignin are involved in the defence response of banana (Musa) plants to Radopholus similis infection. Nematology 16, 565–576. doi: 10.1163/15685411-00002788
Dietrich, P., Cesarz, S., Eisenhauer, N., and Roscher, C. (2020). Effects of steam sterilization on soil abiotic and biotic properties. Soil Org. 92, 99–108. doi: 10.25674/so92iss2pp99
Dita, M., Barquero, M., Heck, D., Mizubuti, E. S. G., and Staver, C. P. (2018). Fusarium wilt of banana: current knowledge on epidemiology and research needs toward sustainable disease management. Front. Plant Sci. 9:1468. doi: 10.3389/fpls.2018.01468
Dubois, T., Coyne, D., Kahangi, E., Turoop, L., and Nsubuga, E. W. N. (2006). Endophyte-enhanced banana tissue culture: technology transfer through public-private partnerships in Kenya and Uganda. ATDF J. 3, 18−24. Available online at: https://hdl.handle.net/10568/91380 (accessed February 14, 2022).
Dubois, T., Coyne, D., and zum Felde, A. (2011). Enhanced Protection for Tissue Cultured Banana Plants. Ibadan: CGIAR Systemwide Program on Integrated Pest Management. Available online at: https://hdl.handle.net/10568/82818 (accessed February 14, 2022).
Dubois, T., Gold, C.S., Coyne, D., Paparu, P., Mukwaba, E., Athman, S., et al. (2004). Merging biotechnology with biological control: banana musa tissue culture plants enhanced by endophytic fungi. Uganda J. Agri. Sci. 9, 445–451. Available online at: https://hdl.handle.net/10568/103293 (accessed February 14, 2022).
FAO-ISPM (2021). International Standards for Phytosanitary Measures. Rome: Secretariat of the International Plant Protection Convection (IPPC), Food and Agriculture Organization (FAO). Available online at: https://assets.ippc.int/static/media/files/publication/en/2021/04/ISPM_List_En_2021-03-17.pdf (accessed February 14, 2022).
Finkel, O. M., Salas-González, I., Castrillo, G., Spaepen, S., Law, T. F., Teixeira, P. J. P. L., et al. (2019). The effects of soil phosphorus content on plant microbiota are driven by the plant phosphate starvation response. PLoS Biol. 17:e3000534. doi: 10.1371/journal.pbio.3000534
Fortuner, R (1988). Nematode Identification and Expert System Technology. Boston, MA: Springer. doi: 10.1007/978-1-4684-9016-9
Freckman, D. W., and Caswell, E. P. (1985). The ecology of nematodes in agroecosystems. Annu. Rev. Phytopathol. 23, 275–296. doi: 10.1146/annurev.py.23.090185.001423
Frew, A., Powell, J. R., Glauser, G., Bennett, A. E., and Johnson, S. N. (2018). Mycorrhizal fungi enhance nutrient uptake but disarm defences in plant roots, promoting plant-parasitic nematode populations. Soil Biol. Biochem. 126, 123–132. doi: 10.1016/j.soilbio.2018.08.019
Gebremikael, M., Steel, H., Buchan, D., et al. (2016). Nematodes enhance plant growth and nutrient uptake under C and N-rich conditions. Sci. Rep. 6:32862. doi: 10.1038/srep32862
Geiser, D. M., Al-Hatmi, A., Aoki, T., Arie, T., Balmas, V., Barnes, I., et al. (2020). Phylogenomic analysis of a 55.1 kb 19-gene dataset resolves a monophyletic Fusarium that includes the Fusarium solani species complex. Phytopathology 111, 1064–1079. doi: 10.1094/PHYTO-08-20-0330-LE
Giné, A., Carrasquilla, M., Martínez-Alonso, M., Gaju, N., and Sorribas, F. J. (2016). Characterization of soil suppressiveness to root-knot nematodes in organic horticulture in plastic greenhouse. Front. Plant Sci. 7:164. doi: 10.3389/fpls.2016.00164
Gniazdowska, A., Szal, B., and Rychter, A. M. (1999). The effect of phosphate deficiency on membrane phospholipid composition of bean (Phaseolus vulgaris L.) roots. Acta Physiol. Plant. 21, 263–269. doi: 10.1007/s11738-999-0041-9
Gundale, M. J., Wardle, D. A., Kardol, P., and Nilsson, M. C. (2019). Comparison of plant–soil feedback experimental approaches for testing soil biotic interactions among ecosystems. New Phytol. 221, 577–587. doi: 10.1111/nph.15367
Haegeman, A., Elsen, A., De Waele, D., and Gheysen, G. (2010). Emerging molecular knowledge on Radopholus similis, an important nematode pest of banana. Mol. Plant Pathol. 11:315. doi: 10.1111/j.1364-3703.2010.00614.x
Hasan, A (1993). “The role of fungi in fungus-nematode interactions,” in Nematode Interactions, ed M. W. Khan (Dordrecht: Springer), 13. doi: 10.1007/978-94-011-1488-2_13
He, Y., Li, R., Lin, F., Xiong, Y., Wang, L., Wang, B., et al. (2020). Transcriptome changes induced by different potassium levels in banana roots. Plants 9:11. doi: 10.3390/plants9010011
Jacobsen, K., Omondi, B. A., Almekinders, C., Alvarez, E., Blomme, G., Dita, M., et al. (2019). Seed degeneration of banana planting materials: strategies for improved farmer access to healthy seed. Plant Pathol. 68, 207–228. doi: 10.1111/ppa.12958
Jayaraman, S., Naorem, A., Lal, R., et al. (2021). Disease-suppressive soils—beyond food production: a critical review. J. Soil Sci. Plant. Nutr. 21, 1437–1465. doi: 10.1007/s42729-021-00451-x
Jones, D. L., Magthab, E. A., Gleeson, D. B., Hill, P. W., Sánchez-Rodríguez, A. R., Roberts, P., et al. (2018). Microbial competition for nitrogen and carbon is as intense in the subsoil as in the topsoil. Soil Biol. Biochem. 117, 72–82. doi: 10.1016/j.soilbio.2017.10.024
Kabuye, D., Tripathi, L., Niyibigira, E., Tripathi, J., and Okori, P. (2011). “Does horizontal gene flow occur in transgenic banana/Fusarium oxysporum (V5W2-9 NH3) associations?” in Proc. Inter. Conf. Agbiotech. Biosaf. Seed Syst (Kampala), 49–53. Available online at: https://www.scifode-foundation.org/attachments/article/38/Kabuye-D-et-al-2011a.pdf
Kaushal, M., Swennen, R., and Mahuku, G. (2020). Unlocking the microbiome communities of banana (Musa spp.) under disease stressed (Fusarium wilt) and non-stressed conditions. Microorganisms 8:443. doi: 10.3390/microorganisms8030443
KEPHIS. (2014). Guidelines for Introduction and Use of Bio-Products, Biological Control Agents and Related Products. Nairobi: Kenya Standing Technical Committee on Imports and Exports (KSTCIE). Kenya Plant Health Inspectorate Service (KEPHIS). Available online at: http://www.kephis.org/images/Guidelines/guidelines.pdf (accessed February 14, 2022).
Kerry, B. R (2000). Rhizosphere interactions and the exploitation of microbial agents for the biological control of plant-parasitic nematodes. Annu. Rev. Phytopathol. 38, 423–441. doi: 10.1146/annurev.phyto.38.1.423
Keswani, C., Singh, H. B., García-Estrada, C., et al. (2020). Antimicrobial secondary metabolites from agriculturally important bacteria as next-generation pesticides. Appl. Microbiol. Biotechnol. 104, 1013–1034. doi: 10.1007/s00253-019-10300-8
Khan, M. R., and Sharma, R. K. (2020). Fusarium-nematode wilt disease complexes, etiology and mechanism of development. Indian Phytopathol. 73, 615–628. doi: 10.1007/s42360-020-00240-z
Kisaakye, J., Fourie, H., Haukeland, S., Kisitu, J., Nakimera, S., Cortada, L., et al. (2022). Endophytic non-pathogenic Fusarium oxysporum-derived dual benefit for nematode management and improved banana (Musa spp.) productivity. Agriculture 12:125. doi: 10.3390/agriculture12020125
Kithaku, E. M., Muigai, A. W., Neondo, J. O., and Mweu, C. M. (2019). Screening of fungal contaminants in banana tissue cultures in Jkuat, Kenya. Afr. J. Microbiol. Res. 13, 675–688. doi: 10.5897/AJMR2019.9182
Kolawole, G. O., Eniola, O., and Oyeyiola, Y. B. (2018). Effects of nutrients omission on maize growth and nutrient uptake in three dominant soil types of southwestern Nigeria. J. Plant Nutr. 41, 1903–1915. doi: 10.1080/01904167.2018.1482909
Kuijken, R. C. P., Snel, J. F. H., Heddes, M. M., et al. (2015). The importance of a sterile rhizosphere when phenotyping for root exudation. Plant Soil 387, 131–142. doi: 10.1007/s11104-014-2283-6
Kumar, K. K., and Dara, S. K. (2021). Fungal and bacterial endophytes as microbial control agents for plant-parasitic nematodes. Int. J. Environ. Res. Public Health 18:4269. doi: 10.3390/ijerph18084269
Kumar, P., Cuervo, M., Kreuze, J. F., Muller, G., Kulkarni, G., Kumari, S. G., et al. (2021). Phytosanitary interventions for safe global germplasm exchange and the prevention of transboundary pest spread: the role of CGIAR germplasm health units. Plants 10:328. doi: 10.3390/plants10020328
Kurtz, A (2010). Endophytic Fusarium oxysporum: Phylogeny and Induced Defense Responses in Banana Plants Against Radopholus similis. (PhD Thesis), University of Bonn, Bonn, Germany. Available online at: https://hdl.handle.net/20.500.11811/4221 (accessed February 14, 2022).
Lancien, M., Ferrario-Méry, S., Roux, Y., Bismuth, E., Masclaux, C., Hirel, B., et al. (1999). Simultaneous expression of NAD-dependent isocitrate dehydrogenase and other Krebs cycle genes after nitrate resupply to short-term nitrogen-starved tobacco. Plant Physiol. 120, 717–726. doi: 10.1104/pp.120.3.717
Lees, K., Fitzsimons, M., Snape, J., Tappin, A., and Comber, S. (2018). Soil sterilisation methods for use in OECD 106: how effective are they? Chemosphere 209, 61–67. doi: 10.1016/j.chemosphere.2018.06.073
Leslie, J. F., and Summerell, B. A. (2008). The Fusarium Laboratory Manual. Hoboken, NJ: John Wiley & Sons. ISBN: 978-0-813-81919-8
Li, J., Zou, C., Xu, J., Ji, X., Niu, X., Yang, J., et al. (2015). Molecular mechanisms of nematode-nematophagous microbe interactions: basis for biological control of plant-parasitic nematodes. Annu. Rev. Phytopathol. 53, 67–95. doi: 10.1146/annurev-phyto-080614-120336
Li, K., DiLegge, M. J., Minas, I. S., Hamm, A., Manter, D., and Vivanco, J. M. (2019). Soil sterilization leads to re-colonization of a healthier rhizosphere microbiome. Rhizosphere 12:100176. doi: 10.1016/j.rhisph.2019.100176
Li, S., Hartman, G. L., Domier, L. L., and Boykin, D. (2008). Quantification of Fusarium solani f. sp. glycines isolates in soybean roots by colony-forming unit assays and real-time quantitative PCR. Theor. Appl. Genet. 117, 343–352. doi: 10.1007/s00122-008-0779-2
Lorenzen, J., Tenkouano, A., Bandyopadhyay, R., Vroh, B., Coyne, D., and Tripathi, L. (2010). Overview of banana and plantain (Musa spp.) improvement in Africa: past and future. Acta Hortic. 879, 595–560. doi: 10.17660/ActaHortic.2010.879.66
Louw-Gaume, A. E., Rao, I. M., Gaume, A. J., and Emmanuel, F. (2010). A comparative study on plant growth and root plasticity responses of two Brachiaria forage grasses grown in nutrient solution at low and high phosphorus supply. Plant Soil 328, 155–164. doi: 10.1007/s11104-009-0093-z
Louw-Gaume, A. E., Schweizer, N., Rao, I. M., Gaume, A. J., and Frossard, E. (2017). Temporal differences in plant growth and root exudation of two Brachiaria grasses in response to low phosphorus supply. Trop. Grassl. 5, 103–116. doi: 10.17138/TGFT(5)103-116
Luc, M., Sikora, R. A., and Bridge, J. (2005). Plant Parasitic Nematodes in Subtropical and Tropical Agriculture. Wallingford: CABI Publishing. doi: 10.1079/9780851997278.0000
MacHardy, W. E., and Beckman, C. H. (1981). “Vascular wilt Fusaria: infection and pathogenesis,” in Fusarium: Diseases, Biology, and Taxonomy, eds P. E. Nelson, T. A. Toussoun, and R. J. Cook (University Park, PA: The Pennsylvania State University Press), 365–391.
Maryani, N., Lombard, L., Poerba, Y. S., Subandiyah, S., Crous, P. W., and Kema, G. H. J. (2019). Phylogeny and genetic diversity of the banana Fusarium wilt pathogen Fusarium oxysporum f. sp. cubense in the Indonesian centre of origin. Stud. Mycol. 92, 155–194. doi: 10.1016/j.simyco.2018.06.003
Mehlich, A (1984). Mehlich 3 soil test extractant: a modification of Mehlich 2 extractant. Comm. Soil Sci. Plant Anal. 15:1409–1416. doi: 10.1080/00103628409367568
Mugo, J. N., Karanja, N. N., Gachene, C. K., Dittert, K., Gitari, H. I., and Schulte-Geldermann, E. (2021). Response of potato crop to selected nutrients in central and eastern highlands of Kenya. Cogent. Food Agric. 7:1898762. doi: 10.1080/23311932.2021.1898762
Mur, L. A. J., Simpson, C., Kumari, A., Gupta, A. K., and Gupta, K. J. (2017). Moving nitrogen to the centre of plant defence against pathogens. Ann. Bot. 119, 703–709. doi: 10.1093/aob/mcw179
Murashige, T., and Skoog, F. (1962). A revised medium for rapid growth and bioassays with tobacco tissue cultures. Physiol Plant 15:473–497. doi: 10.1111/j.1399-3054.1962.tb08052.x
Mwaura, P., Dubois, T., Losenge, T., Coyne, D., and Kahangi, E. (2010). Effect of endophytic Fusarium oxysporum on paralysis and mortality of Pratylenchus goodeyi. Afr. J. Biotechnol. 9:964. doi: 10.5897/AJB09.964
Nakintu, J., Rwakitarara, M. S., and Ochieno, D. M. W. (2008). Occurrence of Soilborne Microbes Invading Roots of Tissue Culture Bananas Inoculated With Endophytic Fusarium oxysporum V5w2. Special Project. Kampala: Faculty of Agriculture, Makerere University.
Neher, D. A (2001). Role of nematodes in soil health and their use as indicators. J. Nematol. 33, 161–168.
Nesme, J., Achouak, W., Agathos, S. N., Bailey, M., Baldrian, P., et al. (2016). Back to the future of soil metagenomics. Front. Microbiol. 7:73. doi: 10.3389/fmicb.2016.00073
Niere, B. I., Speijer, P. R., and Sikora, R. A. (1999). A novel approach to the biological control of banana nematodes. Deutscher Tropentag 1999, 1–9. Available online at: https://ftp.gwdg.de/pub/tropentag/proceedings/1999/referate/STD_C5.pdf (accessed February 14, 2022).
Nunez-Rodriguez, J. C., Ruiz-Roldán, C., Lemos, P., Membrives, S., and Hera, C. (2020). The phosphatase Ptc6 is involved in virulence and MAPK signalling in Fusarium oxysporum. Mol. Plant Pathol. 21, 206–217. doi: 10.1111/mpp.12889
Ochieno, D. M. W (2010). Endophytic Control of Cosmopolites sordidus and Radopholus similis Using Fusarium oxysporum V5w2 in Tissue Culture Banana (Ph.D. thesis and Propositions). Wageningen: Wageningen University and Research Centre. Available online at: http://library.wur.nl/WebQuery/wda/lang/1948566 (accessed February 14, 2022).
Ochieno, D. M. W (2020). Towards consensus on the transfer of Fusarium oxysporum V5w2-enhanced tissue culture banana technology to farmers through public-private partnerships in East Africa. Sci Afr. 10. doi: 10.1016/j.sciaf.2020.e00605
Ochieno, D. M. W (2021). Fusarium oxysporum V5w2 is a non-beneficial endophyte that interacts with Radopholus similis in a wilt disease complex of banana. Sci. Afr. 14:e01014. doi: 10.1016/j.sciaf.2021.e01014
Ochieno, D. M. W., Karoney, E. M., Muge, E. K., Nyaboga, E. N., Baraza, D. L., Shibairo, S. I., et al. (2021). Rhizobium-linked nutritional and phytochemical changes under multitrophic functional contexts in Sustainable Food Systems. Front. Sustain. Food Syst. 4:604396. doi: 10.3389/fsufs.2020.604396
Okalebo, J. K., Gathua, K. W., and Woomer, P. L. (2002). Laboratory Methods of Soil and Plant Analysis: A Working Manual, 2nd Edn. Nairobi: SACRED-Africa.
Olivares, B. O., Rey, J. C., Lobo, D., Navas-Cortés, J. A., Gómez, J. A., and Landa, B. B. (2021). Fusarium wilt of bananas: a review of agro-environmental factors in the Venezuelan production system affecting its development. Agronomy 11:986. doi: 10.3390/agronomy11050986
Onguso, J. M., Kahangi, E. M., Ndiritu, D. W., and Mizutani, F. (2004). Genetic characterization of cultivated bananas and plantains in Kenya by RAPD markers. Sci. Hortic. 99, 9–20. doi: 10.1016/S0304-4238(03)00082-7
Orr, R., and Nelson, P. N. (2018). Impacts of soil abiotic attributes on Fusarium wilt, focusing on bananas. Appl. Soil. Ecol. 132, 20–33. doi: 10.1016/j.apsoil.2018.06.019
Paliwoda, D., and Mikiciuk, G. (2020). Use of rhizosphere microorganisms in plant production–a review study. J. Ecol. Eng. 21, 292–310. doi: 10.12911/22998993/126597
Paparu, P., Adele, M., Dubois, T., Coyne, D., and Viljoen, A. (2009a). Efficacy of chemical and fluorescent protein markers in studying plant colonization by endophytic non-pathogenic Fusarium oxysporum isolates. Biol. Control 54, 709–722. doi: 10.1007/s10526-009-9221-1
Paparu, P., Dubois, T., Coyne, D., and Viljoen, A. (2007). Defense-related gene expression in susceptible and tolerant bananas (Musa spp.) following inoculation with non-pathogenic Fusarium oxysporum endophytes and challenge with Radopholus similis. Physiol. Mol. Plant Pathol. 71, 149–157. doi: 10.1016/j.pmpp.2007.12.001
Paparu, P., Dubois, T., Coyne, D., and Viljoen, A. (2009b). Dual inoculation of Fusarium oxysporum endophytes in banana: effect on plant colonization, growth and control of the root burrowing nematode and the banana weevil. Biocontrol Sci. Technol. 19, 639–655. doi: 10.1080/09583150902972206
Paparu, P., Dubois, T., Coyne, D., and Viljoen, A. (2010). Effect of Fusarium oxysporum endophyte inoculation on the activities of phenylpropanoid pathway enzymes and Radopholus similis numbers in susceptible and tolerant East African highland bananas. Nematology 12, 469–480. doi: 10.1163/138855409X12571623969646
Paparu, P., Dubois, T., Coyne, D., and Viljoen, A. (2013). Differential gene expression in East African highland bananas (Musa spp.): Interactions between non-pathogenic Fusarium oxysporum V5w2 and Radopholus similis. Physiol. Mol. Plant P. 82, 56–63. doi: 10.1016/j.pmpp.2012.10.003
Paparu, P., Dubois, T., Gold, C. S., Niere, B., Adipala, E., and Coyne, D. (2006). Colonisation pattern of non-pathogenic Fusarium oxysporum, a potential biological control agent, in roots and rhizomes of tissue cultured Musa plantlets. Ann. Appl. Biol. 149, 1–8. doi: 10.1111/j.1744-7348.2006.00064.x
Pegg, K. G., Coates, L. M., O'Neill, W. T., and Turner, D. W. (2019). The epidemiology of Fusarium wilt of banana. Front. Plant Sci. 10:1395. doi: 10.3389/fpls.2019.01395
Pfaff, J., Denton, A. K., Usadel, B., and Pfaff, C. (2020). Phosphate starvation causes different stress responses in the lipid metabolism of tomato leaves and roots. Biochim. Biophys. Acta 1865:158763. doi: 10.1016/j.bbalip.2020.158763
Poveda, J., Abril-Urias, P., and Escobar, C. (2020). Biological control of plant-parasitic nematodes by filamentous fungi inducers of resistance: trichoderma, mycorrhizal and endophytic fungi. Front. Microbiol. 11:992. doi: 10.3389/fmicb.2020.00992
Rebouças, T. A., Haddad, F., Ferreira, C. F., de Oliveira, S. A. S., da Silva Ledo, C. A., and Amorim, E. P. (2018). Identification of banana genotypes resistant to Fusarium wilt race 1 under field and greenhouse conditions. Sci. Hort. 239, 308–313. doi: 10.1016/j.scienta.2018.04.037
Reeves, D. W., Mask, P. L., Wood, C. W., and Delaney, D. P. (1993). Determination of wheat nitrogen status with a hand-held chlorophyll meter: influence of management practices. J. Plant Nutr. 16, 781–796. doi: 10.1080/01904169309364574
Rocha, A. d. J., Ferreira, M. d. S., Rocha, L. d. S., Oliveira, S. A. S., Amorim, E. P., Mizubuti, E. S. G., et al. (2020). Interaction between Fusarium oxysporum f. sp. cubense and Radopholus similis can lead to changes in the resistance of banana cultivars to Fusarium wilt. Eur. J. Plant Pathol. 158, 403–417. doi: 10.1007/s10658-020-02081-y
SAS. (2004). Statistical Analysis System User's Guide: Statistics, Version 9.1. Charlotte, NC: SAS Institute. Available online at: https://support.sas.com/documentation/onlinedoc/91pdf/sasdoc_91/stat_ug_7313.pdf
Schouten, A (2016). Mechanisms involved in nematode control by endophytic fungi. Annu. Rev. Phytopathol. 54, 121–142. doi: 10.1146/annurev-phyto-080615-100114
Schulz, B., and Boyle, C. (2006). “What are endophytes?” in Microbial Root Endophytes. Soil Biology, vol 9, eds B. J. E. Schulz, C. J. C. Boyle, and T. N. Sieber (Berlin; Heidelberg: Springer), 9. doi: 10.1007/3-540-33526-9
Schuster, R. P., Sikora, R. A., and Amin, N. (1995). Potential of endophytic fungi for the biological control of plant parasitic nematodes. Commun. Agric. Appl. Biol. Sci. 60,1047–1052.
Seenivasan, N (2017). Identification of burrowing nematode (Radopholus similis) resistance in banana (Musa spp) genotypes for natural and challenge inoculated populations. Arch. Phytopathol. Plant Prot. 50, 415–437. doi: 10.1080/03235408.2017.1321871
Segura-Mena, R. A., Stoorvogel, J. J., García-Bastidas, F., Slacinas-Niez, M., Kema, G. H. J., and Sandoval, J. A. (2021). Evaluating the potential of soil management to reduce the effect of Fusarium oxysporum f. sp. cubense in banana (Musa AAA). Eur. J. Plant Pathol. 160, 441–455. doi: 10.1007/s10658-021-02255-2
Sharpton, T. J (2014). An introduction to the analysis of shotgun metagenomic data. Front. Plant Sci. 5:209. doi: 10.3389/fpls.2014.00209
Shaw, L. J., Beaton, Y., Glover, L. A., Killham, K., and Meharg, A. A. (1999). Re-inoculation of autoclaved soil as a non-sterile treatment for xenobiotic sorption and biodegradation studies. Appl. Soil Ecol. 11, 217–226. doi: 10.1016/S0929-1393(98)00149-8
Shen, Z., Ruan, Y., Xue, C., Zhong, S., Li, R., Shen, Q., et al. (2015). Soils naturally suppressive to banana Fusarium wilt disease harbor unique bacterial communities. Plant Soil 393, 21–33. doi: 10.1007/s11104-015-2474-9
Shin, R., Berg, R. H., and Schachtman, D. P. (2005). Reactive oxygen species and root hairs in Arabidopsis root response to nitrogen, phosphorus and potassium deficiency. Plant Cell Physiol. 46, 1350–1357. doi: 10.1093/pcp/pci145
Siamak, S. B., and Zheng, S. (2018). Banana Fusarium wilt (Fusarium oxysporum f. sp. cubense) control and resistance, in the context of developing wilt-resistant bananas within sustainable production systems. Hortic. Plant J. 4, 208–218. doi: 10.1016/j.hpj.2018.08.001
Siedliska, A., Baranowski, P., Pastuszka-Wozniak, J., Zubik, M., and Krzyszczak, J. (2021). Identification of plant leaf phosphorus content at different growth stages based on hyperspectral reflectance. BMC Plant Biol. 21:28. doi: 10.1186/s12870-020-02807-4
Sikora, R. A., and Pocasangre, L. (2006). “The concept of a suppressive banana plant: root health management with a biological approach,” in Proceedings of the XVII ACROBAT International Congress, Vol. 1. (Joinville–Santa Catarina), p. 241–248.
Sikora, R. A., Pocasangre, L., zum Felde, A., Niere, B., Tam, T. V., and Dababat, A. A. (2008). Mutualistic endophytic fungi and in-planta suppressiveness to plant parasitic nematodes. Biol. Control 46, 15–23. doi: 10.1016/j.biocontrol.2008.02.011
Sinegani, A. A. S., and Sedri, S. (2011). Effects of sterilization and temperature on the decrease kinetic of phosphorus bioavailability in two different soil types. J. Soil Sci. Plant Nutr. 11, 109–122. doi: 10.4067/S0718-95162011000200010
Soumare, A., Diédhiou, A. G., Arora, N. K., Al-Ani, L. K. T., Ngom, M., Fall, S., et al. (2021). Potential role and utilization of plant growth promoting microbes in plant tissue culture. Front. Microbiol. 12:649878. doi: 10.3389/fmicb.2021.649878
Speijer, P. R., and De Waele, D. (1997). Screening of Musa germplasm for Resistance and Tolerance to Nematodes, INIBAP Technical Guidelines 1. Montpellier: The International Network for the Improvement of Banana and Plantain. INIBAP-ISBN 2-910810-16-X
Speijer, P. R., and Sikora, R. A. (1993). “Influence of a complex disease involving Pratylenchus goodeyi and a non-pathogenic strain of Fusarium oxysporum on banana root health,” in Biological and Integrated Control of Highland Banana and Plantain Pests and Diseases: Proceedings of a Research Coordination Meeting. (Cotonou), 218–230. Available online at: https://www.cabdirect.org/cabdirect/abstract/19952307862 (accessed November 12, 1991).
Tarafdar, J. C., Yadav, R. S., and Meena, S. C. (2001). Comparative efficiency of acid phosphatase originated from plant and fungal sources. J. Plant. Nutr. Soil Sci. 164, 279–282. doi: 10.1002/1522-2624(200106)164:3<279::AID-JPLN279>3.0.CO;2-L
Taylor, J. W (2014). Appropriately sized genera and appropriately ranked higher taxa. IMA Fungus 5, A1–A2. doi: 10.1007/BF03449333
Thomas, P., and Sekhar, A. C. (2017). Cultivation versus molecular analysis of banana (Musa sp.) shoot-tip tissue reveals enormous diversity of normally uncultivable endophytic bacteria. Microb. Ecol. 73, 885–899. doi: 10.1007/s00248-016-0877-7
Timmis, K., de Vos, W. M., Ramos, J. L., Vlaeminck, S. E., Prieto, A., Danchin, A., et al. (2017). The contribution of microbial biotechnology to sustainable development goals. Microb. Biotechnol. 10, 984–987. doi: 10.1111/1751-7915.12818
Topalović, O., and Vestergård, M. (2021). Can microorganisms assist the survival and parasitism of plant-parasitic nematodes? Trends Parasitol. 37, 947–958. doi: 10.1016/j.pt.2021.05.007
Tripathi, L., Atkinson, H., Roderick, H., Kubiriba, J., and Tripathi, J. N. (2017). Genetically engineered bananas resistant to Xanthomonas wilt disease and nematodes. Food Energy Secur. 6, 37–47. doi: 10.1002/fes3.101
Tripathi, L., Babirye, A., Roderick, H., Tripathi, J. N., Changa, C., Urwin, P. E., et al. (2015). Field resistance of transgenic plantain to nematodes has potential for future African food security. Sci. Rep. 5, 1–10. doi: 10.1038/srep08127
Tripathi, L., Ntui, V. O., and Tripathi, J. N. (2019). Application of genetic modification and genome editing for developing climate-smart banana. Food Energy Secur. 8:e00168. doi: 10.1002/fes3.168
Tripathi, L., Ntui, V. O., and Tripathi, J. N. (2020). CRISPR/Cas9-based genome editing of banana for disease resistance. Curr. Opin. Plant Biol. 56, 118–126. doi: 10.1016/j.pbi.2020.05.003
Troelstra, S. R., Wagenaar, R., Smant, W., and Peters, B. A. M. (2001). Interpretation of bioassays in the study of interactions between soil organisms and plants: involvement of nutrient factors. New Phytol. 150, 697–706. doi: 10.1046/j.1469-8137.2001.00133.x
van Dam, N. M., and Bouwmeester, H. J. (2016). Metabolomics in the rhizosphere: tapping into belowground chemical communication. Trends Plant Sci. 21, 256–265. doi: 10.1016/j.tplants.2016.01.008
Van der Veken, L., Cabasan, M. N., Elsen, A., Swennen, A., and De Waele, D. (2021). Effect of single or dual inoculation of the arbuscular mycorrhizal fungus Glomus mosseae and root-nodulating rhizobacteria on reproduction of the burrowing nematode Radopholus similis on non-leguminous and leguminous banana intercrops. J. Plant Dis. Prot. 128, 961–971. doi: 10.1007/s41348-021-00429-y
Vieira, F. C. S., and Nahas, E. (2005). Comparison of microbial numbers in soils by using various culture media and temperatures. Microbiol. Res. 160, 197–202. doi: 10.1016/j.micres.2005.01.004
Vives-Peris, V., de Ollas, C., Gómez-Cadenas, A., and Pérez-Clemente, R. M. (2020). Root exudates: from plant to rhizosphere and beyond. Plant Cell Rep. 39, 3–17. doi: 10.1007/s00299-019-02447-5
Vu, T. T., Hauschild, R., and Sikora, R. A. (2006). Fusarium oxysporum endophytes induced systemic resistance against Radopholus similis on banana. Nematology 8, 847–852. doi: 10.1163/156854106779799259
Vuylsteke, D. R (1998). Shoot-Tip Culture for the Propagation, Conservation, and Distribution of Musa Germplasm. Ibadan: International Institute of Tropical Agriculture. ISBN 13:978-92-9043-140-4
Wabule, M. N., Ngaruiya, P. N., Kimmins, F. K., and Silverside, P. J. (2003). “Registration for biocontrol agents in Kenya,” in Proceedings of the Pest Control Products Board/Kenya Agricultural Research Institute/Department for International Development Crop Protection Programme Workshop. (Aylesford: Natural Resources International Ltd). Available online at: https://assets.publishing.service.gov.uk/media/57a08cc040f0b649740013fe/R7299Registration.pdf (accessed February 14, 2022).
Walkley, A (1947). A critical examination of a rapid method for determining organic carbon in soils: effect of variations in digestion conditions and of inorganic soil constituents. Soil Sci. 37, 29–38. doi: 10.1097/00010694-193401000-00003
Walters, D. R (2009). Are plants in the field already induced? Implications for practical disease control. Crop. Prot. 28, 459–465. doi: 10.1016/j.cropro.2009.01.009
Wang, D., Lv, S., Jiang, P., and Li, Y. (2017). Roles, regulation, and agricultural application of plant phosphate transporters. Front. Plant Sci. 8:817. doi: 10.3389/fpls.2017.00817
Wang, Y., Wang, F., Lu, H., Liu, Y., and Mao, C. (2021). Phosphate uptake and transport in plants: an elaborate regulatory system. Plant Cell Physiol. 62, 564–572. doi: 10.1093/plphys/kiab343
Warman, N. M., and Aitken, E. A. B. (2018). The movement of Fusarium oxysporum f. sp. cubense (Sub-Tropical Race 4) in susceptible cultivars of banana. Front. Plant Sci. 9:1748. doi: 10.3389/fpls.2018.01748
Waweru, B. W., Losenge, T., Kahangi, E. M., Dubois, T., and Coyne, D. (2013). Potential biological control of lesion nematodes on banana using Kenyan strains of endophytic Fusarium oxysporum. Nematology 15, 101–107. doi: 10.1163/156854112X645606
Zakeel, M. C. M., and Safeena, M. I. S. (2020). “Metagenomic insights into interactions between plant nematodes and endophytic microbiome,” in Management of Phytonematodes: Recent Advances and Future Challenges, eds R. Ansari, R. Rizvi, and I. Mahmood (Singapore: Springer), 5. doi: 10.1007/978-981-15-4087-5_5
Zhang, Y., Li, S., Li, H., Wang, R., Zhang, K. Q., and Xu, J. (2020). Fungi-nematode interactions: diversity, ecology, and biocontrol prospects in agriculture. J. Fungi 6:206. doi: 10.3390/jof6040206
Zhang, Z., Qu, Y., Li, S., Feng, K., Wang, S., Cai, W., et al. (2017). Soil bacterial quantification approaches coupling with relative abundances reflecting the changes of taxa. Sci. Rep. 7:4837. doi: 10.1038/s41598-017-05260-w
Zinov'eva, S. V., Vasyukova, N. I., and Ozeretskovskaya, O. L. (2004). Biochemical aspects of plant interactions with phytoparasitic nematodes: a review. Appl. Biochem. Microbiol. 40, 111–119. doi: 10.1023/B:ABIM.0000018912.93529.78
zum Felde, A (2011). Endophytes: Novel Weapons in the IPM Arsenal. CGIAR SPIPM Technical Innovation Brief. SP-IPM Secretariat. p. 2. Available online at: https://hdl.handle.net/10568/87941 (accessed February 14, 2022).
Keywords: Fusarium oxysporum V5w2, nitrogen-phosphorus-potassium, Radopholus similis, rhizosphere microbes, soil sterilization, tissue culture
Citation: Ochieno DMW (2022) Soil Microbes Determine Outcomes of Pathogenic Interactions Between Radopholus similis and Fusarium oxysporum V5w2 in Tissue Culture Banana Rhizospheres Starved of Nitrogen, Phosphorus, and Potassium. Front. Sustain. Food Syst. 6:706072. doi: 10.3389/fsufs.2022.706072
Received: 06 May 2021; Accepted: 03 February 2022;
Published: 01 March 2022.
Edited by:
Nicolas Desoignies, HEPH Condorcet, BelgiumReviewed by:
Zongzhuan Shen, Nanjing Agricultural University, ChinaFederico Lopez-Moya, University of Alicante, Spain
Copyright © 2022 Ochieno. This is an open-access article distributed under the terms of the Creative Commons Attribution License (CC BY). The use, distribution or reproduction in other forums is permitted, provided the original author(s) and the copyright owner(s) are credited and that the original publication in this journal is cited, in accordance with accepted academic practice. No use, distribution or reproduction is permitted which does not comply with these terms.
*Correspondence: Dennis M. W. Ochieno, dochieno@mmust.ac.ke; dwochieno@gmail.comorcid.org/0000-0003-3985-9421
†Present address: Dennis M. W. Ochieno, Department of Biological Sciences, School of Natural Sciences, Masinde Muliro University of Science and Technology, Kakamega, Kenya;Biodiversity and Ecosystem Services Section, Centre for African Medicinal and Nutritional Flora and Fauna (CAMNFF), Techno Park, Masinde Muliro University of Science and Technology (MMUST), Kakamega, Kenya