The bacterial world inside the plant
- 1Agricultural and Livestock Microbiology Graduation Program, School of Agricultural and Veterinarian Sciences, São Paulo State University (UNESP), São Paulo, Brazil
- 2Phytopathology, Microbial and Molecular Farming Lab, Center D'Etudes et Recherche Appliquée-Haute Ecole Provinciale du Hainaut Condorcet, Ath, Belgium
Sustainable agriculture requires the recruitment of bacterial agents to reduce the demand for mineral fertilizers and pesticides such as bacterial endophytes. Bacterial endophytes represent a potential alternative to the widespread use of synthetic fertilizers and pesticides in conventional agriculture practices. Endophytes are formed by complex microbial communities and microorganisms that colonize the plant interior for at least part of their life. Their functions range from mutualism to pathogenicity. Bacterial endophytes colonize plant tissues, and their composition and diversity depend on many factors, including the plant organ, physiological conditions, plant growth stage, and environmental conditions. The presence of endophytes influences several vital activities of the host plant. They can promote plant growth, elicit a defense response against pathogen attack, and lessen abiotic stress. Despite their potential, especially with regard to crop production and environmental sustainability, research remains sparse. This review provides an overview of the current research, including the concept of endophytes, endophytes in plant organs, endophyte colonization, nutrient efficiency use, endophytes and crop nutrition, inoculation with synergistic bacteria, the effect of inoculum concentration on plant root microbiota and synthetic communities. It also examines the practical opportunities and challenges when utilizing endophytes in the field of sustainable agriculture. Finally, it explores the importance of these associations with regard to the future of agriculture and the environment.
Introduction
With a growing global population and limited land resources, agricultural intensification is essential for global food security (Jayne et al., 2019; Devika et al., 2021). However, the use of chemical fertilizer to improve crop yield has had a negative effect on soil quality (Adeleke et al., 2021). The consumption of some foods grown in fertilized fields treated with agrochemicals has been associated with human diseases (Babalola, 2010). Additionally, recent reports have shown that 52% of all fertile food-producing soils globally are now classified as degraded, which poses more new challenges for growing crops. Global food production is expected to decline by 12% over the next 25 years (UNCCD Brochure, 2012; Patel and Minocheherhomji, 2018). Therefore, it is imperative that we establish sustainable and non-hazardous agricultural practices.
Microbial communities in plants represent a potential solution to the decline in global food production. Plant growth and health are modulated by microbial communities that colonize their tissues. Although some microbes are detrimental and cause diseases, others may promote plant growth and tolerance to biotic and abiotic stresses by enhancing nutrient acquisition via many mechanisms (Brader et al., 2017).
The application of plant growth-promoting microorganisms (PGPs) may improve plant production under unfavorable conditions, with the potential to reduce the use of chemical fertilizers and pesticides. However, the current application of endophytes, especially in the field, faces a number of challenges. For example, microbial application may not completely replicate the effectiveness of chemical fertilizers (Sessitsch et al., 2019). In addition, PGP may fail to induce plant growth due to insufficient rhizosphere colonization (Lugtenberg et al., 2001). Many mechanisms that are related to plant growth promotion necessitate further investigation, including the steps involved in plant colonization by PGPs, plant–soil interactions of root endophytes, and microbes associated with all plant parts. Additionally, the interaction of microbes living within the same plant, how they modulate above- and belowground communities, and the involved processes all require further examination to improve the efficiency of the inoculant strain (Compant et al., 2016).
Currently, microbiome research has received tremendous attention due to several factors related to plant growth promotion. Usually, this research begins with screening different isolates for specific characteristics that are related to plant growth under controlled conditions, and then further evaluations are conducted under uncontrolled field conditions (Figure 1). Associations between endophytes and their hosts support health and plant growth (Sessitsch et al., 2019). Figure 2 shows a schematic representation comparing uninoculated and inoculated plants with bacterial endophytes.
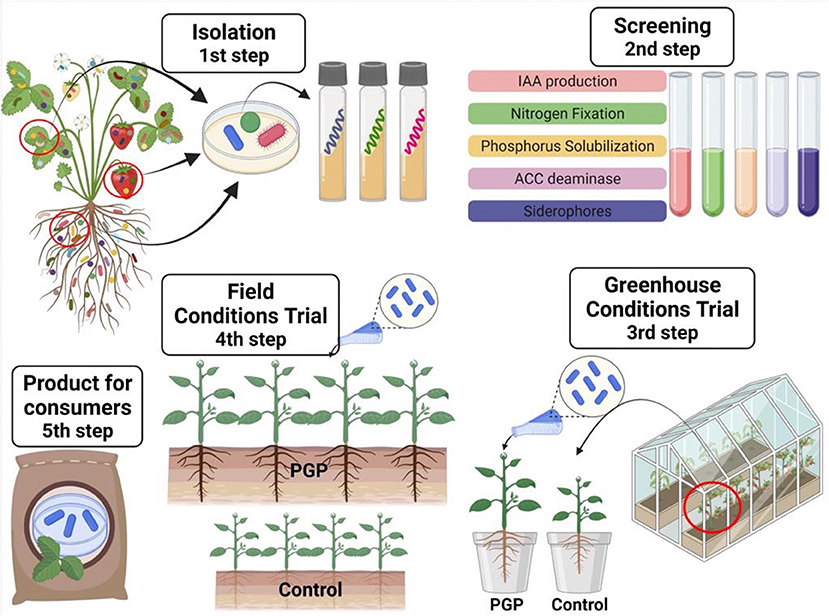
Figure 1. Schematic representation of the steps required to isolate and characterize bacteria that promote plant growth.
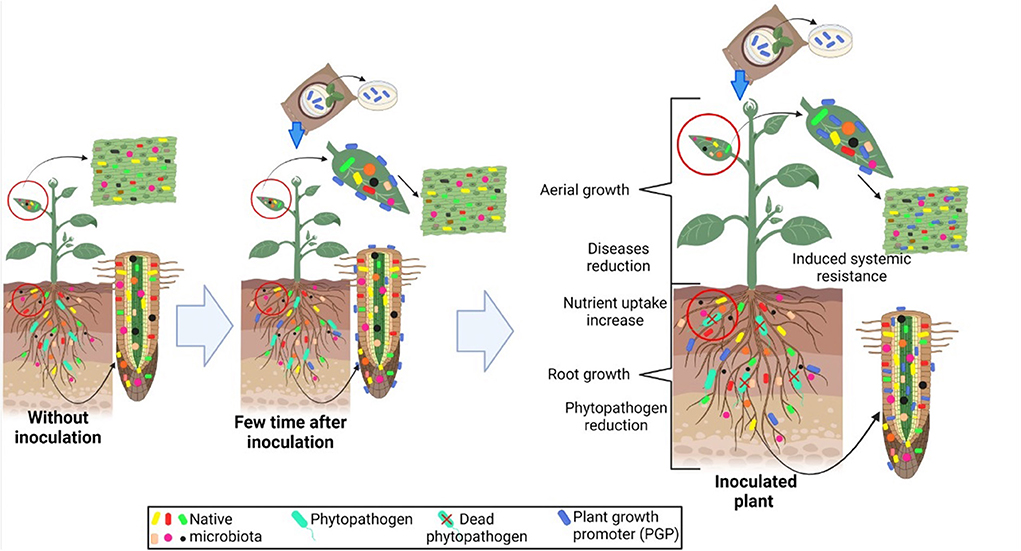
Figure 2. Schematic representation comparing uninoculated and inoculated plants with bacterial endophytes, as well as several abilities related to plant growth promotion in crops, including aerial growth, disease reduction, nutrient uptake increase, root growth, phytopathogen reduction, and induced systemic resistance.
Most plant-associated bacteria are derived from the soil environment (Compant et al., 2010). Microorganisms that live inside the plant are called endophytes. They consist of several microorganisms that spend either their full or partial life cycle colonizing plant tissues. However, the definition of “endophyte” has been modified over time (Hardoim et al., 2015). Endophytes may cause diseases or live as symbionts. They rely on many factors, including the environment, plant genotype, and microbiota (Brader et al., 2017). Hardoim et al. (2015) previously suggested that the term “endophyte” should refer to all microorganisms that colonize the plant tissues for either their whole lifetime or part of their lifetime. One study showed that some species of endophytes may be either pathogenic or beneficial. While most endophytes are innocuous toward select plant species, they may be pathogenic toward other plants (Fadiji and Babalola, 2020). The pathogenicity shown by some endophytes may be attributed to environmental factors. Fluorescent pseudomonads may be pathogenic or beneficial to most plants depending on the conditions. In the present review, the term “endophyte” refers to any microorganism, including pathogens, that can colonize the internal tissues of plants.
Endophytes in plant organs
The best-characterized endophytes are associated with roots and leaves and live on or inside plant organs (Bulgarelli et al., 2013). Different plant organs house a wide range of microbiota that exert a strong influence on each compartment, such as flowers, fruits, and seeds (Compant et al., 2010). Different chemical compositions are found in roots, stems, leaves, fruits, and seeds, which promote the colonization of several bacterial communities responsible for plant growth, stress adaptation and defense (Hounsome et al., 2008). The degree of colonization by microbiota is dependent on the different chemical compositions of each plant organ (Bulgarelli et al., 2013).
The microorganisms associated with plants are essential for seed germination and healthy crop development (Firdous et al., 2019). Plants that allow endophyte entrance, seed colonization, and vertical transmission for subsequent generations benefit from distinct advantages (Truyens et al., 2013). The majority of the microbial genera found in the microbiome are also found in the soil and play an essential role in conservation, germination, and seed development. A fascinating review by Samreen et al. (2021) addressed associated bacterial and fungal endophytes: diversity, life cycle, transmission, and application potential.
Typically, bacterial communities living in different organs of the same plant and in the rhizosphere soil possess similar characteristics, and each group of microbes is determined by the genetics of the plant host, growing conditions, and the interactions between the environment and individual plants (Wearn et al., 2012; Baldan et al., 2014). These results suggest that the plants attract endophytes that will provide certain compounds, hormones, and metabolites. However, chemotactic behavior alone does not fully explain endophyte host levels. Soil microorganisms may sense and respond to multiple chemoattractants simultaneously. The behavior of soil microorganisms may be influenced by abiotic and biotic environmental factors during the colonization of different plant organs. Chemoattractant or repellent molecules may be released in the field to modulate the actions of microorganisms, improving the growth of crop plants. With the identification of more chemoattractants, additional strategies may be used to guarantee endophyte colonization in different host plant organs (Tsai et al., 2020).
Endophyte colonization
The abundance and diversity of endophytes may be modulated by the plant immune system, thereby maintaining an adequate bacterial population in different plant organs (Liu et al., 2017). High bacterial cell density can be detrimental to host organs. For instance, high cell density induces quorum-sensing regulated processes, such as virulence and pathogenicity, that are crucial for beneficial functions (Hartmann et al., 2014).
Bacterial taxa rely on the specific environment promoted by the plant compartment to determine their occurrence, abundance, and activities (Brader et al., 2017).
Typically, three classes of bacterial endophytes are mentioned. They include the following: endophytes that are not able to reproduce in the absence of plant tissue - these endophytes are spread from seed to seed; facultative endophytes - these endophytes typically live in the soil and opportunistically colonize the plant tissues; and passive endophytes - these endophytes do not typically occupy the plant tissues, but depending on the circumstances, they may colonize the tissues via wounds on the root hairs (Hardoim et al., 2015). In the soil environment, microorganisms that reach the rhizosphere are influenced by root exudates supporting the establishment and development of the microbial community (Lemanceau et al., 2017).
Plants have the ability to detect several environmental factors, such as the presence of beneficial microbes, nutrients, and hydric stress, including the presence of pathogens. Plant exudation is modulated by a combination of these factors (Li et al., 2018).
Plants release approximately 10–40% of their fixed carbon made by photosynthesis through their roots in both inorganic and organic forms. The secretion and diffusion of these exudates are chemically diverse and include compounds such as cellulose, fatty acids, phenolics, nucleotides, putrescine, sterols, vitamins, organic acids and amino acids (Sasse et al., 2018). The bacteria must be able to colonize the rhizosphere using the available nutrients and compete with several microorganisms interacting with the plant genotype (Taulé et al., 2021).
The soil microbiome is modulated by the root environment, root exudates, and root morphology. In addition, bacterial endophytes become established in different plant organs (Rodríguez et al., 2018; Sasse et al., 2018). Several factors can shape endophyte colonization in the soil and inside the leaves, such as the plant genotype and environment (Vincent et al., 2018). The success of endophyte colonization within plant leaves can be influenced by competition with other endophytes and their capacity to face imposed challenges (Vacher et al., 2016). The competition for limited resources within communities of organisms determines each species' realized niche (Chase, 2003). The composition of the bacterial communities and their activities limit the species of bacterial endophytes that colonize the plant leaf (Van Bael et al., 2017).
Plants promote a favorable environment for endophyte colonization, and consequently, endophytes will compete with a colonizing pathogen (Ghazi et al., 2021). Kennedy (2010) demonstrated a reduction in pathogen colonization when the establishment of beneficial microbes occurred first. Berg and Raaijmakers (2018) verified that plant seeds may be home to several microbial endophytes whose composition is determined by the environment, agricultural practices and plant genotype.
Thus, some bacterial traits improve the bacterial interaction and colonization of the plant. Endophytic bacteria are great competitors due to their diverse ability to produce secondary metabolites that can reduce several pathogens, protect against predatory organisms, and guarantee their colonization in the plant (Taulé et al., 2021). Specific microbes can colonize plant seeds and are transmitted vertically to various generations (Shade et al., 2017). Additionally, microbes can be transmitted horizontally to different environments Figure 2.
Inoculation with synergistic bacteria
The inoculation with synergistic bacteria with plant growth-promoting abilities is becoming increasingly common. This practice has some advantages, such as an increase in the successful establishment of bacteria in the rhizosphere and in the condition of interaction with plants (Bulgarelli et al., 2013). Some studies on the combination of PGPR have found that most of the microorganisms used in mixtures did not interfere negatively with each other (Kaur et al., 2015; Korir et al., 2017; Vandana et al., 2021). However, few reports indicate that certain mixtures of microbial strains do not show synergistic or at least comparable effects on plant growth promotion concerning the separate application of microorganisms (Felici et al., 2008; Lobo et al., 2022). These authors support the hypothesis that the co-presence of the two microorganisms may alter, directly or indirectly, the internal hormone content of the root, interfering with the normal morphogenesis of the root itself.
Effect of inoculum concentration on plant root microbiota
Under field conditions, PGPMs are applied in the form of formulated products, these new formulations have increased the concentration of microorganisms involved in the field. However, despite the advance in the use of inoculants in agriculture, few studies have evaluated the effect of inoculant concentration on plant growth promotion. Thus, this theme has become essential to define whether the increase in the concentration of microorganisms is an important aspect related to product efficiency or whether it is just an aspect of commercial advantage. Escobar Diaz et al. (2021) evaluated the effect of B. subtilis concentration in cotton crop under field conditions and verified that the parameters that were favored by the highest inoculant concentrations were soil respiratory activity, phosphorus in root dry matter, nitrogen in shoot dry matter, and number of colonies forming units in roots and leaves. Concentrations did not affect nitrogen in root dry matter, phosphorus in shoot dry matter, and microbial biomass carbon. Interestingly, concentrations did not affect cotton fiber or seed yield. dos Santos et al. (2022) evaluated the effect of inoculant concentration based on B. subtilis on soybean crops and verified that B. subtilis inoculations did not affect the endophytic community of roots. Bueno et al. (2022) showed a strong tendency for the inoculant concentration to increase and the necessity of mineral fertilization dose for the plants to achieve the same development. The authors proposed that these high inoculum concentrations promoted an increase in the bacterial rhizospheric population, and as a consequence, more photosynthetic metabolites from the plants and more nutrients from the soil are needed. Thus, the need for mineral fertilization for plant growth must be increased. More studies are needed to understand better the effect of inoculum concentration on the microbiota.
Nutrient use efficiency
Global agriculture suffers a high economic pressure for food production. Thus, a significant number of chemical fertilizers are used annually. These chemical fertilizers use energy extracted from fossil fuels that are pollutant resources and spread gases related to the greenhouse effect. In addition, the indiscriminate use of chemical fertilizers neglects the principles of management and soil conservation, promoting damage to soil health and accelerating nutrient depletion, acidification, and erosion (Brevik, 2012; Sarkar et al., 2017). Moreover, the low use of organic fertilizers has worsened soil fertility (Sarkar et al., 2021a). Chemical fertilizer practices and cereal production worldwide have exacerbated the negative balance of nutrients in soil (Sanyal et al., 2014). Among agricultural crops, nutrient efficiency use is less than 50% in most agricultural regions (Baligar et al., 2001). Factors such as energy crises and reduction of nutrient efficiency form a great challenge for modern agriculture (Sarkar et al., 2021b). Some studies have demonstrated that the consumption of fossil fuels, changes in the climate scenario, soil degradation, and food security have resulted in a cyclical degradation process of natural resources, low productivity, and low nutrient efficiency use (Rakshit, 2019). Therefore, breaking this deleterious cycle is a necessary practice. Technology that promotes nutrient availability in the soil improves the nutrient supply to plants and enhances the plant's capacity to take up nutrients (Aloo et al., 2019).
To face this challenge, using plant growth-promoting microorganisms is an excellent strategy for reducing the use of agrochemicals and the environmental impact without reducing crop yield. Trichoderma harzianum, Pseudomonas fluorescens and Bacillus subtilis have been used to increase macronutrient uptake (N, P, and K), root length, heading percentage, head diameter, head weight, and the total weight of red cabbage (Sarkar et al., 2021c). In another study, Trichoderma harzianum, Pseudomonas fluorescens, and Bacillus subtilis were applied singly and in combination with 75% of the recommended dose fertilizer (RDF). The authors verified that compatible microbes improved the nutrient composition and bioactive compounds of red cabbage, that the performance of the dual consortium was better than that of the triple consortium and that the reduced fertilizer dose (75% RDF) in combination with Trichoderma sp. and Pseudomonas sp. achieved the highest head yield (Sarkar and Rakshit, 2021). Cipriano et al. (2021) inoculated sugarcane with Paraburkholderia caribensis, Kosakonia radicincitans, Paraburkholderia tropica, Pseudomonas fluorescens, and Herbaspirillum frisingense. They showed that the nutrient-use efficiency increased by an average of 250–300% compared the treatments to the control. The integrated use of microbes for nutrient and disease management, along with organic manures and inorganic fertilizers, has been suggested as the most effective method to maintain healthy and sustainable soil while increasing crop productivity. Inoculation with these methods increased crop yields by ~10–15% under farm conditions (Kumar et al., 2021). The application of B. subtilis and P. putida increased the percentage of nitrogen by ~ 40% compared to the control (Mehrasa et al., 2022). Bacillus sp., Pseudomonas sp., Staphylococcus sp., Paenibacillus sp., Stenotrophomonas sp., Sphingobacterium sp., Lysinibacillus sp., Advenella sp., Enterobacter sp., Variovorax sp., and Plantibacter sp. were evaluated in wheat and increased phosphorus acquisition efficiency by 29.5% (Emami et al., 2020). The inoculation of Herbaspirillum seropedica in rice crops increased the shoot nutrient contents of N, P, K, Ca, Mg, and S by 120, 350, 430, 150, 110, and 300%, respectively (Ramos et al., 2020).
Sarkar et al. (2021b) have written a fascinating review concerning nutrient use efficiency. In this review, the author demonstrated that rhizosphere chemistry and other mechanisms of plant–microbial interactions will frame suitable strategies to harness the best ecosystem services, including improved resource use efficiency.
Endophytes and crop nutrition
Nitrogen (N) is essential for maintaining vital activities in the cell. It is an essential component of various important molecules responsible for maintaining cell functions. These molecules include adenosine triphosphate (ATP), nucleic acids, and chlorophyll (Werner and Newton, 2005). This nutrient, as well as oxygen, carbon, and hydrogen, is required in all living biomass (Howarth et al., 2009). The process responsible for converting organic nitrogen to inorganic nitrogen, thereby enabling plant use, is a combination of nitrification and ammonification (Paul and Kumar, 2015). The bacterium Rhizobia is the most studied symbiotic N2-fixing organism in agriculture (Shamseldin et al., 2017). Biological nitrogen fixation (BNF) is a process in which nitrogen is converted to ammonia by nitrogenase activity, thereby becoming available for microbe and plant assimilation (Wichern et al., 2008). The atmosphere is the principal major reservoir of nitrogen. However, for nitrogen to be absorbed by plants, it must first be fixed by bacteria.
Pseudomonas fluorescens has been used to reduce the amount of N fertilizer applied to wheat crops. The fungus Trichoderma harzianum promoted a 25% reduction in the nitrogen dose for the same crop. Duarah et al. (2011) reported a reduction in NPK fertilizer application using seeds inoculated with bacteria and verified a considerable enhancement in NUE.
Phosphorus is essential for plant growth and affects many biological processes. It is required in photosynthesis, signal transduction, molecule biosynthesis, and respiration processes. A tremendous quantity of phosphorus is present in soils in both organic and mineral forms. Nevertheless, the main factor limiting plant growth in different environments is its availability (Mitter et al., 2021). Soil microbes can mineralize phosphorus into plant-mineral forms through several mechanisms of mineralization and solubilization (Alori et al., 2017). Phosphate-solubilizing microbes (PSMs) utilize various mechanisms to produce and release different compounds that solubilize organic phosphorus immobilized in organic matter and inorganic P, such as tricalcium phosphate, rock phosphate, and hydroxyapatite. One such mechanism is the production of organic acids, CO2, and hydroxyl ions (Wei et al., 2018).
Inoculation of wheat seeds with P. fluorescens and NPK fertilizer application at 25, 50, 75, and 100% resulted in enhanced P use efficiency, at 102, 56, 57, and 21%, respectively, when compared with corresponding uninoculated treatments in wheat field studies (Duarah et al., 2011). Seed priming with a conidial suspension of T. harzianum (1 × 108 spores/mL) significantly increased the P uptake by sunflower (Helianthus annuus L.) in a greenhouse study (Nagaraju et al., 2012).
Potassium (K) is another important element for plant growth. It is an essential nutrient in the cell cytoplasm and functions and is related to protein synthesis and photosynthesis. Potassium is the most abundant element on Earth and the second most abundant nutrient in the soil. However, the K present in the soil at approximately 98% is in a non-soluble form. Potassium is trapped within mica and feldspar minerals in the soil, such as muscovite and biotite. Another portion, approximately 1–2%, is adsorbed onto organic matter and clay. Some, 0.1–0.2%, is available in the soil solution and is directly taken up by the plants (Srivastava et al., 2021).
Seed treatment with T. harzianum isolates increased the uptake of K by 62% in sunflower grown in greenhouse conditions (Nagaraju et al., 2012). Bacterial isolates (Pseudomonas orientalis, Rahnella aquatilis, and Pantoea agglomerans) isolated from Iranian soils containing mica and illite minerals proved their K solubilizing ability by augmenting K use efficiency in rice grown under pot conditions (Khanghahi et al., 2018).
Micronutrients are essential for plant growth and development. They participate in many critical enzymatic activities responsible for maintaining cell metabolism. Various studies have shown that micronutrient deficiencies decrease crop yield, mainly in crops under soil with low organic matter (Rashid and Ryan, 2004). Essential micronutrients are nickel, silicon, iron, copper, boron, and molybdenum (Castro et al., 2018; Shukla et al., 2018).
Synthetic communities
The endophytic community originates from bacteria of different sources, mainly soil and seeds. Bacteria from seeds play an important role in early plant stages (Verma et al., 2019). Interestingly, soil is also a source of bacteria during the later plant stages. The recruited bacteria must interact with natural microbiota to perform important functions related to plant development (Lemanceau et al., 2017).
Synthetic definition systems (SynComs) result from the core microbiome responsible for important functions in the microbial community, which acts as a substitute for the natural microbiome (Vorholt et al., 2017). Synthetic microbial communities (SynComs) have been constructed based on several combinations and interactions between plants and their microbiome and are based on various mechanisms that drive microbial communities in different aspects and environments (Vorholt et al., 2017). Information based on microbiome traits, such as microbe–microbe interactions, microbe–plant interactions, redundancy, dominance, and modularity, has been used to assemble these SynComs and optimize their effect on plant growth. Synthetic communities have also been constructed with respect to the important roles played by distinct strains from individual microbial cultures and in higher-complexity interaction networks. This experimental approach enables the inhibition, or specific removal (drop-out), of individual strains, populations, or functions and monitors the whole response of the system. Additionally, this approach allows us to know the individual strains and their prioritization. With the introduction of microorganisms into a preestablished community, their effects can also be evaluated (Vorholt et al., 2017).
Carlström et al. (2019) inoculated Arabidopsis thaliana with 62 native bacterial strains. They verified that some missing strains could interfere, to varying degrees, with specific niches already colonized by the natural microbiome. These results demonstrated that individual phyla, such as Actinobacteria and Proteobacteria, may significantly influence the communities and microbial structure.
Specific and essential plant genes in the community can also be determined. The dynamics of the microbiome can be previously established and then applied using SynCom. This strategy could enable intelligent farming systems (Trivedi et al., 2020). Stochastic events may determine the presence of some microbes; however, substantial evidence has shown that core microorganisms are established according to plant conditions. These microbes are consistently related to the main taxonomic resolution (Müller et al., 2016). Variations due to plant genotype and environment modulate the microbiome composition. However, studies have shown that the establishment of these SynComs has been consistent throughout the years (Schlaeppi et al., 2014; Finkel et al., 2017).
The assemblage of core microbes could exert several benefits related to plant growth and health. However, it is almost impossible to predict whether a microbial consortia can become established in a place where a microbiome is already installed (Liu et al., 2019).
SynCom can reduce the incidence of diseases. It was built based on previously established microbiome characteristics and the analysis of culture collections (Liu et al., 2019). Niu et al. (2017) reported a reduction in diseases caused by Fusarium verticillioides after Syncom application. Interestingly, the severity of the disease was also significantly reduced.
The reductionist synthetic community approach can be used to improve disease suppressing efficacy. Nevertheless, it is necessary to know microbe–microbe interactions and plant–microbe interactions when constructing multiple-strain biological control consortia for assembly (Niu et al., 2020).
Sustainable agriculture
Food security concerns have led to agricultural intensification and widespread use of chemical fertilizers and pesticides to maximize production (Kumar et al., 2015). Therefore, the harmful effects of these chemicals on non-target organisms and the destabilization of the environment through pollution are expected to become higher than they are now (Yu et al., 2010). Endophytes produce several compounds that can be applied for the biocontrol of a wide range of plant diseases and pests (Shafi et al., 2017) and for plant production. The commercialization, formulation, and application of efficient PGP strains have been proposed as a potential solution for improving agricultural yields without the deleterious effects of synthetic fertilizers and pesticides (Glick, 2014).
Studies carried out under controlled conditions have shown promising results (Shafi et al., 2017). However, crops are grown under varying environmental conditions, which produces large differences and inconsistencies in terms of results (Kamilova et al., 2015).
Many researchers around the world are combining different techniques, including hybridization and techniques that are cultivation-independent, to improve the understanding of microbial strains and determine which are cultivable and uncultivable (Reinhold-Hurek and Hurek, 2011; Berg et al., 2014).
Studies showing how plants shape their microbiome, including ecological interactions in agricultural systems, have been crucial. In the context of climate change, soil pollution, and agricultural intensification, soil microorganisms have been intensely affected (Mitter et al., 2019).
The use of biofertilizers and their success as PGPs rely on selecting specific functions or microorganisms and developing new products to enhance the survival of inoculated strains (Mitter et al., 2021).
Bell et al. (2019) reported that due to the complexity of the microbiome, including genetic diversity and the heterogenicity of the soils, the production of different formulations could be an effective strategy for coping with varying environmental conditions. However, it is unrealistic and unfeasible to design specific biofertilizers for each specific field (Mitter et al., 2019).
New technologies should guarantee a higher number of viable cells during storage and application, and the use of carriers and additives that are cost-effective and easy to use is important to support the presence of endophytes in the product (Bashan et al., 2016; Mitter et al., 2019).
The development of new products requires continuous research and validation. The products should be evaluated against different environmental conditions, such as crop, climate, soil type and agricultural practices, to generate ranges of microbial products that could be utilized. This would result in a better understanding of their potentialities and feasible use for sustainable production. Table 1 shows the bacterial species, abilities, experimental conditions, and results promoted by the application in crops.
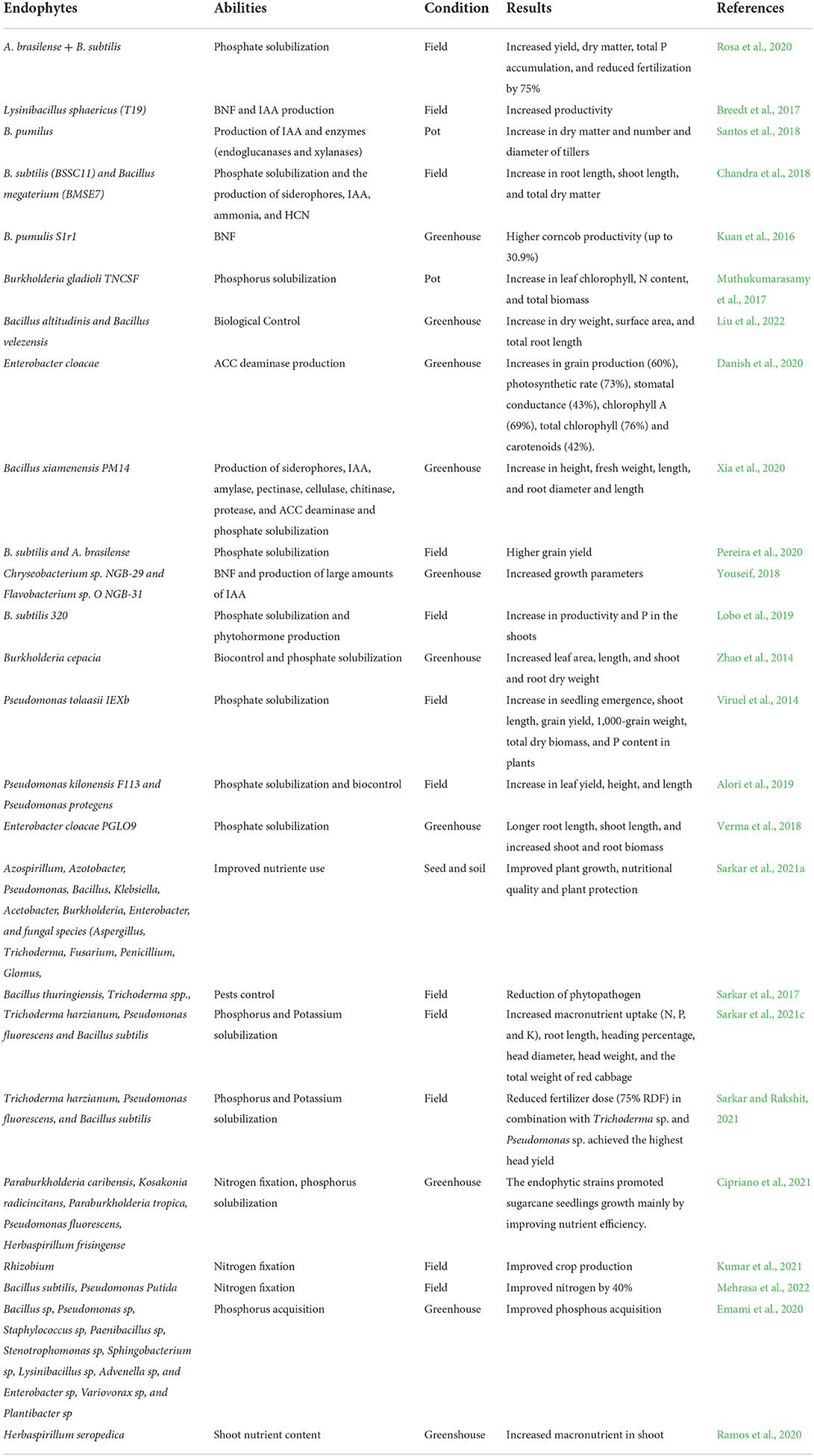
Table 1. Bacterial species, abilities, experimental conditions, and results promoted by the application in crops.
Limitations in the use of endophytes
There are numerous advantages to using endophytes for plant growth promotion, including the low cost of commercialization. However, their use is still not yet widespread because of inconsistent results over varying environmental conditions, plant genotypes and soils. Additionally, agricultural practices and recommendations already exist (Debnath et al., 2019).
To achieve the desired effects related to plant growth and health, microbial products need to overcome many challenges, such as the colonization rate, survival, and shelf life. Typically, endophyte studies have a limited number of variables, making it difficult to understand their performance more fully (Acharya et al., 2017).
Some endophytes show low rhizosphere competence due to competition with the original soil microbiota. Another aspect that can interfere with the success of endophyte colonization and, consequently, their ability to promote plant growth is the complexity of plant–microbe interactions (Fadiji and Babalola, 2020).
Microbial endophytes can show high variability and low reproducibility with regard to their results as plant growth-promoting agents. The reasons for this may include variations in environmental conditions and the edaphic microbiome (Schütz et al., 2018).
Field trials have many uncontrolled aspects that are unable to be evaluated under greenhouse conditions. Additionally, biotic and abiotic factors can interfere with the success of endophytes (Batista and Singh, 2021). The endogenous microbiome may contain antagonistic microbes and competitors that can negatively affect the ability of endophytes to colonize the rhizosphere and endosphere, thus restricting plant growth (Ortas, 2003), and the efficiency of endophytes on agricultural crop production may be reduced (Mitter et al., 2021).
The success of endophyte utilization requires assessing their functionality, production methods and proper formulation. Another potential challenge in using endophytes is that some are opportunistic pathogens for animals, other plants, and humans (Fadiji and Babalola, 2020).
Future and perspectives
Endophytes have attracted attention due to their ability to promote plant growth through different functions and mechanisms, as shown in this review. Microbial endophytes isolated from different plants of agricultural interest play important roles in balancing plant physiology, making nutrients available in the plant, and phytostimulation. Despite recent advances in scientific understanding, many questions remain, including “How can evolutionary relationships and endophyte diversity be determined by metagenomics and bioinformatics tools to predict endophyte functions?” and “How can endophytes be used effectively to reduce production costs and environmental impacts?” Sustainable agriculture requires the reduction of inputs and self-contained functioning, both of which translate to more affordable and eco-friendly solutions. This review highlights the potential of biological-dependent tools, specifically endophytes, to help address global food production challenges. It is clear that there are currently large gaps in our understanding that need to be filled before these tools can be applied to real world situations. Nevertheless, endophytes may be the key to the future of sustainable crop productivity and effective nutrient management.
Conclusion
Microbial endophytes are microorganisms that can colonize different plant tissues. First, this process relies on the specific interaction between the microorganisms from the rhizosphere and the plant host. This interaction may also be influenced by biotic and abiotic factors. Interestingly, these microorganisms have several abilities to promote plant growth, such as nitrogen fixation, phosphorus solubilization, phytohormone and siderophore production, phytopathogen control, and systemic resistance against diseases. These microorganisms are called plant growth-promoting endophytes and may be used in crops to reduce chemical fertilizers, insecticides, and fungicides. Thus, these microorganisms can be used to mitigate environmental impacts and production costs without yield reduction.
Author contributions
All authors listed have made a substantial, direct, and intellectual contribution to the work and approved it for publication.
Conflict of interest
The authors declare that the research was conducted in the absence of any commercial or financial relationships that could be construed as a potential conflict of interest.
Publisher's note
All claims expressed in this article are solely those of the authors and do not necessarily represent those of their affiliated organizations, or those of the publisher, the editors and the reviewers. Any product that may be evaluated in this article, or claim that may be made by its manufacturer, is not guaranteed or endorsed by the publisher.
References
Acharya, J., Bakker, M., Moorman, T., Kaspar, T., Lenssen, A., and Robertson, A. (2017). Time interval between cover crop termination and planting influences corn seedling disease, plant growth, and yield. Plant Dis. 101, 591–600. doi: 10.1094/PDIS-07-16-0975-RE
Adeleke, B. S., Ayangbenro, A. S., and Babalola, O. O. (2021). Genomic analysis of endophytic Bacillus cereus T4S and its plant growth-promoting traits. Plants 10, 1776. doi: 10.3390/plants10091776
Aloo, B., Makumba, B., and Mbega, E. (2019). The potential of bacilli rhizobacteria for sustainable crop production and environmental sustainability. Microbiol. Res. 219, 26–39. doi: 10.1016/j.micres.2018.10.011
Alori, E. T., Babalola, O. O., and Prigent-Combaret, C. (2019). Impacts of microbial inoculants on the growth and yield of maize plant. Open Agri. J. 13, 1–8. doi: 10.2174/1874331501913010001
Alori, E. T., Glick, B. R., and Babalola, O. O. (2017). Microbial phosphorus solubilization and its potential for use in sustainable agriculture. Front. Microbiol. 8, 971. doi: 10.3389/fmicb.2017.00971
Babalola, O. O. (2010). Beneficial bacteria of agricultural importance. Biotechnol. Lett. 32, 1559–1570. doi: 10.1007/s10529-010-0347-0
Baldan, E., Nigris, S., Populin, F., Zottini, M., Squartini, A., and Baldan, B. (2014). Identification of culturable bacterial endophyte community isolated from tissues of Vitis vinifera “Glera”. Plant Biosyst. 148, 508–516. doi: 10.1080/11263504.2014.916364
Baligar, V., Fageria, N., and He, Z. (2001). Nutrient use efficiency in plants. Commun. Soil Sci. Plant Anal. 32, 921–50. doi: 10.1081/CSS-100104098
Bashan, Y., Kloepper, J. W., De-Bashan, L. E., and Nannipieri, P. (2016). A need for disclosure of the identity of microorganisms, constituents, and application methods when reporting tests with microbe-based or pesticide-based products. Biol. Fertil. Soils 52, 283. doi: 10.1007/s00374-016-1091-y
Batista, B. D., and Singh, B. K. (2021). Realities and hopes in the application of microbial tools in agriculture. Microb. Biotechnol. 14, 1258–1268. doi: 10.1111/1751-7915.13866
Bell, T. H., Kaminsky, L. M., Gugino, B. K., Carlson, J. E., Malik, R. J., Hockett, K. L., et al. (2019). Factoring ecological, societal, and economic considerations into inoculant development. Trends Biotechnol. 37, 572–573. doi: 10.1016/j.tibtech.2019.02.009
Berg, G., Grube, M., Schloter, M., and Smalla, K. (2014). Unraveling the plant microbiome: looking back and future perspectives. Front. Microbiol. 5, 148. doi: 10.3389/fmicb.2014.00148
Berg, G., and Raaijmakers, J. M. (2018). Saving seed microbiomes. ISME J. 12, 1167–1170. doi: 10.1038/s41396-017-0028-2
Brader, G., Compant, S., Vescio, K., Mitter, B., Trognitz, F., Ma, L.-J., et al. (2017). Ecology and genomic insights into plant-pathogenic and plant-nonpathogenic endophytes. Annu. Rev. Phytopathol. 55, 61–83. doi: 10.1146/annurev-phyto-080516-035641
Breedt, G., Labuschagne, N., and Coutinho, T. A. (2017). Seed treatment with selected plant growth-promoting rhizobacteria increases maize yield in the field. Ann. Appl. Biol. 171, 229–236. doi: 10.1111/aab.12366
Brevik, E.C. (2012). Soils and climate change: gas fluxes and soil processes. Soil Horizons 53, 12–23. doi: 10.2136/sh12-04-0012
Bueno, C. B., Santos, R. M. D., De Souza Buzo, F., Silva, M. S. R. D. a. D., and Rigobelo, E. C. (2022). Chemical fertilization and microbial inoculum on Bacillus subtilis endophytism in soybean and maize plants. Front. Microbiol. 13, 901157. doi: 10.3389/fmicb.2022.901157
Bulgarelli, D., Schlaeppi, K., Spaepen, S., Van Themaat, E. V. L., and Schulze-Lefert, P. (2013). Structure and functions of the bacterial microbiota of plants. Annu. Rev. Plant Biol. 64, 807–838. doi: 10.1146/annurev-arplant-050312-120106
Carlström, C. I., Field, C. M., Bortfeld-Miller, M., Müller, B., Sunagawa, S., and Vorholt, J. A. (2019). Synthetic microbiota reveal priority effects and keystone strains in the Arabidopsis phyllosphere. Nat. Ecol. Evol. 3, 1445–1454. doi: 10.1038/s41559-019-0994-z
Castro, P. H., Lilay, G. H., and Assunção, A. G. (2018). “Regulation of micronutrient homeostasis and deficiency response in plants,” in Plant Micronutrient Use Efficiency (Elsevier), 1–15.
Chandra, P., Tripathi, P., and Chandra, A. (2018). Isolation and molecular characterization of plant growth-promoting Bacillus spp. and their impact on sugarcane (Saccharum spp. hybrids) growth and tolerance towards drought stress. Acta Physiol. Plant. 40, 199. doi: 10.1007/s11738-018-2770-0
Chase, J. M. (2003). Community assembly: when should history matter? Oecologia 136, 489–498. doi: 10.1007/s00442-003-1311-7
Cipriano, M. A. P., Freitas-Iório, R. D. P., Dimitrov, M. R., De Andrade, S. A. L., Kuramae, E. E., and Silveira, A. P. D. D. (2021). Plant-growth endophytic bacteria improve nutrient use efficiency and modulate foliar N-metabolites in sugarcane seedling. Microorganisms 9, 479. doi: 10.3390/microorganisms9030479
Compant, S., Clément, C., and Sessitsch, A. (2010). Plant growth-promoting bacteria in the rhizo-and endosphere of plants: their role, colonization, mechanisms involved and prospects for utilization. Soil Biol. Biochem. 42, 669–678. doi: 10.1016/j.soilbio.2009.11.024
Compant, S., Saikkonen, K., Mitter, B., Campisano, A., and Mercado-Blanco, J. (2016). Editorial Special Issue: Soil, Plants and Endophytes. Singapore: Springer. doi: 10.1007/s11104-016-2927-9
Danish, S., Zafar-Ul-Hye, M., Mohsin, F., and Hussain, M. (2020). ACC-deaminase producing plant growth promoting rhizobacteria and biochar mitigate adverse effects of drought stress on maize growth. PLoS ONE 15, e0230615. doi: 10.1371/journal.pone.0230615
Debnath, M., Ashwath, N., and Midmore, D. (2019). Physiological and morphological responses to abiotic stresses in two cultivars of Stevia rebaudiana (Bert.) Bertoni. South Afr. J. Botany 123, 124–132. doi: 10.1016/j.sajb.2019.01.025
Devika, O. S., Singh, S., Sarkar, D., Barnwal, P., Suman, J., and Rakshit, A. (2021). Seed priming: a potential supplement in integrated resource management under fragile intensive ecosystems. Front. Sustain. Food Syst. 5, 209. doi: 10.3389/fsufs.2021.654001
dos Santos, R. M., Cueva-Yesquén, L. G., Garboggini, F. F., Desoignies, N., and Rigobelo, E. C. (2022). Inoculum concentration and mineral fertilization: effects on the endophytic microbiome of soybean. Front. Microbiol. 13, 900980. doi: 10.3389/fmicb.2022.900980
Duarah, I., Deka, M., Saikia, N., and Deka Boruah, H. (2011). Phosphate solubilizers enhance NPK fertilizer use efficiency in rice and legume cultivation. 3 Biotech 1, 227–238. doi: 10.1007/s13205-011-0028-2
Emami, S., Alikhani, H. A., Pourbabaee, A. A., Etesami, H., Motasharezadeh, B., and Sarmadian, F. (2020). Consortium of endophyte and rhizosphere phosphate solubilizing bacteria improves phosphorous use efficiency in wheat cultivars in phosphorus deficient soils. Rhizosphere 14, 100196. doi: 10.1016/j.rhisph.2020.100196
Escobar Diaz, P. A., Dos Santos, R. M., Baron, N. C., Gil, O. J. A., and Rigobelo, E. C. (2021). Effect of aspergillus and bacillus concentration on cotton growth promotion. Front. Microbiol. 12, 737385. doi: 10.3389/fmicb.2021.737385
Fadiji, A. E., and Babalola, O. O. (2020). Exploring the potentialities of beneficial endophytes for improved plant growth. Saudi J. Biol. Sci. 27, 3622–3633. doi: 10.1016/j.sjbs.2020.08.002
Felici, C., Vettori, L., Giraldi, E., Forino, L. M. C., Toffanin, A., Tagliasacchi, A. M., et al. (2008). Single and co-inoculation of bacillus subtilis and azospirillum brasilense on lycopersicon esculentum: effects on plant growth and rhizosphere microbial community. Appl. Soil Ecol. 40, 260–270. doi: 10.1016/j.apsoil.2008.05.002
Finkel, O. M., Castrillo, G., Paredes, S. H., González, I. S., and Dangl, J. L. (2017). Understanding and exploiting plant beneficial microbes. Curr. Opin. Plant Biol. 38, 155–163. doi: 10.1016/j.pbi.2017.04.018
Firdous, J., Lathif, N. A., Mona, R., and Muhamad, N. (2019). Endophytic bacteria and their potential application in agriculture: a review. Indian J. Agric. Res. 53, 1–7. doi: 10.18805/IJARe.A-366
Ghazi, A., Atia, E., and Elsakhawy, T. (2021). Evaluation of an endophytic plant growth-promoting bacterium, Klebsiella variicola, in mitigation of salt stress in tuberose (Polianthes tuberosa L.). J. Hortic. Sci. Biotechnol. 96, 770–82. doi: 10.1080/14620316.2021.1926343
Glick, B. R. (2014). Bacteria with ACC deaminase can promote plant growth and help to feed the world. Microbiol. Res. 169, 30–39. doi: 10.1016/j.micres.2013.09.009
Hardoim, P. R., Van Overbeek, L. S., Berg, G., Pirttilä, A. M., Compant, S., Campisano, A., et al. (2015). The hidden world within plants: ecological and evolutionary considerations for defining functioning of microbial endophytes. Microbiol. Mol. Biol. Rev. 79, 293–320. doi: 10.1128/MMBR.00050-14
Hartmann, A., Rothballer, M., Hense, B. A., and Schröder, P. (2014). Bacterial quorum sensing compounds are important modulators of microbe-plant interactions. Front. Plant Sci. 5, 131. doi: 10.3389/fpls.2014.00131
Hounsome, N., Hounsome, B., Tomos, D., and Edwards-Jones, G. (2008). Plant metabolites and nutritional quality of vegetables. J. Food Sci. 73, R48–R65. doi: 10.1111/j.1750-3841.2008.00716.x
Howarth, J. R., Parmar, S., Barraclough, P. B., and Hawkesford, M. J. (2009). A sulphur deficiency-induced gene, sdi1, involved in the utilization of stored sulphate pools under sulphur-limiting conditions has potential as a diagnostic indicator of sulphur nutritional status. Plant Biotechnol. J. 7, 200–209. doi: 10.1111/j.1467-7652.2008.00391.x
Jayne, T. S., Snapp, S., Place, F., and Sitko, N. (2019). Sustainable agricultural intensification in an era of rural transformation in Africa. Global Food Secur. 20, 105–113. doi: 10.1016/j.gfs.2019.01.008
Kamilova, F., Okon, Y., De Weert, S., and Hora, K. (2015). “Commercialization of microbes: manufacturing, inoculation, best practice for objective field testing, and registration,” in Principles of Plant-Microbe Interactions. Singapore: Springer, 319–327. doi: 10.1007/978-3-319-08575-3_33
Kaur, N., Sharma, P., and Sharma, S. (2015). Co-inoculation of Mesorhizobium sp. and plant growth promoting rhizobacteria Pseudomonas sp. as bio-enhancer and bio-fertilizer in chickpea (Cicer arietinum L.). Legume Res. 38, 367–374. Available online at: https://arccarticles.s3.amazonaws.com/webArticle/articles/15LR2975.pdf
Kennedy, P. (2010). Ectomycorrhizal fungi and interspecific competition: species interactions, community structure, coexistence mechanisms, and future research directions. New Phytol. 187, 895–910. doi: 10.1111/j.1469-8137.2010.03399.x
Khanghahi, Y. M., Pirdashti, H., Rahimian, H., Nematzadeh, G., and Ghajar Sepanlou, M. (2018). Potassium solubilising bacteria (KSB) isolated from rice paddy soil: from isolation, identification to K use efficiency. Symbiosis 76, 13–23. doi: 10.1007/s13199-017-0533-0
Korir, H., Mungai, N. W., Thuita, M., Hamba, Y., and Masso, C. (2017). Co-inoculation effect of rhizobia and plant growth promoting rhizobacteria on common bean growth in a low phosphorus soil. Front. Plant Sci. 8, 141. doi: 10.3389/fpls.2017.00141
Kuan, K. B., Othman, R., Rahim, K. A., and Shamsuddin, Z. H. (2016). Plant growth-promoting rhizobacteria inoculation to enhance vegetative growth, nitrogen fixation and nitrogen remobilisation of maize under greenhouse conditions. PLoS ONE 11, e0152478. doi: 10.1371/journal.pone.0152478
Kumar, R., Saurabh, K., Kumawat, N., Sundaram, P. K., Mishra, J. S., Singh, D. K., et al. (2021). “Sustaining productivity through integrated use of microbes in agriculture,” in Role of Microbial Communities for Sustainability. Singapore: Springer, 109–145. doi: 10.1007/978-981-15-9912-5_5
Kumar, V., Singh, S., Singh, J., and Upadhyay, N. (2015). Potential of plant growth promoting traits by bacteria isolated from heavy metal contaminated soils. Bull. Environ. Contam. Toxicol. 94, 807–814. doi: 10.1007/s00128-015-1523-7
Lemanceau, P., Blouin, M., Muller, D., and Moënne-Loccoz, Y. (2017). Let the core microbiota be functional. Trends Plant Sci. 22, 583–595. doi: 10.1016/j.tplants.2017.04.008
Li, S., Tian, Y., Wu, K., Ye, Y., Yu, J., Zhang, J., et al. (2018). Modulating plant growth–metabolism coordination for sustainable agriculture. Nature 560, 595–600. doi: 10.1038/s41586-018-0415-5
Liu, H., Carvalhais, L. C., Crawford, M., Singh, E., Dennis, P. G., Pieterse, C. M., et al. (2017). Inner plant values: diversity, colonization and benefits from endophytic bacteria. Front. Microbiol. 8, 2552. doi: 10.3389/fmicb.2017.02552
Liu, X., Li, Q., Li, Y., Guan, G., and Chen, S. (2019). Paenibacillus strains with nitrogen fixation and multiple beneficial properties for promoting plant growth. PeerJ 7, e7445. doi: 10.7717/peerj.7445
Liu, Z., Zhang, X., Li, L., Xu, N., Hu, Y., Wang, C., et al. (2022). Isolation and characterization of three plant growth-promoting rhizobacteria for growth enhancement of rice seedling. J. Plant Growth Regulat. 41, 1382–1393. doi: 10.1007/s00344-021-10393-4
Lobo, L. L. B., Da Silva, M. S. R. D. A., Carvalho, R. F., and Rigobelo, E. C. (2022). The negative effect of coinoculation of plant growth-promoting bacteria is not related to indole-3-acetic acid synthesis. J. Plant Growth Regul. 40, 1–10. doi: 10.1007/s00344-022-10706-1
Lobo, L. L. B., Dos Santos, R. M., and Rigobelo, E. C. (2019). Promotion of maize growth using endophytic bacteria under greenhouse and field conditions. Aust. J. Crop. Sci. 13, 2067. doi: 10.21475/ajcs.19.13.12.p2077
Lugtenberg, B. J., Dekkers, L., and Bloemberg, G. V. (2001). Molecular determinants of rhizosphere colonization by Pseudomonas. Annu. Rev. Phytopathol. 39, 461–490. doi: 10.1146/annurev.phyto.39.1.461
Mehrasa, H., Farnia, A., Kenarsari, M. J., and Nakhjavan, S. (2022). Endophytic bacteria and SA application improve growth, biochemical properties, and nutrient uptake in white beans under drought stress. J. Soil Sci. Plant Nutr. 22, 1–12. doi: 10.1007/s42729-022-00884-y
Mitter, E. K., Kataoka, R., De Freitas, J. R., and Germida, J. J. (2019). Potential use of endophytic root bacteria and host plants to degrade hydrocarbons. Int. J. Phytoremediat. 21, 928–938. doi: 10.1080/15226514.2019.1583637
Mitter, E. K., Tosi, M., Obregón, D., Dunfield, K. E., and Germida, J. J. (2021). Rethinking crop nutrition in times of modern microbiology: innovative biofertilizer technologies. Front. Sustain. Food Syst. 5, 29. doi: 10.3389/fsufs.2021.606815
Müller, D. B., Vogel, C., Bai, Y., and Vorholt, J. A. (2016). The plant microbiota: systems-level insights and perspectives. Annu. Rev. Genet. 50, 211–234. doi: 10.1146/annurev-genet-120215-034952
Muthukumarasamy, R., Revathi, G., Vadivelu, M., and Aruri, K. (2017). Isolation of bacterial strains possessing nitrogen-fixation, phosphate and potassium-solubilization and their inoculation effects on sugarcane. Indian J. Exp. Biol. 55, 161–170.
Nagaraju, A., Sudisha, J., Murthy, S. M., and Ito, S.-I. (2012). Seed priming with Trichoderma harzianum isolates enhances plant growth and induces resistance against Plasmopara halstedii, an incitant of sunflower downy mildew disease. Aust. Plant Pathol. 41, 609–620. doi: 10.1007/s13313-012-0165-z
Niu, B., Paulson, J. N., Zheng, X., and Kolter, R. (2017). Simplified and representative bacterial community of maize roots. Proc. Nat. Acad. Sci. 114, E2450–E2459. doi: 10.1073/pnas.1616148114
Niu, B., Wang, W., Yuan, Z., Sederoff, R. R., Sederoff, H., Chiang, V. L., et al. (2020). Microbial interactions within multiple-strain biological control agents impact soil-borne plant disease. Front. Microbiol. 11, 585404. doi: 10.3389/fmicb.2020.585404
Ortas, I. (2003). Effect of selected mycorrhizal inoculation on phosphorus sustainability in sterile and non-sterile soils in the harran plain in South Anatolia. J. Plant Nutr. 26, 1–17. doi: 10.1081/PLN-120016494
Patel, T. S., and Minocheherhomji, F. P. (2018). Plant growth promoting Rhizobacteria: blessing to agriculture. Int. J. Pure App. Biosci. 6, 481–492. doi: 10.18782/2320-7051.6383
Paul, A., and Kumar, S. (2015). An A20/AN1-zinc-finger domain containing protein gene in tea is differentially expressed during winter dormancy and in response to abiotic stress and plant growth regulators. Plant Gene. 1, 1–7. doi: 10.1016/j.plgene.2014.12.003
Pereira, N. C. M., Galindo, F. S., Gazola, R. P. D., Dupas, E., Rosa, P. A. L., Mortinho, E. S., et al. (2020). Corn yield and phosphorus use efficiency response to phosphorus rates associated with plant growth promoting bacteria. Front. Environ. Sci. 8, 12. doi: 10.3389/fenvs.2020.00040
Rakshit, A. (2019). “Impact assessment of bio priming mediated nutrient use efficiency for climate resilient agriculture,” in Climate Change and Agriculture in India: Impact and Adaptation. Singapore: Springer, 57–68. doi: 10.1007/978-3-319-90086-5_6
Ramos, A. C., Melo, J., De Souza, S. B., Bertolazi, A. A., Silva, R. A., Rodrigues, W. P., et al. (2020). Inoculation with the endophytic bacterium Herbaspirillum seropedicae promotes growth, nutrient uptake and photosynthetic efficiency in rice. Planta 252, 1–8. doi: 10.1007/s00425-020-03496-x
Rashid, A., and Ryan, J. (2004). Micronutrient constraints to crop production in soils with Mediterranean-type characteristics: a review. J. Plant Nutr. 27, 959–975. doi: 10.1081/PLN-120037530
Reinhold-Hurek, B., and Hurek, T. (2011). Living inside plants: bacterial endophytes. Curr. Opin. Plant Biol. 14, 435–443. doi: 10.1016/j.pbi.2011.04.004
Rodríguez, C. E., Mitter, B., Barret, M., Sessitsch, A., and Compant, S. (2018). Commentary: seed bacterial inhabitants and their routes of colonization. Plant Soil. 422, 129–134. doi: 10.1007/s11104-017-3368-9
Rosa, P. A. L., Mortinho, E. S., Jalal, A., Galindo, F. S., Buzetti, S., Fernandes, G. C., et al. (2020). Inoculation with growth-promoting bacteria associated with the reduction of phosphate fertilization in sugarcane. Front. Environ. Sci. 8, 32.
Samreen, T., Naveed, M., Nazir, M. Z., Asghar, H. N., Khan, M. I., Zahir, Z. A., et al. (2021). Seed associated bacterial and fungal endophytes: diversity, life cycle, transmission, and application potential. Appl. Soil Ecol. 168, 104191. doi: 10.1016/j.apsoil.2021.104191
Santos, R. M., Kandasamy, S., and Rigobelo, E. C. (2018). Sugarcane growth and nutrition levels are differentially affected by the application of PGPR and cane waste. Microbiol. Open. 7, e00617. doi: 10.1002/mbo3.617
Sanyal, S., Majumdar, K., and Singh, V. (2014). Nutrient management in Indian agriculture with special reference to nutrient mining—a relook. J. Indian Soc. Soil Sci. 62, 307–325.
Sarkar, D., Dubey, P. K., Chaurasiya, R., Sankar, A., Chatterjee, N., Ganguly, S., et al. (2021a). Organic interventions conferring stress tolerance and crop quality in agroecosystems during the United Nations decade on ecosystem restoration. Land Degrad. Develop. 32, 4797–4816. doi: 10.1002/ldr.4094
Sarkar, D., Rakesh, S., Ganguly, S., and Rakshit, A. (2017). Management of increasing soil pollution in the ecosystem. Adv. Res. 12, 1–9. doi: 10.9734/AIR/2017/36622
Sarkar, D., and Rakshit, A. (2021). Bio-priming in combination with mineral fertilizer improves nutritional quality and yield of red cabbage under Middle Gangetic Plains, India. Sci. Hortic. 283, 110075. doi: 10.1016/j.scienta.2021.110075
Sarkar, D., Rakshit, A., Al-Turki, A. I., Sayyed, R., and Datta, R. (2021b). Connecting bio-priming approach with integrated nutrient management for improved nutrient use efficiency in crop species. Agriculture 11, 372. doi: 10.3390/agriculture11040372
Sarkar, D., Sankar, A., Devika, O. S., Singh, S., Parihar, M., Rakshit, A., et al. (2021c). Optimizing nutrient use efficiency, productivity, energetics, and economics of red cabbage following mineral fertilization and biopriming with compatible rhizosphere microbes. Sci. Rep. 11, 1–14. doi: 10.1038/s41598-021-95092-6
Sasse, J., Martinoia, E., and Northen, T. (2018). Feed your friends: do plant exudates shape the root microbiome? Trends Plant Sci. 23, 25–41. doi: 10.1016/j.tplants.2017.09.003
Schlaeppi, K., Dombrowski, N., Oter, R. G., Van Themaat, E. V. L., and Schulze-Lefert, P. (2014). Quantitative divergence of the bacterial root microbiota in Arabidopsis thaliana relatives. Proc. Nat. Acad. Sci. 111, 585–592. doi: 10.1073/pnas.1321597111
Schütz, L., Gattinger, A., Meier, M., Müller, A., Boller, T., Mäder, P., et al. (2018). Improving crop yield and nutrient use efficiency via biofertilization—a global meta-analysis. Front. Plant Sci. 8, 2204. doi: 10.3389/fpls.2017.02204
Sessitsch, A., Pfaffenbichler, N., and Mitter, B. (2019). Microbiome applications from lab to field: facing complexity. Trends Plant Sci. 24, 194–198. doi: 10.1016/j.tplants.2018.12.004
Shade, A., Jacques, M.-A., and Barret, M. (2017). Ecological patterns of seed microbiome diversity, transmission, and assembly. Curr. Opin. Microbiol. 37, 15–22. doi: 10.1016/j.mib.2017.03.010
Shafi, J., Tian, H., and Ji, M. (2017). Bacillus species as versatile weapons for plant pathogens: a review. Biotechnol. Biotechnol. Equip. 31, 446–459. doi: 10.1080/13102818.2017.1286950
Shamseldin, A., Abdelkhalek, A., and Sadowsky, M. J. (2017). Recent changes to the classification of symbiotic, nitrogen-fixing, legume-associating bacteria: a review. Symbiosis 71, 91–109. doi: 10.1007/s13199-016-0462-3
Shukla, A. K., Behera, S. K., Pakhre, A., and Chaudhari, S. (2018). Micronutrients in soils, plants, animals and humans. Indian J. Fertil. 14, 30–54.
Srivastava, M., Sadanandom, A., and Srivastava, A. K. (2021). Towards understanding the multifaceted role of SUMOylation in plant growth and development. Physiol. Plant. 171, 77–85. doi: 10.1111/ppl.13204
Taulé, C., Vaz-Jauri, P., and Battistoni, F. (2021). Insights into the early stages of plant–endophytic bacteria interaction. World J. Microbiol. Biotechnol. 37, 1–9. doi: 10.1007/s11274-020-02966-4
Trivedi, P., Leach, J. E., Tringe, S. G., Sa, T., and Singh, B. K. (2020). Plant–microbiome interactions: from community assembly to plant health. Nat. Rev. Microbiol. 18, 607–621. doi: 10.1038/s41579-020-0412-1
Truyens, S., Weyens, N., Cuypers, A., and Vangronsveld, J. (2013). Changes in the population of seed bacteria of transgenerationally Cd-exposed a rabidopsis thaliana. Plant Biol. 15, 971–981. doi: 10.1111/j.1438-8677.2012.00711.x
Tsai, A. Y. L., Oota, M., and Sawa, S. (2020). Chemotactic host-finding strategies of plant endoparasites and endophytes. Front. Plant Sci. 11, 1167. doi: 10.3389/fpls.2020.01167
UNCCD Brochure (2012). Desertification Land Degredation and Drought (DLDD) - Some Global Facts and Figures. Available online at: http://www.un.org/
Vacher, C., Hampe, A., Porté, A. J., Sauer, U., Compant, S., and Morris, C. E. (2016). The phyllosphere: microbial jungle at the plant–climate interface. Annu. Rev. Ecol. Evol. Syst. 47, 1–24. doi: 10.1146/annurev-ecolsys-121415-032238
Van Bael, S., Estrada, C., and Arnold, A. E. (2017). Chapter 6: foliar endophyte communities and leaf traits in tropical trees. Fungal Commun. Organiz. Role Ecosyst. 4, 79–94. doi: 10.1201/9781315119496-7
Vandana, U., Rajkumari, J., Singha, L., Satish, L., Alavilli, H., Sudheer, P., et al. (2021). The endophytic microbiome as a hotspot of synergistic interactions, with prospects of plant growth promotion. Biology 10, 101. doi: 10.3390/biology10020101
Verma, M., Mishra, J., and Arora, N. K. (2019). “Plant growth-promoting rhizobacteria: diversity and applications,” in Environmental Biotechnology: for Sustainable Future. Singapore: Springer, 129–173. doi: 10.1007/978-981-10-7284-0_6
Verma, P., Agrawal, N., and Shahi, S.K. (2018). Enterobacter cloacae strain PGLO9: Potential source of maize growth promoting rhizobacteria. Int. J. Bot. Stud. 3, 172–175.
Vincent, G., Shang, K., Zhang, G., Chazarenc, F., and Brisson, J. (2018). Plant growth and nutrient uptake in treatment wetlands for water with low pollutant concentration. Water Sci. Technol. 77, 1072–1078. doi: 10.2166/wst.2017.624
Viruel, E., Erazzu, L. E., Calsina, L. M., Ferrero, M. A., Lucca, M. E., and Sineriz, F. (2014). Inoculation of maize with phosphate solubilizing bacteria: effect on plant growth and yield. J. Soil Sci. Plant Nutr. 14, 819–831. doi: 10.4067/S0718-95162014005000065
Vorholt, J. A., Vogel, C., Carlström, C. I., and Müller, D. B. (2017). Establishing causality: opportunities of synthetic communities for plant microbiome research. Cell Host Microbe 22, 142–155. doi: 10.1016/j.chom.2017.07.004
Wearn, J. A., Sutton, B. C., Morley, N. J., and Gange, A. C. (2012). Species and organ specificity of fungal endophytes in herbaceous grassland plants. J. Ecol. 100, 1085–1092. doi: 10.1111/j.1365-2745.2012.01997.x
Wei, W., Wang, L., Bao, P., Shao, Y., Yue, H., Yang, D., et al. (2018). Metal-Free C (sp2)–H/N–H cross-dehydrogenative coupling of quinoxalinones with aliphatic amines under visible-light photoredox catalysis. Org. Lett. 20, 7125–7130. doi: 10.1021/acs.orglett.8b03079
Werner, D., and Newton, W. E. (2005). Nitrogen Fixation in agriculture, Forestry, Ecology, and the Environment. Singapore: Springer Science and Business Media. doi: 10.1007/1-4020-3544-6
Wichern, F., Eberhardt, E., Mayer, J., Joergensen, R. G., and Müller, T. (2008). Nitrogen rhizodeposition in agricultural crops: methods, estimates and future prospects. Soil Biol. Biochem. 40, 30–48. doi: 10.1016/j.soilbio.2007.08.010
Xia, Y., Farooq, M. A., Javed, M. T., Kamran, M. A., Mukhtar, T., Ali, J., et al. (2020). Multi-stress tolerant PGPR Bacillus xiamenensis PM14 activating sugarcane (Saccharum officinarum L.) red rot disease resistance. Plant Physiol Biochem. 151, 640–649. doi: 10.1016/j.plaphy.2020.04.016
Youseif, S. H. (2018). Genetic diversity of plant growth promoting rhizobacteria and their effects on the growth of maize plants under greenhouse conditions. Ann. Agri. Sci. 63, 25–35. doi: 10.1016/j.aoas.2018.04.002
Yu, Q., Han, H., Vila-Aiub, M. M., and Powles, S. B. (2010). AHAS herbicide resistance endowing mutations: effect on AHAS functionality and plant growth. J. Exp. Bot. 61, 3925–3934. doi: 10.1093/jxb/erq205
Keywords: plant growth-promoting, endophytes, yield, SynComs, plant growth
Citation: Santos RMd, Desoignies N and Rigobelo EC (2022) The bacterial world inside the plant. Front. Sustain. Food Syst. 6:830198. doi: 10.3389/fsufs.2022.830198
Received: 06 December 2021; Accepted: 11 July 2022;
Published: 28 July 2022.
Edited by:
Autar Krishen Mattoo, Agricultural Research Service (USDA), United StatesReviewed by:
Deepranjan Sarkar, Banaras Hindu University, IndiaJoyce Dória, Universidade Federal de Lavras, Brazil
Copyright © 2022 Santos, Desoignies and Rigobelo. This is an open-access article distributed under the terms of the Creative Commons Attribution License (CC BY). The use, distribution or reproduction in other forums is permitted, provided the original author(s) and the copyright owner(s) are credited and that the original publication in this journal is cited, in accordance with accepted academic practice. No use, distribution or reproduction is permitted which does not comply with these terms.
*Correspondence: Everlon Cid Rigobelo, everlon.cid@unesp.br