Deciphering the role of phosphorus management under conservation agriculture based wheat production system
- 1ICAR Research Complex for NEH Region, Sikkim Center, Gangtok, Sikkim, India
- 2ICAR-Indian Agricultural Research Institute, New Delhi, India
- 3ICAR-Indian Institute of Farming Systems Research, Modipuram, Uttar Pradesh, India
- 4ICAR-National Bureau of Agriculturally Important Microorganisms, Mau, Uttar Pradesh, India
- 5ICAR-Indian Grassland and Fodder Research Institute, Jhansi, Uttar Pradesh, India
- 6Department of Animal Husbandry, Lucknow, India
- 7Spices Park Chhindwara, Spices Board of India, Chhindwara, Madhya Pradesh, India
- 8ICAR Research Complex for NEH Region, Umiam, Meghalaya, India
- 9Chaudhary Charan Singh Haryana Agricultural, University, Hisar, Haryana, India
Phosphorus (P) is a vital element required by all living organism (plants, animals and microbes etc.). Its application in agriculture, whether in conventional or conservation agriculture, requires careful attention due to its low use efficiency, which typically does not exceed 20%. With the increasing acceptance of conservation agriculture (CA), it is crucial to develop protocols for P management to ensure sustainable wheat production. Therefore, a field trial was conducted from 2016–2017 to 2017–2018 in the India's semiarid eco-region to study the role of P on wheat productivity, quality, and resource use efficiency under CA-based production system. We assessed the impact of tillage operations and P management practices on wheat productivity, quality, and resource use efficiency. Three tillage and residue management options such as CT-R (conventional tillage without residue); NT-R (no tillage without maize residue) and NT + R (no tillage with maize residue @ 2.5 Mg ha−1) were laid-out in main plot and five P management options subplots viz. P1 (nitrogen and potash according to recommended but not P); P2 (17.2 kg P ha−1); P3 (17.2 kg of P ha−1 + microbial fertilizer); P4 (17.2 kg P ha−1 + compost inoculant culture) and P5 (34.4 kg P ha−1) in split plot design with three replicates. The results indicates that the combination of no-tillage with residue retention (maize residue @ 2.5 Mg ha−1) (NT + R) and the application of 34.4 kg P ha−1 (P5) significantly improved grain yield by ~43.2% compared to the control treatment (conventional tillage with no residue, CT – R, and no phosphorus application). NT + R also resulted in significantly better amino acid (~22.7%) and net protein yield (~21.2%) compared to CT – R. Regarding the P management strategy, the highest amino acid (49.1%) and protein yield (12.5%) were observed under the P5 treatment compared to the no-phosphorus treatment. Conjoint use of NT – R, along with the application of 17.2 kg P ha−1 and PSB (Phosphorus Solubilizing Bacteria), resulted in a significant increase in energy use efficiency of ~58% over other treatments combination. Furthermore, the NT + R plot that received 17.2 kg P ha−1 + PSB demonstrated higher P agronomic efficiency (~43%) and recovery efficiency (~53%) over control. The study's findings underscore the significance of adopting efficient P management strategies in CA to ensure the sustainable production of wheat.
1. Introduction
Among cereals, wheat (Triticum aestivum L.) is a prime food crop for ~36% of the global population, contributing to nearly 55% of the carbohydrates and 20% of the food calories. Over an extensive area of 31.9 million hectares in India, wheat cultivation yields an impressive 112.7 million tons (AGRICOOP, 2022). The protein content significantly influences the quality of wheat brad, with a low concentration of essential amino acids and a high concentration of nonessential amino acids (Jat et al., 2018; Garutti et al., 2022). Therefore, increasing production and improving wheat protein content have become key concerns. Farmers in the Indo-Gangetic plains (IGPs) of India practice an intensive rice-wheat system (RWS) and often rely on a large amount of urea for nitrogen supplementation, while using minimal amounts of P and potassium (K) and almost no secondary and micronutrients. This ultimately results in impaired soil health, low input use efficiency, a dramatic reduction in crop quality, and diminished crop yields (Kumar et al., 2018). To address these issues and find solutions, scientists have suggested transitioning to a sustainable crop production system. Therefore, understanding the importance of agronomic management that impact the nutritional quality of wheat is crucial for addressing future global food and nutritional security and achieving sustainable agricultural goals.
After the green revolution, researchers have turned their attention to CA due to its potential to enhance agro-ecosystem productivity, improve resource use efficiency, mitigate global climate change, and address soil degradation issues (Babu et al., 2020). Recently, management of nutrient has been recognized as the 4th principle (apart from the three principles of CA i.e., minimal/zero tillage, bio-intensified crop rotation and crop residue retention), considering CA's unique impact on plant nutritional behavior. Conservation agriculture has shown the potential to increase wheat yield (Jat et al., 2020) and improve the pasta making quality of durum wheat (Kerbouai et al., 2023). However, it does not have a significant effect on determining protein content of wheat (Ruiz et al., 2019). On the other hand, CA has been found to enhance maize grain protein content and modify nutrient composition, potentially increasing the risk of iron (Fe) deficiency while decreasing the incidence of selenium (Se) deficiency in Malawian women and children (Galani et al., 2022). Since nutrient requirements can vary depending on crops, cropping systems, and management techniques, it is crucial to identify the specific nutrient needs for different locations, especially within CA-based systems (Kumar et al., 2023a).
P is an essential nutrient for plants and is nearly as important as the primary nutrient elements (Luo et al., 2017). However, currently, a lack of P in the soil remains one of the primary factors limiting crop productivity (Johnston and Poulton, 2019). Under long-term zero-till management, an increase in soluble P can be accredited to the greater soil organic matter (SOM) content and reduced P fixation in maize-based cropping systems in weathered soils of the semiarid tropical climate in the Indian subcontinent (Anil et al., 2022). In the maize-wheat system, residue management combined with site specific nutrient management improves the labile and available soil P fractions under CA (Haokip et al., 2020). This, in turn, increases nutrient use efficiency, leading to improved profitability and reduced environmental footprint in the production of wheat (Rawal et al., 2022). However, lower residue retention rates and limited soil structure improvements under reduced tillage often result in decreased availability of labile and organic P under CA (Margenot et al., 2017). P management in CA differs fundamentally from conventional farming, as reduced or zero tillage alters the soil's condition and nutrient dynamics. Therefore, it is crucial to prioritize the standardization of location-specific P management options (Kumar et al., 2018). While researchers have extensively studied nitrogen, potassium, and other nutrients, limited evidence is available regarding P management in CA-based wheat production systems (Kumawat et al., 2018). In light of these facts, we hypothesize that external P application improves wheat productivity, grain quality, and resource use efficiency in CA-based wheat production systems. With the aforementioned information in mind, a recent study was conducted with specific goals to study the interactive influence of tillage, residue, and P management options on wheat grown in the semi-arid climate of India.
2. Materials and methods
2.1. Experimental site
During two consecutive winter seasons (2016–2017 and 2017–2018), trials were executed at research farm of the Indian Agricultural Research Institute (IARI), New Delhi (located at latitude 28°38′0839″ north and longitude 77°09′1442″ east; Figure 1). The experimental farm is situated in a subtropical semi-arid climate and is classified as the Mehrauli series of the Inceptisol order. The soil of the experimental plot is sandy clay loam and mildly alkaline, with pH 8.10 (non-saline) and electrical conductivity 0.24 dS m−1 (Jackson, 1973). The topsoil (0–15 cm soil depth) contained 0.43% organic carbon (Walkley and Black, 1934), 191 kg ha−1 of available nitrogen (N) (Subbiah and Asija, 1956), 11.9 kg ha−1 of available phosphorus (Olsen et al., 1954), and 208 kg ha−1 of available potassium (K) (Jackson, 1973). Monthly weather data for the experimental period (2016–2017 and 2017–2018) were obtained from a nearby meteorological observatory situated at ICAR-IARI in New Delhi (Figure 2).
2.2. Experimental design
In the experimental field, individual plots (5.6 m × 4.2 m) were prepared by manual laborers with the help of spade to avoid the mixing of the soil in different plots with different nutrient treatments. Experiment was conducted in split plot design, where the main plots consisted of three tillage methods: conventional tillage with no residue (CT – R), no-tillage with no-corn residue (NT – R), and no-tillage with maize residue @ 2.5 Mg ha−1 (NT + R). The subplots were assigned five P management strategies: P1 (Recommended N and K, and No-P), P2 (Recommended N and K, and 17.2 kg P ha−1), P3 (Recommended N and K, and 17.2 kg P ha−1 + PSB culture), P4 (Recommended N and K, and 17.2 kg P ha−1 + compost inoculant culture), and P5 (Recommended N and K, and 34.4 kg P ha−1). Each treatment combination was replicated three times. Under the NT + R treatment, maize residues (from the preceding crop) were incorporated at a rate of 2.50 Mg ha−1. A compost inoculant containing a mixture of Trichoderma viride, Aspergillus awamori, Aspergillus nidulans, and Phanerochaete chrysosporium was used, along with a PSB culture acquired from Microbiology Division of IARI, New Delhi.
2.3. Field experiment
Fertilizers were applied according to the treatment plan, utilizing urea, diammonium phosphate, and potassium chloride as inorganic nutrient sources for nitrogen, phosphorus, and potassium, respectively. The recommended dose of fertilizer (RDF) was 150 kg N ha−1, 34.4 kg P ha−1, and 49.8 kg K ha−1. At sowing, the full dose of K and half dose of N were applied (basal dose), while the remaining nitrogen was top-dressed during the first, second, and third irrigations as per the treatment plan. P doses were administered based on the recommended guidelines of the treatment plan. Prior to sowing the crop, a suitable culture of microbial consortia was used for seed treatment. In a plastic bucket, a mixture of 20–25 L of warm water (60°C) and 2.5 kg of jaggery was prepared, and wheat seeds were soaked in this solution for 30 min. Following the recommended guidelines, the seeds were treated with the appropriate carrier (PSB culture) at a rate of 20 g kg−1 and shade dried for 6–7 h before sowing in their respective treatment plots. In certain plots, immediately after sowing the crop, the compost inoculant culture was mixed with water and applied over the crop residue at a rate of 1,250 ml per hectare. Wheat variety “HD 2967” was sown in Ist week of November by manually at rate of 100–125 kg seed ha−1 with a row spacing of 20 cm and harvested at IInd week of April during both the year. Standardized package and practices of crop cultivation were followed for both test years.
2.4. Crop productivity
The aboveground biomass yield from each net plot area was manually harvested using a sickle and threshed using a pullman thresher after sun-drying for 1 week. The harvested biomass was then oven-dried at 65°C for 72 h. The weight of the grains was recorded after they had been cleaned and sun-dried for 1 week following the final harvest. The wheat grain yield was assessed when the seed's moisture content fell within the range of 12%−14%. The per plot grain yield was measured and converted as Mg ha−1.
2.5. Quality parameters
2.5.1. Analytical procedure for the determination of amino acids and protein content
The determination of amino acids (except tryptophan) was performed according to the methodology described by Szkudzinska et al. (2017). Chromatographic separation was performed on an AccQ-Tag Ultra C-18 column and quantified using a PDA detector at 260 nm. Amino acid identification was performed by comparing the retention times of the peak in the sample with that of the standard. The amino acid content of the sample was calculated using Empower software, using the internal standard. The total protein content of the grains was determined by using the Kjeldahl method (N 6.25) according to the methodology of AACC Method (AACC Method 46-11.02) (AACC, 2010). The grain amino acid yield and protein yield was determined using following equation as suggested by Kaur et al. (2020).
2.6. Phosphorus use efficiency
Agronomic efficiency (AE) was determined using the below formula as given by Karlovsky (1982)
Crop recovery efficiency (CRE) was calculated through below formula as given by Shabnam and Iqbal (2016)
2.7. Energy dynamics
The total amount of energy used in all inputs was calculated by combining their energy equivalents for direct and indirect energy of renewable and non-renewable production inputs as follows
2.8. Statistical analysis
In the current investigation, we analyzed the data from three independent replicates of each treatment using common linear modeling techniques implemented in the Statistical Analysis System 9.3 software package (SAS Institute, Cary, NC). Analysis of variance (ANOVA) was conducted for split plot design to know the significance of the “F” value. For multiple comparisons of treatment means, the critical difference (least significant difference-LSD) at 5% level of significance was employed.
To assess grain quality features, principal component analysis (PCA) was conducted to identify statistically significant Eigenvalues that would help in selecting a minimum dataset of prominent grain quality parameters.
3. Results
3.1. Yield attributes and yield (Mg ha−1)
Significant variations in wheat yield attributes were observed (p < 0.05) under different tillage and residue management options, except for spike length and thousand grain weight, throughout the study period (Table 1). Retention of crop residues increased crop yield by 4.17%−21.6% regardless of tillage treatments without residue retention. The highest significant values for tiller counts (416–423), number of grains per spike (33.8–34.7), and number of spikelets per spike (18.7–19.6) were observed under the NT + R treatment compared to CT – R and NT – R. Similarly, the NT + R treatment showed a significant improvement in grain yield (~23.5%) and straw yield (~18%), followed by NT – R during the study period. The application strategies for P had a significant (p < 0.05) influence on yield-attributing traits and crop production, except for 1,000 grain weight. The P5 treatment exhibited the greatest increase in all harvestable yield attributes. As the rate of P application increased, there was a notable increase in the number of tillers per square meter (2.8%−10.4%), spike length (1.6%−12.5%), number of spikelets per spike (5.62%−57.6%), and number of grains per spike (4.01%−15.6%). The increase in grain yield (34.7%−70.4%) was almost equivalent to the increase in straw yield (33.2%−60.7%) with the rising P application rate. The interaction effect of tillage, residues, and P management practices on wheat yield was significant (p < 0.05) during both years of the study (Figure 3). Among the different treatment combinations, the combined application of NT + R with the P5 treatment resulted in a significant enhancement of grain yield by ~43.2% compared to the CT – R with the P1 treatment combination.
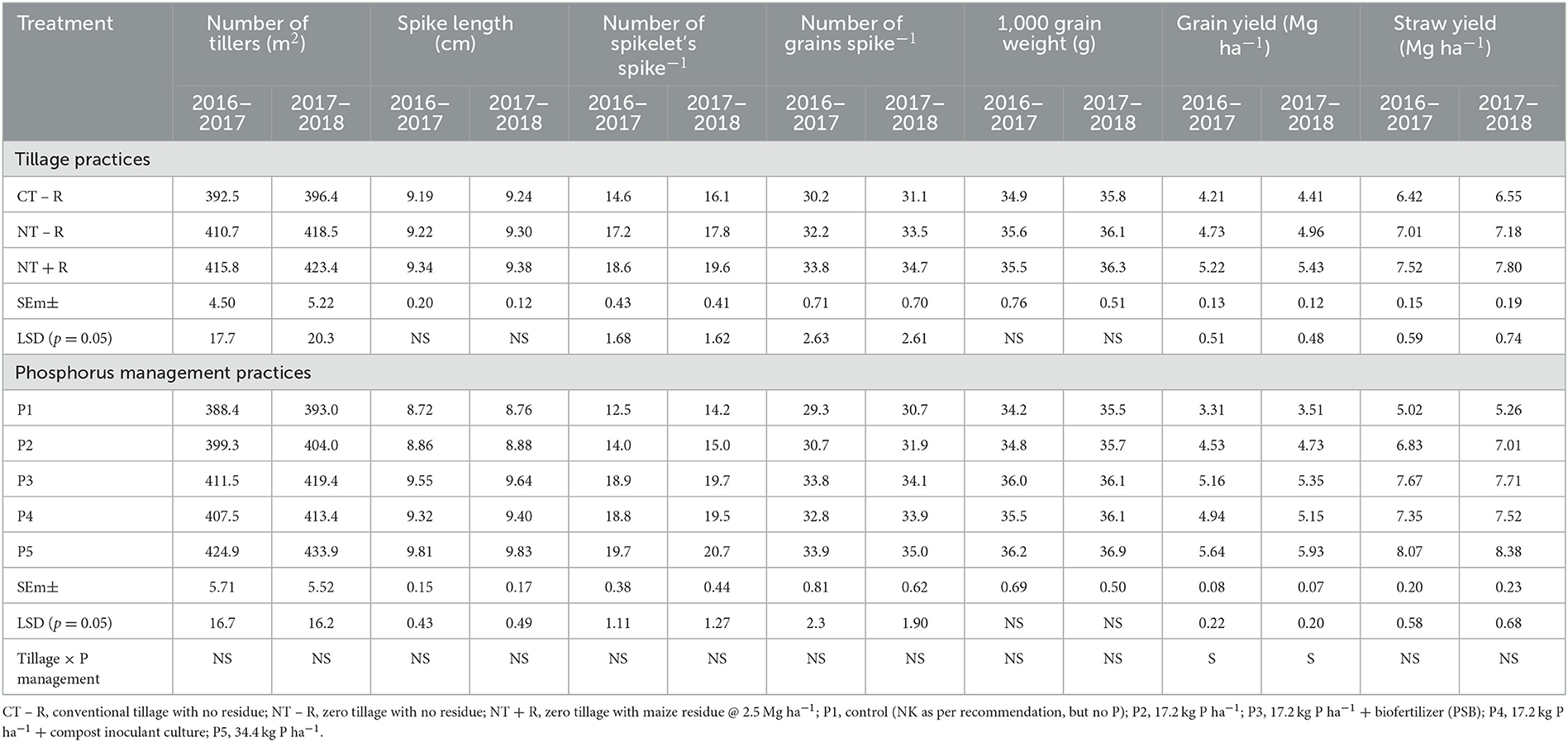
Table 1. Yield attributes and yield of wheat influenced by tillage, residue and phosphorus management practices under conservation agriculture.
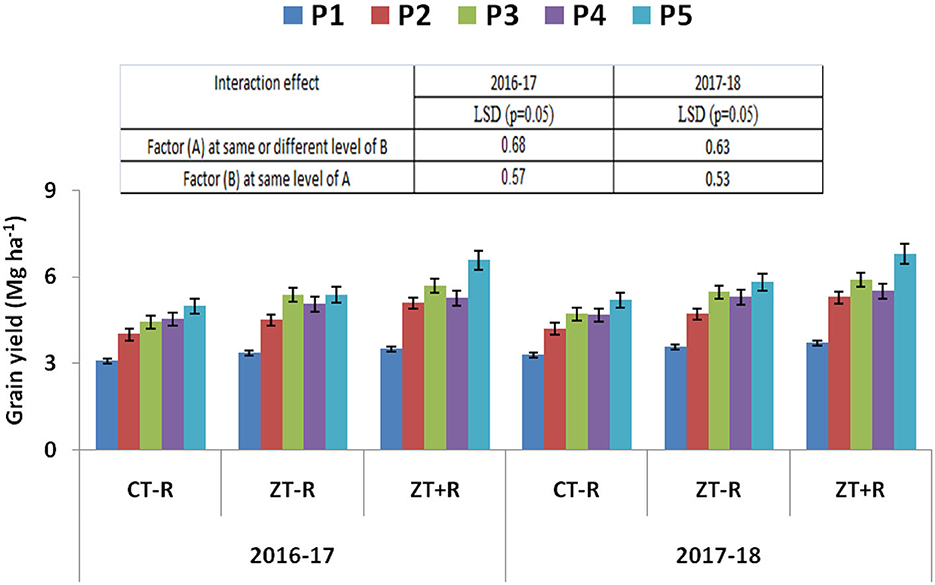
Figure 3. Interaction effects of tillage, residue and phosphorus management practices on grain yield of wheat under conservation agriculture (2016–2017 and 2017–2018). CT – R, conventional tillage with no residue; NT – R, No tillage with no residue; NT + R, no tillage with maize residue @ 2.5 Mg ha−1; P1, control (NK as per recommendation, but no P); P2, 17.2 kg P ha−1; P3, 17.2 kg P ha−1 + biofertilizer (PSB); P4, 17.2 kg P ha−1 + compost inoculant culture; P5, 34.4 kg P ha−1.
3.2. Quality parameters
Tillage and residue management did not have a significant impact (p < 0.05) on amino acid composition and wheat grain protein content (Supplementary Table 2). However, they did have a significant impact on cumulative amino acids and net protein yield throughout the study years, following the order: NT + R > NT – R > CT – R (Table 2). The NT + R treatment showed a notable increase in amino acid yield, including lysine (~25.2%), methionine (~23.1%), proline (~22.0%), glycine (~20.4%), alanine (~31.4%), cystine (~18.7%), phenylalanine (~18.5%), and net protein yield (~21.2%) compared to CT – R. The P management practices had a significant (p < 0.05) impact on amino acid composition and wheat grain protein content (Supplementary Table 2). The P5 treatment exhibited the highest amplification of amino acid content (3.28 and 3.30% lysine, 1.91 and 1.92% methionine, 8.23 and 8.25% proline, 6.26 and 6.27% glycine, 7.76 and 7.78% alanine, 3.58 and 3.60% cystine, 4.91 and 4.95% phenylalanine) and protein content (10.8 and 10.9%), which was statistically similar to P3 and P4. Notably, a significant increase in amino acid yield (~65.7% lysine, ~59.1% methionine, ~60.7% proline, ~59.9% glycine, ~69.1% alanine, ~22.2% cystine, ~7.10% phenylalanine) and net protein yield (~12.6%) was recorded under the P5 treatment, followed by P3 throughout the 2-year experimental period (Table 2).
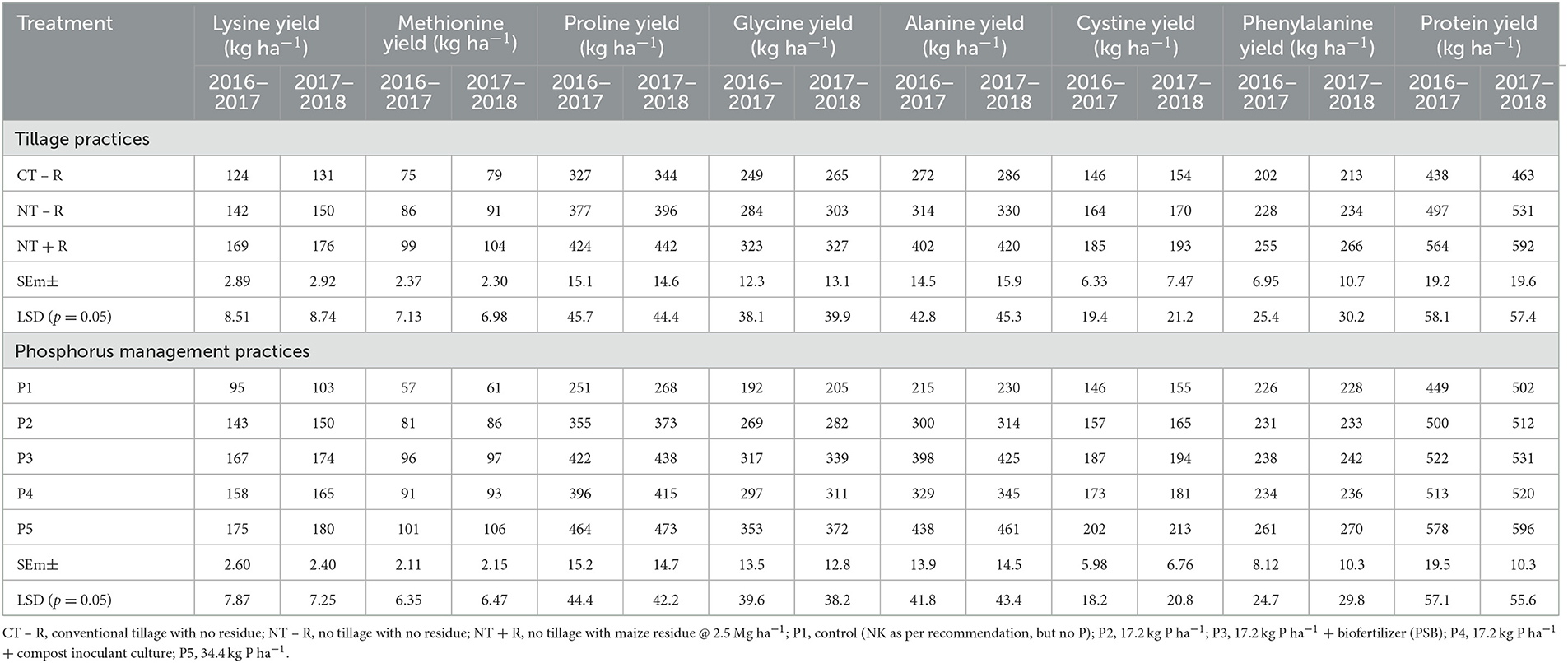
Table 2. Influence of tillage and microbial inoculants mediated integrated P management on quality parameters (amino acids and protein yield) of wheat under conservation agriculture.
Principal component analysis (PCA) was conducted to study the amino acids and net protein yield in wheat grain. Two principal components (PCs) with eigenvalues ≥1 were generated, explaining a total variation of 97.7% of the total variance since they possessed eigenvalues >1.0 (Supplementary Table 2). The loading plot showed that PC1 (80.9%) exhibited significant positive loadings for proline yield, protein yield, glycine yield, and alanine yield. Similarly, PC2 had higher loadings for lysine yield, cystine yield, phenylalanine yield, and methionine yield. The quadrant first score plot identified treatments such as NT + R, P5; NT + R, P3; NT + R, P4; NT – R, P4; and NT + R, P2, showing positive heavy loadings on PC1. Notably, the fourth quadrant included diverse treatments such as NT – R, P5; NT – R, P3; and CT – R, P5 (Figure 4).
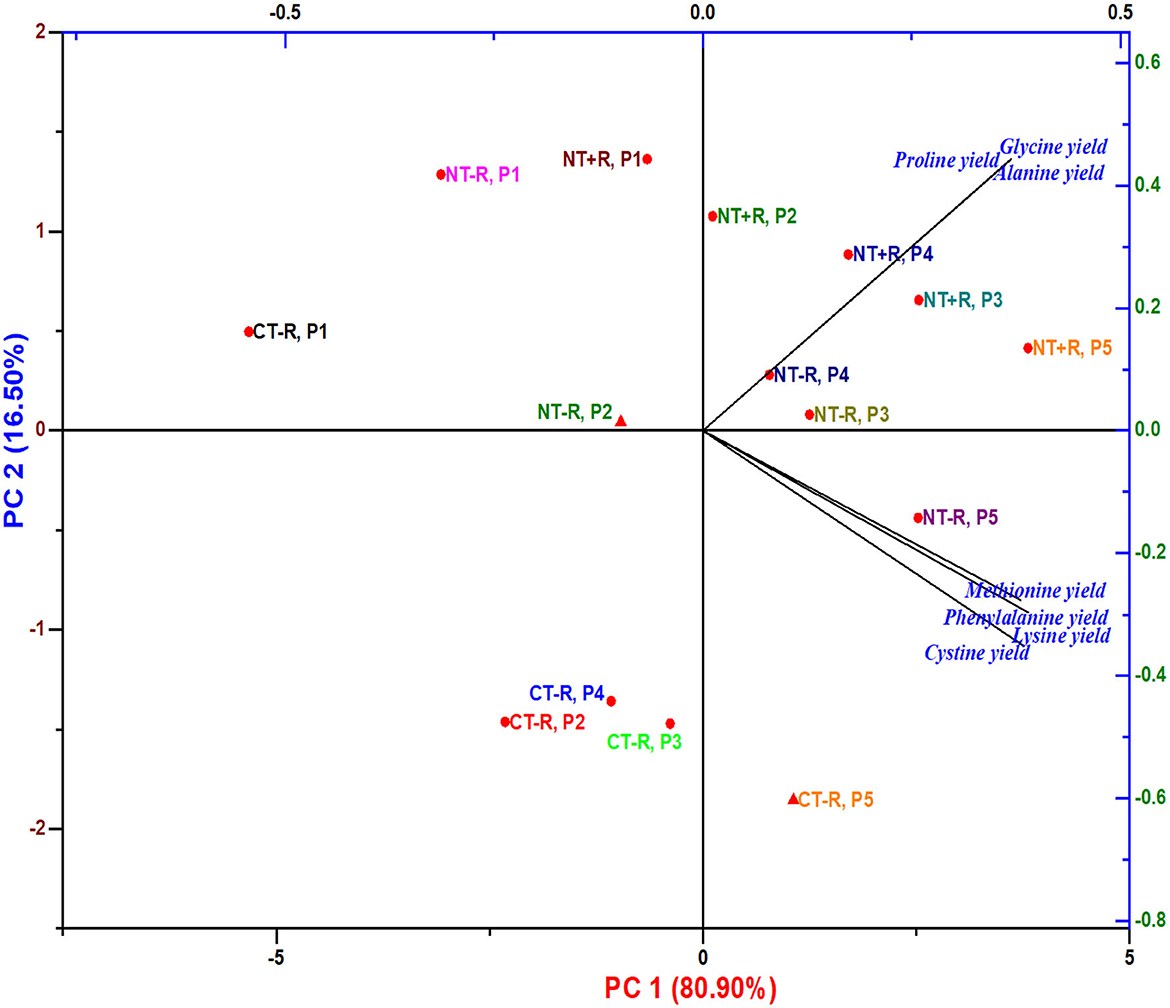
Figure 4. Biplot formed by PC1 and PC2 showing the scores and loadings. CT – R, conventional tillage with no residue; NT – R, no tillage with no residue; NT + R, no tillage with maize residue @ 2.5 Mg ha−1; P1, control (NK as per recommendation, but no P); P2, 17.2 kg P ha−1; P3, 17.2 kg P ha−1 + biofertilizer (PSB); P4, 17.2 kg P ha−1 + compost inoculant culture; P5, 34.4 kg P ha−1.
3.3. Phosphorus use efficiency
A significant interaction was observed between different tillage practices and P management strategies (p < 0.05; Table 3). The P agronomic efficiency was significantly higher for the treatment combination of P3 with NT + R (~128 kg grain kg−1 of applied P) compared to other treatment combinations. In the first year, the highest P recovery efficiency was observed for the combination of NT + R and P3 (77.3 kg P uptake kg−1 of P applied). Similarly, in the second year, a higher P recovery efficiency of 71.4 kg P uptake kg−1 of P applied was observed with the NT + R and P3 treatment combination. This result was not significantly different from the NT – R and P3 treatment combination but was considerably superior to the CT – R and P3 treatment combination.
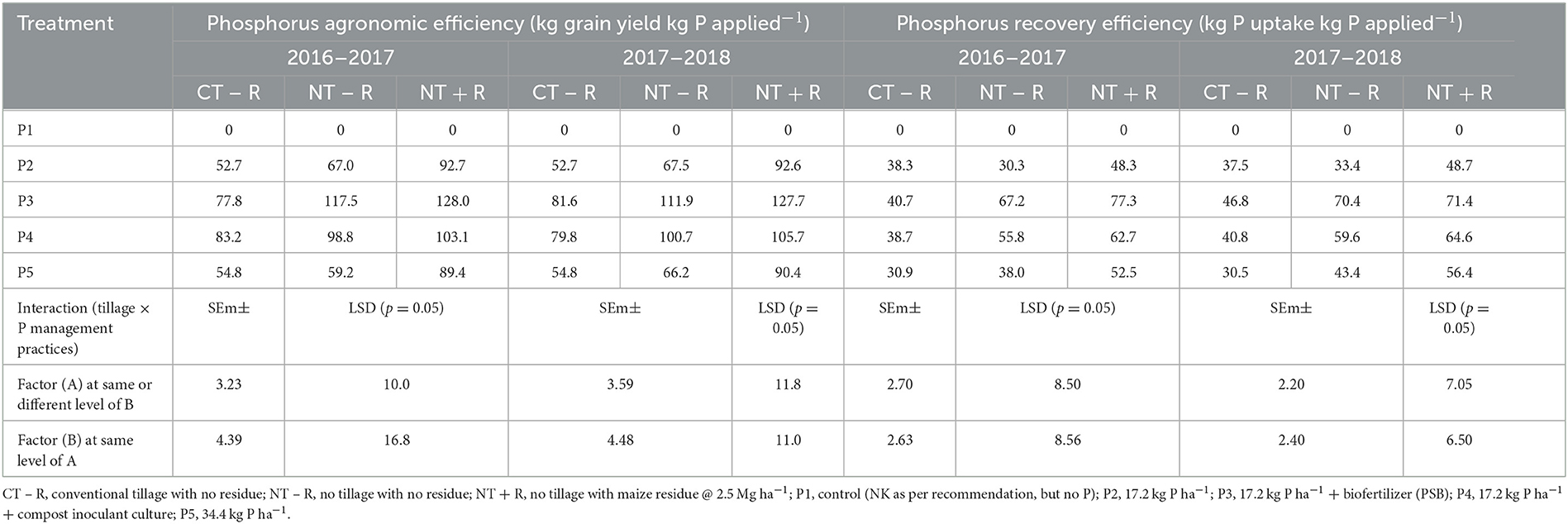
Table 3. Influence of tillage and microbial inoculants mediated integrated P management on phosphorus agronomic and recovery efficiency of wheat under conservation agriculture.
3.4. Energy dynamics
The energy dynamics were significantly influenced by different combinations of tillage practices, residue management, and P management strategies (Figures 5–7). A notable increase of 27% in energy gross return was observed in the NT + R treatment compared to the other tillage practices. The NT + R treatment resulted in the highest energy input, with a maximum of 50.1 and 50.2 × 103 MJ ha−1, while the lowest energy input was observed under NT – R, with 18.8 and 18.8 × 103 MJ ha−1. Implementing NT – R tillage practices led to a significant increase in net energy returns by ~32% and energy use efficiency by ~58% compared to ZT + R and CT – R. Throughout the study period, the P5 treatment exhibited the highest gross energy returns (184 and 192 × 103 MJ ha−1), energy input (30.04 and 30.09 × 103 MJ ha−1), and net energy returns (154 and 162 × 103 MJ ha−1) compared to other treatments in terms of P management options. The interaction effect showed a significant increase (~58%) in energy use efficiency in the NT – R with P3 treatment combination. The next highest energy use efficiency was observed in the NT – R with P4 and NT – R with P5 treatment combinations.
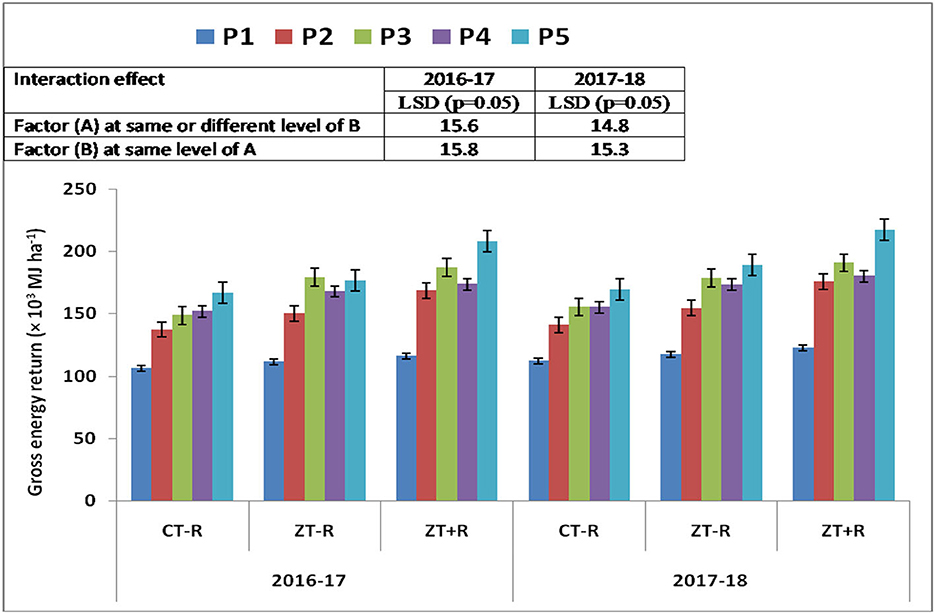
Figure 5. Interaction effects of tillage, residue and phosphorus management practices on energy gross returns of wheat under conservation agriculture (2016–2017 and 2017–2018). CT – R, conventional tillage with no residue; NT – R, no tillage with no residue; NT + R, no tillage with maize residue @ 2.5 Mg ha−1; P1, control (NK as per recommendation, but no P); P2, 17.2 kg P ha−1; P3, 17.2 kg P ha−1 + biofertilizer (PSB); P4, 17.2 kg P ha−1 + compost inoculant culture; P5, 34.4 kg P ha−1.
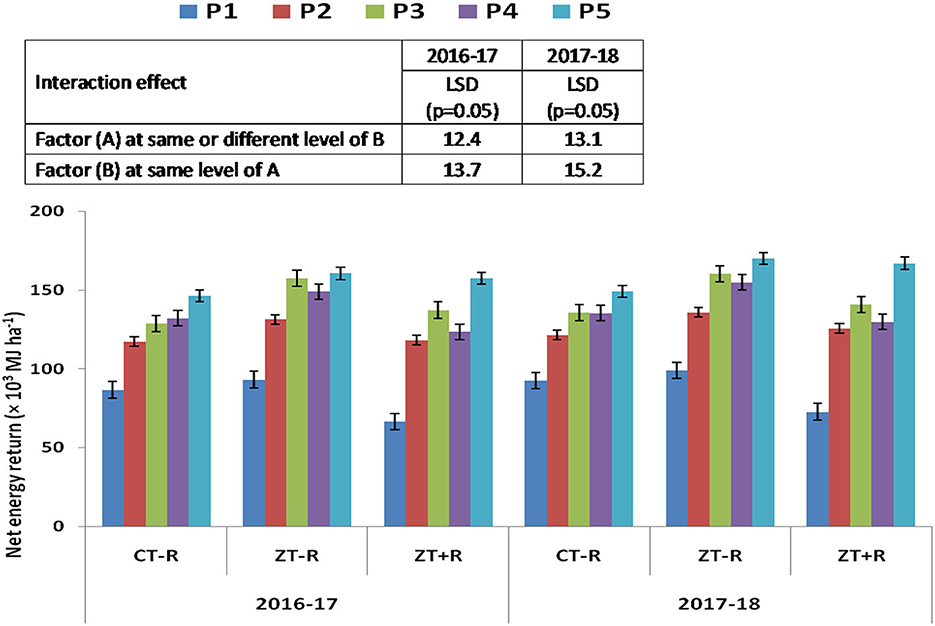
Figure 6. Interaction effects of tillage, residue and phosphorus management practices on energy net returns of wheat under conservation agriculture (2016–2017 and 2017–2018). CT – R, conventional tillage with no residue; NT – R, no tillage with no residue; NT + R, no tillage with maize residue @ 2.5 Mg ha−1; P1, control (NK as per recommendation, but no P); P2, 17.2 kg P ha−1; P3, 17.2 kg P ha−1 + biofertilizer (PSB); P4, 17.2 kg P ha−1 + compost inoculant culture; P5, 34.4 kg P ha−1.
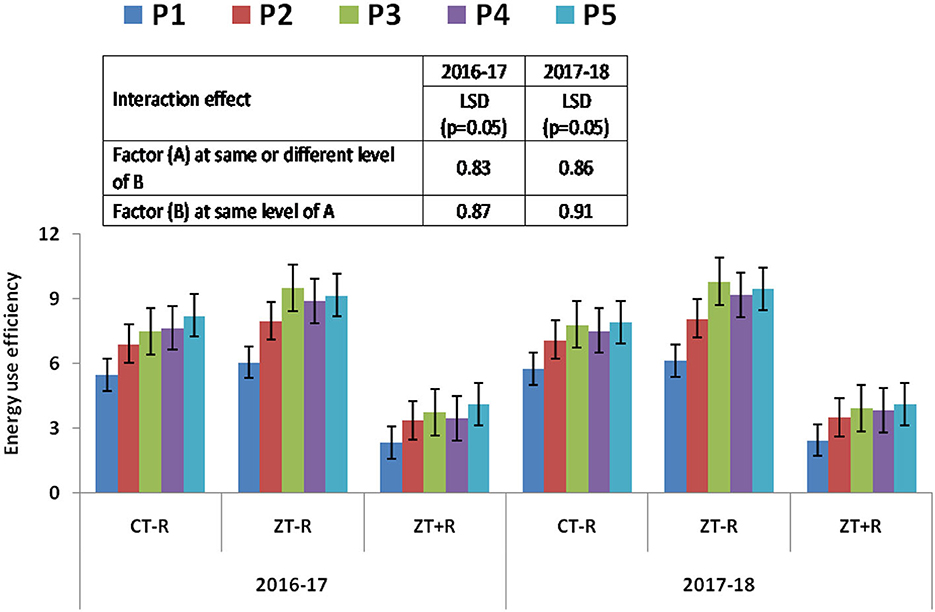
Figure 7. Interaction effects of tillage, residue and phosphorus management practices on energy use efficiency of wheat under conservation agriculture (2016–2017 and 2017–2018). CT – R, conventional tillage with no residue; NT – R, no tillage with no residue; NT + R, no tillage with maize residue @ 2.5 Mg ha−1; P1, control (NK as per recommendation, but no P); P2, 17.2 kg P ha−1; P3, 17.2 kg P ha−1 + biofertilizer (PSB); P4, 17.2 kg P ha−1 + compost inoculant culture; P5, 34.4 kg P ha−1.
4. Discussion
4.1. Yield attributes and yield
In our study, CA improved crop productivity and sustainability of wheat in maize-wheat cropping systems. The NT + R treatment ensured higher nutrient availability (N, P, K), leading to better crop establishment, reduced weed infestation, and efficient nutrient utilization, resulting in higher grain yield and straw yield compared to the NT – R treatment during both years of experimentation (Busari et al., 2015; Page et al., 2019). The addition of crop residues played a role in preserving soil moisture and enhancing nutrient availability, promoting cellular reproduction, expansion, and overall crop growth, resulting in higher economic yield (Jinger et al., 2020; Jat et al., 2021). Additionally, the P management strategies significantly influenced yield-attributing traits and crop production throughout the trial period. The P5 treatment performed better in terms of yield attributes compared to P1, as higher available P in less weathered Inceptisols facilitates plant metabolism, energy conversion, and photosynthetic assimilation, leading to improved aboveground biomass assimilation, root growth, and supportive yield attributes (Ahmed et al., 2017). In our study, the combination of NT + R with P5 resulted in significantly superior grain production (6.56 and 6.80 Mg ha−1, respectively) compared to CT – R along with P1 treatment combination (Figure 3) due to the balanced acquisition of available N, P, and K under conservation agriculture (Anwar et al., 2016; Rehim et al., 2016).
4.2. Quality parameters
Amino acids and protein content in crops are the end products of successful growth and development, often regulated by the rate of carbon uptake and conversion into harvestable products. In our study, wheat grain quality in terms of lysine, methionine, proline, glycine, alanine, cystine, phenylalanine, and protein content was assessed. Wheat grown under NT + R treatment yielded significantly higher amino acids and net grain protein content. This can be attributed to increased root activity and improved soil aeration, facilitating nutrient uptake, particularly nitrogen, and its translocation from vegetative parts to the grain in standing wheat crop (Fernandez et al., 2018). Enhanced growth, productivity, and elevated levels of amino acids and protein ultimately led to increased overall yields of amino acids and protein (Babu et al., 2020; Kumar et al., 2023a). The observed improvements in amino acids and net protein yield may be due to the rising P levels, which could result in increased amino acids and improved nitrogen nutrition for the crops. This could be attributed to greater root development, more effective nutrient and water uptake by plants, and higher grain yield (Jamal and Fawad, 2019; Garutti et al., 2022). Under higher P nutrition levels (P5), maximal values of amino acids (lysine, methionine, proline, glycine, alanine, cystine, and phenylalanine) and protein content were observed, which were comparable to P3 and P4 treatments. The increase in the limiting amino acids of wheat grain, lysine (8.20%−11.6%), and methionine (4.02%−11.7%), was more prominent with the increase in P application levels. However, tillage practices had minimal impact on modifying grain amino acid content in wheat. The increase in external P application promoted wheat growth and enhanced crop nutrient uptake, leading to the observed increase in wheat grain amino acid content (Yi et al., 2023). The increasing P integration rates stimulated root activity, nutrient absorption from the soil solution, and translocation from source to sink, indirectly influencing protein and amino acid levels (Kumar and Saini, 2022). Moreover, the abundant P supply may require the breakdown of carbohydrates into acetyl co-enzyme A, an essential component for the production of amino acids and proteins (Haokip et al., 2019; Ali et al., 2020). Thus, external application of P amplified the grain amino acids and net protein yield in our study. Similar to Babicki et al. (2016), the PCA score plot displayed that treatments such as NT + R, P5; NT + R, P3; NT + R, P4; NT – R, P4; and NT + R, P2 showed positive heavy loading on PC1. Notably, the fourth quadrant included diverse treatments such as NT – R, P5; NT – R, P3; and CT – R, P5.
4.3. Phosphorus use efficiency
The P agronomic efficiency was significantly higher under the NT + R with P3 treatment combination compared to other combinations, as it resulted in enhanced grain yield per unit application of external P fertilizers (Johnston and Poulton, 2019). Furthermore, the inclusion of PSB in the P3 treatment led to higher P agronomic efficiency, saving 50% of P fertilizers while increasing grain yield. This increased wheat yield per unit P nutrient utilization contributed to improved P agronomic efficiency (Guelfi et al., 2022). In the first year, the NT + R and P3 treatment combination exhibited notably higher P recovery efficiency compared to other combinations. Similarly, in the second year, the same combination of NT + R and P3 treatment showed a higher P recovery efficiency, which was not significantly different from the NT – R and P3 treatment, but was significantly superior to the CT – R and P3 treatment combination. The solubilization of fixed P by PSB and the band placement of P fertilizers may explain this, as the amount of P sorbed is influenced by the soil-to-applied P ratio (Bijarniya et al., 2020). The higher grain yield and increased P uptake led to improved root growth dynamics, resulting in greater efficiency in P recovery and higher P utilization efficacy under the P3 treatment (Salim et al., 2023). This enhanced nutrient cycling enhances phosphorus utilization by plants, particularly when combined with a reduced amount of chemical fertilizer (Luo et al., 2017; Ven et al., 2019). The combination of chemical fertilizer and biofertilizer (PSB) can have synergistic effects on phosphorus availability and plant uptake. PSB facilitate the release of phosphorus and promote nutrient cycling through the decomposition of organic matter, releasing nutrients into the soil solution, thereby making them more available to plants (Nunes et al., 2022). The use of biofertilizers can potentially reduce phosphorus fixation by producing organic acids and enzymes that can dissolve or chelate soil-bound phosphorus, making it more accessible to plants (Yuan et al., 2016). Therefore, when biofertilizers are combined with a reduced amount of chemical fertilizer, overall P availability and recovery efficiency can be improved. By reducing the amount of chemical fertilizer applied and supplementing it with biofertilizers, nutrient losses can be minimized. This reduction in nutrient losses ensures that more phosphorus remains available in the soil for plant uptake, resulting in a higher phosphorus recovery efficiency for the P3 treatment compared to the P4 and P5 treatments. Additionally, at higher levels of applied P, plants utilize a smaller proportion of the added P, leading to a decreased P recovery efficiency (Kumar et al., 2023a,b).
4.4. Energy dynamics
In our study, the energy dynamics were significantly influenced by diverse tillage practices, residue management, and P management strategies. The implementation of NT + R tillage practices resulted in a notable increase in net energy returns by 87.9% compared to CT – R, while the gross energy returns were statistically similar to NT – R. The higher energy input in the NT + R system was attributed to the addition of residues (2.5 Mg ha−1), whereas the NT – R and CT – R systems had lower energy inputs due to reduced tillage practices. Consistent with Kumar et al. (2021), the higher energy demand was primarily driven by the high energy value of the residues (12.5 MJ kg−1). During both years, the NT – R method achieved the highest net energy returns and energy use efficiency, which were significantly greater than those achieved through NT + R and CT – R. This was mainly due to reduced energy usage and increased energy production resulting from higher grain and stover harvest. However, the excessive addition of crop residues can reduce the effectiveness in achieving energy balance and energy use efficiency, as the residues have a high potential energy value (Jat et al., 2018). Therefore, our results indicate that reduced tillage methods significantly increase yield and net energy income compared to conventional tillage (Kumar et al., 2023b). In terms of P management approaches, the P5 treatment consistently exhibited the highest gross returns of energy, energy input, and net returns of energy over the 2-year period. The interaction effect confirmed a percent increment of 56.3 in energy use efficiency for the NT – R with P3 treatment combination, followed by NT – R with P4 and NT – R with P5 (Kumawat et al., 2018; Haokip et al., 2020).
5. Conclusion
Based on 2 years of experimentation it was summarized that NT + R in combination with P5 resulted in significantly superior grain yield compared to CT – R. Additionally, NT + R exhibited noticeably higher amino acid and protein yield compared to CT – R. Among the P nutrition treatments, the highest amino acid and protein yield was observed in the P5 treatment, followed by the P3 treatment. The NT + R with P3 treatment combination showed substantially higher P agronomic efficiency and P recovery efficiency compared to the other treatment combinations. While, energy use efficiency was significantly higher under NT – R with P3 treatment combination. Hence, adopting a sustainable agricultural approach that combines microbiota-based integrated phosphorus management with no-tillage practices and the retention of crop residues represents an energy-efficient and ecologically sound method to support the cultivation of premium-quality wheat in semi-arid regions.
Data availability statement
The original contributions presented in the study are included in the article/Supplementary material, further inquiries can be directed to the corresponding author.
Author contributions
Conceptualization, methodology and visualization, validation, and visualization: AK, SD, and UKB. Software: SB, RS, and SK. Formal analysis: AK, AdK, and GG. Investigation: AK. Writing original draft preparation: AK, PKU, BAG, SS, GV, and RS. Writing review and editing: PKU, AK, RKS, SS, GV, and RD. Supervision and research administration: UKB and SD. All authors contributed to the article and approved the submitted version.
Funding
The author(s) declare that no financial support was received for the research, authorship, and/or publication of this article.
Acknowledgments
Authors are highly thankful to the Director and Vice chancellor of Indian Agricultural Research Institute for all the support to conduct the experiment.
Conflict of interest
The authors declare that the research was conducted in the absence of any commercial or financial relationships that could be construed as a potential conflict of interest.
Publisher's note
All claims expressed in this article are solely those of the authors and do not necessarily represent those of their affiliated organizations, or those of the publisher, the editors and the reviewers. Any product that may be evaluated in this article, or claim that may be made by its manufacturer, is not guaranteed or endorsed by the publisher.
Supplementary material
The Supplementary Material for this article can be found online at: https://www.frontiersin.org/articles/10.3389/fsufs.2023.1235141/full#supplementary-material
References
AACC (2010). “AACC method 46-11.02. Crude protein–improved Kjeldahl method, copper catalyst modyfication,” in Official Methods of the American Association of Cereal Chemists, 11th ed. (St. Paul, MN: AACC), 39, 72.
AGRICOOP (2022). Directorate of Economics and Statistics Department of Agriculture, Cooperation and Farmers Welfare Second Advance Estimates of Production of Food Grains for 27/02/2018. Available online at: http://agricoop.gov.in/sites/default/files/2ND_ADV_EST_APY_201718_E.pdf (accessed May, 2023).
Ahmed, K., Qadir, G., Nawaz, M. Q., Saqib, A. I., Rizwan, M., Zaka, M. A., et al. (2017). Integrated phosphorus management improves production of rice–wheat cropping system under salt affected conditions. Int. J. Plant Prod. 12, 25–32. doi: 10.1007/s42106-017-0003-x
Ali, A., Asif, M., Adnan, M., Aziz, A., Hayyat, M. S., Saleem, M. W., et al. (2020). Effect of different levels of phosphorus on growth, yield and quality of wheat (Triticum aestivum L.). Int. J. Bot. Stud. 5, 64–68.
Anil, A. S., Sharma, V. K., Jiménez-Ballesta, R., Parihar, C. M., Datta, S. P., Barman, M., et al. (2022). Impact of long-term conservation agriculture practices on phosphorus dynamics under maize-based cropping systems in a sub-tropical soil. Land 11, 1488. doi: 10.3390/land11091488
Anwar, S., Israeel Babar, I., Khan, A. S., Imran, Shah, W. A., et al. (2016). Nitrogen and phosphorus fertilization of improved varieties for enhancing phenological traits of wheat. Pure Appl. Biol. 5, 511–519. doi: 10.19045/bspab.2016.50065
Babicki, S., David, A., Ana, M., Liang, Y., Jason, R., Grant, A. M., et al. (2016). Heatmapper: web-enabled heat mapping for all. Nucleic Acids Res. 44, 147–153. doi: 10.1093/nar/gkw419
Babu, S., Singh, R., Avasthe, R. K., Yadav, G. S., Das, A., Singh, V. K., et al. (2020). Impact of land configuration and organic nutrient management on productivity, quality and soil properties under baby corn in Eastern Himalayas. Sci. Rep. 10, 1–14. doi: 10.1038/s41598-020-73072-6
Bijarniya, D., Parihar, C. M., Jat, R. K., Kalvania, K., Kakraliya, S. K., Jat, M. L., et al. (2020). Portfolios of climate smart agriculture practices in smallholder rice-wheat system of eastern Indo-Gangetic Plains-crop productivity, resource use efficiency and environmental foot prints. Agronomy 10, 1561. doi: 10.3390/agronomy10101561
Busari, M. A., Kukal, S. S., Kaur, A., Bhatt, R., and Duazi, A. A. (2015). Conservation tillage impacts on soil, crop and the environment. Soil Water Conserv. Res. 3, 119–129. doi: 10.1016/j.iswcr.2015.05.002
Fernandez, M. R., Zentner, R. P., Schellenberg, M. P., Aladenola, O., Leeson, J. Y., Luce, M. S., et al. (2018). Soil fertility and quality response to reduced tillage and diversified cropping under organic management. Agron. J. 111, 781–792. doi: 10.2134/agronj2018.01.0028
Galani, Y. J. H., Ligowe, I. S., Kieffer, M., Kamalongo, D., Kambwiri, A. M., Kuwali, P., et al. (2022). Conservation agriculture affects grain and nutrient yields of maize (Zea mays L.) and can impact food and nutrition security in Sub-Saharan Africa. Front. Nutr. 8, 1291. doi: 10.3389/fnut.2021.804663
Garutti, M., Nevola, G., Mazzeo, R., Cucciniello, L., Totaro, F., Bertuzzi, C. A., et al. (2022). The impact of cereal grain composition on the health and disease outcomes. Front. Nutr. 9, 888974. doi: 10.3389/fnut.2022.888974
Guelfi, D., Nunes, A. P. P., Sarkis, L. F., and Oliveira, D. P. (2022). Innovative phosphate fertilizer technologies to improve phosphorus use efficiency in agriculture. Sustainability 14, 14266. doi: 10.3390/su142114266
Haokip, I. C., Dwivedi, B. S., Meena, M. C., Datta, S. P., Jat, H. S., Dey, A., et al. (2020). Effect of conservation agriculture and nutrient management options on soil phosphorus fractions under maize-wheat cropping system. J. Indian Soc. Soil Sci. 68, 45–53. doi: 10.5958/0974-0228.2020.00005.5
Haokip, I. C., Dwivedi, B. S., Meena, M. C., Datta, S. P., Sharma, V. K., Saharawat, Y. S., et al. (2019). Effect of phosphorus fertilization and microbial inoculants on yield, phosphorus use-efficiency and available phosphorus in maize–wheat cropping system. Indian J. Agric. Sci. 89, 806–812. doi: 10.56093/ijas.v89i5.89662
Jackson, M. L. (1973). Soil Chemical Analysis. New Delhi: Prentice Hall of India Private Limited, 187.
Jamal, A., and Fawad, M. (2019). Effectiveness of phosphorous fertilizers in wheat crop production in Pakistan. J. Hort. Plant Res. 5, 25–29. doi: 10.18052/www.scipress.com/JHPR.5.25
Jat, M. L., Chakraborty, D., Ladha, J. K., Rana, D. S., Gathala, M. K., McDonald, A., et al. (2020). Conservation agriculture for sustainable intensification in South Asia. Nat. Sustain. 3, 336–343. doi: 10.1038/s41893-020-0500-2
Jat, R. C., Sharma, Y., Jakhar, R. K., and Sharma, R. K. (2018). Effect of phosphorus, zinc and iron on yield and quality of wheat in western Rajasthan, India. Int. J. Curr. Microbiol. App. Sci. 7, 2055–2062. doi: 10.20546/ijcmas.2018.703.241
Jat, R. K., Bijarniya, D., Kakraliya, S. K., Sapkota, T. B., Kakraliya, M., Jat, M. L., et al. (2021). Precision nutrient rates and placement in conservation maize-wheat system: effects on crop productivity, profitability, nutrient-use efficiency, and environmental footprints. Agronomy 11, 2320. doi: 10.3390/agronomy11112320
Jinger, D., Dhar, S., Dass, A., Sharma, V. K., Vijayakumar, S., Gupta, G., et al. (2020). Influence of residual silicon and phosphorus on growth, productivity, lodging and grain quality of succeeding wheat under rice-wheat cropping system. J. Environ. Biol. 41, 1676–1684. doi: 10.22438/jeb/41/6/SI-250
Johnston, A. E., and Poulton, P. R. (2019). Phosphorus in agriculture: a review of results from 175 years of research at Rothamsted, UK. J. Environ. Qual. 48, 1133–1144. doi: 10.2134/jeq2019.02.0078
Karlovsky, J. (1982). The balance sheet approach to determination of phosphate maintenance requirements. Fert. Res. 3, 111–125. doi: 10.1007/BF01082972
Kaur, N., Kaur, H., and Mavi, G. S. (2020). Assessment of nutritional and quality traits in biofortified bread wheat genotypes. Food Chem. 302, 125342. doi: 10.1016/j.foodchem.2019.125342
Kerbouai, I., Sfayhi, D., Sassi, K., M'hamed, H. C., Jenfaoui, H., Riahi, J., et al. (2023). Influence of conservation agriculture on durum wheat grain, dough texture profile and pasta quality in a Mediterranean region. Agriculture 13, 908. doi: 10.3390/agriculture13040908
Kumar, A., Behera, U., Dhar, S., Shukla, L., Singh, R., Babu, S., et al. (2023a). Conservation tillage and microbially mediated integrated phosphorus management enhance productivity, profitability, and energy use efficiency of maize. J. Agric. Sci. 161, 373–386. doi: 10.1017/S0021859623000266
Kumar, A., Behera, U. K., Dhar, S., Shukla, L., Bhatiya, A., Meena, M. C., et al. (2018). Effect of tillage, crop residue and phosphorus management practices on the productivity and profitability of maize cultivation in Inceptisols. Indian J. Agric. Sci. 88, 1558–1167. doi: 10.56093/ijas.v88i10.84223
Kumar, A., and Saini, K. S. (2022). Conservation agriculture effects on root traits, productivity and profitability of rice in rice-wheat-summer moong cropping system. AMA-Agric. Mech. Asia Afr. Lat. Am. 53, 5245–5254.
Kumar, A., Saini, K. S., Dasila, H., Kumar, R., Devi, K., Bisht, Y. S., et al. (2023b). Sustainable intensification of cropping systems under conservation agriculture practices: impact on yield, productivity and profitability of wheat. Sustainability 15, 7468. doi: 10.3390/su15097468
Kumar, M., Mitra, S., Mazumdar, S. P., Majumdar, B., Saha, A. R., Singh, S. R., et al. (2021). Improvement of soil health and system productivity through crop diversification and residue incorporation under jute-based different cropping systems. Agronomy 11, 1622. doi: 10.3390/agronomy11081622
Kumawat, C., Sharma, V. K., Meena, M. C., Dwivedi, B. S., Barman, M., Kumar, S., et al. (2018). Effect of crop residue retention and phosphorus fertilization on P use efficiency of maize and biological properties of soil under maize-wheat cropping system in an Inceptisol. Indian J. Agric. Sci. 88, 1184–1189. doi: 10.56093/ijas.v88i8.82527
Luo, G., Ling, N., Nannipieri, P., Chen, H., Raza, W., Wang, M., et al. (2017). Long-term fertilization regimes affect the composition of the alkaline phosphomonoesterase encoding microbial community of a vertisol and its derivative soil fractions. Biol. Fert. Soils 53, 375–388. doi: 10.1007/s00374-017-1183-3
Margenot, A. J., Paul, B. K., Sommer, R. R., Pulleman, M. M., Parikh, S. J., Jackson, L. E., et al. (2017). Can conservation agriculture improve phosphorus (P) availability in weathered soils? Effects of tillage and residue management on soil P status after 9 years in a Kenyan Oxisol. Soil Till. Res. 166, 157–166. doi: 10.1016/j.still.2016.09.003
Nunes, A. P. P., Santos, C. F., and Guelfi, D. (2022). Interfaces between biodegradable organic matrices coating and MAP fertilizer for improve use efficiency. Sci. Total Environ. 804, 149896. doi: 10.1016/j.scitotenv.2021.149896
Olsen, S. R., Cole, C. V., Watanabe, F. S., and Dean, L. A. (1954). Estimation of Available Phosphorus in Soils by Extraction with Sodium Bicarbonate. USDA Circular No. 939. Washington, DC: Gov. Printing Office, 1–19.
Page, K. L., Dang, Y. P., Dalal, R. C., Reeves, S., Thomas, G., Wang, W., et al. (2019). Changes in soil water storage with no-tillage and crop residue retention on a vertisol: impact on productivity and profitability over a 50 year period. Soil Till. Res. 194, 104319. doi: 10.1016/j.still.2019.104319
Rawal, N., Pande, K. R., Shrestha, R., and Vista, S. P. (2022). Nutrient use efficiency (NUE) of wheat (Triticum aestivum L.) as affected by NPK fertilization. PLoS ONE 17, e0262771. doi: 10.1371/journal.pone.0262771
Rehim, A., Hussain, M., Hussain, S., Noreen, I., Dogan, H., Zia-Ul-Haq, M., et al. (2016). Band-application of phosphorus with farm manure improves phosphorus use efficiency, productivity, and net returns of wheat on sandy clay loam soil. Turk. J. Agric. Agrofore. 40, 1–9. doi: 10.3906/tar-1505-133
Ruiz, M., Zambrana, E., Fite, R., Sole, A., Tenorio, J. L., Benavente, E., et al. (2019). Yield and quality performance of traditional and improved bread and durum wheat varieties under two conservation tillage systems. Sustainability 11, 4522. doi: 10.3390/su11174522
Salim, M., Chen, Y., Solaiman, Z. M., and Siddique, K. H. M. (2023). Phosphorus application enhances root traits, root exudation, phosphorus use efficiency, and seed yield of soybean genotypes. Plants 12, 1110. doi: 10.3390/plants12051110
Shabnam, R., and Iqbal, M. T. (2016). Phosphorus use efficiency by wheat plants that grown in an acidic soil. Braz. J. Sci. Technol. 3, 18. doi: 10.1186/s40552-016-0030-7
Subbiah, B. V., and Asija, G. L. (1956). A rapid procedure for estimation of available nitrogen in soils. Current Sci. 25, 259–260.
Szkudzinska, K., Smutniak, I., Rubaj, J., Korol, W., and Bielecka, G. (2017). Method validation for determination of amino acids in feed by UPLC. Accred. Qual. Assur. 22, 247–252. doi: 10.1007/s00769-017-1281-9
Ven, A., Verlinden, M. S., Verbruggen, E., Vicca, S., and Hart, M. (2019). Experimental evidence that phosphorus fertilization and arbuscular mycorrhizal symbiosis can reduce the carbon cost of phosphorus uptake. Funct. Ecol. 33, 2215–2225. doi: 10.1111/1365-2435.13452
Walkley, A., and Black, I. A. (1934). An examination of the Degtjorett method for determining soil organic matter and a proposal for modification of the chromic acid titration method. Soil Sci. 37, 29–38. doi: 10.1097/00010694-193401000-00003
Yi, H., Hu, S., Zhang, Y., Wang, X., Xia, Z., Lei, Y., et al. (2023). Proper delay of phosphorus application promotes wheat growth and nutrient uptake under low phosphorus condition. Agriculture 13, 884. doi: 10.3390/agriculture13040884
Keywords: conservation agriculture, energy use efficiency, phosphorus use efficiency, productivity, quality, wheat
Citation: Kumar A, Behera UK, Dhar S, Babu S, Singh R, Upadhyay PK, Saha S, Devadas R, Kumar A, Gupta G, Singh RK, Gudade BA, Karan S and Verma G (2023) Deciphering the role of phosphorus management under conservation agriculture based wheat production system. Front. Sustain. Food Syst. 7:1235141. doi: 10.3389/fsufs.2023.1235141
Received: 28 July 2023; Accepted: 12 September 2023;
Published: 05 October 2023.
Edited by:
Matteo Balderacchi, Independent Researcher, Piacenza, ItalyReviewed by:
Gong Haiqing, China Agricultural University, ChinaApurbo Chaki, Bangladesh Agricultural Research Institute, Bangladesh
Copyright © 2023 Kumar, Behera, Dhar, Babu, Singh, Upadhyay, Saha, Devadas, Kumar, Gupta, Singh, Gudade, Karan and Verma. This is an open-access article distributed under the terms of the Creative Commons Attribution License (CC BY). The use, distribution or reproduction in other forums is permitted, provided the original author(s) and the copyright owner(s) are credited and that the original publication in this journal is cited, in accordance with accepted academic practice. No use, distribution or reproduction is permitted which does not comply with these terms.
*Correspondence: Amit Kumar, amitkumaricar13@gmail.com