Effects of short-term drought, nitrogen application and their interactions on the composition and functional genes of soil microbial communities in alfalfa grassland on the Loess Plateau
- 1State Key Laboratory of Herbage Improvement and Grassland Agro-Ecosystems, Lanzhou University, Lanzhou, China
- 2College of Pastoral Agricultural Science and Technology, Lanzhou University, Lanzhou, China
- 3Qingyang National Field Scientific Observation and Research Station of Grassland Agro-Ecosystems, Lanzhou University, Qingyang, China
- 4Management Center for Scientific Observing Stations, Lanzhou University, Lanzhou, China
- 5Institute of Soil and Water Conservation Science of Gansu Province, Lanzhou, China
- 6College of Grassland Agriculture, Northwest A&F University, Yangling, Shaanxi, China
Drought and nitrogen addition are important factors influencing soil microorganisms and changes in the soil environment. In the future, droughts will become more frequent, shorter, and more severe. However, little is known about the soil organic carbon components, enzyme activity, and composition, structure, and functional genes of soil microbial communities under short-term drought and nitrogen application conditions. In this study, we used metagenomics sequencing technology to explore the changes in the composition and functional genes of soil microbial communities under short-term drought, nitrogen application, and their interactions in the artificial grasslands of the Loess Plateau. The results indicated that (I) short-term drought, nitrogen application, and their interactions all increased the particulate organic carbon (POC) content. (II) Short-term drought increased the contents of soil microbial biomass carbon (MBC) and microbial biomass nitrogen (MBN), as well as soil enzyme activity. (III) Short-term drought significantly increased the bacterial alpha diversity, whereas the interactions of short-term drought and nitrogen application enhanced the fungal alpha diversity. (IV) The interactions of short-term drought and nitrogen application inhibited Fusarium to prevent plant diseases. (V) Short-term drought enriched the relative abundance of genes related to carbon cycling and amino acid metabolism, while nitrogen application reduced genes related to carbon cycling but enriched genes related to glycan biosynthesis and metabolism. These results clearly showed that, short-term drought altered the composition and functional genes of soil microbial communities. Our research suggests that in the event of frequent short-term droughts in the future, nitrogen addition can be considered to maintain the diversity of soil microbial communities and sustain soil carbon and nitrogen cycling.
1 Introduction
Drought is one of the most important factors affecting soil microorganisms, plants, and the soil environment (Bogati and Walczak, 2022). The emergence of extreme weather events, especially drought, puts pressure on the function and composition of soil microorganisms, reducing their activity and abundance and affecting enzyme production and nutrient cycling, thereby leading to a decrease in soil fertility and inhibition of plant growth (Geisen et al., 2019; Jansson and Hofmockel, 2020; Bogati and Walczak, 2022). Climate models predict an increase in the frequency and intensity of extreme drought weather events (Iglesias and Garrote, 2015; Spinoni et al., 2015). In arid and semi-arid areas, extreme drought events may become shorter but more severe and frequent (Ochoa-Hueso et al., 2018). Such frequent short-term drought may increase the risk of dryness and high temperatures in specific regions or seasons. Plants need sufficient water during the growing season, resulting in plant damage and reduced yields. Moreover, short-term drought may lead to changes in soil organic carbon composition and extracellular enzyme activity related to soil organic matter decomposition (Barnard et al., 2013), as well as changes in microbial communities and functions (Hueso et al., 2012; Ochoa-Hueso et al., 2018). Therefore, it is necessary to explore the impact of short-term drought on soil microbial communities and functions for agricultural development.
The impacts of drought on microorganisms are very complex and may be influenced by the frequency, intensity, and duration of drought (Kundel et al., 2020). Short-term drought is not a “weakened version” of long-term drought; it has its own unique features (Yakushev et al., 2023). At present, the assembly of microbial communities is easily affected by short-term climate change (especially drought), which may affect the participation of microorganisms in nutrient decomposition and cycling (Ochoa-Hueso et al., 2018). In particular, the structure of bacterial communities is more easily affected by short-term environmental changes (Yuste et al., 2014). Although the changes caused by extreme drought have negative impacts, they may induce the adaptation of microorganisms and plants to the environment by altering related functional genes, allowing them to continue to survive and reproduce (Sayer et al., 2021). In addition, the impacts of extreme drought on microorganisms can lead to changes in soil extracellular enzyme activity and redistribute resources through feedback mechanisms that affect carbon and nitrogen cycling in the soil (Austin et al., 2004; Barnard et al., 2013; Schimel, 2018). At present, it is not yet clear how short-term drought affects soil carbon cycling.
Nitrogen addition also has a major impact on soil properties and soil microorganisms. The application of nitrogen can be used to improve soil fertility and plant productivity and further reduce the negative effects of extreme weather events on soil fertility (Lu et al., 2015; Nkebiwe et al., 2016). However, the impacts of nitrogen addition on soil organic carbon components and extracellular enzyme activity are contradictory, and some studies have shown that soil organic carbon components and extracellular enzyme activity increase (Maaroufi et al., 2015; Jian et al., 2016), decrease (Ochoa-Hueso et al., 2018), or stay the mostly the same (Chen et al., 2015). These differences may be due to differences in ecosystem types, nitrogen fertilizer methods, and experimental times (Lu et al., 2011). Moreover, the responses of the composition and structure of soil microbial communities to nitrogen addition are inconsistent (Roberts et al., 2011). For example, the composition of microbial communities did not respond to nitrogen addition in soil (Roberts et al., 2011), or nitrogen addition led to a decrease in bacterial diversity in grassland soil (Zeng et al., 2016). Therefore, further exploration of the effects of nitrogen application on soil organic carbon components, soil enzyme activity, and soil microorganisms based on specific circumstances is necessary.
Surprisingly, the negative impact of drought on the soil can be reduced by adding nutrients. The addition of nitrogen generally improves soil fertility, which may alleviate the negative effects of drought on soil function and plant productivity. The addition of nitrogen alleviates but cannot fully offset the legacy effects of extreme weather events (Nguyena et al., 2018). The functions of soil microbial communities, as well as changes in the composition and structure of communities, importantly influence soil function (Sayer et al., 2021). However, there is currently little understanding of the effects of extreme drought events and nitrogen addition on soil microbial communities and the processes involved in carbon cycling (Nguyena et al., 2018). It is still unknown whether the impacts of short-term drought on microbial communities and carbon cycling can be regulated by adding appropriate amounts of nitrogen. Therefore, continuing to explore the interaction between drought and nitrogen application is crucial for understanding carbon cycling in agricultural systems under extreme climate conditions.
Due to limited knowledge of how short-term drought, nitrogen addition, and their interactions affect soil carbon components, soil enzyme activity, the composition of soil microbial communities and their functional genes, we designed an experiment involving short-term drought and nitrogen application on artificial alfalfa grasslands in the Loess Plateau of China. In addition, we used a metagenomic sequencing strategy to explore the changes in functional genes involved in carbon and nitrogen cycling. Therefore, in our study, we aim to address the following questions: (I) How do short-term drought and nitrogen application and their interactions affect changes in soil organic carbon components, MBC, MBN, and soil enzyme activity? (II) How do short-term drought and nitrogen application and their interactions change the composition and structure of soil microbial communities? (III) Do the relative abundances of functional genes related to carbon and nitrogen cycling change under short-term drought, nitrogen application and their interactions? (IV) Is it possible to mitigate the effects of drought on both soil microbial communities and soil functions by adding a suitable amount of nitrogen over a short period of time?
2 Materials and methods
2.1 Experimental location and design
This experiment was conducted at the National Field Scientific Observation and Research Station of Grassland Agricultural Ecosystem in Qingyang, Gansu Province, China (35°40’N, 107°51′E, altitude 1,297 m), which belongs to the hinterland on the Longdong Loess Plateau, with a warm temperate semi-arid continental monsoon climate. The average temperature is 9.4°C, and the average potential evaporation is 1,500 mm. The average annual rainfall is 527.6 mm, mainly concentrated during the growth period, with July to September accounting for approximately 60% of the annual rainfall. The research object of the experiment is the artificial grassland dominated by alfalfa, which exhibits strong regeneration and ecological adaptability. It can be harvested three times a year, every time during the early flowering period.
To explore the effects of short-term drought, nitrogen application and their interactions on soil organic carbon components, soil enzyme activity, and composition and structure of soil microbial communities, the study adopted a completely randomized block design with two factors (short-term drought and nitrogen application) and four treatments (C: blank control, P: short-term drought, N: nitrogen application, NP: nitrogen application × short-term drought), with four zones per treatment, totaling 16 experimental plots, each with an area of 4 m × 4 m (16 m2). A distance of 1 m was reserved between the plots to facilitate experimental sampling and avoid interference from human activities on adjacent experimental plots (Figure 1).
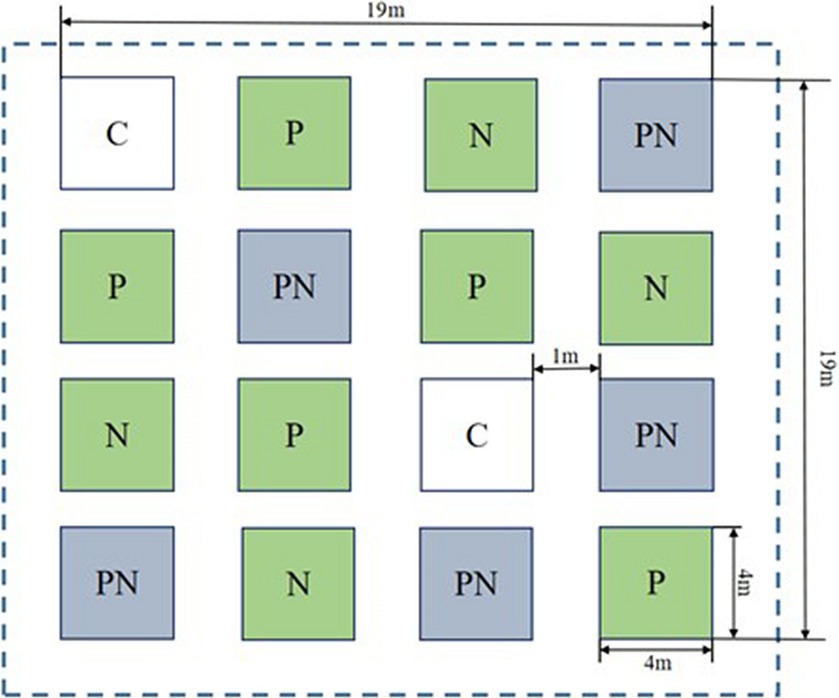
Figure 1. Design drawing of the sample plot for this experiment. (C: blank control, N: nitrogen application, P: short-term drought, NP: short-term drought × nitrogen application).
The experiment included two rainfall scenarios, natural rainfall and drought (reducing rainfall by 50%), and used canopies to achieve the drought treatment. The height of canopies from the ground was 1.30–1.60 m. Tile-shaped transparent groove plates measuring 20 cm in width were used to intercept rainwater and channel it into the rainwater collection device through PVC sinks and conduits. To eliminate the differences between the treatments caused by the light transmittance of PVC pipes in the canopies, the canopies were also placed in the area without short-term drought treatment, and the pipes were inverted to achieve the effect of natural precipitation. Trenches were dug around the sampling area and PVC boards were buried at a depth of 60 cm, exposing the surface for 10 cm to prevent soil moisture from migrating between the samples. There were two levels of nitrogen addition, namely, nitrogen application and no nitrogen application. Based on the soil nitrogen content in the Loess Plateau region, development trends, and relevant research cases, the nitrogen application rate was set at 10 g N m−2 yr.−1. Based on the nitrogen content of urea, the needed amount of urea for each experimental plot was calculated and applied evenly in the treatment area using the dry method before rainfall each year (divided into three separate times).
2.2 Soil sampling
This experiment was conducted in April 2022. The soil was sampled on 4 September 2022. After harvesting alfalfa, we randomly selected four sampling locations within each plot and collected soil cores with a 38 mm inner diameter at depths of 0–10, 10–20, 20–30, and 30–50 cm. Soil samples from the same soil layer were mixed. Four biological replicates were performed per group.
2.3 Methods for measuring soil carbon components
Method for determining total soil organic carbon (TOC): soil samples from each soil layer were ground through a 0.25 mm soil sieve under external heating (oil bath temperature, 180°C; boiling time, 5 min). Soil organic carbon was oxidized with a certain concentration of potassium dichromate and sulfuric acid solutions, the remaining potassium dichromate was titrated with ferrous sulfate, and the content of organic carbon was calculated from the amount of potassium dichromate consumed. Compared with the dry burning method, only 90% of organic carbon is oxidize using this method. Therefore, the measured organic carbon was multiplied by a correction factor of 1.1 to calculate the amount of organic carbon. This experiment used a ferrous solution of o-phenanthroline as an indicator.
Easily oxidized organic carbon (EOC) refers to the organic carbon in soil that is easily oxidized and unstable after interacting with oxidants. Based on the total organic carbon content of the soil, the amount of soil sample containing 15 mg of carbon was calculated as the weight of the sample to be tested (approximately 2.0–2.5 g of soil), and then the sample was transferred to 50 ml plastic centrifuge tubes with covers, with no soil sample added to the blank sample (three biological replicates). Then, 25 mL of potassium permanganate solution with a concentration of 333 mmol/L was added to the centrifuge tubes, the centrifuge tubes were shaken for 1 h (normal shaking was sufficient), and the tubes were centrifuged at a speed of 4,000 r/min for 5 min. The supernatant was diluted with deionized water (1:250), and the absorbance at 565 nm was measured by a spectrophotometer. The change in potassium permanganate concentration was calculated from the difference between the absorbance of the blank without soil and that of the soil sample, and then the amount of oxidized carbon could be calculated.
Particulate organic carbon (POC) refers to organic particulate matter insoluble in water, which plays an important role in the carbon cycle. Air-dried soil was passed through a 2 mm sieve to remove visible plant roots and other debris. The samples then dried overnight and stored at 4°C. Ten grams of pre-treated soil sample was weighed, put into reagent bottles, 30 mL of 5 g/L sodium hexametaphosphate solution was added, and the sample was shaken on a reciprocating oscillator for 15 h to disperse it. The dispersed solution was then passed through a 53 μm sieve and rinsed with clean water until the leachate clarified. The separated components were dried at 60°C and weighed, and their percentage in the soil was calculated.
Dissolved organic carbon (DOC) refers to the organic carbon extracted with water, dilute salt, and other extractants, followed by passage through a 0.45 μm pore filter membrane. Each layer of soil sample was ground through a 2 mm sieve, 5 g of soil sample was weighed, and the sample was transferred to 100-mL plastic bottles with covers. K2SO4 solution (0.5 mol/L) was added to complete 25 mL (soil: water ratio 1:5), and the mixture was shaken for 30 min. The mixture was left to settle for 30 min after the end of the shock. A pipette gun was then used to accurately transfer 2.5 ml of the supernatant of the original solution to 25 ml volumetric flasks (diluted to 5 ~ 15 mg/L, depending on the concentration of the original solution and diluted approximately 10 times in the Loess Plateau area). Then, the constant-volume solution was drawn into a 30 ml syringe and passed through a 0.45 μm pore filter membrane, and the absorbance of the filtered solution was measured by a UV spectrophotometer at a wavelength of 254 nm. Finally, a regression equation was established with the concentration and absorbance value to calculate the results.
2.4 Determination of MBC and MBN
The MBC was determined by weighing six fresh soil samples (passed through a 2 mm soil sieve) each to 12.5 g and placing them in small beakers. The three small beakers were then placed into a vacuum dryer, with three beakers placed at the bottom of the dryer, each containing chloroform, water, and low-concentration sodium hydroxide solution (with a small amount of glass beads in the beakers). When vacuuming, chloroform was allowed to boil vigorously for 3–5 min, the vacuum dryer valve was then closed, and the sample was placed in a dark room for 24 h. After the fumigation was completed, the dryer valve was opened, and the chloroform was removed (and placed in a fume hood). The other three portions of soil were put into another dryer, and the above operation was repeated, but chloroform was not added. All the fumigated soil samples were then transferred to 150 ml triangular flasks, 50 mL of 0.5 mol/L K2SO4 (K2SO4: water ratio 1:4) was added, the samples were shaken for 30 min and filtered. The operation for non-fumigated soil samples was the same, and blanks were made at the same time. The determination of MBN required repeating the above steps to obtain the extraction solution and then using a Kjeldahl azotometer for determination.
2.5 Enzyme activity
This experiment used the fluorescence microplate assay to measure the activity of soil enzymes (Qi et al., 2016). The enzymes selected for measurement in this experiment were β-glucosidase (Glu), β-cellobiosidase (β-CB), β-1,4-xylosidase (Xyl), leucine aminopeptidase (LAP), peroxidase (POD), and N-acetylamino glucosidase (NAG).
2.6 Experimental procedures of metagenomic sequencing
DNA was extracted from soil samples using the CTAB method. The DNA degradation degree, potential contamination and DNA concentration were measured using the Agilent 5,400 system. The sequencing library was generated using the NEBNext® Ultra™ DNA Library Prep Kit for Illumina (NEB, USA, Catalogue#: E7370L) following the manufacturer’s recommendations. Briefly, genomic DNA samples were fragmented by sonication to a size of 350 bp. Then, DNA fragments were end-polished, A-tailed, and ligated with the full-length adapter for Illumina sequencing, followed by further PCR amplification. After PCR, the products were purified by the AMPure XP system (Beckman Coulter, USA). Subsequently, library quality was assessed on the Agilent 5,400 system (Agilent, USA) and quantified by qPCR (1.5 nM). The qualified libraries were pooled and sequenced on Illumina platforms with the PE150 strategy, according to the effective library concentration and data amount needed. The raw data of bacteria, fungi, and viruses in soil samples were obtained by metagenomic sequencing using the Illumina NovaSeq high-throughput sequencing platform.
2.7 Statistical analysis
First, the experimental data were processed in Excel 2013, which included data import, cleaning, conversion and formatting to ensure the accuracy and consistency of the data. Then, statistical tests were conducted using SPSS 25.0 software, and soil carbon components, soil enzyme activity, MBC, and MBN data were analyzed using t-tests, analysis of variance, and other functions (statistical significance was determined at a level of p ≤ 0.05). Microbial data were analyzed using R software (version 3.1.1). We used the “vegdist,” “adonis” and “capscale” functions in the vegan package and used the ggplot2 package for data visualization1.
3 Results
3.1 Changes in soil organic carbon components, MBC, MBN, and pH under short-term drought and nitrogen application
The differences in soil organic carbon components among the four treatments are shown in Figure 2. There was no significant change in TOC or DOC among the four treatments. Compared with that in the C treatment, the POC in all treatments increased, with the increase in the surface layer being the most significant (p < 0.05). The NP treatment had the highest POC content. In the four treatments, the contents of EOC in the 0–10 and 10–20 cm soil layers showed significant changes (p < 0.05). Compared with that in the C treatment, the content of EOC in the N treatment increased.
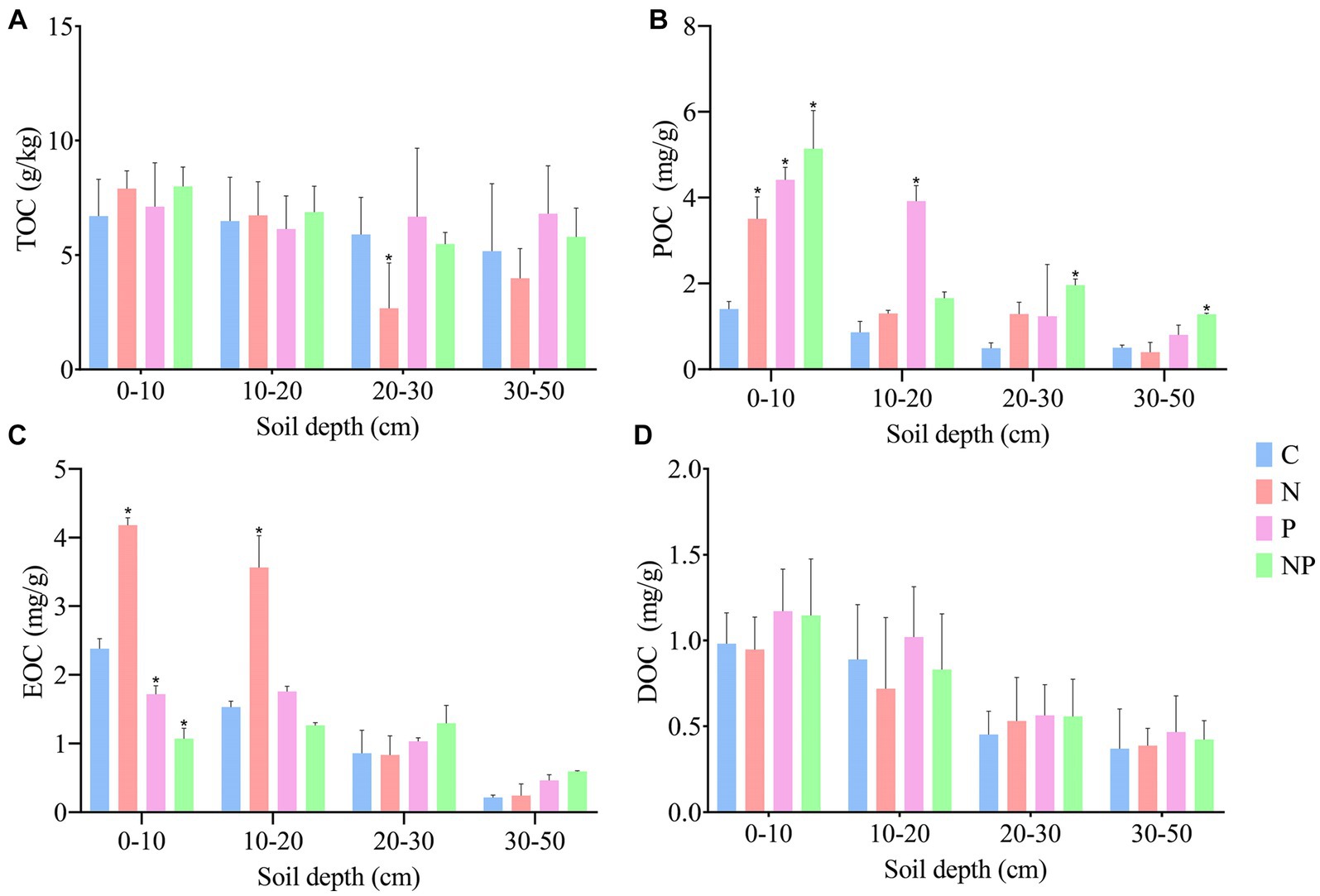
Figure 2. Differences in the contents of soil organic carbon components in C (blank control), N (nitrogen application), P (short-term drought), NP (short-term drought × nitrogen application), (A) TOC content, (B) POC content, (C) EOC content, and (D) DOC content. (*) Represents significant differences between treatments (p < 0.05).
Compared with that in the C treatment, the contents of MBC and MBN in the N treatment, P treatment and NP treatment were slightly increased. Moreover, the increase in MBC and MBN in the P treatment was higher than that in the other treatments (Figure 3). The minimum MBC and MBN values were 5.80 and 2.19 mg/kg, respectively, and the maximum MBC and MBN values were 139.78 and 20.67 mg/kg, respectively.
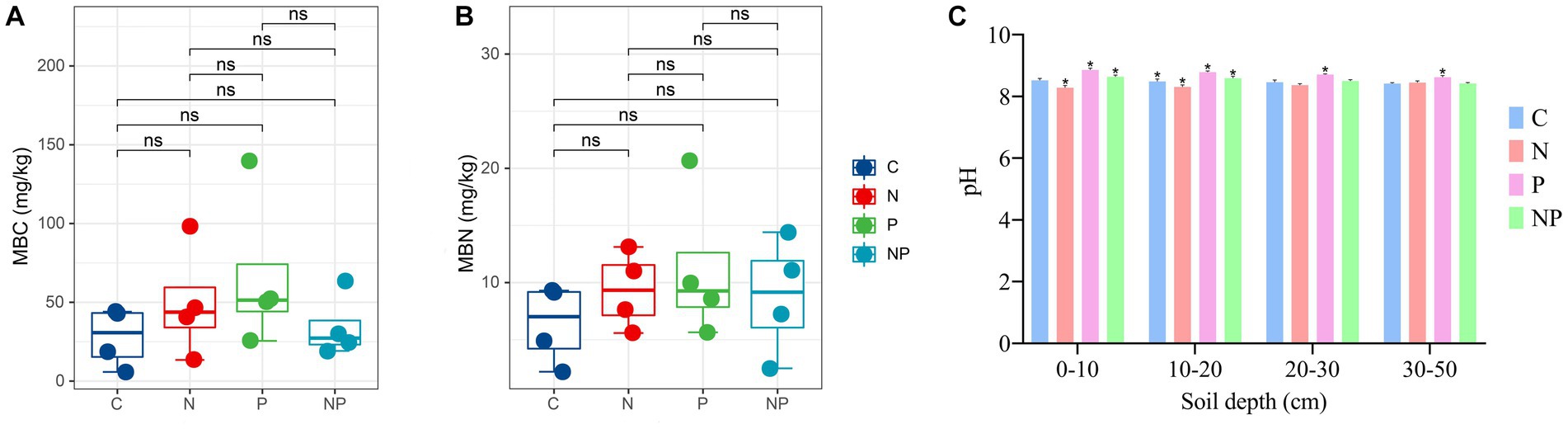
Figure 3. The contents of MBC, MBN, and soil pH in C (blank control), N (nitrogen application), P (short-term drought), and NP (short-term drought × nitrogen application), (A) MBC content, (B) MBN content, (C) pH value. (*) Represents significant differences between treatments (p < 0.05).
The pH values of the four treatments were similar, but compared with the C treatment, the N treatment showed a downwards trend, the P treatment showed an upwards trend, and the NP treatment was similar to the C treatment (Figure 3).
3.2 Short-term drought stimulates enzyme activity
The soil enzyme activities in the four treatments are shown in Figure 4. Compared with those in the C treatment, the activities of Xyl, Glu, and LAP increased in the P and NP treatments but were not different in the N treatment. The activities of POD, NAG and β-CB did not change significantly among the four treatments.
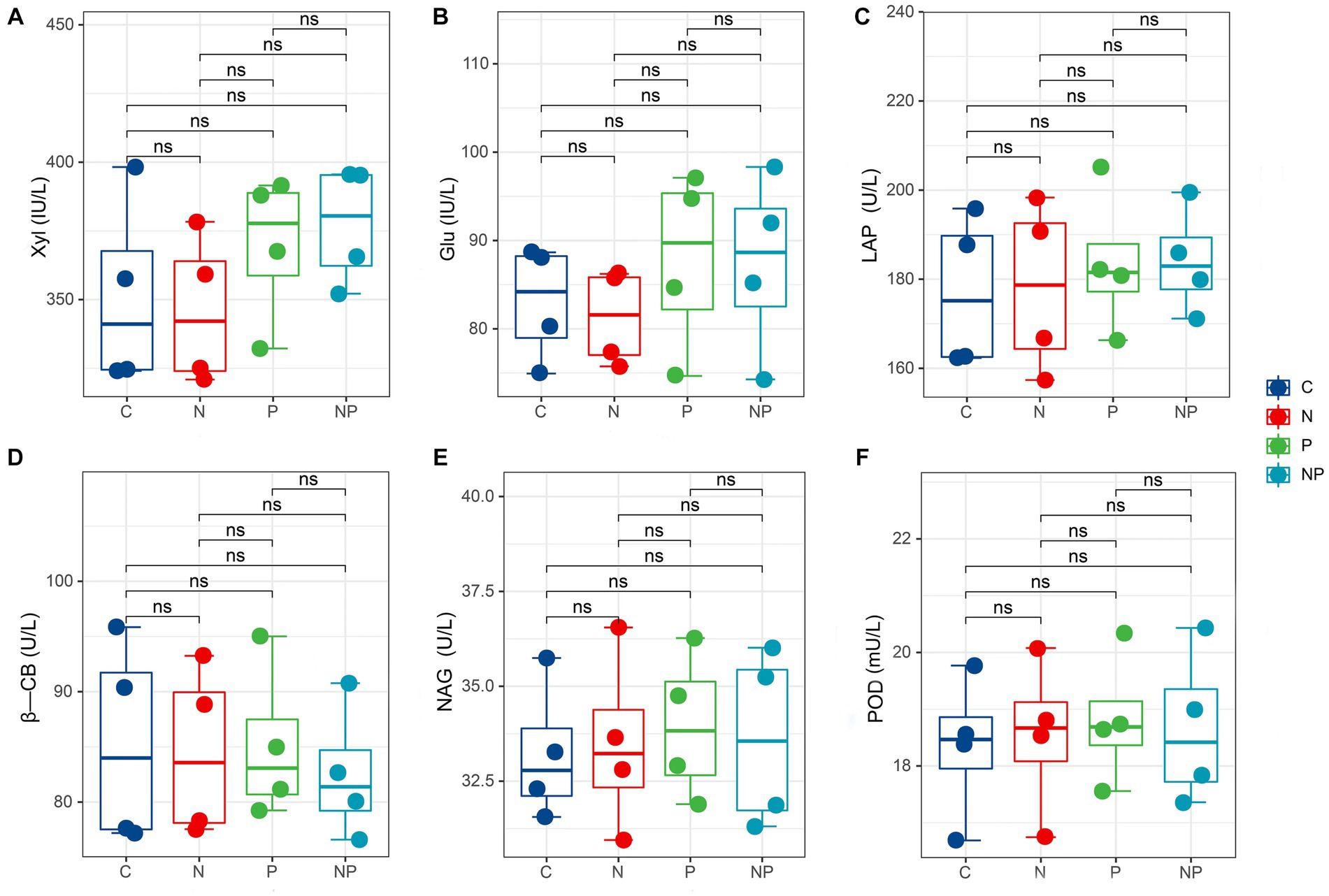
Figure 4. Box plots show the activities of soil enzymes among C (blank control), N (nitrogen application), P (short-term drought), NP (short-term drought × nitrogen application). (A) Xyl activity, (B) Glu activity, (C) LAP activity, (D) β-CB activity, (E) NAG activity, (F) POD activity.
3.3 Changes in the diversity of soil microbial communities under short-term drought and nitrogen application
We used Venn diagrams (Figure 5) to show the numbers of common and unique species in the four treatments. Compared to the C treatment, the numbers of operational taxonomic units (OTUs) of fungi and bacteria were increased in the other three treatments. Bacterial OTU analysis showed that all treatments shared 1,399 OTUs, among which the P treatment had the highest number of bacterial OTUs (2,485). Fungal OTU analysis showed that all treatments shared 88 OTUs, among which the NP treatment had the highest number of fungal OTUs (212). This indicated that the fungal community diversity of the NP treatment was higher, while the bacterial community diversity of the P treatment was higher. As shown in Figure 6, the P treatment significantly enhanced bacterial alpha diversity (Chao1, Ace, and Shannon) (p < 0.05). However, the NP treatment increased fungal alpha diversity (Ace and Shannon), while the N treatment had almost no effect on bacterial or fungal alpha diversity.
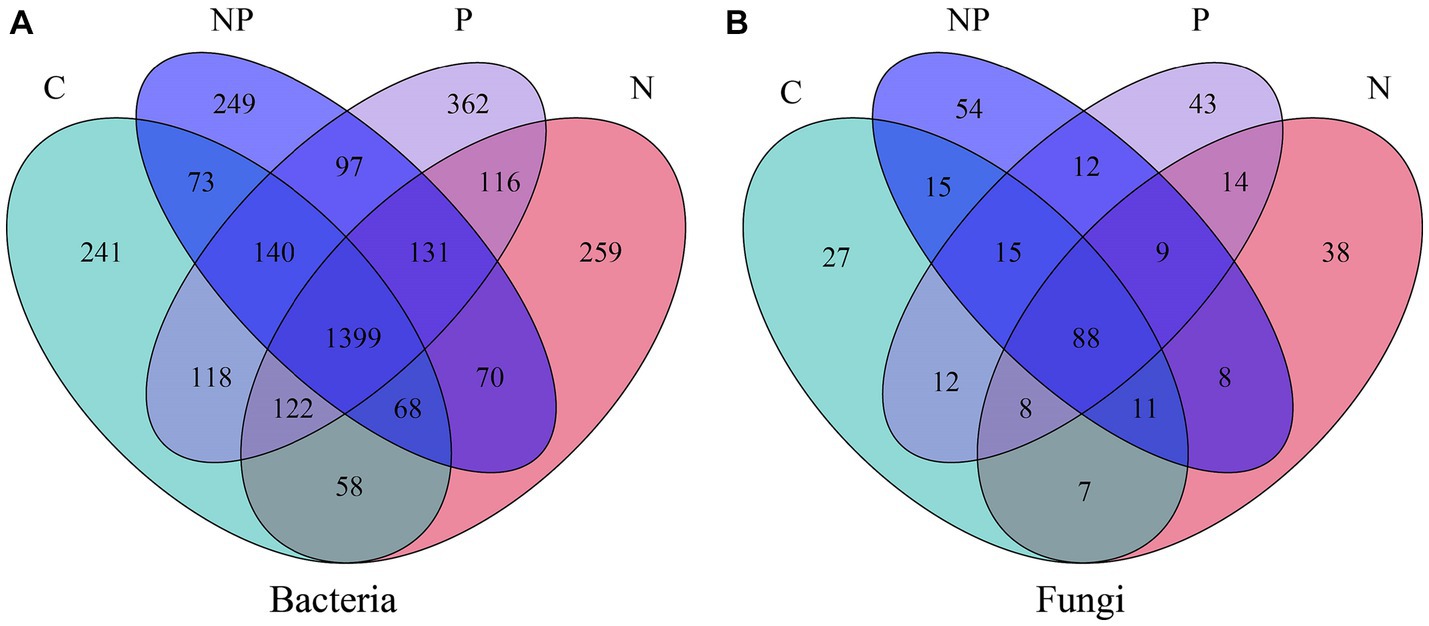
Figure 5. Venn diagrams of the unique and shared operational taxonomic units (OTUs) between C (blank control), N (nitrogen application), P (short-term drought), NP (short-term drought × nitrogen application). (A) Venn diagrams of bacterial OTUs. (B) Venn diagrams of fungal OTUs.
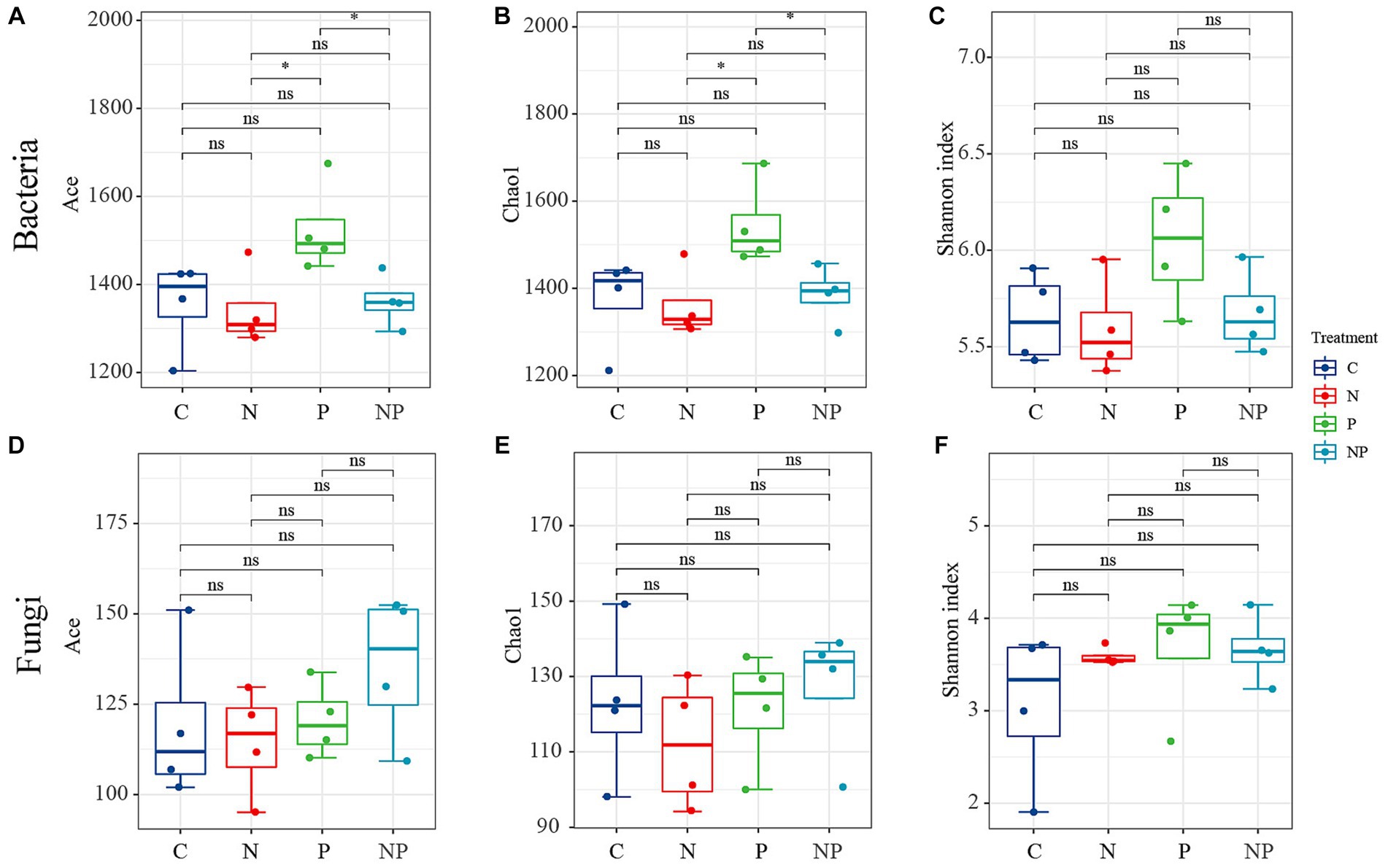
Figure 6. Box plots of bacteria and fungi alpha diversities among the four treatments, (*) represents significant differences between treatments (p < 0.05). (C: blank control, N: nitrogen application, P: short-term drought, NP: short-term drought × nitrogen application.) (A) ACE index in bacterial alpha diversity, (B) Chao1 index in bacterial alpha diversity, (C) Shannon index in bacterial alpha diversity, (D) ACE index in fungi alpha diversity, (E) Chao1 index in fungi alpha diversity, (F) Shannon index in fungi alpha diversity.
3.4 Changes in the composition and structure of soil microbial communities under short-term drought and nitrogen application
The relative abundances of the top 10 bacterial and fungal phyla and genera among the four treatments are shown in Figure 7. At the bacterial phylum level, Proteobacteria accounted for the highest proportion of bacterial communities in all treatments, but the proportion of Proteobacteria remained almost unchanged among the four treatments. The other two dominant bacterial phyla among the four treatments were Actinobacteria and Firmicutes. The proportions of Actinobacteria in the C, N, P, and NP treatments were 24.8, 24.18, 29.99, and 27.38%, respectively. The proportions of Firmicutes in the C, N, P, and NP treatments were 1.32, 1.51, 2.05, and 1.73%, respectively. Compared with those in the C treatment, the proportions of Actinobacteria and Firmicutes in the P and NP treatments increased, while there was no significant change in the N treatment. In addition, the proportion of Cyanobacteria in the NP treatment was higher than that in the other three treatments. At the fungal phylum level, the top three dominant fungal communities among the four treatments were Ascomycota, Mucoromycota, and Basidiomycota. Their proportions were 52.95, 9.9, 2.07% in the C treatment, 49.4, 6.38, 4.6% in the N treatment, 50.87, 19.39, 3.76% in the P treatment, and 53.86, 13.08, 2.35% in the NP treatment, respectively. Compared with that in the C treatment, the proportions of Mucoromycota in the P and NP treatments increased, while those in the N treatment decreased, and the proportions of Basidiomycota in the N, P, and NP treatments increased.
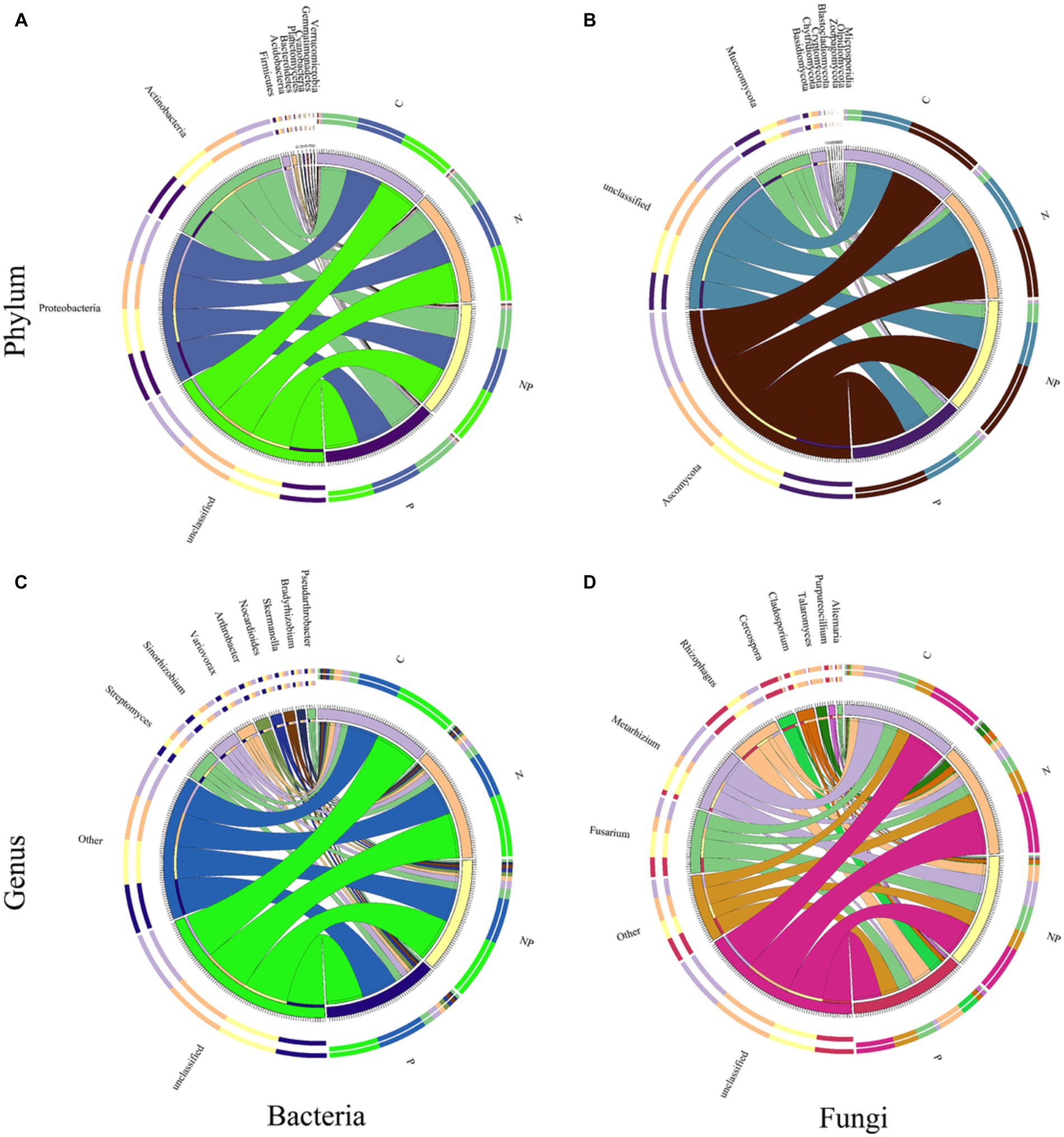
Figure 7. The relative abundances of dominant bacterial and fungal communities at the phylum and genus levels in C: blank control, N: nitrogen application, P: short-term drought and NP: short-term drought × nitrogen application. (A,C) The relative abundances of the top 10 bacterial phyla and genera among the four treatments. (B,D) The relative abundances of the top 10 fungal phyla and genera among the four treatments.
At the genus level, in terms of the composition and structure of bacterial communities, there was no significant change in the relative abundance of the top 10 species under the N, P, and NP treatments compared to the C treatment. At the fungal genus level, the top four dominant fungal communities in the four treatments were Metarhizium, Fusarium, Rhizophagus, and Cladosporium, and their proportions were 24.57, 13.45, 8.6, and 0.99% in the C treatment, 8.86, 10.26, 5.29, and 6.91% in the N treatment, 34.5, 17.74, 18.44, and 3.96% in the P treatment, and 17.7, 13.44, 11.16, and 3.28% in the NP treatment, respectively. Compared with that in the C treatment, the proportion of Metarhizium in the P treatment increased, while that in the N and NP treatments decreased. The proportion of Rhizophagus in the P and NP treatments increased, while that in the N treatments decreased. The proportions of Cladosporium in the N, P, and NP treatments increased. Furthermore, it is worth noting that Purpureocillium accounted for 0.58% of the community in the C treatment and 2.94% in the P treatment.
3.5 Changes in functional genes under short-term drought and nitrogen application
The metagenomic reads were annotated based on the KEGG database (Figure 8). The P treatment increased the relative abundances of pathway genes related to the carbon cycle and amino acid metabolism. The N treatment decreased the abundances of pathway genes related to the carbon cycle, such as starch and sucrose metabolism, inositol phosphate metabolism, pentose phosphate pathway, pentose and glucuronic acid transformation, ascorbate and aldarate metabolism, but increased the relative abundances of genes related to glycan biosynthesis and metabolism, such as glycosphingolipid biosynthesis-globo and isoglobo series, other glycan degradation, lipopolysaccharide biosynthesis, glycosaminoglycan degradation, and various types of N-glycan biosynthesis. Moreover, N treatment increased the abundances of genes related to the nitrogen cycle. There were no significant changes in the NP treatment.
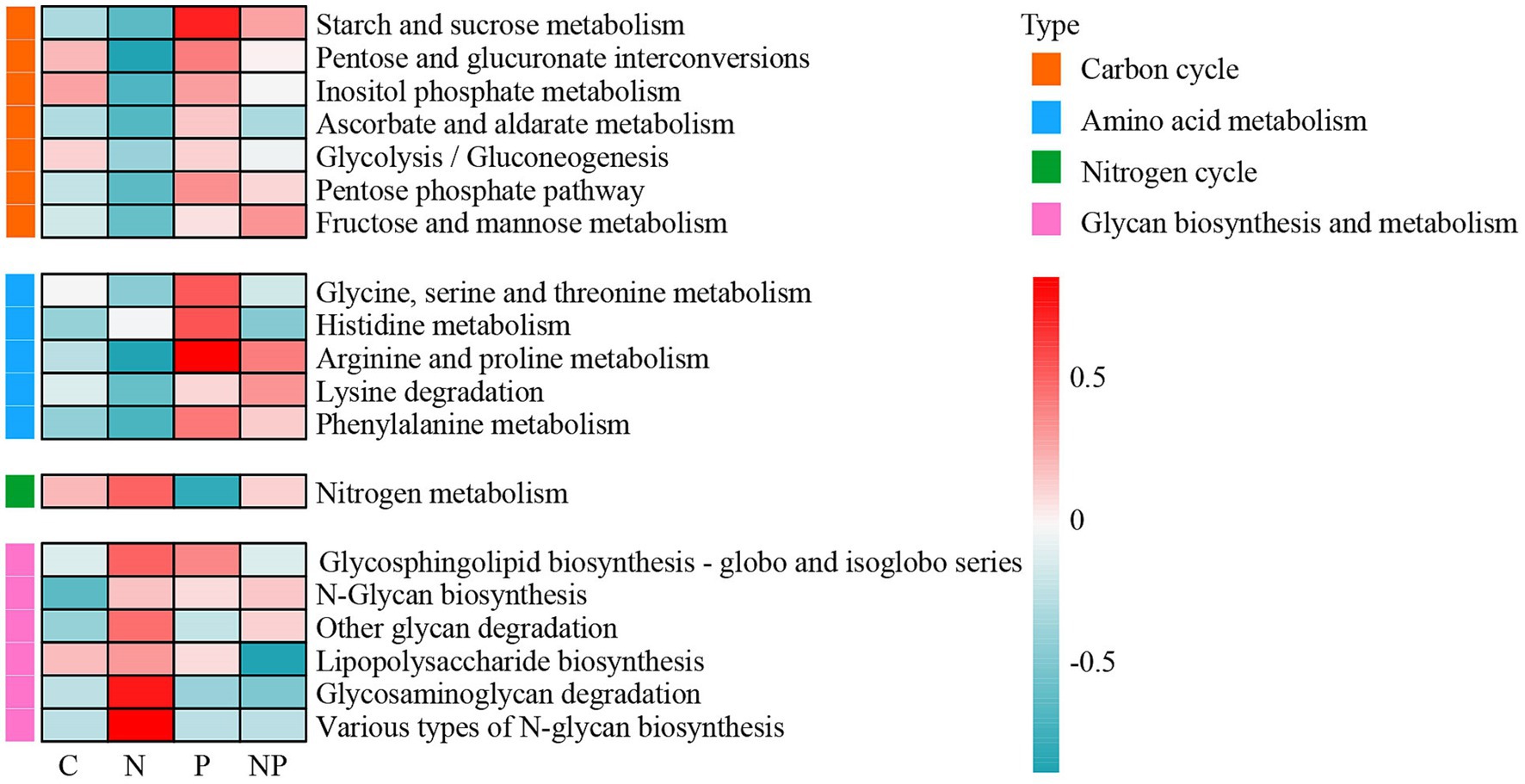
Figure 8. Heatmaps of the relative abundances of functional genes related to the carbon and nitrogen cycles for all treatments based on KEGG database. (C: blank control, N: nitrogen application, P: short-term drought, NP: short-term drought × nitrogen application). The blue and red colors indicate abundances from low to high, respectively.
4 Discussion
4.1 Effects on soil physicochemical properties and soil enzyme activities
Soil is a large carbon pool and its carbon content is three times that of the atmosphere (Lal, 2004). The amount of soil organic carbon directly affects soil quality and productivity (Liu et al., 2014) and reflects the relationship between input and decomposition rates, but it is difficult to detect changes under short-term climate change (Yang et al., 2005). Recently, many studies have found that unstable organic carbon, such as POC, DOC, EOC, and MBC, is a more sensitive and useful indicator (Plaza-Bonilla et al., 2014). In this study, we found that the N, P and NP treatments increased POC (Figure 2). The short-term droughts led to an increase in POC, which may be due to POC being mainly imported from fresh plant stubble and the return of litter and dead fine roots. Nitrogen application also increased POC, possibly due to nitrogen enrichment promoting the accumulation of soil POC by increasing large aggregate carbon and causing acidification of the soil (Wang et al., 2020).
Both the N and P treatments increased the soil MBC and MBN (Figure 3). Nitrogen application increased soil MBC and MBN, which may be because appropriate nitrogen application can meet the nitrogen demand of soil animal, plant and microbial life activities and stimulate soil microbial activities (Zhu et al., 2021). At the same time, the sufficient supply of soil nutrients after nitrogen application alleviates the competition between plants and soil microorganisms, thus promoting the accumulation of MBC and MBN (Zhu et al., 2021). We also found that short-term drought increased soil microbial biomass carbon and nitrogen, which may be caused by the fact that under drought conditions, plant growth is inhibited, and a large number of plants dry and fall to the ground. The nutrient competition between plants and soil microorganisms decreases, and the litter input into the soil is decomposed by microorganisms, which further promotes the increase in microbial biomass (Zhang et al., 2017; Li et al., 2024).
In our study, soil enzyme activity was increased under short-term drought treatment (Figure 4), which may be due to the accumulation of enzymes and their reaction substrates under low moisture conditions, thus driving enzyme reactions under more arid conditions (Austin et al., 2004). The increase in soil enzyme activity may also be related to reduced competition between plants and microorganisms for soil resources and nutrients under drought conditions (Schwinning and Sala, 2004). Usually, the water limitation threshold of microbes is much lower than that of plants (Schwinning and Sala, 2004; Delgado-Baquerizo et al., 2013). Higher enzyme activity may be associated with higher organic matter input and rhizosphere activity, while drought can lead to root death and leaf shedding, resulting in more input of additional organic matter into the soil (Ogaya and Peñuelas, 2004; Sinsabaugh et al., 2008) and thus enhancing soil enzyme activity. The activity of Glu and Xyl increased under short-term drought conditions because cellulose accounts for 40% of plant tissue, and drought may increase the input of cellulose to soil, ultimately increasing Glu activity (Toberman et al., 2010). The LAP activity in the P treatment also increased, which may be due to the short-term drought increasing the nitrogen requirements of soil microorganisms and limiting their nutrient metabolism (Xiao et al., 2022).
4.2 Short-term drought increases bacterial diversity, while its interaction with nitrogen application increases fungal diversity
Under short-term drought conditions, more dead plants drop their branches, resulting in higher soil organic matter (Ogaya and Peñuelas, 2004). In this study, alfalfa, a leguminous plant rich in nutrients such as amino acids, sugars, and flavonoids, was more conducive to bacterial growth and development, resulting in the increased species diversity of bacteria in the P treatment (Isobe et al., 2001; Figures 5, 6). Moreover, the input of organic matter also provides more carbon for bacterial growth and promotes an increase in bacterial diversity (Liao et al., 2021). Similarly, we found that short-term drought resulted in an increase in the alpha diversity of soil bacteria, possibly due to the decrease in pore connectivity caused by low water content, and dry pore spaces further created isolated habitats and niches that could accommodate less competitive bacteria. Compared with bacteria, fungi are less restricted under short-term drought, which may be related to their mycelium system connecting through dry pore spaces (Tecon and Or, 2017). Compared with the C treatment, the change in microbial species diversity in the N treatment was minimal (Figures 5, 6), revealing that the overall response of the microbial communities was not sensitive to nitrogen addition (McHugh et al., 2017). Generally, nitrogen-induced soil acidification is an important mechanism for altering the composition of bacterial communities (Zhang et al., 2017). However, our experimental site was located on the Loess Plateau, and as nitrogen application can only cause a slight decrease in soil pH, the soil remained alkaline (Liu et al., 2015; Figure 3C). In addition, we also found that the fungal species diversity increased under the NP treatment (Figures 5, 6), indicating that under short-term drought, nitrogen application may increase fungal diversity. This is mainly because, the fungal communities were more abundant and diverse in the area with less rainfall, and adding an appropriate amount of nitrogen fertilizer can further provide nutrients for fungi (Classen et al., 2015; Kaisermann et al., 2015).
4.3 Impact on the composition and structure of soil microbial communities
Drought is a major climatic driver of soil microbial community composition and structure (Maestre et al., 2015). Our study showed that the microbial community composition and structure were highly susceptible to short-term drought, which was consistent with a previous study (Ochoa-Hueso et al., 2018). This may affect microbiome-mediated soil nutrient cycling, with implications for ecosystem function. The common explanation for changes in microbial composition is niche selection, where the relative abundance of drought-tolerant taxa increases compared to drought-sensitive taxa (Figure 7). Over time, the entire soil microbial community can develop drought resistance, which may lead to an increase in microbial activity rates at low soil moisture (Goransson et al., 2013). In the P treatments, the relative abundance of Actinobacteria and Firmicutes increased, and Actinobacteria typically exhibited drought resistance. Actinobacteria decompose organic matter in soil, such as chitin and cellulose, and have strong resistance to dry and low-resource conditions, which may explain why they outperform other microorganisms under drought conditions. The majority of Firmicutes are gram-positive bacteria, which possess thick cell walls (Aislabie et al., 2009) and are able to form spores under extreme conditions (Singh et al., 2007), allowing them to survive under drought conditions. Compared to that in the other treatments, the relative abundance of Cyanobacteria increased in the NP treatment, this is mainly because Cyanobacteria had strong stress resistance and drought tolerance, when nitrogen was added it increased the response ratio of the relative abundance of blue–green algae (She et al., 2018).
Compared to Bacteria, at the phylum level, the number of Mucoromycota treated with P and NP increased, while the number of Mucoromycota treated with N decreased (Figure 7). This may be due to the evolution of three life forms in Mucoromycota: saprotrophic, mycorrhizal, and parasitic. The saprophytic form survives by obtaining nutrients via the breakdown of dead and decaying organic matter. Short-term drought increased the number of dead plants in the soil, providing more organic matter for mainly saprophytic microbes. The addition of nitrogen may inhibit the activity of saprophytic fungi, which also causes the relative abundance of Mucoromycota treated with NP to be lower than that treated with P (Wen et al., 2018). Basidiomycetes can tolerate drought and may be superior to other taxa in water-scarce environments due to their advanced osmoregulatory functions and strong cell walls that allow them to resist limiting conditions. Under nitrogen application conditions, the relative abundance of Basidiomycetes increased, which may be due to the provision of nutrients for Basidiomycetes (Bogati and Walczak, 2022).
In this study, changes at the fungal genus level were also observed. The relative abundance of Fusarium decreased under nitrogen application but increased under drought treatment. Fusarium includes plant pathogens of many important crops, many of them are soil-borne, and their accumulation in the soil may exacerbate crop diseases (Li et al., 2022). Drought is one of the factors that increases the incidence rate and severity of Fusarium-induced diseases. The relative abundance of Purpureocillium in the P treatment also increased compared with that in the other three treatments. Purpureocillium has an inhibitory effect on a variety of plant pathogenic bacteria and viruses and can reduce Fusarium abundance. The increase in the relative abundance of Fusarium under drought conditions also promotes the increase in Purpureocillium, which may be due to the self-regulation of the microbial community (Hao et al., 2018). However, nitrogen application reduced the relative abundance of Fusarium in soil. According to the theory of hypotrophic replicative nutrition, the tendency of Fusarium to be hypotrophic is negatively correlated with the content of inorganic nitrogen (Yang et al., 2019). In our study, we found no change in the relative abundance of Fusarium in the NP treatment compared to the C treatment (Figure 7). This suggests a potential strategy: appropriate nitrogen application in times of drought may inhibit Fusarium and contribute to plant protection.
The relative abundance of Metarhizium increased under drought treatment, decreased under nitrogen treatment, and remained unchanged under NP treatment. Metarhizium is a broad-spectrum insect pathogen. According to statistics, Metarhizium is the host of more than 200 insects, can cause insect disease, and can cause repeated infections within populations. At present, Metarhizium has been developed into a fungal insecticide second only to Beauveria bassiana (Zimmermann, 2007). Drought, pests and diseases, and pathogen-related damage often occur at the same time, and additional stress from climate change and drought may make plants more vulnerable to pests and pathogens. The increase in Metarhizium content under drought conditions may indicate an increase in the soil pest population, and the decrease in Metarhizium content under nitrogen application conditions may indicate a decrease in the soil pest population. Therefore, appropriate nitrogen application during drought may inhibit the occurrence of pests.
4.4 Short-term drought enriches pathway genes related to the carbon cycle and amino acid metabolism
The crop planted in the experimental site was alfalfa, which contains a large amount of protein and amino acids and is a high-quality forage. Short-term drought caused alfalfa litter to enter the soil, and the litter was decomposed by microorganisms, which promoted the increase in genes related to amino acid metabolism. At this time, microorganisms can easily obtain nutrients, further promoting the soil carbon cycle (Figure 8). Moreover, plant litter is the main source of soil organic carbon and has a positive effect on microbial carbon cycling genes (Zhong et al., 2018). The input of plant litter to soil stimulated the enrichment of soil microbial carbon cycling genes. The decrease in the abundance of pathway genes associated with the carbon cycle after nitrogen treatment may be due to the addition of nitrogen inhibiting the activity of saprophytic fungi, which produce enzymes to decompose organic matter that is difficult to degrade, which may not be conducive to carbon decomposition, resulting in a decrease in the abundance of pathway genes associated with the carbon cycle. However, the addition of nitrogen reduced the decomposition rate of plant litter, and the microbial communities under nitrogen treatment utilized sugar as a carbon source to maintain activity (Zhang et al., 2016; Wen et al., 2018; Wang et al., 2020). Thus, nitrogen fertilization enriched the abundance of genes associated with glycan biosynthesis and metabolism. In addition, appropriate nitrogen application may also promote an increase in the abundance of nitrogen cycling-related genes in soil, which is conducive to the nitrogen cycle (Figure 8).
5 Conclusion
The soil organic carbon components, soil enzyme activity, and composition and functional genes of the soil microbial community of alfalfa artificial grassland on the Loess Plateau show different responses to short-term drought, nitrogen application and their interactions. Short-term drought, nitrogen application and their interactions all increased soil POC, while only nitrogen application increased the soil EOC content. Short-term drought increased soil MBC and MBN and promoted an increase in soil enzyme activity. Additionally, short-term drought increased the alpha diversity of soil bacteria, and the interaction between short-term drought and nitrogen application increased the alpha diversity of soil fungi. Short-term drought stress also increased the relative abundance of drought-resistant bacteria such as Actinobacteria and Firmicutes. During short-term drought, moderate nitrogen application reduced the relative abundance of the Fusarium and Metarhizium genera, which may prevent plant diseases during droughts and pest outbreaks. Short-term drought enriched the abundance of genes related to the carbon cycle and amino acid metabolism, while nitrogen application decreased the abundance of genes related to the carbon cycle and enriched the abundance of genes related to glycan biosynthesis and metabolism and the nitrogen cycle. Overall, short-term drought altered the composition and functional genes of soil microbial communities. Moreover, in the case of frequent short-term drought in the future, nitrogen addition can be considered to maintain the diversity of the soil microbial community and sustain soil carbon cycling.
Data availability statement
The datasets presented in this study can be found in online repositories. The names of the repository/repositories and accession number(s) can be found at: NCBI BioProject, accession number PRJNA1046644, http://www.ncbi.nlm.nih.gov/bioproject/1046644.
Author contributions
RW: Conceptualization, Data curation, Formal analysis, Investigation, Methodology, Project administration, Resources, Software, Writing – original draft, Writing – review & editing. JGZ: Data curation, Software, Writing – review & editing. TM: Resources, Supervision, Writing – review & editing. WL: Funding acquisition, Supervision, Writing – review & editing. YS: Project administration, Visualization, Writing – review & editing. QY: Methodology, Resources, Writing – review & editing. XW: Project administration, Writing – review & editing. JL: Formal analysis, Methodology, Writing – review & editing. QX: Formal analysis, Methodology, Writing – review & editing. LL: Formal analysis, Methodology, Writing – review & editing. JJZ: Data curation, Formal analysis, Writing – review & editing. JM: Conceptualization, Funding acquisition, Investigation, Writing – review & editing. ZZ: Analyzed data and created figures.
Funding
The author(s) declare financial support was received for the research, authorship, and/or publication of this article. This research was supported by grants from National Natural Science Foundation of China (NSFC, 32371969, 32001403), the Science and Technology Planning Project of Gansu Province (Basic Research Program, grant number 23JRRA1039, and Technology Innovation Guidance Program, grant number 20CX9NA105), and the Soil and Water Conservation Compensation Fee Project of Gansu Province in 2023 (Gan Shui Bao Fa [2022] No. 467).
Acknowledgments
The authors wish to thank the editors and reviewers for their valuable comments and suggestions.
Conflict of interest
The authors declare that the research was conducted in the absence of any commercial or financial relationships that could be construed as a potential conflict of interest.
Publisher’s note
All claims expressed in this article are solely those of the authors and do not necessarily represent those of their affiliated organizations, or those of the publisher, the editors and the reviewers. Any product that may be evaluated in this article, or claim that may be made by its manufacturer, is not guaranteed or endorsed by the publisher.
Footnotes
References
Aislabie, J., Jordan, S., Ayton, J., Klassen, J. L., Barker, G. M., and Turner, S. (2009). Bacterial diversity associated with ornithogenic soil of the Ross Sea region, Antarctica. Can. J. Microbiol. 55, 21–36. doi: 10.1139/W08-126
Austin, A. T., Yahdjian, L., Stark, J. M., Belnap, J., Porporato, A., Norton, U., et al. (2004). Water pulses and biogeochemical cycles in arid and semiarid ecosystems. Oecologia 141, 221–235. doi: 10.1007/s00442-004-1519-1
Barnard, R. L., Osborne, C. A., and Firestone, M. K. (2013). Responses of soil bacterial and fungal communities to extreme desiccation and rewetting. ISME J. 7, 2229–2241. doi: 10.1038/ismej.2013.104
Bogati, K., and Walczak, M. (2022). The impact of drought stress on soil microbial community, enzyme activities and plants. Agronomy 12:189. doi: 10.3390/agronomy12010189
Chen, H., Li, D. J., Gurmesa, G. A., Yu, G. R., Li, L. H., Zhang, W., et al. (2015). Effects of nitrogen deposition on carbon cycle in terrestrial ecosystems of China: a meta-analysis. Environ. Pollut. 206, 352–360. doi: 10.1016/j.envpol.2015.07.033
Classen, A. T., Sundqvist, M. K., Henning, J. A., Newman, G. S., Moore, J. A. M., Cregger, M. A., et al. (2015). Direct and indirect effects of climate change on soil microbial and soil microbial-plant interactions: what lies ahead? Ecosphere 6, 1–21. doi: 10.1890/ES15-00217.1
Delgado-Baquerizo, M., Maestre, F. T., Rodrıguez, J. G. P., and Gallardo, A. (2013). Biological soil crusts promote N accumulation in response to dew events in dryland soils. Soil Biol. Biochem. 62, 22–27. doi: 10.1016/j.soilbio.2013.02.015
Geisen, S., Wall, D. H., and van der Putten, W. H. (2019). Challenges and opportunities for soil biodiversity in the Anthropocene. Curr. Biol. 29, R1036–R1044. doi: 10.1016/j.cub.2019.08.007
Goransson, H., Godbold, D. L., Jones, D. L., and Rousk, J. (2013). Bacterial growth and respiration responses upon rewetting dry forest soils: impact of drought-legacy. Soil Biol. Biochem. 57, 477–486. doi: 10.1016/j.soilbio.2012.08.031
Hao, Z. T., Hao, X. H., Yan, J., Xie, B. Y., and Liang, L. Q. (2018). Research progress on the secondary metabolite of Purpureocillium lilacinum. Biol. Bull. 34, 11–17. doi: 10.13560/j.cnki.biotech.bull.1985.2018-0049
Hueso, S., García, C., and Hernández, T. (2012). Severe drought conditions modify the microbial community structure, size and activity in amended and unamended soils. Soil Biol. Biochem. 50, 167–173. doi: 10.1016/j.soilbio.2012.03.026
Iglesias, A., and Garrote, L. (2015). Adaptation strategies for agricultural water management under climate change in Europe. Agric. Water Manage. 155, 113–124. doi: 10.1016/j.agwat.2015.03.014
Isobe, K., Tateishi, A., Nomura, K., Inoue, H., and Tsuboki, Y. (2001). Flavonoids in the extract and exudate of the roots of leguminous crops. Plant Prod. Sci. 4, 278–279. doi: 10.1626/pps.4.278
Jansson, J. K., and Hofmockel, K. S. (2020). Soil microbiomes and climate change. Nat. Rev. Microbiol. 18, 35–46. doi: 10.1038/s41579-019-0265-7
Jian, S. Y., Li, J. W., Chen, J., Wang, G. S., Mayes, M. A., Dzantor, K. E., et al. (2016). Soil extracellular enzyme activities, soil carbon and nitrogen storage under nitrogen fertilization: a meta-analysis. Soil Biol. Biochem. 101, 32–43. doi: 10.1016/j.soilbio.2016.07.003
Kaisermann, A., Maron, P. A., Beaumelle, L., and Lata, J. C. (2015). Fungal communities are more sensitive indicators to non-extreme soil moisture variations than bacterial communities. Appl. Soil Ecol. 86, 158–164. doi: 10.1016/j.apsoil.2014.10.009
Kundel, D., Bodenhausen, N., Jørgensen, H. B., Truu, J., Birkhofer, K., Hedlund, K., et al. (2020). Effects of simulated drought on biological soil quality, microbial diversity and yields under long-term conventional and organic agriculture. FEMS Microbiol. Ecol. 96, fiaa205. doi: 10.1093/femsec/fiaa205
Lal, R. (2004). Soil carbon sequestration impacts on global climate change and food security. Science 304, 1623–1627. doi: 10.1126/science.1097396
Li, Y., Li, Y., Zhang, Q. P., Xu, G., Liang, G. P., Kim, D., et al. (2024). Enhancing soil carbon and nitrogen through grassland conversion from degraded croplands in China: assessing magnitudes and identifying key drivers of phosphorus reduction. Soil Tillage Res. 236:105943. doi: 10.1016/j.still.2023.105943
Li, Q., Wu, Q., Zhang, T., Xiang, P., Bao, Z. J., Tu, W. Y., et al. (2022). Phosphate mining activities affect crop rhizosphere fungal communities. Sci. Total Environ. 838:156196. doi: 10.1016/j.scitotenv.2022.156196
Liao, L. R., Wang, X. T., Wang, J., Liu, G. B., and Zhang, C. (2021). Nitrogen fertilization increases fungal diversity and abundance of saprotrophs while reducing nitrogen fixation potential in a semiarid grassland. Plant Soil 465, 515–532. doi: 10.1007/s11104-021-05012-w
Liu, J. B., Fa, K. Y., Zhang, Y. Q., Wu, B., Qin, S. G., and Jia, X. (2015). Abiotic CO2 uptake from the atmosphere by semiarid desert soil and its partitioning into soil phases. Geophys. Res. Lett. 42, 5779–5785. doi: 10.1002/2015GL064689
Liu, E., Teclemariam, S. G., Yan, C., Yu, J., Gu, R., Liu, S., et al. (2014). Long-term effects of no-tillage management practice on soil organic carbon and its fractions in the northern China. Geoderma 213, 379–384. doi: 10.1016/j.geoderma.2013.08.021
Lu, Y. H., Sun, Y. T., Liao, Y. L., Nie, J., Xie, J., Yang, Z., et al. (2015). Effects of the application of controlled release nitrogen fertilizer on rapeseed yield, agronomic characters and soil fertility. J. Agric. Sci. Technol. 16, 1216–1221. doi: 10.16175/j.cnki.1009-4229.2015.06.031
Lu, M., Zhou, X. H., Luo, Y. Q., Yang, Y. H., Fang, C. M., Chen, J. K., et al. (2011). Minor stimulation of soil carbon storage by nitrogen addition: a meta-analysis. Agric. Ecosyst. Environ. 140, 234–244. doi: 10.1016/j.agee.2010.12.010
Maaroufi, N. I., Nordin, A., Hasselquist, N. J., Bach, L. H., Palmqvist, K., and Gundale, M. J. (2015). Anthropogenic nitrogen deposition enhances carbon sequestration in boreal soils. Glob. Chang. Biol. 21, 3169–3180. doi: 10.1111/gcb.12904
Maestre, F. T., Delgado-Baquerizo, M., Jeffries, T. C., Eldridge, D. J., Ochoa, V., Gozalo, B., et al. (2015). Increasing aridity reduces soil microbial diversity and abundance in global drylands. Proc. Natl. Acad. Sci. U. S. A. 112, 15684–15689. doi: 10.1073/pnas.1516684112
McHugh, T. A., Morrissey, E. M., Mueller, R. C., Gallegos-Graves, V., Kuske, C. R., and Reed, S. C. (2017). Bacterial, fungal, and plant communities exhibit no biomass or compositional response to two years of simulated nitrogen deposition in a semiarid grassland. Environ. Microbiol. 19, 1600–1611. doi: 10.1111/1462-2920.13678
Nguyena, L. T. T., Osanaia, Y., Laia, K., Andersona, I. C., Bangeb, M. P., Tissuea, D. T., et al. (2018). Responses of the soil microbial community to nitrogen fertilizer regimes and historical exposure to extreme weather events: flooding or prolongeddrought. Soil Biol. Biochem. 118, 227–236. doi: 10.1016/j.soilbio.2017.12.016
Nkebiwe, P. M., Weinmann, M., Bar-Tal, A., and Müller, T. (2016). Fertilizer placement to improve crop nutrient acquisition and yield: a review and meta-analysis. Field Crop Res. 196, 389–401. doi: 10.1016/j.fcr.2016.07.018
Ochoa-Hueso, R., Collins, S. L., Delgado-Baquerizo, M., Hamonts, K., Pockman, W. T., Sinsabaugh, R. L., et al. (2018). Drought consistently alters the composition of soil fungal and bacterial communities in grasslands from two continents. Glob. Chang. Biol. 24, 2818–2827. doi: 10.1111/gcb.14113
Ogaya, R., and Peñuelas, J. (2004). Phenological patterns of Quercus ilex, Phyllerea latifolia and Arbutus unedo growing under a field experimental drought. Ecoscience 11, 263–270. doi: 10.1080/11956860.2004.11682831
Plaza-Bonilla, D., Álvaro-Fuentes, J., and Cantero-Martínez, C. (2014). Identifying soil organic carbon fractions sensitive to agricultural management practices. Soil Tillage Res. 139, 19–22. doi: 10.1016/j.still.2014.01.006
Qi, R., Li, J., Lin, Z., Li, Z., Li, Y., and Yang, X. (2016). Temperature effects on soil organic carbon, soil labile organic carbon fractions, and soil enzyme activities under long-term fertilization regimes. Appl. Soil Ecol. 102, 36–45. doi: 10.1016/j.apsoil.2016.02.004
Roberts, B. A., Fritschi, F. B., Horwath, W. R., Scow, K. M., Rains, W. D., and Travis, L. R. (2011). Comparisons of soil microbial communities influenced by soil texture, nitrogen fertility, and rotations. Soil Sci. 176, 487–494. doi: 10.1097/SS.0b013e31822769d3
Sayer, E. J., Crawford, J. A., Edgerley, J., Askew, A. P., Hahn, C. Z., Whitlock, R., et al. (2021). Adaptation to chronic drought modifies soil microbial community responses to phytohormones. Commun. Biol. 4:516. doi: 10.1038/s42003-021-02037-w
Schimel, J. P. (2018). Life in dry soils: effects of drought on soil microbial communities and processes. Annu. Rev. Ecol. Evol. Syst. 49, 409–432. doi: 10.1146/annurev-ecolsys-110617-062614
Schwinning, S., and Sala, O. E. (2004). Hierarchy of responses to resource pulses in arid and semi-arid ecosystems. Oecologia 141, 211–220. doi: 10.1007/s00442-004-1520-8
She, W. W., Bai, Y. X., Zhang, Y. Q., Qin, S. G., Feng, W., Sun, Y. F., et al. (2018). Resource availability drives responses of soil microbial communities to short-term precipitation and nitrogen addition in a desert Shrubland. Front. Microbiol. 9:186. doi: 10.3389/fmicb.2018.00186
Singh, B. L., Munro, S., Potts, J. M., and Millard, P. (2007). Influence of grass species and soil type on rhizosphere microbial community structure in grassland soils. Appl. Soil Ecol. 36, 147–155. doi: 10.1016/j.apsoil.2007.01.004
Sinsabaugh, R. L., Lauber, C. L., Weintraub, M. N., Ahmed, B., Allison, S. D., Crenshaw, C., et al. (2008). Stoichiometry of soil enzyme activity at global scale. Ecol. Lett. 11, 1252–1264. doi: 10.1111/j.1461-0248.2008.01245.x
Spinoni, J., Naumann, G., and Vogt, J. (2015). Spatial patterns of European droughts under a moderate emission scenario. Adv. Sci. Res. 12, 179–186. doi: 10.5194/asr-12-179-2015
Tecon, R., and Or, D. (2017). Biophysical processes supporting the diversity of microbial life in soil. FEMS Microbiol. Rev. 41, 599–623. doi: 10.1093/femsre/fux039
Toberman, H., Laiho, R., Evans, C. D., Artz, R. R. E., Fenner, N., Straková, P., et al. (2010). Long-term drainage for forestry inhibits extracellular phenol oxidase activity in Finnish boreal mire peat. Eur. J. Soil Sci. 61, 950–957. doi: 10.1111/j.1365-2389.2010.01292.x
Wang, Y. J., Liu, L., Yang, J. F., Duan, Y. M., Luo, Y., Taherzadeh, M. J., et al. (2020). The diversity of microbial community and function varied in response to different agricultural residues composting. Sci. Total Environ. 715:136983. doi: 10.1016/j.scitotenv.2020.136983
Wen, X., Xiao, C., Xin, J., and Biao, Z. A. (2018). Meta-analysis of soil extracellular enzyme activities in response to global change. Soil Biol. Biochem. 123, 21–32. doi: 10.1016/j.soilbio.2018.05.001
Xiao, L., Zhao, M., Liu, G. B., Li, P., Liu, F. Y., and Xue, S. (2022). Soil biochemical index-based assessment of the effect of drought stress on the rhizosphere soil quality in three typical grass species in the loess plateau, China. J. Soils Sediments 22, 2982–2994. doi: 10.1007/s11368-022-03291-z
Yakushev, A. V., Zhuravleva, A. I., and Kuznetsova, I. N. (2023). Effects of long-term and short-term droughts on the hydrolytic enzymes in haplic Luvisol. Eurasian Soil Sci. 56, 782–792. doi: 10.1134/S1064229323600380
Yang, X. D., Ma, L. F., Ji, L. F., Shi, Y. Z., Yi, X. Y., Yang, Q. L., et al. (2019). Long-term nitrogen fertilization indirectly affects soil fungi community structure by changing soil and pruned litter in a subtropical tea (Camellia sinensis L.) plantation in China. Plant Soil 444, 409–426. doi: 10.1007/s11104-019-04291-8
Yang, C. M., Yang, L. Z., and Ouyang, Z. (2005). Organic carbon and its fractions in paddy soil as affected by different nutrient and water regimes. Geoderma 124, 133–142. doi: 10.1016/j.geoderma.2004.04.008
Yuste, G. C., Fernandez-Gonzalez, A. J., Fernandez-Lopez, M., Ogaya, R., Penuelas, J., Sardans, J., et al. (2014). Strong functional stability of soil microbial communities under semiarid Mediterranean conditions and subjected to long-term shifts in baseline precipitation. Soil Biol. Biochem. 69, 223–233. doi: 10.1016/J.SOILBIO.2013.10.045
Zeng, J., Liu, X., Song, L., Lin, X., Zhang, H., Shen, C., et al. (2016). Nitrogen fertilization directly affects bacterial community composition. Soil Biol. Biochem. 92, 41–49. doi: 10.1016/j.soilbio.2015.09.018
Zhang, W. D., Chao, L., Yang, Q. P., Wang, Q. K., Fang, Y. T., and Wang, S. L. (2016). Litter quality mediated nitrogen effect on plant litter decomposition regardless of soil fauna presence. ESA 97, 2834–2843. doi: 10.1002/ecy.1515
Zhang, Y., Shen, H., He, X., Thomas, B. W., Lupwayi, N. Z., and Hao, X. (2017). Fertilization shapes bacterial community structure by alteration of soil pH. Front. Microbiol. 8:1325. doi: 10.3389/fmicb.2017.01325
Zhong, Y., Yan, W., Wang, R., Wen, W., and Shangguan, Z. (2018). Decreased occurrence of carbon cycle functions in microbial communities along with long-term secondary succession. Soil Biol. Biochem. 123, 207–217. doi: 10.1016/j.soilbio.2018.05.017
Zhu, L., Li, Y. X., Wang, Y., Wang, Y. Y., Zhang, X. Y., Chen, A. J., et al. (2021). Effects of nitrogen application on maize nitrogen uptake and soil biological and chemical properties under drought stresses at seedling stage. J. Soil Water Conserv. 35, 267–274. doi: 10.13870/j.cnki.stbcxb.2021.04.037
Keywords: short-term drought, nitrogen application, soil enzyme activity, alpha diversity, bacterial and fungal communities, functional genes
Citation: Wang R, Zhang J, Ma T, Lv W, Zhang Z, Shen Y, Yang Q, Wang X, Li J, Xiang Q, Lv L, Zhang J and Ma J (2023) Effects of short-term drought, nitrogen application and their interactions on the composition and functional genes of soil microbial communities in alfalfa grassland on the Loess Plateau. Front. Sustain. Food Syst. 7:1332683. doi: 10.3389/fsufs.2023.1332683
Edited by:
Zhou Li, Guizhou University, ChinaReviewed by:
Xiaonan Guo, Hebei GEO University, ChinaRamadoss Dhanuskodi, Massey University, New Zealand
Copyright © 2023 Zhang, Ma, Lv, Zhang, Shen, Yang, Wang, Li, Xiang, Lv, Zhang and Ma. This is an open-access article distributed under the terms of the Creative Commons Attribution License (CC BY). The use, distribution or reproduction in other forums is permitted, provided the original author(s) and the copyright owner(s) are credited and that the original publication in this journal is cited, in accordance with accepted academic practice. No use, distribution or reproduction is permitted which does not comply with these terms.
*Correspondence: Jingyong Ma, majy@lzu.edu.cn