The fate of nitrogen in animal urine patch as affected by trees and nitrogen supply on integrated crop-livestock systems
- 1Academic Department of Agricultural Sciences, Federal University of Technology - Parana (UTFPR), Campus Pato Branco, Brazil
- 2Animal Production Technical Area Department, Rural Development Institute of Paraná IAPAR-Emater, Ponta Grossa, Brazil
- 3Animal Production Technical Area Department, Rural Development Institute of Paraná IAPAR-Emater, Pato Branco, Brazil
- 4Department of Agricultural Sciences, Universidad Nacional del Altiplano - Puno, Puno, Peru
Nitrogen (N) losses by leaching are significant in terms of environmental contamination. Thus, attention has been given to urination points of ruminant urine because they contain high levels of N. Integrated crop-livestock systems (ICLS), in turn, seek to intensify production and, at the same time, make production systems more sustainable. Therefore, it is crucial to know the behavior of N to boost its cycling and, consequently, minimize its impacts (environmental and economic). The objective of this study was to evaluate N leaching from bovine urine in a mixed pasture of Lolium multiflorum x Avena strigosa in two different integrated systems, crop-livestock (CL) and crop-livestock with trees (CLT), and two N doses (90 and 180 kg N ha−1). During the winter 2018, the study was conducted in the Brazilian subtropics, in sandy texture soil. The application of higher doses of nitrogen fertilizer (180 kg N ha−1) causes an increase in the concentration of nitrogen excreted in urine and, consequently, increases nitrate and ammonium contents in the soil at the urine points. Higher nitrogen contents were observed at the urine points, since the uptake of nutrients by the forage plants was reduced due to shading of the plants, leading to a reduction in dry matter production of the pasture. The area of action/diffusion of urinary N does not exceed 25 cm from the center point of urination. No leaching of nitrate or ammonium was observed at the sampled urine points, up to a depth of 40 cm. However, the adoption of more intensified production systems (i.e., with the inclusion of grazing animals and increased nitrogen fertilization) should be conducted under conditions that provide high plant uptake of soil nutrients to reduce the risk of losses.
1. Introduction
The integrated crop-livestock system has been highlighted in relation to sustainable soil management. These systems are based on the biological cycling of nutrients, and grazing can boost this process, since the animal acts as a catalyst in the system, consuming forage and depositing feces and urine in the soil (Ribeiro et al., 2021). Many studies have been conducted in integrated crop-livestock system relating the effect of grazing on the dynamics of decomposition and release of nutrients left by the pasture (residual dry matter) and by the excreta of grazing animals (feces and urine) on the successor grain crop. However, the cycling of nutrients through urine is still little known, being an important source of nutrients for the system, especially nitrogen and potassium (Devasena and Sangeetha, 2022).
Ruminants convert a low quantity of nutrients consumed via pasture into animal product, varying from 5 to 30%, making the return of nutrients to the soil, through animal excreta, an important event in the nutrient cycle (Dubeux and Sollenberger, 2020). In the case of urine, there can be high rates of N at urination points, ranging from 200 to 2,000 kg N ha−1 (Selbie et al., 2015), usually exceeding the ability of plants and microorganisms to utilize these nutrients for growth, resulting in losses to the environment (Hoogendoorn et al., 2010; Cameron et al., 2013). Thus, the large amount of nutrients entering at localized points may be susceptible to losses through leaching, since they may exceed the plant's demand.
There are several factors that may affect the loss of N. These may be related to the nitrogen fertilization performed in the pasture, which promotes gains in plant and animal production and in the improvement of soil fertility, by intensifying the processes of cycling and recycling nutrients. Another factor that may affect the reduction of N losses in integrated crop-livestock system is the presence of trees in the system, which may contribute to the cycling of nutrients through the addition of litter and the absorption of nutrients in deeper soil layers. Furthermore, the intrinsic characteristics of the soil, such as low clay content in sandy soils, can directly influence the leaching process (Tahir and Marschner, 2017). In contrast, there are incipient studies regarding early fertilization in sandy soils. Therefore, it is important to understand the behavior of N from animal urine in integrated crop-livestock system to mitigate losses and maximize the use of this nutrient, making the use of these systems an important tool for agricultural sustainability.
Therefore, we hypothesized that: (1) the application of nitrogen to pasture increases the rate of nutrient input into the soil via urine, and (2) the introduction of trees to pasture affects the behavior of nitrogen in the soil. This study aimed to evaluate the effect of bovine urination and nitrogen application on the behavior of N-mineral in the soil in an integrated crop-livestock-tree system compared with an integrated crop-livestock system.
2. Materials and methods
The experiment was conducted at the Rural Development Institute of Paraná-IAPAR-Emater, Ponta Grossa-PR (25° 07' 22" S, 50° 03' 01" W) in southern Brazil. The local climate is humid subtropical, or Cfb, in the Köppen classification system (Koppen and Geinger, 1928), with frequent occurrence of frosts and an average annual temperature of 17.6°C, ranging from 14°C in July to 21°C in January. The average annual rainfall is 1,400 mm. The meteorological data during the experimental period are shown in Figure 1. The accumulated precipitation during the experiment was 528 mm. The average maximum and minimum temperatures were 23°C and 12°C, respectively.
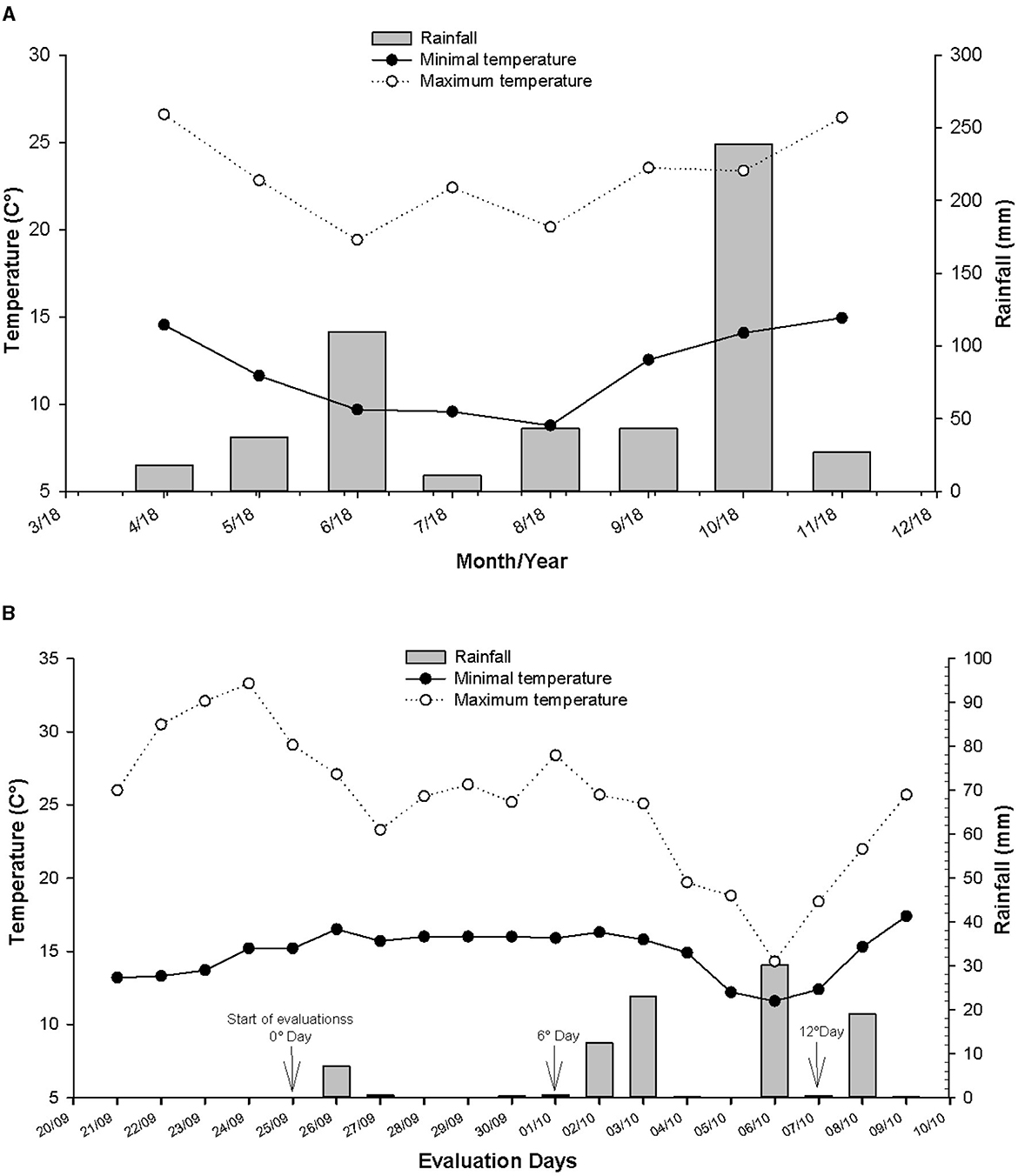
Figure 1. Meteorological data during the period of pasture development (A) and meteorological data during the period of soil collection at urine urination points (B). Ponta Grossa - PR, Brazil. Source: SIMEPAR, Ponta Grossa-PR, (2020).
The experimental area is 13.07 ha, 6.09 ha of which is wooded. These areas are contiguous, containing an association of soils classified as Typic Distrudept and Rhodic Hapludox variety, with sandy texture (19%, 3%, and 78% of clay, silt, and sand in the upper 20 cm, respectively). The relief has between 4 and 9% slope.
Chemical properties of the 0–0.2 m layer of soil at the beginning of the experiment were pH (CaCl2) = 4.97, organic matter (OM) = 24.96 g dm−3, P = 71.16 mg dm−3, K = 98.40 mg dm−3, Ca = 2.16 cmol(c) dm−3, Mg = 1.27 cmol(c) dm−3, base saturation = 47.09%, CEC = 7.73 cmol(c) dm−3, NH4+ = 11.93 mg kg−1 soil, NO3−= 9.07 mg Kg−1 soil, and N-Mineral total= 21.00 mg Kg−1 soil. Moreover, in the 0–0.4 m layer of soil: NH4+= 9.89 mg Kg−1 de solo, NO3−= 6.45 mg Kg−1 soil, and N-Mineral = 16.34 mg Kg−1 soil.
2.1. Area history
In 2006, three tree species: eucalyptus (Eucalyptus dunnii Maiden), pink pepper (Schinus terebinthifolius Raddi) and grevillea (Grevillea robusta A. Cunn. ex R. Br.), were planted in 6 of the 12 experimental units, each with 0.99 ± 0.231 ha. The experimental units were considered like areas conducted in CL and CLT systems and their replications. The species were interspersed in the same row, transversely about the slope, with a predominantly northeast–southwest orientation, with 3×14 m spacing (238 trees ha−1).
The experimental area has been conducted in an integrated crop-livestock system, using no-till farming since 2009. In winter 2010, the production system integrated cool-season pastures (black oat + ryegrass) and warm-season corn or soybean in the same area and crop year.
During the summer 2013, we reduced the number of trees in the area to 159 trees ha−1 with the removal of the pink pepper trees. In November 2014, thinning of the grevillea trees occurred to reduce the negative effect of high shading. Thus, only eucalyptus trees remained in the experimental area (i.e., 79 trees ha−1). In November 2015, another thinning occurred due to the high negative impact of shading by eucalyptus trees, thus remaining 40 trees per ha, at a spacing of 28 m between rows and 9 m between plants (Pontes et al., 2021).
2.2. Treatments and experimental design
The experimental design was a randomized block (blocking factor: slope of 5%), with three repetitions in factorial scheme (2 × 2 × 3 × 4 × 4), where are defined, respectively: nitrogen doses (90 and 180 kg N ha−1) applied to pasture; integrated systems, crop-livestock (CL) and crop-livestock with trees (CLT); days that collections were performed after urination (0, 6, and 12, being these equidistant), horizontal distances from the central point of urination (0, 25, 50, and 75 cm, being these equidistant) and soil collection depths (0–5, 5–10, 10–20, and 20–40 cm collection). According to Bernardon et al. (2021), the highest N requirements in pasture occur during early pasture development (i.e., early tillering), justifying the single application at this stage and soil (Pontes et al., 2018).
Nitrate and ammonium soil concentration was determined in the sample collected immediately, on the 6th and 12th day after the urination, respectively, denominated 0. On these dates, we collected samples from 0 (the central point of the urine urination site), 25, 50, and 75 cm from the central point, as well as in different depths along with the soil profile, 0–5, 5–10, 10–20, and 20–40 cm (Figure 2).
2.3. Establishment of pasture
Sowing of black oat (Avena strigosa Schreb) + annual ryegrass (Lolium multiflorum Lam.) took place on 16 May 2018 in no-till system, with inter-row spacing of 17 cm and sowing density of 45 and 15 kg ha−1, respectively, being applied 400 kg ha−1 commercial formula 4-30-10 (NPK), which represents 16 kg N ha −1, 120 kg P2O5 ha−1, and 40 kg K2O ha−1 at seeding. Urea application (45% N) occurred on 18 June 2018 (33 days after pasture seeding), using 90 kg N ha−1 or 180 kg N ha−1, according to the treatments.
The crop used previously (summer 2017-2018) was corn (Zea mays L., Balu 280 Pro) was sown previously in summer, at the beginning of November 2017, in a no-till system, with row spacing of 0.80 m, with six seeds per linear meter, configuring an initial population of 75,000 plants ha−1, 400 kg ha−1 of commercial formula 10-30-10 (NPK; 40 kg N ha−1, 120 kg P2O5 ha−1 and 40 kg K2O ha−1) was used at sowing and 270 kg of urea ha−1 as topdressing 40 days after sowing, in a single dose in both systems.
2.4. Management pasture height
The grazing system used was continuous stocking with the use of the “put and take” technique, described by Mott and Lucas (1952), to adjust the stocking rate and maintain a constant pasture height of approximately 20 cm by regulating animals.
In each experimental area, three Purunã breed animals (heifers between 8 and 10 months old and 225 kg of body weight) were evaluated during the livestock phase of the experiment (Pontes et al., 2020). Adjustments were made regularly, based on weekly evaluations of pasture height, measured with the aid of a graduated ruler at 100 points per experimental unit. The animals entered into the pasture on 5 July 2018 (50 days after pasture sowing), when ryegrass + oats had an overall average height of all treatments of 24.3 cm, remaining until 17 October 2018.
2.5. Urine collection and analysis
We collected urine samples following the spot sampling methodology, which is performed by spontaneous urina. The collection was performed at the time of weighing the animals (two collections at an interval of 28 days each), using three test animals from each treatment. Immediately after urine sampling, the liquid was filtered with filter paper and acidified with 40 ml of sulfuric acid (0.036 N) in 10 ml of urine (Valadares et al., 1999), identified, and immediately frozen (–20 °C) until analyzed. The cooled urine samples were analyzed for total N concentration using the direct distillation method of Bremmer and Kenney (1966), described by Tedesco et al. (1995). The concentration of total N in urine is expressed as grams of N per liter (g N L−1) of fresh urine. The N contents in urine differed according to the different N doses (90 vs. 180 kg N ha−1), in which 2.05 g N L−1 and 4.06 g N L−1 were found, respectively.
2.6. Nitrate and ammonium
Soil collection occurred on 25 September 2018 (day 0), 1 October 2018 (day 06), and 13 October 2018 (day 12). For soil collection, the animals were initially observed in the field, identifying three urination points within each plot, demarcating the central point of the urine site; then, the urination area was isolated with cages measuring 40 cm by 40 cm. The averages for evaluating nitrate and ammonium in the soil on the evaluation days are averages of three collections per experimental unit.
We took three soil samples per experimental unit with the help of a caliper auger, stratified at depths of 0–5, 5–10, 10–20, and 20–40 cm and different distances from the central urine point (CPS), being at 0 (midpoint), 25, 50, and 75 cm from the main point. Samples were collected 2 h after urination of the animals. The means for evaluating nitrate and ammonium at different soil depths and distance from the central point are means of three collections per experimental unit.
The samples were dried in an oven with forced air circulation at 55 °C for approximately 120 h, ground on a sieve with a 2 mm mesh, and taken for analysis. The analyses for the determination of nitrate and ammonium were performed according to the methodology by Keeney and Bremner (1966), by the Kjeldahl steam distillation method using 1M KCl as described by Tedesco et al. (1995).
2.7. Statistical procedures
After checking that the assumptions of the analysis of variance had been met, the data for all the variables were subjected to an analysis of variance using a factorial mathematical model (2 × 2 × 3 × 4 × 4, i.e., N levels, systems, days after urination, distance from the central point of urination and soil depth), with three replications in a randomized block design (=5%). All the analyses were done using Statgraphics statistical software, and the images were analyzed using SigmaPlot R version 12.5 (Systat Software, San Jose, CA).
3. Results
3.1. Ammonium
In the CL, no significant variation of values was observed at the different N doses applied (90 and 180 kg N ha−1) and as a function of the days of evaluation after urination (Figures 3A, B). At day zero, the central point of urination presented higher ammonium values, which decreased over the course of days (6 and 12 days). Increases in ammonium levels do not occur in the CL system beyond 25 cm from the central point of urination (Table 1).
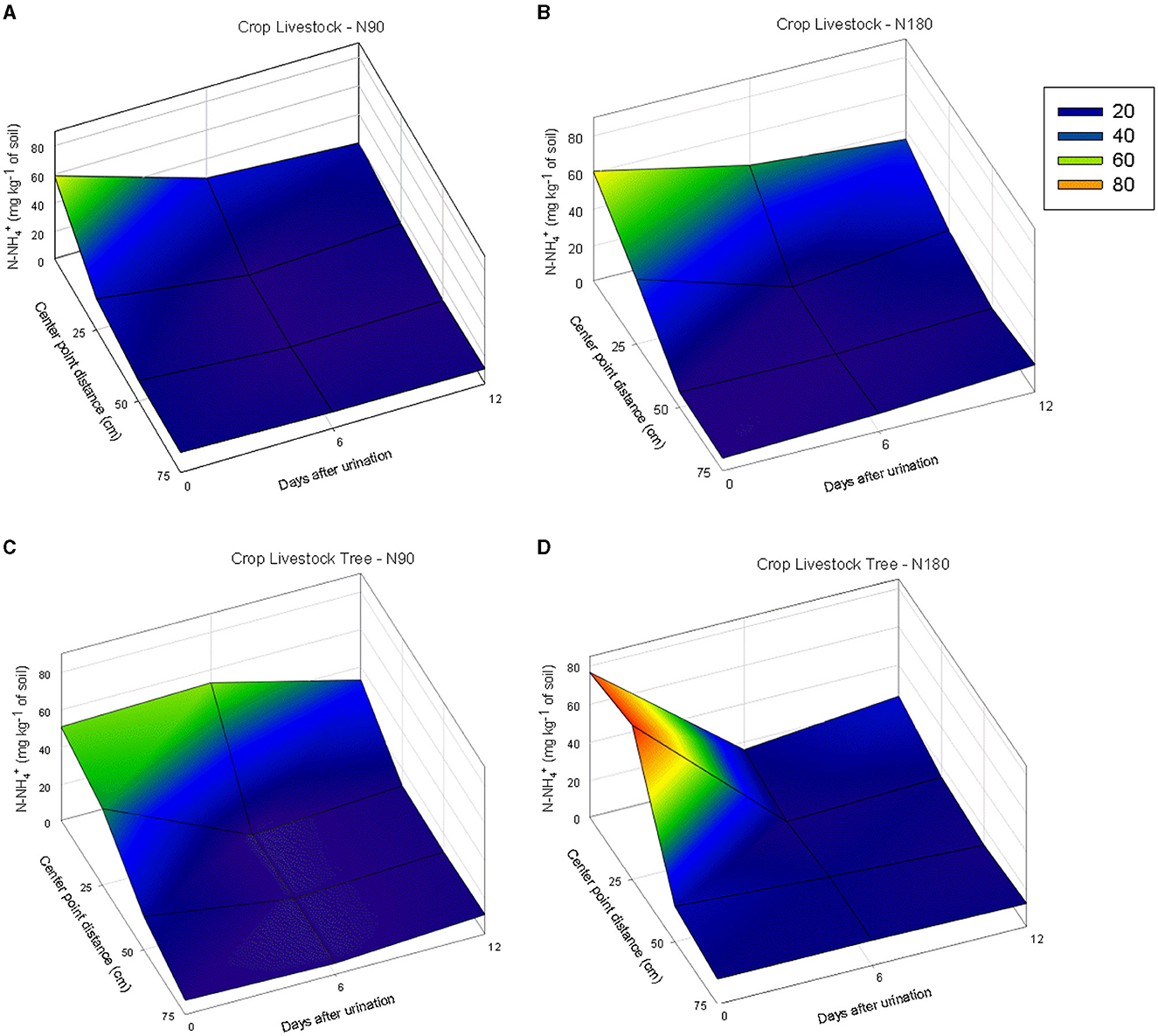
Figure 3. Ammonium concentrations ( mg kg−1; P = 0.0111) after urine urination in Lolium multiflorum + Avena strigosa pastures under two different integrated systems, crop-livestock [CL, (A, B)] and crop-livestock-tree [CLT, (C, D)], and two nitrogen doses (90 and 180 kg N ha−1; N 90 and N 180, respectively) as a function of days after urine urination and distance from the central urination point.
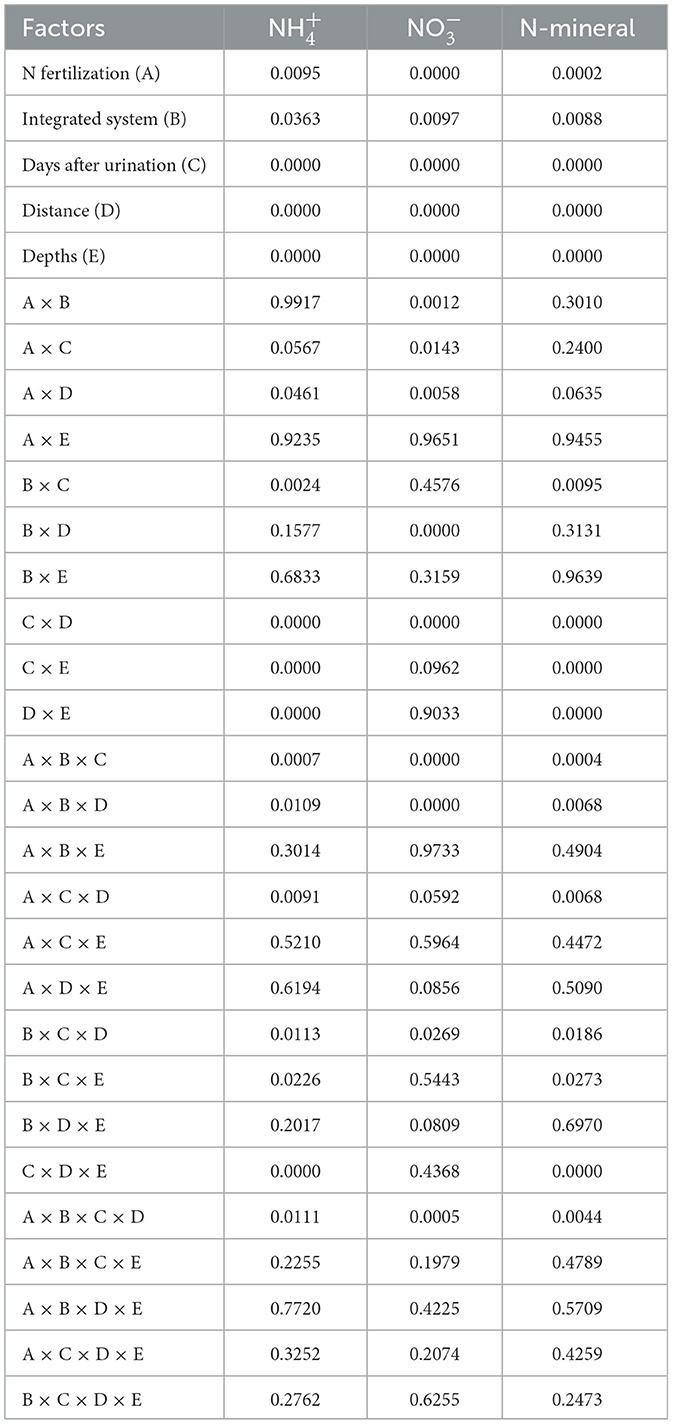
Table 1. Analysis of variance of ammonium (), nitrate (), and N-mineral contents at micturition points as a function of N application, production systems, collection days, distance, and depth.
On the other hand, in CLT areas, greater variations in soil contents were observed (Figures 3C, D). At N90 treatments, increases in soil concentration were observed (Figure 3C) at the central urination point, which extended until sampling day 6 (53.03 mg kg−1 soil) with these values decreasing only on sampling day 12 (16.5 mg kg−1 soil). CLT-N180 presented the highest soil concentration Figure 3D). On day 0 of sampling, the central point showed a concentration of 76,8 mg kg−1 of soil, reducing this value to 50 mg kg−1 soil at 25 cm distance, decreasing to even lower values on the other days and distances evaluated.
3.2. Nitrate
Nitrate () soil concentration changes occurred up to a distance of 25 cm from the central point of urination (Figure 4), as for ammonium soil concentration (Figure 3). Increases in nitrate contents up to 25 cm from the central urination point were observed only in areas with trees at the highest dose of nitrogen fertilization (Figure 4D). On the 6th day after urination, we observed a peak in soil nitrate contents at the distance of 50 cm from the central sampling point (26.9 mg kg−1 soil). At a distance of 25 cm until the 12th day of evaluation, increases of are observed in Figure 4B (CL-N180) and Figure 4C (CLT-N90), indicating that N-urine was still in the process of transformation.
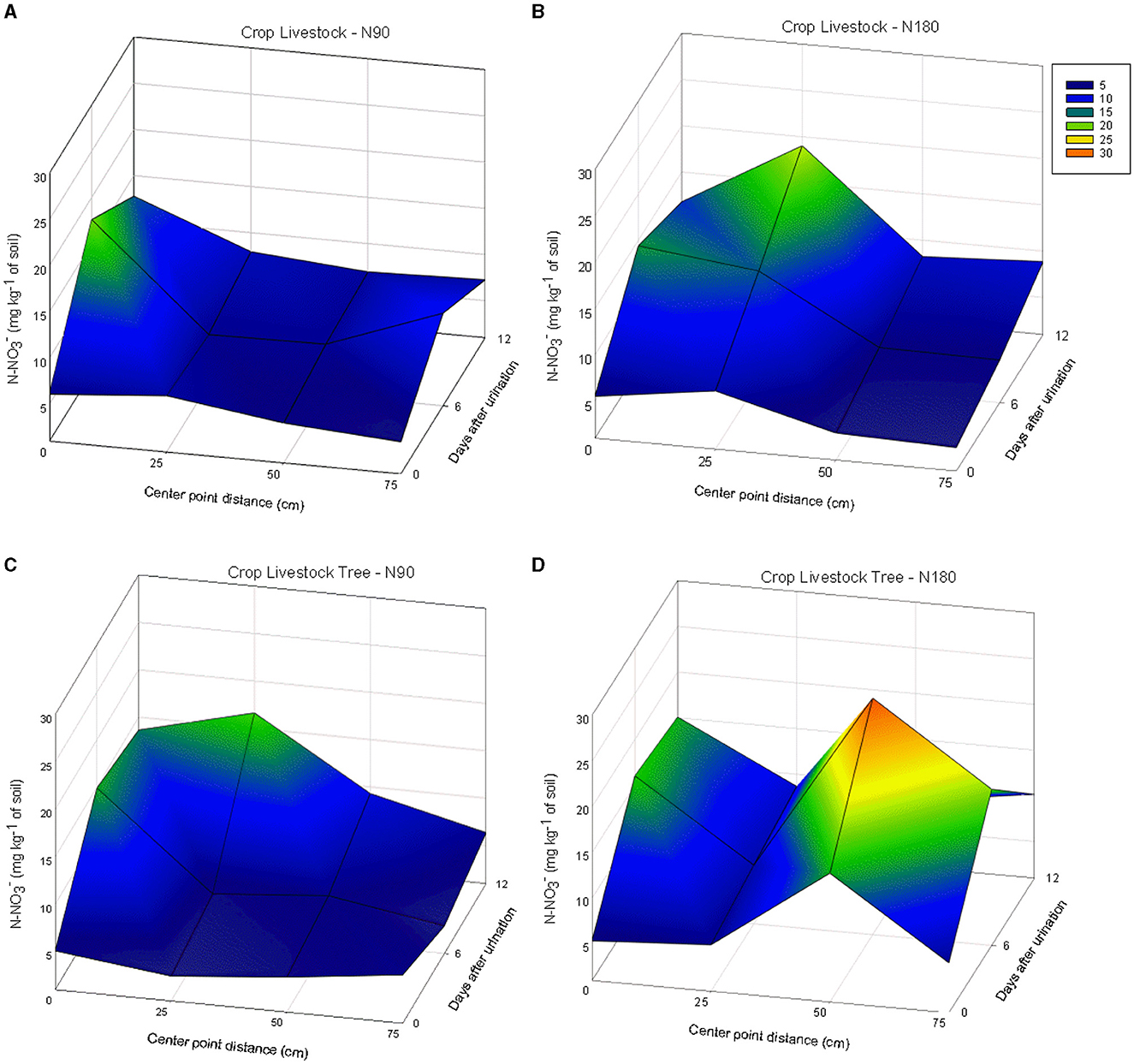
Figure 4. Nitrate concentrations ( mg kg−1; P = 0.0005) after urine urination in Lolium multiflorum + Avena strigosa pastures under two different integrated systems, crop-livestock [CL, (A, B)] and crop-livestock-tree [CLT, (C, D)], and two nitrogen doses (90 and 180 kg N ha−1; N90 and N180, respectively) as a function of days after urine urination and distance from the central urination point.
3.3. Nitrate and ammonium concentration in function of soil depth
There was no evidence of ammonium and nitrate leaching up to 40 cm soil depth (P = 0.0000) (Figure 5). In both chemical forms, there was a decrease in the contents with increasing soil depth, with the 0–5 and 20–40 cm layers presenting 10.9 mg and 7.3 mg of kg−1 of soil, respectively (Figure 5). While presents in the 0–5 and 20–40 cm layer, was 47 and 20.8 mg of kg−1 of soil.
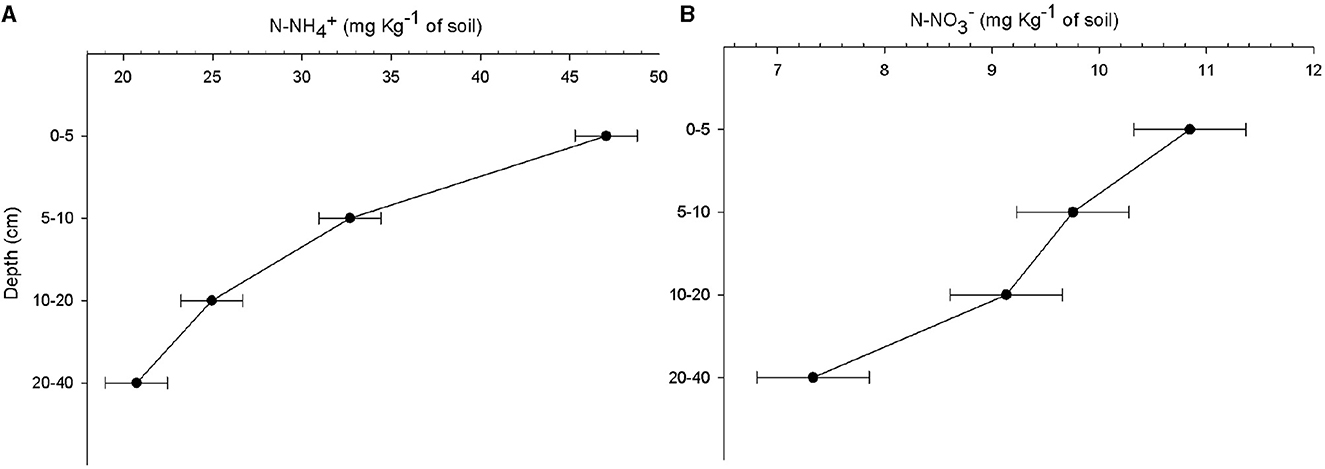
Figure 5. Ammonium (A) ( mg kg−1; P = 0.0000) and nitrate (B) ( mg kg−1; P = 0.0000) concentration at different soil depths at urine urination points of cattle on Lolium multiflorum + Avena strigosa pasture. Vertical bars indicate the standard error of the mean ( ±1.74; ± 0.53).
The range of action of the N-urinary in our study does not exceed 25 cm, resulting in an effective area of 0.197 m2.
4. Discussion
The shading effect resulted in a higher N concentration in the soil by lower extraction of N by plants. Carpinelli et al. (2020) verified, in the same year and experiment, a 42% reduction in light input in the system with trees. This lower forage production in shaded areas is mainly due to the lower tillering and stretch of the plants (Oliveira et al., 2007; Belesky et al., 2011, 2017; Paciullo et al., 2011).
Integrated systems become more complex as more components are included in the system, as in the case of CLT, where the tree component can alter some factors, such as temperature and soil moisture, due to the changes in light entering the system. This fact can alter the chemical, physical, and biological characteristics of the soil (Dias et al., 2007; Sales et al., 2018; Assis et al., 2019), modifying the flow of nutrients and, consequently, their contents in the soil.
In both systems, the higher N application in the system provided higher availability of ammonium (>80 mg kg−1 of soil) and soil nitrate (19 mg kg−1 of soil), possibly due to the higher amount of N via urine added to the soil, more than 98%. After urination, the area affected by N-urinary is dependent on several factors that can modify the area of action of N coming from the urine, such as (i) urinary volume; (ii) solute concentrations; (iii) soil texture (related to cation exchange capacity); (iv) soil moisture content; (v) topography, (vi) weather; (vii) vegetation type and, (viii) root architecture (Marsden et al., 2016).
The effective area of a urine patch is defined by the wet area added to the peripheral area outside, which is affected by the urinary N (Selbie et al., 2015). Our observed value is in agreement with those observed in several studies, where the effective area ranges from 0.03 to 1.1 m2 for cattle (Selbie et al., 2015). Therefore, knowing the effective area of a urine patch (25 cm), the concentration of urinary N contained in the urine (2.05 and 4.06 g N L−1 of urine) (McAuliffe et al., 2020), and the average volume of urine per urination (1.9 L) (Whitehead, 2000), it is possible to calculate the application rate of urinary N per hectare. In the present study, the application rate of N via urine was therefore 197.7 and 391.6 kg N ha−1, between doses of N.
After N enters the soil through urine, urea is rapidly hydrolyzed from ammonium to the subsequent nitrate. The nitrification process results in an increase in content (Whitehead, 2000). Soil moisture causes the resumption of the nitrification process (Bissani et al., 2008) and is a determining factor in the leaching of available nutrients in the soil solution, mainly N in the form of . According to Allende-Montalbán et al. (2021), after nitrification of N, most of the N-mineral, mainly in the form of , can be leached out under conditions of excessive precipitation. Considering that the accumulated rainfall for the period of the experiment was 528 mm (Figure 1A), it can be said that this value is low. Given that in the previous year, in the same experimental area, an accumulated average of 934 mm was obtained (Pontes et al., 2020). These values contribute to understanding the data, mainly because there was no leaching up to the 40 cm depth of the soil. Although, even with the 66.4 mm precipitation (Figure 1) before the third collection, such precipitation was insufficient for leaching losses, keeping this nutrient in the topsoil layers. According to Fichtner et al. (2019), with the more significant number of empty pores in dry soil conditions, there is less infiltration of water and loading of available nutrients in the soil solution unlike what happens in wet soils.
Marsden et al. (2016), considering the horizontal diffusion area of sheep urine (load equivalent to 200 kg N ha−1) in a Cambisol, maintained at 50% or 70% moisture, found small variations in diffusion (6 cm) and only in the first days of evaluation (3 days), due to the rapid hydrolysis of urea. Subsequently, decrease in contents, and a progressive increase in occured. Nitrate peak happened at 19 days of evaluation as far as nitrification proceeds, with a decreasing trend after this evaluation. In addition, higher concentrations were found in soils with 50% water (268 mg kg−1 soil) compared with 70% moisture (207 mg kg−1 soil), indicating that high moisture can increase the dilution and diffusion of , decreasing its concentration in soil. However, the study conducted by Marsden et al. (2016) disregards the effects of the presence of preferential flows, roots, and plant uptake and vertical diffusion of in soil, among other factors, which may result in lower availability in soil, as verified in our study.
In sandy soil, ammonium is also poorly retained the negative charges in the soil (Alrashidi et al., 2020) due to the low clay and MO content usually found in these soils, which also increases the possibility of losses in this chemical form. Soil texture is, therefore, an essential factor in the behavior of N because it directly influences the dynamics of water in the soil. In sandy soils, on average, 70% of its composition is sand that make them highly permeable, with low water retention capacity, low organic matter contents and, consequently, low cation adsorption capacity (He et al., 2014; Centeno et al., 2017). Dunbabin et al. (2003) suggest that high soil rooting density is vital for reducing leaching rates in coarse-textured soils and show the ability of highly branched root architectures to deplete from the surface mineralization zone. N uptake by forage plants at urine urination points can reach 300–700 kg N ha−1 year−1 (During and McNaught, 1961; Ball et al., 1979; Ledgard, 2001).
Some studies demonstrated that significant amounts of N-urine reach greater depths by preference fluxes through soil macro-pores. Menneer et al. (2008), working with a lysimeter (450 mm depth), measured losses of 109 kg in a urine application (775 kg N ha−1) and attributed this result to macro-pore flux and high precipitation/drainage. The same authors, detected the movement of below 700 mm in urine-treated lysimeters and attributed it to macropore flux. Although N is leached primarily as , losses are significant in some circumstances if the soil fails to retain it in the upper soil layers (Selbie et al., 2015).
5. Conclusion
The application of nitrogen fertilizer (180 kg N ha−1) causes an increase in the concentration of nitrogen excreted in urine and, consequently, an increase in nitrate and ammonium contents in the soil at the urination points.
The presence of trees in the system was the most important factor for higher ammonium and nitrate levels observed in the soil.
Horizontally, the area of action/diffusion of urinary N does not exceed 25 cm from the central point of urination. The highest soil N concentration was observed at the central point of urination.
We did not observe the evidence of nitrate and ammonium leaching up to a depth of 40 cm.
Data availability statement
The raw data supporting the conclusions of this article will be made available by the authors, without undue reservation.
Ethics statement
The animal study was reviewed and approved by Ethics Committee of IDR - Paraná. The studies were conducted in accordance with the local legislation and institutional requirements. Written informed consent was obtained from the owners for the participation of their animals in this study.
Author contributions
RL, TA, AA, and LP contributed to conception and design of the study. RL, LK, MM, and PB organized the database. TA and RL performed the statistical analysis. RL wrote the first draft of the manuscript. RL, TA, IS, and LP wrote sections of the manuscript. All authors contributed to manuscript revision, read, and approved the submitted version.
Conflict of interest
The authors declare that the research was conducted in the absence of any commercial or financial relationships that could be construed as a potential conflict of interest.
Publisher's note
All claims expressed in this article are solely those of the authors and do not necessarily represent those of their affiliated organizations, or those of the publisher, the editors and the reviewers. Any product that may be evaluated in this article, or claim that may be made by its manufacturer, is not guaranteed or endorsed by the publisher.
References
Allende-Montalbán, R., Martín-Lammerding, D., Delgado, M. M., Porcel, M. A., and Gabriel, J. L. (2021). Urease inhibitors effects on the nitrogen use efficiency in a maize-wheat rotation with or without water deficit. Agriculture 11, 684. doi: 10.3390/agriculture11070684
Alrashidi, S. H., Sallam, A. S., and Usman, A. R. (2020). Acid-modified and unmodified natural clay deposits for in situ immobilization and reducing phytoavailability of molybdenum in a sandy loam calcareous soil. Sustainability 12, 8203. doi: 10.3390/su12198203
Assis, P. C. R., Stone, L. F., Oliveira, J. M., Wruck, F. J., Madari, B. E., and Heinemann, A. B. (2019). Atributos físicos, químicos e biológicos do solo em sistemas de integração lavoura-pecuária- floresta. Revista Agrarian 12, 57–70. doi: 10.30612/agrarian.v12i43.8520
Ball, P. R., Keeney, D. R., Theobald, P. W., and Nes, P. (1979). Nitrogen balance in urine-affected areas of a New Zealand pasture. Agron. J. 7, 133–175.
Belesky, D. P., Burner, D. M., and Ruckle, J. (2011). Tiller production in cocksfoot (Dactylis glomerata) and tall fescue (Festuca arundinacea) growing along a light gradient. Grass Forage Sci. 66, 370–380. doi: 10.1111/j.1365-2494.2011.00796.x
Belesky, D. P., Burner, D. M., and Ruckle, J. (2017). Relationship between sward height and herbage mass for integrated crop-livestock systems with trees. Grassland Sci. 63, 29–35. doi: 10.1111/grs.12147
Bernardon, A., Simioni Assmann, T., Brugnara Soares, A., Franzluebbers, A., Maccari, M., and de Bortolli, M. A. (2021). Carryover of n-fertilization from corn to pasture in an integrated crop-livestock system. Arch. Agron. Soil Sci. 67, 687–702. doi: 10.1080/03650340.2020.1749268
Bissani, C. A., Gianello, C., Camargo, F. O., and Tedesco, M. J. (2008). Fertilidade dos solos e manejo da adubaçāao de culturas. Porto Alegre: Metrópole.
Bremmer, J. M., and Kenney, D. R. (1966). Determination and isotope-ratio analysis of different forms of nitrogen in soils: 3. exchangeable ammonium, nitrate, and nitrite by extraction-distillation methods. Soil Sci. Soc. Am. J. 30, 577–582. doi: 10.2136/sssaj1966.03615995003000050015x
Cameron, K. C., Di, H. J., and Moir, J. L. (2013). Nitrogen losses from the soil/plant system: a review. Ann. Appl. Biol. 162, 145–173. doi: 10.1111/aab.12014
Carpinelli, S., Fonseca, A. F., d., Neto, P. H. W., Dias, S. H. B., Pontes, L., et al. (2020). Spatial and temporal distribution of cattle dung and nutrient cycling in integrated crop–livestock systems. Agronomy 10, 672–691. doi: 10.3390/agronomy10050672
Centeno, L. N., Guevara, M. D. F., Cecconello, S. T., Sousa, R. O. d., and Timm, L. C. (2017). Textura do solo: Conceitos e aplicaçães em solos arenosos. Revista Brasileira de Engenharia e Sustentabilidade 4, 31–37. doi: 10.15210/rbes.v4i1.11576
Devasena, M., and Sangeetha, V. (2022). “Cow urine: Potential resource for sustainable agriculture,” in Emerging Issues in Climate Smart Livestock Production. Amsterdam: Elsevier, 247–262.
Dias, P. F., Souto, S. M., Resende, A. S., S, U., Rocha, G. P., Moreira, J. F., et al. (2007). Transferência do N fixado por leguminosas arbóreas para o capim survenola crescido em consórcio. Ciência Rural 37, 352–356. doi: 10.1590/S0103-84782007000200009
Dubeux, J. C., and Sollenberger, L. E. (2020). “Nutrient cycling in grazed pastures,” in Management Strategies for Sustainable Cattle Production in Southern Pastures. Amsterdam: Elsevier, 59–75.
Dunbabin, V., Diggle, A., and Rengel, Z. (2003). Is there an optimal root architecture for nitrate capture in leaching environments? Plant, Cell Environ. 26, 835–844. doi: 10.1046/j.1365-3040.2003.01015.x
During, C., and McNaught, K. J. (1961). Effects of cow urine on growth of pasture and uptake of nutrients. New Zeal. J. Agricult. Res. 4, 591–605. doi: 10.1080/00288233.1961.10431617
Fichtner, T., Goersmeyer, N., and Stefan, C. (2019). Influence of soil pore system properties on the degradation rates of organic substances during soil aquifer treatment (sat). Appl. Sci. 9, 496. doi: 10.3390/app9030496
He, H., Wang, H., Hu, K., and Mcconkey, B. (2014). A modelling approach to evaluate the long-term effect of soil texture on spring wheat productivity under a rainfed condition. Agric. Ecosyst. Environ. 4, 1–10. doi: 10.1038/srep05736
Hoogendoorn, C. J., Betteridge, K., Costall, D. A., and Ledgard, S. F. (2010). Nitrogen concentration in the urine of cattle, sheep and deer grazing a common ryegrass/cocksfoot/white clover pasture. New Zeal. J. Agricult. Res. 53, 235–243. doi: 10.1080/00288233.2010.499899
Keeney, D., and Bremner, J. (1966). A chemical index of soil nitrogen availability. Nature 211, 892–893. doi: 10.1038/211892a0
Koppen, W., and Geinger, R. (1928). Klimate der Erde. Alemanha: Verlag Justus Perthes, Gotha, Gotha.
Ledgard, S. F. (2001). Nitrogen cycling in low input legume based agriculture, with emphasis on legume/grass pastures. Plant Soil 228, 43–59. doi: 10.1023/A:1004810620983
Marsden, K. A., Jones, D. L., and Chadwick, D. R. (2016). The urine patch diffusional area: An important N 2 O source? Soil Biol. Biochem. 92, 161–170. doi: 10.1016/j.soilbio.2015.10.011
McAuliffe, G., López-Aizpún, M., Blackwell, M., Castellano-Hinojosa, A., Darch, T., Evans, J., et al. (2020). Elucidating three-way interactions between soil, pasture and animals that regulate nitrous oxide emissions from temperate grazing systems. Agricult. Ecosyst. Environ. 300, 106978. doi: 10.1016/j.agee.2020.106978
Menneer, J. C., Ledgard, S., and Sprosen, M. (2008). Soil N process inhibitors alter nitrogen leaching dynamics in a pumice soil. Austral. J. Soil Res. 46, 323–331. doi: 10.1071/SR06180
Mott, G. O., and Lucas, H. L. (1952). “The design conduct and interpretation of grazing trials on cultivated and improved pastures,” in Internation Grassland Congress. Pensylvania: Estados Unidos, 1380–1395.
Oliveira, T. K., Macedo, R. L. G., Santos, P. A., Higashikawa, E. M., and Venturin, N. (2007). Produtividade de Brachiaria brizantha (Hochst. ex A. Rich.) Stapf cv. Marandu sob diferentes arranjos estruturais de sistema agrossilvipastoril com eucalipto. Ciência e Agrotecnologia 31, 748–757. doi: 10.1590/S1413-70542007000300022
Paciullo, D. S. C., Castro, C. R. T., Fernandes, P. B., Müller, M. D., Pires, M. F., Fernandes, E. N., et al. (2011). Características produtivas e nutricionais do pasto em sistema agrossilvipastoril, conforme a distância das árvores. Pesquisa Agropecuária Brasileira 46, 1176–1183. doi: 10.1590/S0100-204X2011001000009
Pontes, L. d,. S, Porfírio-da Silva, V., Moletta, J. L., and Telles, T. S. (2021). Long-term profitability of crop-livestock systems, with and without trees. Agric. Syst. 192, 103204. doi: 10.1016/j.agsy.2021.103204
Pontes, L. d,. S, Stafin, G., Moletta, J. L., and Porfírio-da Silva, V. (2020). Performance of purun a beef heifers and pasture productivity in a long-term integrated crop-livestock system: the effect of trees and nitrogen fertilization. Agrofor. Syst. 94, 1713–1723. doi: 10.1007/s10457-020-00491-9
Pontes, L. S., Tullio, G. F., Martins, A. S., Moletta, J. L., and Porfírio-da Silva, V. (2018). Corn yield for silage and grains in different integrated crop-livestock systems. Revista Ciência Agronômica 49, 315–323. doi: 10.5935/1806-6690.20180036
Ribeiro, R. H., Ibarr, M. A., Bratti, F., and Dieckow, J. (2021). “Integrated crop-livestock systems on SOC sequestration in subtropical Brazil,” in Recarbonizing Global Soils—A Technical Manual of Recommended Sustainable Soil Management: Cropland, Grassland, Integrated Systems and Farming Approaches—Case Studies. Food and Agriculture Organization and Intergovernmental Technical Panel on Soils. pp. 385–403.
Sales, A., Silva, A. R., Veloso, C. A. C., Carvalho, E. J. M., and Miranda, B. M. (2018). Carbono orgânico e atributos do solo sob manejo agropecuário sustentável na amazônia legal. Colloquium Agrariae 14, 1–15. doi: 10.5747/ca.2018.v14.n1.a185
Selbie, D. R., Buckthought, L. E., and Shepherd, M. A. (2015). The challenge of the urine patch for managing nitrogen in grazed pasture systems. Adv. Agron. 129, 229–292. doi: 10.1016/bs.agron.2014.09.004
Tahir, S., and Marschner, P. (2017). Clay addition to sandy soil reduces nutrient leaching–effect of clay concentration and ped size. Commun. Soil Sci. Plant Anal. 48, 1813–1821. doi: 10.1080/00103624.2017.1395454
Tedesco, M., Gianello, C., Bissani, C., Bohnen, H., and Volkweiss, S. (1995). Análise de solo, plantas e outros materiais. Porto Alegre: Departamento de Solos – UFRGS.
Valadares, R., Broderick, G., Valadares Filho, S., and Clayton, M. (1999). Effect of replacing alfalfa silage with high moisture corn on ruminal protein synthesis estimated from excretion of total purine derivatives. J. Dairy Sci. 82, 2686–2696. doi: 10.3168/jds.S0022-0302(99)75525-6
Keywords: crop-livestock forestry, nutrient cycling, nitrogen leaching, pasture, sandy soil
Citation: Lima RCd, Assmann TS, Pontes LdaS, Mensor M, Assmann AL, Kagimura LT, Barriga PAB and Severo IK (2023) The fate of nitrogen in animal urine patch as affected by trees and nitrogen supply on integrated crop-livestock systems. Front. Sustain. Food Syst. 7:940535. doi: 10.3389/fsufs.2023.940535
Received: 10 May 2022; Accepted: 25 September 2023;
Published: 27 October 2023.
Edited by:
Dipayan Sarkar, United States Department of Agriculture, United StatesReviewed by:
Julio Cesar Pascale Palhares, Brazilian Agricultural Research Corporation (EMBRAPA), BrazilCarlos Alexandre Costa Crusciol, São Paulo State University, Brazil
Copyright © 2023 Lima, Assmann, Pontes, Mensor, Assmann, Kagimura, Barriga and Severo. This is an open-access article distributed under the terms of the Creative Commons Attribution License (CC BY). The use, distribution or reproduction in other forums is permitted, provided the original author(s) and the copyright owner(s) are credited and that the original publication in this journal is cited, in accordance with accepted academic practice. No use, distribution or reproduction is permitted which does not comply with these terms.
*Correspondence: Tangriani Simioni Assmann, tangriani@professores.utfpr.edu.br