Pelagic fish spared from ocean catch by integrating Black Soldier Fly Larvae in U.S. aquaculture production
- Department of Agricultural Sciences, Texas State University, San Marcos, TX, United States
The sustainability challenges associated with utilizing forage fish sourced from ocean catch as fish meal and fish oil in the aquaculture industry has increased the demand for alternative feeds. Previous research indicates that Black Soldier Fly Larvae (BSFL; Hermetia illucens) can partially replace fish meal and/or fish oil in the diets of farmed aquaculture species without compromising fish growth or efficiency. The objective of our study was to identify the amount of pelagic fish from ocean catch that could be spared from fish meal and fish oil production by integrating BSFL in the diets of three aquaculture species, Atlantic Salmon (Salmo salar), Rainbow Trout (Oncorhynchus mykiss), and Whiteleg Shrimp (Litopenaeus vannamei) in the United States. Annual output for these aquaculture species was collected for 2017–2019. For each specie, we calculated the total metric tons (MT) of spared pelagic fish, by taxa, from fish meal and fish oil production based on total lifetime food intake and dietary replacement rates of fish meal and fish oil by BSFL as established in previous literature. At the highest level of dietary BSFL substitution for fish meal and/or fish oil that did not sacrifice performance of the three aquaculture species, 40,843 MT of pelagic fish could be spared from ocean catch in the U.S. per year. Therefore, integrating BSFL in the diets of aquaculture species could reduce the demand for pelagic fish sourced from ocean catch and positively contribute to the sustainability of aquaculture production.
1 Introduction
Projections indicate the global population will reach 9 billion by 2050 (United Nations Department of Economic and Social Affairs, 2019). Together with increased wealth and per capita consumption of fish (Little et al., 2016), this population growth has positioned the aquaculture industry as one of the fastest growing food production sectors in the world (Stankus, 2021). From 1990 to 2020, global aquaculture production grew an average of 6.7% per year (Food and Agriculture Organization (FAO), 2022). Meanwhile, fed-based aquaculture production, for which aquaculture species were farmed and provided formulated feed, grew faster than the non-fed counterpart, reaching approximately 72.2% of total production in 2020 (Food and Agriculture Organization (FAO), 2022). As the market for aquaculture products and fed-based production grows, there is increased concern over the sustainability of using fish meal and fish oil as feed (Malcorps et al., 2019).
Fish meal is the optimal protein feed in aquaculture production (Renna et al., 2017) while fish oil is used as an additive to increase dietary fatty acid content and provide omega-3 fatty acids [National Oceanic and Atmospheric Administration (NOAA), 2023]. Currently, 71% of fish meal and 74% of fish oil are produced from ocean catch of pelagic fish (Boyd et al., 2022), 90% of which are from food-grade fish such as sardines and anchovies (Cashion et al., 2017). As the aquaculture industry utilizes an estimated 46% of fish meal and fish oil produced globally (Malherbe, 2005), the growing demand for aquaculture products will increase the pressure placed on pelagic fish populations. Further, using fish meal and fish oil in farmed salmon production is associated with a carbon footprint of 3,532 kg of carbon dioxide per ton, which is much higher than that from dried BSFL, less than 30 kg of carbon dioxide per ton (Parodi et al., 2020; Wang et al., 2022).
Pelagic fish (e.g., Peruvian Anchoveta, Pacific Sardine, Atlantic Herring), also referred to as forage fish, play vital roles in marine ecosystems by transferring energy from low trophic levels to higher and more valued carnivorous fish (Siple et al., 2019). While pelagic fish undergo cyclical population shifts due to natural stock productivity, increased fishing rates for fish meal and fish oil production exacerbate declining populations (Essington et al., 2015) as previously documented for the Pacific Sardine (Barnes et al., 1992; Baumgartner et al., 1992). Threats and actual realization of pelagic fish population collapse has negative ecosystem consequences, such as decreased utility of the fish as prey and decreased nutrient cycling (Nissar et al., 2022). The global economic value of pelagic fish is an estimated 18.7 billion USD annually, which is more than triple the catch value of these fish (Konar et al., 2019). Diminished pelagic fish populations could harm industries that capture economic value from them, including marine-based ecotourism businesses that are valued at 4.6 trillion USD (Northrop et al., 2022). Besides the ecologic and economic losses, declining pelagic fish populations could also contribute to climate change as pelagic fish sequester carbon from the atmosphere by storing carbon dioxide, a major greenhouse gas (Konar et al., 2019).
In addition to the environmental sustainability and socioeconomic issues of using pelagic fish as feed, fluctuations in supply, price, and quality of fish meal have increased the demand for alternative feeds (Luthada-Raswiswi et al., 2021). Plant-based alternatives and Black Soldier Fly Larvae (BSFL; Hermetia illucens) are among potential alternative feeds. However, plant-based proteins may not fully meet the dietary needs of carnivorous farmed fish (Daniel, 2018) and researchers have reported lower feed conversion ratio (FCR) and growth rate from feeding carnivorous fish plant proteins in place of fish meal (Daniel, 2018).
Due to the nutritional profile, there has been recent interest in utilizing BSFL as an alternative to fish meal in aquaculture production, particularly for carnivorous fish (Priyadarshana et al., 2021). BSFL is an advantageous feed due to the ability of the larvae to convert organic waste into biomass, thus minimizing the natural resource inputs required for rearing (Goyal et al., 2021) and contributing to waste valorization (Siddiqui et al., 2022). Further, BSFL has been approved by the Association of American Feed Control Officials (AAFCO) as a feed ingredient for certain aquaculture species in the U.S. [Association of American Feed Control Officials (AAFCO), 2021] and is being assessed by the European Feed Safety Authority (EFSA) for market integration in the E.U. (Liguori et al., 2022).
Research has been conducted to evaluate BSFL as a feed for three commonly reared and harvested farmed fish species in the United States (U.S.): Atlantic Salmon (Salmo salar; Nøkland, 2019), Rainbow Trout (Oncorhynchus mykiss; Renna et al., 2017), and Whiteleg Shrimp (Litopenaeus vannamei; Cummins et al., 2017). Ultimately, this research demonstrates that BSFL can be used in partial dietary replacement of fish meal and/or fish oil without compromising FCR, fish growth, or survival. As farmed Atlantic Salmon alone requires 60% of global supplies of fish oil and 23% of fish meal (Willer et al., 2022), the benefits of incorporating BSFL into aquaculture production could be significant.
Due to the sustainability concerns around fish meal and fish oil and the demonstrable ability of BSFL to replace these feeds, research that evaluates the impact of integrating BSFL in aquaculture production is warranted. Accordingly, the objective of this study was to estimate the amount of pelagic fish sourced from ocean catch that could be spared from fish meal and fish oil production by integrating BSFL into the diets of farmed Atlantic Salmon (Salmo salar), Rainbow Trout (Oncorhynchus mykiss), and Whiteleg Shrimp (Litopenaeus vannamei) in the U.S.
2 Materials and methods
Table 1 outlines the parameters used in our model; all were derived from existing literature and are described in further detail in this section. The average weight gain per fish achieved during rearing (ΔWeight) was determined by calculating the difference between the average market weight and fry weight for each of the three farmed fish species included in our model in Equation (1). Fry are young fish that have absorbed nutrients from the attached yolk sac and can eat autonomously. Accordingly, Fry Weight is the starting weight of the fish entering aquaculture production. Fry Weight and Average Market Weight were collected for Atlantic Salmon (Maine Aquaculture Innovation Center, 2023), Rainbow Trout (Towers, 2023), and Whiteleg Shrimp [Food and Agriculture Organization (FAO), 2023].
We calculated the total kilograms (KG) of feed consumed per fish during the rearing process for each aquaculture species by multiplying the weight gain per fish, ΔWeight (Equation 1), and average feed conversion ratio, Average FCR, in Equation (2). Average FCR is the amount of feed required per unit of weight gain; this was collected for Atlantic Salmon from Nøkland (2019), for Rainbow Trout from Renna et al. (2017), and for Whiteleg Shrimp from Cummins et al. (2017).
We then calculated the annual total metric tons (MT) of feed consumed ( ) for each of the three modeled aquaculture species produced commercially in the U.S. by multiplying (Equation 2) and the total annual MT of production in Equation (3). The total annual MT of production was averaged over a 3-year period (2017–2019) for Atlantic Salmon, Rainbow Trout, and Whiteleg Shrimp [Food and Agriculture Organization (FAO), 2021].
Output from Equation (3) includes intake of all ingredients that comprise a ration, including fish meal and fish oil. From this, we calculated the total annual MT of fish meal (Equation 4) and fish oil (Equation 5) required for commercial farmed fish production in the U.S. for each modeled species based on the inclusion rates of BSFL under each dietary replacement scenario i outlined in Table 1. Each dietary replacement scenario i was characterized by a specific combination of BSFL, fish meal, and fish oil for each of the three aquaculture species we modeled as implemented in previous research (Cummins et al., 2017; Renna et al., 2017; Nøkland, 2019). A dietary replacement scenario was only included if there was not a significant decrease in FCR, growth rate, or survival associated with that level of dietary BSFL substitution from previous literature.
In Equations (4) and (5), %FM and %FO are the percentages of fish meal and fish oil, respectively, in the diet of each farmed fish species under a dietary replacement scenario i. Based on previous literature, we included four dietary replacement scenarios for both Atlantic Salmon and Whiteleg Shrimp and three scenarios for Rainbow Trout.
For Atlantic Salmon, Nøkland (2019) evaluated four BSFL inclusion rates: 0, 8, 16, and 32% with corresponding fish meal inclusion rates of 25, 23, 22, and 18% and fish oil inclusion rates of 15, 13, 11, and 6%. Thus, dietary replacement scenarios 1–4 correspond with 0, 8, 16, and 32% of BSFL inclusion with the baseline being scenario 1 (i.e., 0% BSFL, 25% fish meal, and 15% fish oil). For Rainbow Trout, Renna et al. (2017) substituted fish meal with increasing BSFL at 0, 20, and 40% of dietary inclusion. As the level of BSFL increased in the diet, fish meal inclusion decreased from 60 to 30% and fish oil inclusion decreased from 9 to 5%. Thus, dietary replacement scenarios 1–3 correspond with 0, 20, and 40% of BSFL inclusion with the baseline being scenario 1 (i.e., 0% BSFL, 60% fish meal, and 9% fish oil). For Whiteleg Shrimp, the four BSFL inclusion scenarios from Cummins et al. (2017) were 0, 7, 14, and 21%. As the level of dietary BSFL increased, the level of fish meal decreased, from 25 to 10%. However, as dietary BSFL increased, fish oil also increased from 1 to 2.5%. Thus, dietary replacement scenarios 1–4 correspond with 0, 7, 14, and 21% of BSFL inclusion with the baseline being scenario 1 (i.e., 0% BSFL, 25% fish meal, and 1% fish oil).
The number of studies investigating BSFL as a feed substitute in aquaculture production has rapidly increased in recent years. We chose the above three studies based on their experimental design and dietary substitution rates. Specifically, each study substituted BSFL for fish meal and/or fish oil and reported FCR, fish growth, and survival. The BSFL inclusion rate in Nøkland (2019) ranges from 0 to 32% which is greater than the range of 4 to 15% in Mikołajczak et al. (2022) and Eide et al. (2024) for Atlantic Salmon. For Rainbow Trout, the BSFL inclusion rates were 15 and 30% in Bolton et al. (2021) and 0 to 15% in Caimi et al. (2021) which are narrower than that in Renna et al. (2017). For Whiteleg Shrimp, a narrow BSFL inclusion was used in Richardson et al. (2021) and salmon oil was utilized in Nunes et al. (2023).
Based on calculated in Equation (4) and in Equation (5) for each aquaculture fish species under a dietary replacement scenario i, we determined the total MT of pelagic fish needed to produce fish meal ( ) by dividing by 22.5%, the conversion rate of pelagic fish to fish meal [Food and Agriculture Organization (FAO), 1986; Equation 6], and the MT of pelagic fish needed to produce fish oil ( ) by dividing by 7.2%, the conversion rate of pelagic fish to fish oil (The Fish Site, 2011; Equation 7).
Fish used to produce fish meal and fish oil are sourced from both ocean catch and fresh fish scraps. In Equation (8), we calculated the MT of pelagic fish sourced from ocean catch that is used for FM production for each farm fish species under scenario i ( ) by multiplying Equation (6) with 71%, the percent of fish sourced from ocean catch for fish meal production (Boyd et al., 2022). Similarly, to determine the (Equation 9), we multiplied Equation (7) by 74%, the percent of fish sourced from ocean catch for fish oil production (Boyd et al., 2022).
Using the outcome from Equations (8) and (9) for each farmed fish species under scenario i, we calculated the MT of spared pelagic fish for each of the top 10 taxa commonly utilized in global fish meal and fish oil production (Cashion et al., 2017) in Equations (10) and (11). The MT of each taxa is calculated using the percentage the taxa ( ) multiplied by the total MT of ocean catch used to produce fish meal ( ) or fish oil ( ) under each scenario. Importantly, only 53% of taxa were defined by Cashion et al. (2017) with 47% categorized as “other”. Therefore, we represented the 53% defined taxa as 100% of those used for fish meal and fish oil production, removing the undefined “other” taxa.
As the same individual fish is used for concurrent fish meal and fish oil production (Ockerman and Basu, 2014), we took the greater of Equations (10) and (11) to represent the total MT of ocean catch by each pelagic fish taxa used as feed in aquaculture production (Equation 12).
We then calculated the MT of spared pelagic fish by taxa for each BSFL inclusion rate (scenarios j with j > 1) by subtracting the MT of pelagic fish sourced from ocean catch under scenarios j ( ) from that of the baseline scenario, for which there was 0% dietary BSFL inclusion ( ), in Equation (13).
3 Results and discussion
The objective of our study was to identify the amount of ocean catch pelagic fish that could be spared from feed production by integrating BSFL into the diets of aquaculture species commonly reared in the U.S. Specifically, we focused on Atlantic Salmon (Salmo salar), Rainbow Trout (Oncorhynchus mykiss), and Whiteleg Shrimp (Litopenaeus vannamei). We then developed a model that used the BSFL replacement scenarios from previous feeding trials where BSFL was substituted for dietary fish meal and/or fish oil without adversely affecting fish growth, FCR, or survival rate.
Our model included published estimates of fry weight, market weight, FCR, and annual output for each of the three farmed aquaculture species. Further, we included adjustments for the percent of fish meal and fish oil originating from ocean catch, rather than fresh fish scraps, and for the conversion rate from pelagic fish to fish meal or fish oil. Our model also assumed the same fish is dually used for fish meal and fish oil feed production, ensuring ‘double counting’ did not occur. Thus, we calculated the amount of the top ten pelagic fish taxa that would be spared from ocean catch when BSFL was substituted for fish meal and/or fish oil in the diet.
For the baseline replacement scenario (0% BSFL), 15,534 MT of pelagic fish would be required annually for fish meal production to support Atlantic Salmon farming in the U.S. (Table 2). At the maximum dietary replacement level (32% BSFL), 10,998 MT of pelagic fish would be required for fish meal production, a difference of 4,536 MT associated with integrating BSFL in the diet of Atlantic Salmon. These required amounts would be less than that required for fish oil production (Table 3), in which 30,314 MT would be required in the baseline scenario and 12,126 MT in the maximum dietary replacement scenario to support Atlantic Salmon farming. Therefore, the MT of pelagic fish used for fish oil, rather than fish meal, production was used in our final calculation.
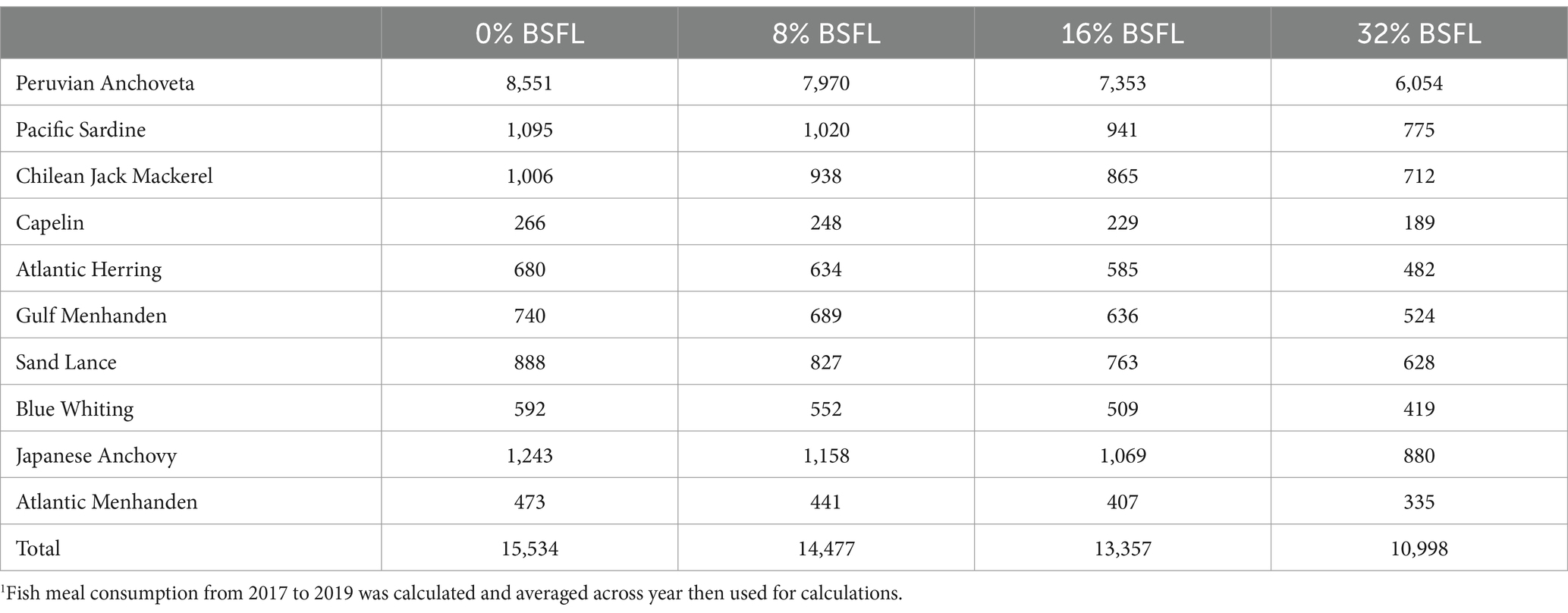
Table 2. Metric tons of pelagic fish from ocean catch used for fish meal with varying inclusions of Black Soldier Fly larvae (BSFL) in the diet of Atlantic Salmon (Salmo salar)1.
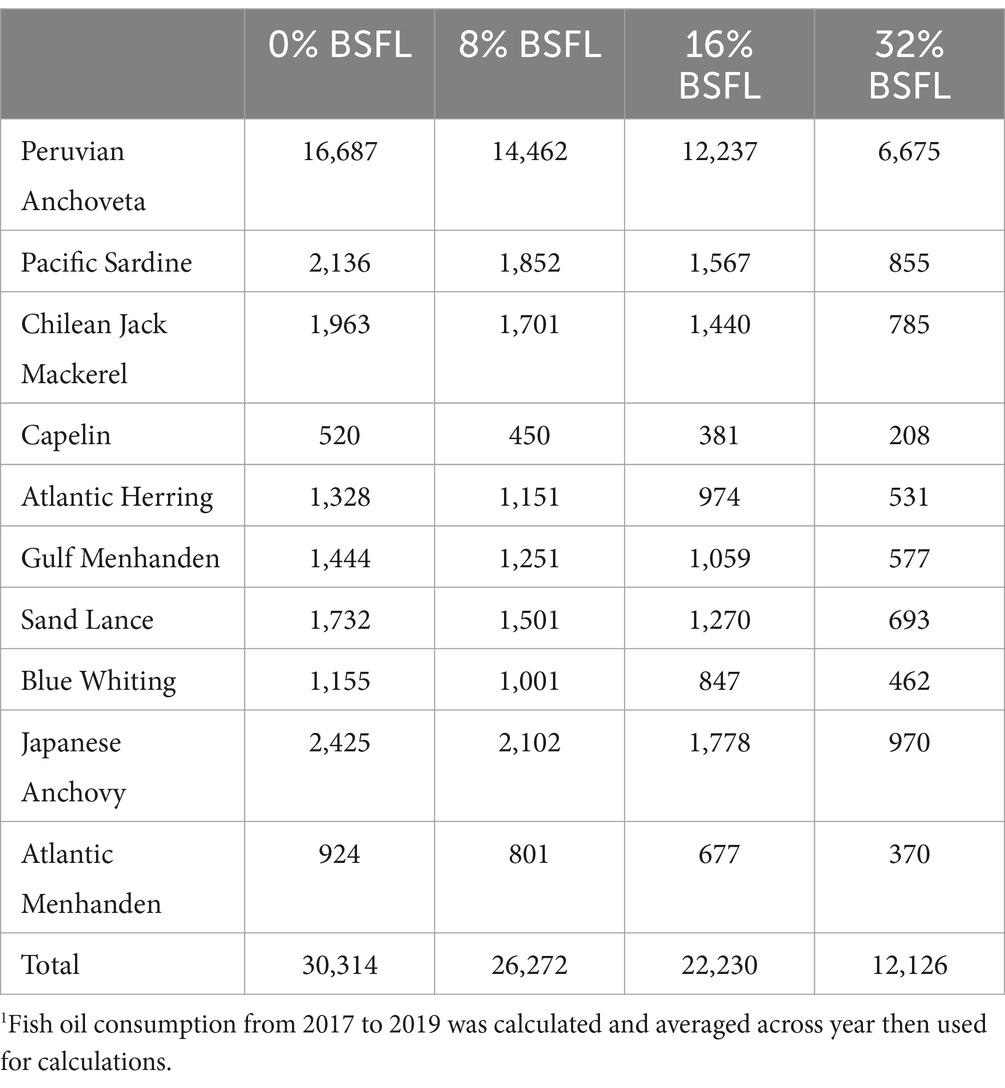
Table 3. Metric tons of pelagic fish from ocean catch used for fish oil with varying inclusions of Black Soldier Fly larvae (BSFL) in the diet of Atlantic Salmon (Salmo salar)1.
At an 8% dietary inclusion rate of BSFL, 4,042 MT of pelagic fish could be spared from ocean catch annually with potential to spare 18,188 MT annually at the maximum dietary inclusion rate, 32%. Most of the spared pelagic fish would be Peruvian Anchoveta, as this taxon is the most heavily utilized for fish meal and fish oil production, followed by Pacific Sardine (Cashion et al., 2017).
To support Rainbow Trout production in the U.S., 42,595 MT of pelagic fish would be required for fish meal production annually (Table 4) and 20,783 MT for fish oil (Table 5) under the baseline replacement scenarios, for which 0% BSFL was included in the diet. These estimates are more than for Atlantic Salmon, likely because the annual output of Rainbow Trout is greater than that of Atlantic Salmon in the U.S., but also because of the dietary inclusion levels of fish meal that were used in the feeding trials included in our model. Specifically, Nøkland (2019) included 25% of fish meal in the baseline scenario for Atlantic Salmon while Renna et al. (2017) included 60% of fish meal. We assume that the diets evaluated in these feeding trials accurately reflect those implemented in aquaculture production.
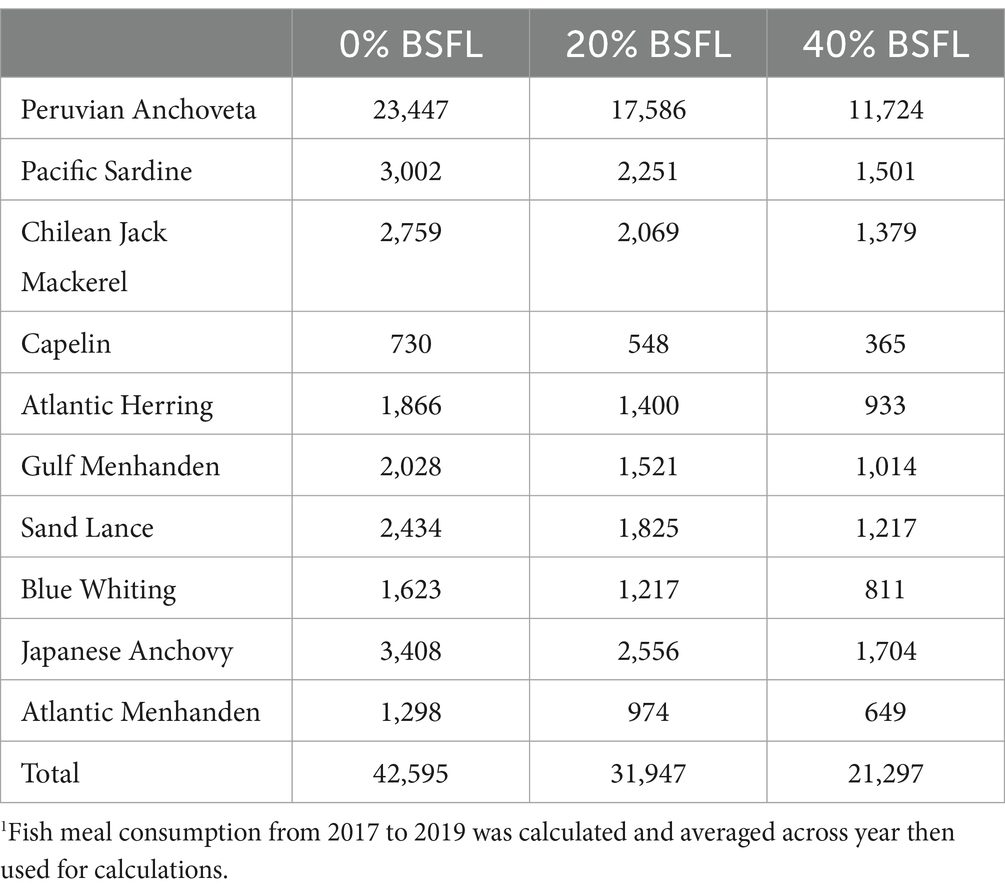
Table 4. Metric tons of pelagic fish from ocean catch used for fish meal with varying inclusions of Black soldier fly larvae (BSFL) in the diet of Rainbow trout (Oncorhynchus mykiss)1.
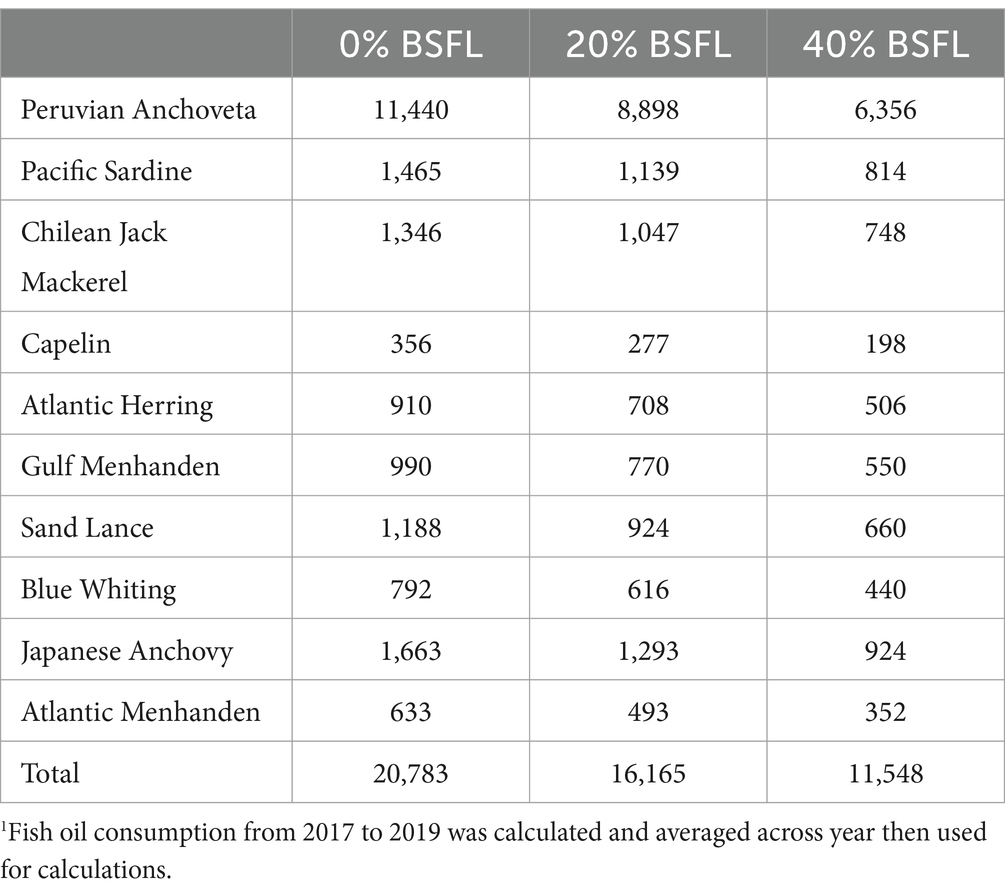
Table 5. Metric tons of pelagic fish from ocean catch used for fish oil with varying inclusions of Black soldier fly larvae (BSFL) in the diet of Rainbow trout (Oncorhynchus mykiss)1.
When including BSFL in the diet of Rainbow Trout at 20% or 40%, the MT of pelagic fish required for fish meal production was greater than the MT of pelagic fish required for fish oil production. Therefore, we used the requirements for fish meal production in our final calculation. By including BSFL in the diet of Rainbow Trout at a 20 and 40% inclusion rate, 10,648 and 21,298 MT of pelagic fish could be spared from ocean catch, respectively.
In the baseline scenario for Whiteleg Shrimp (i.e., 0% of BSFL in the diet), 2,265 MT of pelagic fish would be required for fish meal (Table 6) and 295 MT would be required for fish oil production every year (Table 7). Therefore, we used the required amounts of pelagic fish sourced from ocean catch for production of fish meal, rather than fish oil, in our final calculation of spared ocean catch fish associated with integrating BSFL in Whiteleg Shrimp production.
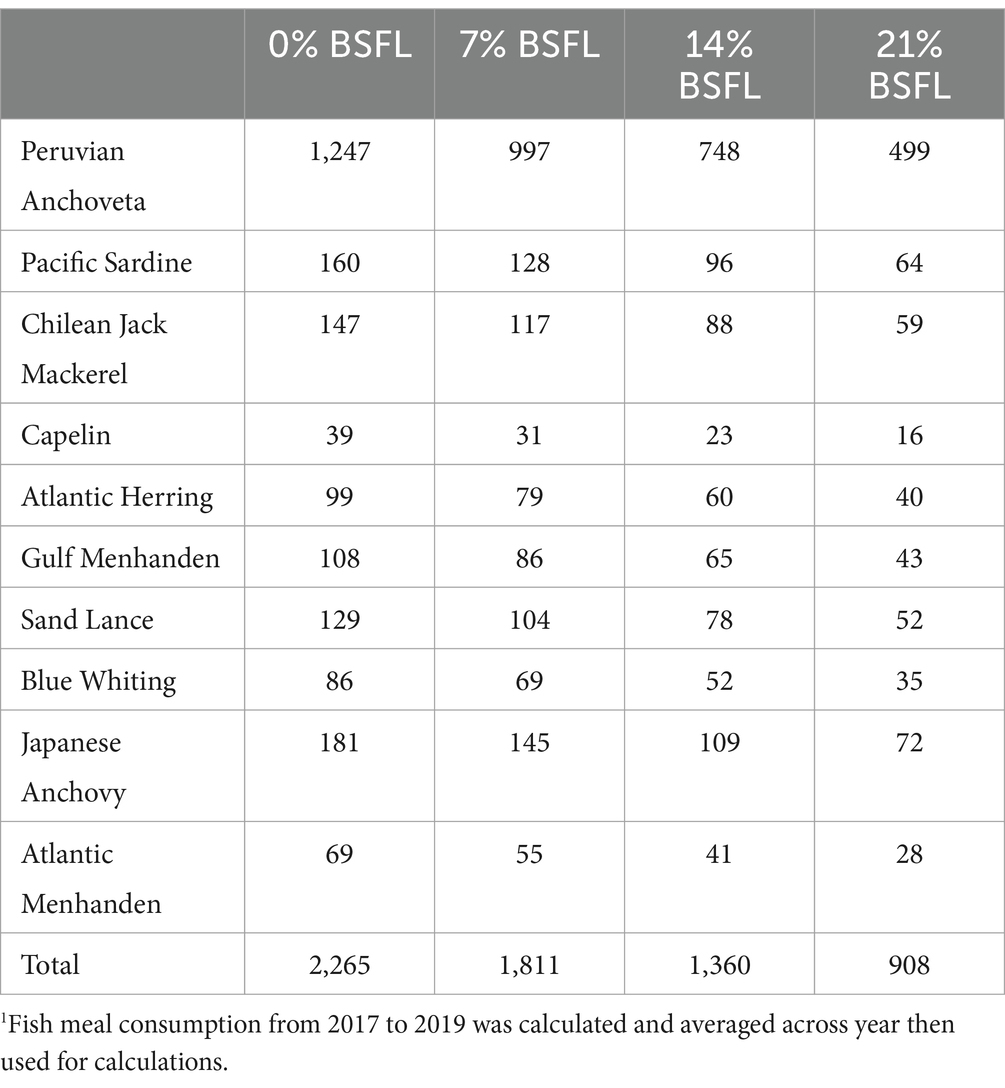
Table 6. Metric tons of pelagic fish from ocean catch used for fish meal with varying inclusions of Black soldier fly larvae (BSFL) in the diet of Whiteleg shrimp (Litopenaeus vannamei)1.
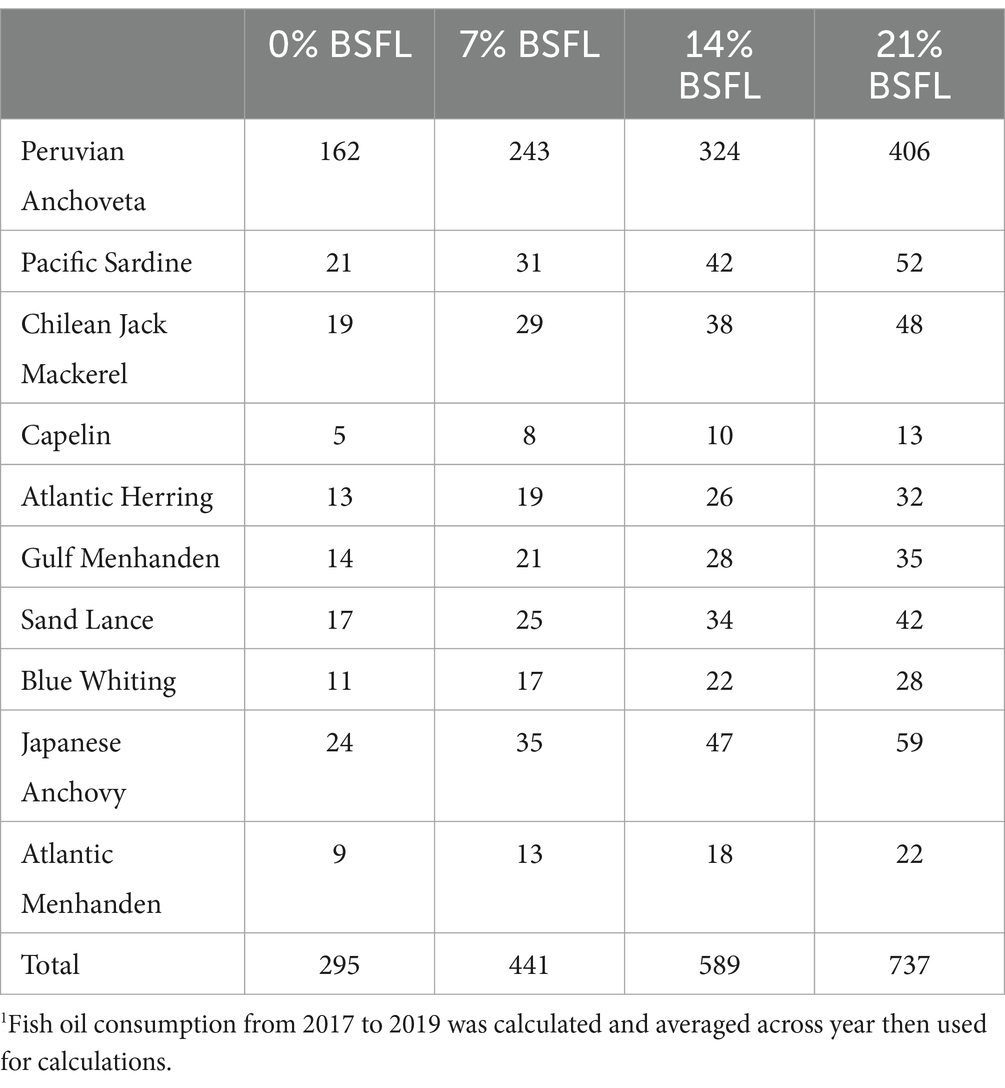
Table 7. Metric tons of pelagic fish from ocean catch used for fish oil with varying inclusions of Black soldier fly larvae (BSFL) in the diet of Whiteleg shrimp (Litopenaeus vannamei)1.
In our dietary substitution scenarios for Whiteleg Shrimp, we modeled 7, 14, and 21% dietary inclusion of BSFL. At 7% dietary inclusion of BSFL, 454 MT of pelagic fish would be spared from ocean catch; at 14% inclusion, 905 MT would be spared; and at 21%, 1,357 MT would be spared. Therefore, there is less potential for BSFL to spare pelagic fish from ocean catch when integrated in Whiteleg Shrimp diets compared to Atlantic Salmon or Rainbow Trout, likely due to the size and intake of Whiteleg Shrimp versus other farmed aquaculture species.
By integrating BSFL in the diets of three major farmed fish species in the U.S. (i.e., Atlantic Salmon, Rainbow Trout, and Whiteleg Shrimp) at the maximum dietary levels that did not sacrifice fish performance, there is potential to spare approximately 41,000 MT of ocean catch pelagic fish from fish meal and fish oil production (Table 8). Based on aquaculture production from the Food and Agriculture Organization (FAO) (2021), the three farmed fish species in our study account for approximately 14% of all farmed carnivorous fish and crustaceans in the U.S. Therefore, the total spared pelagic fish could be greater than the amount estimated in our study.
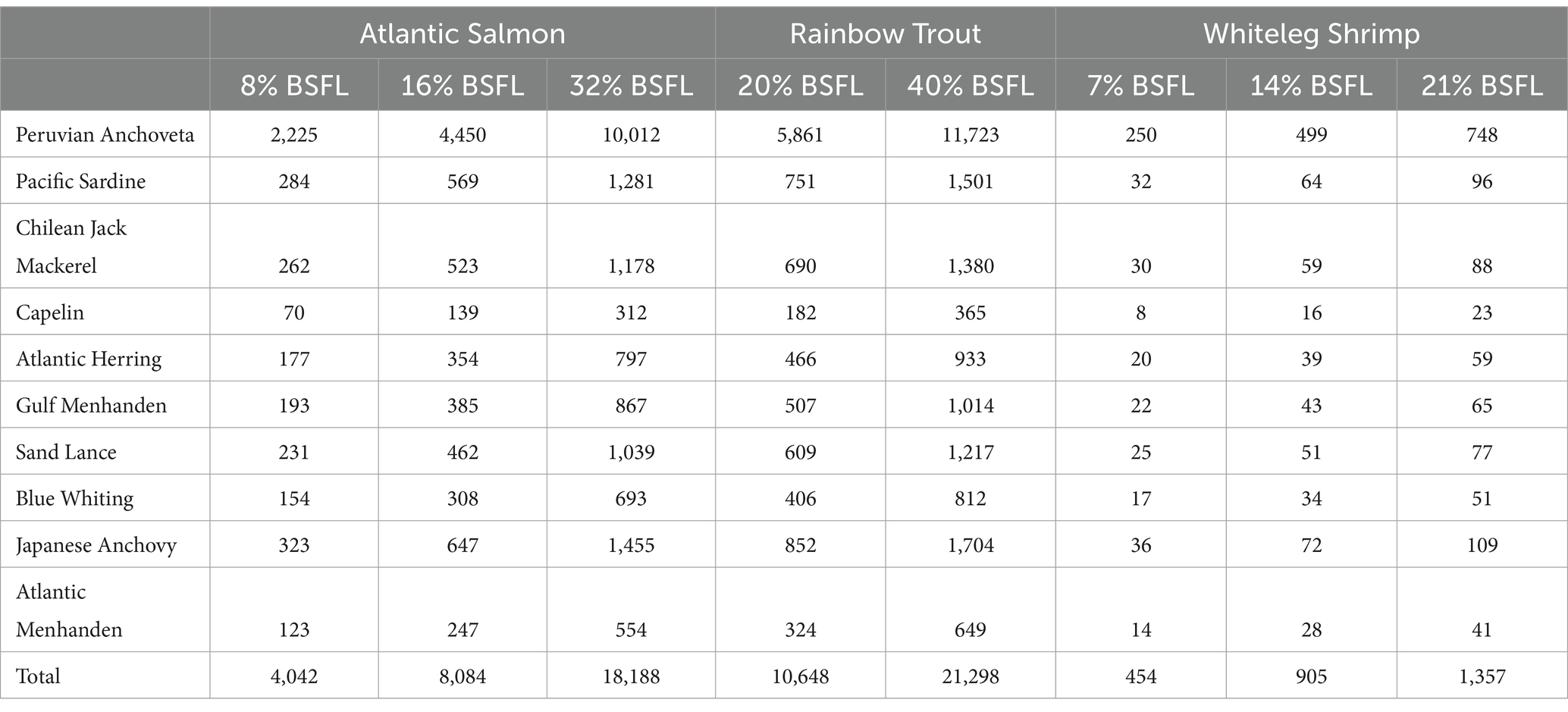
Table 8. Metric tons of pelagic fish spared from ocean catch with varying inclusions of Black soldier fly larvae (BSFL).
Alder et al. (2008) estimated 20–30 million MT of fish are used to produce fish meal and fish oil globally every year. More recent estimates from the Food and Agriculture Organization (FAO) (2022) indicated that 17.7 million MT of finfish from ocean catch were reduced to fish meal and fish oil in 2018, representing 80% of finfish use for non-food purposes. The aquaculture industry is the major global consumer of fish meal and fish oil; other industries have historically utilized fish meal, although estimates are not well established. Malherbe (2005) estimated that fish meal and fish oil were utilized by the swine and poultry industries at 24 and 22% of global production, respectively, whereas the >Food and Agriculture Organization (FAO) (2015) estimated a reduced utilization of fish meal for both industries, 20% for swine and 5% for poultry. To have an impact on pelagic fish populations that translates to positive ecological, economic, and/or environmental outcomes, BSFL will likely need to be integrated into the diets of many farmed carnivorous fish species across several major aquaculture-producing countries.
The two most utilized pelagic fish taxa for fish meal and fish oil are the Peruvian Anchoveta and the Pacific Sardine (Cashion et al., 2017). Although populations of the Peruvian Anchoveta have rebounded or been compensated for by other pelagic fish species (Bakun et al., 2010), their biomass and carrying capacity are sensitive to environmental conditions (Bertrand et al., 2011) and climate change can severely reduce the maximum catch potential (Lam et al., 2016). As 98% of anchoveta landings are used to produce fish meal and fish oil, the use of pelagic fish for this production exacerbates the already severe children malnourishment situation in the Amazon and Andes regions and results in over-fishing (Majluf et al., 2017). Similarly, populations of the Pacific Sardine almost collapsed in 1950 due to a combination of worsening environmental conditions and over-fishing (Barnes et al., 1992; Baumgartner et al., 1992). The sardine population recovered temporarily in the 1990s but began to decline again in the mid-2000s until a major fishery was closed in 2015 (Pacific Fishery Management Council, 2017). These incidences of population collapse are concerning given the many ecosystem services that pelagic fish provide, recently reviewed by Nissar et al. (2022).
Our data indicate that approximately 22,500 MT of Peruvian Anchoveta and 2,900 MT of Pacific Sardine could be spared through utilizing BSFL in place of fish meal and/or fish oil in our modeled fish species, which could mitigate future population collapses. However, our findings could have over-emphasized the potential sparing effect of integrating BSFL as aquaculture feed on a given taxon because approximately half of the taxa of pelagic fish used for fish meal and fish oil production are not known or defined (Cashion et al., 2017) and we represented the top 10 known taxa out of 100%.
In the last 15 years, insect farming has emerged as a rapidly scaling branch of agriculture; by 2030, the estimated output for the E.U. is 250,000 MT annually (International Platform of Insects for Food and Feed (IPIFF), 2020). In the U.S., a BSFL-rearing facility was recently developed with an estimated annual output of 60,000 MT of insect protein and 20,000 MT of insect oil (Carpenter, 2020). While the cost of BSFL and other insect proteins is not currently known and/or cannot compete with conventional feeds, availability is expected to increase with concomitant decreases in cost. Further, E.U. and U.S. legislation approves of the use of insect-derived feed materials in aquaculture production (Official Journal of the European Union (EU), 2017; Association of American Feed Control Officials (AAFCO), 2021), although the E.U. is still investigating the safety of BSFL (Liguori et al., 2022). Ultimately, it is expected that adequate volumes of BSFL will soon be available for integration in the aquaculture industry which should have positive sustainability benefits, given our data.
It is worth noting that, in the feeding trials we based our model on, diets were isonitrogenous, but not isoenergetic, and BSFL was substituted for fish meal and/or fish oil. For Atlantic Salmon (Nøkland, 2019) and Rainbow Trout (Renna et al., 2017), dietary inclusion of both fish meal and fish oil decreased in accordance with increasing BSFL; however, for Whiteleg Shrimp, inclusion of fish meal decreased and fish oil increased with increasing BSFL (Cummins et al., 2017). It is unclear why certain researchers replaced fish oil with BSFL (Renna et al., 2017; Nøkland, 2019) while others increased fish oil in accordance with BSFL (Cummins et al., 2017). While the nutritional value of BSFL depends on the larval diet, it regularly contains 35% fat (Drewery et al., 2022), indicating it has potential to simultaneously displace both dietary fish meal and fish oil if it is not defatted. However, the fatty acid profile of BSFL is predominantly saturated, especially in medium-chain fats, while fish oil contains significant amounts of omega-3 polyunsaturated fatty acids that are not synthesized de novo and are, thus, a dietary requirement of many aquaculture species. It is likely that BSFL has more value to aquaculture production as a replacement for protein-rich fish meal, rather than fish oil.
Our data are limited in that we assumed the diets evaluated in the three feeding trials we based on model on reflect those implemented in aquaculture production across the entire lifespan of each fish species. Additional data were not available with parameters necessary for our model (i.e., farmed fish species relevant to U.S. aquaculture, replacement of fish meal and/or fish oil with BSFL, reported fish growth and FCR) and not across different life stages (i.e., juvenile and adult). Further, while we collected data that are important for efficient aquaculture production (i.e., FCR, fish growth, and survival rate), the reported outcomes may not be applied across all production scenarios. For example, while survival rate was not different across dietary treatment groups in previous research we based our model on (Cummins et al., 2017; Renna et al., 2017; Nøkland, 2019), other studies document changes in survival, sometimes favorable, associated with BSFL supplementation to other farmed aquaculture species (Alvanou et al., 2023).
We encourage further in vivo feeding trials of BSFL across different aquaculture species and life stages with attention to growth and FCR. As these data become available, they can be evaluated through the model described here to estimate the potential impact of using BSFL as a dietary substitute to spare ocean catch pelagic fish. We also encourage the development of a similar model as ours for other livestock industries that are major consumers of fish meal and fish oil (i.e., swine, poultry). Finally, a cost–benefit analysis should be conducted, focusing on the net benefit of BSFL substitution for conventional protein feeds in livestock diets.
Data availability statement
The raw data supporting the conclusions of this article will be made available by the authors, without undue reservation.
Author contributions
EM: Data curation, Investigation, Writing – original draft. XL: Conceptualization, Investigation, Methodology, Resources, Supervision, Writing – original draft, Writing – review & editing. MD: Conceptualization, Funding acquisition, Methodology, Project administration, Supervision, Writing – original draft, Writing – review & editing.
Funding
The author(s) declare that financial support was received for the research, authorship, and/or publication of this article. The authors acknowledge funding from United States Department of Agriculture (USDA NIFA AFRI 2020-02682) Hispanic Serving Institutions program (grant 2020-01979).
Conflict of interest
The authors declare that the research was conducted in the absence of any commercial or financial relationships that could be construed as a potential conflict of interest.
Publisher’s note
All claims expressed in this article are solely those of the authors and do not necessarily represent those of their affiliated organizations, or those of the publisher, the editors and the reviewers. Any product that may be evaluated in this article, or claim that may be made by its manufacturer, is not guaranteed or endorsed by the publisher.
References
Alder, J., Campbell, B., Karpouzi, K., Kaschner, K., and Pauly, D. (2008). Forage fish: from ecosystems to markets. Ann. Rev. Environ. Res. 33, 153–166. doi: 10.1146/annurev.environ.33.020807.143204
Alvanou, M. V., Kyriakoudi, A., Makri, V., Lattos, A., Feidantsis, K., Papadopoulos Dimitrios, K., et al. (2023). Effects of dietary substitution of fishmeal by black soldier fly (Hermetia illucens) meal on growth performance, whole-body chemical composition, and fatty acid profile of Pontastacus leptodactylus juveniles. Front. Physiol. 14:1156394. doi: 10.3389/fphys.2023.1156394
Association of American Feed Control Officials (AAFCO). (2021). Ingredient definitions committee report. AAFO, Champaign, IL, US.
Bakun, A., Babcock, E. A., Lluch-Cota, S. E., Santora, C., and Salvadeo, C. J. (2010). Issues of ecosystem-based management of forage fisheries in “open” non-stationary ecosystems: the example of the sardine fishery in the Gulf of California. Rev. Fish Biol. Fish. 20, 9–29. doi: 10.1007/s11160-009-9118-1
Barnes, J. T., MacCall, A. D., Jacobson, L. D., and Wolf, P. (1992). Recent population trends and abundance estimates for the Pacific sardine (Sardinops sagax). CalCOFI Rep. 33, 60–75.
Baumgartner, T. R., Soutar, A., and Ferreira-Bartrina, V. (1992). Reconstruction of the history of Pacific sardine and northern anchovy of the past two millennia from sediments of the Santa Barbara Basin California. CalCOFI Rep. 33, 24–40.
Bertrand, A., Chaigneau, A., Peraltilla, S., Ledesma, J., Graco, M., Monetti, F., et al. (2011). Oxygen: a fundamental property regulating pelagic ecosystem structure in the coastal southeastern tropical Pacific. PLoS One 6:e29558. doi: 10.1371/journal.pone.0029558
Bolton, C. M., Muller, N., Hyland, J., Johnson, M. P., Souza Valente, C., Davies, S. J., et al. (2021). Black soldier fly larval meal with exogenous protease in diets for rainbow trout (Oncorhynchus mykiss) production meeting consumer quality. J. Agric. Food Res. 6:100232. doi: 10.1016/j.jafr.2021.100232
Boyd, C. E., McNevin, A. A., and Davis, R. P. (2022). The contribution of fisheries and aquaculture to the global protein supply. Food Sec. 14, 805–827. doi: 10.1007/s12571-021-01246-9
Caimi, C., Biasato, I., Chemello, G., Oddon, S. B., Lussiana, C., Malfatto, V. M., et al. (2021). Dietary inclusion of a partially defatted black soldier fly (Hermetia illucens) larva meal in low fishmeal-based diets for rainbow trout (Oncorhynchus mykiss). J. Anim. Sci. Biotechnol. 12, 1–15. doi: 10.1186/s40104-021-00575-1
Carpenter, S. (2020). World’s largest insect protein farm signals future of food supply. Jersey City, NJ, Unites States: Forbes.
Cashion, T., le Manach, F., Zeller, D., and Pauly, D. (2017). Most fish destined for fishmeal production are food-grade fish. Fish and Fisher. 18, 837–844. doi: 10.1111/faf.12209
Cummins, V., Rawles, S., Thompson, K., Velasquez, A., Kobayashi, Y., Hager, J., et al. (2017). Evaluation of black soldier fly (Hermetia illucens) larvae meal as partial or total replacement of marine fish meal in practical diets for Pacific white shrimp (Litopenaeus vannamei). Aquac. 473, 337–344. doi: 10.1016/j.aquaculture.2017.02.022
Daniel, N. (2018). A review on replacing fish meal in aqua feeds using plant protein sources. Int. J. Fish. Aqua. Studies 6, 164–179.
Drewery, M. L., Liu, X., and Wickersham, T. A. (2022). Black soldier fly larvae (BSFL) as a feed for beef cattle: a hedonic pricing model. J. Ins. Food Feed 8, 743–751. doi: 10.3920/JIFF2021.0166
Eide, H. L., Rocha, S. D. C., Morales-Lange, B., Kuiper, R. V., Dale, O. B., Djordjevic, B., et al. (2024). Black soldier fly larvae (Hermetia illucens) meal is a viable protein source for Atlantic salmon (Salmo salar) during a large-scale controlled field trial under commercial-like conditions. Aquac. 579:740194. doi: 10.1016/j.aquaculture.2023.740194
Essington, T. E., Moriarty, P. E., Froehlich, H. E., Hodgson, E. E., Koehn, L. E., Oken, K. L., et al. (2015). Fishing amplifies pelagic fish population collapses. Proc. Nat. Acad. Sci. U.S.A. 112, 6648–6652. doi: 10.1073/pnas.1422020112
Food and Agriculture Organization (FAO). (1986). The production of fish meal and oil, FAO fisheries technical paper – 142. Rome: FAO of the United Nations.
Food and Agriculture Organization (FAO). (2015). Summary tables of fishery statistics: Capture, aquaculture, commodity and food balance sheets. Rome: FAO of the United Nations.
Food and Agriculture Organization (FAO). (2021). FAO yearbook. Fishery and aquaculture statistics 2019. FAO of the United Nations, Rome: FAO of the United Nations.
Food and Agriculture Organization (FAO). (2022). The state of world fisheries and aquaculture 2022. Sustainability in action. Rome: FAO of the United Nations
Food and Agriculture Organization (FAO). (2023). Cultured aquatic species fact sheets: Penaeus vannamei. Rome: FAO of the United Nations.
Goyal, S., Ott, D., Liebscher, J., Höfling, D., Müller, A., Dautz, J., et al. (2021). Sustainability analysis of fish feed derived from aquatic plant and insect. Sustain. For. 13:7371. doi: 10.3390/su13137371
International Platform of Insects for Food and Feed (IPIFF). (2020). Edible insects on the European market. Brussels, Belgium: IPIFF.
Konar, M., Qiu, S., Tougher, B., Vause, J., Tlusty, M., Fitzsimmons, K., et al. (2019). Illustrating the hidden economic, social and ecological values of global forage fish resources. Res. Conserv. Recycl. 151:104456. doi: 10.1016/j.resconrec.2019.104456
Lam, V. W. Y., Cheung, W. L. W., Reygondeau, G., and Sumaila, R. U. (2016). Projected change in global fisheries revenues under climate change. Sci. Rep. 6:32607. doi: 10.1038/srep32607
Liguori, B., Sancho, A. I., Poulsen, M., and Lindholm Bøgh, K. (2022). Novel foods: Allergenicity assessment of insect proteins. EFSA J. 20:e200910. doi: 10.2903/j.efsa.2022.e200910
Little, D., Newton, R., and Beveridge, M. (2016). Aquaculture: a rapidly growing and significant source of sustainable food? Status, transitions and potential. Proc. Nutr. Soc. 75, 274–286. doi: 10.1017/S0029665116000665
Luthada-Raswiswi, R., Mukaratirwa, S., and O’Brien, G. (2021). Animal protein sources as a substitute for fish meal in aquaculture diets: a systematic review and meta-analysis. Appl. Sci. 11:3854. doi: 10.3390/app11093854
Maine Aquaculture Innovation Center. (2023). Atlantic salmon. Available at: https://www.maineaquaculture.org/atlantic-salmon/ (Accessed July 27 2023).
Majluf, P., De la Puente, S., and Christensen, V. (2017). The little fish that can feed the world. Fish Fisheries 18, 772–777. doi: 10.1111/faf.12206
Malcorps, W., Kok, B., van‘t Land, M., Fritz, M., van Doren, D., Servin, K., et al. (2019). The sustainability conundrum of fish meal substitution by plant ingredients in shrimp feeds. Sustain. For. 11:1212. doi: 10.3390/su11041212
Malherbe, S. (2005). The world market for fishmeal. International Fishmeal Fish Oil Organization (IFFO). Proceedings of World Pelagic Conference, Cape town, South Africa. Tunbridge Wells, United Kingdom.
Mikołajczak, Z., Mazurkiewicz, J., Rawski, M., Kierończyk, B., Józefiak, A., Świątkiewicz, S., et al. (2022). Black soldier fly full-fat meal in Atlantic salmon nutrition – part a: effects on growth performance, feed utilization, selected nutriphysiological traits and production sustainability in fries. Annals Anim. Sci. doi: 10.2478/aoas-2022-0070
National Oceanic and Atmospheric Administration (NOAA). (2023). Feeds for aquaculture. Washington D.C., United States: NOAA.
Nissar, S., Bakhtiyar, Y., Arafat, M. Y., Andrabi, S., Bhat, A. A., and Yousuf, T. (2022). A review of the ecosystem services provided by the marine forage fish. Hydrobiology 850, 2871–2902. doi: 10.1007/s10750-022-05033-1
Nøkland, D. (2019). Black soldier fly larvae (acid conserved or dry meal) in extruded salmon diets: Effects on feed processing, pellet quality, growth, and nutrient digestibility. Master's thesis, Norwegian University of Life Sciences, Ås, Norway.
Northrop, E., Schuhmann, P.W., Burke, L., Fyall, A., Alvarez, S., Spenceley, A., et al. (2022). Opportunities for transforming coastal and marine tourism: towards sustainability, regeneration and resilience. High level panel for a Sustainable Ocean economy. Available at: https://oceanpanel.org/opportunity/sustainable-coastal-marine-tourism/ (Accessed May 16 2023).
Nunes, A. J. P., Yamamoto, H., Simões, J. P., Pisa, J. L., Miyamoto, N., and Leite, J. S. (2023). The black soldier Fly (Hermetia illucens) larvae meal can cost-effectively replace fish meal in practical nursery diets for post-larval Penaeus vannamei under high-density culture. Aust. Fish. 8:605. doi: 10.3390/fishes8120605
Ockerman, H. W., and Basu, L. (2014). “Inedible” in Encyclopedia of meat sciences. eds. C. Dikemann and C. Devine. 2nd Edn. (London, UK: Elsevier Science Ltd.)
Official Journal of the European Union (EU), (2017). Commission regulation (EU) 2017/893. Available at: https://eur-lex.europa.eu/legal-content/EN/TXT/PDF/?uri=CELEX:32017R0893&from=EN (accessed on 19 January 2021).
Pacific Fishery Management Council. (2017). Status of the Pacific coast coastal pelagic species fishery and recommended acceptable biological catches. Portland, OR: Pacific Fishery Management Council
Parodi, A., De Boer, I., Gerrits, W., Van Loon, J., Heetkamp, M., Van Schelt, J., et al. (2020). Bioconversion efficiencies, greenhouse gas and ammonia emissions during black soldier fly rearing – a mass balance approach. J. Clean. Prod. 271:122488. doi: 10.1016/j.jclepro.2020.122488
Priyadarshana, M. K. C., Walpita, C. N., Naveenan, M., Magamage, M. P. S., and Ruwandeepika, H. A. D. (2021). Substitution of fish meal with black soldier Fly Hermetia illucens Linnaeus, 1758 larvae in finfish aquaculture–a review. Asian Fish. Sci. 34, 114–126.
Renna, M., Schiavone, A., Gai, F., Dabbou, S., Lussiana, C., Malfatto, V., et al. (2017). Evaluation of the suitability of a partially defatted black soldier fly (Hermetia illucens L.) larvae meal as ingredient for rainbow trout (Oncorhynchus mykiss Walbaum) diets. J. Anim. Sci. Biotech 8:57. doi: 10.1186/s40104-017-0191-3
Richardson, A., Dantas-Lima, J., Lefranc, M., and Walraven, M. (2021). Effect of a black soldier Fly ingredient on the growth performance and disease resistance of juvenile Pacific white shrimp (Litopenaeus vannamei). Animals 11:1450. doi: 10.3390/ani11051450
Siddiqui, S. A., Ristow, B., Rahayu, T., Putra, N. S., Yuwono, N. W., Nisa', K., et al. (2022). Black soldier fly larvae (BSFL) and their affinity for organic waste processing. Waste Manag. 140, 1–13. doi: 10.1016/j.wasman.2021.12.044
Siple, M. C., Essington, T. E., and Plagányi, É. E. (2019). Forage fish fisheries management requires a tailored approach to balance trade-offs. Fish Fisher. 20, 110–124. doi: 10.1111/faf.12326
Stankus, A. (2021). State of world aquaculture 2020 and regional reviews: food and agriculture organization (FAO) webinar series. FAO Aquacult. Newslett. 63, 17–18.
The Fish Site. (2011). The fish feed story. The Fish Site, Ireland. Available at: https://thefishsite.com/articles/the-fish-feed-story (Accessed April 24 2023).
United Nations Department of Economic and Social Affairs. (2019). World population prospects: the 2017 revision. Available at: https://www.un.org/en/desa/world-population-projected-reach-98-billion-2050-and-112-billion-2100 (Accessed June 3 2023).
Wang, S., Cheng, L., and Liu, X. (2022). Comparative study on the carbon footprints of extruded and pelleted feed and their potential for carbon reduction: a case study of grass carp feed. J. Clean. Prod. 381:135192. doi: 10.1016/j.jclepro.2022.135192
Keywords: sustainability, alternative feeds, forage fish, fish meal, fish oil, climate change
Citation: Moore E, Liu X and Drewery ML (2024) Pelagic fish spared from ocean catch by integrating Black Soldier Fly Larvae in U.S. aquaculture production. Front. Sustain. Food Syst. 8:1297414. doi: 10.3389/fsufs.2024.1297414
Edited by:
Roberto Anedda, Porto Conte Ricerche, Parco Scientifico e Tecnologico della Sardegna, ItalyReviewed by:
Miguel A. Olvera-Novoa, CINVESTAV - Marine Resources Dept., MexicoIoannis A. Giantsis, Aristotle University of Thessaloniki, Greece
Copyright © 2024 Moore, Liu and Drewery. This is an open-access article distributed under the terms of the Creative Commons Attribution License (CC BY). The use, distribution or reproduction in other forums is permitted, provided the original author(s) and the copyright owner(s) are credited and that the original publication in this journal is cited, in accordance with accepted academic practice. No use, distribution or reproduction is permitted which does not comply with these terms.
*Correspondence: Merritt L. Drewery, m_d553@txstate.edu