Crop–livestock-integrated farming system: a strategy to achieve synergy between agricultural production, nutritional security, and environmental sustainability
- 1Department of Agronomy, Tamil Nadu Agricultural University, Coimbatore, Tamil Nadu, India
- 2Water Technology Centre, Tamil Nadu Agricultural University, Coimbatore, Tamil Nadu, India
- 3Indian Council of Agricultural Research - Krishi Vigyan Kendra, Tamil Nadu Agricultural University, Coimbatore, Tamil Nadu, India
- 4Project Coordination Unit, Indian Council of Agricultural Research-Indian Institute of Farming Systems Research, Modipuram, Uttar Pradesh, India
- 5Agricultural Research Station, Tamil Nadu Agricultural University, Coimbatore, Tamil Nadu, India
- 6Maize Research Station, Tamil Nadu Agricultural University, Coimbatore, Tamil Nadu, India
- 7Tapioca and Castor Research Station, Tamil Nadu Agricultural University, Coimbatore, Tamil Nadu, India
- 8Division of Animal Nutrition, Indian Council of Agricultural Research-National Institute of Animal Nutrition and Physiology, Bengaluru, India
Introduction: Climate change, nutritional security, land shrinkage, and an increasing human population are the most concerning factors in agriculture, which are further complicated by deteriorating soil health. Among several ways to address these issues, the most prominent and cost-effective means is to adopt an integrated farming system (IFS). Integrating farming systems with livestock enables a way to increase economic yield per unit area per unit of time for farmers in small and marginal categories. This system effectively utilizes the waste materials by recycling them via linking appropriate components, thereby minimizing the pollution caused to the environment. Further integrating livestock components with crops and the production of eggs, meat, and milk leads to nutritional security and stable farmer's income generation. So, there is a dire need to develop an eco-friendly, ecologically safe, and economically profitable IFS model.
Methods: An experiment was conducted to develop a crop–livestock-based integrated farming system model for the benefit of irrigated upland farmers in the semi-arid tropics for increasing productivity, farm income, employment generation, and food and nutritional security through efficient utilization of resources in the farming system.
Results and discussion: The IFS model has components, viz., crop (0.85 ha) + horticulture (0.10 ha) + 2 cattles along with 2 calves in dairy (50 m2) + 12 female goats and 1 male goat (50 m2) + 150 numbers of poultry birds (50 m2) + vermicompost (50 m2) + kitchen garden (0.02 ha) + boundary planting + supporting activities (0.01 ha) in a one-hectare area. The model recorded a higher total MEY (162.31 t), gross return (689,773), net return (317,765), and employment generation (475 mandays). Further negative emissions of −15,118 CO2-e (kg) greenhouse gases were recorded under this model. The study conclusively reveals that integration of crop, horticulture, dairy, goat, poultry, vermicompost production, kitchen garden, and boundary planting models increases the net returns, B:C ratio, employment generation, nutritional security, and livelihoods of small and marginal farmers.
1 Introduction
Agriculture and livestock are the key contributors to a country's economy and agricultural occupation. However, changing climate and erratic distribution of rainfall negatively affect the agriculture and livestock sectors. Since the 1980s, climate change has already reduced crop yields by about 5%−10% in notable areas (Iizumi and Ramankutty, 2016). The overall production of maize, rice, wheat, and soya is projected to fall to 9% by 2030 and 23% by 2050 worldwide (Haile et al., 2017). Major coastal areas with their high levels of agriculture are currently prone to be mainly affected; this includes Bangladesh and Vietnam, and drought-prone locations such as large parts of East and West Africa, Morocco, and parts of South Asia and China. This not only damages agriculture but also has a huge economic impact since climate change leads to fluctuating food prices, which have an indirect negative effect on production as these fluctuations signal risk and reduce incentives to invest in agriculture. At present, employment in agriculture has been drastically reduced, and there is practically no scope for horizontal growth of land for food production; which is mostly owing to the ever-increasing human population and diminishing land resources. To ensure that farm households receive a sufficient amount of money on a regular basis, only vertical expansion is feasible by integrating appropriate farming components that demand less area and time. Further global warming is a major threat to humans for sustainable food production. Approximately 20% of the yearly contribution of carbon dioxide (CO2), methane (CH4), and nitrous oxide (N2O) gas emissions is from the agriculture sector. So, it is necessary to develop alternative strategies to mitigate GHG emissions as well as to improve the production and productivity of crops. Studies conducted in Indonesia on reducing greenhouse gas emissions by adopting IFS practices also show promising results (Munandar et al., 2015).
Integrated farming system (IFS) with livestock is one of the traditional practices in rural India among farmers of small and medium categories. It is very important for farmers to diversify their crops with dairy, goatery, fishery, poultry, duckery, and so on to increase income (Ray et al., 2012). The holdings should be evenly distributed compared to land holdings among the farmers (Kochewad et al., 2017). An IFS places an emphasis on recycling farm wastes and reducing the hurdles of their management in an effective and useful way. This can be achieved by making various components of the farming system work together for higher total productivity than individual productions. The wastes/and by-products from one enterprise are used as inputs for another to enhance productivity and lower the cost of production. With India's shifting agrarian situation, the IFS appears to be a viable option. Overall, an IFS achieves numerous goals, including helping farmers become self-sufficient by ensuring that family members have balanced food, raising living standards by increasing employment and total net income, reducing risk and uncertainty, and maintaining environmental harmony (Mali et al., 2014). Furthermore, IFS is considered to be the most viable and efficient option in Southeast Asian regions, especially for enhancing the productivity of small and marginal farmers; similarly, IFS experiments conducted throughout various countries also showed a positive impact on farm productivity. In Indonesia, it has been found that the IFS integration of corn/horticulture-cow, supported by irrigation ponds, can increase income from IDR 4,094,000 in 2005 to IDR 9,696,300 in 2008, an increase of 136.84 percent (Adijaya et al., 2009). Further experiments with integrated farming systems in the Philippines and Thailand also registered higher yields with improved net returns. In Bangladesh, the integration of different components such as crops (0.65 ha), vegetable farms (0.12 ha), other crops fields (0.09 ha), and a homestead (0.08 ha) with 2.17 numbers of cattle and 11.69 numbers of poultry birds recorded higher profitability (Uddin and Takeya, 2006).
In African countries, integrated farming systems can contribute positively to increasing the productivity of agricultural systems and improving the overall biomass and ecosystem (Duncan et al., 2013). Erick et al. (2013) concluded in their research that livestock-based integration not only helps agriculture but also helps mitigate the most important problems in Africa, such as food security and malnutrition in both animals and humans. This was supported by the findings of Ezeaku et al. (2015). Similarly, results were also observed by Ugwumba et al. (2010) in crop + livestock IFS in the Awka South Agricultural Zone of Anambra State, Nigeria. Integrated crop and livestock systems in Ghana, West Africa, improve overall farm productivity and income through effective recycling of available resources on the farm (Asante et al., 2020). Othman (2006) revealed that adopting IFS increased food production and enhanced economic profitability through the integration of different allied enterprises into the ecosystem. Hence, IFS experiments were conducted to improve productivity, farm income, employment generation, nutritional food security, and reduce GHG emissions for the livelihood improvement of small and marginal farmers in irrigated upland.
2 Materials and methods
2.1 Site description and prevailing weather conditions
The experiments were conducted on the irrigated upland of Tamil Nadu Agricultural University, Coimbatore, from 2017 to 2022. The experimental site was located at 10°12′ and 11°24′ N latitude and between 76°39′ and 77°30′ E longitude of 426.7 m above MSL. The experimental site has a tropical wet and dry climate. During the study, the mean maximum and minimum temperatures were 32.0°C and 22.8°C, respectively. Similarly, the mean morning and evening relative humidity were 84.6% and 54.1%, respectively. A total rainfall of 3,778.9 mm was recorded with 272 rainy days (Figures 1A, B). A mean sunshine hour of 6.5 and a mean evaporation of 5.9 mm were also registered during the cropping period.
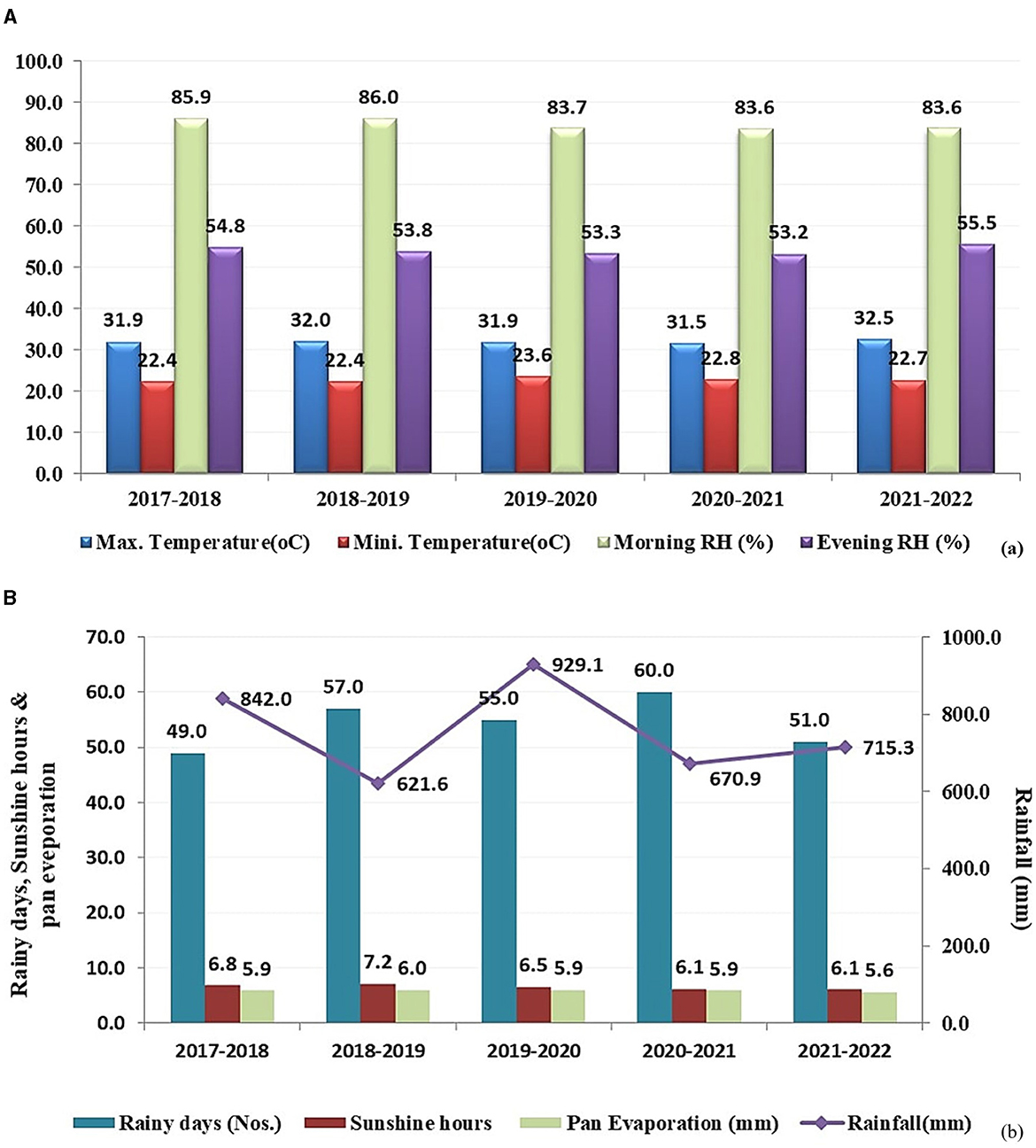
Figure 1. (A) Mean meteorological weather data (maximum, maximum temperature and morning, evening relative humidity) for the crop season from 2017 to 2022. (B) Mean meteorological weather data (rainy days, sunshine hours, pan evaporation, and rainfall) for the crop season from 2017 to 2022.
2.2 Soil characteristics
The soil of the experimental field was clay loam in texture, with the underlying Periyanaikenpalayam soil series having a bulk density (mg m−2) of 1.24. The soil was alkaline (8.49), medium in organic carbon (0.60), medium in available N (250 kg/ha), medium in P2O5 (21.9 kg/ha), and high in K2O (624 kg/ha).
2.3 Enterprises details
The IFS model was established during 2017–2018 in a 1.0 ha area. Six components were included, viz., crop (0.85 ha) + horticulture (0.10 ha) + 2 cattles along with 2 calves in dairy (50 m2) + 12 female goats and one male goat (50 m2) + 150 numbers of poultry birds (50 m2) + kitchen garden (200 m2) + boundary planting + vermicompost (50 m2) + value addition. In addition to this, a 100 m2 area was allotted for supporting activities (100 m2) (Table 1). A benchmark survey of 150 farmers from three districts (Erode, Salem, and Coimbatore) in the western zone of Tamil Nadu has been taken as the data for conventional farming systems.
2.3.1 Cropping system, horticultural, kitchen garden, and border planting
In the cropping component, five cropping systems were included in a total area of 0.85 ha. After assessing the fodder needs of the dairy and goat components in terms of green fodder, dry fodder, and concentrated feed requirements, the promising fodder components were identified and included in the cropping system. Considering the annual green fodder requirement of the dairy and goat units, an area of 0.25 ha was allotted for the Bajra Napier grass (CO (BN) 5) and leguminous Desmanthus virgatus (CO1) to get a year-round green fodder supply. To supplement the fodder requirement during lean summer months, Sesbania grandiflora (Agathi) and Gliricidia sepium were raised along the field boundary. To effectively utilize the field boundaries, annual moringa, curry leaf, and agathi were planted. In the horticultural component fruit trees, viz., sapota, guava, amla, and pomegranate, were planted (Table 2). Intercropping with vegetables like bottle guard, pumpkin, snake guard, and bitter guard was raised in between the space of fruit trees to reduce evaporation losses, weed population, and gain additional income. In the kitchen garden, vegetables (cluster beans, brinjal, radish, lablab, bhindi, and tomato) and greens (fenugreek, coriander, amaranthus, and palak) were raised to meet the nutritional balance of members. A recommended package of practices was followed for agricultural, horticultural crops, and border planting. The surface irrigation system was used to irrigate both horticultural and agricultural crops. Weeds were managed by spraying pre-emergence herbicides. Pests and diseases were controlled by spraying insecticides and seed treatments with biocontrol agents.
2.3.2 Dairy
The dairy unit was maintained with two desi-breed cows (Gir and Kankrej) and two calves. For the feeding schedule, 25 kg of green fodder, 7 kg of dry fodder, and 3.5 kg of concentrate feed were fed daily. FMD vaccinations were administered every 6 months, and artificial insemination was used to reproduce. Deworming was done at a 2-month interval. Milk obtained from the dairy animals was sold regularly. Cow dung obtained from the unit is weighed regularly and used for vermicompost production.
2.3.3 Goat
In the goat unit, Salem black (12 female and 1 male) was maintained on an elevated platform raised 7–8 feet above ground level with good ventilation. In the feeding schedule, 3.5 kg of grass fodder, 2.0 kg of legume fodder, and 1.5 kg of tree fodder were fed. The green grass and dry fodder obtained from crop components and border planting were recycled and utilized as feed for animals. The feed was given at 1.0%−1.5% of their body weight. In addition to this, to maintain health and improve the digestive system, the goats were allowed to graze for 2–3 h in Cenchrus ciliaris grazing area. Vaccination for FMD was done once every 6 months and for enterotoxemia once a year, respectively. Furthermore, deworming was given at the 1st month of age and then once a month up to 6 months of age. After attaining a body weight of 15–20 kg, goats were sold periodically.
2.3.4 Poultry
In poultry rearing, 150 Assel desi birds were reared with a deep litter system. The litter was spread to a depth of 5 cm on the floor before introducing chicks. The litter was stirred once a week. The vaccination was done as per the standard schedule. The poultry bird was fed with a combination of nutrients of carbohydrate, protein, fiber, and mineral salts. Grains such as maize, ragi, prosomillet, cumbu, and cowpea obtained from the IFS are grained in the required proportion and used as feed. After attaining a body weight of 2 kg, the birds were sold.
2.3.5 Vermicompost production
A vermicompost shed was constructed in a 50-m2 area, and earthworms were released at 1 kg/m2. For effective utilization of resources, the animal waste from the dairy and goat units, along with the unutilized feed wastes and crop residues or by-products, were collected for preparing vermicompost. Periodical harvesting of vermicompost was used for agricultural, horticultural, and kitchen gardens.
2.4 Observations recording
2.4.1 Productivity
The system was analyzed by quantifying productivity and income to what extent. The productivity of various allied enterprises was converted to maize grain equivalent yield (MEY) based on farm price.
2.4.2 Economics
The cost of inputs and the price of produce at prevailing market rates were considered for working out the cost of cultivation, returns, and B:C ratio as below.
2.4.2.1 Net return
Net returns were obtained by subtracting the cost of cultivation from the gross return for each component.
2.4.2.2 Benefit-cost ratio (BCR)
The benefit-cost ratio was worked out using the following formula.
2.4.3 Greenhouse gas emissions
The information was calculated using previously forecasted values, fertilizer, machinery, and chemical usage for various crops. For easier comparison, the greenhouse gas emissions from various cropping sequences and other components were converted to carbon dioxide equivalents between components.
were,
Emission = Annual emissions in units of kg of CO2 eq. per farm.
A = Activity data (kg of N used, liters of fuel used, etc.).
EF-Emission factor = IPCC default emission factors or country-specific emission factors.
The IPCC uses CO2 as a reference gas; hence, all other GHGs are converted into carbon dioxide equivalents to ensure uniformity.
2.4.4 Data interpretation
The production data of different enterprises for five consecutive years were presented year-wise and average under respective parameters. The conventional farming system (crop + horticulture + livestock) was considered as a control to compare with the integrated farming system.
The difference between the two farming systems was expressed as a fold change (conventional farming systems were considered as 1).
3 Results and discussion
3.1 Productivity and economics of crop components
The ever-increasing demand for food grain production requires crop diversification, which is essential to increasing productivity, profitability, and sustainability. In this study, a higher maize equivalent yield of 1.86 t in a 1,500 m2 area was obtained from prosomillet-chillies-dhaincha (Tables 3, 4). This might be due to the rooting systems of the crops; the shallow and fibrous root systems of the prosomillet and deep root systems of the chillies enable for better uptake of nutrients from the top and bottom layers of the soil in their consecutive seasons of cropping. Prosomillet is included in the cropping system for its nutritional content and significance. At present, millet is considered an important source of energy and protein for African and Asian countries (Amadou et al., 2013), and considering its nutritional superiority and climate-resilient features of prosomillet, it can be a better climate-smart alternative to the predominant cereals (Rajasekaran et al., 2023).
Similarly, vegetable-based cropping systems like prosomillet-chillies-dhaincha will provide year-round production with additional revenue, thereby guaranteeing nutritional security (Arti et al., 2019). This result was followed by maize-sunflower-dhaincha (1.85 t.). Integration of maize-sunflower cropping in the IFS system plays a pivotal role in animal nutrition. The maize grain and sunflower oil cake produced from the cropping system can be utilized as concentrate feed for livestock and feed for poultry, thus reducing the overall cost of production via better recycling. Experiments conducted in southern Africa revealed that maize-sunflower rotation produced significantly higher yields (Thierfelder et al., 2013). Furthermore, Shanmugam and Ramamoorthy (2014) in their study concluded that the maize-sunflower cropping system in a sericulture-based integrated farming system increased the productivity of maize as well as that of sunflowers with enhanced net return and B:C ratio. Similarly, crop rotation with dhaincha significantly improved the yield. This can be attributed to the efficient and adequate nutrients supply from dhaincha biomass decomposition and the released nutrients for the crop (Sarwar et al., 2017).
The overall crop productivity of 10.78 t. recorded in 8,500 m2. Similarly, Meena et al. (2022) revealed that crop diversification with multiple crops like sugarcane-ratoon and sugarcane-wheat registered a higher sugarcane equivalent yield of 26.83 t./year. Further conventional farming systems, or monocropping, resulted in lower productivity and economic returns. Monocropping with rice is less economical when compared with a diversified cropping system. This is mainly due to the fact that the cultivation of a larger number of crops on the same piece of land has a synergistic impact on each other and compensates for nutrients requirements with minimal environmental risk (Mahapatra and Behera, 2011). Based on the economics of the cropping component, the highest net return of/28,149/ha was recorded with prosomillet-chillies-dhaincha with a B:C ratio of 2.19; however, pearlmillet-cotton-dhaincha recorded a higher B:C ratio of 2.25 with a net return of/21,842/ha. In the fodder system, Bajra Napier hybrid grass, Desmanthus, and Cenchrus recorded a net return of/36,185/ha with a B:C ratio of 1.87.
3.2 Productivity
The productivity of the various components in the integrated farming system was converted into maize equivalent yield (Table 5). Among the different enterprises, crop components alone recorded a higher maize equivalent yield (10.78 t./ha), followed by dairy (7.17 t./ha), goat (5.02 t./ha), vermicompost (4.14 t./ha), poultry (2.39 t./ha), boundary planting (1.18 t./ha), horticulture (1.05 t./ha), and kitchen garden (0.72 t./ha). Higher productivity was due to residue recycling, increased nutrient addition from vermicompost, and the incorporation of green manure in the summer, which improved soil fertility and subsequently increased crop productivity. A higher total rice equivalent yield of 20.83 t. was recorded due to the application of organic manures, viz., farmyard manure, vermicompost, and azolla by recycling, which might have contributed to better crop productivity in IFS (Porpavai and Marimuthu, 2018). The total productivity of the developed IFS was 162.31 t./ha, which was higher when compared with the conventional farming system (crop+horticulture+livestock) (98.27 t./ha). This result is in agreement with the findings of Goverdhan et al. (2020), who opined that integration of crop+horticulture+dairy+goat+vermicompost+backyard poultry fowl recorded higher productivity (26.58 t. of total maize equivalent yield), which was higher than conventional farming systems.
3.3 Economics
Gross return, net return, production cost, and B:C ratio were worked out as individual components in the IFS model. A total gross return of ₹689,773/- and a net return of ₹317,765/- were recorded with the IFS model. The integrated farming system, which includes crops, livestock, poultry, and fisheries, provided a net return of ₹189,069/ha/year as opposed to ₹74,552/ha/year in conventional cotton, which was 2.5 times less than the IFS system (Vinodakumar et al., 2017; Paramesh et al., 2022). This might be due to the inclusion of livestock in the system, which produced higher returns for the farmer.
Among the different components, cropping recorded a higher net return of ₹106,098/- (33.39%) (Table 6). This was followed by a goat, which recorded a net return of ₹73,479/- (23.12%), and the cost of production was lower when compared with the dairy unit. Dairy recorded a net return of ₹30,240/-. This was mainly due to reduced production costs. Expenditure was reduced in the farming system mainly due to the reduction of fodder costs since fodder was grown on the farm itself and fed to animals and birds. Besides, vermicompost produced from the waste was well utilized for the production of fodder. Maintaining goats as an allied enterprise generated year-round income for the farmers with less investment. The goat component contributed to 33.1% of the total gross income. Next to goat, vermicompost production recorded a net return of ₹39,916/-, which contributed about 12.56%. For effective utilization of resources, the animal waste from dairy and goat units, along with the unutilized feed and crop residues/and by-products was used for the production of vermicompost. This provided an excellent opportunity for organic recycling and reduced farmers' dependency on externally purchased inputs.
The IFS models with coconut + goat + cow + turkey + azolla + vermicompost recorded a higher gross return, net return, and B:C ratio compared to conventional cultivation (Thavaprakaash and Premavathi, 2019). Similarly, in the crop + dairy + poultry + vermicompost + azolla IFS model with a net return of ₹10,525/-, the dairy unit contributed a higher net income of 9.52% due to the supply of balanced nutrition by green and dry fodder produced in the system (Shankar et al., 2017). The dairy component produced a considerably higher net return of ₹79,476/- in the IFS model with crop + dairy + poultry + fishery (Singh et al., 2007). Kumara et al. (2017) and Vinodakumar and Desai (2017) reported that the IFS model in a 1-ha area with crop + horticulture + dairy (one buffalo + two HF cows) + sheep (10 + 1) + vermicompost + azolla produced a higher net return from crops (₹80,795/-), followed by horticulture (₹38,526/-), dairy (₹47,278/-), and sheep unit (₹17,876/-).
The poultry component also recorded a considerable net return of ₹22,998/-, with a share of 7.24%. This was mainly due to reduced production costs from reduced purchases of feed from outside markets. The grains such as maize, cumbu, and prosomillet obtained from the farming system are used as feed for poultry. This will create interest among the farmers in rearing poultry as a component of the IFS model. The meat and eggs obtained from the poultry unit generate additional income for the farmers. Similarly, Kumar et al. (2017) reported that the integration of crops with poultry, goats, and mushrooms was more suitable for achieving better income for the farmers.
Boundary planting, horticulture, and the kitchen garden generated a net return of ₹19,923/-, 15,506/-, and 9,605/-, respectively. Integrating horticulture, the kitchen garden, and boundary planting are the sources for increasing family income and the nutritive values of foods and fodder.
The conventional farming system with crop + horticulture + livestock in 1-ha area of the farm recorded a lower gross return (₹266,236/-), a lower net return (₹102,005/-), and a lower B:C ratio (1.62). Lower economic returns in the conventional farming system are mainly due to minimum component integration. Dey et al. (2010) and Sachinkumar et al. (2012) have reported similar findings.
3.4 Employment generation and percentage contribution
On employment generation, cropping activity in the IFS model generates 175 mandays of employment, and multi-enterprise included in the farming system generates 300 mandays of employment with even distribution over the course of the year (Figure 2). Total employment generation from the IFS model was 475 mandays, which was higher when compared with the conventional farming system (232 mandays). This was primarily due to the diversified nature of the numerous activities related to various allied enterprises included in integrated farming systems, which offer a lot of employment opportunities, keep farmers and their families engaged for a longer period of time, and help to improve employment for rural poor people. Integration of cropping with different enterprises such as dairy, mushroom, and biogas recorded higher employment generation (875 mandays) (Sivamurugan, 2001). Combining crops with other enterprises such as horticulture + goat + poultry increases labor requirements, which provide scope to employ more labor throughout the year without giving breaks in the lean period as in conventional agriculture (Ravisankar et al., 2007; Kumar et al., 2012). Sharma et al. (2017) observed that crop + horticulture + kitchen garden + dairy + goat + poultry + duck + vermicompost in irrigated conditions of Chhattisgarh recorded higher employment generation for 1,033 mandays for 1.5 ha area and also in gardenland (Siddeswaran et al., 2012). This is mainly due to the diversified nature of multifarious activities related to different enterprises included in the integrated farming system.
3.5 Food and nutritional security
The integrated farming system fulfills the basic family needs of cereals, pulses, oil, fruits, vegetables, and proteins through milk and meat, which improves the family income status and maintains balanced nutrition for the farm family members. It also alleviates malnutrition deficiencies in rural areas. In this study, adopting an integrated farming system (Table 7) with different components produced 1,679 kg of cereals, 1,918 kg of vegetables, 1,497 L of milk, and 300 kg of meat, which is more than sufficient for an average farm family of six members. Further production of green fodder (46,624 kg) and dry fodder (2,434 kg) was also used as feed for livestock units such as cattle and goats, which reduced the production cost considerably in livestock rearing. IFS strengthens food and nutritional security more than conventional systems. A field experiment conducted in Modipuram reported that integrating crops with dairy, fish, and boundary planting produced 3,040 kg of cereals, 289.9 kg of pulses, 4,228 L of milk, 5,560 kg of fruits, and 2,960 kg of vegetables (Panwar et al., 2019). Higher nutritional security was achieved by producing 1,738 kg of cereals, 239 kg of pulses, 283 kg of oilseeds, 5,902 L of milk, 162 kg of fruits, 1,581 kg of vegetables, 675 kg of eggs, 26,560 kg of green fodder, 3,761 kg of dry fodder, and 810 kg of fuel wood by adopting crops with dairy, horticulture, goat, poultry, and biocompost in a 1.45-ha area (Ravisankar et al., 2019).
3.6 GHG emissions
The high utilization of agricultural chemicals in crop production produced higher GHG emissions from crop fields and other allied enterprises (Figure 3). It was demonstrated that in the integrated farming system, the highest GHG was emitted by livestock components measured at 2,480 CO2-e (kg), followed by goats at 1,958 CO2-e (kg) and horticultural vegetable crops and cropping systems. Among the different cropping systems, cowpea-ragi-dhaincha released a minimum of 143 CO2-e (kg) into the atmosphere. On the contrary, prosomillet-chillies-dhaincha released a maximum of 218 CO2-e (kg), followed by maize-sunflower-dhanicha, which released 213 CO2-e (kg), and pearl millet-cotton-dhaincha, which released 196 CO2-e (kg). The total sink in the IFS unit was 21,008 CO2-e (kg), and the total GHG emission from the IFS unit was −15,118 CO2-e (kg). Fruit trees and boundary plantations, which resulted in a negative GHG emission and a greater carbon sink in the IFS model, allow for further agricultural or enterprise intensification. The integrated farming system is considered a viable strategy to reduce greenhouse gas emissions through the adoption of suitable nutrient recycling (Barbosa et al., 2015; Sharma and Sharma, 2018). Considerably, IFS produced low greenhouse gas emissions. These results are in close conformity with the findings of Meena et al. (2022), who opined that crop + dairy + horticulture + fish pond + mushroom + poultry + kitchen garden + vermicompost + boundary planting registered negative GHG emissions. Negative (−3,175 kg CO2 eq./ha) GHG emissions were recorded in crops with dairy, horticulture, fishery, poultry, duckery, goatery, apiary, vermicompost, biogas, liquid manure, and FYM production integration (Ravisankar et al., 2019). Perennial forages combined with cropping techniques including agroforestry, alley cropping, and intercropping provide a variety of choices for mitigating the effects of climate change by enhancing carbon sequestration and nutrient availability with better grazing management (Bell et al., 2014; Ericksson and Crane, 2018). Further border planting of trees reduces CH and CO emissions by sequestering carbon as biomass and modifying the soil environment, and the roots act as sinks of carbon (Franzluebbers et al., 2016; MacCarthy et al., 2018; Shyam et al., 2023). Therefore, it can be said that IFS is a prominent means to reduce the effects of climate change.
3.7 Limitations and Barriers
Although the IFS system overall is efficient, it also has certain limitations in terms of implementation and practicality. The major limitation has to be the high initial cost associated with various components of the system as well as the requirement of manpower. Furthermore, the system also needs a farm with a sizable area to accommodate all the components, which is a major barrier among small- and medium-scale farmers. The system also needs its initial operational time to become efficient and effective, and the economic stability of the farmers during this time period is also a major concern, especially for small farmers. This was further supported by the findings of Pandey et al. (2019). The availability of suitable planting materials and their combination of plants, animals, and inputs, as well as the complexities of managing, are also constraints, as mentioned in the findings of Nurcholis and Supangkat (2011) and Pushpa (2010). During the course of this study, it was understood that the limitations of the integrated farming system, although prominent, outweigh the advantages, and as the system progresses in the long run, it becomes more efficient and effective than the conventional farming system, thereby providing a stable and sustainable income for the farmers.
4 Conclusion
The developed IFS integrated farming system in 1.0-ha integrating agriculture crops, horticulture, dairy, goat, poultry, vermicompost, kitchen garden, and boundary planting registered higher productivity with generating more mandays employment and fetched a higher gross return and net return. In addition, it also provides nutritional security to the farm household by producing a high amount of cereals, vegetables, milk, and meat, which alleviates nutritional deficiency. This IFS model also helps to reduce the impact of global warming by reducing negative GHG, thus becoming eco-friendly. Thereby, it can be considered the economically feasible, ecologically sustainable, and efficient IFS model for the resource-poor farmers of irrigated upland. This model is developed under on-farm conditions in the western zone of Tamil Nadu, and the results thus obtained are based on the regional-specific conditions. Therefore, in future studies, more emphasis needs to be provided on understanding the variabilities associated with the combinations of the integrated farming system under different climatic and regional conditions, as well as identifying the possibilities and potentials of recycling and optimizing the available resources in a wider national-specific context.
Data availability statement
The original contributions presented in the study are included in the article/supplementary material, further inquiries can be directed to the corresponding author/s.
Author contributions
PS: Conceptualization, Formal analysis, Funding acquisition, Methodology, Validation, Writing – original draft, Writing – review & editing. SS: Validation, Conceptualization, Formal analysis, Funding acquisition, Methodology, Writing – original draft. PCP: Conceptualization, Funding acquisition, Methodology, Writing – review & editing. SV: Writing – original draft, Writing – review & editing. AR: Formal analysis, Validation, Writing – review & editing. NR: Conceptualization, Funding acquisition, Writing – review & editing. PP: Funding acquisition, Writing – review & editing. TP: Funding acquisition, Writing – review & editing. NS: Funding acquisition, Writing – review & editing. SN: Funding acquisition, Writing – review & editing. MG: Validation, Writing – review & editing.
Funding
The author(s) declare financial support was received for the research, authorship, and/or publication of this article. This study was funded by Indian Institute of Farming System Research (IIFSR), Modipuram, Meerut, Uttar Pradesh, India and Tamil Nadu Agricultural University, Coimbatore, Tamil Nadu, India.
Acknowledgments
The authors express special heartfelt thanks to the Indian Institute of Farming System Research (IIFSR), Modipuram, Meerut, Uttar Pradesh, and the Department of Agronomy, Tamil Nadu Agricultural University, Coimbatore, Tamil Nadu, India, for their valuable support and suggestions during the course of study.
Conflict of interest
The authors declare that the research was conducted in the absence of any commercial or financial relationships that could be construed as a potential conflict of interest.
Publisher's note
All claims expressed in this article are solely those of the authors and do not necessarily represent those of their affiliated organizations, or those of the publisher, the editors and the reviewers. Any product that may be evaluated in this article, or claim that may be made by its manufacturer, is not guaranteed or endorsed by the publisher.
References
Adijaya, N., Trisnawati, W., Mahaputra, K., and Agus, K. (2009). Laporan Akhir Prima Tani Lahan Kering Dataran Rendah Iklim Kering di Kabupaten Buleleng. Balai Pengkajian Teknologi Pertanian, Bali: Denpasar.
Amadou, I., Gounga, M. E., and Le, G. W. (2013). Millets: nutritional composition, some health benefits and processing-A review. Emirates J. Food Agric. 25, 501–508. doi: 10.9755/ejfa.v25i7.12045
Arti, B. E., Jha, K. K., Lily, M., and Kispotta, M. (2019). Identification of suitable vegetable based cropping sequences in relation to economic yield and profitability. J. Pharmacogn. Phytochem. 5, 37–41.
Asante, B. O., Villano, R. A., and Battese, G. E. (2020). Evaluating complementary synergies in integrated crop-livestock systems in Ghana. Int. J. Soc. Econ. 47, 72–85. doi: 10.1108/IJSE-04-2019-0274
Barbosa, F. A., SoaresFilho, B. S., Merry, F. D., de Oliveira Azevedo, H., Costa, W. L. S., Coe, M. T., et al. (2015). Cenários para a pecuária de corteamazônica. Universidade Federal de Minas Gerais: Belo Horizonte, Brazil, 154.
Bell, L. W., Moore, A. D., and Kirkegaard, J. A. (2014). Evolution in crop– livestock integration systems that improve farm productivity and environmental performance in Australia. Eur. J. Agron. 57, 10–20. doi: 10.1016/j.eja.2013.04.007
Dey M. M. Paraguas F. J. Kambewa P. Pemsl D. E. (2010) The impact of integrated aquaculture–agriculture on small-scale farms in Southern Malawi. Agric. Econ. 41, 67–79. 10.1111/j.1574-0862.2009.00426.x
Duncan, J., Tarawali, S. A., Thorne, P. J., Valbuena, D., Descheemaeker, K., and Tut, S. H. K. (2013). Integrated Crop-Livestock Systems-a Key to Sustainable Intensification in Africa, Zimbabwe. Trop. Grassl.- Forrajes Trop. 1, 202–206. doi: 10.17138/TGFT(1)202-206
Erick, O. O., Mlingi, F. T., Nyonje, B. M., Charo-Karisa, H., and Munguti, J. M. (2013). Can integrated livestock-fish culture be a solution to East Africa's food insecurity? A review. African J. Food, Agric. Nutr. Dev. 13, 8058–8076. doi: 10.18697/ajfand.59.12920
Ericksson, P., and Crane, T. (2018). The feasibility of low emissions development interventions for the East African livestock sector: Lessons from Kenya and Ethiopia. International Livestock Research Institute, Research Report 46. Nairobi, Kenya. Available online at: https://cgspace.cgiar.org/bitstream/handle/10568/91527/RR46.pdf (accessed October 15, 2023).
Ezeaku, I. E., Mbah, B. N., Baiyeri, K. P., and Okechukwu, E. C. (2015). Integrated crop livestock farming system for sustainable agricultural production in Nigeria. Afr. J. Agric. Res. 10, 4268–4274. doi: 10.5897/AJAR2015.9948
Franzluebbers, A. J., Chappell, J. C., Shi, W., and Cubbage, F. W. (2016).Greenhouse gas emissions in an agroforestry system of the Southeastern USA. Nutr. Cycl. Agroecosyst. 108, 85–100. doi: 10.1007/s10705-016-9809-7
Goverdhan, M., Kumari, C. P., Sridevi, S., Ramana, M. V., and Suresh, K. (2020). Evaluation of integrated farming system model for small and marginal farmers of Telangana State. Curr. Appl. Sci. 39, 26–134. doi: 10.9734/cjast/2020/v39i1030636
Haile, M. G., Wossen, T., Tesfaye, K., and von Braun, J. (2017). Impact of climate change, weather extremes, and price risk on global food supply. Econ. Disaster Clim. Chang. 1, 55–75. doi: 10.1007/s41885-017-0005-2
Iizumi, T., and Ramankutty, N. (2016). Changes in yield variability of major crops for 1981–2010 explained by climate change. Environ. Res. Lett. 11:034003. doi: 10.1088/1748-9326/11/3/034003
Kochewad S. A. Meena L. R. Devender K.umar, Sanjeev, K.umar, Meena, L. K. Singh S. P. and Singh K. (2017). Livestock based integrated farming systems for livelihood improvement of small and marginal farmers. SAJFTE. 3, 526–532. doi: 10.46370/sajfte.2017.v03i01.09
Kumar, S., Samal, S., Dwivedi, S. K., and Manibhushan. (2017). Enhancement in productivity and income sustainability through Integrated farming system approaches for small and marginal farmers of Eastern India: Integrated farming system approaches for small and marginal farmers of Eastern India. J. Agri. Search. 4, 85–91. doi: 10.21921/jas.v4i2.7777
Kumar, S., Singh, S., Meena, M., Shivani, S., and Dey, A. (2012). Resource recycling and their management under integrated farming system for lowlands of Bihar lowlands of Bihar. Indian J. Agric. Sci. 82, 504–510. doi: 10.56093/ijas.v82i6.18889
Kumara, O., Sannathimmappa, H., Basavarajappa, D., Danaraddi, V., Pasha, A., and Rajani, S. (2017). Integrated farming system-An approach towards livelihood security, resource conservation and sustainable production for small and marginal farmers. Int. J. Plant Soil Sci. 15, 1–9. doi: 10.9734/IJPSS/2017/31994
MacCarthy, D. S., Zougmore, R. B., Akponikpe, P. B. I., Koomson, E., Savadogo, P., and Adiku, S. G. K. (2018). Assessment of greenhouse gas emissions from different land-use systems: a case study of CO2 in the Southern Zone of Ghana. Appl. Environ. Soil Sci. 2018, 1–12. doi: 10.1155/2018/1057242
Mahapatra, I. C., and Behera, U. K. (2011). Rice based farming systems for livelihood improvement of Indian farmer. Indian J. Agron. 56, 1–19. doi: 10.59797/ija.v56i1.4660
Mali, H., Kumar, A., and Katara, P. (2014). “Integrated farming system for irrigated and rainfed conditions,” in Proceedings of the National Symposium on Agricultural Diversification for Sustainable Livelihood and Environmental Security, India.
Meena, L. R., Kochewad, S. A., Prusty, A. K., Bhanu, C., and Kumar, K. (2022). Sustainable integrated farming system model for small farm holders of Uttar Pradesh. Indian J. Agric. Sci. 92, 1080–1085. doi: 10.56093/ijas.v92i9.110742
Munandar, G.ustiar, F., Hayati, R., and Munawar, A. I. (2015). Crop-cattle integrated farming system: an Alternative of climatic change mitigation. Trop. Anim. Sci. J. 38, 95–103. doi: 10.5398/medpet.2015.38.2.95
Nurcholis, M., and Supangkat, G. (2011). Pengembangan Integrated Farming System untuk Pengendalian Alih Fungsi Lahan Pertanian. Dalam: Prosiding Seminar Nasional Budidaya Pertanian: Urgensi dan Strategi Pengendalian Alih Fungsi Lahan Pertanian. Bengkulu, ID.
Othman, K. (2006). “Integrated farming system and multifunctionality of agriculture in Malaysia,” in XV International Symposium on Horticultural Economics and Management 655 291–296. Available online at: http://www.actahort.org/books/655/655-36.html. doi: 10.17660/ActaHortic.2004.655.36
Pandey, P. R., Gupta, J. K., Narvariya, R. K., Meena, S. C., and Narwariya, D. (2019). Constraints faced by farmers in adoption of integrated farming system in Vindhyan Plateau of Madhya Pradesh. Plant Arch. 19, 512–514.
Panwar, A. S., Ravisankar, N., Singh, R., Prusty, A. K., Shamim, M., Tripathi, D., et al. (2019). AICRP on integrated farming systems: salient achievements and future directions. Indian J.Fertilisers 15, 338–353.
Paramesh, V., Ravisankar, N., Behera, U., Arunachalam, V., Kumar, P., Solomon Rajkumar, R., et al. (2022). Integrated farming system approaches to achieve food and nutritional security for enhancing profitability, employment, and climate resilience in India. Food Energy Secur. 1:321. doi: 10.1002/fes3.321
Porpavai, S., and Marimuthu, R. (2018). Development of integrated farming system model for marginal farmers of cauvery delta zone. Indian J. Ecol. 45, 183–186.
Rajasekaran, R., Francis, N., Mani, V., and Ganesan, J. (2023). “Proso millet (Panicum miliaceum L.),” in Neglected and Underutilized Crops (New York: Academic Press), 247–278. doi: 10.1016/B978-0-323-90537-4.00005-3
Ravisankar, N., Pramanik, S. C., Rai, R. B., Nawaz, S., Biswas, T. K., and Bibi, N. (2007).Study on integrated farming system in hilly upland areas of Bay Islands. Indian J. Agron. 52, 7–10. doi: 10.59797/ija.v52i1.4879
Ravisankar, N., Singh, P., Mishra, R. P., Prusty, A. K., Shamim, M., Singh, R., et al. (2019). Annual report of AICRP on Integrated farming system. Available online at: https://iifsr.icar.gov.in/icar-iifsr/AICRP/pdf/AICRPIFS_Annual_Report_2018-2019.pdf (accessed October 15, 2023).
Ray, M., Samanta, B., Haldar, P., Chatterjee, S., and Khan, D. K. (2012). Agriculture practices and its association with livestock in hilly areas of West Bengal. Indian J. Hill Farm. 25, 53–57.
Sachinkumar, T. N., Basavaraja, H., Kunnal, L. B., Kulkarni, G. N., Ahajanashetty, S. B., et al. (2012). Economics of farming systems in northern transitional zone of Karnataka. Karnataka J. Agric. Sci. 25, 350–358.
Sarwar, A. K. M. G., Hossain, S. Z., and Chanda, S. C. (2017). Effect of Dhaincha accessions on soil health and grain yield of rice. J. Biosci. Agric. Res. 13, 1140–1145. doi: 10.18801/jbar.130217.139
Shankar, K. A., Yogeesh, L. N., Prashant, S. M., Peer, P. S., and Desai, B. K. (2017). Integrated farming system: profitable farming to small farmers. Int. J. Curr. Microbiol. Appl. Sci. 6, 2819–2824. doi: 10.20546/ijcmas.2017.610.330
Shanmugam, R., and Ramamoorthy, K. (2014). Effect of nutrient recycling in seri-based integrated farming system on soil fertility, productivity and profitability in maize-sunflower cropping system. Trends Biosci. 7, 317–321.
Sharma, R. L., Abraham, S., Bhagat, R., and Prakash, O. (2017). Comparative performance of integrated farming system models in Gariyaband region under rainfed and irrigated conditions. Indian J. Agric. Res. 51, 64–68. doi: 10.18805/ijare.v51i1.7064
Sharma, U. C., and Sharma, V. (2018). Greenhouse gases emissions from agriculture sector in Northeastern region of India. Acta Sci. Microbiol. 1, 36–43.
Shyam, C. S., Shekhawat, K., Rathore, S. S., Babu, S., Singh, R. K., and Upadhyay, P. K. (2023). Development of integrated farming system model - a step towards achieving biodiverse, resilient and productive green economy in agriculture for small holdings in India. Agron. 13:955. doi: 10.3390/agronomy13040955
Siddeswaran, K., Sangeetha, S. P., and Shanmugam, P. M. (2012). “Integrated farming system for the small irrigated upland farmers of Tamil Nadu,” in Extended Summaries: Third International Agronomy Congress on Agriculture diversification, Climate change management and Livelihoods, New Delhi, India.
Singh, K., Bohra, J. S., Singh, T. K., Singh, J. P., Singh, Y., and Singh, C. S. (2007). Productivity and economics of integrated farming system in irrigated agroecosystem of eastern Uttar Pradesh. Indian J. Agron. 52, 11–15.
Sivamurugan, A. P. (2001). Sustainable farming system under irrigated upland situation. Ph.D Thesis, Tamil Nadu Agricultural University, Coimbatore, Tamil Nadu.
Thavaprakaash, N., and Premavathi, R. (2019). Farmer's participatory coconut based integrated farming systems for sustainable farm productivity in high rainfall zone of Tamil Nadu. Madras Agric. J. 106, 576–581. doi: 10.29321/MAJ.2019.000313
Thierfelder, C., Cheesman, S., and Rusinamhodzi, L. (2013). Benefits and challenges of crop rotations in maize-based conservation agriculture (CA) cropping systems of southern Africa. Int. J. Agric. Sustain. 11, 108–124. doi: 10.1080/14735903.2012.703894
Uddin, M. T., and Takeya, H. (2006). Comparative study on integrated farming in Bangladesh and other countries. Bangladesh J. Agric. Econ. 29, 81–92.
Ugwumba, C. O. A., Okoh, R. N., Ike, P. C., Nnabuife, E. L. C., and Orji, E. C. (2010). Integrated farming system and its effect on farm cash income in Awka south agricultural zone of Anambra State, Nigeria. Am.-Eurasian J. Agric. Environ. Sci. 8, 1–6.
Vinodakumar, S. N., and Desai, B. K. (2017). Dairy based farming system models for livelihood security of small and marginal farmers of NE Karnataka. Int. J. Conserv. Sci. 5, 805–808.
Vinodakumar, S. N., Desai, B. K., Channabasavanna, A. S., Satyanarayana, R., Patil, M. G., and Patil, S. S. (2017). Relative performance of various integrated farming system models with respect to system productivity, economics and employment generation. Int. J. Agric. Sci. 13, 348–352. doi: 10.15740/HAS/IJAS/13.2/348-352
Keywords: employment generation, greenhouse gas emission, nutritional security, profitability, sustainability
Citation: Shanmugam PM, Sangeetha SP, Prabu PC, Varshini SV, Renukadevi A, Ravisankar N, Parasuraman P, Parthipan T, Satheeshkumar N, Natarajan SK and Gopi M (2024) Crop–livestock-integrated farming system: a strategy to achieve synergy between agricultural production, nutritional security, and environmental sustainability. Front. Sustain. Food Syst. 8:1338299. doi: 10.3389/fsufs.2024.1338299
Received: 14 November 2023; Accepted: 29 January 2024;
Published: 21 February 2024.
Edited by:
Bin Yao, Chinese Academy of Forestry, ChinaReviewed by:
Nilhari Neupane, International Water Management Institute (Nepal), NepalKochewad Sanjivkumar, National Institute of Abiotic Stress Management (ICAR), India
Copyright © 2024 Shanmugam, Sangeetha, Prabu, Varshini, Renukadevi, Ravisankar, Parasuraman, Parthipan, Satheeshkumar, Natarajan and Gopi. This is an open-access article distributed under the terms of the Creative Commons Attribution License (CC BY). The use, distribution or reproduction in other forums is permitted, provided the original author(s) and the copyright owner(s) are credited and that the original publication in this journal is cited, in accordance with accepted academic practice. No use, distribution or reproduction is permitted which does not comply with these terms.
*Correspondence: Marappan Gopi, getgopi72@gmail.com; S. P. Sangeetha, sangeetha.sp@tnau.ac.in