An evaluation of energy and carbon budgets in diverse cropping systems for sustainable diversification of rainfed uplands in India's eastern hill and plateau region
- 1ICAR-Research Complex for Eastern Region, Farming System Research Centre for Hill and Plateau Region, Ranchi, India
- 2ICAR-Research Complex for Eastern Region, Patna, Bihar, India
- 3Division of Agronomy, ICAR-Indian Agricultural Research Institute, New Delhi, India
With increasing cost and use of energy in agriculture, the traditional practice of mono-cropping of rice in upland is neither sustainable nor eco-friendly. It is necessary to identify crop diversification options with high energy efficiency, productivity, and low global warming potential (GWP). In this experiment, an inclusive system analysis was accomplished for 3 years (2016–2019) of five mono-cropping production (MCP) systems namely rice (R), finger millet (FM), black gram (BG), horse gram (HG), pigeon pea (PP), and four intercropped systems viz. R+BG, R+HG, FM+ BG, and FM + HG. The key objective was to evaluate the flow of energy, carbon balance, and GWP of these varied production systems. Puddled rice was recorded as an energy-exhaustive crop (27,803 MJ ha−1), while horse gram was noted to have the lowest energy use (26,537 MJ ha−1). The total energy output from pigeon pea (130,312 MJ ha−1) and diversified intercropped systems (142,135 MJ ha−1) was 65.3% and 80.3% higher than mono-cultured systems, respectively. Rice and rice-based intercropping production systems showed higher carbon footprints (1,264–1,392 kg CO2 eq. ha−1). Results showed that R+BG and R+HG were the most energy-efficient production systems, having higher energy ratio (5.8 and 6.0), higher carbon efficiency (7.41 and 8.24), and carbon sustainability index (6.41 and 7.24) as against 3.30, 3.61, and 2.61 observed under sole cropping production systems. On average, rice and rice-based production systems had 7.4 times higher GWP than other production systems. In productivity terms, pigeon pea and FM+HG had higher rice equivalent yields of 8.81 and 5.79 t ha−1 and benefit-cost ratios of 2.29 and 1.87, respectively. Thus, the present study suggests that pigeon pea and finger millet-based intercropping systems were the most appropriate crop diversification options for the rainfed upland agro-ecosystem of the eastern region of India.
1 Introduction
Energy use efficiency (Fatima et al., 2023), economic returns, and environmental sustainability (Shyam et al., 2023) of agriculture enterprises are greatly influenced by the quantum and form of energy used in a cropping system. A production system with comparatively lower input requirements and higher outputs is the most efficient one (Upadhyay et al., 2022). The sustainability and profitability of the production systems in the rainfed upland of India's eastern region are in question due to unsustainable energy use practices, the backdrop of widespread natural resource degradation, climate aberrations, and low crop productivity (Praharaj et al., 2016; Singh et al., 2017). The principal cropping system in the rainfed upland of India's eastern region is rice-fallow, constituting ~83% (9.7 M ha) of the nation's total rice-fallow area (11.7 M ha) (Ali et al., 2014; Ghosh et al., 2016; Li et al., 2024). The sustainability of conventional production systems in rainfed upland ecosystems is threatened by declining water availability, limited soil residual moisture, free grazing of cattle, and the poor socio-economic condition of the farming communities (NAAS, 2013).
Energy is a fundamental component of modern agriculture, as it relies significantly on fossil fuels and other energy resources. Production and environmental sustainability are directly related to the type of energy inputs and the total energy output from a production system. Studies have shown that crop production systems are very energy-intensive. In the eastern plateau region, rice-rice systems and rice-fallow-fallow systems recorded energy use of 2.14 and 1.91 MJ kg−1, respectively (Ray et al., 2020). Research into energy flows within diverse production systems gained prominence among the researchers in 1970s, driven by the worldwide fossil-fuel crunch and the growing demand for food to sustain the constantly growing population (Alam et al., 2019). Evaluating the energy dynamics of a system and conducting carbon auditing are crucial steps in choosing the most suitable crop production system, considering factors such as energy efficiency, carbon footprints (CFs), and their environmental implications (Babu et al., 2020). Effective use of energy in agriculture is a prerequisite for sustainable agricultural production which will ensure higher production and productivity, save financial resources, and conserve fossil fuels. Following the Green Revolution era, Indian agriculture has been characterized by the promotion of high input (John and Babu, 2021), mechanized, and irrigated cropping systems. This has led to substantial energy consumption, both directly and indirectly, due to the energy-intensive nature of production activities, inputs, and supplementary requirements (Soni et al., 2018). Hence, enhancing energy efficiency has emerged as a primary goal for both farmers and policymakers. Nevertheless, ongoing initiatives have not fully realized the comprehensive economic potential of energy utilization in agriculture (World Energy Outlook, 2012). Agricultural production systems should be smart enough to sensibly use all the energy inputs so that the twin goals of environmental sustainability and system productivity can be achieved at minimal energy cost.
The agricultural sector is anticipated to play an important role in the share of greenhouse gas (GHG) emissions attributed to climate change. In 2018, global agricultural and associated land use emissions amounted to 9.30 billion tons of carbon dioxide equivalent (FAO, 2018). With growing demands for food grains production, energy use in the agriculture sector will amplify significantly leading to increased greenhouse gas (GHG) emissions (FAO, 2022). As per Czyzewski and Kryszak (2018), agricultural practices account for ~25–30% of GHG emissions. Furthermore, it is well-established that rice cultivation is a significant source of GHGs, including methane (CH4), nitrous oxide (N2O), and carbon dioxide (CO2). This phenomenon is believed to be partially responsible for global warming and subsequent climate change, as indicated by Linquist et al. (2012). Evidence from eastern India showed that GHG emissions were highest (1.265 ± 0.29 t CO2eq. t−1) for rice-fallow-fallow systems and lowest for rice-vegetable systems (Ray et al., 2020;). Rice-based cropping systems have always been predominant in India and are believed to be a major contributor to CH4 and N2O emissions (Yadav et al., 2017; Ray et al., 2018). Rice fields can alone contribute to ~19% and 11% of the total N2O and CH4 emissions, respectively (IPCC, 2007). The impact of crop production systems on GHG emissions is multifaceted, influenced by factors such as variations in energy efficiency, temperature and water management, carbon sequestration, nutrient inputs, fossil fuel utilization for machinery and pesticides, differing crop growth durations, and variations in the crop yields (Alam et al., 2019). Due to increasing environmental impacts and rising energy costs, upland rice-based production systems of eastern India are becoming unsustainable and non-profitable. In this context, the diversification of crops has been recognized as a significant agricultural practice aimed at enhancing agroecosystem productivity while reducing CF (Singh et al., 2017).
There are a few studies focusing on energy budgeting and CFs of cropping systems in India, the majority of which accounted for energy and carbon budgeting in a sole rice production system (Chaudhary et al., 2017; Singh et al., 2019) and sole wheat system (Singh et al., 2020). Some studies considered different cropping systems such as maize-wheat (Saad et al., 2016), pearl millet-wheat (Choudhary et al., 2017), and maize-wheat-mungbean (Parihar et al., 2018). However, the main aim of these studies was to assess the energy and carbon budgeting under the varying tillage, crop establishment, and residue management practices. Studies focusing on the evaluation of diverse cropping systems in a region with consideration of millet and pulse-based systems as alternate cropping systems are lacking for the eastern part of the country. There is an immediate need to identify the most environmentally friendly and efficient millet-based production systems that offer increased productivity and profitability, while also being economical in their use of energy, water, and carbon inputs, all in an environmentally sustainable manner in longer perspectives. We hypothesized that diversified crop rotations and intercropping production systems result in a reduction in energy consumption and carbon footprints and provide more yields and income in the climate change scenario. Over a span of 3 years, from 2016 to 2019, a comprehensive study was undertaken to intensify the cropping systems in South Asia, with the objective of replacing the extensively practiced rice-fallow production system. The outcomes of this study can be applied to enhance the utilization of unutilized rice-fallow lands by introducing the appropriate post-rainy-season/winter crops and employing effective crop establishment practices. Additionally, a deeper understanding of the ecology of rice-fallow areas can facilitate the development of suitable crop rotations and moisture conservation practices to enhance the income of the farmers in eastern India.
2 Materials and methods
2.1 Experiment site
The field study was conducted at a research farm situated in Ranchi, Jharkhand, India (23.35°N and 85.33°E at an altitude of 629 m), spanning kharif seasons from 2016 to 2019. The soil of the experimental site was Typic Haplustalf type (Order: Alfisol) and sandy loam in texture (sand: 69%; silt: 20%; clay: 11%)/The soil exhibited low fertility, characterized by low organic carbon content at 0.43% and a deficiency in available nitrogen (N) (195.5 kg ha−1) and was acidic in nature. However, it had moderate levels of available phosphorus (P) (35.7 kg ha−1) and potassium (K) (241.2 kg ha−1). Monsoon rainfall is the main source of water in the region which is concentrated mostly in 4 months (June–September). During the other periods, agriculture is largely dependent on the groundwater sources.
2.2 Experiment design
The experiment was laid out in a randomized block design with three replications. The treatments consisted of five sole corps and four intercropped systems. The experiment included five sole crop-based production systems namely, rice (Oryza sativa) (T1), finger millet (Eleusine coracana) (T2), black gram (Vigna mungo) (T3), horse gram (Macrotyloma uniflorum) (4), and pigeon pea (Cajanus cajan) (T5) and four diversified intercropped systems, namely, Rice+ Black gram (T6), Rice+ Horse gram (T7), Finger millet + Black gram (T8), and Finger millet + Horse gram (T9). Crops were sown at their respective recommended plant geometry and fertilizer rate (Table 1). In diversified production systems, individual crops were planted in a ratio of 1:1. The selection of sole crops was based on nutritional requirements and the preference of farm families for a particular crop. The cultivars/varieties of the particular crops were the same across all the treatments during the experimentation.
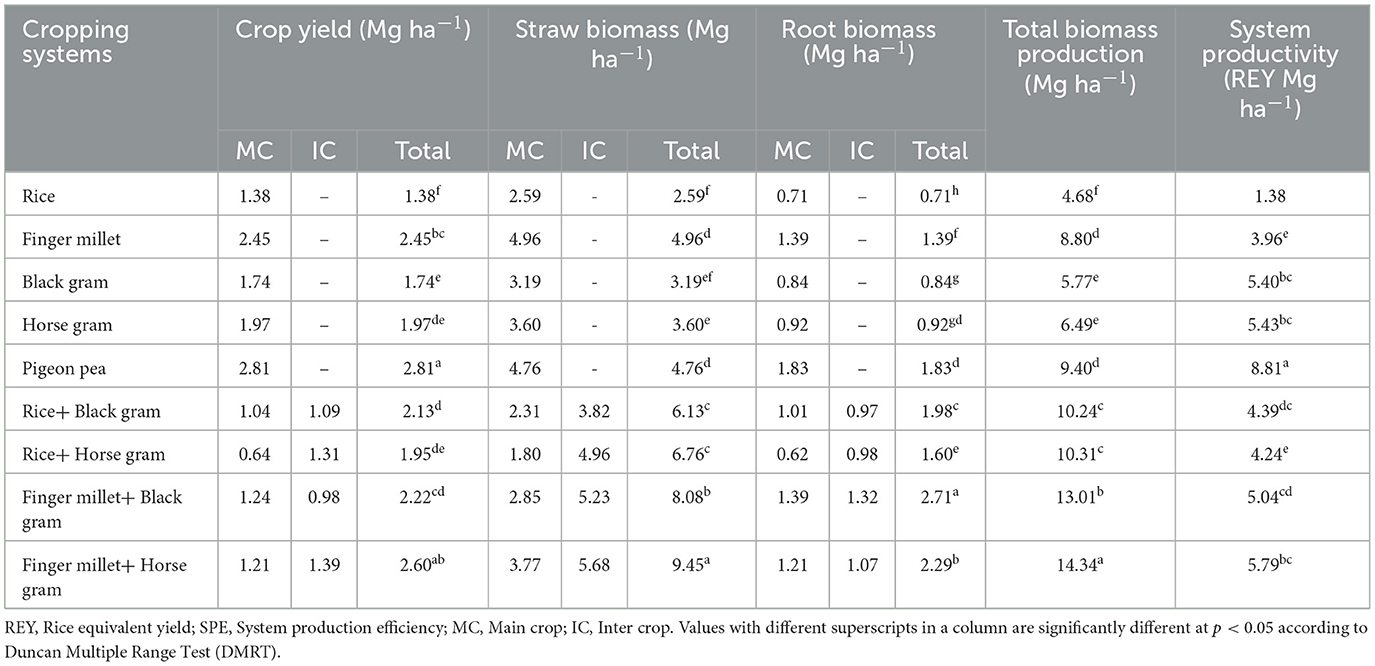
Table 1. Crop yield, total biomass production and system productivity of diverse cropping systems in rice-fallow systems in the eastern hill and plateau region of eastern India (average of 3 years).
2.3 Crop management
Prior to the start of the experiment each year, the experimental plots were plowed using a tractor-drawn plow and subsequently harrowed to pulverize the soil. In rice-based treatments, the initiation of nursery raising started at the same time as the planting date of other intercropping and sole crop treatments. A uniform dose of 1.0 t ha−1 of vermicompost was applied across all the treatment plots before the start of the experiment. In rice-based treatments, 21-day-old seedlings (2–3 seedlings per hill) with a spacing of 30 × 10 cm were used. The recommended dose of fertilizers (RDF) was applied in each of the cropping systems (Supplementary Table 1). For nutrient application, 50% of the required N, along with a full dosage of P and K, was applied as a basal dose. The outstanding 50% of N was administered in two equal portions, divided into applications at maximum tillering and panicle initiation stages of respective crop growth. Weed control was done through manual weeding at the relevant growth stages of crops. Details of crops/cultivars are provided in Supplementary Table 1. Except for sole rice and sole finger millet, an insecticide spray of Imidacloprid 200 SL (17.8 % w w−1) at the rate of 1 ml l−1 was advocated in all treatments.
2.4 Energy budgeting
In the current investigation, an analysis of energy input-output flows was conducted and compared across varied tillage systems. The management of energy input flows in different tillage systems was determined based on their input intensiveness. Energy flows in various tillage systems were calculated by considering the crop management practices, which encompassed machinery operations and input utilization, as well as the quantity of biomass produced. Energy inputs were categorized into direct (operational) and indirect (non-operational) categories. Direct energy inputs encompassed labor (manual), fuel, and farm implements usage, while indirect energy inputs included seeds, farmyard manure (FYM), fertilizers, and pesticides. A comprehensive inventory was prepared encompassing inputs of different crops (such as seeds, pesticides, fertilizers, manpower, farm implements, etc.) and principle/by-product (output) to establish the energy input-output flow for individual crops. Based on total fuel consumption, diesel energy was calculated. Soil fertility, solar radiation, wind, etc. (renewable and natural energy sources) were not considered, as they are not associated with opportunity costs and are not contingent on experiments. Manpower (human labor) and the input of draft animals (bullock power) were measured during the study, as these inputs played a significant role in conventional tillage (CT) production systems. Physical units of total input and output were transformed into energy units using available energy equivalents (published data) (Supplementary Table 2). Various energy use indices were computed using the below formulas (See Equations 1–8).
Energy inputs: The energy equivalent for all inputs was totaled to give an estimation of total energy inputs in respective crop production.
Energy outputs: The energy output from grains/seeds and straw/haulm was computed by multiplying its consequent energy equivalents.
2.5 GHG emissions and GWP
The impact on the environment by the diverse tillage systems was measured by computing the carbon footprint in spatial scale (CFs) and yield scale carbon footprint (CFy). CFs is the total greenhouse gas emissions which include CO2, N2O, and CH4 emitted (directly and indirectly) in carbon dioxide equivalent (CE). The CO2, CH4, and N2O emissions were altered into CE use of GWP equivalents factor of 1, 28, and 265 for CO2, CH4, and N2O, respectively (Padre et al., 2016). The C-footprint (CF) was assessed through the emission of GHG from fossil fuels (diesel) various agronomic operations (tillage, insecticide, plantings, fertilizers) (operational GHG flux), and the production of fertilizer/seed (input GHG flux). The amount of GHG emissions in CE is linked with agronomic input and different operations computed by multiplied inputs (diesels, fertilizers) with corresponding carbon-emission coefficients (C-emission coefficient) (Supplementary Table 3). However, C-emission coefficients were not available for specific applied pesticides. Hence, it is presumed that emissions associated with diverse stages of pesticide production, its transportation, storage, and application in the field were comparable for similar groups of pesticides (Lal, 2004). The periodic CH4 emissions from puddled transplanted rice (PTR) and direct seeded rice (DSR) were 13 and 6 kg cycle−1 hectare−1, respectively (Padre et al., 2016). GHG emissions resulting from nitrogenous fertilizers (Equation 9) were computed by the formula suggested by Padre et al. (2016).
The emission factor for N2O (% of applied N) for a rice paddy was 0.51 (Padre et al., 2016; Kumar et al., 2018). Data on GHG (CO2, CH4, N2O) emissions were used to compute the GWP as shown in the Equation 10:
The cumulative photosynthates of the crops, which represent the total carbon (C) output, were calculated by multiplying them with their respective crop yield, considering the total above-ground biomass and the average carbon content of biomass, ~44% on a dry weight (DW) basis (Lal, 2004). The carbon budgeting for various tillage production systems was computed using the Equations 11–14.
Where, CFs: carbon footprint in spatial scale
The Eco-efficiency Index (EEI) considers both economic and ecological aspects in the context of diverse cropping systems. EEI1 (Equation 15) represents the ratio of economic returns to the overall environmental impact (Gómez-Limón et al., 2012) (Equation 15). Sustainable agricultural production aims to enhance the EEI by reducing the environmental impact, which includes factors such as energy input and greenhouse gas emission, while simultaneously improving the economic outputs (Cicek et al., 2011). The EEI expresses how efficient a diverse production system is, linked to its impact on nature. EEI2 (Equation 16) can also be computed considering GHG emissions (EEI2), representing economic returns per kilogram of GHG emitted. These indices were computed by the following formula:
In the present investigation, the environmental impacts of diverse tillage production systems were computed using energy input (MJ) and the amount of greenhouse gas emitted (kg CE ha−1) during the investigation.
2.6 Yield attributing traits and yield
The economic yield and by-product (straw/haulm) of each crop in diverse production systems were calculated by harvest of 50 m2 areas in all plots. The root biomass was estimated using destructive sampling of five plants from each experimental plot. The extracted roots were placed in a paper bag and dried in an oven at 60°C for 48 h. The dry weight of five plants was upscaled to obtain the per hectare root biomass under each treatment. Economic yields were obtained by threshing at a moisture content of ~12% (ww−1). Rice equivalent yield (REY) of different crops was calculated by adapting grain yields to rice yields with MSP factors using the Equation 17:
The minimum support price (MSP) of crops for the corresponding year (Indian rupee, INR) was taken into account for the calculation of REY. The calculation of system rice equivalent yield (SREY) was done using the following formula (See Equation 18):
Emissions from vermicompost were calculated by scaling the equivalent compost emissions by the relative N content (Equation 19).
Where, Wvermi is the amount of vermicompost applied, kg; EFcompost is the CO2 emission factor for compost, and Nvermi and Ncompost(%) are the nitrogen contents in vermicompost and compost, respectively.
2.7 Economics
The cultivation cost of various production systems in different tillage practices was calculated by adding the cost of the entire input (seed, fertilizer, pesticide, fuels, labor) and farm machinery (Mandal et al., 2015). These inputs cost was computed based on prevailing market rates. The price of economic yield was acquired from the minimum support price (MSP) from the Government of India (GOI). Selling charges of straw (by-products) were computed based on native price. The following economic parameters were calculated based on input cost and gross return (Equations 20, 21):
2.8 Data analysis
Collected data underwent an analysis of variance (ANOVA) to facilitate comparisons between treatments and to draw statistical interpretations. The treatment comparison was made using Duncan's Multiple Range Test (DMRT) post hoc with the help of the SPSS program (version 16.0) with a windows-based interface. Principal Component Analysis (PCA) was conducted using PAST 3.14 statistical software with a windows-based interface.
3 Results
3.1 Crop yield, total biomass production, and system productivity
In the present investigation, crop economic yields and total biomass production were significantly influenced by diverse cropping systems due to differentiation in bio-physical and management factors (Table 1). The yield of rice varied from 1.38 Mg ha−1 in sole cropping systems to 0.64–1.04 Mg ha−1 in intercropping systems. Among the intercropped systems, the finger millet + horse gram system had higher crop yield, which was 22.2, 33.2, and 17.3% higher than the rice + black gram, rice + horse gram, and finger millet + black gram systems, respectively. On average, intercropped systems recorded 99% and 88.6% higher straw and root biomass, respectively, over mono-cropped systems. Finger millet-based intercropping systems, finger millet + black gram and finger millet + horse gram, showed significantly higher (p < 0.05) straw as well as root biomass yields. The annual biomass production varied across the crops and intercropping systems (Table 1). Intercropping systems produced higher total biomass (10.24–14.34 Mg ha−1 year−1). The highest biomass production was noted in the finger millet + horse gram system (T9:14.34 Mg ha−1 year−1), which was higher by 206.4, 63, 148.5, 121, 52.6, 40, 39.1, and 10.2% than T1, T2, T3, T4, T5, T6, T7, and T8, respectively. Regardless of the cropping system, treatments T9 and T8 exhibited higher root biomass (2.29–2.74 Mg ha−1 year−1). Among the different crops, pigeon pea (2.81 Mg ha−1), finger millet (24.5 Mg ha−1), horse gram (1.97 Mg ha−1), and black gram (1.74 Mg ha−1) were the most productive. However, rice had the lowest crop yield (1.38 Mg ha−1). System productivity of rice and finger millet-based intercropping systems was 3.2 and 1.34 times higher than respective sole cropping system productivity.
3.2 Energy use and input-output relationships
In general, millet-based intercropping production systems exhibited lower energy input requirements compared to rice-based intercropping systems (Table 2). Based on the energy use patterns, T1 was the most energy-intensive (27,803 MJ ha−1), followed by T5 (27,289 MJ ha−1). In contrast, production systems based on millet (T9: 26,714 and T8: 26,885 MJ ha−1) required lower energy. Amongst the diverse intercropping systems, treatments T4, T3, T8, and T2 demonstrated the lowest energy requirements.
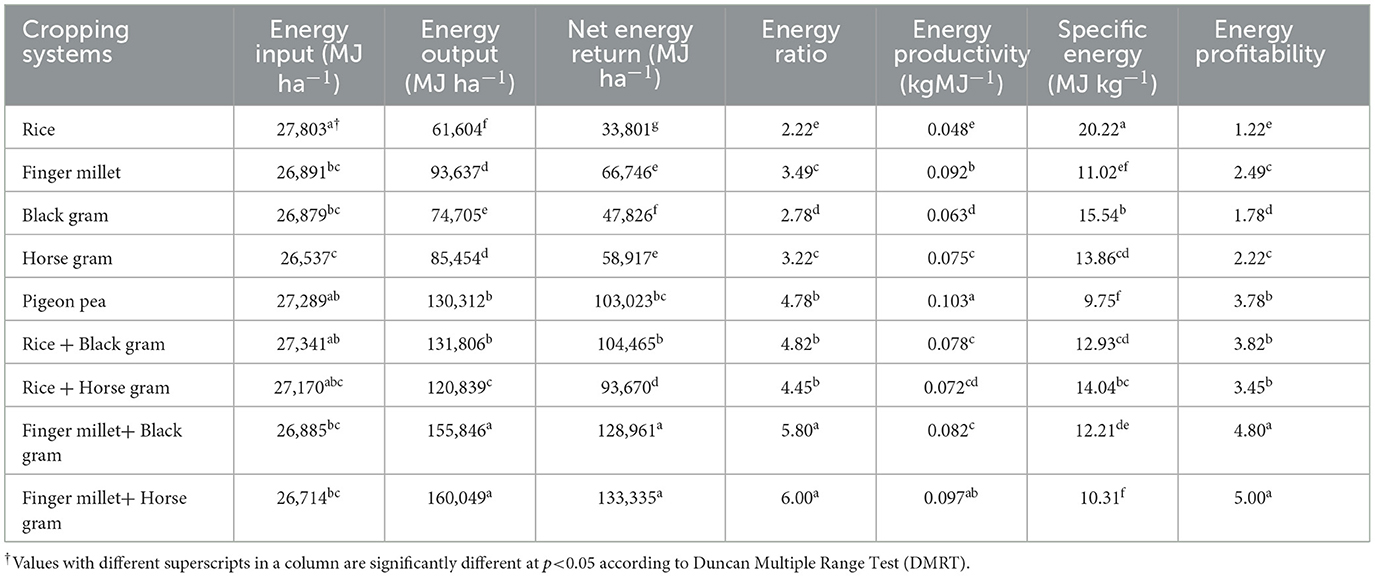
Table 2. Energy flow as influenced by diverse cropping systems in rice-fallow systems in the eastern hill and plateau region of eastern India (average of 3 years).
The total energy output varied across a range from T1 (61,604 MJ ha−1) to T9 (160,049 MJ ha−1) (Table 2). Irrespective of the crops/intercropping system, the highest energy output was noted in T9 (160,049 MJ ha−1). The same trends were observed for the net returns. Additionally, energy ratio and energy profitability were notably higher in T9 (6.0 and 5.0), with T8 following closely (5.8 and 4.8). Similar trends were followed in energy productivity also. As evident from the data, the specific energy was significantly higher in the case of sole crop-based systems (Table 2). Among the intercropping systems, millet-based systems had markedly lower specific energy compared to rice-based intercropping systems. The lowest specific energy (10.3 MJ ha−1) was recorded in the millet-based system treatment (T9).
It was observed that human power comprised the highest percentage of total energy inputs (54.8%-60.1%). The energy input used for diesel in the millet-based intercropping system was 7,602 MJ ha−1 (Table 3). Energy inflow through crop nutrition, i.e., fertilizers, ranged between 8.5 and 12.7%, being higher in rice (3,430 MJ ha−1) and finger millet (3,409 MJ ha−1). Among the intercropping system, maximum energy input was noted in the rice-based system compared to the millet-based production system (Table 3). Irrespective of intercropping systems, higher energy input was used through human power followed by diesel and fertilizer.
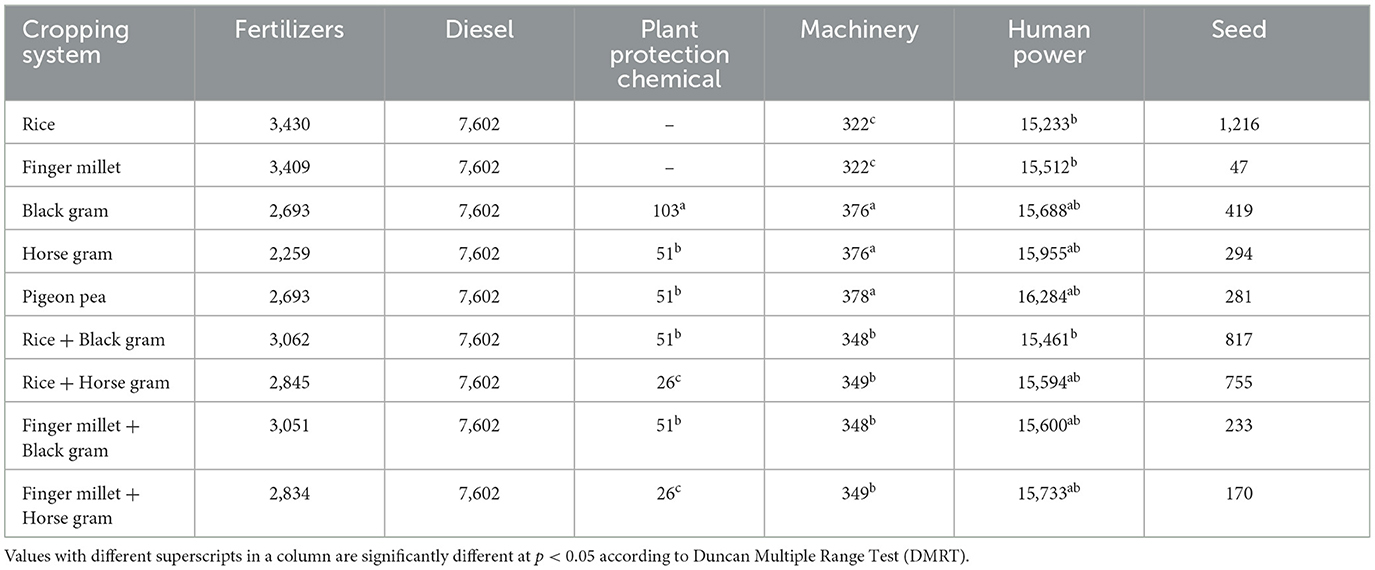
Table 3. Share of various energy inputs (MJ ha−1) under diverse cropping systems in rice-fallow systems in the eastern hill and plateau region of eastern India (average of 3 years).
Among the different agronomic management practices, land preparation was the most energy-intensive (10,650–11,481 MJ ha−1). The maximum consumption of energy input was noted in intercropping systems compared to sole cropping (Table 4). Irrespective of the crops/intercropping system, harvesting, threshing, and storage consumed the highest energy input (4,875–6,020 MJ ha−1). Seed and intercultural operations shared similar energy inputs toward total energy inputs in cropping systems.

Table 4. Energy consumption (MJ ha−1) under different agronomic management practices of diverse cropping systems in rice-fallow systems in the eastern hill and plateau region of eastern India (average of 3 years).
Direct and non-renewable energy sources represented 86–88.8% and 59.7–63.1% of the energy inputs, respectively. In general, these categories constituted the most substantial portions of the total energy inputs, followed by non-direct and renewable energy sources (Figure 1). Irrespective of the crops/intercropping system, the highest human energy profitability (HEP) was noted in intercropping systems in comparison to sole-cropping systems. Amongst the intercropping systems, finger millet + horse gram had the highest HEP and the lowest was the rice-monocropping system. The millet-based production systems had a higher HEP than the rice-based intercropping systems (Figure 2). Intercropping systems recorded higher renewable and non-renewable energy efficiency over the rice-sole system (Figure 3). These attributes were highest for T9 (finger millet + horse gram) and lowest for T1 (rice-sole cropping). Energy intensiveness (EI) was markedly influenced by diverse cropping systems (Figure 4). Among the sole cropping, T1 (rice sole) recorded the highest EI whereas the lowest EI was pigeon pea (T5). Similarly, the rice-based intercropping systems had a comparatively higher EI in comparison to the millet-based systems. The rice + horse gram system had the highest EI. A comparable pattern was also observed within the millet-based systems (Figure 4).
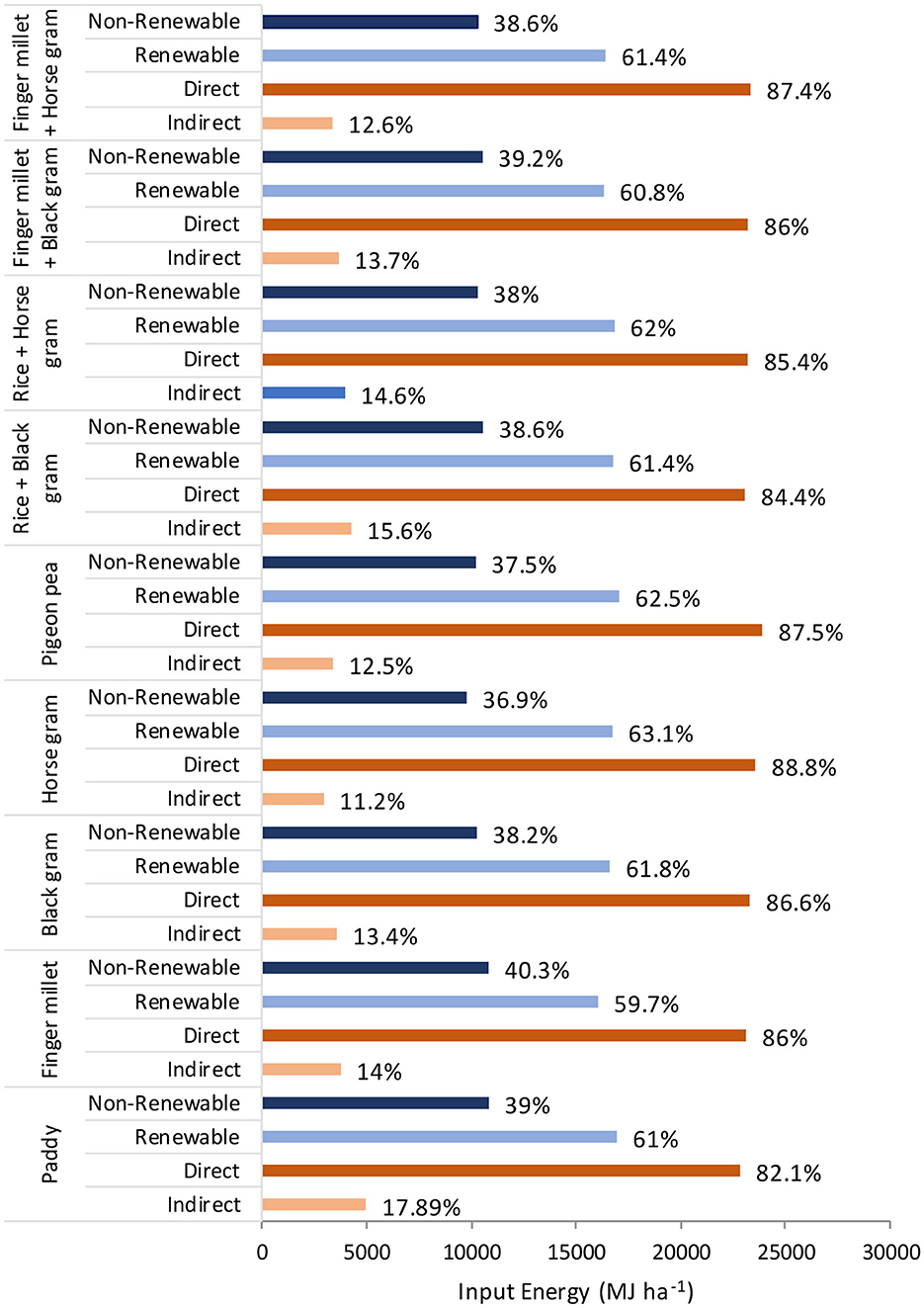
Figure 1. Source-wise distribution of direct, indirect, renewable, and non-renewable input energy as influenced by diverse cropping systems in rice-fallow systems in the eastern hill and plateau region of eastern India (average of 3 years).
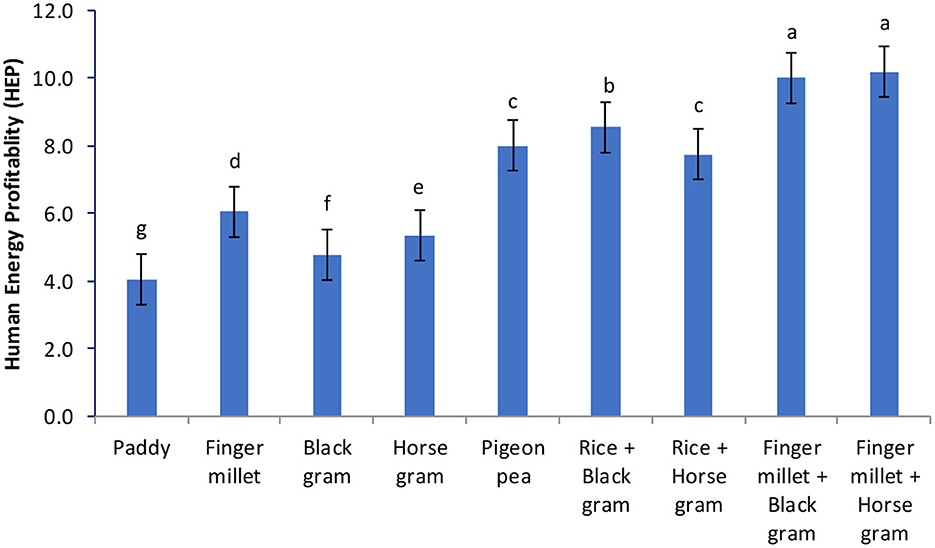
Figure 2. Human energy profitability as influenced by diverse cropping systems in rice-fallow systems in the eastern hill and plateau region of eastern India (average of 3 years). Values with lower case letters provided in graph are significantly different at p < 0.05 according to Duncan Multiple Range Test (DMRT).
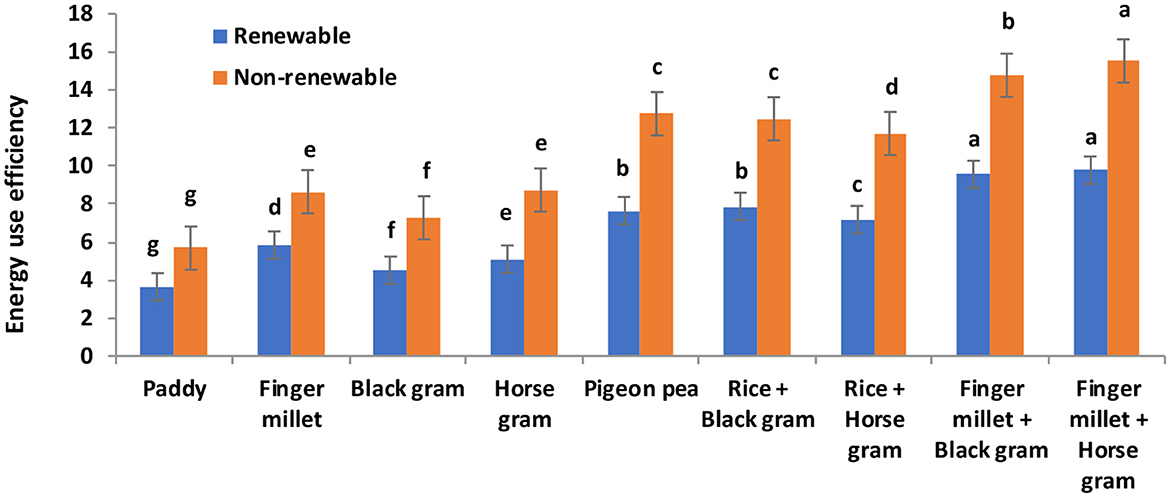
Figure 3. Renewable and non-renewable energy use efficiency as influenced by diverse cropping systems in rice-fallow systems in the eastern hill and plateau region of eastern India (average of 3 years). Values with lower case letters provided in graph are significantly different at p < 0.05 according to Duncan Multiple Range Test (DMRT).
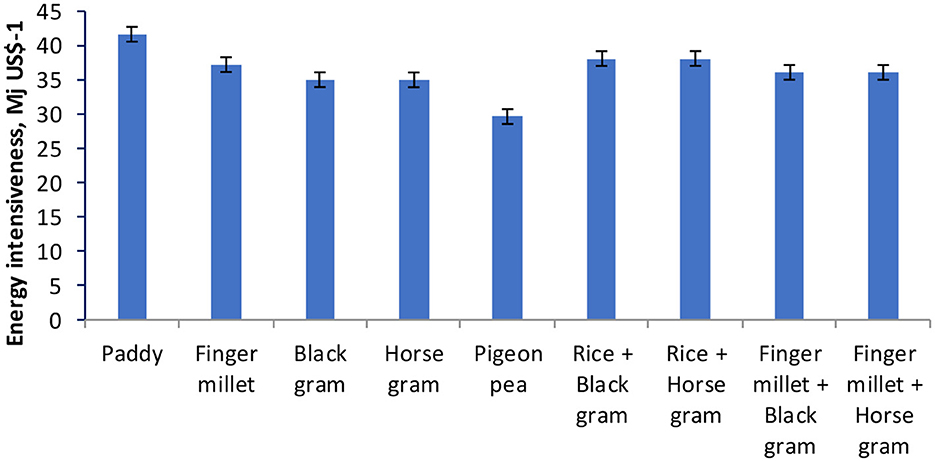
Figure 4. Energy intensiveness as influenced by diverse cropping systems in rice-fallow systems in the eastern hill and plateau region of eastern India (average of 3 years).
Among the different cropping systems, the total energy input requirement was highest in the rice-sole cropping system for T1 (27,803 Mg ha−1), notably surpassing that of the millet-based production systems (Table 1). In terms of diverse farm operations, diesel (27.3–28.5%) and inorganic fertilizers (8.5–12.7%) constituted the most substantial share of the energy input, following land preparation (Table 3).
Generally, millet-based production systems demonstrated higher energy outputs compared to rice-sole cropping. The system-based energy ratio ranged from 2.22 to 6.0, with the values contingent on the total biomass production and energy input utilization. Among the treatments, T9 was recognized as the most energy-efficient. In the current investigation, the millet-based production systems displayed lower energy requirements, ranging from 26,714 to 26,891 MJ ha−1, in contrast to the rice-based systems (27,803 MJ ha−1).
3.3 Carbon budgeting
The CF was markedly influenced by diverse crop and intercropping production systems. Irrespective of the crops/intercropping system, land preparation, fertilizer application, and seed sowing contributed ~82.2–89.5% toward the total carbon footprint (Table 5). Among these, land preparation had the highest share of 59.2–69.3%. Intercultural operations, chemical plant protection, and harvesting/threshing operations had comparatively lower contributions toward the total carbon footprint. Among the various crops/intercropping systems, rice had the highest total carbon footprint (814 kg CE ha−1). The millet-based intercropping systems had a comparatively lower total carbon footprint in comparison to the rice-based production systems (Table 5).
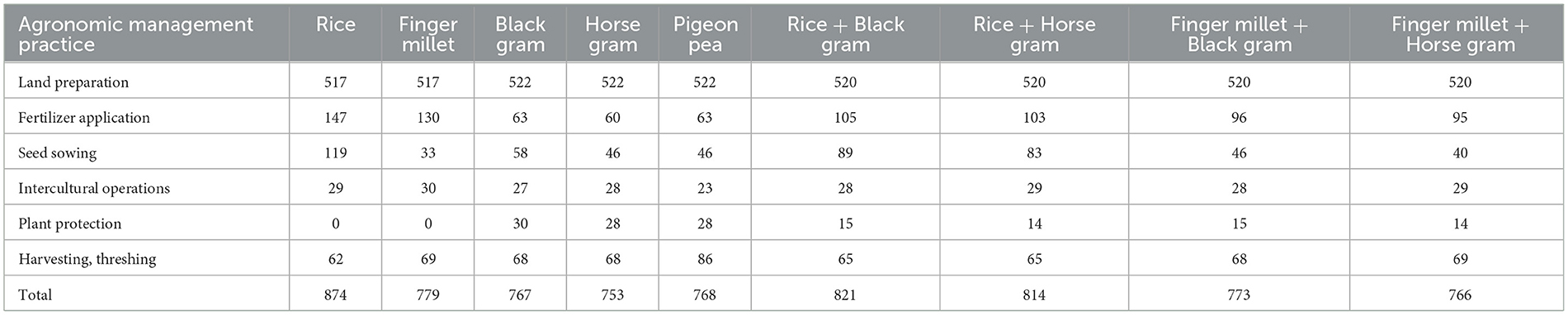
Table 5. C-footprint (kg CO2 eq. ha−1) in different agronomic management practices of diverse cropping systems in rice-fallow systems in the eastern hill and plateau region of eastern India (average of 3 years).
Irrespective of crops/cropping system, rice had the highest share toward the total carbon input (Figure 5). Among the tested crops, pulses (black gram, horse gram, pigeon pea) contributed comparatively lower carbon inputs to the total carbon input due to being low input requiring crops. As the cropping system intensified, overall contribution toward C-input was increased markedly during the present study. Sole cropping had comparatively lower C-input as compared to intercropping systems. Millet-based intercropping systems (T8 and T9) had comparatively lower C-input than rice and or cereal-based systems. The lowest carbon output was recorded with sole rice (T1). The millet-based intercropping systems had markedly higher carbon output compared to cereals-based systems. The highest levels of C-outputs were observed in T9. Conversely, the highest CF was recorded in T1 (1.4 kg CE kg SREY−1).
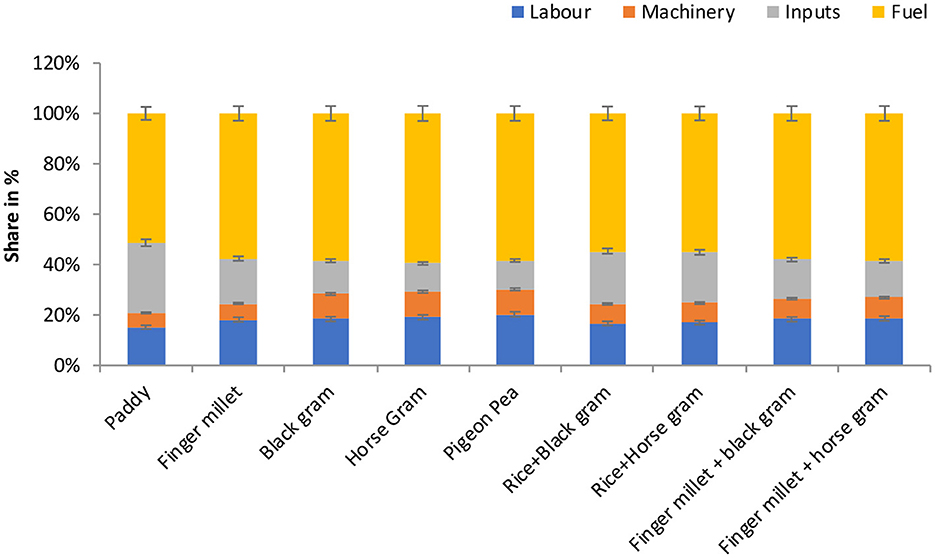
Figure 5. Percentage share of different inputs toward carbon input under diverse tillage production systems in rice-fallow systems in the eastern hill and plateau region of eastern India (average of 3 years).
Diesel usage during land preparation and irrigation represented the most substantial contributor to carbon emissions in cereal-based production systems, while these values were minimized with the adoption of a millet-based cropping system (Table 6). The total CE emissions were higher in cereal-based cropping systems (814–874 kg CE ha−1) compared to the millet-based production systems (766–779 kg CE ha−1). Cereal-based production systems exhibited significantly higher (p < 0.05) C-footprints (1.04–2.05 CE kg SREY−1) compared to the millet-based production systems (0.23–0.44 CE kg SREY−1). An interesting observation is that pulses had the lowest CFs (778 kg CE ha−1) due to their inherently low input requirements (Table 6).
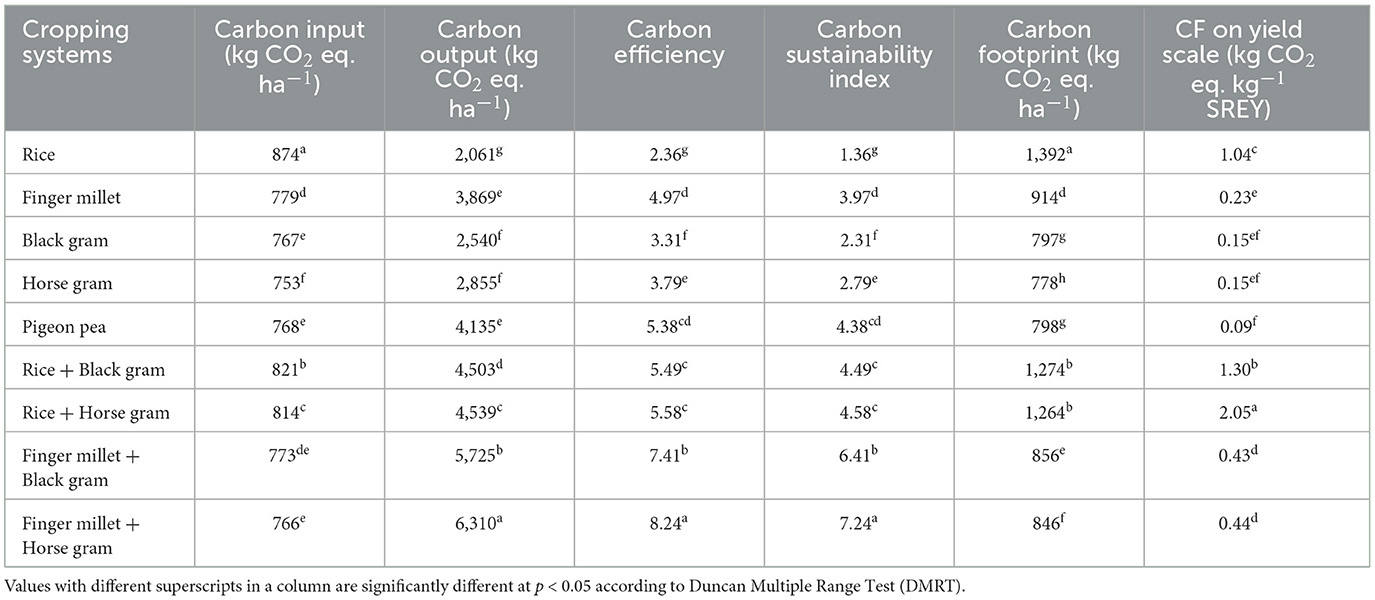
Table 6. Carbon input and output efficiency of diverse cropping systems in rice-fallow systems in the eastern hill and plateau region of eastern India (average of 3 years).
3.4 Eco-efficiency index (EEI)
In general, pulses (black gram, horse gram, and pigeon pea) recorded a higher EEI in economic (0.02 and−0.04 US $ MJ−1) as well as in ecological terms (0.7 and 1.75 US $ kg CO2eq−1) (Figure 6). In terms of input energy and GHG emission, millet-based intercropping had a lower EEI than cereal-based production systems. The EEI (economic terms) in pulses and millet was found to be 1.92 and 2.64 times higher, respectively, than rice alone. A similar pattern was noted in the case of the EEI concerning GHG emissions.
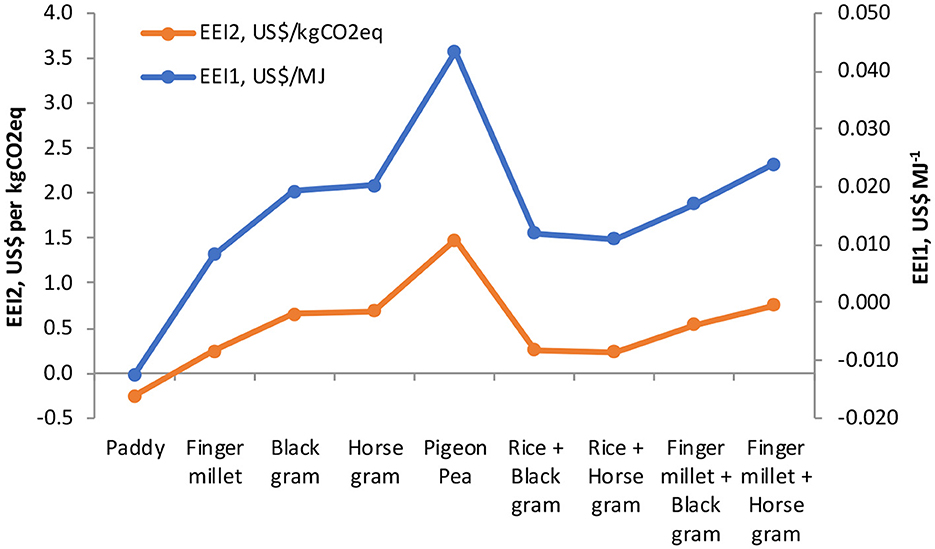
Figure 6. Eco-efficiency index (EEI) as influenced by diverse cropping systems in rice-fallow systems in the eastern hill and plateau region of eastern India (average of 3 years).
3.5 Environmental impact
Assessment of GHG emissions and evaluation of GWP were conducted to determine the effect of diverse cropping systems on environmental sustainability. Horse gram, black gram, and pigeon pea had the lowest N2O emissions (0.09–0.11 kg ha−1 season−1) as against finger millet and rice crops (0.51–0.60 kg ha−1 season−1) (Table 7), while only rice-based systems were assessed for CH4 emissions with 12.8 kg ha−1 season−1. The N2O-GWP of rice and finger millet-based sole cropping systems was 1.7 to 6.6 times higher than other sole or intercropped production systems. The N2O-GWP of intercropped systems ranged between 80.0 and 95.0 kg CO2 eq. ha−1 season−1. Among the sole crop systems, the GWP of black gram (30.08 kg CO2 eq. ha−1 season−1) and horse gram (24.42 kg CO2 eq. ha−1 season−1) was 5.8% and 22.2% of the GWP of sole rice, while in comparison to finger millet, it was 4.7% and 18%, respectively.
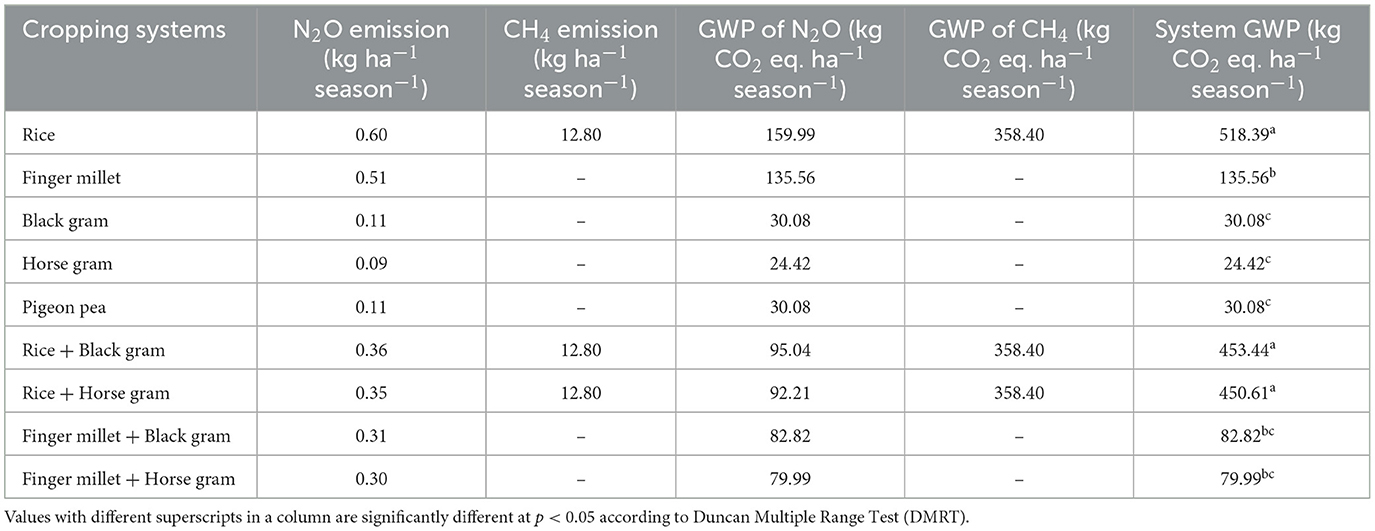
Table 7. Greenhouse gas emission and GWP of diverse cropping systems in rice-fallow systems in the eastern hill and plateau region of eastern India (average of 3 years).
3.6 Production economics
The higher average expenditures that were incurred in cereal-based production systems compared to pulse-based production systems were attributed to excessive tillage operations, increased fertilizer and irrigation usage, and higher labor costs (Table 8). Rice-based production systems recorded comparatively less B:C while millet-based systems observed higher net returns.
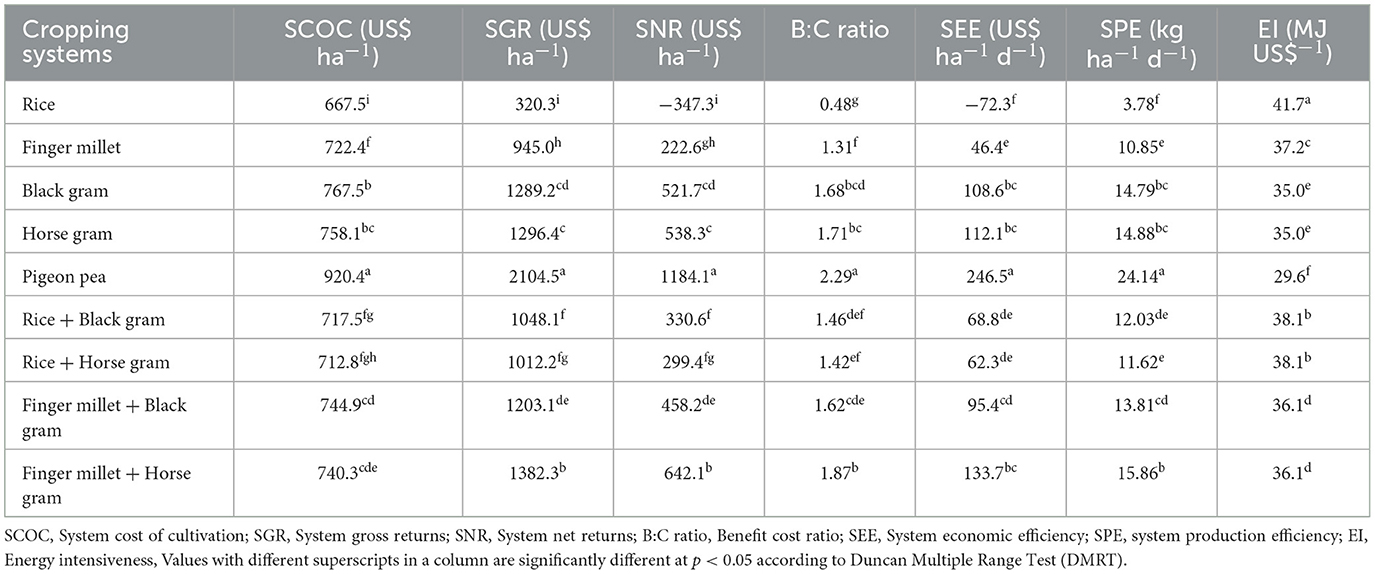
Table 8. Production economics of diverse cropping systems in rice-fallow systems in the eastern hill and plateau region of eastern India (average of 3 years).
4 Discussion
4.1 Productivity of the cropping systems
In the present investigation, crop yields and total biomass production were significantly affected by diverse cropping systems due to variations in bio-physical and management factors (Table 1). Pan et al. (2022) also observed that the introduction of black gram and horse gram as intercrops in paddy and finger millet crops increased the system productivity and efficiency as compared to sole-cropping systems. Crop rotations involving a variety of crop cultivars and cropping systems, a widely adopted agricultural practice globally, have proven effective in addressing the challenges posed by the adverse climatic changes in intensive production systems, ultimately promoting environmental sustainability (Lal et al., 2020). Crop diversification through climate-resilient cropping systems represents a primary strategy for enhancing overall ecosystem productivity and mitigating ecological sustainability concerns (Chaudhary et al., 2009). This approach also contributes to a reduction in CFs and energy use (Yang et al., 2014). Consequently, the design of a resilient crop production system should prioritize the optimization of energy inputs and CFs throughout the entire production process. Assessment of GHG emissions and CFs for individual crops is imperative when creating an efficient cropping system. Selection of crops and cultivars that demand minimal input and have lesser carbon footprints is essential, regardless of the cropping systems (Yadav et al., 2017).
Across all the cropping systems, the T9 treatment consistently exhibited the highest biomass production. Variations in biomass production can be attributed to differences in the genetic potential of individual crops (Lal et al., 2020). Increased total biomass production in finger millet and horse gram systems can be attributed to the superior production capabilities of C4 plants. Finger millet (dual purpose) and horse gram efficiently harness solar energy, resulting in an overall higher total biomass production (Tuti et al., 2012). This study has been also authenticated under upland rainfed conditions, where soil moisture is a primary constraint to achieving a higher crop yield (Choudhary et al., 2017). Furthermore, improved crop management practices, including residual fertility and moisture management, significantly contributed to higher yield benefits (Samal et al., 2017).
4.2 Energy and input use efficiencies
Energy requirements for crop production are directly influenced by the management techniques applied and inputs utilized. In general, millet-based intercropping systems exhibited lower energy input requirements compared to rice-based intercropping systems (Table 2). The need for energy and its production potential is significantly influenced by factors such as inputs used, choice of crops and cultivars, type of cropping system, and crop establishment methods (Kumar et al., 2020). Among the different cropping systems, the total energy input requirement was higher in rice alone (27,803 MJ ha−1), notably surpassing millet-based production systems (Table 1). The primary factor contributing to the increase in energy input was the intensive crop management practices involving human labor, diesel, fertilizers, seeds, and machinery to enhance crop productivity in the rice-sole cropping system (Kumar et al., 2019). Crop production based on conventional tillage systems exhibited high energy input requirements and relatively lower resource use efficiency. Approximately 54.8–57.4% of energy input was allocated for land preparations and crop establishment management, a trend supported by various researchers (Yadav et al., 2017). In terms of diverse farm operations, crop diesel (27.3–28.5%) and inorganic fertilizers (8.5–12.7%) constituted the most substantial share of energy inputs, following land preparation (Table 3). After fertilizers, diesel was the most energy-intensive item, and this variance stemmed from the adoption of high-yielding crop cultivars and various farm operations, including plowing, irrigation, and machinery, under different production systems (Chaudhary et al., 2017). However, millet-based production systems exhibited lower energy requirements due to reduced tillage and minimal inter-cultural operations (Saad et al., 2016). Tuti et al. (2012) also reported that land preparation, fertilizer, and seeds were primary contributors to energy consumption, accounting for ~83% of the total energy use.
Under the varied production system, the energy output is majorly determined by the total biomass production, which includes the main product as well as by-products (Fatima et al., 2023). Intercropping systems exhibit more energy productivity by yielding higher grain, seeds, straw, and total biomass production (Table 1). Regardless of the cropping systems, the highest energy input contribution was attributed to land preparation, followed by diesel and fertilizers, particularly due to the elevated levels of nitrogenous fertilization in rice cultivation. In terms of energy productivity, millet-based production systems outperformed rice-based production systems. Generally, millet-based production systems demonstrated higher energy outputs compared to rice-sole cropping. The system-based energy ratio ranged from 2.22 to 6.0, with values contingent on total biomass production and energy input utilization. The energy ratio of millet-based cropping systems was notably higher, primarily due to the production of more biomass with minimal energy input (Choudhary et al., 2017; Pan et al., 2022). Among the treatments, T9 was identified as the most energy-efficient. In contrast, rice-sole cropping (T1) exhibited the lowest energy efficiency, largely owing to the increased energy input in terms of fertilizer and human power (Kumar et al., 2019). The treatment T1, which involved sole cropping, exhibited higher energy input and relatively lower energy output, leading to a reduced energy ratio. Notably, a higher energy ratio and productivity were observed in the treatments with higher economic yields (Tuti et al., 2012). Rice treatment (T1) demonstrated the lowest energy productivity. Efficiency of energy utilization was more pronounced in millets and pulses, as evidenced by improved energy ratios. The treatment T1 emerged as an energy-intensive system due to the utilization of increased energy inputs to generate comparable energy outputs (Yadav et al., 2020). Consequently, judicious selection of crops and varieties is essential for designing resource-efficient, energy-efficient, and carbon-efficient production systems (Benbi, 2018).
Millet-based production systems exhibited the highest energy outputs while utilizing lower energy inputs. The superior energy output of millet-based production systems was attributed to higher yields of finger millet, expressed in terms of REY (Chaudhary et al., 2017). The primary factors contributing to higher energy inputs in conventional rice-production systems were increased energy input in terms of fertilizers, machinery, diesel, and weeding (Bohra and Kumar, 2015). Therefore, minimizing these constituents in crop management is essential to enhance the energy ratio.
In the current investigation, millet-based production systems displayed lower energy requirements mainly due to reduced fertilizer usage and intercultural operations, while intensive tillage in rice as a sole cropping system increased energy consumption (Nassiri and Singh, 2009; Houshyar et al., 2015). Adoption of the millets in cropping systems led to a partial reduction in energy use, although the use of pesticides somewhat increased energy consumption compared to conventional tillage (Choudhary et al., 2017). Consequently, a greater biological yield of millet-based systems resulted in elevated energy outputs, even under conditions of limited resources (Barut et al., 2011).
4.3 Carbon footprints
Carbon footprints were markedly influenced by diverse crops and intercropping systems (Table 5). Rice-based intercropping systems exhibited the highest levels of C-inputs (Table 6). This could be attributed to extensive land preparation and increased use of fertilizers. Variations in C-inputs were primarily the result of changes in crop and cropping systems (Yadav et al., 2020). In general, C-inputs in cereals, including rice-based production systems, were significantly consumed during land preparation, fertilizer application, and seed sowing. Amongst the various agronomic management practices, the largest shares of C-inputs were attributed to land preparation and fertilizer application (Jat et al., 2019).
Irrespective of the crops/cropping system, rice had the highest share of the total C-input (Figure 5). Millet-based intercropping systems (T8 and T9) had comparatively lower C-input compared to rice/cereal-based systems. Field investigations by Babu et al. (2020) also reported that non-renewable energy sources, mainly chemical fertilizer applications, had a major share in total C-input followed by diesel use in all the cropping systems. Compared to cereals-based systems, intercropping systems based on millet had markedly higher C-output which may primarily be attributed to substantial biomass production. The transition from a cereal-based system to a millet-based system resulted in a significant reduction in CFs. This trend underscores the idea that the CF of crop production largely depends on the crop/cultivar's ability to efficiently convert mineral nutrition into total biomass (Yadav et al., 2020). These findings align with the perspective of others who have emphasized that the CF of cereal-based production systems can be notably reduced through improved management approaches and sustainable cropping intensification, particularly through the adoption of climate-resilient production systems (Babu et al., 2020). Irrespective of crops or cropping systems, the greatest contributions to GHG emissions came from land preparation, fertilizer application, and seed sowing, followed by harvesting, threshing, and plant protection chemicals. Consequently, focus should be directed toward the selection of crops/cultivars for intensification of rice-based systems that require reduced land preparation and fertilizer inputs while exhibiting comparatively higher conversion efficiency of inputs into outputs.
Enhancing energy use efficiency (EUE) and carbon use efficiency (CUE) in all crops within diverse crop/intercropping production systems plays a pivotal role in promoting environmental sustainability by reducing carbon emissions. The elevated CUE/CSI observed in T8 and T9 can be attributed to lower C-inputs resulting from reduced input usage, including fertilizers, pesticides, and irrigations. Conversely, the highest C-footprint recorded for treatment T1 (1.4 kg CE kg SREY−1) may be due to minimal emissions of carbon from fossil fuels (Choudhary et al., 2017). These findings are consistent with the results reported by Jat et al. (2019). Increased C-input in cereal-based production systems primarily resulted from higher inorganic fertilizer application. Higher values of CE/CSI in millet-based systems were associated with reduced C-inputs during land preparation, fertilizer application, and fossil fuel (diesel) usage, in addition to increasing the production of C-outputs (Yadav et al., 2020).
Diesel usage during land preparation and irrigation represented the most substantial contributor to carbon emissions in cereal-based rotation, while these values were minimized by the inclusion of a millet-based production system (Table 6). This difference in GHG emissions amongst these cropping systems may be attributed to differences in the amount of diesel used (Pratibha et al., 2015). Notably, minimum tillage practices resulted in lower GHG emissions compared to conventional tillage systems (Yadav et al., 2018). Pulse-based cropping systems had the lowest CFs due to their inherently low input requirements (Table 6) which is consistent with the finding reported by Choudhary et al. (2017). The substantial impact of crop establishment and tillage practices on CE/CSI has been emphasized by other researchers (Singh et al., 2020).
Considering the backdrop of climate change and human-induced emissions, the feasibility of diverse cropping systems hinges on carbon efficiency. Consequently, the transition from a rice-based production system to a millet-based cropping system reduces the reliance on fossil fuels and enhances environmental sustainability. Therefore, the implementation and advancement of millet-based production systems contribute to energy conservation, improved EUE, reduced CFs, and the harmonization of food security, nutritional quality, and environmental sustainability in the Indo-Gangetic Plain of South Asia.
4.4 Environmental sustainability
Assessment of environmental sustainability for any system can be achieved through the application of eco-efficiency indices, which encompass both economic and ecological dimensions of a production system (Keating et al., 2010). In general, the EEI (economic term) in pulses and millets was 1.92 and 2.64 times higher than the rice alone system, respectively. This pattern underscores that rice monocropping is not environmentally robust because it significantly degrades the ecosystem (Gupta et al., 2016). An improved EEI in terms of economic gains suggests that pulses and millets generate higher net income over their adverse impact on the environment (Lal et al., 2020). These findings indicate that the addition of pulses/millet in intercropping systems enhances economic profitability while minimizing environmental losses. Several studies have also recommended incorporating energy-efficient crops into cereal-based cropping systems to enhance the EEI (Kulak et al., 2013; Babu et al., 2020). Therefore, the adoption of a pulse/millet production system under a rice-fallow system in eastern India significantly enhances the EEI in terms of energy use and GHG emissions when compared to rice monocropping.
Elevated GWP observed in rice cultivation can be attributed to the increased CH4 emissions from anaerobic paddy fields. The practice of puddling and flooding in paddy fields promotes methanogenesis, resulting in heightened CH4 emissions. Conventional-till-direct-seeded rice (CTDSR) recorded lower CH4 emissions due to the prevalence of aerobic conditions in this practice (Chaudhary et al., 2017). Comparatively, lower energy intensiveness of the management practices and reduced CH4 emission due to foregoing puddling led to significantly lower GWP in solely millet-based as well as intercropped cropping production systems (Babu et al., 2020).
4.5 Comparative economic gains
Net returns serve as a primary evaluation tool for assessing the efficiency of enterprises, management strategies, and production economics (Yadav et al., 2017). The 3-year average expenditures incurred in the rice-based production systems were higher, attributed to excessive tillage operations, increased fertilizer and irrigation usage, and higher labor costs (Table 8). Comparatively lower B:C ratios in the rice-based production systems were due to lower returns and higher per-unit production expenditures (Kumar et al., 2018). Consequently, the higher net returns observed in the millet-based intercropping production systems in this study suggest that these alternative production systems are highly profitable due to their lower investments and equivalent economic yields as compared to cereal-based production systems. Therefore, efficient utilization of natural resources (energy, water, labor) through the adoption of millet-based production systems in diverse agro-ecosystems offers a feasible and viable option for enhancing productivity and profitability, and providing a cleaner and safer environment for resource-poor farmers in the region (Lal et al., 2020).
4.6 Millet-based diversification—barriers and policy
While millet-based production systems have demonstrated remarkable efficiency in terms of system productivity, profitability, energy ratio, and the reduction of C-footprints and GHG emissions, their adoption and substitution for rice-based cropping systems with millet face challenges on the eastern Indo-Gangetic Plains (IGP). This is primarily because rice and wheat are the major staple food in the region. Diversification with new crops or changing the existing cropping systems to environment-friendly and productive ones requires changing the mindset of the farmers which can be achieved through large-scale participatory technology demonstration programs across the region. Apart from these social concerns, some advanced research on millet-based cropping systems is also required. For example, considering increasing temperatures and rainfall variability, it is an imperative to identify and target areas suitable for millet-based production systems. Millet, being rich in nutrients and dietary fiber, should be actively promoted as a nutri-cereal in the diets of the rural poor. Government policies need to be oriented toward millet-based production systems to facilitate wider promotion and adoption. The Food and Agriculture Organization's (FAO) recognition of the year 2023 as the International Year of Millets has left a footprint in people's minds about the benefits and potential of millet.
5 Conclusion
The study demonstrated that adopting a millet-based production system resulted in a ~12% reduction in carbon input and a 14.2% reduction in energy intensiveness compared to cereal-based cropping systems. The CF and GWP of millet-based systems were 38.9 and 15.7% of the values observed in rice-based cropping systems. This study recommends that existing rice-fallow systems be diversified by incorporating climate-resilient millet-based production systems such as Finger millet + Horse gram and Finger millet + Black Gram. The addition of millet in prevailing systems can enhance energy and system productivity, and economic efficiency. The cultivation of pigeon pea as a sole crop also showed the potential for environmental as well as monetary gains. These climate-resilient production systems are not only more productive but are also resource-efficient, energy-efficient, and C-efficient, and exhibit lower GHG emissions. These systems can be incorporated into the policy for large upscaling and out-scaling in the eastern plateau region of the country. The persisting mindset of the farmers and the lack of technological innovations can be the major barriers in achieving the suggested crop diversification at regional scales. This calls for policy interventions (perhaps in terms of subsidy programs and irrigation schemes) for the promotion and adoption of millet and pulse-based cropping systems. The insights generated from this study have the potential to enhance the knowledge base and empower policymakers and researchers to promote safer, cleaner, more sustainable, and more productive climate-resilient cropping systems in the Indo-Gangetic Plains of South Asia, aligning with the United Nations Sustainable Development Goals (SDGs).
Data availability statement
The original contributions presented in the study are included in the article/supplementary material, further inquiries can be directed to the corresponding author/s.
Author contributions
RP: Formal analysis, Investigation, Writing – review & editing, Conceptualization, Writing – original draft. SM: Conceptualization, Investigation, Methodology, Writing – original draft, Writing – review & editing. RK: Data curation, Formal analysis, Investigation, Writing – review & editing, Writing – original draft. SN: Data curation, Formal analysis, Investigation, Methodology, Writing – review & editing, Writing – original draft. RS: Formal analysis, Investigation, Writing – original draft, Writing – review & editing. PU: Data curation, Formal analysis, Investigation, Writing – review & editing. BJ: Data curation, Formal analysis, Writing – original, Writing – review & editing draft. PJ: Data curation, Formal analysis, Writing – original, Writing – review & editing draft. AD: Data curation, Supervision, Formal analysis, Writing – original, Writing – review & editing draft.
Funding
The author(s) declare that no financial support was received for the research, authorship, and/or publication of this article.
Acknowledgments
Authors are grateful to the ICAR-Research Complex for Eastern Region, Patna, Bihar, India for providing necessary facilities during the experimentation.
Conflict of interest
The authors declare that the research was conducted in the absence of any commercial or financial relationships that could be construed as a potential conflict of interest.
Publisher's note
All claims expressed in this article are solely those of the authors and do not necessarily represent those of their affiliated organizations, or those of the publisher, the editors and the reviewers. Any product that may be evaluated in this article, or claim that may be made by its manufacturer, is not guaranteed or endorsed by the publisher.
Supplementary material
The Supplementary Material for this article can be found online at: https://www.frontiersin.org/articles/10.3389/fsufs.2024.1340638/full#supplementary-material
References
Alam, M. K., Bell, R. W., and Biswas, W. K. (2019). Decreasing the carbon footprint of an intensive rice-based cropping system using conservation agriculture on the Eastern Gangetic Plains. J. Clean. Prod. 218, 259–272. doi: 10.1016/j.jclepro.2019.01.328
Ali, M., Ghosh, P. K., and Hazra, K. K. (2014). “Resource conservation technologies in rice fallow,” in Resource Conservation Technology in Pulses, eds P. K. Ghosh, N. Kumar, M. S. Venkatesh, K. K. Hazra, N. Nadarajan (Jodhpur: Scientific Publishers), 83–88.
Babu, S., Mohapatra, K. P., Das, A., Yadav, G. S., Tahasildar, M., Singh, R., et al. (2020). Designing energy-efficient, economically sustainable, and environmentally safe cropping system for the rainfed maize–fallow land of the Eastern Himalayas. Sci Total Environ. 722, 137874. doi: 10.1016/j.scitotenv.2020.137874
Barut, Z. B., Ertekin, C., and Karaagac, H. A. (2011). Tillage effects on energy use for corn silage in Mediterranean Coastal of Turkey. Energy 36, 5466–5475. doi: 10.1016/j.energy.2011.07.035
Benbi, D. K. (2018). Carbon footprint and agricultural sustainability nexus in an intensively cultivated region of Indo-Gangetic Plains. Sci. Total Environ. 644, 611–623. doi: 10.1016/j.scitotenv.2018.07.018
Bohra, J. S., and Kumar, R. (2015). Effect of crop establishment methods on productivity, profitability, and energetics of rice (Oryza sativa)-wheat (Triticum aestivum) system. Ind. J. Agric. Sci. 85, 217–223.
Chaudhary, V. P., Gangwar, B., Pandey, D. K., and Gangwar, K. S. (2009). Energy auditing of diversified rice–wheat cropping systems in Indo-Gangetic plains. Energy 34, 1091–1096. doi: 10.1016/j.energy.2009.04.017
Chaudhary, V. P., Singh, K. K., Pratibha, G., Bhattacharyya, R., Shamim, M., Srinivas, I., et al. (2017). Energy conservation and greenhouse gas mitigation under different production systems in rice cultivation. Energy 130, 307–317. doi: 10.1016/j.energy.2017.04.131
Choudhary, M., Rana, K. S., Bana, R. S., Ghasal, P. C., Choudhary, G. L., Jakhar, P., et al. (2017). Energy budgeting and carbon footprint of pearl millet–mustard cropping system under conventional and conservation agriculture in rainfed semi-arid agro-ecosystem. Energy 141, 1052–1058. doi: 10.1016/j.energy.2017.09.136
Cicek, A., Altintas, G., and Erdal, G. (2011). Energy consumption patterns and economic analysis of irrigated wheat and rain-fed wheat production: case study for Tokat Region, Turkey. Bulgarian J. Agric. Sci. 17, 378–388.
Czyzewski, B., and Kryszak, L. (2018). Impact of different models of agriculture on greenhouse gases (GHG) emissions: a sectoral approach. Outlook Agric. 47, 68–76. doi: 10.1177/0030727018759092
FAO (2022). Greenhouse Gas Emissions From Agrifood Systems. Global, Regional and Country Trends, 2000-2020. FAOSTAT Analytical Brief Series No. 50. Rome:FAO.
Fatima, A., Singh, V. K., Babu, S., Singh, R. K., Upadhyay, P. K., Rathore, S. S., et al. (2023). Food production potential and environmental sustainability of different integrated farming system models in northwest India. Front. Sustain. Food Syst. 7, 959464. doi: 10.3389/fsufs.2023.959464
Ghosh, P. K., Hazra, K. K., Nath, C. P., Das, A., and Acharya, C. L. (2016). Scope, constraints and challenges of intensifying rice (Oryza sativa) fallows through pulses. Indian J. Agron. 61, S122–128.
Gómez-Limón, J. A., Picazo-Tadeo, A. J., and Reig-Martínez, E. (2012). Eco-efficiency assessment of olive farms in Andalusia. Land Use Policy 29, 395–406. doi: 10.1016/j.fcr.2012.06.007
Gupta, D. K., Bhatia, A., Kumar, A., Das, T. K., Jain, N., Tomer, R., et al. (2016). Mitigation of greenhouse gas emission from rice–wheat system of the Indo-Gangetic plains: through tillage, irrigation, and fertilizer management. Agric. Ecosyst. Environ. 230, 1–9. doi: 10.1016/j.agee.2016.05.023
Houshyar, E., Dalgaard, T., Tarazkar, M. H., and Jørgensen, U. (2015). Energy input for tomato production. What economy says, and what is good for the environment? J. Clean Prod. 89, 99–109. doi: 10.1016/j.jclepro.2014.11.022
IPCC (2007). Climate Change 2007: Synthesis Report Contribution of Working Groups I, II and III to the Fourth Assessment Report of the Intergovernmental Panel on Climate Change. Geneva: IPCC, 104.
Jat, S. L., Parihar, C. M., Singh, A. K., Kumar, B., Choudhary, M., Nayak, H. S., et al. (2019). Energy auditing and carbon footprint under long-term conservation agriculture-based intensive maize systems with diverse inorganic nitrogen management options. Sci. Total Environ. 664, 659–668. doi: 10.1016/j.scitotenv.2019.01.425
John, D. A., and Babu, G. R. (2021). Lessons from the aftermaths of green revolution on food system and health. Front. Sustain. Food Syst. 5:644559. doi: 10.3389/fsufs.2021.644559
Keating, B. A., Carberry, P. S., Bindraban, P. S., Asseng, S., Meinke, H., and Dixon, J. (2010). Eco-efficient agriculture: concepts, challenges, and opportunities. Crop Sci. 50, S-109–S-119. doi: 10.2135/cropsci2009.10.0594
Kulak, M., Nemecek, T., Frossard, E., and Gaillard, G. (2013). How eco-efficient are low-input cropping systems in Western Europe, and what can be done to improve their eco-efficiency? Sustainability 5, 3722–3743. doi: 10.3390/su5093722
Kumar, R., Mishra, J. S., Rao, K. K., Bhatt, B. P., Hazra, K. K., Hans, H., et al. (2019). Sustainable intensification of rice fallows of Eastern India with suitable winter crop and appropriate crop establishment technique. Environ. Sci. Poll. Res. 26, 29409–29423. doi: 10.1007/s11356-019-06063-4
Kumar, R., Mishra, J. S., Rao, K. K., Mondal, S., Hazra, K. K., Choudhary, J. S., et al. (2020). Crop rotation and tillage management options for sustainable intensification of rice-fallow agro-ecosystem in eastern India. Sci. Rep. 10, 1–15. doi: 10.1038/s41598-020-67973-9
Kumar, V., Jat, H. S., Sharma, P. C., Gathala, M. K., Malik, R. K., Kamboj, B. R., et al. (2018). Can productivity and profitability be enhanced in intensively managed cereal systems while reducing the environmental footprint of production? Assessing sustainable intensification options in the breadbasket of India. Agric. Ecosyst. Environ. 252, 132–147. doi: 10.1016/j.agee.2017.10.006
Lal, B., Gautama, P., Panda, B. B., Tripathi, R., Shahid, M., Bihari, P., et al. (2020). Identification of energy and carbon efficient cropping system for ecological sustainability of rice fallow. Ecol. Indic. 115, 106431. doi: 10.1016/j.ecolind.2020.106431
Lal, R. (2004). Carbon emission from farm operations. Environ. Int. 30, 981–990. doi: 10.1016/j.envint.2004.03.005
Li, Y., Li, Y., Zhang, Q., Xu, G., Liang, G., Kim, D. G., et al. (2024). Enhancing soil carbon and nitrogen through grassland conversion from degraded croplands in China: assessing magnitudes and identifying key drivers of phosphorus reduction. Soil Till. Res. 236, 105943. doi: 10.1016/j.still.2023.105943
Linquist, B. A., Adviento-Borbe, M. A., Pittelkow, C. M., Van Kessel, C., and Van Groenigen, K. J. (2012). Fertilizer management practices and greenhouse gas emissions from rice systems: a quantitative review and analysis. Field Crops Res. 135, 10–21.
Mandal, S., Roy, S., Das, A., Ramkrushna, G. I., Lal, R., Verma, B. C., et al. (2015). Energy efficiency and economics of rice cultivation systems under sub-tropical Eastern Himalaya. Energy Sustain. Dev. 28, 115–121. doi: 10.1016/j.esd.2015.08.002
NAAS (2013). Improving Productivity of Rice Fallows. Policy Paper No. 64. New Delhi: National Academy of Agricultural Sciences.
Nassiri, S. M., and Singh, S. (2009). Study on energy use efficiency for paddy crop using data envelopment analysis (DEA) technique. Appl. Energy 86, 1320–1325. doi: 10.1016/j.apenergy.2008.10.007
Padre, T. A., Rai., M, Kumar, V., Gathala, M., Sharma, P. C., Sharma, S., et al. (2016). Quantifying changes to the global warming potential of rice-wheat systems with the adoption of conservation agriculture in north-western India. Agric. Ecosyst. Environ. 219, 125–137. doi: 10.1016/j.agee.2015.12.020
Pan, R. S., Kumar, R., Bhatt, B. P., Mishra, J. S., Singh, A. K., Naik, S. K., et al. (2022). Production potential and soil health of diversified production system of hill and plateau region of eastern India. Indian J. Agric. Sci. 92, 101–104. doi: 10.56093/ijas.v92i1.120849
Parihar, C. M., Jat, S. L., Singh, A. K., Kumar, B., Rathore, N. S., Jat, M. L., et al. (2018). Energy auditing of long-term conservation agriculture based irrigated intensive maize systems in semi-arid tropics of India. Energy 142, 289–302. doi: 10.1016/j.energy.2017.10.015
Praharaj, C. S., Singh, U., Singh, S. S., Singh, N. P., and Shivay, Y. S. (2016). Supplementary and life-saving irrigation for enhancing pulses production, productivity and water-use efficiency in India. Ind. J. Agric. Sci. 61, 249–261.
Pratibha, G., Srinivas, I., Rao, K. V., Raju, B. M. K., Thyagaraj, C. R., and Korwar, G. R. (2015). Energy auditing of long-term conservation agriculture based irrigated intensive maize systems in semi-arid tropics of India. Energy 142, 289–302.
Ray, K., Hasan, S. S., and Goswami, R. (2018). Techno-economic and environmental assessment of different rice-based cropping systems in an inceptisol of West Bengal, India. J Clean Prod. 205, 350–363. doi: 10.1016/j.jclepro.2018.09.037
Ray, K., Sen, P., Goswami, R., Sarkar, S., Brahmachari, K., Ghosh, A., et al. (2020). Profitability, energetics and GHGs emission estimation from rice-based cropping systems in the coastal saline zone of West Bengal, India. PLoS ONE 15, e0233303. doi: 10.1371/journal. pone.0233303
Saad, A. A., Das, T. K., Rana, D. S., Sharma, A. R., Bhattacharyya, R., and Lal, K. (2016). Energy auditing of a maize–wheat–greengram cropping system under conventional and conservation agriculture in irrigated north-western Indo-Gangetic Plains. Energy 116, 293–305. doi: 10.1016/j.energy.2016.09.115
Samal, S. K., Rao, K. K., Poonia, S. P., Kumar, R., Mishra, J. S., Prakash, V., et al. (2017). Evaluation of long-term conservation agriculture and crop intensification in rice-wheat rotation of Indo–Gangetic Plains of South Asia: carbon dynamics and productivity. Eur. J. Agron. 90, 98–208. doi: 10.1016/j.eja.2017.08.006
Shyam, C. S., Shekhawat, K., Rathore, S. S., Babu, S., Singh, R. K., Upadhyay, P. K., et al. (2023). Development of integrated farming system model-a step towards achieving biodiverse, resilient and productive green economy in agriculture for small holdings in India. Agronomy 13:955. doi: 10.3390/agronomy13040955
Singh, P., Singh, G., and Sodhi, G. P. S. (2019). Energy auditing and optimization approach for improving energy efficiency of rice cultivation in south-western Punjab, India. Energy 174, 269–279. doi: 10.1016/j.energy.2019.02.169
Singh, P., Singh, G., and Sodhi, G. P. S. (2020). Energy and carbon footprints of wheat establishment following different rice residue management strategies vis-à-vis conventional tillage coupled with rice residue burning in north-western India. Energy 200, 117554. doi: 10.1016/j.energy.2020.117554
Singh, R. N., Praharaj, C. S., Kumar, R., Singh, S. S., Kumar, N., and Singh, U. (2017). Influence of rice (Oryza sativa) habit groups and moisture conservation practices on soil physical and microbial properties in rice + lathyrus relay cropping system under rice fallows in Eastern Plateau of India. Ind. J. Agric. Sci. 87, 1633–1639.
Soni, P., Sinha, R., and Perret, S. R. (2018). Energy use and efficiency in selected rice-based cropping systems of Middle-Indo Gangetic Plains in India. Energy Rep. 4, 554–564. doi: 10.1016/j.egyr.2018.09.001
Tuti, M. D., Prakash, V., Pandey, B. M., Bhattacharya, R., Mahanta, D., Bisht, J. K., et al. (2012). Energy budgeting of colocasia–based cropping systems in the Indian sub-Himalayas. Energy 45, 986–993. doi: 10.1016/j.energy.2012.06.056
Upadhyay, P. K., Sen, A., Singh, Y., Singh, R. K., Prasad, S. K., Sankar, A., et al. (2022). Soil health, energy budget, and rice productivity as influenced by cow products application with fertilizers under south Asian Eastern Indo-Gangetic plains zone. Front. Agron. 3, 758572. doi: 10.3389/fagro.2021.758572
Yadav, G. S., Babu, S., Das, A., Mohapatra, K. P., Singh, R., Avasthe, R. K., et al. (2020). No-till and mulching enhance energy use efficiency and reduce carbon footprint of a direct-seeded upland rice production system. J. Clean. Prod. 271, 122700. doi: 10.1016/j.jclepro.2020.122700
Yadav, G. S., Das, A., Lal, R., Babu, S., Meena, R. S., Saha, P., et al. (2018). Energy budget and carbon footprint in a no-till and mulch-based rice-mustard cropping system. J. Clean. Prod. 191, 144–157. doi: 10.1016/j.jclepro.2018.04.173
Yadav, G. S., Lal, R., Meena, R. S., Datta, M., Babu, S., Das, A., et al. (2017). Energy budgeting for designing sustainable and environmentally clean/safer cropping systems for rainfed rice fallow lands in India. J. Clean. Prod. 158, 29–37. doi: 10.1016/j.jclepro.2017.04.170
Keywords: carbon budgeting, carbon sustainability index, climate resilient cropping system, energy productivity, global warming potential, system productivity
Citation: Pan RS, Mali SS, Kumar R, Naik SK, Upadhyay PK, Shinde R, Jha BK, Jeet P and Das A (2024) An evaluation of energy and carbon budgets in diverse cropping systems for sustainable diversification of rainfed uplands in India's eastern hill and plateau region. Front. Sustain. Food Syst. 8:1340638. doi: 10.3389/fsufs.2024.1340638
Received: 18 November 2023; Accepted: 29 January 2024;
Published: 27 February 2024.
Edited by:
Ashim Datta, Central Soil Salinity Research Institute (ICAR), IndiaReviewed by:
Jayanta Layek, The ICAR Research Complex for North Eastern Hill Region (ICAR RC NEH), IndiaJatish Chandra Biswas, Bangladesh Rice Research Institute, Bangladesh
Yuan Li, Lanzhou University, China
Copyright © 2024 Pan, Mali, Kumar, Naik, Upadhyay, Shinde, Jha, Jeet and Das. This is an open-access article distributed under the terms of the Creative Commons Attribution License (CC BY). The use, distribution or reproduction in other forums is permitted, provided the original author(s) and the copyright owner(s) are credited and that the original publication in this journal is cited, in accordance with accepted academic practice. No use, distribution or reproduction is permitted which does not comply with these terms.
*Correspondence: Pravin Kumar Upadhyay, pravin.ndu@gmail.com; Rakesh Kumar, rakeshbhu08@gmail.com; Santosh S. Mali, santosh.icar@gmail.com