Analysis of supplemental wildlife feeding in Mississippi and environmental gastrointestinal parasite load
- 1Department of Wildlife, Fisheries and Aquaculture, Mississippi State University, Mississippi State, MS, United States
- 2Department of Clinical Sciences, College of Veterinary Medicine, Mississippi State University, Mississippi State, MS, United States
Wildlife species are host to a variety of gastrointestinal parasites (GIPs). Artificially concentrating animals may increase the risk of disease spread due to increased GIP load and associated environmental load. Supplemental feeding of deer is common among hunters and known to concentrate animals, but there is limited knowledge of how it affects GIP environmental load. GIP load was compared between ecologically-equivalent pairs of sites in Mississippi with and without year-round supplemental feeding (average distance between pairs = 147 m). During May-August in 2019 and 2020, feces from white-tailed deer and raccoons were collected and examined for the presence of nematodes, coccidia, Giardia spp., Cryptosporidium spp., and Baylisascaris procyonis. On average, fed sites had 8 more deer (241% increase) and 2 more raccoon fecal piles (540% increase) than unfed sites. Average parasite loads for individual fecal samples did not differ between fed and unfed sites, but the greater number of deer and raccoon fecal piles at fed sites (p < 0.0001) produced 231% and 308% greater environmental loads of nematodes and coccidia, respectively. Spin feeders, the only feeder type that distributed feed on the ground, had 326% more coccidia in feces on average compared to other feeder types (p < 0.03). These results show that supplemental feeding of white-tailed deer, especially with spin feeders, increases environmental loads of GIP and the potential for transmission of parasitic diseases.
Introduction
Supplemental wildlife feeding is a popular management action for white-tailed deer, Odocoileus virginianus, (WTD) enthusiasts, practiced by 89% of hunters in Arkansas (1) and about half of Mississippi hunting clubs (W. McKinley, personal communication). Hunters and managers use feeding with various goals including increasing recreational opportunities, food resources during times of scarcity, and body condition (2). It is known to concentrate animals in a small area which can intensify otherwise insignificant infections (3). Anthropogenic food sources (e.g., supplemental feeding) have been implicated in the transmission of many wildlife diseases such as bovine tuberculosis in WTD (4), raccoon roundworm (Baylisascaris procyonis) in raccoons [Procyon lotor; (5, 6)], and endoparasites in wild boar [Sus scrofa; (7)].
Gastrointestinal parasites (GIPs) are notable due to their potential to infect many different species (8). Though many parasite genera or species are host-species specific (e.g., Eimeria spp.), others such as Cryptosporidium parvum and B. procyonis are not. C. parvum has reservoirs in humans and domestic animals and has been documented in a variety of wildlife species including WTD (9, 10). B. procyonis is a well-documented zoonotic threat, and it is transmitted by many animals that typically visit supplemental wildlife feeding stations [e.g., rodents, turkeys (Meleagris gallopavo), raccoons, and many others] (11–13).
Wildlife's contribution to landscape-level parasite contamination, and thus transmission risk, can be calculated as environmental load (14). Environmental load calculations quantify parasites by wildlife population or geographic area and may indicate potential for transfer, especially for fecal-oral transmitted species (15, 16). Though past research correlated wildlife GIPs and human-supplied food, there is little research directly comparing wildlife with and without feeding sites (17) or addressing how environmental load changes over time at long-term feeding sites.
To address these gaps in the literature, this study compared the environmental load of gastrointestinal parasites at paired fed and unfed sites. Eggs and oocysts were identified to genus level, and categorized as either “nematode” or “coccidia” based on microscopic morphology.
In previous fecal parasite prevalence studies in WTD, nematode eggs identified by morphology were consistent with a broad range of strongylid species in three super familes: Ancylostomatoidea, Strongyloidea, and Trichostrongyloidea (18). In WTD, coccidia oocytes identified by morphology were consistent with the Eimeria spp. (19). In previous fecal parasite prevalance studies in raccoons, nematode eggs identified by morphology were consistent with Physaloptera rara and Gnathostoma procyonis. Though not a nematode, Macracanthorhynchus ingen is common in raccoons in Mississippi, and the eggs have similar morphology to some raccoon nematodes. (6, 20–22). In raccoons, oocysts identified as coccidia were morphologically consistent with Eimeria spp. and Sarcocystis spp. (23).
Nematode eggs and coccidia oocytes from WTD and raccoon feces were chosen to estimate envirnomental load because of their high expected prevalance at supplemental WTD feeding sites, ease of identification with routine laboratory techniques, and likely increased transmission risk with increased envirnomental contamination. Coccidia species are transmitted by the fecal oral route (24), while many nematode species are transmitted through direct contact with larvae in the environment (25). Because of these transmission routes, it is likely that increased environmental loads are associated with increased transmission risk. Gastrointestinal parasite load was also assessed by feeder type and duration of feeding. Finally, feces collected from both fed and unfed sites were tested for Giardia spp., Cryptosporidium spp., and B. procyonis. It was hypothesized that environmental load of gastrointestinal parasites would be greater at fed sites compared to unfed sites (6, 7) as well as with longer duration of feeding compared to short-term feeding because WTD use of feeders increases over time (26).
Materials and methods
WTD feeders that were in year-round use for at least 1 year were sampled on 17 properties (Figure 1) in Mississippi, USA. Each feeder had been in year-round use for 1–10 years (mean ± SD; 3.9 ± 2.3 years). Feeding duration was categorized into short term (<5 years) and long term (≥5 years). The types of feeders included spin (n = 31), trough (n = 26), and gravity (n = 22; Figure 2). Most feeders were privately-owned and operated, so their placement was non-random.
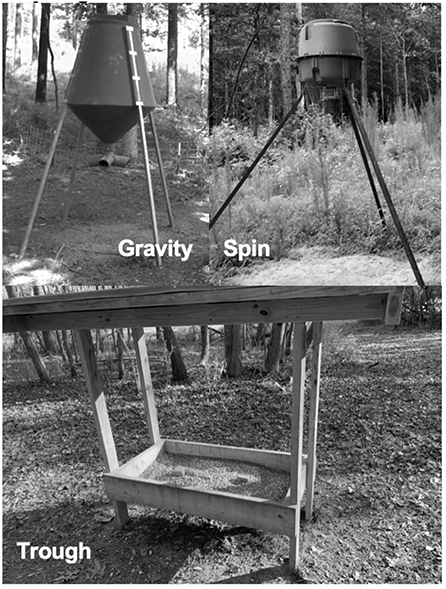
Figure 2. Feeder types (gravity, spin, and trough) sampled in Mississippi, USA during May-August of 2019 and 2020.
Each fed site (i.e., area with a feeder) was paired with an ecologically-equivalent, unfed reference site. Reference sites were areas without feeders but with similar characteristics (e.g., plant community, prescribed fire regime, and proximity to bodies of water, agronomic food plantings, and infrastructure). Seventy-nine feeder sites had viable, ecologically-equivalent unfed pairs. Feeders and their reference sites were 147 ± 70 m apart (average ± SD). To match characteristics as closely as possible between fed and unfed sites, reference sites were chosen non-randomly.
Site pairs were established in a variety of vegetation types: closed canopy pines, thinned pines, and bottomland hardwoods. The pairs were in seven different soil resource regions: Blackland Prairie, Interior Flatlands, Lower Coastal Plain, Lower Thin Loess, Upper Coastal Plain, Upper Thick Loess, and Upper Thin Loess (27).
Visitation by animals and humans (researchers excluded) was monitored at each site using camera traps (Bushnell Trophy Cam HD Vital V3 Game Camera, Bushnell, Overland Park, Kansas, USA) placed 4–5 m from site center. Increased visitation around feeders was expected so cameras settings to reduce photo redundancy and double counting of individuals were chosen. A 25-min interval between trigger events was used with one photo per event and a scanning photo every hour. Species and number of individuals from each photo were recorded.
Sampling occurred on the same day for each pair of sites to limit the influence of factors beyond feeding status (e.g., seasonal differences, weather). From May to August of 2019 and 2020, feces were counted and collected along concentric, circular transects out to a 25 m radius from site center. Feces were identified by visual characteristics to species level (28) and samples deemed fresh enough for GIP counts (moist and soft to the touch) were collected. Samples were placed on ice in the field and refrigerated in the lab until processing, which occurred within 48 h of collection.
To quantify WTD and raccoon fecal parasites, the McMaster's technique was used to determine the number of nematode eggs per gram of feces (EPG) and coccidia oocytes per gram of feces (OPG) in all fresh WTD and raccoon feces (29). Non-quantitiave test tube flotation testing with Sheather's solution was performed on all raccoon feces collected to surveil for B. procyonis. All samples with eggs suggestive of possible B. procyonis were submitted for confirmation by RT-PCR to Zoologix (Chatsworth, CA, USA). Additional quantitave tests of WTD feces included direct fluorescent antibody (DFA) testing to detect Giardia spp. and Cryptosporidium spp. conducted by the Mississippi Veterinary Research and Diagnostic Laboratory (Pearl, MS, USA).
For statistical analyses, version 9.4 of the SAS System for Windows (©2013 SAS Institute Inc.) was utilized. Differences between each fed and unfed pair for all variables were calculated and Shapiro-Wilk tests were used to determine that all data were non-normally distributed. Therefore, Wilcoxon signed-rank tests were utilized to compare the number of fecal piles and average EPG and OPG of nematodes and coccidia in WTD and raccoon feces. To examine the relationship between coccidia and nematode OPG/EPG and feeder characteristics (feeder type and duration of feeding), generalized linear models (GLMs) using PROC GENMOD were used. A zero-inflated negative binomial distribution was selected for the model due to the number of zeros and the non-normal distribution.
Environmental load was calculated using the mean EPG /OPG of nematodes and coccidia per WTD and raccoon fecal pile, the mean number of fresh feces for these two species per site, and the mean weight of fresh feces for each species (30). A mean weight of 40.8 g was used for raccoon feces (31). To determine mean WTD feces weight, 10 freshly deposited fecal piles were collected from captive WTD at the Mississippi State University deer research facility. The mean weight of these 10 samples was 122.4 g.
Results
Over the course of the study, 2,133 total fecal piles were counted, of which 288 were deemed fresh enough for fecal egg/oocyte counts. More fecal piles were found at fed sites from WTD (fed-unfed = 8.2 ± 20.8, p < 0.0001) and raccoons (2.2 ± 4.2, p < 0.0001) (Table 1). Fecal piles from wild pigs (Sus scrofa) and rabbits (Sylvilagus spp.), were also found at study sites, but they were not included in statistical analyses. Mean number of fresh feceal samples per species per site was <2 (Table 2), but the large number of site pairs allowed us to detect differences in GIP ecology between fed and unfed sites.
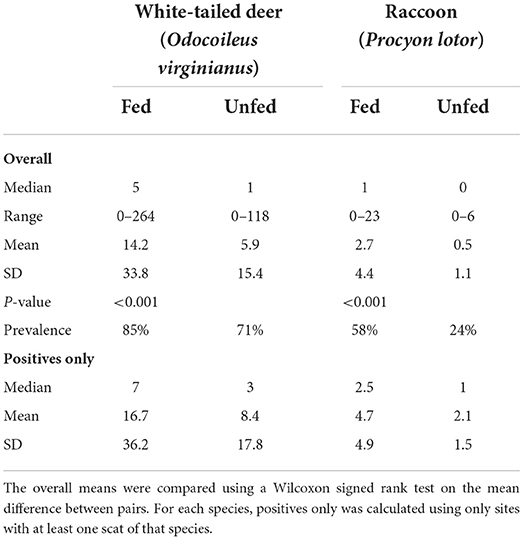
Table 1. Prevalence, median, and mean number of fecal piles by species found at 79 pairs of fed and unfed sites sampled on 17 properties in Mississippi, USA during May-August of 2019 and 2020.
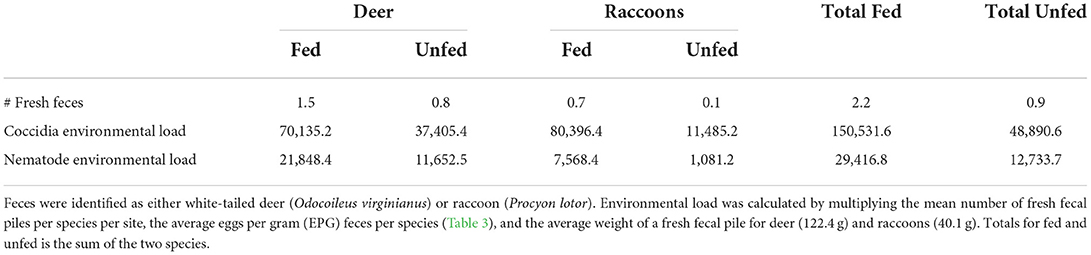
Table 2. Estimated environmental load of nematodes and coccidia per site at fed and unfed sites in Mississippi, USA sampled during May-August of 2019 and 2020.
Gastrointestinal parasite loads for individual fecal samples did not differ between fed and unfed sites for WTD or raccoons (Table 3). Nematodes were not affected by feeder type or feeding duration (Table 4). For coccidia, there was also no effect of feeding duration. However, WTD parasite loads within individual fecal samples were greater around spin feeders (Table 4), with 217% more coccidia oocysts in WTD feces at spin feeders than at gravity and 650% more than at trough on average.
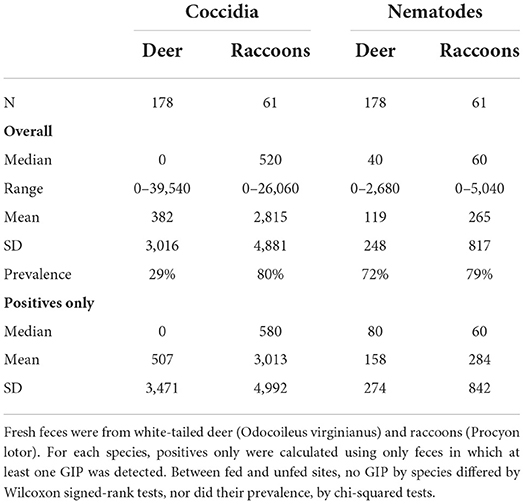
Table 3. Summary statistics for coccidia oocysts per gram (OPG) and nematode eggs per gram (EPG) in scat by host species collected during May-August of 2019 and 2020 at 79 site pairs on 17 properties in Mississippi, USA.
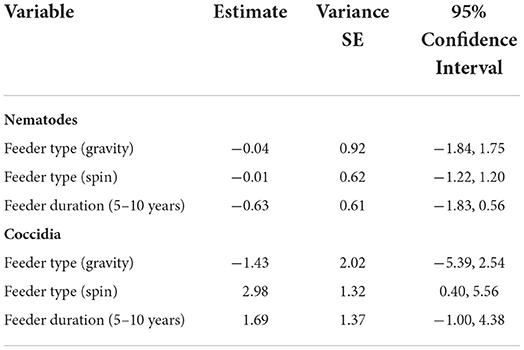
Table 4. Generalized linear model-generated coefficients for the effects of feeder type (gravity and spin relative to trough) and duration of feeding (5–10 years, 1–4 years) on the infection intensity of feces with coccidia and nematodes collected in Mississippi, USA during May-August of 2019 and 2020.
Environmental parasite load varied more between paired sites for coccidia than nematodes, but in both cases were greater at fed sites than unfed sites (Table 2). Despite no difference in the average GIP load for each individual fecal pile, the increased number of feces at fed sites led to 231 and 308% greater environmental loads of nematodes and coccidia, respectively, compared to unfed sites.
Human visitation differed in prevalence (p < 0.01) and average daily visits (p < 0.01) at fed (54%, 0.2 ± 0.3 visits/day) and unfed sites (29%, 0.1 ± 0.2 visits/day). In addition to humans and wildlife, domestic dogs (Canis familiaris) were also documented more at fed sites than unfed sites (11 vs. 2 visits), though the sample size was too low for statistical comparison.
Sixty-two sites had at least one fresh WTD scat and composite samples from each of these sites were tested for Giardia spp. and Cryptosporidium spp. Two composite samples, one fed and one unfed site on different properties, were positive for both Giardia spp. and Cryptosporidium spp. (Figure 3). Due to a low detection rate, statistical analyses could not be performed. The assemblage/species of Giardia spp. and Cryptosporidium spp. was not detected in this study; therefore, the zoonotic potential of the parasites detected is unknown. Two of 61 individual, fresh raccoon scats contained eggs morphologically suggestive of a roundworm species, but neither were positive for B. procyonis by RT-PCR.
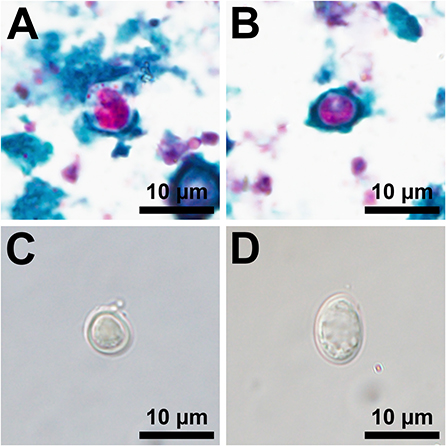
Figure 3. Photomicrographs of acid-fast stained oocysts consistent with a Cryptosporidium sp. (A,B) and isolations by fecal floatation using Sheather's saturated sugar solution of an unstained oocyst consistent with a Cryptosporidium sp. (C) and an unstained cyst consistent with a Giardia sp. (D).
Discussion
For many individuals who supplementally feed wildlife, the goal of the feeding program is to improve wildlife health (2). However, these results suggest supplemental feeding increases environmental load of gastrointestinal parasites, which may undermine nutritional gains from feeding (32) and risk increasing disease transmission. Previous studies have reported similar results, such as increased GIPs with presence of supplemental feeding (6, 7) and greater host density (33).
It is unsurprising that fecal EPG counts in this study did not differ by feeding status given that paired fed and unfed sites averaged only 147 m apart. Not all WTD in a population will visit feeders (26), but the same individual could visit both fed and unfed sites within a pair since bucks travel 6 km/day on average (34). Similarly, average raccoon home ranges in Mississippi range from 1.3 to 2.8 km2 (35), demonstrating that the same individual could travel between fed and unfed sites. The difficulty in finding a large sample size of ecologically-equivalent unfed sites that were also spatially independent from feeder locations prevented us from using populations with no access to feed as past research has (5, 36). We were also limited in how far away we could establish unfed sites while matching the ecological and management traits of fed sites.
Environmental load calculations demonstrated one potential effect of concentrating wildlife at a supplemental feeding site. The increased environmental load of GIPs that are transmitted through fecal oral or direct contact with larva in the environment (e.g., coccidia and nematodes, respectively) suggests supplemental feeding may affect disease transmission. The increased visitation to supplemental feeding sites by humans, wildlife, and possibly domestic pets, coupled with greater environmental parasite loads, may promote the transmission of zoonotic diseases such as Cryptosporidium parvum or B. procyonis, both of which have been detected in many wildlife species, humans, and domestic animals (9, 10, 13). While these specific zoonotic parasites were not detected in this study, they have been detected in numerous other wildlife species that would likely be attracted to supplemental feedings sites (13, 37). This suggests that supplemental feeding could affect transmission risk of zoonotic parasitic diseases in geographic areas where they are present. This increased risk, coupled with increased human visitation to feeding sites, could be a public health concern.
Interestingly, there was an effect of feeder type with increased coccidia in feces around spin feeders, where feed is consumed off the ground, compared to gravity and trough feeders, where feed is designed to be consumed directly from the feeder (Figure 2). This suggests an elevated transmission risk from feeding practices that distribute food directly onto the ground, which is plausible particularly for parasites with fecal-oral transmission, such as coccidia (24).
Despite our hypothesis that gastrointestinal parasitism would increase over time at feeding sites, there was no effect of time for either nematodes or coccidia. It was expected that GIPs would increase with long-term feeding because the proportion of WTD using feeders increased over time in Hubert and others' study (26). However, these results were over the first 2 years of feeding whereas this study compared 1–4 years of feeding to 5–10 years of feeding. At this scale, there may not be meaningful differences in the proportion of a WTD population using feeders. Alternatively other wildlife use may not differ in time in the same way and so mitigate any effect of changing WTD use.
Giardia spp. and Cryptosporidium spp. were detected in only 3.2% of samples, which is similar to previously reported surveillance data for Mississippi WTD (Giardia spp. and Cryptosporidium spp. prevalence of 1.1 and 5.0%, respectively; (38). Giardia spp. is typically transmitted through water sources, but it can also be through direct contact with feces (39). Future studies with more samples that determine Giardia spp. and Cryptosporidum spp. species/assemblage should be conducted to determine the effects of supplemental wildlife feeding on specifically zoonotic species/assemblages. To the authors' knowledge, Baylisascaris procyonis has still not been found in Mississippi (13). This study should be repeated in a geographic area with high B. procyonis prevalence, such as Texas, to determine the effects of supplemental feeding on this parasite of high public health importance. Sampling GIPs from feces found in the field has limitations. First, freshness of collected feces was unknown, unlike studies which used freshly-deposited samples or samples collected directly from the animal (38, 40). Additionally, for Giardia, cysts are excreted sporadically during infection (41), so prevalence may have been under-estimated.
In conclusion, supplemental feeding, like other actions that concentrate animals, increases GIP environmental load which may increase disease transmission risk to wildlife, domestic pets, and humans. This effect may be mitigated by using feeders that keep feed off the ground, like gravity and trough feeders. Future research can further elucidate the relationship between feeding and GIPs by comparing isolated populations with and without feeding.
Data availability statement
The raw data supporting the conclusions of this article will be made available by the authors, without undue reservation.
Author contributions
MH contributed to project design, data collection, statistical analysis, and drafting the manuscript. SD contributed to project design and implementation. WCB contributed to project design, data collection, and drafting the manuscript. BS contributed to project design, implementation, and statistical analysis. All authors contributed to manuscript review and revision. All authors contributed to the article and approved the submitted version.
Funding
This publication is a contribution of the Forest and Wildlife Research Center, Mississippi State University and was supported by the Mississippi Department of Wildlife, Fisheries, and Parks using US Fish and Wildlife Service (Pittman-Robertson) Federal Aid in Wildlife Restoration funding.
Acknowledgments
The project would not have been possible without help from numerous landowners. We thank our collaborator, B. Navarre, and technicians: L. Resop, H. Deasy, C. Deignan, H. Miller, A. Porter, and K. Smith. We also thank T. Graham Rosser for his assistance speciating Giardia and Cryptosporidium positives.
Conflict of interest
The authors declare that the research was conducted in the absence of any commercial or financial relationships that could be construed as a potential conflict of interest.
Publisher's note
All claims expressed in this article are solely those of the authors and do not necessarily represent those of their affiliated organizations, or those of the publisher, the editors and the reviewers. Any product that may be evaluated in this article, or claim that may be made by its manufacturer, is not guaranteed or endorsed by the publisher.
References
1. Arkansas Game and Fish Commission. Think Twice Before Filling the Corn Feeder. (2019). Available online at: https://www.agfc.com/en/news/2019/08/14/think-twice-before-filling-the-corn-feeder/ [accessed June 29, 2022].
2. Inslerman RA, Miller JE, Baker DL, Kennamer JE, Cumberland R, Stinson ER, et al. Baiting and Supplemental Feeding of Game Wildlife Species. Bethesda, MD: The Wildlife Society (2006). p. 66.
3. Yuill TM. Forward. In: Friend M, Franson JC, editors. Field Manual of Wildlife Diseases. Madison, WI: United States Geological Survey (1999). p. 1.
4. Miller R, Kaneene JB, Fitzgerald SD, Schmitt SM. Evaluation of the influence of supplemental feeding of white-tailed deer (Odocoileus virginianus) on the prevalence of bovine tuberculosis in the Michigan wild deer population. J Wild Dis. (2003) 39:84–95. doi: 10.7589/0090-3558-39.1.84
5. Gompper ME, Wright AN. Altered prevalence of raccoon roundworm (Baylisascaris procyonis) owing to manipulated contact rates of hosts. J Zool. (2005) 266:215–9. doi: 10.1017/S0952836905006813
6. Brookshire WC, Dykstra A, Loftin C, Lashley M. Wildlife baiting is associated with an increased parasite intensity in raccoons (Procyon lotor) in Mississippi, USA. J Wild Dis. (2020) 56:724–6. doi: 10.7589/2019-08-197
7. Navarro-Gonzalez N, Fernandez-Llario P, Enrique P-MJ, Mentaberre G, Lopez-Martin JM, Lavin S, et al. Supplemental feeding drives endoparasite infection in wild boar in Western Spain. Vet Parasitol. (2013) 196:114–23. doi: 10.1016/j.vetpar.2013.02.019
8. Hatam-Nahavandi K, Ahmadpour E, Carmena D, Spotin A, Bangoura B, Xiao L. Cryptosporidium infections in terrestrial ungulates with focus on livestock: a systematic review and meta-analysis. Parasit Vectors. (2019) 12:23. doi: 10.1186/s13071-019-3704-4
9. Perz JF, le Blancq SM. Cryptosporidium parvum infection involving novel genotypes in wildlife from lower New York State. Appl Environ Microbiol. (2001) 67:1154–62. doi: 10.1128/AEM.67.3.1154-1162.2001
10. Smith K, Fischer J, Little S, Lockhart J, Yabsley MJ, Stallknecht DE. Diseases with implications for human health. In: Davidson W, editor. Field Manual of Wildlife Diseases in the Southeastern United States. Athens, GA: Southeastern Cooperative Wildlife Disease Study (2006). p. 405–28.
11. Lambert BC, Demarais S. Use of supplemental feed for ungulates by non-target species. Southwest Nat. (2001) 46:118–21. doi: 10.2307/3672387
12. Campbell TA, Long DB, Shriner SA. Wildlife contact rates at artificial feeding sites in Texas. Environ Manage. (2013) 51:1187–93. doi: 10.1007/s00267-013-0046-4
13. Kazacos K. Baylisascaris Larva Migrans. Reston, VA: United State Geological Survey (2016). p. 122.
14. Atwill ER, Pereira MDGC, Alonso LH, Elmi C, Epperson WB, Smith R, et al. Environmental load of Cryptosporidium parvum oocysts from cattle manure in feedlots from the central and western United States. J Environ Qual. (2006) 35:200–6. doi: 10.2134/jeq2005.0099
15. Atwill ER, Hoar B, das Graças Cabral Pereira M, Tate KW, Rulofson F, Nader G. Improved quantitative estimates of low environmental loading and sporadic periparturient shedding of Cryptosporidium parvum in adult beef cattle. Appl Environ Microbiol. (2003) 69:4604–10. doi: 10.1128/AEM.69.8.4604-4610.2003
16. Cox P, Griffith M, Angles M, Deere D, Ferguson C. Concentrations of pathogens and indicators in animal feces in the Sydney watershed. Appl Environ Microbiol. (2005) 71:5929–34. doi: 10.1128/AEM.71.10.5929-5934.2005
17. Sorensen A, van Beest FM, Brook RK. Impacts of wildlife baiting and supplemental feeding on infectious disease transmission risk: a synthesis of knowledge. Prev Vet Med. (2014) 113:356–63. doi: 10.1016/j.prevetmed.2013.11.010
18. Hoberg EP, Alan Kocan A, Rickard LG, Alan A. Gastrointestinal strongyles in wild ruminants. In: Samuel WM, Pybus MJ, Kocan AA, editors. Parasitic Diseases of Wild Mammals. Ames, IA: Iowa State University Press (2001). p. 193–227.
19. Campbell TA, VerCauteren KC. Diseases and parasites. In: Hewitt DG, editor. Biology and Management of White-Tailed Deer. Boca Raton, FL: CRC Press (2011). p. 219–50.
20. American Association of Veterinary Parasitologists. Physaloptera Rara. (2022). Available online at: https://www.aavp.org/wiki/nematodes/spirurida/physalopteroidea/physaloptera-rara/ (accessed June 23, 2022).
21. Ash LR. Development of Gnathostoma procyonis Chandler, 1942, in the first and second intermediate hosts. J Parasitol. (1962) 48:298–305. doi: 10.2307/3275591
22. Hartnett EA, Léveillé AN, French SK, Clow KM, Shirose L, Jardine CM. Prevalence, distribution, and risk factors associated with Macracanthorhynchus ingens infections in raccoons from Ontario, Canada. J Parsitol. (2018) 104:457–64. doi: 10.1645/17-202
23. Adams JH, Levine ND, Todd KS. Eimeria and sarcocystis in raccoons in Illinois. J Protozool. (1981) 28:221–2. doi: 10.1111/j.1550-7408.1981.tb02836.x
24. Hamond D. Life cycles and development of coccidia. In: Hammond D, Long P, editors. The Coccidia: Eimeria, Isospora, Toxoplasma, and Related Genera. Baltimore: University Park Press (1973). p. 46–73.
25. Gutierrez Y. Other tissue nematode infections. In: Guerrant R, Walker D, Weller P, editors. Tropical Infectious Diseases: Principles, Pathogens and Practice. Edinburgh: W.B. Saunders (2011). p. 778–87.
26. Hubert GF, Woolf A, Post G. Food habits of a supplementally-fed captive herd of white-tailed deer. J Wildl Manag. (1980) 44:740–6. doi: 10.2307/3808032
27. Kushla JD, Oldham L. Forest Soils of Mississippi. Starkville: Mississippi State University Extension Service (2017). p. 7.
28. Internet Center for Wildlife Damage Management. Scat ID (2021). Available online at: https://icwdm.org/identification/feces/scat-id/ (accessed June 29, 2022).
29. Roepstorff A, Nansen P. Epidemiology, Diagnosis and Control of Helminth Parasites of Swine. Rome: Food and Agriculture Organization of the United Nations (1998). p. 171.
30. Oates SC, Miller MA, Hardin D, Conrad PA, Melli A, Jessup DA, et al. Prevalence, environmental loading, and molecular characterization of Cryptosporidium and Giardia isolates from domestic and wild animals along the central California coast. Appl Environ Microbiol. (2012) 78:18762–72. doi: 10.1128/AEM.02422-12
31. Reed C, Henke SE, Kresta AE. Frequency of deposition and location of Baylisascaris Procyonis eggs in raccoon feces. J Wildl Dis. (2012) 48:190–4. doi: 10.7589/0090-3558-48.1.190
32. Hoskin S, Johnson M, Swanson J. Internal parasites and productivity in farmed deer. Proc NZ Soc Anim Prod. (2007) 67:102–6. Available online at: http://www.nzsap.org/proceedings/2007/internal-parasites-and-productivity-farmed-deer
33. Atwill ER, Camargo SM, Phillips R, Alonso LH, Tate KW, Jensen WA, et al. Quantitative shedding of two genotypes of Cryptosporidium parvum in California ground squirrels (Spermophilus beecheyi). Appl Environ Microbiol. (2001) 67:2840–3. doi: 10.1128/AEM.67.6.2840-2843.2001
34. Webb SL, Gee KL, Strickland BK, Demarais S, Deyoung RW. Measuring fine-scale white-tailed deer movements and environmental influences using GPS collars. Int J Ecol. (2010) 2010:12. doi: 10.1155/2010/459610
35. Chamberlain MJ, Leopold BD. Spatio-temporal relationships among adult raccoons (Procyon lotor) in central Mississippi. Am Midl Nat. (2002) 148:297–308. doi: 10.1674/0003-0031(2002)148[0297:STRAAR]2.0.CO;2
36. Mukul-Yerves JM, Zapata-Escobedo MR, Montes-Pérez RC, Rodríguez-Vivas RI, Torres-Acosta JF. Parásitos gastrointestinales y ectoparásitos de ungulados silvestres en condiciones de vida libre y cautiverio en el trópico mexicano. Rev Mex Cienc Pecu. (2015) 5:459–69. doi: 10.22319/rmcp.v5i4.4017
37. Zahedi A, Paparini A, Jian F, Robertson I, Ryan U. Public health significance of zoonotic Cryptosporidium species in wildlife: critical insights into better drinking water management. Int J Parasitol Parasites Wildl. (2016) 5:88–109. doi: 10.1016/j.ijppaw.2015.12.001
38. Rickard LG, Siefker C, Boyle CR, Gentz EJ. The prevalence of Cryptosporidium and Giardia spp. in fecal samples from free-ranging white-tailed deer (Odocoileus virginianus) in the southeastern United States. J Vet Diagn Invest. (1999) 11:65–72. doi: 10.1177/104063879901100111
39. Thompson RCA. The zoonotic significance and molecular epidemiology of Giardia and giardiasis. Vet Parasitol. (2004) 126:15–35. doi: 10.1016/j.vetpar.2004.09.008
40. Schultz SR, Barry RX, Johnson MK, Miller JE, Forbes WA. Effects of feed plots on fecal egg counts of white-tailed deer. Small Rumin Res. (1994) 13:93–7. doi: 10.1016/0921-4488(94)90036-1
Keywords: Baylisascaris procyonis, coccidia, Cryptosporidium, deer, gastrointestinal parasites, Giardia, supplemental feeding, nematodes
Citation: Huang MHJ, Demarais S, Brookshire WC and Strickland BK (2022) Analysis of supplemental wildlife feeding in Mississippi and environmental gastrointestinal parasite load. Front. Vet. Sci. 9:995437. doi: 10.3389/fvets.2022.995437
Received: 15 July 2022; Accepted: 15 August 2022;
Published: 26 September 2022.
Edited by:
Ferran Jori, UMR ASTRE–CIRAD, FranceReviewed by:
Jacob L. Bowman, University of Delaware, United StatesLuis Garcia Prieto, Universidad Nacional Autónoma de México, Mexico
Copyright © 2022 Huang, Demarais, Brookshire and Strickland. This is an open-access article distributed under the terms of the Creative Commons Attribution License (CC BY). The use, distribution or reproduction in other forums is permitted, provided the original author(s) and the copyright owner(s) are credited and that the original publication in this journal is cited, in accordance with accepted academic practice. No use, distribution or reproduction is permitted which does not comply with these terms.
*Correspondence: Miranda H. J. Huang, miranda@mirandahuang.com