Evaluation of the role of clathrin and bacterial viability in the endocytosis of Lawsonia intracellularis
- 1Department of Clinic and Surgery, Escola de Veterinária, Universidade Federal de Minas Gerais, Belo Horizonte, Minas Gerais, Brazil
- 2Department of Veterinary and Biomedical Sciences, College of Veterinary Medicine, University of Minnesota, St. Paul, MN, United States
Lawsonia intracellularis is an obligate intracellular bacterium and causative agent of proliferative enteropathy. The pathogenesis of L. intracellularis is not completely understood, including the endocytic mechanisms to access the host cell cytoplasm. In this study, we evaluated the mechanisms involved in endocytosis of L. intracellularis in vitro using intestinal porcine epithelial cells (IPEC-J2). Confocal microscopy was used to co-localize L. intracellularis and clathrin. Clathrin gene knockdown was then applied to verify whether L. intracellularis endocytosis is clathrin-dependent. Finally, internalization of viable and non-viable (bacteria were inactivated by heat) L. intracellularis organisms were assessed to study the role of the host cell during bacterial endocytosis. L. intracellularis organisms were observed co-localized with clathrin by confocal microscopy but the amount of L. intracellularis internalized in cells, with and without clathrin knockdown, did not differ statistically. The internalization of non-viable L. intracellularis showed a decrease in the internalization in cells with less clathrin synthesis (P<0.05). The present study is the first to elucidate the involvement of clathrin in the endocytosis of L. intracellularis. Clathrin-mediated endocytosis was shown to be an important, but not required, process for L. intracellularis internalization in porcine intestinal epithelial cells. Independence of bacterial viability for host cell internalization was also confirmed.
1. Introduction
Lawsonia intracellularis is an obligate intracellular, microaerophilic, Gram-negative bacterium that causes proliferative enteropathy (PE) (1). This disease is endemic in swine herds worldwide, and is also reported in other species, including non-human primates, horses, rabbits, wild mammals, and birds (2, 3).
The pathogenesis of L. intracellularis is still poorly understood. L. intracellularis infection occurs via the fecal-oral route. In the gastrointestinal tract, the microorganism overcomes the hostile environment of the stomach and localizes in the aboral region of the small intestine (ileum) (4). The bacterium uses a single polar flagellum (5) to cross the intestinal mucus barrier, allowing intimate contact with the enterocytes and subsequent host cell invasion.
Endocytosis of intracellular bacteria can occur via different routes. The zipper mechanism does not require metabolically active invaders for its initiation. The zipper endocytosis process is associated with proteins or structures of the outer membrane of the bacterium that interact directly with specific host cells receptors. In this process, there is a clustering of eukaryotic cell receptors, associated with changes in the actin cytoskeleton resulting in the microorganism being enwrapped by cytoplasmic membrane (6, 7). Then, several downstream mechanisms are activated resulting in pathogen endocytosis. In the zipper endocytosis process, there is involvement of clathrin-dependent machinery, associated with the formation of vesicles from the endocytosis of exogenous material. The involvement of this machinery occurs in the initial stage of the endocytosis process, shortly after the adhesion of the bacterium to the surface of the host cell (8). Another method used by bacteria for internalization into cells is the so-called trigger endocytosis mechanism. This process is dependent on bacterial effector proteins that are introduced into the host cells, generally through the type III secretion system (T3SS) (9). These proteins are responsible for mobilizing the actin for rearrangement of the cytoskeleton and pathogen internalization (9).
The mechanisms involved in the endocytosis of L. intracellularis are not yet fully understood. Lawson et al. (10) have demonstrated that L. intracellularis can be internalized even after its inactivation. This finding indicates that the process of L. intracellularis internalization in the host cells is dependent on the recognition of bacterial membrane proteins by the host, and hence, the clathrin-machinery would be potentially involved. Therefore, the objective of the present study was to evaluate the involvement of clathrin in the endocytosis process of viable and non-viable L. intracellularis, as well as to determine whether the bacterium uses other mechanisms for its internalization in the host cell.
2. Results
2.1. L. intracellularis and clathrin were co-localizated by confocal microscopy
The co-localization of L. intracellularis and clathrin was observed by confocal microscopy and indicates an activation of the clathrin-dependent endocytosis mechanism to internalize L. intracellularis (Figure 1).
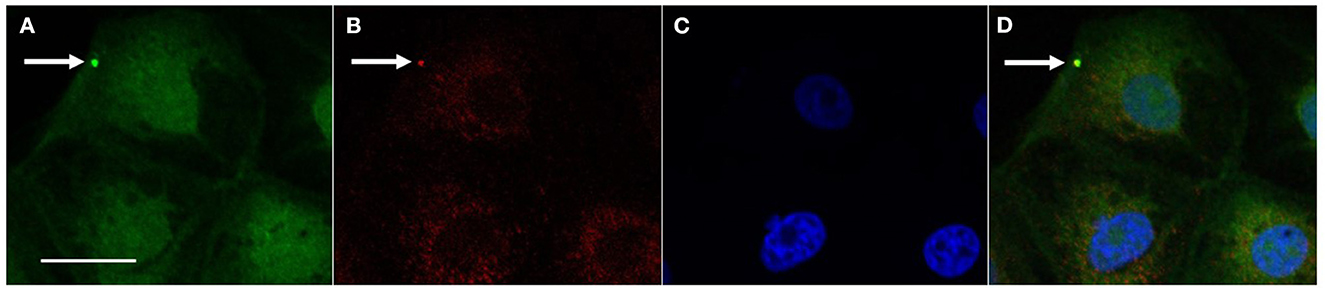
Figure 1. Co-localization of L. intracellularis and clathrin in IPEC-J2 cells 1 h after inoculation. (A) L. intracellularis fluorescent positive signal (arrow, green signal, AlexaFluor 488) and (B) Clathrin fluorescent positive signal (arrow, red signal, Cy3) were observed in the cell cytoplasm. (C) Cell nuclei (blue—DAPI) and (D) Co-localization of the fluorescent signals for L. intracellularis and clathrin (arrow, yellow signal). Confocal microscopy„ scale bar: 10 μm.
2.2. Internalization of L. intracellularis is affected by bacterial viability and clathrin expression
Clathrin knockdown by siRNA transfection was successfully demonstrated by the western blot technique (Figure 2A), and relative protein levels were reduced by 68.9 ± 2% (Figure 2B). Quantification of bacterial internalization was evaluated by cell culture lysis (control and siRNA) and bacterial extraction and quantification through RT-PCR. We observed that viable L. intracellularis had a numerically higher internalization capacity than heat-killed L. intracellularis (but not statistically, P > 0.05) in cells able to synthesize normal levels of clathrin (Figure 2C). Conversely, internalization of heat-killed L. intracellularis was significantly decreased (P < 0.05) in clathrin knockdown IPEC-J2 cells (Figure 2D), compared to non-treated cells.
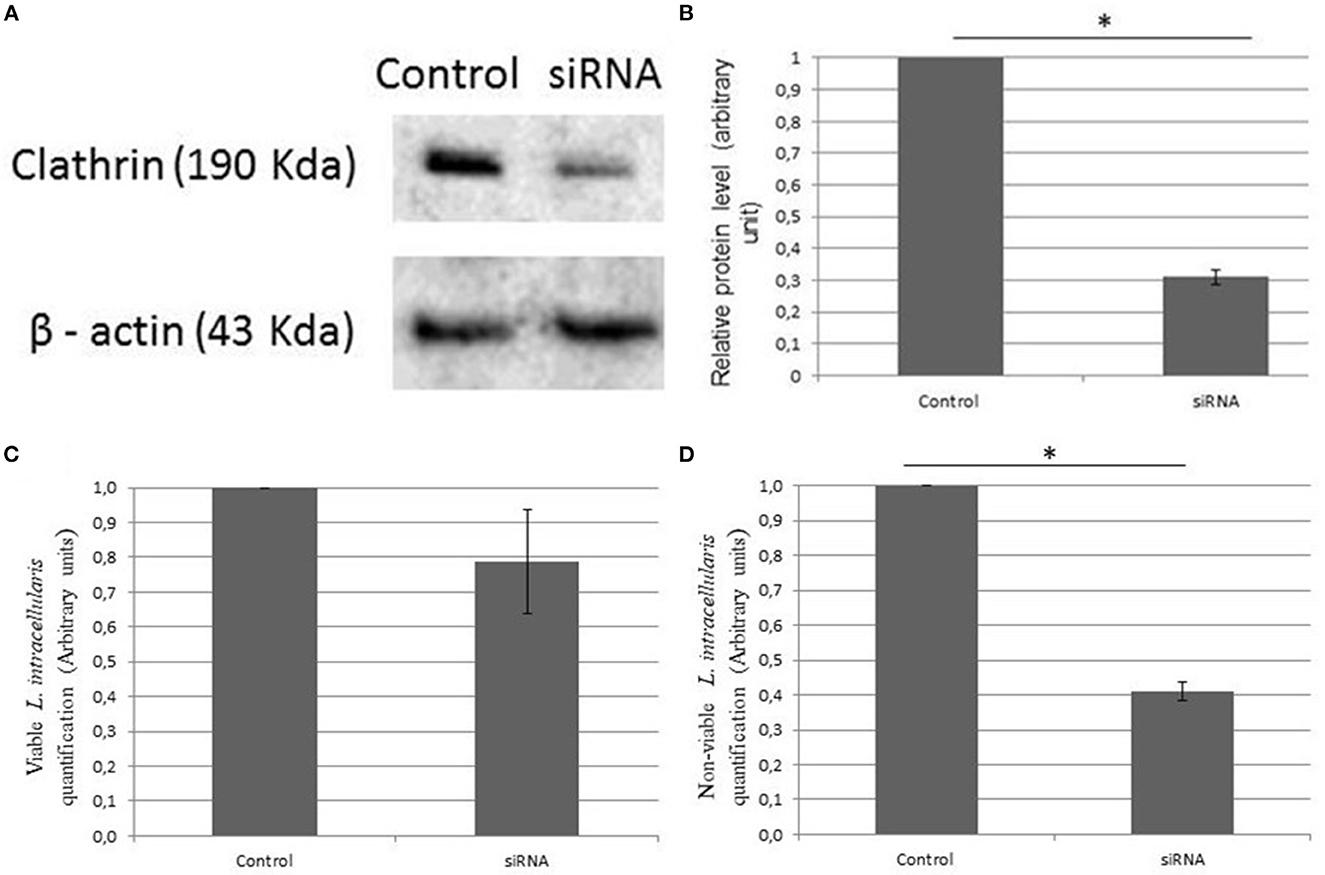
Figure 2. (A) Effect of clathrin knockdown using Western blot. The expression of clathrin in culture of IPEC-J2 infected with L. intracellularis demonstrated a lower expression rate of clathrin in the transfected group (siRNA). β-actin was used as loading control. (B) The relative protein levels analyzed by ImageJ and standard deviation. (C, D) The evaluation of the amount of L. intracellularis endocytosis in the different groups was performed using RT-PCR. Results are presented as the means ± SD of three independent experiments, using control groups (IPEC—J2 infected with L. intracellularis) and siRNA groups (IPEC—J2 knockdown to clathrin and infected with L. intracellularis). (C) Live L. intracellularis amounts did not differ statistically in the control and transfected group and (D) heat killed L. intracellularis had a reduced internalization rate (*P < 0.05) in the transfected group when compared to the control.
3. Discussion
L. intracellularis is a relatively “new” bacterium species with the first report of isolation and in vitro propagation dating from 1993 (1), and its recognition as a genus and species in 1995 (11). L. intracellularis is the single species in its genus and shares only 92% of identity with Bilophila wadsworthia, the closest bacterium species in the same family. In addition, although available in public databases, L. intracellularis genome still lacks characterization of various sequences. The reproduction of pathogenesis published studies performed with other enteropathogens such as in Salmonella enterica, Listeria monocytogenes and Escherichia coli is very difficult due to growth particularities of L. intracellularis. The lack of understanding about the molecular biology of L. intracellularis, associated with the microaerophilic and intracellular requirements for L. intracellularis in vitro growth, make L. intracellularis a unique pathogen and dictate the realization of proof-of-concept experiments before any deeper and more comprehensive experiments can be performed.
In the present study, internalization of heat-killed L. intracellularis was significantly lower (P < 0.05) when infecting IPEC-J2 cells under a clathrin gene silencing condition. The method of bacterial inactivation used in the present study (65°C/30 min) would have likely minimal impact on surface structure (12), and hence, would likely not interfere on the recognition of membrane proteins as it was evidenced by the success of the internalization of heat-killed Lawsonia intracellularis in IPEC-J2 cells with full expression of clathrin. Altogether our results showed evidence that L. intracellularis can be internalized by clathrin-dependent mechanisms without losing the property of being endocytosed by other alternative active pathway processes. In other words, based in our results it is possible that viable L. intracellularis could also be internalized by a mechanism that does not involve clathrin pathway. Further studies are needed to evaluate the intracellular traffic of L. intracellularis within epithelial cells.
The importance of L. intracellularis as a pathogen capable of causing disease of economic importance in the swine industry is well-acknowledged. Nevertheless, little information on the pathogenesis and, more specifically, on the mechanisms of entry of the bacterium into eukaryotic cells, is available. In a previous study, Lawson et al. (10) have observed that dead L. intracellularis could be visualized inside cells, suggesting that the process of endocytosis could be dependent on the eukaryotic cell only. In the present study, we evaluated the mechanisms involved in the endocytosis of L. intracellularis and confirmed, by confocal microscopy, that L. intracellularis can be internalized through clathrin-dependent mechanisms. However, the amount of live L. intracellularis organisms recovered from transfected and non-transfected IPEC-J2 cells did not differ statistically. Thus, the capacity of L. intracellularis to be internalized by other active (self-dependent) mechanisms is very likely.
The main mechanisms involved in bacterial endocytosis are the zipper and the trigger processes. The zipper process is related to the presence of proteins or outer membrane compounds on the bacterium surface that interact directly with host receptors and induce a cascade reaction that culminates with bacterial endocytosis (6, 13). The zipper mechanism has been demonstrated in Listeria monocytogenes, which expresses two proteins involved in the internalization mechanism (Internalins A and B—InlA and InlB). Those proteins mimic some host cell proteins and initiate the process of clathrin-mediated endocytosis (8, 14). Although neither InlA, InlB have been identified in L. intracellularis genome, as there still are hypothetical and uncharacterized L. intracellularis proteins in L. intracellularis genome, it is possible that some of them could participate in the bacterial endocytosis. In our study, the presence of clathrin, topographically co-localized with L. intracellularis as demonstrated by confocal microscopy, suggests that the L. intracellularis internalization process may be clathrin-dependent.
Confocal microscopy has been used to prove the colocalization of bacterial organisms with host cell markers (14–16). In these studies, a semi-quantitative analysis for the confocal microscopy was performed to reinforce the proportion of single positive signals for the bacterial organism, the host cell markers and the double positive signals, allowing more inferences from the data. In the present study the use of confocal microscopy—which specificity is high but lacks sensitivity—was designed to serve as a proof-of-concept for the colocalization of L. intracellularis and clathrin, however, a semi-quantitative analysis was not performed. Future studies should consider numerical data to support further colocalization of L. intracellularis and host cell markers. Presence of L. intracellularis in cytoplasm of IPEC-J2 cells treated with specific siRNA indicates potential internalization by other non-clathrin-dependent mechanisms. To evaluate this, IPEC-J2 cell cultures (control and knockdown for clathrin) were infected with dead L. intracellularis. This experiment resulted in a decreased internalization of heat-killed bacteria in cells with diminished clathrin. Based on this experiment results it is plausible to hypothesize that live L. intracellularis can be internalized through self-dependent mechanisms, that do not depend on clathrin expression, possibly through the process of endocytosis called trigger. In the trigger endocytosis' process, there is an initial pathogen-host interaction and, from there, bacterial proteins are secreted into the eukaryotic cell cytosol through the T3SS, an apparatus that has already been shown to be present in L. intracellularis (17). The secreted bacterial proteins (also called effector proteins) are responsible for host cytoplasmic organization by rearrangements in the actin cytoskeleton (7). This trigger mechanism is well-described in bacteria of the genus Salmonella. Salmonella species adhere to the cells through a fimbrial adhesion and then, through the T3SS-1, synthesize effector proteins responsible for actin polymerization and bacterial envelopment (18). It has been demonstrated (15) that Salmonella species can also be internalized by the zipper mechanism mediated by the Rck membrane protein, in addition to the trigger mechanism.
The present study is a proof-of-concept that L. intracellularis can be endocytosed by a clathrin-dependent mechanism and/or by mechanisms that require the bacterium's viability. Further studies are needed to determine which outer membrane proteins present in L. intracellularis have the ability to interact with host cell receptors to initiate the endocytosis process. Moreover, it is necessary to evaluate whether the T3SS, present in L. intracellularis, is involved with the internalization process and to determine the likely effector proteins synthetized by L. intracellularis.
4. Materials and methods
4.1. Cell cultures and in vitro infection
Intestinal porcine epithelial cells (IPEC-J2) (19) were cultured in T25 flasks with Dulbecco's Modified Eagle Medium (DMEM)/F12, supplemented with 5% fetal bovine serum, 5 ng/mL epidermal growth factor (E9644, Sigma-Aldrich), and 5 ng/mL mixture of insulin, transferrin and selenium (BD Biosciences), without antibiotics, at 37°C in a humidified atmosphere with 5% CO2, as previously described (20). L. intracellularis strain PHE/MN1-00 (ATCC PTA-3457), previously isolated from swine with the hemorrhagic form of proliferative enteropathy, was used at passages 12 to 14 to infect the IPEC-J2 cells. A pure culture of the bacterium was thawed and grown in McCoy cell culture incubated in a bag with an atmosphere of ~6% oxygen and 8% carbon dioxide (19, 20), for three consecutive passages to allow bacteria to recover from the freezing condition. Subsequently, the culture supernatant was filtered (0.8 μm, Millipore) to remove McCoy cells which was used as inoculum (21–23), and added into 24-well plates containing IPEC-J2 at ~30% of confluence. As some of the treatment groups were designed to verify the influence of bacterial viability in the outcomes, L. intracellularis aliquots were heat-killed by 30 min of heating at 65°C (24). To prove the efficiency of this method, an aliquot of heat-killed L. intracellularis was added to a permissive cell culture (McCoy cells at 30% confluence). After monitoring the culture for 6 days, an immunocytochemistry was performed and there were not labeling for L. intracellularis antigens (data not shown). As the aim of the immunocytochemistry is to stain L. intracellularis in the cytoplasm of the inoculated cells, a negative stain proves that there were no bacteria in the inoculum able to infected and to propagate in the IPEC-J2 cells. Experiments were performed three times, each time with triplicates, with the inoculum containing 2.1 × 105, 3.4 × 105, and 1.8 × 105 L. intracellularis/ml and 1.7 × 104, 2.4 × 104, and 2 × 104 IPEC-J2 cells/ml, in each triplicate, respectively.
The experimental groups were: (a) IPEC-J2 knockdown for clathrin (described below) inoculated with viable L. intracellularis; (b) IPEC-J2 knockdown for clathrin (described below) inoculated with heat-killed L. intracellularis (described above); (c) IPEC-J2 not transfected (control) inoculated with live L. intracellularis; and (d) IPEC-J2 not transfected (control) inoculated with heat-killed L. intracellularis. The cell cultures were allowed to be in contact with L. intracellularis microorganisms for 1 h. After that period, the supernatant was removed, and the cell monolayer was washed three times with PBS and fixed with 4% paraformaldehyde.
4.2. RNA silencing
The process of gene silencing through siRNA was performed to evaluate the requirement for clathrin-dependent endocytosis for L. intracellularis internalization. The knockdown of the gene clathrin heavy chain like 1 (CLTCL1) responsible for the synthesis of this protein was performed via silencing RNA (siRNA) followed by the inoculation of the bacteria (16).
IPEC-J2 cells were transfected using the following oligonucleotide sequences for clathrin synthesis: clathrin heavy chain sense, 5′-GGCCCAGGUGGUAAUCAUUTT-3′; clathrin heavy chain antisense, 3′-AAUGAUUACCACCUGGGCCTG-5 (16). Two days before infection, IPEC-J2 cells were incubated for 15 min with 2.5 μg of siRNA, using lipofectamine 3000 (# L3000008, Invitrogen, USA), according to the manufacturer's instructions. Then, the media was replaced with fresh IPEC-J2 media without the oligonucleotides and the cell culture was infected with L. intracellularis.
4.3. Western blot
IPEC-J2 cells transfected with siRNA and non-transfected IPEC-J2 cells were washed three times with PBS (25) and lysed with M-PER (#78501, Invitrogen, USA) for 5 min. The lysate was purified by centrifugation at 12,000 g for 10 min. Protein lysates were separated by SDS-PAGE and transferred onto a nitrocellulose membrane and labeled with anti-clathrin antibody (P1663—Cell Signaling, Danvers, MA, USA). β-actin detection was used as a loading control for Western blot experiments with anti-β-actin antibody (C4—sc47778, Santa Cruz Biotechnology). Semi-quantification of protein concentration was performed using imageJ software (National Institute of Health; download from http://rsbweb.nih.gov/ij/).
4.4. Confocal microscopy
IPEC-J2 cells were grown on coverslips in 24-well plates and infected with L. intracellularis at a multiplicity of infection of 10 at 37°C for 1 h. This period of incubation was chosen after performing a pilot test was performed in which four periods of incubation were tested (15, 30 min, 1 and 2 h) and the highest number of fluorescent signals (L. intracellularis and clathrin) was observed at 1 h (data not shown). Subsequently, the cells were washed three times with PBS and fixed in 4% paraformaldehyde for 10 min at room temperature. Thereafter, further washing with PBS was carried out to remove excess paraformaldehyde. Fixed cells were incubated for 30 min at 37°C with the following primary antibodies: anti-clathrin (P1663—Cell Signaling, Danvers, MA, USA; source: rabbit), and anti-L. intracellularis (source: mouse) (20). After washing with PBS, the secondary antibodies goat anti-rabbit IgG conjugated to Cy3 (ab97075—Abcam) and goat anti-mouse IgG conjugated to AlexaFluor 488 (ab150113—Abcam) were added and incubated for 30 min at 37°C. Finally, the coverslips were mounted on glass slides with mounting media containing DAPI (ProLong Diamond Antifade Mountant—P36971—ThermoFisher, USA) and imaged under confocal microscope (FV500, Olympus).
4.5. Quantification of L. intracellularis
To quantify L. intracellularis after 1 h of culture, the IPEC-J2 cells were washed three times with PBS to remove extracellular bacteria (25), lysed and processed for intracellular bacterial DNA extraction using DNeasy Blood & Tissue Kit (#D6321-01, Qiagen, Valencia, CA, USA), according to instructions from the manufacturer. The extracted DNA was quantified by real time PCR (RT-PCR) at the University of Minnesota Veterinary Diagnostic Laboratory (UMN/VDL) using standardized methods to estimate the quantity of L. intracellularis. The Ct values obtained from the RT-PCR were converted to numbers of bacteria/ml according to an equation previously validated by the UMN/VDL (26). The mean of the results was converted to arbitrary unit (AU) to simplify data presentation, with the results of the positive control group converted to 1 AU and the results of the other treatment group were proportionally converted to the positive control AU.
4.6. Statistical analysis
Quantitative data were presented with mean and standard deviation. Statistical analysis was performed using Student's paired t-test and considered significant when P < 0.05. The software Prism GraphPad version 5.0 was used for statistical analysis.
5. Conclusions
In conclusion, the present study has demonstrated the involvement of clathrin in the endocytic process of L. intracellularis and has also confirmed that L. intracellularis can be internalized through mechanisms that depend on its viability.
Data availability statement
The datasets presented in this study can be found in online repositories. The names of the repository/repositories and accession number(s) can be found in the article/supplementary material.
Author contributions
CP, TR, FV, CG, and RG: conceptualization and formal analysis. CP, TR, and AD: methodology. CP and TR: software. CP, TR, and FV: validation. CP, TR, CG, and RG: data curation. CP: writing—original draft preparation. TR, AD, FV, CG, and RG: writing, reviewing, and editing. CG and RG: funding acquisition. All authors have read and agreed to the published version of the manuscript.
Funding
TR had a scholarship from Coordenação de Aperfeiçoamento de Pessoal de Nível Superior (CAPES), Brazil. RG had a research fellowship from Conselho Nacional de Desenvolvimento Científico e Tecnológico (CNPq). CP was supported by the Capes Foundation and Ministry of Education of Brazil.
Conflict of interest
The authors declare that the research was conducted in the absence of any commercial or financial relationships that could be construed as a potential conflict of interest.
Publisher's note
All claims expressed in this article are solely those of the authors and do not necessarily represent those of their affiliated organizations, or those of the publisher, the editors and the reviewers. Any product that may be evaluated in this article, or claim that may be made by its manufacturer, is not guaranteed or endorsed by the publisher.
References
1. Lawson GH, McOrist S, Jasni S, Mackie RA. Intracellular bacteria of porcine proliferative enteropathy: cultivation and maintenance in vitro. J Clin Microbiol. (1993) 31:1136–42. doi: 10.1128/jcm.31.5.1136-1142.1993
2. Cooper DM, Swanson DL, Gebhart CJ. Diagnosis of proliferative enteritis in frozen and formalin-fixed, paraffin-embedded tissues from a hamster, horse, deer and ostrich using a Lawsonia intracellularis-specific multiplex PCR assay. Vet Microbiol. (1997) 54:47–62. doi: 10.1016/S0378-1135(96)01264-3
3. Lafortune M, Wellehan JFX, Jacobson ER, Troutman JM, Gebhart CJ, Thompson MS. Proliferative enteritis associated with Lawsonia intracellularis in a Japanese macaque (Macaca fuscata). J Zoo Wildl Med. (2004) 35:549–52. doi: 10.1638/03-080
4. Vannucci FA, Gebhart CJ. Recent advances in understanding the pathogenesis of Lawsonia intracellularis infections. Vet Pathol. (2014) 51:465–77. doi: 10.1177/0300985813520249
5. Lawson GHK, Gebhart CJ. Proliferative enteropathy. J Comp Pathol. (2000) 122:77–100. doi: 10.1053/jcpa.1999.0347
6. Pizarro-Cerdá J, Cossart P. Subversion of phosphoinositide metabolism by intracellular bacterial pathogens. Nat Cell Biol. (2004) 6:1026–33. doi: 10.1038/ncb1104-1026
7. Cossart P, Helenius, A. Endocytosis of viruses and bacteria. CSH Perspect Biol. (2014) 6:a016972. doi: 10.1101/cshperspect.a016972
8. Bonazzi M, Vasudevan L, Mallet A, Sachse M, Sartori A, Prevost M-C, et al. Clathrin phosphorylation is required for actin recruitment at sites of bacterial adhesion and internalization. J Cell Biol. (2011) 195:525–36. doi: 10.1083/jcb.201105152
9. Misselwitz B, Kreibich SK, Rout S, Stecher B, Periaswamy B, Hardt W-D. Salmonella enterica serovar typhimurium binds to HeLa cells via fim-mediated reversible adhesion and irreversible type three secretion system 1-mediated docking. Infect Immun. (2011) 79:330–41. doi: 10.1128/IAI.00581-10
10. Lawson GH, Mackie RA, Smith DG, McOrist S. Infection of cultured rat enterocytes by Ileal symbiont intracellularis depends on host cell function and actin polymerisation. Vet Microbiol. (1995) 45:339–50. doi: 10.1016/0378-1135(94)00142-J
11. McOrist S, Gebhart CJ, Boid R, Barns M. Characterization of Lawsonia intracellularis gen. nov., sp. nov., the obligately intracellular bacterium of porcine proliferative enteropathy. Int J Syst Bacteriol. (1995) 45:820–5. doi: 10.1099/00207713-45-4-820
12. Rathman M, Sjaastad MD, Falkow, S. Acidification of phagosomes containing Salmonella typhimurium in murine macrophages. Infect. Immunity. (1996) 64:2765–73. doi: 10.1128/iai.64.7.2765-2773.1996
13. Pizarro-Cerdá J, Cossart P. Bacterial adhesion and entry into host cells. Cell. (2006) 124:715–27. doi: 10.1016/j.cell.2006.02.012
14. Veiga E, Guttman JA, Bonazzi M, Boucrot E, Toledo-Arana A, Lin AE, et al. Invasive and adherent bacterial pathogens co-Opt host clathrin for infection. Cell Host Microbe. (2007) 2:340–51. doi: 10.1016/j.chom.2007.10.001
15. Cain RJ, Hayward RD, Koronakis V. Deciphering interplay between Salmonella invasion effectors. PLoS Pathog. (2008) 4:e1000037. doi: 10.1371/journal.ppat.1000037
16. Veiga E, Cossart P. Listeria hijacks the clathrin-dependent endocytic machinery to invade mammalian cells. Nat Cell Biol. (2005) 7:894–900. doi: 10.1038/ncb1292
17. Alberdi MP, Watson E, McAllister GEM, Harris JD, Paxton EA, Thomson JR, et al. Expression by Lawsonia intracellularis of type III secretion system components during infection. Vet Microbiol. (2009) 139:298–303. doi: 10.1016/j.vetmic.2009.06.022
18. Berschneider HM. Development of normal cultured small intestinal epithelial cell lines which transport Na and Cl. Gastroenterol. (1989) 96 (Suppl. 2):A41.
19. Vannucci FA, Wattanaphansak S, Gebhart CJ. An alternative method for cultivation of Lawsonia intracellularis. Clin Microbiol. (2012) 50:1070–2. doi: 10.1128/JCM.05976-11
20. Guedes RMC, Gebhart CJ. Preparation and characterization of polyclonal and monoclonal antibodies against Lawsonia intracellularis. J Vet Diagn Inv. (2003) 15:438–46. doi: 10.1177/104063870301500506
21. Resende TP, Pereira CER, Daniel AGd, Vasquez E, Saqui-Salces M, Vannucci FA, et al. Effects of Lawsonia intracellularis infection in the proliferation of different mammalian cell lines. Vet. Microbiol. (2019) 228:157–64. doi: 10.1016/j.vetmic.2018.11.029
22. Resende TP, Medida RL, Guo Y, Vannucci FA, Saqui-Salces M, Gebhart C. Evaluation of mouse enteroids as a model for Lawsonia intracellularis infection. Vet. Res. (2019) 50:1–11. doi: 10.1186/s13567-019-0672-9
23. Resende TP, Medida RL, Vannucci FA, Saqui-Salces M, Gebhart C. Evaluation of swine enteroids as in vitro models for Lawsonia intracellularis infection. J An Sci. (2020) 98:skaa011. doi: 10.1093/jas/skaa011
24. Li X-M, Srivastava K, Grishin A, Huang C-K, Schofield B, Burks W, et al. Persistent protective effect of heat-killed Escherichia coli producing “engineered,” recombinant peanut proteins in a murine model of peanut allergy. J Allergy Clin. Immunol. (2003) 112:159–67. doi: 10.1067/mai.2003.1622
25. Pereira CER, Resende TP, Armién AG, Laub RP, Vannucci FA, Santos RL, et al. Survival of Lawsonia intracellularis in porcine peripheral blood monocyte-derived macrophages. PLoS ONE. (2020) 15:e0236887. doi: 10.1371/journal.pone.0236887
Keywords: endocytosis, intracellular bacteria, infection, pathogenesis, proliferative enteropathy
Citation: Pereira CER, Resende TP, Daniel AGdS, Vannucci FA, Gebhart C and Guedes RMC (2023) Evaluation of the role of clathrin and bacterial viability in the endocytosis of Lawsonia intracellularis. Front. Vet. Sci. 10:1005676. doi: 10.3389/fvets.2023.1005676
Received: 28 July 2022; Accepted: 10 January 2023;
Published: 30 January 2023.
Edited by:
Anbu K. Karuppannan, Tamil Nadu Veterinary and Animal Sciences University, IndiaReviewed by:
Allen Page, University of Kentucky, United StatesFernando L. Leite, Boehringer Ingelheim Animal Health Business Unit, United States
Copyright © 2023 Pereira, Resende, Daniel, Vannucci, Gebhart and Guedes. This is an open-access article distributed under the terms of the Creative Commons Attribution License (CC BY). The use, distribution or reproduction in other forums is permitted, provided the original author(s) and the copyright owner(s) are credited and that the original publication in this journal is cited, in accordance with accepted academic practice. No use, distribution or reproduction is permitted which does not comply with these terms.
*Correspondence: Roberto Mauricio Carvalho Guedes, guedes@vet.ufmg.br