Increased thrombin activatable fibrinolysis inhibitor activity is associated with hypofibrinolysis in dogs with sepsis
- 1Department of Clinical Sciences, College of Veterinary Medicine, Cornell University, Ithaca, NY, United States
- 2Comparative Coagulation Laboratory, Animal Health Diagnostic Center, Cornell University, Ithaca, NY, United States
Introduction: Disorders of coagulation are well-recognized in dogs with sepsis, but data regarding fibrinolysis disorders are limited. We aimed to characterize fibrinolysis in dogs with sepsis compared to healthy controls. We hypothesized that dogs with sepsis would be hypofibrinolytic, and that hypofibrinolysis would be associated with non-survival.
Methods: This was a prospective observational cohort study. We enrolled 20 client-owned dogs with sepsis admitted to the Cornell University Hospital for Animals and 20 healthy pet dogs. Coagulation and fibrinolytic pathway proteins including antiplasmin activity (AP), antithrombin activity (AT), thrombin activatable fibrinolysis inhibitor activity (TAFI), D-dimer concentration, fibrinogen concentration, and plasminogen activity were measured and compared between groups. Overall coagulation potential, overall fibrinolysis potential, and overall hemostatic potential were calculated from the curve of fibrin clot formation and lysis over time.
Results: Compared to healthy controls, dogs with sepsis had lower AT (P = 0.009), higher AP (P = 0.002), higher TAFI (P = 0.0385), and higher concentrations of fibrinogen (P < 0.0001) and D-dimer (P = 0.0001). Dogs with sepsis also had greater overall coagulation potential (P = 0.003), overall hemostatic potential (P = 0.0015), and lower overall fibrinolysis potential (P = 0.0004). The extent of fibrinolysis was significantly negatively correlated with TAFI. No significant differences were observed between survivors and non-survivors.
Discussion: Dogs with sepsis were hypercoagulable and hypofibrinolytic compared to healthy dogs, suggesting potential utility of thromboprophylaxis in this patient population. The association between high TAFI and low overall fibrinolysis potential might provide a potential mechanism for this hypofibrinolysis.
Introduction
Sepsis is a major cause of morbidity and mortality in dogs (1), and is defined as the dysregulated host response to infection that causes organ dysfunction (2), including disorders of the hemostatic system (3). Sepsis is associated with development of a procoagulant state (4, 5), that can manifest as disseminated intravascular coagulation (DIC) (6), perpetuating organ damage (7), and causing clinical thrombosis (8–11). This procoagulant state results from activity of proinflammatory cytokines (12–15), that induce de novo intravascular tissue factor expression (16, 17), and diminish concentrations of endogenous inhibitors and anticoagulants, including thrombin-activatable fibrinolysis inhibitor (TAFI), antithrombin (AT) and protein C (3, 5, 18).
Suppression of the fibrinolytic system is an important predictor of development of multiple organ dysfunction syndrome (MODS) and mortality from sepsis in humans (19–21). The mechanism of fibrinolytic dysregulation in sepsis is complex. Plasmin, the primary effector of fibrinolysis, is generated from cleavage of plasminogen by tissue plasminogen activators (tPA). Low plasminogen concentrations preceded development of thrombocytopenia in a case series of humans with sepsis (22), and have been described in dogs with DIC due to cancer, pancreatitis and sepsis (23). Increased tPA activity occurs during sepsis to promote fibrinolysis (21), however simultaneous upregulation of fibrinolysis inhibitors oppose this action (24). The primary inhibitor of tPA is plasminogen activator inhibitor-1 (PAI-1). PAI-1 is an acute phase reactant and increased PAI-1 activity has been documented in humans with sepsis (24, 25). These patients also have decreased antiplasmin (AP) activities (19), attributed to increased formation and clearance of plasmin-antiplasmin complexes (26–28). In humans with sepsis, alterations in fibrinolytic pathway proteins predict thrombocytopenia (22), and have prognostic value (29, 30). Fibrinolysis is also inhibited by the plasma carboxypeptidase, thrombin activatable fibrinolysis inhibitor (TAFI). Activated TAFI removes the carboxyl-terminal lysine residues from fibrin that promote plasminogen binding to fibrin and enhance plasmin-mediated fibrinolysis (21). In humans with sepsis, decreased TAFI activity is associated with organ dysfunction (31, 32), and consumption of TAFI is an independent predictor of mortality (29).
Fibrinolytic pathway proteins including AP, plasminogen and TAFI have been studied in dogs with babesiosis, cancer and endocrinopathies (33–35), but similar studies of fibrinolysis in dogs with sepsis have not been performed. Given the complexity of the coagulation system (36, 37), simultaneous analysis of multiple fibrinolysis proteins might aid assessment of the disturbances present in dogs with sepsis. The main objective of our study was to characterize fibrinolysis in dogs with sepsis through measurement of individual fibrinolytic pathway proteins, combined with assessment of a global test of fibrinolysis, referred to as overall hemostasis potential (OHP) (38, 39). We hypothesized that dogs with sepsis are hypofibrinolytic, have fibrinolysis profiles distinct from those of healthy dogs, and that hypofibrinolysis is associated with non-survival.
Materials and methods
Study design
This was a prospective observational cohort study of client-owned dogs with sepsis admitted to the Cornell University Hospital for Animals. Dogs were eligible for enrollment if they weighed >5 kg, had a documented clinical syndrome associated with systemic infection (such as pyometra, septic peritonitis or pneumonia) and satisfied ≥2 systemic inflammatory response syndrome (SIRS) criteria, specifically: hypo- or hyper-thermia, temperature <37.8 or >39.4°C (<100.0 or >102.9°F); tachycardia, heart rate >140 bpm; tachypnea, respiratory rate >20 bpm; leukopenia or leukocytosis, <6 × 103/μL or >16 × 103/μL or >3% band neutrophils (3, 40). When bacterial cultures were not performed, or were negative, sepsis was confirmed by alternative means e.g., confirmation of gastrointestinal content leakage, surgical lesion identification, or determination through radiographic criteria. Dogs were ineligible if they had sepsis due to viral disease e.g., parvovirus or fungal disease e.g., candidiasis. To protect enrolled animals from potential complications associated with venipuncture, dogs with severe anemia, coagulopathy, or thrombocytopenia (Hb <5 g/dL; PT or aPTT >150 % normal; platelets <30 × 103/μL) were excluded. Dogs not expected to live more than 12 h were also ineligible. Dogs were enrolled with written informed client consent. The local Institutional Animal Care and Use Committee approved the study protocol (Cornell IACUC Protocol #2014-0053). Healthy dogs were recruited from staff-owned pets and were eligible for the study if they weighed >5 kg, were aged between 1 and 9 y, had no chronic or recent illness, and had received no medications other than preventative healthcare (e.g., parasiticides) in the preceding 3 months. Dogs were classified as healthy based on history, physical examination, and the results of complete blood count and serum biochemistry profile results.
Case management and evaluation
Primary clinicians determined all aspects of case management. Signalment and physical examination findings at hospital admission were recorded. Blood gases, electrolytes and lactate concentrations were measured immediately after sample collection with a point-of-care device (RapidPoint 500, Siemens Healthcare, Malvern, PA). Complete blood counts (CBC) (ADVIA 2120, Siemens Healthcare) with clinical pathologist review and serum biochemistry profiles (Cobas C501, Roche Diagnostics, Indianapolis, IN) were analyzed immediately whenever possible, but always within 48 h of collection. Mentation score, blood glucose, albumin and lactate concentrations and platelet counts were used to calculate the acute patient physiologic and laboratory evaluation illness severity score (APPLEfast) (41, 42). Outcome status at discharge was recorded as survived, died, or euthanized. Blood samples were collected at study entry, and prior to administration of any antithrombotic medications, into evacuated tubes (Vacutainer, BD and Co, Franklin Lakes, NJ) containing no-additive (for serum biochemistry analyses), 3.2% sodium citrate (1:9 ratio) (for coagulation testing) and K2-EDTA (for complete blood counts). Citrate plasma was prepared from whole blood by centrifugation for 10 min at 1,370 g (Ultra-8V Centrifuge, LW Scientific, Lawrenceville, GA). Plasma was transferred into polypropylene freezer tubes (Polypropylene Screw-Cap Microcentrifuge Tubes, VWR, Radnor, PA) with some plasma deliberately left in each tube to minimize the risk of cell contamination and frozen at −80°C pending batch analysis.
Coagulation and fibrinolysis testing
Determination of antiplasmin activity (AP), antithrombin activity (AT), D-dimer concentration, fibrinogen concentration (Clauss fibrinogen) and plasminogen activity was performed using an automated instrument with mechanical and spectrophotometric endpoint detection modes (STA Compact Max, Diagnostica Stago, Parsippany, NJ). Plasma AP and AT were measured with the manufacturer's synthetic chromogenic substrate kits (Stachrom Antiplasmin and Stachrom AT III, Diagnostica Stago). The AP assay is configured with a plasmin substrate and human plasmin reagent such that residual cleavage of the substrate is inversely proportional to AP in the test plasma. The AP standard curve was derived from a human plasma standard, with results reported as percentage of the human standard. The AT assay was modified using a pooled canine plasma standard (prepared at the Coagulation Laboratory from 20 healthy dogs). The pooled canine plasma had an assigned value of 100% AT and results were reported as percentage of the canine standard. Plasma D-dimer concentration was measured using a quantitative, turbidimetric immunoassay and the manufacturer's human D-dimer calibration standard (HemosIL D-dimer and Calibrator, Instrumentation Laboratory, Lexington, MA). Fibrinogen concentrations were measured via the Clauss method using a human thrombin reagent (STA Fibrinogen 100 U/mL, Diagnostica Stago) and the canine plasma standard. The fibrinogen content of the plasma standard was determined by gravimetric method (43). Concentrations of D-dimer and fibrinogen were reported as ng/mL and mg/dL, respectively. Plasminogen activity was measured based on cleavage of a chromogenic plasmin substrate (S-2251, Diapharma, West Chester, OH) following sample incubation with urokinase (Prospec, East Brunswick, NJ), as previously described (44). The assay was modified by an initial acidification/neutralization step and results were reported as the percentage plasminogen activity of a pooled canine plasma standard with an assigned value of 100%.
Quantitation of TAFI activity was performed as previously reported, with minor modifications (18). Briefly, canine TAFI activity was analyzed using a commercial kinetic chromogenic assay kit (Pefakit TAFI, Pentafarm, Basel, Switzerland). The assay uses a thrombin-thrombomodulin complex reagent to activate TAFI in the test plasma. Activated TAFI then acts on a synthetic chromogenic TAFI substrate. Plasma samples were diluted 1:2 in 0.9% sodium chloride prior to analysis. Diluted plasma samples were combined with the thrombin-thrombomodulin reagent in a 1:10 ratio in a 96-well microtiter plate and incubated for 3 min at 37°C before addition of the synthetic substrate. Upon addition of the substrate, the absorbance at 405 nm of each well was monitored every 10 s for 5 min in an automated plate reader (Cytation 1, BioTek Agilent, Santa Clara, CA). All measurements were run in duplicate. The activity of TAFI was expressed as percent activity of a pooled human plasma provided as a calibrator.
Fibrin clot formation and lysis over time in patient plasma was evaluated in the OHP assay (38, 39). The assay was configured with paired reaction mixtures containing test plasma and thrombin to generate a coagulation curve, and test plasma with thrombin and tPA to generate a fibrinolysis curve (39, 45). The assay was performed as previously described (45). In brief, coagulation and lysis reactions were performed in flat-bottom 96-well microtiter plates containing seventy-five microliters of plasma and a buffer containing bovine alpha-thrombin (final concentration 0.05 U/mL). The lysis reactions also contained human recombinant tPA (final concentration 350 ng/mL). After addition of the buffer containing coagulation and fibrinolysis activators to the test plasma, absorbance at 405 nm was measured every minute for 60 min and plotted over time to visualize changes in turbidity related to fibrin formation and degradation. The parameter overall coagulation potential (OCP) was defined as the area under the coagulation curve. This parameter is a measure of the rate and amount of fibrin formation. The OHP parameter was defined as the area under the curve in the lysis reaction containing thrombin and tPA, and thus depends on both fibrin formation and fibrinolysis. The overall fibrinolysis potential (OFP) parameter is derived from the OCP and OHP values. The OFP is calculated as the relative difference in area between the coagulation and lysis curves: OFP% = (OCP – OHP)/OCP × 100.
Statistical methods
Continuous data (e.g., dog characteristics, physical examination findings and clinicopathologic values) were assessed for normality using the D'Agostino Pearson test and appropriate descriptive statistics calculated. Comparisons between groups were performed using unpaired t-tests with Welch's correction for normally distributed data or the Mann-Whitney U test when data were non-parametric. Correlations between coagulation parameters were evaluated using Spearman's correlation coefficients, associated P-values and scatterplots. Strength of correlation was assessed as follows: <0.5 weak, 0.5–0.6 mild, 0.6–0.7 moderate, 0.7–0.8 strong, 0.8–0.9 very strong, 0.9–1.0 excellent. The overall pattern of coagulation values in dogs with sepsis were compared with those in healthy dogs using radar plots (Excel for Mac, Microsoft, Redmond, WA). Coagulation variable data for each dog was ratioed against the midpoint of the corresponding reference interval. The within-group means of these fold values corresponding to the degree of divergence from the reference interval were then overlaid on a hexagonal reference chart to allow visual comparison of dogs with sepsis with healthy controls. Using comparisons against the relevant reference interval, the coagulation disturbances in each dog with sepsis was classified as hyperfibrinolytic, hypofibrinolytic, mixed disturbance, or no disorder. Dogs satisfying 3/5 of the following criteria: low TAFI, low fibrinogen, high D-dimer, low AP, and low plasminogen were classified as hyperfibrinolytic, most consistent with a bleeding risk. Dogs satisfying 3/5 of the following criteria: high TAFI, high fibrinogen, high AP, low AT, and high plasminogen were classified as hypofibrinolytic, most consistent with a thrombotic risk. Dogs with disturbances characteristic of more than one type of disturbance were categorized as having a mixed disorder. Dogs with 4/6 parameters within the reference interval were classified as no disorder. The association between coagulation status classification (hypofibrinolytic versus other) and outcome was assessed with Fisher's exact test. Statistical analyses were performed using commercial software (Prism 9 for macOS, GraphPad, La Jolla, CA) with alpha set at 0.05. No post-hoc corrections were made for multiple comparisons because all between group comparisons were based on a priori hypotheses.
Results
Animals
A total of 40 dogs were enrolled; 20 dogs with sepsis and 20 healthy controls. The 20 dogs with sepsis had a variety of different diseases, specifically four dogs had abscesses or cellulitis, three dogs had peritonitis, three dogs had pneumonia, three dogs had pyometra, and two dogs had mastitis. Other causes included anaplasmosis, gastroenteritis (with bacteremia), osteomyelitis, pyothorax and urosepsis (all n = 1). Of the 20 dogs, three were euthanized for disease severity prior to discharge, the remainder survived to discharge, equivalent to a 15% case fatality rate. Of the 17 dogs that survived to hospital discharge, 16 dogs were alive at day 28, with 1 dog lost to follow up, equivalent to a 16% 28-day case fatality rate. Demographic characteristics, initial assessments and clinicopathologic variables are summarized in Table 1. Dogs had been treated with a variety of medications prior to study enrolment, summarized in Table 2. Positive cultures were obtained in 64% (9/14) dogs for which culture samples were submitted. Bacterial organisms cultured from the dogs included Escherichia coli (n = 4), Staphylococcus pseudintermedius (n = 2), Actinomyces canis, Bacteroides spp., Clostridium perfringens, Enterococcus faecium, Fusobacterium sp., Microbacterium phyllosphaerae, Mycoplasma sp., Peptostreptococcus sp., and Pseudarthrobacter sp. (all n = 1).
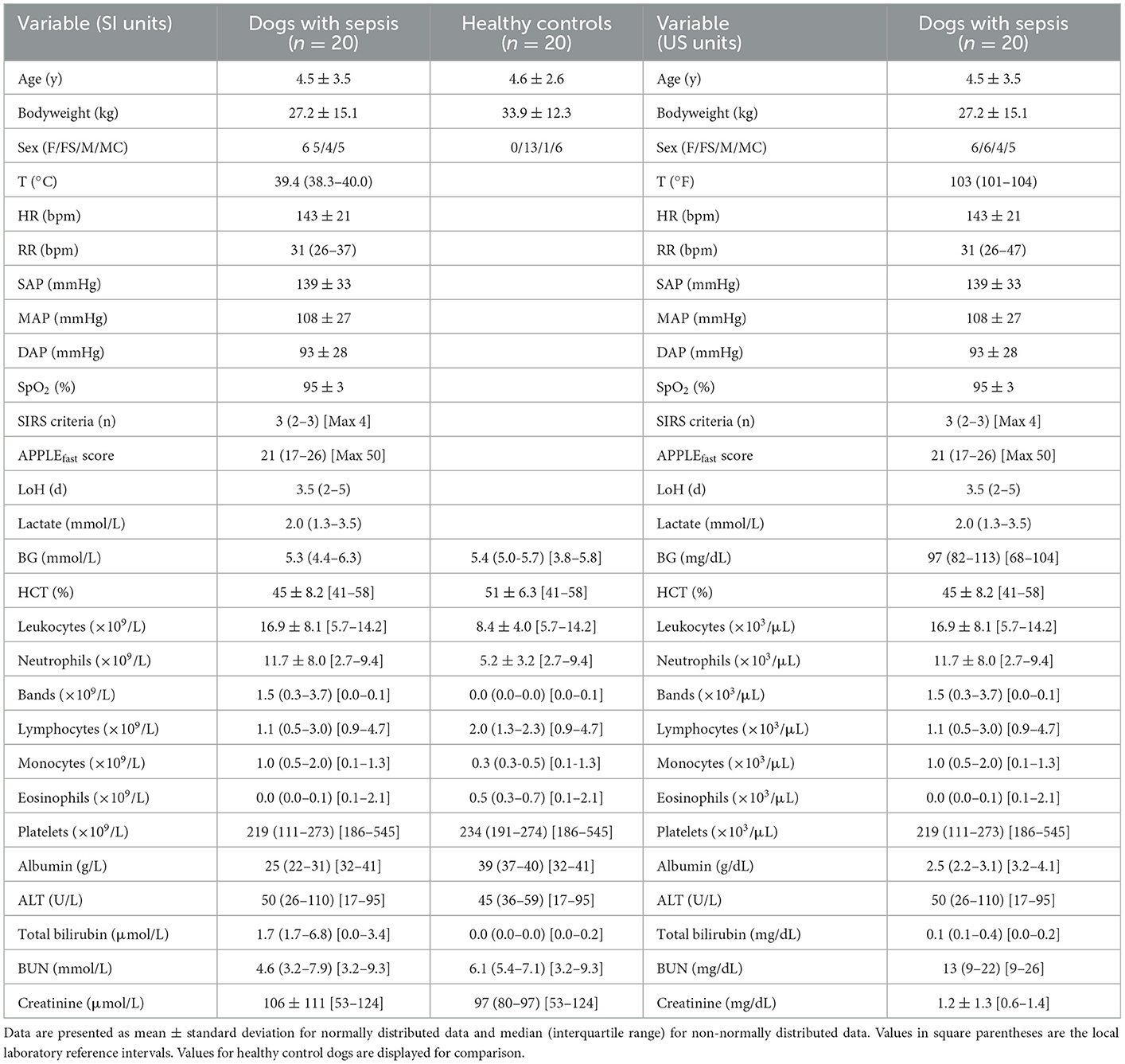
Table 1. Summary of population characteristics including complete blood count and serum biochemistry data from study entry for dogs with sepsis in both SI and US units.
Coagulation and fibrinolysis assays
All coagulation and fibrinolysis test results are summarized in Table 3. Compared to healthy controls, dogs with sepsis had significantly lower AT, higher AP and TAFI, and higher concentrations of clottable fibrinogen and D-dimer. No difference in plasminogen activity was observed between the two groups (Figure 1). Control samples on two OHP assay plates generated inappropriately low OHP values, indicating an assay error. Study samples quantitated on these plates were excluded from subsequent analyses and lack of sample volume precluded repeating these tests. Comparison of OHP assay results for the remaining septic dogs (n = 9) and controls (n = 10), revealed that septic dogs had significantly greater OHP, OCP and smaller OFP compared to healthy controls (Figure 2). Only two pairs of variables were associated with a Spearman correlation coefficient >0.7 (strong or better). The activity of the fibrinolysis inhibitor, TAFI, was negatively correlated with the extent of fibrinolysis, measured as % OFP (rs −0.817, P = 0.011, Figure 3A), and the OCP was positively correlated with illness severity as assessed by APPLEfast score (rs 0.729, P = 0.033, Figure 3B). A single graphical display of the 6 individual tests revealed that fibrinogen and D-dimer concentrations in the septic dog group demonstrated the most profound deviations from reference intervals (Figure 4). No significant differences between the test values of septic dog survivors and non-survivors were observed (Supplementary material S1). Similarly, none of the coagulation variables were significantly associated with duration of hospitalization. Classification of dogs with sepsis based on the number of test parameters outside of their corresponding reference intervals suggested that seven dogs had a mixed disorder, seven dogs had no disorder, five dogs appeared hypofibrinolytic, and one dog appeared hyperfibrinolytic. There was no association between hypofibrinolytic status and non-survival to hospital discharge, relative risk 1.56 (0.97–4.07), P = 0.140.
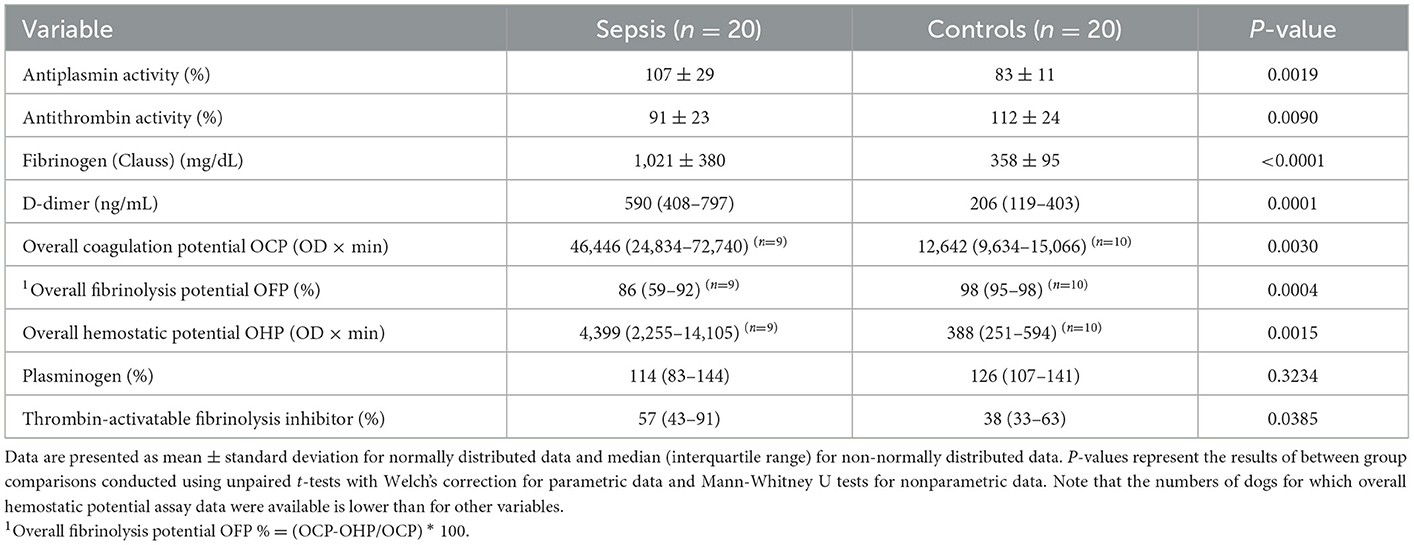
Table 3. Descriptive statistics summarizing the coagulation and fibrinolysis test results from dogs with sepsis compared to healthy controls.
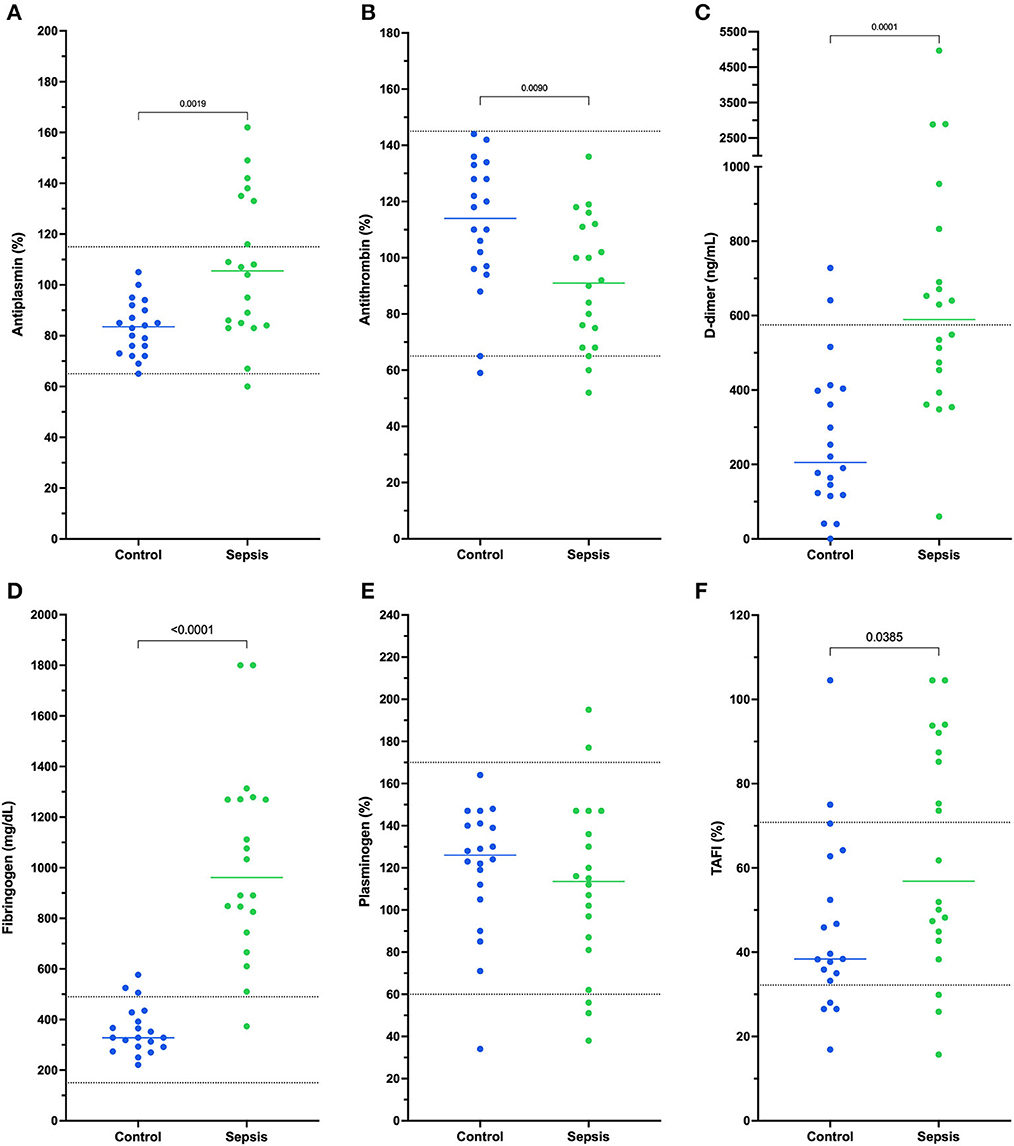
Figure 1. Dotplots of the activities of (A) antiplasmin (%), (B) antithrombin (%), concentrations of (C) D-dimer (ng/mL) and (D) fibrinogen (mg/dL) and activities of (E) plasminogen (%), and (F) thrombin-activatable fibrinolysis inhibitor (TAFI, %) in dogs with sepsis compared to healthy controls. Comparisons were performed using unpaired t-tests with Welch's correction or the Mann-Whitney U-test as appropriate based on data distribution. Compared to healthy controls, dogs with sepsis have significantly increased activities of antiplasmin and TAFI, significantly decreased antithrombin activity, and significantly increased concentrations of fibrinogen and D-dimer. Horizontal dotted lines represent laboratory reference interval bounds.
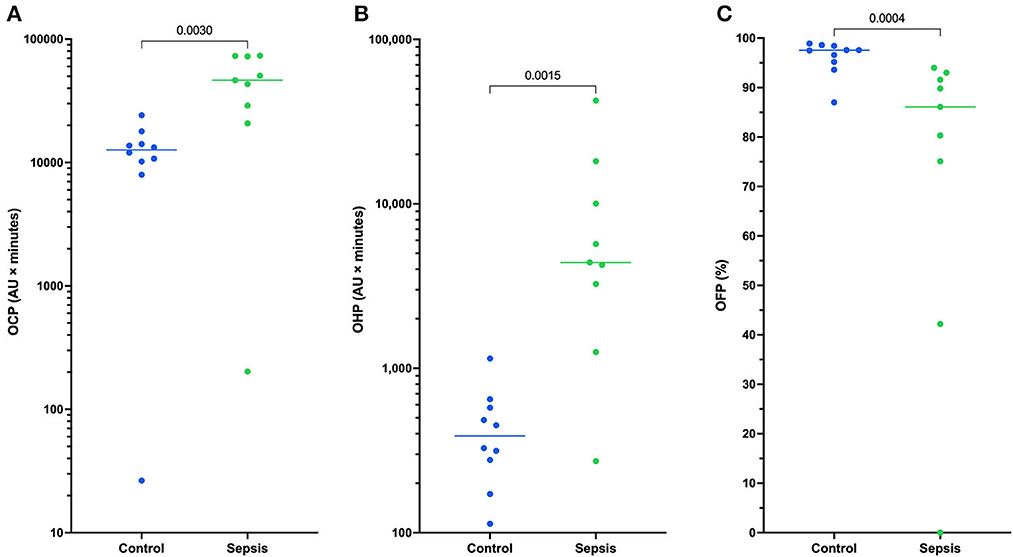
Figure 2. Dotplots of (A) the overall coagulation potential (OCP), (B) overall hemostatic potential (OHP), and (C) overall fibrinolytic potential (OFP) for 9 dogs with sepsis compared to controls (n = 10). Comparisons were performed using unpaired t-tests with Welch's correction or the Mann-Whitney U-test as appropriate based on data distribution. Dogs with sepsis have significantly greater OCP and OHP compared to healthy control dogs, and significantly lower OFP. This suggests dogs with sepsis have a greater propensity for clot formation and are hypofibrinolytic compared to control dogs. For 11 dogs with sepsis there was insufficient plasma sample volume available to perform the OHP/OFP assay.
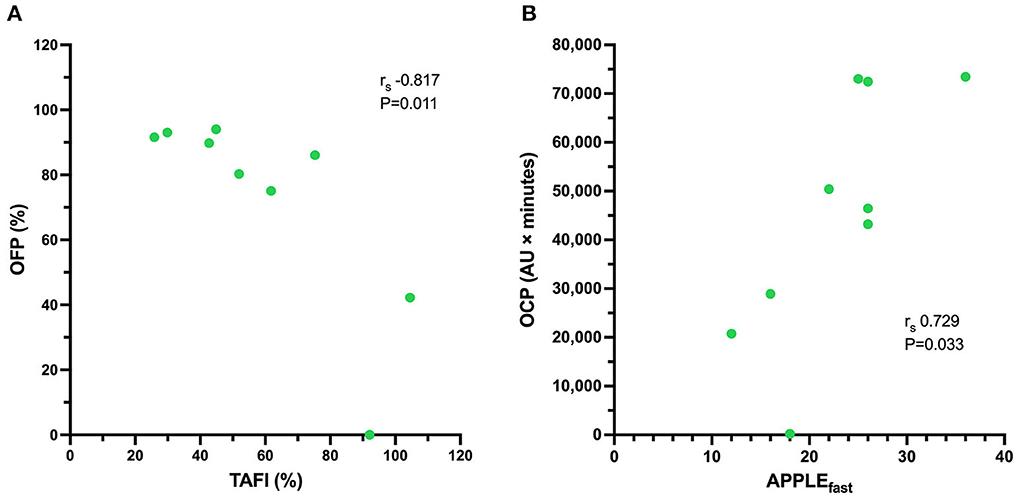
Figure 3. Scatterplot of (A) the activity of thrombin-activatable fibrinolysis inhibitor (TAFI, %, abscissa) against the overall fibrinolysis potential (OFP, %, ordinate) for nine dogs with sepsis. There is a significant and very strong negative correlation between the activity of TAFI and the OFP. Scatterplot of (B) the illness severity score (APPLEfast, abscissa) against the overall coagulation potential (OCP, AU × minutes, ordinate) for nine dogs with sepsis. There is a significant, strong positive correlation between illness severity and the OCP. For the remaining 11 dogs with sepsis there was insufficient plasma sample volume available to perform the OHP/OFP assay.
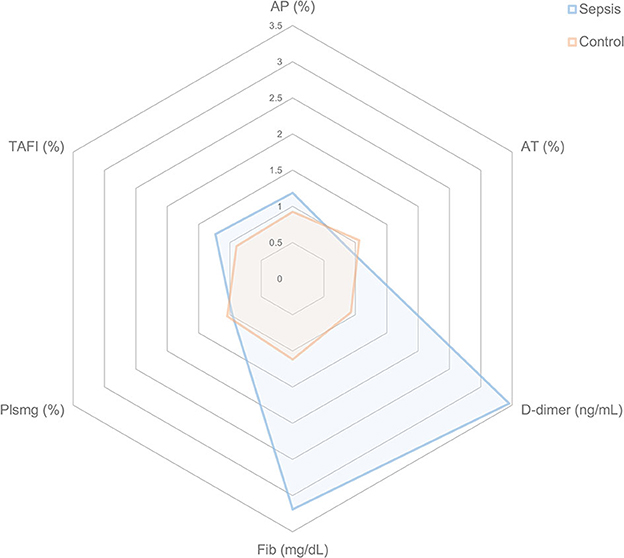
Figure 4. Radar plots representing the coagulation disturbances in dogs with sepsis compared to healthy control dogs. For each coagulation variable and for each individual dog, the fold change from the midpoint of the reference interval was calculated for dogs with sepsis and for healthy controls. The mean fold change for each coagulation variable was then plotted. A value of 1.0 indicates no mean deviation from the midpoint of the reference interval, while a value of 3.0 indicates there was a mean 3-fold increase above the midpoint of the reference interval for that variable. Consistent with their healthy, normal status, the mean values for control dogs are all close to 1.0. By contrast, dogs with sepsis have large mean deviations for D-dimer and Fib in particular. AP, antiplasmin; AT, antithrombin; Fib, fibrinogen; Plsmg, plasminogen; TAFI, thrombin-activatable fibrinolysis inhibitor.
Discussion
We aimed to characterize the fibrinolytic system in dogs with sepsis. Although there was some heterogeneity in the study population, dogs with sepsis were hypercoagulable and hypofibrinolytic relative to normal dogs. Depressed fibrinolysis combined with increased fibrinogen thus contributes to a prothrombotic risk in dogs with sepsis, as it does in humans (46, 47). Our results support recommendations that sepsis represents a risk factor for thrombosis in dogs that can warrant thromboprophylaxis (48, 49).
We observed numerous significant differences in the activities and concentrations of pro- and anti-fibrinolytic proteins in dogs with sepsis compared to healthy controls, most consistently increased fibrinogen and D-dimer concentrations. Concentrations of the fibrinolysis inhibitor TAFI were also significantly increased in dogs with sepsis and were very strongly correlated with the OFP. This association provides potential mechanistic insight, because high TAFI concentrations were correlated with low OFP values. High TAFI activity prevents tPA from colocalizing with plasminogen on fibrin thereby limiting plasmin generation and suppressing fibrinolysis. Increased TAFI concentrations are associated with increased risk of venous thrombosis and stroke in humans (50–52), although typically TAFI concentrations are either unchanged (53, 54), or decreased in humans with sepsis (55, 56). High TAFI concentrations were reported in dogs with babesiosis (33), and in dogs with sepsis (18), with concentrations comparable with those we observed. The cause of high TAFI concentrations in dogs with sepsis is uncertain. Increased hepatic synthesis of TAFI combined with decreased endothelial expression of thrombomodulin might result in a relative imbalance between production and consumption, thereby favoring higher plasma TAFI concentration (5, 57).
The increased AP activities observed in dogs with sepsis likely resulted from an acute phase response and further contributed to the hypofibrinolytic phenotype. In dogs, AP activity is also increased following minor and major surgery (58, 59), postoperative hemorrhage (60), and with protein-losing disease (61). AP is a serine-protease inhibitor (serpin) produced by the liver that binds plasmin, leading to its own cleavage and the subsequent formation of inactive antiplasmin-plasmin complexes, thereby limiting fibrinolysis (62). Additionally FXIIIa cross-links AP into growing thrombi (63), slowing plasmin-mediated clot lysis (64). AP may help prevent hyperfibrinolysis, and studies in various human diseases suggests that increased AP concentrations potentiate and perpetuate pathologic thrombi causing stroke, deep vein thrombosis and pulmonary embolism (65).
Prior studies have demonstrated that dogs with sepsis have increased fibrinogen concentrations consistent with the acute phase, evidence of consumption of the endogenous anticoagulants AT and protein C, and increased concentrations of D-dimers indicating fibrin degradation (3, 66, 67). Consistent with these reports, we identified significantly decreased AT and significantly increased fibrinogen and D-dimer concentrations. Dogs in our study had evidence of thrombin generation (decreased AT) and activation of the fibrinolytic system (increased D-dimer), but the increased fibrinogen concentrations do not suggest that a consumptive coagulopathy or sufficient criteria for diagnosis of overt DIC occurred in any dog (6). Rather, the typical phenotype of dogs in our study was consistent with inflammation, hypercoagulability and hypofibrinolysis. The OHP assay parameters OHP and OCP were increased, consistent with the increased fibrinogen concentration. Notably, the balance of fibrin formation and lysis described by the OFP parameter was low in septic dogs, confirming hypofibrinolysis. Assays of the individual components of the pathway suggest reduced plasmin generation and there may also be a structural explanation for the observed hypofibrinolysis. Thrombi formed when the initial rate of thrombin generation is high are dense, formed of tightly packed thin fibrin fibers that resist fibrinolysis, while those formed by lower rates of thrombin generation are looser with fibers that are coarse and more readily degraded by plasmin (68). This ultrastructural phenomenon may have in vivo consequences (69), and suggests that in the future, concurrent measurements of thrombin generation and plasmin generation potential could offer further insights into the pro-vs. anti-thrombotic balance present in patients in vivo (70).
There are limitations to the present study. The sample size in our study was small, restricted by costs and sample volumes required to perform the panel of coagulation and fibrinolysis assays. Low sample size may have increased the rates of type I and type II errors (e.g., for survival analyses) and precluded identification of discrete sub-populations. In addition, we did not evaluate every protein involved in the fibrinolytic system (47), in part due to lack of available or applicable assays. For instance, we measured plasminogen and AP, but did not determine the concentration of plasmin-antiplasmin (PAP) complexes or plasmin generation itself. Plasmin generation assays (70, 71) are in development but are presently not readily available for clinical studies in dogs (72). Similarly, PAP complexes have been assessed in humans with sepsis (26, 73), but a canine PAP assay is not presently available. Finally, assays to measure tPA and PAI-1 were unavailable (35), and might have provided valuable insights into the regulation of fibrinolysis in dogs with sepsis.
Most dogs in the study survived, likely due to low overall illness severity, moreover all three deaths were due to euthanasia, which could have biased survival analyses. As with all studies measuring blood biomarker concentrations, plasma levels of precursor coagulation proteins, their activated forms and their inhibitors are dynamic over time and are affected by fluid administration, blood product transfusion, disease-specific treatment, and administration of drugs such as aminocaproic acid and tranexamic acid that may directly influence the fibrinolytic system. Concurrent conditions including neoplasia, trauma, and liver disease and the effects of surgical interventions will inevitably affect the measured concentrations of pro- and antifibrinolytic proteins and in vivo hemostatic balance. Our study provides only a single time point observation precluding assessment of temporal changes or determination if the abnormalities identified represented the maximal disturbances associated with the disease. Experimental sepsis models enable sequential observations at defined times within the course of the syndrome, but do not replicate all features of naturally occurring disease.
In summary dogs with sepsis were hypercoagulable and hypofibrinolytic, characterized by increased plasma AP, D-dimer, fibrinogen and TAFI, and relative suppression of clot lysis in a global hemostasis assay, the OHP. These results support an increased potential for thrombotic complications in this patient population. Monitoring changes over time in the most readily available of these assays, such as fibrinogen and D-dimer, might provide guidance on individual patient risk and enable clinicians to individualize therapy with antithrombotic drugs. Future studies might include analyses of individual fibrinolytic pathway proteins, particularly tPA and PAI-1 and activated TAFI (53), and global assays, such as thrombin and plasmin generation, and viscoelastic measures of fibrinolysis to assess the overall balance of the fibrinolytic system (74, 75). Comparisons of the coagulation disturbances between underlying causes of sepsis might determine if all dogs with sepsis are comparably affected thereby improving our understanding of the causes of the coagulation dysfunction in dogs with sepsis. Identifying the underlying causes of hypofibrinolysis in dogs with sepsis through mechanistic studies (76, 77), might enable alternative therapeutic strategies to be employed in the future (78, 79).
Data availability statement
The original contributions presented in the study are included in the article/Supplementary material, further inquiries can be directed to the corresponding author.
Ethics statement
The animal study was reviewed and approved by the local Institution Animal Care and Use Committee (Protocol #2014-0053). Written informed consent was obtained from the owners for the participation of their animals in this study.
Author contributions
KS recruited and enrolled patients, collected and analyzed data, and co-wrote the manuscript. RG conceived the study, analyzed data, and co-wrote the manuscript. AS collected and analyzed data and edited the manuscript. MB collected and analyzed data and edited the manuscript. All authors contributed to the article and approved the submitted version.
Conflict of interest
The authors declare that the research was conducted in the absence of any commercial or financial relationships that could be construed as a potential conflict of interest.
Publisher's note
All claims expressed in this article are solely those of the authors and do not necessarily represent those of their affiliated organizations, or those of the publisher, the editors and the reviewers. Any product that may be evaluated in this article, or claim that may be made by its manufacturer, is not guaranteed or endorsed by the publisher.
Supplementary material
The Supplementary Material for this article can be found online at: https://www.frontiersin.org/articles/10.3389/fvets.2023.1104602/full#supplementary-material
References
1. Kenney EM, Rozanski EA, Rush JE, deLaforcade-Buress AM, Berg JR, Silverstein DC, et al. Association between outcome and organ system dysfunction in dogs with sepsis: 114 cases (2003–2007). J Am Vet Med Assoc. (2010) 236:83–7. doi: 10.2460/javma.236.1.83
2. Singer M, Deutschman CS, Seymour CW, Shankar-Hari M, Annane D, Bauer M, et al. The third international consensus definitions for sepsis and septic shock. JAMA. (2016) 315:801–10. doi: 10.1001/jama.2016.0287
3. de Laforcade AM, Freeman LM, Shaw SP, Brooks MB, Rozanski EA, Rush JE. Hemostatic changes in dogs with naturally occurring sepsis. J Vet Intern Med. (2003) 17:674–9. doi: 10.1111/j.1939-1676.2003.tb02499.x
4. Kuzi S, Segev G, Haruvi E, Aroch I. Plasma antithrombin activity as a diagnostic and prognostic indicator in dogs: a retrospective study of 149 dogs. J Vet Intern Med. (2010) 24:587–96. doi: 10.1111/j.1939-1676.2010.0497.x
5. Kim SD, Baker P, DeLay J, Wood RD. Thrombomodulin expression in tissues from dogs with systemic inflammatory disease. Vet Pathol. (2016) 53:797–802. doi: 10.1177/0300985815626571
6. Goggs R, Mastrocco A, Brooks MB. Retrospective evaluation of 4 methods for outcome prediction in overt disseminated intravascular coagulation in dogs. (2009-2014): 804 cases. J Vet Emerg Crit Care. (2018) 28:541–50. doi: 10.1111/vec.12777
7. Vignolo-Scalone WH, Vignolo-Puglia WH, Kitchens CS. Microvascular alterations in thrombin-induced experimental disseminated intravascular coagulation in the dog. Angiology. (1984) 35:261–8.
8. LaRue MJ, Murtaugh RJ. Pulmonary thromboembolism in dogs: 47 cases (1986–1987). J Am Vet Med Assoc. (1990) 197:1368–72.
9. Van Winkle TJ, Liu SM, Hackner SG. Clinical and pathological features of aortic thromboembolism in 36 dogs. J Vet Emerg Crit Care. (1993) 3:13–21. doi: 10.1111/j.1476-4431.1993.tb00099.x
10. Palmer KG, King LG, Van Winkle TJ. Clinical manifestations and associated disease syndromes in dogs with cranial vena cava thrombosis: 17 cases (1989–1996). J Am Vet Med Assoc. (1998) 213:220–4.
11. Respess M, O'Toole TE, Taeymans O, Rogers CL, Johnston A, Webster CR. Portal vein thrombosis in 33 dogs: 1998–2011. J Vet Intern Med. (2012) 26:230–7. doi: 10.1111/j.1939-1676.2012.00893.x
12. DeClue AE, Sharp CR, Harmon M. Plasma inflammatory mediator concentrations at ICU admission in dogs with naturally developing sepsis. J Vet Intern Med. (2012) 26:624–30. doi: 10.1111/j.1939-1676.2012.00895.x
13. Goggs R, Letendre JA. Evaluation of the host cytokine response in dogs with sepsis and noninfectious systemic inflammatory response syndrome. J Vet Emerg Crit Care. (2019) 29:593–603. doi: 10.1111/vec.12903
14. Johnson V, Burgess B, Morley P, Bragg R, Avery A, Dow S. Comparison of cytokine responses between dogs with sepsis and dogs with immune-mediated hemolytic anemia. Vet Immunol Immunopathol. (2016) 180:15–20. doi: 10.1016/j.vetimm.2016.08.010
15. Karlsson I, Hagman R, Johannisson A, Wang L, Sodersten F, Wernersson S. Multiplex cytokine analyses in dogs with pyometra suggest involvement of KC-like chemokine in canine bacterial sepsis. Vet Immunol Immunopathol. (2016) 170:41–6. doi: 10.1016/j.vetimm.2016.01.005
16. O'Brien M. The reciprocal relationship between inflammation and coagulation. Top Companion Anim Med. (2012) 27:46–52. doi: 10.1053/j.tcam.2012.06.003
17. Ogasawara S, Stokol T. Interleukin-10 inhibits lipopolysaccharide-induced upregulation of tissue factor in canine peripheral blood monocytes. Vet Immunol Immunopathol. (2012) 148:331–6. doi: 10.1016/j.vetimm.2012.04.023
18. Jessen LR, Wiinberg B, Kjelgaard-Hansen M, Jensen AL, Rozanski E, Kristensen AT. Thrombin-activatable fibrinolysis inhibitor activity in healthy and diseased dogs. Vet Clin Pathol. (2010) 39:296–301. doi: 10.1111/j.1939-165X.2010.00230.x
19. Hesselvik JF, Blombäck M, Brodin B, Maller R. Coagulation, fibrinolysis, and kallikrein systems in sepsis: relation to outcome. Crit Care Med. (1989) 17:724–33. doi: 10.1097/00003246-198908000-00002
20. Madoiwa S, Nunomiya S, Ono T, Shintani Y, Ohmori T, Mimuro J, et al. Plasminogen activator inhibitor 1 promotes a poor prognosis in sepsis-induced disseminated intravascular coagulation. Int J Hematol. (2006) 84:398–405. doi: 10.1532/IJH97.05190
21. Madoiwa S. Recent advances in disseminated intravascular coagulation: endothelial cells and fibrinolysis in sepsis-induced DIC. J Intensive Care. (2015) 3:8. doi: 10.1186/s40560-015-0075-6
22. Koyama K, Madoiwa S, Tanaka S, Koinuma T, Wada M, Sakata A, et al. Evaluation of hemostatic biomarker abnormalities that precede platelet count decline in critically ill patients with sepsis. J Crit Care. (2013) 28:556–63. doi: 10.1016/j.jcrc.2012.10.069
23. Feldman BF, Madewell BR, O'Neill S. Disseminated intravascular coagulation: antithrombin, plasminogen, and coagulation abnormalities in 41 dogs. J Am Vet Med Assoc. (1981) 179:151–4.
24. Schmitt FCF, Manolov V, Morgenstern J, Fleming T, Heitmeier S, Uhle F, et al. Acute fibrinolysis shutdown occurs early in septic shock and is associated with increased morbidity and mortality: results of an observational pilot study. Ann Intensive Care. (2019) 9:19. doi: 10.1186/s13613-019-0499-6
25. Hoshino K, Kitamura T, Nakamura Y, Irie Y, Matsumoto N, Kawano Y, et al. Usefulness of plasminogen activator inhibitor-1 as a predictive marker of mortality in sepsis. J Intensive Care. (2017) 5:42. doi: 10.1186/s40560-017-0238-8
26. Stief TW, Ijagha O, Weiste B, Herzum I, Renz H, Max M. Analysis of hemostasis alterations in sepsis. Blood Coagul Fibrinolysis. (2007) 18:179–86. doi: 10.1097/MBC.0b013e328040bf9a
27. Kario K, Matsuo T, Kodama K, Matsuo M, Yamamoto K, Kobayashi H. Imbalance between thrombin and plasmin activity in disseminated intravascular coagulation. Assessment by the thrombin-antithrombin-III complex/plasmin-alpha-2-antiplasmin complex ratio. Haemostasis. (1992) 22:179–86.
28. López-Aguirre Y, Páramo JA. Endothelial cell and hemostatic activation in relation to cytokines in patients with sepsis. Thromb Res. (1999) 94:95–101. doi: 10.1016/s0049-3848(98)00200-x
29. Semeraro F, Colucci M, Caironi P, Masson S, Ammollo CT, Teli R, et al. Platelet drop and fibrinolytic shutdown in patients with sepsis. Crit Care Med. (2018) 46:e221–e8. doi: 10.1097/ccm.0000000000002919
30. Park KJ, Kim HJ, Hwang SC, Lee SM, Lee YH, Hahn MH, et al. The imbalance between coagulation and fibrinolysis is related to the severity of the illness and the prognosis in sepsis. Korean J Intern Med. (1999) 14:72–7. doi: 10.3904/kjim.1999.14.2.72
31. Wada H, Nobori T, Watanabe R, Shiku H, Sakuragawa N. Plasma levels of plasminogen activator inhibitor-1. (PAI-1) and thrombin activatable fibrinolysis inhibitor (TAFI) in patients with disseminated intravascular coagulation (DIC). Turk J Haematol. (2002) 19:235–7.
32. Hayakawa M, Sawamura A, Gando S, Jesmin S, Naito S, Ieko M, et al. low TAFI activity and insufficient activation of fibrinolysis by both plasmin and neutrophil elastase promote organ dysfunction in disseminated intravascular coagulation associated with sepsis. Thromb Res. (2012) 130:906–13. doi: 10.1016/j.thromres.2012.01.015
33. Kules J, Gotic J, Mrljak V, Baric Rafaj R. Blood markers of fibrinolysis and endothelial activation in canine babesiosis. BMC Vet Res. (2017) 13:82. doi: 10.1186/s12917-017-0995-6
34. Vilar Saavedra P, Lara Garcia A, Zaldivar Lopez S, Couto G. Hemostatic abnormalities in dogs with carcinoma: a thromboelastographic characterization of hypercoagulability. Vet J. (2011) 190:e78–83. doi: 10.1016/j.tvjl.2011.02.025
35. Wong CJ, Koch M, Behling-Kelly EL. Development of a plasminogen activator inhibitor. (PAI-1) assay and comparison of plasma PAI-1 activity in hyperlipidemic/dyslipidemic dogs with either hyperadrenocorticism or diabetes mellitus, and healthy dogs. Res Vet Sci. (2017) 111:1–8. doi: 10.1016/j.rvsc.2016.11.004
36. Longstaff C, Kolev K. Basic mechanisms and regulation of fibrinolysis. J Thromb Haemost. (2015) 13 Suppl 1:S98–105. doi: 10.1111/jth.12935
37. Ekdahl KN, Teramura Y, Hamad OA, Asif S, Duehrkop C, Fromell K, et al. Dangerous liaisons: complement, coagulation, and kallikrein/kinin cross-talk act as a linchpin in the events leading to thromboinflammation. Immunol Rev. (2016) 274:245–69. doi: 10.1111/imr.12471
38. Antovic A. The overall hemostasis potential: a laboratory tool for the investigation of global hemostasis. Semin Thromb Hemost. (2010) 36:772–9. doi: 10.1055/s-0030-1265294
39. Dengate AL, Morel-Kopp MC, Beatty JA, Barrs V, Braddock JA, Churcher RK, et al. Evaluation and modification of the overall hemostasis potential assay for use with canine plasma. Am J Vet Res. (2013) 74:1493–8. doi: 10.2460/ajvr.74.12.1493
40. Hauptman JG, Walshaw R, Olivier NB. Evaluation of the sensitivity and specificity of diagnostic criteria for sepsis in dogs. Vet Surg. (1997) 26:393–7.
41. Hayes G, Mathews K, Doig G, Kruth S, Boston S, Nykamp S, et al. The acute patient physiologic and laboratory evaluation. (APPLE) score: a severity of illness stratification system for hospitalized dogs. J Vet Intern Med. (2010) 24:1034–47. doi: 10.1111/j.1939-1676.2010.0552.x
42. Pashmakova MB, Bishop MA, Steiner JM, Suchodolski JS, Barr JW. Evaluation of serum thyroid hormones in dogs with systemic inflammatory response syndrome or sepsis. J Vet Emerg Crit Care. (2014) 24:264–71. doi: 10.1111/vec.12172
43. Whitton CM, Sands D, Hubbard AR, Gaffney PJ. A collaborative study to establish the 2nd International Standard for Fibrinogen, Plasma. Thromb Haemost. (2000) 84:258–62.
44. Lanevschi A, Kramer JW, Greene SA, Meyers KM. Evaluation of chromogenic substrate assays for fibrinolytic analytes in dogs. Am J Vet Res. (1996) 57:1124–30.
45. Dengate AL, Morel-Kopp MC, Beatty JA, Barrs V, Braddock JA, Churcher RK, et al. Differentiation between dogs with thrombosis and normal dogs using the overall hemostasis potential assay. J Vet Emerg Crit Care. (2016) 26:446–52. doi: 10.1111/vec.12442
46. Tuan TA, Ha NTT, Xoay TD, My TTK. Fibrinolytic impairment and mortality in pediatric septic shock: a single-center prospective observational study. Pediatr Crit Care Med. (2021) 22:969–77. doi: 10.1097/pcc.0000000000002759
47. Larsen JB, Hvas AM. Fibrinolytic alterations in sepsis: biomarkers and future treatment targets. Semin Thromb Hemost. (2021) 47:589–600. doi: 10.1055/s-0041-1725096
48. deLaforcade A, Bacek L, Blais MC, Goggs R, Lynch A, Rozanski E. Consensus on the rational use of antithrombotics in veterinary critical care. (CURATIVE): domain 1-defining populations at risk. J Vet Emerg Crit Care. (2019) 29:37–48. doi: 10.1111/vec.12797
49. Goggs R, Blais MC, Brainard BM, Chan DL, deLaforcade AM, Rozanski E, et al. American college of veterinary emergency and critical care (ACVECC) consensus on the rational use of antithrombotics in veterinary critical care (CURATIVE) guidelines: small animal. J Vet Emerg Crit Care. (2019) 29:12–36. doi: 10.1111/vec.12801
50. van Tilburg NH, Rosendaal FR, Bertina RM. Thrombin activatable fibrinolysis inhibitor and the risk for deep vein thrombosis. Blood. (2000) 95:2855–9.
51. Eichinger S, Weltermann A, Minar E, Stain M, Schönauer V, Schneider B, et al. Symptomatic pulmonary embolism and the risk of recurrent venous thromboembolism. Arch Intern Med. (2004) 164:92–6. doi: 10.1001/archinte.164.1.92
52. Leebeek FW, Goor MP, Guimaraes AH, Brouwers GJ, Maat MP, Dippel DW, et al. High functional levels of thrombin-activatable fibrinolysis inhibitor are associated with an increased risk of first ischemic stroke. J Thromb Haemost. (2005) 3:2211–8. doi: 10.1111/j.1538-7836.2005.01484.x
53. Park R, Song J, An SS. Elevated levels of activated and inactivated thrombin-activatable fibrinolysis inhibitor in patients with sepsis. Korean J Hematol. (2010) 45:264–8. doi: 10.5045/kjh.2010.45.4.264
54. Chen CC, Lee KD, Gau JP Yu YB, You JY, Lee SC, et al. Plasma antigen levels of thrombin-activatable fibrinolysis inhibitor did not differ in patients with or without disseminated intravascular coagulation. Ann Hematol. (2005) 84:675–80. doi: 10.1007/s00277-005-1079-4
55. Zeerleder S, Schroeder V, Hack CE, Kohler HP, Wuillemin WA, TAFI. and PAI-1 levels in human sepsis. Thromb Res. (2006) 118:205–12. doi: 10.1016/j.thromres.2005.06.007
56. Emonts M, de Bruijne EL, Guimarães AH, Declerck PJ, Leebeek FW, de Maat MP, et al. Thrombin-activatable fibrinolysis inhibitor is associated with severity and outcome of severe meningococcal infection in children. J Thromb Haemost. (2008) 6:268–76. doi: 10.1111/j.1538-7836.2007.02841.x
57. Faust SN, Levin M, Harrison OB, Goldin RD, Lockhart MS, Kondaveeti S, et al. Dysfunction of endothelial protein C activation in severe meningococcal sepsis. N Engl J Med. (2001) 345:408–16. doi: 10.1056/nejm200108093450603
58. Lanevschi A, Kramer JW, Greene SA, Meyers KM. Fibrinolytic activity in dogs after surgically induced trauma. Am J Vet Res. (1996) 57:1137–40.
59. Moldal ER, Kristensen AT, Peeters ME, Nødtvedt A, Kirpensteijn J. Hemostatic response to surgical neutering via ovariectomy and ovariohysterectomy in dogs. Am J Vet Res. (2012) 73:1469–76. doi: 10.2460/ajvr.73.9.1469
60. Lara-García A, Couto CG, Iazbik MC, Brooks MB. Postoperative bleeding in retired racing greyhounds. J Vet Intern Med. (2008) 22:525–33. doi: 10.1111/j.1939-1676.2008.0088.x
61. Donahue SM, Brooks M, Otto CM. Examination of hemostatic parameters to detect hypercoagulability in dogs with severe protein-losing nephropathy. J Vet Emerg Crit Care. (2011) 21:346–55. doi: 10.1111/j.1476-4431.2011.00656.x
62. Singh S, Saleem S, Reed GL. Alpha2-antiplasmin: the devil you don't know in cerebrovascular and cardiovascular disease. Front Cardiovasc Med. (2020) 7:608899. doi: 10.3389/fcvm.2020.608899
63. Fraser SR, Booth NA, Mutch NJ. The antifibrinolytic function of factor XIII is exclusively expressed through α2-antiplasmin cross-linking. Blood. (2011) 117:6371–4. doi: 10.1182/blood-2011-02-333203
64. Reed GL, Matsueda GR, Haber E. Platelet factor XIII increases the fibrinolytic resistance of platelet-rich clots by accelerating the crosslinking of alpha 2-antiplasmin to fibrin. Thromb Haemost. (1992) 68:315–20.
65. Singh S, Houng AK, Reed GL. Venous stasis-induced fibrinolysis prevents thrombosis in mice: role of α2-antiplasmin. Blood. (2019) 134:970–8. doi: 10.1182/blood.2019000049
66. de Laforcade AM, Rozanski EA, Freeman LM Li W. Serial evaluation of protein C and antithrombin in dogs with sepsis. J Vet Intern Med. (2008) 22:26–30. doi: 10.1111/j.1939-1676.2007.0021.x
67. Bentley AM, Mayhew PD, Culp WT, Otto CM. Alterations in the hemostatic profiles of dogs with naturally occurring septic peritonitis. J Vet Emerg Crit Care. (2013) 23:14–22. doi: 10.1111/vec.12013
68. Wolberg AS. Thrombin generation and fibrin clot structure. Blood Rev. (2007) 21:131–42. doi: 10.1016/j.blre.2006.11.001
69. Zabczyk M, Undas A. Plasma fibrin clot structure and thromboembolism: clinical implications. Pol Arch Intern Med. (2017) 127:873–81. doi: 10.20452/pamw.4165
70. Matsumoto T, Nogami K, Shima M. Simultaneous measurement of thrombin and plasmin generation to assess the interplay between coagulation and fibrinolysis. Thromb Haemost. (2013) 110:761–8. doi: 10.1160/th13-04-0345
71. Miszta A, Ahmadzia HK, Luban NLC Li S, Guo D, Holle LA, et al. Application of a plasmin generation assay to define pharmacodynamic effects of tranexamic acid in women undergoing cesarean delivery. J Thromb Haemost. (2021) 19:221–32. doi: 10.1111/jth.15114
72. Miszta A, Huskens D, Donkervoort D, Roberts MJM, Wolberg AS, de Laat B. Assessing plasmin generation in health and disease. Int J Mol Sci. (2021) 22:2758. doi: 10.3390/ijms22052758
73. Semeraro F, Ammollo CT, Caironi P, Masson S, Latini R, Panigada M, et al. D-dimer corrected for thrombin and plasmin generation is a strong predictor of mortality in patients with sepsis. Blood Transfus. (2020) 18:304–11. doi: 10.2450/2019.0175-19
74. Spodsberg EH, Wiinberg B, Jessen LR, Marschner CB, Kristensen AT. Endogenous fibrinolytic potential in tissue-plasminogen activator-modified thromboelastography analysis is significantly decreased in dogs suffering from diseases predisposing to thrombosis. Vet Clin Pathol. (2013) 42:281–90. doi: 10.1111/vcp.12068
75. Panigada M, Zacchetti L., L'Acqua C, Cressoni M, Anzoletti MB, Bader R, et al. Assessment of fibrinolysis in sepsis patients with urokinase modified thromboelastography. PLoS ONE. (2015) 10:e0136463. doi: 10.1371/journal.pone.0136463
76. Gould TJ, Vu TT, Stafford AR, Dwivedi DJ, Kim PY, Fox-Robichaud AE, et al. Cell-free DNA modulates clot structure and impairs fibrinolysis in sepsis. Arterioscler Thromb Vasc Biol. (2015) 35:2544–53. doi: 10.1161/atvbaha.115.306035
77. Cruz DBD, Helms J, Aquino LR, Stiel L, Cougourdan L, Broussard C, et al. DNA-bound elastase of neutrophil extracellular traps degrades plasminogen, reduces plasmin formation, and decreases fibrinolysis: proof of concept in septic shock plasma. FASEB J. (2019) 33:14270–80. doi: 10.1096/fj.201901363RRR
78. Denorme F, Rustad JL, Portier I, Crandell JL, de Araujo CV, Cody MJ, et al. Neutrophil extracellular trap inhibition improves survival in neonatal mouse infectious peritonitis. Pediatr Res. (2022) 3:1–8. doi: 10.1038/s41390-022-02219-0
Keywords: sepsis, antithrombin, antiplasmin, TAFI, fibrinogen, D-dimer, dogs, fibrinolysis
Citation: Sotos KE, Goggs R, Stablein AP and Brooks MB (2023) Increased thrombin activatable fibrinolysis inhibitor activity is associated with hypofibrinolysis in dogs with sepsis. Front. Vet. Sci. 10:1104602. doi: 10.3389/fvets.2023.1104602
Received: 21 November 2022; Accepted: 30 January 2023;
Published: 16 February 2023.
Edited by:
Heiko Rühl, University Hospital Bonn, GermanyReviewed by:
Stefano Cortellini, Royal Veterinary College (RVC), United KingdomIvanio Teixeira Borba Junior, State University of Campinas, Brazil
Copyright © 2023 Sotos, Goggs, Stablein and Brooks. This is an open-access article distributed under the terms of the Creative Commons Attribution License (CC BY). The use, distribution or reproduction in other forums is permitted, provided the original author(s) and the copyright owner(s) are credited and that the original publication in this journal is cited, in accordance with accepted academic practice. No use, distribution or reproduction is permitted which does not comply with these terms.
*Correspondence: Robert Goggs, r.goggs@cornell.edu