Neglected zoonotic helminthiases in wild canids: new insights from South America
- 1Biomedical Research Center Seltersberg (BFS), Institute of Parasitology, Justus Liebig University Giessen, Gießen, Germany
- 2CIBAV Research Group, Veterinary Medicine School, Universidad de Antioquia, Medellín, Colombia
- 3Institute of Parasitology, Biology Centre of the Czech Academy of Sciences, České Budějovice, Czechia
The global threat of neglected tropical diseases (NTDs) constitutes a public health issue in underdeveloped countries. Zoonotic helminthiases are the most common human NTD agents in developing countries in sub-Saharan Africa, Asia, and the Americas, causing a global burden of disease that exceeds that of more recognized infectious diseases such as malaria and tuberculosis. Wild canids are well-known mammals that act as natural reservoirs of zoonotic-relevant helminthiasis worldwide, thus playing a pivotal role in their epidemiology and transmission to humans. Here we evaluate the occurrence of zoonotic gastrointestinal helminths in two Neotropical wild canid species from the Amazonian and Andean regions of Colombia, i.e., the bush dog (Speothos venaticus) and the crab-eating fox (Cerdocyon thous). We recovered tapeworm proglottids from bush dog fecal samples and identified them molecularly as the canine-specific lineage of Dipylidium caninum by using cytochrome c oxidase subunit I (cox1) gene sequences. Moreover, examination of a crab-eating fox during necropsy revealed the presence of non-embryonated eggs of the neglected nematode Lagochilascaris cf. minor, in addition to eggs and gravid proglottids of the cestode Spirometra mansoni. These findings represent the first report of zoonotic-relevant cestodes, i.e., D. caninum (“canine genotype”), S. mansoni, and the nematode L. cf. minor, in bush dogs and crab-eating foxes as final hosts. The occurrence of these zoonotic helminthiases in wild canid species calls for regular monitoring programs to better understand the epidemiology and transmission routes of neglected dipylidiasis, lagochilascariosis, and sparganosis in South America.
1. Introduction
Zoonoses account for approximately 60% of emerging human infectious diseases, and among these, up to 70% are wildlife-derived pathogens (1, 2). In addition, the global threat of neglected tropical diseases (NTDs) constitutes a public health issue in underdeveloped countries in sub-Saharan Africa, Asia, and the Americas. Among NTDs, zoonotic helminthiases are the most common human pathogens, causing a global burden of disease exceeding that of better-known infectious diseases such as malaria and tuberculosis. On a global scale, helminth infections account for over 75% of disability-adjusted life years lost. However, many of them have fallen into oblivion as neglected diseases (3, 4). Wild canids are well-known natural reservoirs of zoonotic parasites (5–10), which include numerous helminth species, and thus play a pivotal role in the life cycle, epidemiology, and transmission routes of human infections (11–14). The forested tropical regions with high mammalian species richness are facing the emergence of zoonotic disease hotspots under ongoing land use changes, giving rise to an increased disease transmission risk at the human-animal interface (15, 16). Lower-latitude developing countries (e.g., Neotropical territories) have a concentration of emerging zoonotic pathogens, while scientific studies and surveillance efforts that focus on this issue remain scarce (17). Knowledge of zoonotic cestodes is limited to the genera Dibothriocephalus (diphyllobothriosis), Hymenolepis, and Taenia, leaving uncommon neglected cestode infections such as bertielliosis, dipylidiasis, echinococcosis, inermicapsiferosis, raillietinosis, mesocestoidiosis, and sparganosis, which are rarely reported clinically and underestimated even by specialists (4, 18, 19). Wild canids comprise a large group of carnivores that are distributed throughout the world, often living in close proximity to human populations (20–22). The Neotropics are home to a total of 10 wild canid species with varied behaviors, habitats, and forms (Table 1). The current study presents the findings on the gastrointestinal helminth parasite in two highly divergent free-ranging Neotropical wild canid (NWC) species: the elusive semiaquatic diurnal/crepuscular bush dog and the nocturnal ground-dwelling crab-eating fox. Furthermore, we examine the potential role that NWC may play as definitive hosts (DH) in the transmission and maintenance of neglected zoonotic helminthiases, providing new insights into this unresolved issue.
2. Materials and methods
2.1. Study areas and sample collection
Based on the Köppen–Geiger classification system, the Amazonian and Andean sampling areas were found in tropical rainforests and temperate, warm summers without dry climates, respectively (23). Animal sampling was focused on the northern regions of South America (Figure 1) and within the distribution range of bush dogs and crab-eating foxes in the Neotropical area. The current study included samples collected between 2019 and 2021 as part of a national wildlife conservation and monitoring program carried out by veterinarians/mammologists in Colombia. Additionally, animals found dead and collected by indigenous peoples and local communities were included in the study. Due to the evasive behavior of bush dogs, parasite specimens were collected from direct sampling sites on trails that were systematically monitored by trap cameras. The general features and morphometric characteristics of wild carnivore deposits were followed for fecal identification (24). Moreover, associated tracks and local traditional ecological knowledge were also used to sample these elusive individuals, as previously described (25, 26). Therefore, fecal samples were collected as fresh as possible. No bush dog carcasses were examined during the study period. However, scattered proglottids and cestode strobila segments were found partially dehydrated during the macroscopical examination of feces from two monitored bush dogs (n = 2) in the Amazonian municipality of Puerto Santander, Colombia. Parasite collection from crab-eating foxes was carried out during the necropsy of a dead animal (n = 1) in the Andean municipality of Ciudad Bolívar., Colombia, and in an environment where grass-fed cattle are raised. The head, thoracic, and abdominal cavities were extensively examined for the presence of ecto- and endoparasites. The entire gastrointestinal tract, heart, spleen, kidneys, and respiratory tract were removed and thoroughly inspected in situ for the presence of macroscopic parasites using a 40X-25 mm glass magnifier. An adult cestode specimen was carefully recovered from fresh feces collected from the gut lumen after a longitudinal intestinal incision. Fecal samples were then collected directly from the gastrointestinal tract and dry-preserved until examination.
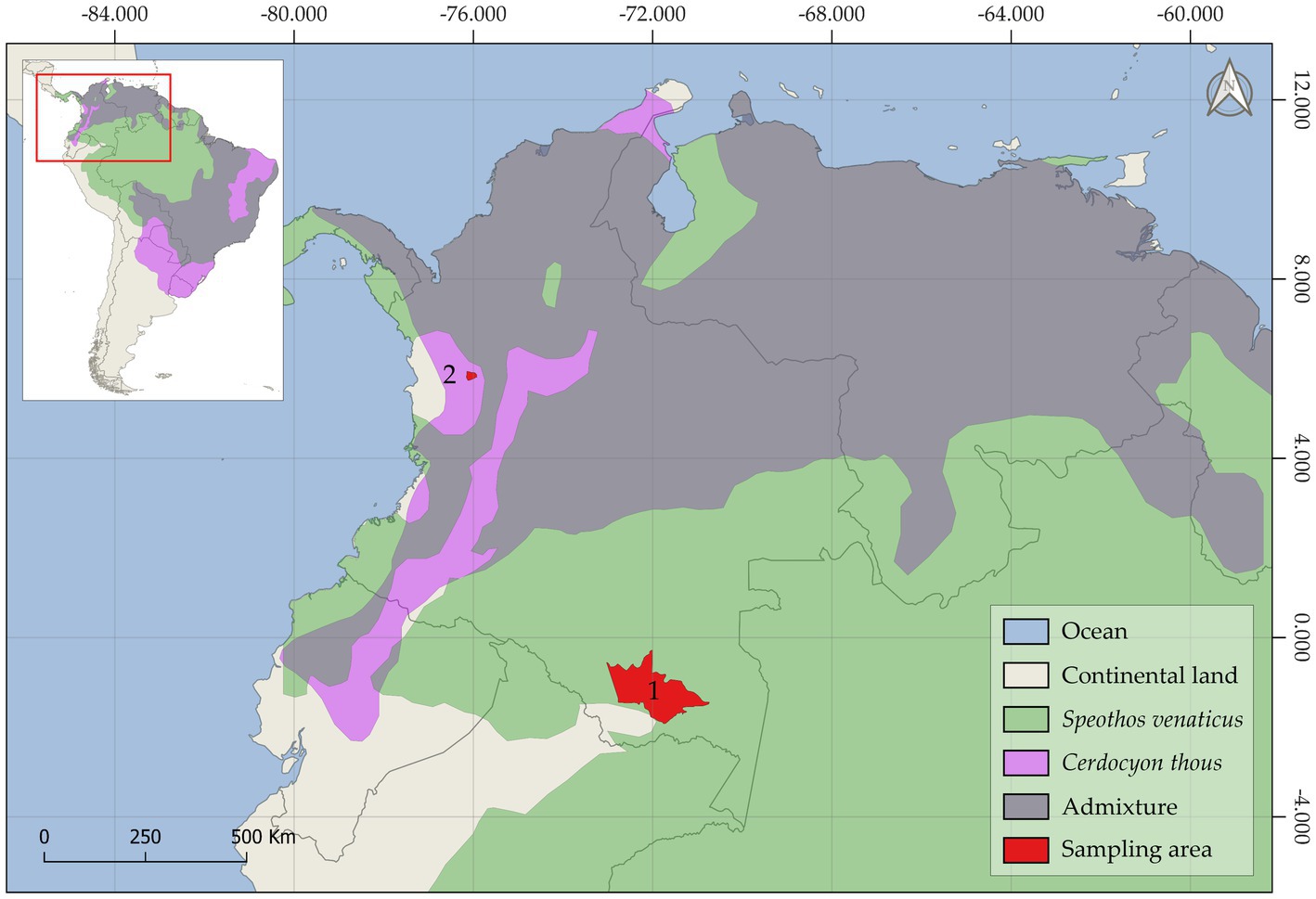
Figure 1. The geographic map depicts the historical distribution range of the bush dog (Speothos venaticus), the crab-eating fox (Cerdocyon thous), and the admixture zone where both species are found. The Amazonian (1) and Andean (2) sampling areas of this study are shown in red.
After macroscopic observation of cestode strobila and free proglottids in the feces, all collected parasite specimens were handled using fine entomological tweezers, gently rinsed, washed three times with 0.9% pre-warmed phosphate-buffered saline (PBS), and subsequently preserved in ~96% EtOH until microscopic and molecular evaluation. Combined sedimentation-flotation and modified sodium acetate–acetic acid-formalin standardized parasitological techniques were used to analyze wild canid fecal samples (27). In addition, gravid proglottids obtained from the crab-eating fox tapeworm strobila were dissected and wet mounted on slides. This non-invasive method for fecal collection allowed for the recovery of adult cestode specimens without unnecessary manipulation, trapping, or disturbance of these free-ranging canids (25, 28).
2.2. Phenotypic evaluation of adult cestode specimens
General morphologic and morphometric taxonomic traits were observed, and parasite stage identification was conducted under microscopic analysis using an Olympus BX53™ semi-motorized light microscope (Olympus Corporation, Tokyo, Japan) at 400 and 1,000X magnification. The Olympus DP74™ digital camera was used to capture photomicrographs of eggs, adult strobila, and proglottids. Parasites were measured using the cellSens™ standard imaging software. Additionally, cestode proglottids were dehydrated in ethanol series (75, 80, 85, 90, 96, and 100%), transferred to a fixative solution (i.e., formalin, 95% EtOH, glacial acetic acid, glycerine, and Milli-Q ultrapure distilled water; 10:25:5:10:50 parts, respectively), clarified with lactophenol, and stained with Semichon’s acetocarmine. Finally, the proglottids were wet-mounted and Berlese’s fluid-mounted on slides as described previously (29).
2.3. Molecular phylogenetics
The complete coding sequence of the cytochrome c oxidase subunit I (cox1) gene was amplified in two overlapping fragments with the primers cox1F and JB4.5, and JB3 and cox1R, respectively (30, 31) using Phusion High-Fidelity DNA Polymerase (New England Biolabs, Inc., Ipswich, USA) and the following cycling conditions: 35 cycles of 10 s at 98°C, 15 s at 50°C (cox1F + JB4.5) or 60°C (JB3 + cox1R), and 50 s at 72°C. PCR products were gel-checked, purified with Exonuclease I and FastAP alkaline phosphatase (Thermo Fisher Scientific, Waltham, USA), and directly Sanger-sequenced at SeqMe (Dobříš, Czech Republic). Contiguous gene sequences were assembled, visually checked, and trimmed to the cox1 coding region in Geneious Prime 2020.0.51 and deposited in GenBank under accession numbers OR251823 and OR251823. The resulting sequences were aligned with previously published cox1 data from Dipylidium specimens in addition to other closely related species using MAFFT’s (32) L-INS-i translational align plugin of Geneious. The use of Nippotaenia chaenogobii (JQ2685509) and Nippotaenia mogurndae (ON640728) as outgroup taxa and the selection of relevant ingroup representatives were based on previous phylogenetic estimates, most notably by Waeschenbach et al. (33) and Guo et al. (34). The phylogenetic tree was estimated under the maximum likelihood criterion in IQ-TREE (35). The best-fitting model of nucleotide evolution was selected according to the corrected Akaike information criterion in IQ-TREE (36), and nodal supports were estimated by running 1,000 standard nonparametric bootstrap replicates and 10,000 repetitions of the SH-like approximated likelihood ratio test.
3. Results
3.1. Morphological and morphometric parasite identification
Morphological identification of the whitish, flat, barrel-shaped segments recovered from bush dog feces (Figure 2) was based on observation of the typical longer-than-wide shape, with each proglottid having two bilateral genital pores, one at the center of each lateral margin. The mean gravid proglottid measurements (n = 10) were 12.082 mm (SD ± 0.542 mm) in length and 3.996 mm (SD ± 0.344 mm) in width. The phenotypic evaluation corresponds well with Dipylidium caninum s.l. (Dipylidiidae). Thin-shelled capsules (ovigerous capsules) containing eggs were also noticed inside gravid proglottids (Figure 2D). Regarding the parasitological evaluation of the crab-eating fox, non-embryonated ascarid-type eggs (51.21 × 51.57 μm) with a thick eggshell and a coarsely pitted surface containing multiple excavations were observed (Figure 3A). The morphological traits of the egg correspond well to Lagochilascaris minor, previously described in South American wild carnivore definitive hosts (37, 38). Additionally, parasite stages (i.e., adults and eggs) of the diphyllobothriidean species Spirometra mansoni were detected. A weakly muscled, medium-sized, pink-colored cestode (89.73 cm in length) with a long, prominent neck was also recorded. External segmentation of the strobila was noted throughout the specimen. The cestode showed a well-developed spoon-shaped scolex without inrolling bothrial edges. The mature and gravid proglottids were serrated, and the eggs presented a clearly visible unique operculum and an oval shape with a pointed end (Figures 3B–E and Supplementary Video S1). The average proglottid measurements (n = 483) were 454.14 μm (SD ± 207.16 μm) in length and 1.78 mm (SD ± 0.73 mm) in width. The strobila segments of this parasite specimen were previously used for the molecular identification of S. mansoni reported by Brabec et al. (39).
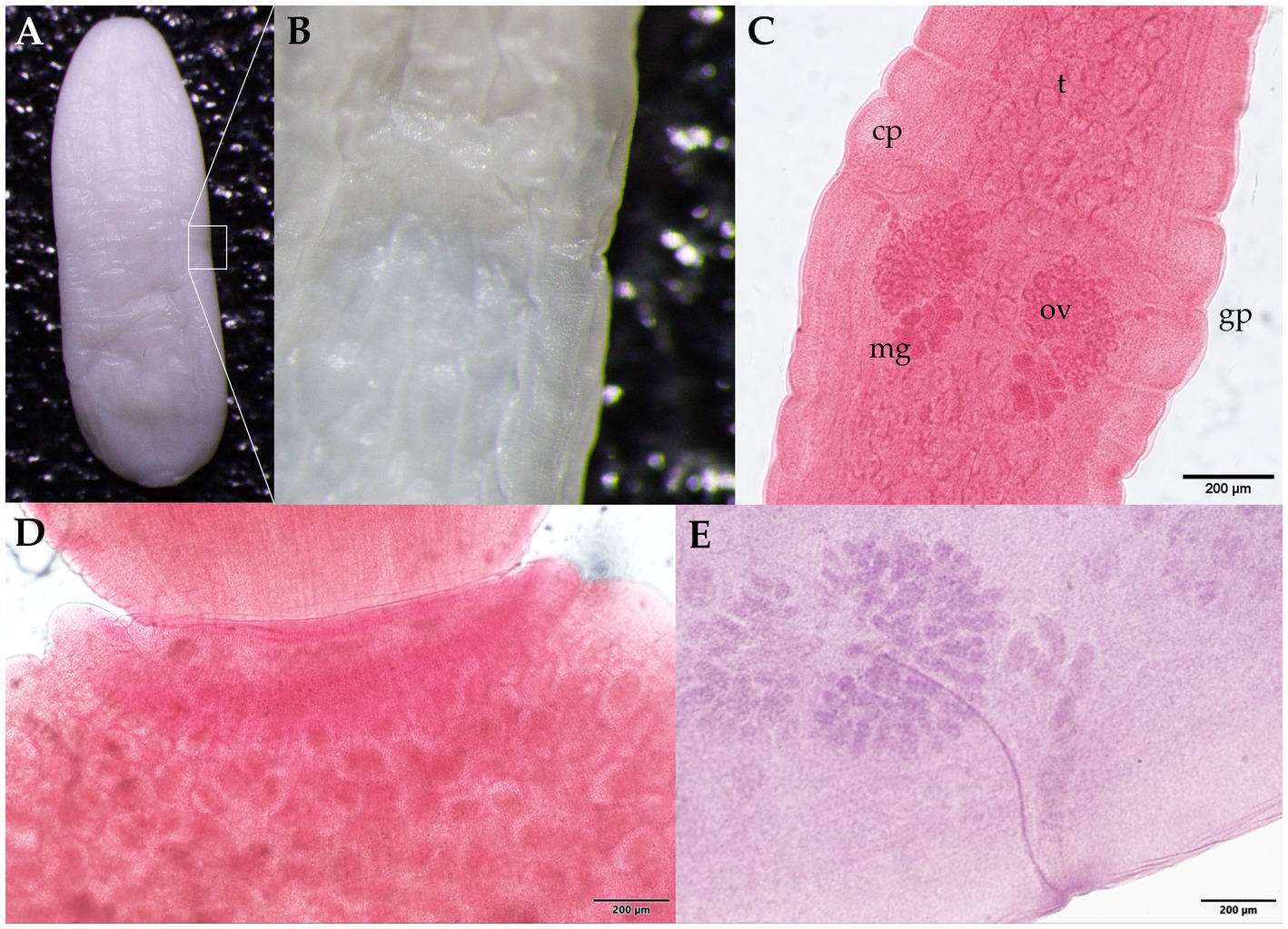
Figure 2. Proglottids of Dipylidium caninum s.l. (family Dipylidiidae) collected from the feces of the Amazonian bush dog (Speothos venaticus). (A) Wet mount unstained gravid proglottid. The white square indicates (B) a lateral magnified view of the genital pore. (C) Mature proglottids stained with Semichon’s acetocarmine; two sets of symmetrically distributed genital organs are visible, with the testis parenchyma (t), cirrus pouch (cp), genital pore (gp), ovaries (ov), and the Mehlis glands (mg). (D) Close-up of the seed-shaped ovigerous proglottid end with round to oval egg capsules (packets) with an average length of 31–50 μm and a width of 27–48 μm (n = 88). (E) Photomicrograph of the parasite showing details of one of two sets of male and female reproductive organs. Scale bars: (C–E) 200 μm.
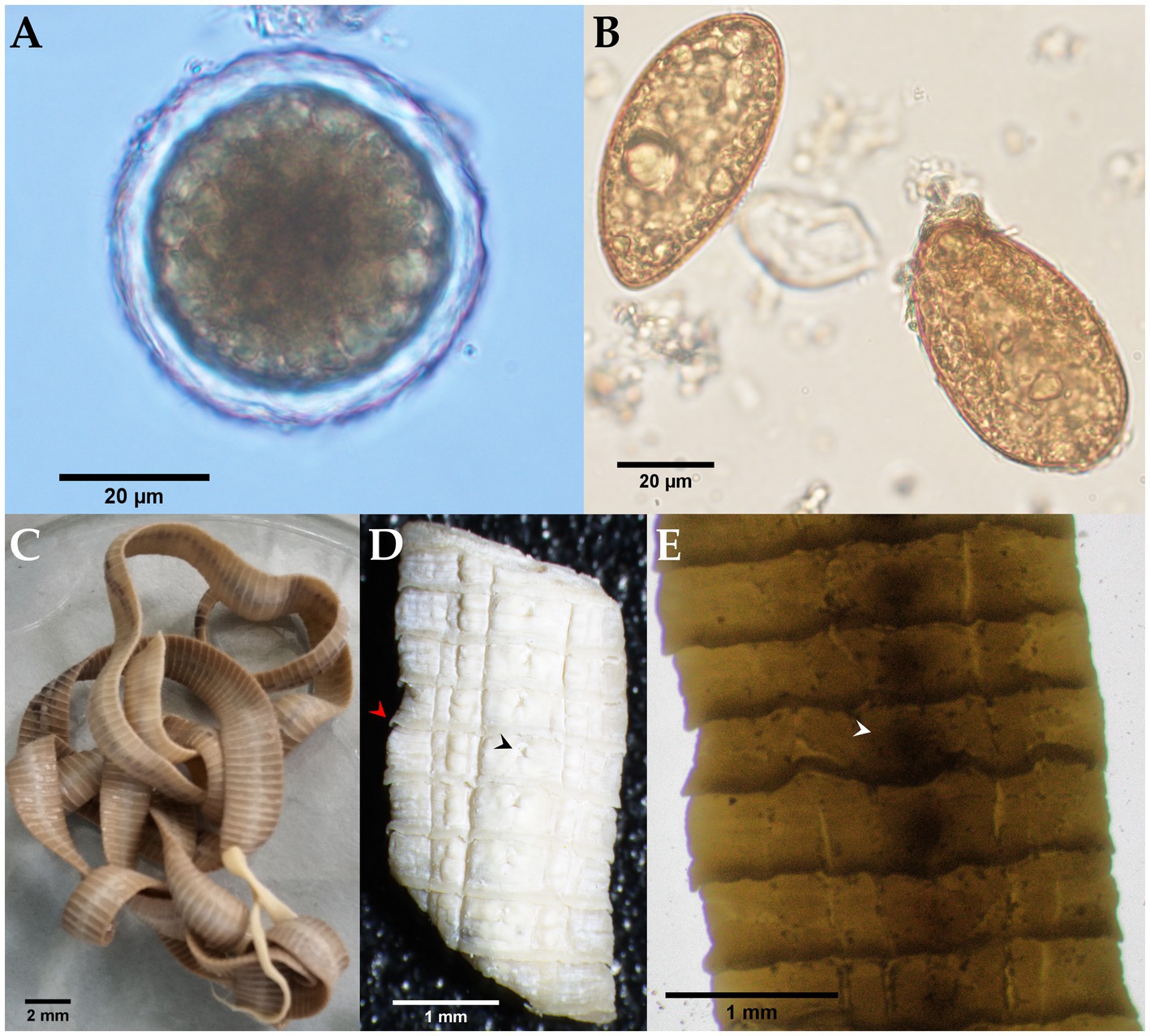
Figure 3. Microscopic and stereomicroscopic morphological examination of parasite stages found in the feces of a free-ranging crab-eating fox (Cerdocyon thous). (A) Non-embryonated egg of Lagochilascaris cf. minor (51.21 × 51.57 μm) with an evident 5.79 μm thick eggshell. (B) Yellowish-brown, cone-shaped operculated eggs of Spirometra mansoni (61.67 × 34.97 μm). (C) Adult S. mansoni (from the Andes) with a spoon-shaped scolex and characteristic pink color due to the presence of host vitamin B12. (D) Close-up photograph of serrated gravid proglottids (red arrowhead); a genital pore is indicated by the black arrowhead. (E) Whole-mounted strobilus segment showing the centrally located spiralled uterus (white arrowhead).
3.2. Molecular characterization of Dipylidium caninum
Strobila segments isolated from two separately collected Andean bush dog fecal samples were molecularly characterized by cox1 sequencing. Maximum likelihood phylogenetic analysis confirmed the species identification as D. caninum, placing both specimens at the base of a well-defined group composed exclusively of D. caninum representatives (Figure 4). The lineage of the D. caninum group consists of two genetically differentiated subgroups corresponding to the previously described canine- and feline-specific genotypes of D. caninum (40). Within these, five canine-specific genotype representatives formed a relatively basal, non-monophyletic, statistically unsupported assemblage of specimens, while the feline-specific genotype represented by two specimens (MG587892 and OK523385) formed a relatively derived, well-supported internal lineage. The Andean bush dog isolates are grouped basally within the canine-specific genotype representatives (Figure 4).
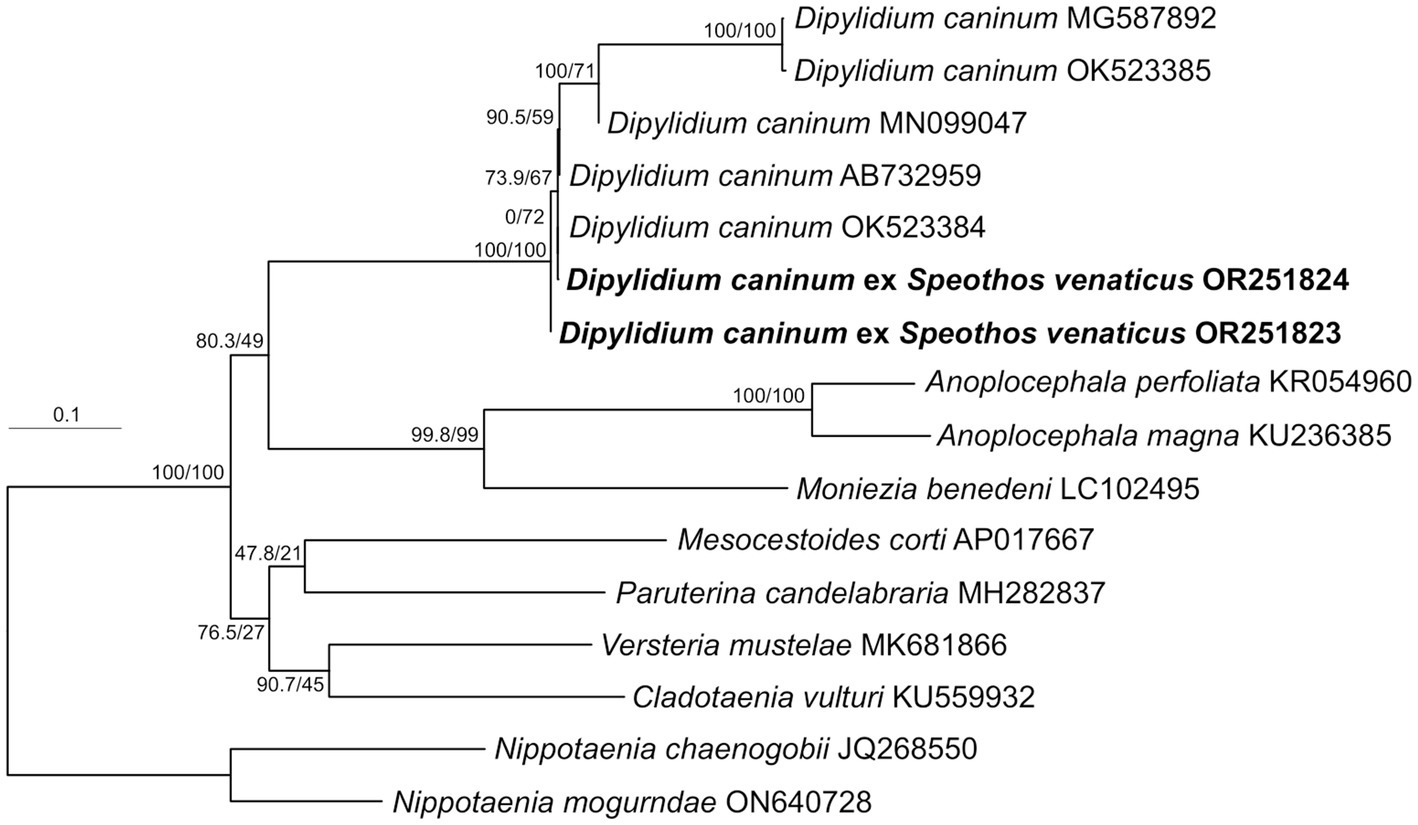
Figure 4. Phylogenetic position of Dipylidium caninum isolates obtained from two Amazonian bush dog hosts. Maximum likelihood tree from IQ-TREE based on nearly complete (1,563 bp) cox1 gene sequences analyzed as a single partition using the TIM + F + R5 model. Nodal values show SH-like approximated likelihood ratio test values (10,000 replicates) and standard nonparametric bootstrap supports (1,000 repetitions). Newly characterized specimens are shown in bold. GenBank accessions are shown after taxon names. The branch length scale bar indicates the number of substitutions per site.
4. Discussion
In an increasingly globalized world, anthropogenic factors have intensified the human-wildlife interface, thus increasing the risk of disease spillover, reduction of biodiversity, and food web collapse (41–43). Among carnivores, only 54.3% of the global species’ distribution range comprises high-quality habitats due to landscape fragmentation and loss of connectivity (44). Nowadays, surveillance of wildlife-derived infectious diseases is imperative to better understand the impact of disease on populations, eco-epidemiology, and biodiversity conservation (45–47). As already stated, synanthropic wild canids have also been reported as natural reservoirs of novel helminth parasites (48–50).
Parasitological surveys of bush dogs are still limited due to their elusive nature and crepuscular behavior, and thus they remain one of the lesser-known wild canid species. Nonetheless, the causative agent of chronic polycystic human echinococcosis (i.e., Echinococcus vogeli) was described for the first time in 1972 in a wild bush dog captured in South America (51, 52). Furthermore, other zoonotic-relevant parasites such as Toxocara canis, Lagochilascaris sp., and Spirometra sp. have been reported in wild bush dogs along with the occurrences of Spirocerca lupi, Ancylostoma caninum, Taenia sp., and the apicomplexan Cystoisospora caninum (53, 54). Additionally, parasites such as Dioctophyme renale, Dirofilaria immitis, Neospora caninum, Rangelia vitalii, the cyst-forming coccidia Hammondia heydorni, and the zoonotic parasites Angiostrongylus cantonensis, Dipylidium caninum, Leishmania infantum (syn. L. chagasi), Toxoplasma gondii, and various important ticks have been reported for the crab-eating fox (55–63). Thus, both NWC species may contribute to the environmental maintenance and transmission of human and domestic animal parasitoses. Given the lack of information regarding the occurrence and distribution of helminthiases of public health concern, such as dipylidiasis, lagochilascariosis, and sparganosis, the results presented here collectively provide new insights into the potential of NWC in the emergence and transmission of zoonotic infectious diseases. Here, we successfully perform the identification of neglected zoonotic helminths harbored by bush dogs and crab-eating foxes.
The worldwide occurrence of D. caninum s.l. in domestic dogs and cats is well documented, as is human dipylidiasis, particularly in young children (64–68). Humans become infected by accidental ingestion of D. caninum-cysticercoid-carrying intermediate hosts (IH), most commonly fleas or chewing lice (69, 70). Based on molecular techniques that allow for the differentiation of cryptic species and hidden genetic lineages, two genetically distinct lineages, i.e., the so-called D. caninum canine and D. caninum feline genotypes, have been proposed within the genus (40, 71). In contrast to human dipylidiasis, D. caninum infections rarely produce clinical manifestations in canids or felids. Nonetheless, animals that frequently carry D. caninum-infected IH contribute to human parasite transmission (72, 73). Wild carnivores such as dingoes (Canis dingo), golden jackals (Canis aureus), jaguars (Panthera onca), red foxes (Vulpes vulpes), and spotted hyenas (Crocuta crocuta) are wild natural reservoir hosts and thus essential for the maintenance of the parasite life cycle (72, 74–77). Dipylidium caninum infections in crab-eating fox populations have been suggested as a possible consequence of anthropogenic expansion into the natural habitats of wild hosts (60). A study in the rural high-mountain region of Colombia reported the occurrence of D. caninum with an estimated prevalence of 20% (SD ± 8.7%) in free-roaming and peri-domestic dog populations (78). In Colombia, the parasite has only previously been reported in humans and domestic hosts (79). Therefore, to the best of our knowledge, the results presented here expand the geographic distribution range of wildlife dipylidiasis to the Pan-Amazonian and northern Andean regions, providing the first host record for bush dogs. Additionally, we establish here that the analyzed cestode proglottids from bush dogs correspond to the D. caninum canine genotype, which occurs at a higher frequency in canids, has a shorter pre-patency, and has a longer life span than the D. caninum feline genotype (40).
The detection of the ascarid nematode genus Lagochilascaris raises public health concerns since human lagochilascariosis, mainly due to L. minor, is still an extremely neglected zoonotic disease of the Neotropics. The definitive hosts are carnivores (i.e., canids and felids) carrying intestinal adults that shed highly resistant ascarid-like eggs with a thick and rough eggshell (37, 80). Humans acquire lagochilascariosis through the ingestion of infected rodent IH (e.g., agoutis, mice, rats) containing third-stage larvae (L3), but there is also evidence that humans might become infected after ingestion of embryonated eggs of Lagochilascaris (81). So far, more than 100 human cases of lagochilascariosis have been recorded in the Americas (80). Three cases of human lagochilascariosis have been documented in the Caribbean/Pacific, and Amazonian regions of Colombia (82, 83). Regardless, the present study constitutes the first non-human report of this parasite in Colombia. Because the amount of DNA obtained from the isolated Lagochilascaris cf. minor eggs was extremely low and showed partial degradation, subsequent phylogenetic analysis was not feasible. Nevertheless, surveillance for human lagochilascariosis by local public health authorities should be recommended.
Globally distributed sparganosis is a neglected food- and waterborne zoonotic disease caused by infection with cestodes of the genus Spirometra (Diphyllobothriidea), which is frequently reported in numerous wildlife species (14, 18, 39, 84). Sparganosis manifests as muscular and subcutaneous larvae (spargana), but brain invasion has also been reported (85). The obligate heteroxenous parasite life cycle involves carnivores, where intestinal adults shed eggs that are subsequently released into the environment with feces. In the aqueous environment, the eggs hatch into coracidia, which are ingested by copepods as the first IH in which a procercoid larva develops. These larvae are infective to the second tetrapod IH (e.g., frogs, snakes, and birds), where maturation into plerocercoid larvae takes place (86). Humans become infected by eating raw IH flesh, using it in traditional poultices, or drinking water containing infected copepods (87). In South America, a total of 16 human cases of sparganosis have been reported, one of them in Colombia (87, 88). The cestode specimen of the crab-eating fox morphologically described here was molecularly identified as Spirometra mansoni, the first report of the species in South America (39). As sparganosis remains one of the least studied diseases, the morphological data presented here support the findings of Brabec et al. in 2022 (39), which effectively enlarged the distribution range of S. mansoni for the Neotropics, and call for further investigation of human sparganosis.
Here, we have provided evidence for important zoonotic helminth infections in highly divergent free-ranging NWC species. Consequently, investigation of the potential role of the elusive semiaquatic bush dog, the synanthropic/peri-domestic crab-eating fox, and other poorly studied NWCs in the transmission cycle of these parasites to humans seems essential. Additionally, future ectoparasite research on different lice and flea taxa (e.g., Felicola subrostratus, Trichodectes canis, Archaeopsyllinae, and Pulicinae subfamilies) infesting wild carnivore populations is urgently needed to identify the IH harboring D. caninum cysticercoids and thus enabling zoonotic transmission of dipylidiasis. The consequences of coinfections on epidemiology and host fitness require better knowledge of NWC-associated infectious agents to understand their role in the emergence of dipylidiasis, lagochilascariosis, and sparganosis (89). Based on these findings, we encourage further parasitologic investigations to be conducted among NWCs, specifically regarding their endo- and ectoparasites. In conclusion, an ongoing parasitological survey of wildlife is critical for implementing public health strategies to avoid zoonotic spillover in a pathogen-related surveillance network.
Data availability statement
The datasets presented in this study can be found in online repositories. The names of the repository/repositories and accession number(s) can be found at: https://www.ncbi.nlm.nih.gov/nuccore/OR251824, OR251824; and https://www.ncbi.nlm.nih.gov/nuccore/OR251823, OR251823.
Ethics statement
The animal study was approved by the Ethics Committee for Animal Experimentation (CEEA) of the Universidad de Antioquia, Colombia (AS No. 132) under collection permit No. 0524 of 2014 (IDB0321), procedures were conducted according to the Guidelines of the American Society of Mammologists for the use of wild mammals in research and education, and the EU Directive 2010/63/EU. The study was conducted in accordance with the local legislation and institutional requirements.
Author contributions
MU: conceptualization, investigation, writing-original draft preparation. MU and JB: methodology, software, and visualization. JB, JC-G, and CH: validation. JB and MU: formal analysis. CH and JC-G: resources and funding acquisition. JB, CH, and JC-G: data curation and writing – review and editing. JC-G and CH: supervision. All authors have read and agreed to the published version of the manuscript.
Funding
The project in which the samples were collected was funded by the CIBAV Research Group-Centro de Investigaciones Básicas y Aplicadas en Veterinaria (COL0153246), Universidad de Antioquia, Consolidation Strategy 2018–2019. The molecular characterization of the specimens was funded by the Czech Science Foundation, Project No. 19-28399X. The APC was funded by Justus Liebig University in Giessen. We would like to extend our thanks to the Bicentennial Doctoral Excellence Scholarship Program of Colombia (“Programa de becas de excelencia doctoral del bicentenario”) for the financial support to the Ph.D. student MU.
Acknowledgments
We would like to thank the Amazonian native local communities of Araracuara, Amazonas, Colombia, the rural communities, and the cattle ranchers of Ciudad Bolívar.
Conflict of interest
The authors declare that the research was conducted in the absence of any commercial or financial relationships that could be construed as a potential conflict of interest.
Publisher’s note
All claims expressed in this article are solely those of the authors and do not necessarily represent those of their affiliated organizations, or those of the publisher, the editors and the reviewers. Any product that may be evaluated in this article, or claim that may be made by its manufacturer, is not guaranteed or endorsed by the publisher.
Supplementary material
The Supplementary material for this article can be found online at: https://www.frontiersin.org/articles/10.3389/fvets.2023.1235182/full#supplementary-material
Footnotes
References
1. Rahman, MT, Sobur, MA, Islam, MS, Ievy, S, Hossain, MJ, El Zowalaty, ME, et al. Zoonotic diseases: etiology, impact, and control. Microorganisms. (2020) 8:1405. doi: 10.3390/microorganisms8091405
2. Salyer, SJ, Silver, R, Simone, K, and Barton, BC. Prioritizing zoonoses for global health capacity building—themes from one health zoonotic disease workshops in 7 countries, 2014–2016. Emerg Infect Dis. (2017) 23:S55–64. doi: 10.3201/eid2313.170418
3. Xiao, N, Yao, J-W, Ding, W, Giraudoux, P, Craig, PS, and Ito, A. Priorities for research and control of cestode zoonoses in Asia. Infect Dis Poverty. (2013) 2:16. doi: 10.1186/2049-9957-2-16
4. Sapp, SGH, and Bradbury, RS. The forgotten exotic tapeworms: a review of uncommon zoonotic Cyclophyllidea. Parasitology. (2020) 147:533–58. doi: 10.1017/S003118202000013X
5. Irie, T, Uraguchi, K, Ito, T, Yamazaki, A, Takai, S, and Yagi, K. First report of Sarcocystis pilosa sporocysts in feces from red fox, Vulpes vulpes schrencki, in Hokkaido, Japan. Int J Parasitol Parasites Wildl. (2020) 11:29–31. doi: 10.1016/j.ijppaw.2019.12.001
6. Elmore, SA, Lalonde, LF, Samelius, G, Alisauskas, RT, Gajadhar, AA, and Jenkins, EJ. Endoparasites in the feces of arctic foxes in a terrestrial ecosystem in Canada. Int J Parasitol Parasites Wildl. (2013) 2:90–6. doi: 10.1016/j.ijppaw.2013.02.005
7. Otranto, D, and Deplazes, P. Zoonotic nematodes of wild carnivores. Int J Parasitol Parasites Wildl. (2019) 9:370–83. doi: 10.1016/j.ijppaw.2018.12.011
8. Myšková, E, Brož, M, Fuglei, E, Kvičerová, J, Mácová, A, Sak, B, et al. Gastrointestinal parasites of arctic foxes (Vulpes lagopus) and sibling voles (Microtus levis) in Spitsbergen. Svalbard Parasitol Res. (2019) 118:3409–18. doi: 10.1007/s00436-019-06502-8
9. Duscher, GG, Leschnik, M, Fuehrer, H-P, and Joachim, A. Wildlife reservoirs for vector-borne canine, feline and zoonotic infections in Austria. Int J Parasitol Parasites Wildl. (2015) 4:88–96. doi: 10.1016/j.ijppaw.2014.12.001
10. Karamon, J, Samorek-Pieróg, M, Sroka, J, Bilska-Zając, E, Dąbrowska, J, Kochanowski, M, et al. The first record of Echinococcus ortleppi (G5) tapeworms in grey wolf (Canis lupus). Pathogens. (2021) 10:853. doi: 10.3390/pathogens10070853
11. Macchioni, F, Coppola, F, Furzi, F, Gabrielli, S, Baldanti, S, Boni, CB, et al. Taeniid cestodes in a wolf pack living in a highly anthropic hilly agro-ecosystem. Parasite. (2021) 28:10. doi: 10.1051/parasite/2021008
12. Oudni-M’rad, M, Chaâbane-Banaoues, R, M’rad, S, Trifa, F, Mezhoud, H, and Babba, H. Gastrointestinal parasites of canids, a latent risk to human health in Tunisia. Parasit Vectors. (2017) 10:280. doi: 10.1186/s13071-017-2208-3
13. Guerra, D, Armua-Fernandez, MT, Silva, M, Bravo, I, Santos, N, Deplazes, P, et al. Taeniid species of the Iberian wolf (Canis lupus signatus) in Portugal with special focus on Echinococcus spp. Int J Parasitol Parasites Wildl. (2013) 2:50–3. doi: 10.1016/j.ijppaw.2012.11.007
14. Bagrade, G, Králová-Hromadová, I, Bazsalovicsová, E, Radačovská, A, and Kołodziej-Sobocińska, M. The first records of Spirometra erinaceieuropaei (Cestoda: Diphyllobothriidae), a causative agent of human sparganosis, in Latvian wildlife. Parasitol Res. (2021) 120:365–71. doi: 10.1007/s00436-020-06957-0
15. Allen, T, Murray, KA, Zambrana-Torrelio, C, Morse, SS, Rondinini, C, Di Marco, M, et al. Global hotspots and correlates of emerging zoonotic diseases. Nat Commun. (2017) 8:1124. doi: 10.1038/s41467-017-00923-8
16. Namusisi, S, Mahero, M, Travis, D, Pelican, K, Robertson, C, and Mugisha, L. A descriptive study of zoonotic disease risk at the human-wildlife interface in a biodiversity hot spot in South Western Uganda. PLoS Negl Trop Dis. (2021) 15:e0008633. doi: 10.1371/journal.pntd.0008633
17. Jones, KE, Patel, NG, Levy, MA, Storeygard, A, Balk, D, Gittleman, JL, et al. Global trends in emerging infectious diseases. Nature. (2008) 451:990–3. doi: 10.1038/nature06536
18. Kuchta, R, Kołodziej-Sobocińska, M, Brabec, J, Młocicki, D, Sałamatin, R, and Scholz, T. Sparganosis (Spirometra) in Europe in the molecular era. Clin Infect Dis. (2021) 72:882–90. doi: 10.1093/cid/ciaa1036
19. Dhaliwal, BBS, and Juyal, PD. Cestode zoonoses In:. Parasitic Zoonoses. New Delhi: Springer India (2013). 65–82. doi: 10.1007/978-81-322-1551-6
20. Schipper, J, Chanson, JS, Chiozza, F, Cox, NA, Hoffmann, M, Katariya, V, et al. The status of the world’s land and marine mammals: diversity, threat, and knowledge. Science. (2008) 322:225–30. doi: 10.1126/science.1165115
21. Brandão, EMV, Xavier, SCC, Rocha, FL, Lima, CFM, Candeias, ÍZ, Lemos, FG, et al. Wild and domestic canids and their interactions in the transmission cycles of Trypanosoma Cruzi and Leishmania spp. in an area of the Brazilian Cerrado. Pathogens. (2020) 9:818. doi: 10.3390/pathogens9100818
22. Macpherson, CNL. Human behaviour and the epidemiology of parasitic zoonoses. Int J Parasitol. (2005) 35:1319–31. doi: 10.1016/j.ijpara.2005.06.004
23. Beck, HE, Zimmermann, NE, McVicar, TR, Vergopolan, N, Berg, A, and Wood, EF. Present and future Köppen-Geiger climate classification maps at 1-km resolution. Sci Data. (2018) 5:180214. doi: 10.1038/sdata.2018.214
24. Chame, M. Terrestrial mammal feces: a morphometric summary and description. Mem Inst Oswaldo Cruz. (2003) 98:71–94. doi: 10.1590/S0074-02762003000900014
25. Uribe, M, Payán, E, Brabec, J, Vélez, J, Taubert, A, Chaparro-Gutiérrez, JJ, et al. Intestinal parasites of neotropical wild jaguars, pumas, ocelots, and jaguarundis in Colombia: old friends brought back from oblivion and new insights. Pathogens. (2021) 10:822. doi: 10.3390/pathogens10070822
26. Zuercher, GL, Gipson, PS, and Stewart, GC. Identification of carnivore feces by local peoples and molecular analyses. Wildl Soc Bull. (2003) 31:961–70.
27. Yang, J, and Scholten, T. A fixative for intestinal parasites permitting the use of concentration and permanent staining procedures. Am J Clin Pathol. (1977) 67:300–4. doi: 10.1093/ajcp/67.3.300
28. Uribe, M, Hermosilla, C, Rodríguez-Durán, A, Vélez, J, López-Osorio, S, Chaparro-Gutiérrez, JJ, et al. Parasites circulating in wild synanthropic capybaras (Hydrochoerus hydrochaeris): a one health approach. Pathogens. (2021) 10:1152. doi: 10.3390/pathogens10091152
29. Swan, DC. Berlese’s fluid: remarks upon its preparation and use as a mounting medium. Bull Entomol Res. (1936) 27:389–91. doi: 10.1017/S0007485300058259
30. Bowles, J, Blair, D, and McManus, DP. Genetic variants within the genus Echinococcus identified by mitochondrial DNA sequencing. Mol Biochem Parasitol. (1992) 54:165–73. doi: 10.1016/0166-6851(92)90109-W
31. Wicht, B, Yanagida, T, Scholz, T, Ito, A, Jiménez, JA, and Brabec, J. Multiplex PCR for differential identification of broad tapeworms (Cestoda: Diphyllobothrium) infecting humans. J Clin Microbiol. (2010) 48:3111–6. doi: 10.1128/JCM.00445-10
32. Katoh, K, and Standley, DM. MAFFT multiple sequence alignment software version 7: improvements in performance and usability. Mol Biol Evol. (2013) 30:772–80. doi: 10.1093/molbev/mst010
33. Waeschenbach, A, Webster, BL, and Littlewood, DTJ. Adding resolution to ordinal level relationships of tapeworms (Platyhelminthes: Cestoda) with large fragments of mtDNA. Mol Phylogenet Evol. (2012) 63:834–47. doi: 10.1016/j.ympev.2012.02.020
34. Guo, X, Liu, J, Hao, G, Zhang, L, Mao, K, Wang, X, et al. Plastome phylogeny and early diversification of Brassicaceae. BMC Genomics. (2017) 18:176. doi: 10.1186/s12864-017-3555-3
35. Nguyen, L-T, Schmidt, HA, von Haeseler, A, and Minh, BQ. IQ-TREE: a fast and effective stochastic algorithm for estimating maximum-likelihood phylogenies. Mol Biol Evol. (2015) 32:268–74. doi: 10.1093/molbev/msu300
36. Kalyaanamoorthy, S, Minh, BQ, Wong, TKF, von Haeseler, A, and Jermiin, LS. ModelFinder: fast model selection for accurate phylogenetic estimates. Nat Methods. (2017) 14:587–9. doi: 10.1038/nmeth.4285
37. Rodriguez-Vivas, RI, Salazar-Grosskelwing, E, Ojeda-Chi, MM, Flota-Burgos, GJ, Solano-Barquero, A, Trinidad-Martínez, I, et al. First morphological and molecular report of Lagochilascaris minor (Nematoda, Ascarididae) in a domestic cat from Veracruz, Mexico. Vet Parasitol (Amst). (2023) 37:100823. doi: 10.1016/j.vprsr.2022.100823
38. Trindade, MAC, MRP, DM, Drehmer, CJ, and Muller, G. First record of Lagochilascaris minor (Nematoda: Ascarididae) in Leopardus geoffroyi (Carnivora: Felidae) in Brazil. Rev Bras Parasitol Veterinária. (2019) 28:812–5. doi: 10.1590/s1984-29612019087
39. Brabec, J, Uribe, M, Chaparro-Gutiérrez, JJ, and Hermosilla, C. Presence of Spirometra mansoni, causative agent of sparganosis, in South America. Emerg Infect Dis. (2022) 28:2347–50. doi: 10.3201/eid2811.220529
40. Beugnet, F, Labuschagne, M, Vos C,, Crafford, D, and Fourie, J. Analysis of Dipylidium caninum tapeworms from dogs and cats, or their respective fleas. Parasite. (2018) 25:31. doi: 10.1051/parasite/2018029
41. Fricke, EC, Hsieh, C, Middleton, O, Gorczynski, D, Cappello, CD, Sanisidro, O, et al. Collapse of terrestrial mammal food webs since the late Pleistocene. Science. (2022) 377:1008–11. doi: 10.1126/science.abn4012
42. Magouras, I, Brookes, VJ, Jori, F, Martin, A, Pfeiffer, DU, and Dürr, S. Emerging zoonotic diseases: should we rethink the animal–human interface? Front Vet Sci. (2020) 7:582743. doi: 10.3389/fvets.2020.582743
43. El Bizri, HR, Morcatty, TQ, Valsecchi, J, Mayor, P, Ribeiro, JES, Vasconcelos Neto, CFA, et al. Urban wild meat consumption and trade in Central Amazonia. Conserv Biol. (2020) 34:438–48. doi: 10.1111/cobi.13420
44. Crooks, KR, Burdett, CL, Theobald, DM, Rondinini, C, and Boitani, L. Global patterns of fragmentation and connectivity of mammalian carnivore habitat. Philos Trans R Soc B Biol Sci. (2011) 366:2642–51. doi: 10.1098/rstb.2011.0120
45. Grogan, LF, Berger, L, Rose, K, Grillo, V, Cashins, SD, and Skerratt, LF. Surveillance for emerging biodiversity diseases of wildlife. PLoS Pathog. (2014) 10:e1004015. doi: 10.1371/journal.ppat.1004015
46. Martinez, ME. The calendar of epidemics: seasonal cycles of infectious diseases. PLoS Pathog. (2018) 14:e1007327. doi: 10.1371/journal.ppat.1007327
47. Uribe, M, Rodríguez-Posada, ME, and Ramirez-Nieto, GC. Molecular evidence of orthomyxovirus presence in Colombian Neotropical bats. Front Microbiol. (2022) 13:845546. doi: 10.3389/fmicb.2022.845546
48. Nascimento Gomes, AP, dos Santos, MM, Olifiers, N, do Val Vilela, R, Guimarães Beltrão, M, Maldonado Júnior, A, et al. Molecular phylogenetic study in Spirocercidae (Nematoda) with description of a new species Spirobakerus sagittalis sp. nov. in wild canid Cerdocyon thous from Brazil. Parasitol Res. (2021) 120:1713–25. doi: 10.1007/s00436-021-07106-x
49. Rojas, A, Sanchis-Monsonís, G, Alić, A, Hodžić, A, Otranto, D, Yasur-Landau, D, et al. Spirocerca vulpis sp. nov. (Spiruridae: Spirocercidae): description of a new nematode species of the red fox, Vulpes vulpes (Carnivora: Canidae). Parasitology. (2018) 145:1917–28. doi: 10.1017/S0031182018000707
50. Gomes, APN, Olifiers, N, Souza, JGR, Barbosa, HS, D’Andrea, PS, and Maldonado, A. A new acanthocephalan species (Archiacanthocephala: Oligacanthorhynchidae) from the crab-eating fox (Cerdocyon thous) in the Brazilian Pantanal wetlands. J Parasitol. (2015) 101:74–9. doi: 10.1645/13-321.1
51. Rausch, RL, and Bernstein, JJ. Echinococcus vogeli sp. n. (Cestoda: Taeniidae) from the bush dog, Speothos venaticus (Lund). Z Tropenmed Parasitol. (1972) 23:25–34.
52. Tappe, D, Stich, A, and Frosch, M. Emergence of polycystic neotropical echinococcosis. Emerg Infect Dis. (2008) 14:292–7. doi: 10.3201/eid1402.070742
53. Rinas, MA, Nesnek, R, Kinsella, JM, and DeMatteo, KE. Fatal aortic aneurysm and rupture in a neotropical bush dog (Speothos venaticus) caused by Spirocerca lupi. Vet Parasitol. (2009) 164:347–9. doi: 10.1016/j.vetpar.2009.05.006
54. Vizcaychipi, KA, Rinas, M, Irazu, L, Miyagi, A, Argüelles, CF, and Dematteo, KE. Neotropical zoonotic parasites in bush dogs (Speothos venaticus) from upper Paraná Atlantic forests in Misiones, Argentina. Vector-Borne Zoonotic Dis. (2016) 16:664–72. doi: 10.1089/vbz.2015.1929
55. Ribeiro, CT, Verocal, GG, and Tavares, LER. Dioctophyme renale (Nematoda, Dioctophymatidae) infection in the crab-eating fox (Cerdocyon thous) from Brazil. J Wildl Dis. (2009) 45:248–50. doi: 10.7589/0090-3558-45.1.248
56. Almeida,, Kim, PCP, Melo,, Nogueira, JF, Martins, FDC, Garcia, JL, et al. Neospora caninum DNA in feces of crab-eating fox (Cerdocyon thous – Linnaeus, 1776) from northeastern Brazil. Acta Trop (2019) 197:105068. doi: 10.1016/j.actatropica.2019.105068
57. Copat, B, Bastiani, PV, Castellarin Jaconi, F, Wallyson Damarem, W, Streck, AF, de Oliveira, EC, et al. Presentation of hemolytic and hemorrhagic rangeliosis in Cerdocyon thous. Ticks Tick Borne Dis. (2019) 10:690–3. doi: 10.1016/j.ttbdis.2019.02.010
58. Soares, RM, Cortez, LRPB, Gennari, SM, Sercundes, MK, Keid, LB, and Pena, HFJ. Crab-eating fox (Cerdocyon thous), a south American canid, as a definitive host for Hammondia heydorni. Vet Parasitol. (2009) 162:46–50. doi: 10.1016/j.vetpar.2009.02.003
59. Caprioli, RA, De Andrade, CP, Argenta, FF, Ehlers, LP, Soares, JF, Pavarini, SP, et al. Angiostrongylosis in Cerdocyon thous (crab-eating fox) and Lycalopex gymnocercus (pampas fox) in southern Brazil. Parasitology. (2019) 146:617–24. doi: 10.1017/S0031182018001865
60. Vieira, FM, Luque, JL, de Souza, LS, de Moraes Neto, AHA, and Muniz-Pereira, LC. Dipylidium caninum (Cyclophyllidea, Dipylidiidae) in a wild carnivore from Brazil. J Wildl Dis. (2012) 48:233–4. doi: 10.7589/0090-3558-48.1.233
61. Almeida, JC, Melo, RPB, Kim, PCP, Guerra, NR, Alves, LC, Costa, DF, et al. Molecular and serological investigation of infectious diseases in captive and free-range crab-eating fox (Cerdocyon thous - Linnaeus, 1776) from northeastern Brazil. Acta Parasitol. (2018) 63:184–9. doi: 10.1515/ap-2018-0021
62. Ramos, VN, Lemos, FG, Azevedo, FC, Arrais, RC, Lima, CFM, Candeias, IZ, et al. Wild carnivores, domestic dogs and ticks: shared parasitism in the Brazilian Cerrado. Parasitology. (2020) 147:689–98. doi: 10.1017/S0031182020000335
63. Fiorello, CV, Robbins, RG, Maffei, L, and Wade, SE. Parasites of free-ranging small canids and felids in the Bolivian Chaco. J Zoo Wildl Med. (2006) 37:130–4. doi: 10.1638/05-075.1
64. Ilić, T, Nišavić, U, Gajić, B, Nenadović, K, Ristić, M, Stanojević, D, et al. Prevalence of intestinal parasites in dogs from public shelters in Serbia. Comp Immunol Microbiol Infect Dis. (2021) 76:101653. doi: 10.1016/j.cimid.2021.101653
65. Felsmann, M, Michalski, M, Felsmann, M, Sokół, R, Szarek, J, and Strzyżewska-Worotyńska, E. Invasive forms of canine endoparasites as a potential threat to public health – a review and own studies. Ann Agric Environ Med. (2017) 24:245–9. doi: 10.5604/12321966.1235019
66. Dantas-Torres, F, and Otranto, D. Dogs, cats, parasites, and humans in Brazil: opening the black box. Parasit Vectors. (2014) 7:22. doi: 10.1186/1756-3305-7-22
67. Mulinge, E, Zeyhle, E, Mpario, J, Mugo, M, Nungari, L, Ngugi, B, et al. A survey of intestinal helminths in domestic dogs in a human–animal–environmental interface: the Oloisukut conservancy, Narok County, Kenya. J Helminthol. (2021) 95:e59. doi: 10.1017/S0022149X21000547
68. Yu, Z, Ruan, Y, Zhou, M, Chen, S, Zhang, Y, Wang, L, et al. Prevalence of intestinal parasites in companion dogs with diarrhea in Beijing, China, and genetic characteristics of Giardia and Cryptosporidium species. Parasitol Res. (2018) 117:35–43. doi: 10.1007/s00436-017-5631-7
69. Pilarczyk, BM, Tomza-Marciniak, AK, Pilarczyk, R, Rząd, I, Bąkowska, MJ, Udała, JM, et al. Infection of raccoon dogs (Nyctereutes procyonoides) from northern Poland with gastrointestinal parasites as a potential threat to human health. J Clin Med. (2022) 11:1277. doi: 10.3390/jcm11051277
70. Darabi, E, Beigom Kia, E, Mohebali, M, Mobedi, I, Zahabiun, F, Zarei, Z, et al. Gastrointestinal helminthic parasites of stray cats (Felis catus) in Northwest Iran. Iran J Parasitol. (2021) 16:418–25. doi: 10.18502/ijpa.v16i3.7095
71. Labuschagne, M, Beugnet, F, Rehbein, S, Guillot, J, Fourie, J, and Crafford, D. Analysis of Dipylidium caninum tapeworms from dogs and cats, or their respective fleas. Parasite. (2018) 25:30. doi: 10.1051/parasite/2018028
72. García-Agudo, L, García-Martos, P, and Rodríguez-Iglesias, M. Dipylidium caninum infection in an infant: a rare case report and literature review. Asian Pac J Trop Biomed. (2014) 4:S565–7. doi: 10.12980/APJTB.4.2014APJTB-2014-0034
73. Hogan, CA, and Schwenk, H. Dipylidium caninum infection. N Engl J Med. (2019) 380:e39. doi: 10.1056/NEJMicm1813985
74. Smout, F, Skerratt, L, Johnson, C, Butler, J, and Congdon, B. Zoonotic helminth diseases in dogs and dingoes utilising shared resources in an Australian aboriginal community. Trop Med Infect Dis. (2018) 3:110. doi: 10.3390/tropicalmed3040110
75. Ćirović, D, Pavlović, I, Penezić, A, Kulišić, Z, and Selaković, S. Levels of infection of intestinal helminth species in the golden jackal Canis aureus from Serbia. J Helminthol. (2015) 89:28–33. doi: 10.1017/S0022149X13000552
76. East, ML, Kurze, C, Wilhelm, K, Benhaiem, S, and Hofer, H. Factors influencing Dipylidium sp. infection in a free-ranging social carnivore, the spotted hyaena (Crocuta crocuta). Int J Parasitol Parasites Wildl. (2013) 2:257–65. doi: 10.1016/j.ijppaw.2013.09.003
77. Erol, U, Sarimehmetoglu, O, and Utuk, AE. Intestinal system helminths of red foxes and molecular characterization Taeniid cestodes. Parasitol Res. (2021) 120:2847–54. doi: 10.1007/s00436-021-07227-3
78. Peña-Quistial, MG, Benavides-Montaño, JA, Duque, NJR, and Benavides-Montaño, GA. Prevalence and associated risk factors of intestinal parasites in rural high-mountain communities of the Valle del Cauca—Colombia. PLoS Negl Trop Dis. (2020) 14:e0008734. doi: 10.1371/journal.pntd.0008734
79. Rousseau, J, Castro, A, Novo, T, and Maia, C. Dipylidium caninum in the twenty-first century: epidemiological studies and reported cases in companion animals and humans. Parasit Vectors. (2022) 15:131. doi: 10.1186/s13071-022-05243-5
80. Campos, DMB, Barbosa, AP, de Oliveira, JA, Tavares, GG, Cravo, PVL, and Ostermayer, AL. Human lagochilascariasis—a rare helminthic disease. PLoS Negl Trop Dis. (2017) 11:e0005510. doi: 10.1371/journal.pntd.0005510
81. Scioscia, NP, Olmos, L, Gorosábel, A, Bernad, L, Pedrana, J, and Denegri, GM. Natural infection in pampas fox (Lycalopex gymnocercus) by Lagochilascaris major Leiper, 1910 (Nematoda: Ascarididae) in Buenos Aires, Argentina. Zeitschrift für Parasitenkunde (Berlin, Germany). (2018) 117:3023–7. doi: 10.1007/s00436-018-5978-4
82. Moncada, LI, Alvarez, CA, Castellanos, C, Caceres, E, Nicholls, S, and Corredor, A. Lagochilascaris minor in a patient from the Colombian amazon: a case report. Rev Inst Med Trop Sao Paulo. (1998) 40:387–9. doi: 10.1590/s0036-46651998000600009
83. Little, MD, and Botero, D. Two cases of human Lagochilascaris infection in Colombia. Am J Trop Med Hyg. (1984) 33:381–6. doi: 10.4269/ajtmh.1984.33.381
84. Scholz, T, Kuchta, R, and Brabec, J. Broad tapeworms (Diphyllobothriidae), parasites of wildlife and humans: recent progress and future challenges. Int J Parasitol Parasites Wildl. (2019) 9:359–69. doi: 10.1016/j.ijppaw.2019.02.001
85. Hwang, Y-H, Son, W, Kim, Y-W, Kang, D-H, Chang, H-H, Goo, Y-K, et al. A retrieved sparganum of Spirometra erinaceieuropaei from a Korean man during mechanical thrombectomy. Korean J Parasitol. (2020) 58:309–13. doi: 10.3347/kjp.2020.58.3.309
87. Kuchta, R, Scholz, T, Brabec, J, and Narduzzi-Wicht, B. Chapter 17: Diphyllobothrium, Diplogonoporus and Spirometra In: L Xiao, U Ryan, and Y Feng, editors. Biology of foodborne parasites. Section III: Important foodborne helminths. Boca Raton: CRC Press (2015). 299–326.
88. Gomez, JJ, and Botero, D. The first case of sparganosis in Colombia. Am J Trop Med Hyg. (1958) 7:597–9. doi: 10.4269/ajtmh.1958.7.597
Keywords: neglected, zoonosis, wild canids, Neotropics, dipylidiasis, lagochilascariosis, sparganosis
Citation: Uribe M, Brabec J, Chaparro-Gutiérrez JJ and Hermosilla C (2023) Neglected zoonotic helminthiases in wild canids: new insights from South America. Front. Vet. Sci. 10:1235182. doi: 10.3389/fvets.2023.1235182
Edited by:
Georgiana Deak, University of Agricultural Sciences and Veterinary Medicine of Cluj-Napoca, RomaniaReviewed by:
Calin Mircea Gherman, University of Agricultural Sciences and Veterinary Medicine of Cluj-Napoca, RomaniaHarold Salant, Hebrew University of Jerusalem, Israel
Copyright © 2023 Uribe, Brabec, Chaparro-Gutiérrez and Hermosilla. This is an open-access article distributed under the terms of the Creative Commons Attribution License (CC BY). The use, distribution or reproduction in other forums is permitted, provided the original author(s) and the copyright owner(s) are credited and that the original publication in this journal is cited, in accordance with accepted academic practice. No use, distribution or reproduction is permitted which does not comply with these terms.
*Correspondence: Carlos Hermosilla, carlos.r.hermosilla@vetmed.uni-giessen.de