Ex vivo pharmacokinetic/pharmacodynamic of hexahydrocolupulone against Clostridium perfringens in broiler chickens
- 1Guangdong Provincial Key Laboratory of Veterinary Pharmaceutics Development and Safety Evaluation, College of Veterinary Medicine, South China Agricultural University, Guangzhou, China
- 2National Risk Assessment Laboratory for Antimicrobial Resistance of Animal Original Bacteria, Guangzhou, China
- 3Guangzhou Insighter Biotechnology Co., Ltd., Guangzhou, China
The economic impact of necrotizing enteritis (NE) resulting from Clostridium perfringens infection has been significant within the broiler industry. This study primarily investigated the antibacterial efficacy of hexahydrocolupulone against C. perfringens, and its pharmacokinetics within the ileal contents of broiler chickens. Additionally, a dosing regimen was developed based on the pharmacokinetic/pharmacodynamic (PK/PD) model specific to broiler chickens. Results of the study indicated that the minimum inhibitory concentration (MIC) of hexahydrocolupulone against C. perfringens ranged from 2 mg/L to 16 mg/L in MH broth. However, in ileal content, the MIC ranged from 8 mg/L to 64 mg/L. The mutation prevention concentration (MPC) in the culture medium was found to be 128 mg/L. After oral administration of hexahydrocolupulone at a single dosage of 10–40 mg/kg bodyweight, the peak concentration (Cmax), maximum concentration time (Tmax), and area under the concentration-time curve (AUC) in ileal content of broiler chickens were 291.42–3519.50 μg/g, 1–1.5 h, and 478.99–3121.41 μg h/g, respectively. By integrating the in vivo PK and ex vivo PD data, the AUC0-24h/MIC values required for achieving bacteriostatic, bactericidal, and bacterial eradication effects were determined to be 36.79, 52.67, and 62.71 h, respectively. A dosage regimen of 32.9 mg/kg at 24 h intervals for a duration of 3 days would yield therapeutic efficacy in broiler chickens against C. perfringens, provided that the MIC below 4 mg/L.
1 Introduction
The incidence of Clostridium perfringens-induced necrotic enteritis (NE) in the broiler industry, as well as other subclinical diseases associated with the bacteria, has increased (1, 2). Due to the significant costs associated with disease in broiler production (3), the annual cost of NE to the global poultry industry is estimated to be approximately $6 billion, including production losses and the cost of management measures (4). Antibiotic growth promoters (AGPs) can sustain intestinal well-being and modify the composition of resident microorganisms, consequently enhancing production efficiency and ameliorating intestinal health in broiler chickens (5). In recent years, numerous countries worldwide, including the European Union and the United States, have ceased the utilization of antibiotics as growth promoters in poultry feed due to the escalating resistance of C. perfringens to these medications (6, 7). When high concentrations of antibiotics were used, their residues can be found in the blood, the other tissues of the poultry and feces (8). The drug excreted in the feces of treated animals, and can contaminate the feed of other untreated animals (9). Vegetables may also be contaminated from feces especially in countries in where feces are generally used as a fertilizer (10). This presents a significant risk to public health, underscoring the urgency to find herbal or natural products to replace antibiotics.
As research progresses, certain natural antimicrobial agents utilized in food preservation have demonstrated exceptional efficacy in suppressing microbial proliferation (11). Some of the active ingredients extracted from the plant are considered safe and reliable (12). Hop (Humulus lupulus L.) is a dioecious vine belonging to the genus Humulus of the Cannabis family and widely cultivated around the world (13). Hop-derived bitter acids and their oxidation products not only give the unique bitter taste and aroma of beer but also exert a wide range of biological effects, including antibacterial (14), anti-inflammation (15), antifibrogenesis (16), and they have been considered as chemopreventive agents. β-acid, a member of bitter acids (17), contains a blend of lupulone homologs such as lupulone, colupulone, and adlupulone (13). Although β-acids have antimicrobial effects, they are unstable and are easily oxidized (18). However, as hydrogenated derivatives of β-acids, hexahydro-β-acids (HBA) are mixtures of analogues such as hexahydrolupulone, hexahydrocolupulone, and hexahydroadlupulone (19). The stability, antibacterial and antioxidant activity of HBA are better than those of β-acids (19, 20). HBA can inhibit the expression of proteins related to DNA replication, transcription, translation, and proteins related to ribosome synthesis of Listeria monocytogenes, resulting in a decrease in protein content in cells, thereby hindering normal life activities and physiological metabolism (21). Based on the Federal Regulations of Food and Drug Administration (FDA), hops and their extracts are widely acknowledged as safe (22). The structural formula of hexahydrocolupulone is shown in Figure 1.
The objective of this study was to investigate the pharmacokinetic (PK) and pharmacodynamic (PD) properties hexahydrocolupulone in the ileal content. The inhibitory Imax model was employed to compute the PK/PD indices necessary for varying levels of antibacterial efficacy. Moreover, the dosage regimen of hexahydrocolupulone in broiler chickens facilitated the determination of an efficacious dose for NE.
2 Materials and methods
2.1 Chemicals
Hexahydrocolupulone (98.6%) was provided by Guangzhou Insighter Biotechnology (Guangzhou, China). Mueller–Hinton (MH) broth and MH agar were obtained from Qingdao Hope Bio-Technology Co., Ltd (Qingdao, China). Tryptone–sulfite–cycloserine (TSC) agar was obtained from Guangdong Huankai Microbial Technology (Guangdong, China).
2.2 Bacteria
A total of 26 isolates of C. perfringens were employed in this study, comprising one standard strain (ATCC 13124) procured from the Chinese Veterinary Culture Collection Center and 25 strains derived from broiler chickens in Guangdong Province from 2021 to 2023. All strains were stored at −80°C until use. Before each experiment, these bacteria cultures were subcultured on TSC agar and incubated at 37°C for 18–24 h.
2.3 Animals
This study utilized two-week-old Sanhuang broiler chickens with a weight of 50 ± 5 g, which were in a healthy condition. Prior to conducting the experiments, the broiler chickens underwent a 7 days acclimation period. Throughout the study, the broiler chickens were provided with unrestricted access to antibiotic-free food and water. Food, but not water, was withheld for 12 h before dosing and until 4 h after drug administration. All procedures conducted in this study were approved by the Institutional Animal Care and Use Committee of South China Agricultural University, with the assigned approval number of 2022A016.
2.4 Determination of MIC, MBC, and MPC
The sensitivity of hexahydrocolupulone selected in MH broth was evaluated using the microdilution method recommended by CLSI (23). Following a 24 h incubation period, the MIC was established as the lowest concentration of hexahydrocolupulone that effectively hindered observable bacterial growth. Additionally, the ileal contents were assessed for MIC through the microdilution technique. To determine the minimal bactericidal concentration (MBC), 100 μL suspension from the MIC determination wells was consecutively diluted 10 fold in broth. The colony-forming unit of each dilution was counted by spreading 20 μL onto TSC agar plates after 24 h incubation at 37°C in anaerobic condition. The MBC was determined as the concentration at which a 99.9% reduction in the bacterial counts was achieved. The agar method was employed to determine the MPC of hexahydrocolupulone (24). The C. perfringens strains with a concentration of 1010 CFU/mL were inoculated to agar plates that contained varying concentrations of hexahydrocolupulone (1 MIC, 2 MIC, 4 MIC, 8 MIC, 16 MIC, and 32 MIC). These plates were then incubated at 37°C for 72 h. The MPC was defined as the concentration of hexahydrocolupulone that did not facilitate the growth of bacteria on the agar plates.
2.5 In vitro and ex vivo time-killing curves
Different concentrations of hexahydrocolupulone (1/4 MIC, 1/2 MIC, 1 MIC, 2 MIC, and 4 MIC) were prepared in MH broth. Test tubes were inoculated with 106 CFU/mL C. perfringens and incubated at 37°C. Bacterial counts (CFU/mL) were determined at 0, 1, 2, 4, 8, 12, and 24 h of incubation. Specifically, 100 μL of culture was collected at each time point and serially diluted. Colony counts were performed the following morning with a limit of detection (LOD) of 50 CFU/mL. All experiments were conducted in triplicate.
The ileal contents were collected at different intervals after oral administration of 20 mg/kg hexahydrocolupulone in a PK test for high-speed centrifugation and sterile filter treatment. Subsequently, an ex vivo time-kill curve was established. Viable bacteria were enumerated at specific time points (0, 1, 2, 4, 8, 12, and 24 h) by incubating tubes containing the bacterial culture and intestinal contents at 37°C. The LOD for viable bacteria was set at 50 CFU/mL.
2.6 Pharmacokinetic of hexahydrocolupulone in broiler chickens ileum content
Following a period of 7 days of acclimatization, the 144 chickens were subjected to random allocation into three distinct groups, each comprising 48 chickens. The administered doses were 10, 20, and 40 mg/kg. Subsequently, at specific time intervals after the oral administration of hexahydrocolupulone (0.083, 0.25, 0.5, 0.75, 1, 1.5, 2, 4, 6, 8, 12, and 24 h), four broiler chickens were euthanized in each group to collect ileum contents.
The ileum content was accurately weighed to 0.2 ± 0.02 g and then added with 600 μL of 1% formic acid methanol. The mixture was vortexed for 1 min and centrifuged at 13,000 rpm for 10 min. About 200 μL of supernatant was added with 400 μL of 1% formic acid methanol. The mixture was vortexed for 1 min at 13,000 rpm, centrifuged for 10 min, passed through a 0.22 μm filter membrane, and analyzed by high-performance liquid chromatography (HPLC).
The determination of hexahydrocolupulone concentrations in intestinal contents by using HPLC and UV detectors involved the following conditions: UV detection at 341 nm, a column temperature of 30°C, a mobile phase consisting of water with 0.05% phosphoric acid and methanol, and a sample size of 20 μL injected into the HPLC system (Shimazu LC-20A) with a flow rate of 1 mL/min. Separation was achieved using an A R D-C18 (250 mm × 4.6 mm, 5 μm) column. The calibration range for this analysis was 0.25–20 μg/g. The precision levels for intraday and interday measurements ranged from 1.54 to 6.74% and from 4.82 to 7.43%, respectively. The LOD and limit of quantification (LOQ) were determined to be 0.10 and 0.25 μg/g, respectively. A non-compartmental analysis of hexahydrocolupulone concentrations in the intestinal content was conducted using Phoenix WinNonlin® 8.4 (Certara, L.P., Princeton, NJ, United States).
2.7 Analysis of the PK/PD relationship
The ex vivo PK/PD relationships of hexahydrocolupulone in the intestine were simulated using the Imax model in WinNonlin® 8.4 (Certara, L.P., Princeton, NJ, United States) with the following equation (25):
The potential optimal dosage can be calculated using the following equation (26, 27):
3 Results
3.1 MIC, MBC, and MPC of hexahydrocolupulone against C. perfringens
A range of 2–16 mg/L was observed in the MIC of hexahydrocolupulone against 26 strains of C. perfringens. The percentages of each MIC (2, 4, 8, and 16 mg/L) were 26.92, 42.31, 26.92, and 3.85%, respectively. The distribution of MICs is depicted in Figure 2. In MH broth, the MIC and MBC of hexahydrocolupulone against C. perfringens ATCC13124 were 4 and 16 mg/L, respectively; however, in ileal content, these concentrations were sixteen times higher at 16 and 64 mg/L, respectively. The MPC in the culture medium was found to be 128 mg/L, which was 32 times the MIC. The MIC of hexahydrocolupulone against C. perfringens ATCC13124 in the ileum content was determined to be 16 μg/mL, as shown in Table 1.
The calculated MIC of hexahydrocolupulone for C. perfringens ATCC13124 in the ileum content (16 mg/L) was found to be four times higher than that in MH broth (4 mg/L), indicating a strong reinforcing effect of MH broth. To further validate the effects of MH broth, we determined the MICs of hexahydrocolupulone in MH broth and ileum content against 26 selected C. perfringens isolates. Interestingly, a significant difference in the geometric mean MIC values was observed between MH broth and ileal content, resulting in an ileal content/MH ratio of 5.57 for MICs (p < 0.01; Table 2).
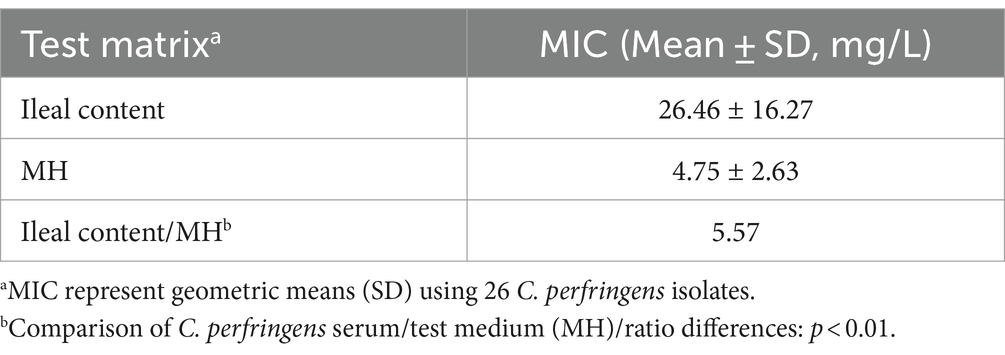
Table 2. Attenuative effect of matrix on in vitro susceptibility of hexahydrocolupulone against C. perfringens (n = 26).
3.2 In vitro and ex vivo antimicrobial activity
The time-kill curves of hexahydrocolupulone against C. perfringens ATCC13124 and GDZ21C61W in MH broth are depicted in Figure 3. The curves revealed that hexahydrocolupulone exhibited a concentration-dependent bactericidal effect. Notably, a sustained inhibitory impact on bacterial growth was observed when C. perfringens was exposed to hexahydrocolupulone concentrations exceeding 8 mg/L.
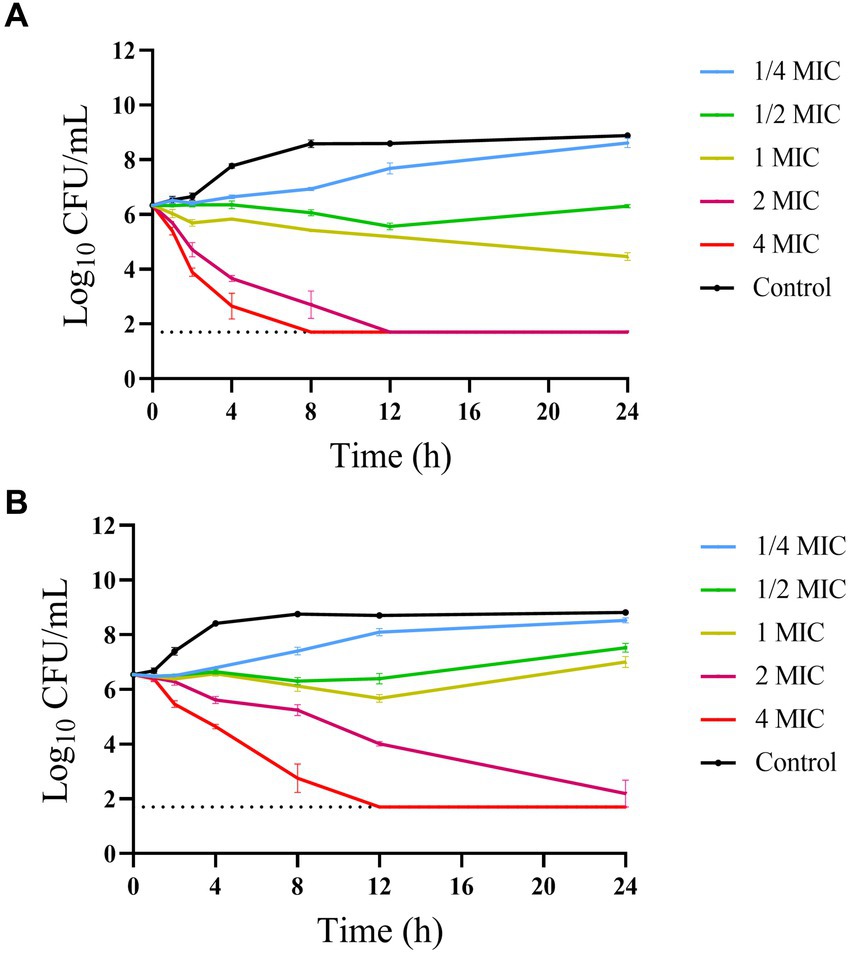
Figure 3. In vitro time-kill curve of hexahydrocolupulone against C. perfringens ATCC13124 (A) and GDZ21C61W (B).
The ex vivo time-kill curves were used to assess the effects of hexahydrocolupulone on samples collected at various time points. The ex vivo time-kill curves of hexahydrocolupulone against C. perfringens ATCC13124, GDZ21C61W, and GDZ21C222W are presented in Figure 4. The results indicated that hexahydrocolupulone exhibited a concentration-dependent effect in ex vivo, which was consistent with the observed time-kill curves in vitro. Notably, a significant reduction in bacterial count was observed in the high-concentration group (at 0.75–2 h), with no detectable bacteria at 24 h.
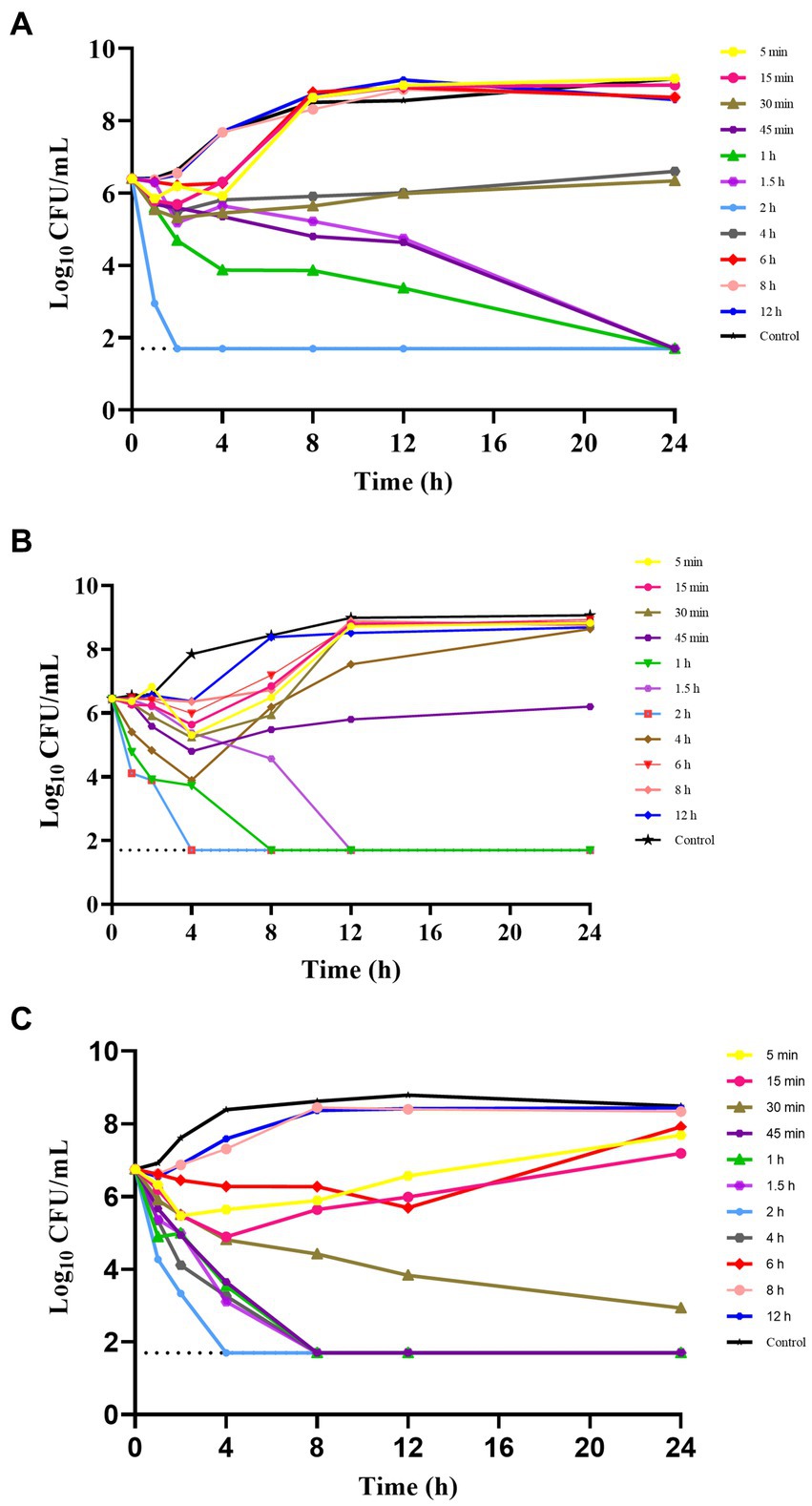
Figure 4. Ex vitro time-kill curve of hexahydrocolupulone against C. perfringens ATCC13124 (A), GDZ21C61W (B), and GDZ21C222W (C).
3.3 Pharmacokinetics analysis
The concentration-time curve of intestinal contents in broiler chickens after a single oral gavage at a dose of 10, 20, and 40 mg/kg was shown in Figure 5. Table 3 presents the PK parameters of hexahydrocolupulone in intestinal content. The Tmax in 10 mg/kg, 20 mg/kg, and 40 mg/kg were 1.38, 1.50, and 1 h, respectively. The Cmax were 291.42 ± 90.01, 440.88 ± 181.09, and 3519.50 ± 752.01 μg/g, respectively. And the AUClast were 478.99 ± 149.92, 779.48 ± 210.59, and 3121.41 ± 895.08 μg h/g, respectively.
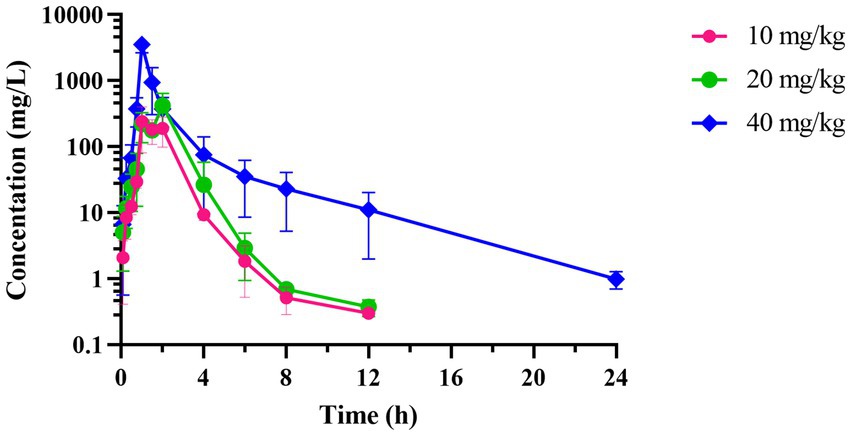
Figure 5. The time-concentration profile of hexahydrocolupulone in intestinal contents of broiler chickens following a single oral administration of 10, 20, and 40 mg/kg (n = 4).
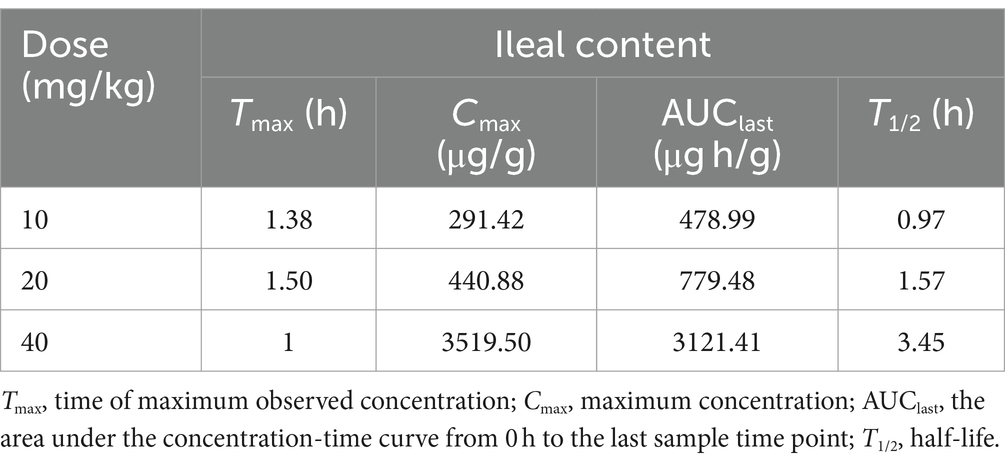
Table 3. Pharmacokinetic parameters of hexahydrocolupulone in ileal content following single gavage in healthy broiler chickens (n = 4, mean ± SD).
3.4 PK/PD analysis
In ileum content, the Imax model effectively elucidated the correlation between the antimicrobial effectiveness of hexahydrocolupulone and the PK/PD parameter represented by the AUC0-24h/MIC ratio in the ileum. The correlation between the efficacy of hexahydrocolupulone against C. perfringens and each of the PK/PD indices is depicted in Figure 6. Table 4 shows the AUC0-24h/MIC ratios required to achieve various efficacy targets. The AUC0-24h/MIC values for bacteriostatic activity, bactericidal action, and virtual eradication in the ileum were 36.79, 52.67, and 62.71 h, respectively.
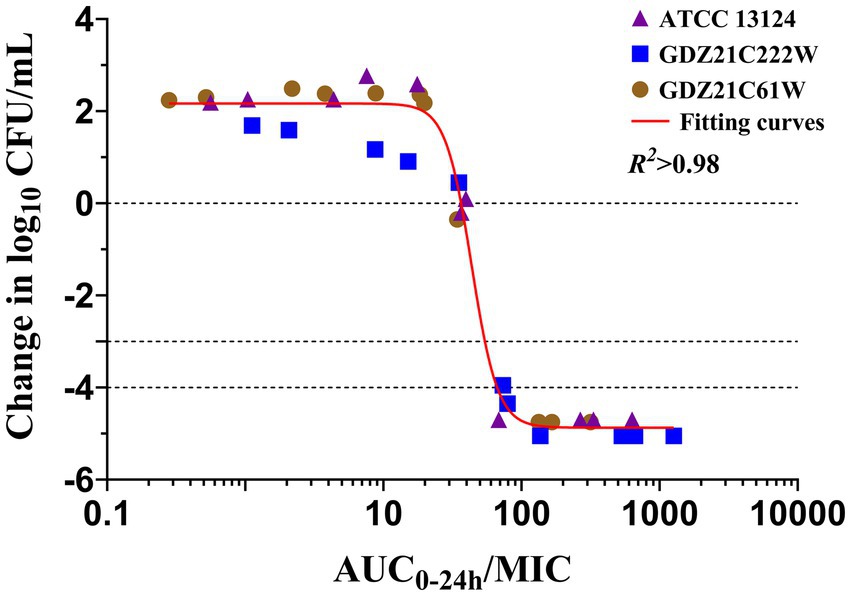
Figure 6. Relationships between the effect of hexahydrocolupulone against C. perfringens and PK/PD indices AUC0–24h/MIC in the ex vivo model. R2 is the coefficient of determination.
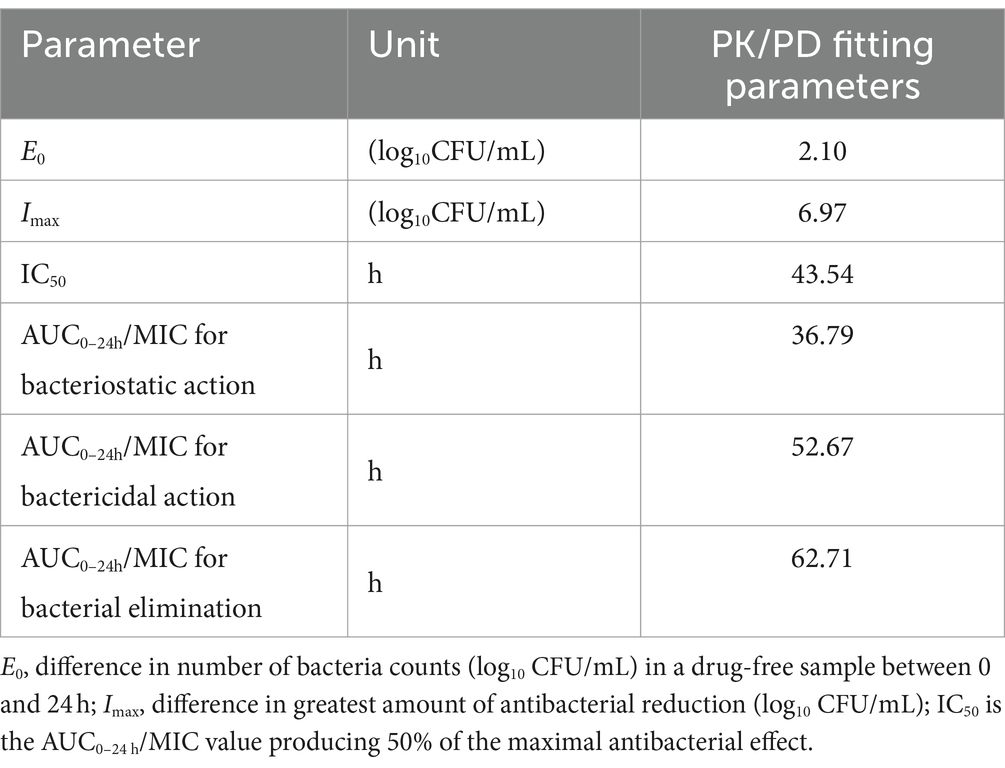
Table 4. PK/PD parameter of ex vivo data after oral administration hexahydrocolupulone in broiler chickens.
4 Discussion
Given the increasing apprehension among consumers regarding the presence of antibiotic residues in poultry products and the emergence of antibiotic-resistant strains, substitutes for antibiotics are necessary (28, 29). Previous studies have confirmed that the active ingredients, derived or separated from hops, exhibit a substantial inhibitory effect on various pathogenic microorganisms [Escherichia coli, S. aureus, Listeria monocytogenes (21), and C. perfringens (14), and they are considered safe and reliable (12)]. The antibacterial activity of hexahydrocolupulone was evaluated using the broth microdilution method to determine the MICs of hexahydrocolupulone against 26 strains of C. perfringens. The MIC range of hexahydrocolupulone against clinical C. perfringens strains was found to be 2–32 mg/L, with more than half of the strains exhibiting MICs in the range of 2–4 μg/mL. This result indicated the strong sensitivity of C. perfringens to hexahydrocolupulone. This study also investigated the effect of different ex vivo and in vitro conditions, such as MH broth and ileal content, on bacterial growth and determination of MIC. The MIC of hexahydrocolupulone against C. perfringens ATCC13124 was found to be 4 mg/L in MH broth and 32 mg/L in the ileum. To assess the effectiveness of hexahydrocolupulone against a broad range of C. perfringens isolates, we determined MICs in MH broth and ileum for 26 selected isolates. The geometric means of the MICs differed significantly between MH broth and ileal content, with an ileal content/MH ratio of 5.57 for MICs. This result suggested that the ileal content has a substantial attenuative effect on the efficacy of hexahydrocolupulone. The utilization of the PK/PD index, specifically the AUC0–24h/MIC ratio, is highly suitable when the MIC is based on the ileal content.
On the basis of the PK findings of hexahydrocolupulone, the absorption and distribution of hexahydrocolupulone in broiler intestine were found rapid after oral administration, as evidenced by peak concentrations attained within 1.5 h; this pattern was similar to that observed with non-oral absorption medications like cyadox (30). Thus, the utilization of hexahydrocolupulone as a potential therapeutic agent against C. perfringens is justified, given that this bacterium predominantly targets the intestinal tract of humans and animals. AUClast and Cmax ranged from 478.88 μg h/g to 3,121.41 μg h/g and from 291.42 μg/g to 3,519.50 μg/g, respectively. This study aimed to investigate the PK data of hexahydrocolupulone in the ileum of healthy broiler chickens for PK/PD studies. Following intragastric administration, the concentrations of hexahydrocolupulone in the broiler chickens’ ileum exhibited a rapid decrease due to chyme transport, which was consistent with the PK properties observed in other orally administered non-absorbable drugs.
The approach to drug development has evolved from an empirical methodology to a modeling and simulation-based methodology, wherein the interplay between PK and PD governs the correlation between dosage and response (31). In this study, a strong correlation (R2 > 0.98) was observed between the PK/PD index of AUC0-24h/MIC and antibacterial activity in the ex vivo model. The AUC0-24h/MIC targets necessary to achieve bacteriostatic, bactericidal, and virtual eradication effects were determined to be 36.79, 52.67, and 62.71 h, respectively. To calculate the dosage, we multiplied the MIC distribution in MH broth by a scaling factor of 5.57 to account for the differences between MH and ileal content, and the Cl/F in ileal content was measured to be 28.01 ± 9.06 mL/kg h. The use of fu was not necessary for the utilization of PD data generated in the small intestine (32). The recommended therapeutic dosage of hexahydrocolupulone for the treatment of C. perfringens with an MIC of ≤4 mg/L was 32.9 mg/kg, to be administered every 24 h.
Some compounds of hops have potential to be used as feed additives to broiler chickens. Some references in the literature indicate that some compounds of hops can replace antimicrobial performance enhancers in the diets of broiler chickens (33). Nevertheless, hop supplementation at the highest concentration influenced the performance of broiler chickens (34). The potency of hop as an antimicrobial agent has also been shown in poultry. The results have demonstrated that hop ß-acid lupulone supplementation to drinking water decreased caecal C. perfringens counts in challenged chickens in both jejunal and caecal sampling sites across all lupulone dosages tested (14). On the other hand, no significant changes were noted in the overall microbiota of the caecum or the midgut when lupulone was added to the water (35). Therefore, it is significant to investigate the PK and PD properties hexahydrocolupulone in broiler chickens.
In this study, we successfully demonstrated the efficacy of hexahydrocolupulone against C. perfringens through in vitro and ex vivo experiments. Additionally, we determined the AUC0-24h/MIC targets of hexahydrocolupulone in simulated broiler intestines. Although HBA exhibit lipophilicity, rendering them insoluble in water, which hinders homogenous dispersion (36), Lu et al. (36) successfully prepared the inclusion complex of HBA/M-β-CD so that the water solubility of HBA was enhanced by CD inclusion. These findings strongly suggested that hexahydrocolupulone holds significant promise as a novel therapeutic agent for the treatment of C. perfringens infection in broiler chickens.
Data availability statement
The raw data supporting the conclusions of this article will be made available by the authors, without undue reservation.
Ethics statement
The animal study was reviewed and approved by Institutional Animal Care and Use Committee of South China Agricultural University, with the assigned approval number of 2022A016. The study was conducted in accordance with the local legislation and institutional requirements.
Author contributions
WZ: Writing – original draft, Writing – review & editing, Conceptualization, Data curation, Formal analysis, Methodology. YL: Conceptualization, Data curation, Formal analysis, Writing – review & editing, Investigation, Methodology. MM: Conceptualization, Data curation, Investigation, Methodology, Writing – review & editing. JY: Methodology, Writing – review & editing. HH: Methodology, Writing – review & editing. XP: Project administration, Writing – review & editing. ZZ: Funding acquisition, Project administration, Resources, Validation, Visualization, Writing – review & editing. DZ: Funding acquisition, Project administration, Resources, Validation, Visualization, Writing – review & editing.
Funding
The author(s) declare financial support was received for the research, authorship, and/or publication of this article. This research was supported by the National Key R&D Program of China (grant 2022YFD 1802100).
Conflict of interest
XP was employed by Guangzhou Insighter Biotechnology Co., Ltd.
The remaining authors declare that the research was conducted in the absence of any commercial or financial relationships that could be construed as a potential conflict of interest.
Publisher’s note
All claims expressed in this article are solely those of the authors and do not necessarily represent those of their affiliated organizations, or those of the publisher, the editors and the reviewers. Any product that may be evaluated in this article, or claim that may be made by its manufacturer, is not guaranteed or endorsed by the publisher.
Supplementary material
The Supplementary material for this article can be found online at: https://www.frontiersin.org/articles/10.3389/fvets.2024.1362292/full#supplementary-material
SUPPLEMENTARY FIGURE S1 | Chromatogram of a standard solution of hexahydrocolupulone.
SUPPLEMENTARY FIGURE S2 | Blank ileal content chromatogram.
SUPPLEMENTARY FIGURE S3 | Chromatogram of addition to the blank ileal contents with 10 μg/g of hexahydrocolupulone.
SUPPLEMENTARY FIGURE S4 | Chromatogram of ileal contents sample after 1.5 h of oral administration.
References
1. Emami, NK, and Dalloul, RA. Centennial review: recent developments in host-pathogen interactions during necrotic enteritis in poultry. Poult Sci. (2021) 100:101330. doi: 10.1016/j.psj.2021.101330
2. Yang, Q, Liu, J, Robinson, KJ, Whitmore, MA, Stewart, SN, and Zhang, G. Perturbations of the ileal mycobiota by necrotic enteritis in broiler chickens. J Anim Sci Biotechnol. (2021) 12:107. doi: 10.1186/s40104-021-00628-5
3. Lee, KW, Lillehoj, HS, Jeong, W, Jeoung, HY, and An, DJ. Avian necrotic enteritis: experimental models, host immunity, pathogenesis, risk factors, and vaccine development. Poult Sci. (2011) 90:1381–90. doi: 10.3382/ps.2010-01319
4. Timbermont, L, Haesebrouck, F, Ducatelle, R, and Van Immerseel, F. Necrotic enteritis in broilers: an updated review on the pathogenesis. Avian Pathol. (2011) 40:341–7. doi: 10.1080/03079457.2011.590967
5. Haque, MH, Sarker, S, Islam, MS, Islam, MA, Karim, MR, Kayesh, MEH, et al. Sustainable antibiotic-free broiler meat production: current trends, challenges, and possibilities in a developing country perspective. Biology. (2020) 9:110411. doi: 10.3390/biology9110411
6. Dibner, JJ, and Richards, JD. Antibiotic growth promoters in agriculture: history and mode of action. Poult Sci. (2005) 84:634–43. doi: 10.1093/ps/84.4.634
7. Castanon, JI. History of the use of antibiotic as growth promoters in European poultry feeds. Poult Sci. (2007) 86:2466–71. doi: 10.3382/ps.2007-00249
8. Bacanli, M, and Basaran, N. Importance of antibiotic residues in animal food. Food Chem Toxicol. (2019) 125:462–6. doi: 10.1016/j.fct.2019.01.033
9. Darwish, WS, Eldaly, EA, E-Abbasy, MT, Ikenaka, Y, Nakayama, S, and Ishizuka, M. Antibiotic residues in food: the african scenario. Jpn J Vet Res. (2013) 61:S13–22.
10. Phillips, I, Casewell, M, Cox, T, De Groot, B, Friis, C, Jones, R, et al. Does the use of antibiotics in food animals pose a risk to human health? A critical review of published data. Antimicrob Chemother. (2004) 172:136–52. doi: 10.1136/vr.f624
11. Efenberger-Szmechtyk, M, Nowak, A, and Czyzowska, A. Plant extracts rich in polyphenols: antibacterial agents and natural preservatives for meat and meat products. Crit Rev Food Sci Nutr. (2021) 61:149–78. doi: 10.1080/10408398.2020.1722060
12. Ahmadifar, E, Yousefi, M, Karimi, M, Raieni, RF, Dadar, M, Yilmaz, S, et al. Benefits of dietary polyphenols and polyphenol-rich additives to aquatic animal health: an overview. Rev Fish Sci Aquac. (2021) 29:478–511. doi: 10.1080/23308249.2020.1818689
13. Karabin, M, Hudcova, T, Jelinek, L, and Dostalek, P. Biologically active compounds from hops and prospects for their use. Compr Rev Food Sci Food Saf. (2016) 15:542–67. doi: 10.1111/1541-4337.12201
14. Siragusa, GR, Haas, GJ, Matthews, PD, Smith, RJ, Buhr, RJ, Dale, NM, et al. Antimicrobial activity of lupulone against Clostridium perfringens in the chicken intestinal tract jejunum and caecum. J Antimicrob Chemother. (2008) 61:853–8. doi: 10.1093/jac/dkn024
15. Van Cleemput, M, Heyerick, A, Libert, C, Swerts, K, Philippe, J, De Keukeleire, D, et al. Hop bitter acids efficiently block inflammation independent of gralpha, pparalpha, or ppargamma. Mol Nutr Food Res. (2009) 53:1143–55. doi: 10.1002/mnfr.200800493
16. Saugspier, M, Dorn, C, Thasler, WE, Gehrig, M, Heilmann, J, and Hellerbrand, C. Hop bitter acids exhibit anti-fibrogenic effects on hepatic stellate cells in vitro. Exp Mol Pathol. (2012) 92:222–8. doi: 10.1016/j.yexmp.2011.11.005
17. Duarte, LM, Adriano, L, and de Oliveira, M. Capillary electrophoresis in association with chemometrics approach for bitterness hop (humulus lupulus L.) classification. Electrophoresis. (2018) 39:1399–409. doi: 10.1002/elps.201700420
18. De Almeida, NEC, Nascimento, ESPD, and Cardoso, DR. On the reaction of lupulones, hops β-acids, with 1-hydroxyethyl radical. J Agric Food Chem. (2012) 60:10649–56. doi: 10.1021/jf302708c
19. Ruckle, L, and Senn, T. Hop acids can efficiently replace antibiotics in ethanol production. Int Sugar J. (2006) 108:139–47.
20. Yajima, H, Ikeshima, E, Shiraki, M, Kanaya, T, Fujiwara, D, Odai, H, et al. Isohumulones, bitter acids derived from hops, activate both peroxisome proliferator-activated receptor alpha and gamma and reduce insulin resistance. J Biol Chem. (2004) 279:33456–62. doi: 10.1074/jbc.M403456200
21. Tian, B, Xu, D, Li, W, Wang, J, Cheng, J, and Liu, Y. Proteomic analysis of hexahydro-beta-acids/hydroxypropyl-beta-cyclodextrin inhibit Listeria monocytogenes. Appl Microbiol Biotechnol. (2022) 106:755–71. doi: 10.1007/s00253-022-11764-x
22. Stirting, L, Mcconathy, L, Bettardo, B, Yasbar, S, Franco, J, Saverson, E, et al. Code of federal regulations. (2001)
23. CLSI. Performance standards for antimicrobial disk and dilution susceptibility tests for bacteria isolated from animals: Approved standard. 5th ed. Wayne: CLSI supplement VET01S (2020).
24. Kong, L, Lu, Y, Yang, L, Zhang, W, Zuo, B, Peng, X, et al. Pharmacokinetics and pharmacodynamics of colistin combined with isopropoxy benzene guanidine against mcr-1–positive Salmonella in an intestinal infection model. Front Microbiol. (2022) 13:907116. doi: 10.3389/fmicb.2022.907116
25. Riviere Je, TP. Chapter 13: simultaneous pharmacokinetic pharmacodynamic modeling. Riviere Je, (Ed.) Comparative pharmacokinetics: principles, techniques & applications. 2nd edn, Ames, IA: Wiley-Blackwell, John Wiley & Sons, Ltd., 255–294. (2011)
26. Toutain, PL, Del, CJ, and Bousquet-Melou, A. The pharmacokinetic-pharmacodynamic approach to a rational dosage regimen for antibiotics. Res Vet Sci. (2002) 73:105–14. doi: 10.1016/s0034-5288(02)00039-5
27. Toutain, P, Bousquet-Melou, A, and Martinez, M. AUC/MIC: a PK/PD index for antibiotics with a time dimension or simply a dimensionless scoring factor? J Antimicrob Chemother. (2007) 60:1185–8. doi: 10.1093/jac/dkm360
28. Hosny, RA, Gaber, AF, and Sorour, HK. Bacteriophage mediated control of necrotic enteritis caused by C. perfringens in broiler chickens. Vet Res Commun. (2021) 45:409. doi: 10.1007/s11259-021-09821-3
29. Parent, E, Archambault, M, Charlebois, A, Bernier-Lachance, J, and Boulianne, M. A chicken intestinal ligated loop model to study the virulence of Clostridium perfringens isolates recovered from antibiotic-free chicken flocks. Avian Pathol. (2017) 46:138–49. doi: 10.1080/03079457.2016.1228825
30. Wang, F, Mi, K, Ahmad, I, Xie, S, Hussain, HI, Yuan, Z, et al. Antibacterial activity of cyadox against Clostridium perfringens in broilers and a dosage regimen design based on pharmacokinetic-pharmacodynamic modeling. Microb Pathog. (2020) 141:103981. doi: 10.1016/j.micpath.2020.103981
31. Dingemanse, J, and Appel-Dingemanse, S. Integrated pharmacokinetics and pharmacodynamics in drug development. Clin Pharmacokinet. (2007) 46:713–37. doi: 10.2165/00003088-200746090-00001
32. Lu, Y, Yang, L, Zhang, W, Li, J, Peng, X, Qin, Z, et al. Pharmacokinetics and pharmacodynamics of isopropoxy benzene guanidine against Clostridium perfringens in an intestinal infection model. Front Vet Sci. (2022) 9:4248. doi: 10.3389/fvets.2022.1004248
33. Cornelison, J, Yan, F, Watkins, S, Rigby, L, Segal, J, and Waldroup, P. Evaluation of hops (Humulus Lupulus) as an antimicrobial in broiler diets. Int J Poultry Sci. (2006) 5:134–6. doi: 10.3923/ijps.2006.134.136
34. Rezar, V, Levart, A, Cerenak, A, Salobir, J, and Pirman, T. Effect of hop (Humulus Lupulus) supplementation on performance, oxidative stress, and oxidative stability of broiler chicken meat. Eur Poult Sci. (2020) 84:e299. doi: 10.1399/eps.2020.299
35. Tillman, GE, Haas, GJ, Wise, MG, Oakley, B, Smith, MA, and Siragusa, GR. Chicken intestine microbiota following the administration of lupulone, a hop-based antimicrobial. FEMS Microbiol Ecol. (2011) 77:395–403. doi: 10.1111/j.1574-6941.2011.01119.x
Keywords: hexahydrocolupulone, Clostridium perfringens, pharmacokinetic/pharmacodynamic (PK/PD), broiler, ex vivo
Citation: Zhang W, Lu Y, Ma M, Yang J, Huang H, Peng X, Zeng Z and Zeng D (2024) Ex vivo pharmacokinetic/pharmacodynamic of hexahydrocolupulone against Clostridium perfringens in broiler chickens. Front. Vet. Sci. 11:1362292. doi: 10.3389/fvets.2024.1362292
Edited by:
Arturo Anadón, Complutense University of Madrid, SpainReviewed by:
Cengiz Gokbulut, Balıkesir University, TürkiyeZigang Qu, Lanzhou Veterinary Research Institute (CAAS), China
Nora Mestorino, National University of La Plata, Argentina
Copyright © 2024 Zhang, Lu, Ma, Yang, Huang, Peng, Zeng and Zeng. This is an open-access article distributed under the terms of the Creative Commons Attribution License (CC BY). The use, distribution or reproduction in other forums is permitted, provided the original author(s) and the copyright owner(s) are credited and that the original publication in this journal is cited, in accordance with accepted academic practice. No use, distribution or reproduction is permitted which does not comply with these terms.
*Correspondence: Zhenling Zeng, zlzeng@scau.edu.cn; Dongping Zeng, donytsang@scau.edu.cn
†These authors have contributed equally to this work