Clinical validation of circulating immune complexes for use as a diagnostic marker of canine leishmaniosis
- 1Department of Animal Health, Faculty of Veterinary, Universidad Complutense de Madrid, Madrid, Spain
- 2R&D Unit Allergy and Immunology, LETI Pharma S.L.U., Madrid, Spain
- 3Small Animal Emergency and ICU Service, Veterinary Teaching Hospital, Faculty of Veterinary, Universidad Complutense de Madrid, Madrid, Spain
Introduction: Canine leishmaniosis (CanL) is a systemic disease that affects dogs. When multiplication of the parasite cannot be controlled, dogs consistently show high levels of antigen and IgG antibodies, which lead to the formation of circulating immune complexes (CIC). Timely intervention to reduce the parasite load and CIC levels is crucial for preventing irreversible organ damage. However, a diagnostic test to quantify CIC levels is currently lacking.
Methods: In this real-world study, we aimed to examine the performance of a new ELISA to measure CIC levels in dogs naturally infected with Leishmania infantum. Thirty-four dogs were treated according to their clinical condition and followed for 360 days. Before (day 0) and after treatment (days 30, 90, 180, 270, and 360), all dogs underwent a physical examination, and blood samples were obtained for CBC, biochemical profile, serum protein electrophoresis and IFAT. Serum PEG-precipitated CIC were determined by ELISA.
Results: Our results indicate higher CIC levels in dogs in advanced disease stages showing higher antibody titres (p < 0.0001, r = 0.735), anemia (p < 0.0001), dysproteinemia (p < 0.0001), and proteinuria (p = 0.004). Importantly, dogs responding well to treatment exhibited declining CIC levels (p < 0.0001), while in poor responders and those experiencing relapses, CIC were consistently elevated. CIC emerged as a robust discriminator of relapse, with an area under the curve (AUC) of 0.808. The optimal cut-off to accurately identify relapse was an optical density of 1.539.
Discussion: Our findings suggest that declining CIC levels should be expected in dogs showing a favorable treatment response. Conversely, in dogs displaying a poor response and recurrent clinical relapses, CIC levels will be high, emphasizing the need for vigilant monitoring. These findings suggest that CIC could serve as a valuable biomarker for disease progression, treatment efficacy, and relapse detection in CanL. Our study contributes to enhancing diagnostic approaches for CanL and underscores the potential of CIC as a complementary tool in veterinary practice. As we move forward, larger studies will be essential to confirm these findings and establish definitive cut-offs for clinical application.
1 Introduction
Canine leishmaniosis (CanL), a life-threatening zoonotic disease caused by an obligate intracellular protozoan Leishmania infantum, is transmitted to humans and other animals by blood-sucking phlebotomine sand flies. Dogs are considered the main peridomestic reservoir of infection (1, 2).
Leishmania may infect any organ, tissue or biological fluid, determining that clinical signs of CanL are non-specific and include lethargy, loss of appetite, weight loss, cutaneous lesions, onychogryphosis, generalized lymphadenomegaly, polyuria and polydipsia, ocular lesions, epistaxis, lameness, and other less common clinical signs like vomiting and diarrhea (3). However, many dogs show no apparent clinical signs or clinical-pathological abnormalities for several months or years after infection is confirmed. These dogs are defined as clinically healthy infected dogs. As for sick dogs, a classification system proposed by the LeishVet group helps guide practitioners in the clinical management of these animals and offers prognostic information (3). In this system, four clinical stages are described according to disease severity based on serological status, clinical signs, and laboratory findings.
After infection, clinically healthy infected and sick dogs show different immune responses to the parasite. Healthy dogs mount a robust Th1 response, which is crucial to halt parasite multiplication and for intracellular parasite elimination. In contrast, sick dogs mostly show a Th2 response, which leads to immune suppression and parasite proliferation (4, 5). In these animals, Leishmania antigens and non-specific IgG antibodies gradually build-up in the bloodstream as the parasite multiplies, leading to the formation of circulating immune complexes (CIC). These large molecules are deposited in the endothelium of several organs including the skin, eyes, joints, and renal glomeruli, and cause severe, often irreversible, organ damage. When this occurs, dogs can develop immune-mediated polyarthritis, vasculitis, indolent ulcers, and chronic uveitis, which can lead to blindness requiring the enucleation of one or both eyes. In LeishVet stages III or IV, CIC deposition in renal glomeruli can lead to chronic kidney disease (CKD) with life-threatening consequences (6–8).
The use of antimonials or miltefosine in combination with allopurinol is the standard treatment for CanL. In some cases, steroids are also needed to control immune-mediated clinical signs (9, 10). Prompt treatment is vital to reduce the parasite load and CIC levels, and thus mitigate the risk of irreversible organ damage. However, a diagnostic test to quantify CIC levels before and after treatment is currently lacking. Parody et al. (11) developed a method to quantify serum levels of CIC in dogs with CanL that was recently laboratory validated (12). In their work, Parody et al. (11) confirmed a clear association between disease progression and increasing CIC levels, suggesting that CIC could serve as a valuable biomarker for diagnostic purposes and also to track disease progression and assess treatment efficacy (13). Validating these findings in a real-world study is the first step toward making this method available to practitioners. The aim of the present study was to examine the performance of this new biomarker in a clinical setting by measuring CIC levels in dogs naturally infected with L. infantum.
2 Materials and methods
2.1 Study design
This longitudinal cohort study was conducted at the Infectious Diseases Unit of the Veterinary Teaching Hospital, Universidad Complutense de Madrid (UCM), Madrid, Spain. The study protocol was approved by this University’s Research Ethics Committee. Participants were dogs brought to the hospital by their owners over the period May 2021 to April 2022 for evaluation after a first diagnosis of CanL, or follow-up for check-ups or due to a clinical relapse. Dogs were considered eligible if naturally infected with L. infantum [positive serology for Leishmania by indirect fluorescent antibody test (IFAT >1:100, positive cytology and/or positive PCR result)] and their disease was then graded as LeishVet stages I–IV. Before enrolment, we informed all dog owners about the study protocol, including the option to withdraw their dogs from the study at any time, and obtained their written consent.
2.2 Study protocol
Dogs were included in the study on day 0 (Visit 1 enrolment pre-treatment) and thereafter followed for 12 months. Appropriate treatment was started shortly after enrolment. Visits to our service were then scheduled for post-treatment onset days 30, 90, 180, 270, and 360 (Figure 1).
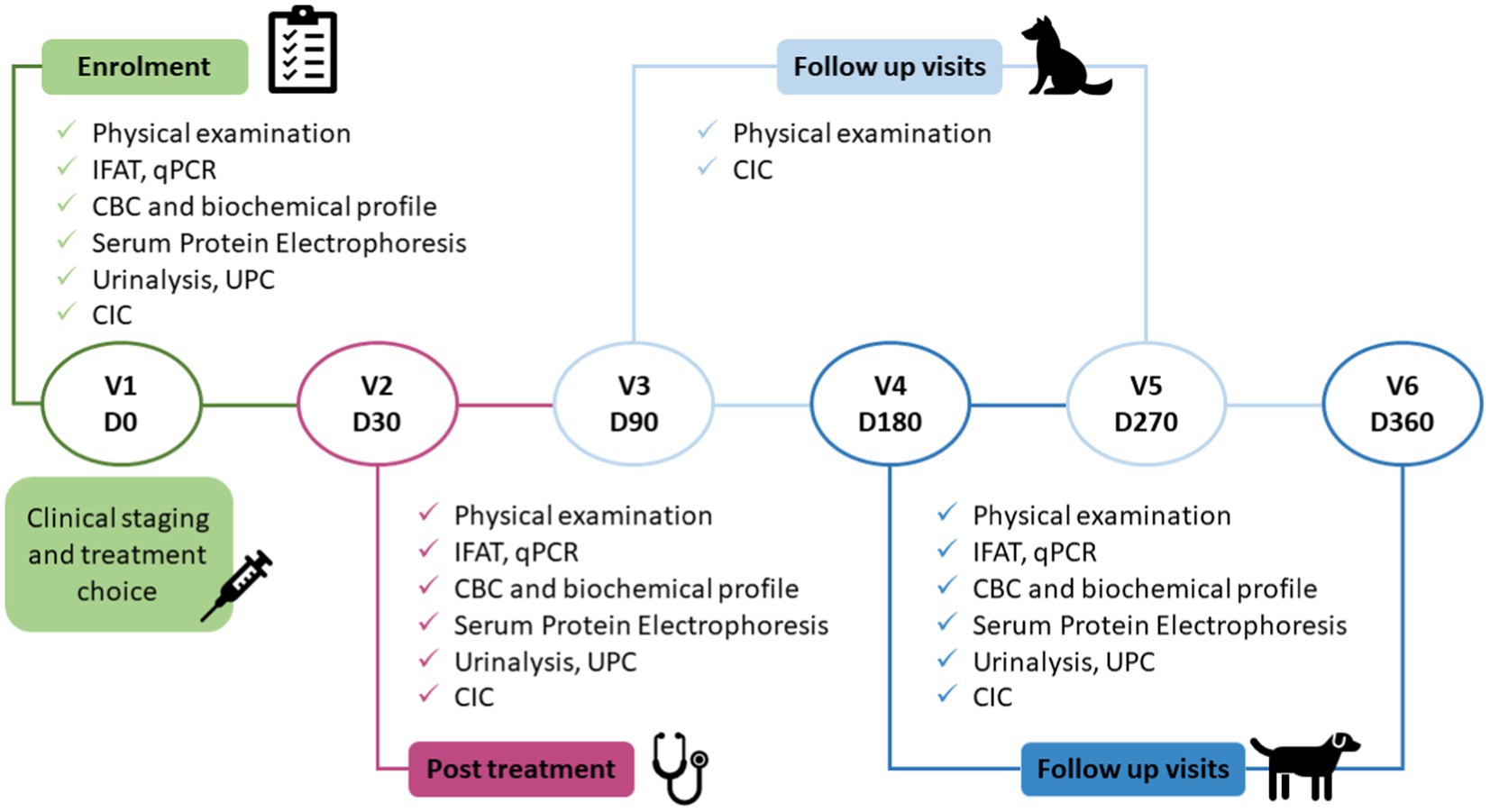
Figure 1. Study protocol. The clinical study consisted of six visits, enrolment (pre-treatment), after completing 30 days of treatment, and four follow-up visits. IFAT, indirect fluorescent antibody test; CBC, complete blood count; UPC, urinary protein/creatinine ratio; CIC, circulating immune complexes; qPCR, quantitative polymerase chain reaction.
Dogs underwent a thorough physical examination in each visit. Data were compiled on clinical signs including signs of CIC deposition (Figure 2). Each clinical sign was graded from 0 to 3 (low to high severity) to give an overall clinical score for each animal (maximum 68) (Table 1).
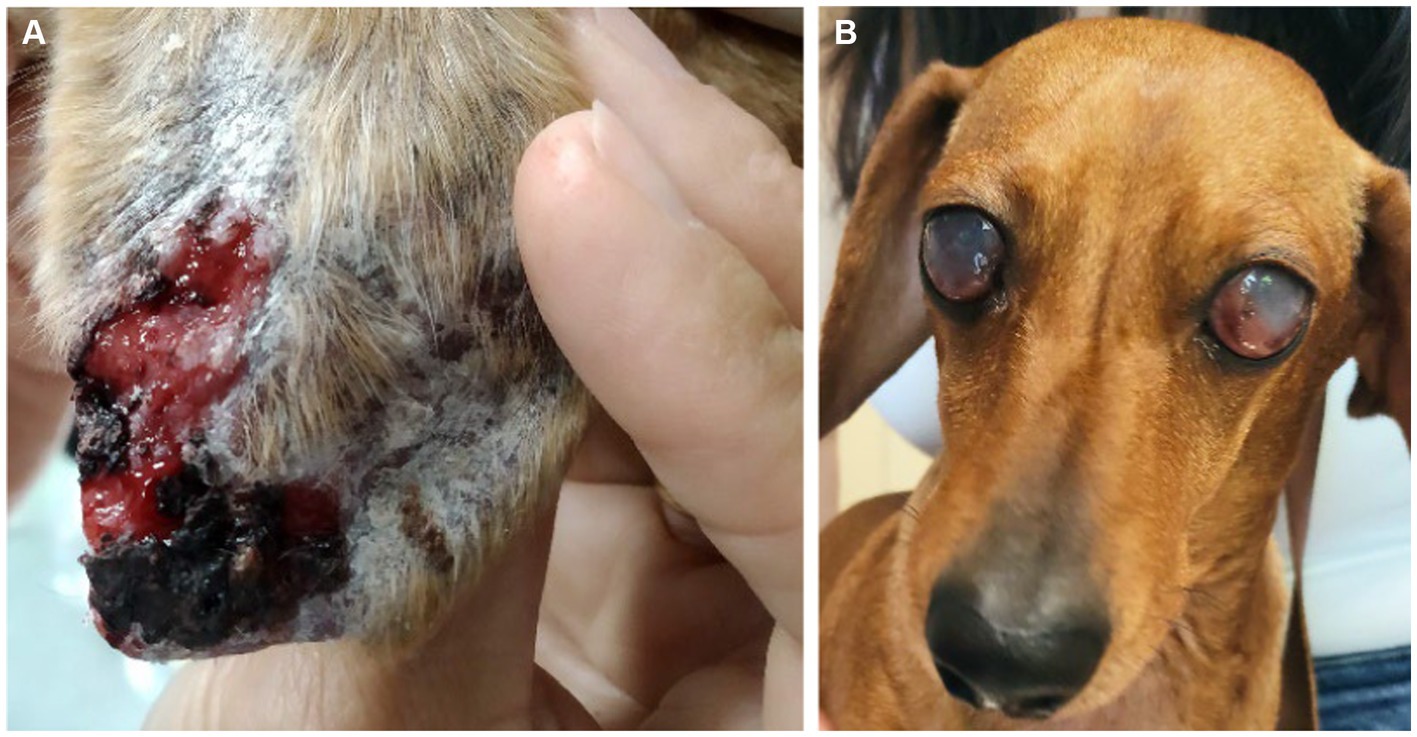
Figure 2. Clinical signs of CIC deposition in dogs with CanL. (A) Ulcer in the pinna associated with vasculitis. (B) Blindness associated with bilateral uveitis.
At each visit, blood samples were collected to determine serum CIC concentrations by PEG ELISA (11) (Figure 1). On days 0, 30, 180, and 360, routine laboratory tests were also performed to determine the clinical condition of the dog, which included complete blood count (CBC), biochemical profile (creatinine, urea, and alanine aminotransferase), serum protein electrophoresis, and anti-Leishmania immunofluorescence antibody test (IFAT) (15). Serial dilutions from 1:50 to 1:6400 were used and seropositivity defined by a cut-off ≥1:200. Urinalysis, including urine density, sediment analysis and protein/creatinine ratio (UPC), were also undertaken on days 0, 30, 180, and 360 on urine samples obtained preferably by cystocentesis, or by free catch into a sterile container. Quantitative PCR was also performed to confirm the infection on day 0, and to quantify parasite load at 6 and 12 months post enrolment. Based on physical examination and clinical-pathological findings, dogs were assigned to LeishVet clinical disease stages I–IV (3).
2.3 CIC isolation and quantification
A modified precipitation method with polyethylene glycol (PEG; Sigma-Aldrich, St. Louis, MO, United States) was used to separate free antigens and antibodies from CIC (11). PEG-precipitated CIC were pelleted by centrifugation, then reconstituted in 0.01 M phosphate-buffered saline (PBS) and stored at −80°C for further use. CIC were quantified using a Leishmania-specific ELISA method as described by Parody et al. (11). All samples were tested in duplicate, and the mean value was recorded. A mean OD of 0.274 + 3 standard deviations of negative samples was considered as the cut-off value; any sample exhibiting absorbance above the cut-off value was considered positive.
2.4 Treatment
The treatment for each dog was determined by one of the authors (a veterinarian specialist in infectious diseases) and was based on clinical history, physical examination, and laboratory findings. Clinically healthy infected dogs or those at stage I received no treatment or received only allopurinol for 6–12 months. Dogs in stages II or III were treated with a combination of meglumine antimoniate (MGA) (50 mg/kg subcutaneously q12h for 28 days) and allopurinol (10 mg/kg orally q12h for 6–12 months). When considered necessary, prednisone (0.5 mg/kg orally q12h for 3–4 weeks) was added to the treatment regimen in dogs in stage III to control signs of CIC deposition (10). Proteinuric dogs were treated following International Renal Interest Society Guidelines for CKD in dogs (16).
Relapses were also recorded and a second course of leishmanicide was administered if needed. Disease relapse was defined as significant clinical worsening and/or laboratory abnormalities prompting a new course of treatment with antimonials.
The clinical response to treatment was assessed by examining clinical records, changes produced in clinical scores and laboratory variables over time, and the number of relapses produced during the study period. Good responders were dogs that showed improvement in clinical signs and laboratory abnormalities and no relapses in the 12 months following treatment onset. Dogs were considered poor responders if they experienced one or more relapses during the study or showed no improvement in clinical-pathological variables by the end of the study.
2.5 Statistical analysis
All statistical tests were performed using the software packages SAS (SAS Institute Inc., Cary, NC, United States) version 9.4, and SPSS Statistics version 28 (SPSS Inc., Chicago, IL, United States). Graphs were created using GraphPad Prism 10. Significance was set at p ≤ 0.05.
Some variables were categorized for analysis: age (2–4 years: young adult, 5–8 years: mature adult, ≥9 years: senior) (17), IFAT titer according to cut-off values established by the laboratory (<200 negative, 200–400 low positive, 800–1,600 medium positive, >1,600 high positive) (3, 15, 18, 19), and albumin/globulin ratio (A/G) (>0.8 normal, 0.7–0.8 mild dysproteinemia, 0.5–0.69 moderate dysproteinemia, <0.5 severe dysproteinemia) (19–21).
As CIC values within groups were not normally distributed (Kolmogorov–Smirnov test, p < 0.001), non-parametric tests were used. The Wilcoxon test was used to compare categorical variables between two established groups and the Kruskal–Wallis test with Bonferroni correction when there were more than two groups. The association between CIC levels and the clinical signs from the scoring system used in this study was evaluated using the Chi-Square test, specifically analyzing the 2 × 2 contingency table formed by the variables. From this analysis, relative risks (RR) for each clinical sign were calculated, accompanied by their respective 95% confidence intervals (CI). These measures serve to quantify the strength and direction of the association between CIC and the clinical signs, providing valuable insights into their relationship.
To assess changes in CIC levels over time, we used the Friedman test for repeated measures also with Bonferroni correction. Spearman’s correlation test was used to examine possible correlations between CIC levels and continuous variables. We also assessed the capacity of CIC level to accurately identify relapse by calculating the area under the receiver operating characteristic (ROC) curve (AUC).
3 Results
3.1 Study population
Of the 44 dogs enrolled, 23 were male and 21 female. Fourteen dogs were classified as young adults (2–4 years), 18 as mature adults (5–8 years), and 11 as senior (mean age 6.6 years; 95% CI: 1.6–14.64). During the study, three dogs died of a comorbidity (2 lymphoma, 1 carcinoma), and seven were lost to follow-up as owners failed to return for the re-visits. Even though only 34 dogs completed the 12 months of the study, data for all 44 dogs enrolled were included in the analysis until the loss of follow-up.
The CIC levels were significantly higher in young adult dogs compared to senior (p < 0.001), and low negative correlation was found between CIC level and age (r = −0.273). No differences in CIC levels were detected between mongrels and pure breeds (p = 0.107) or between male and female dogs (p = 0.416).
3.2 CIC and clinical scores, and LeishVet stages
The mean clinical score awarded to the 34 dogs that completed the 12 months of follow up gradually decreased throughout the study (Figure 3), and clinical scores and CIC levels showed positive correlation (Figure 3) (r = 0.5135, p < 0.0001). Dogs showing clinical signs were also found to have a greater risk of having elevated CIC levels. Table 2 displays the relative risks (RR) for various clinical signs evaluated in association with the presence of CIC in dogs. Interestingly, 10/44 dogs (22.72%) had a clinical score of five or less, but high CIC levels (≥2 OD).
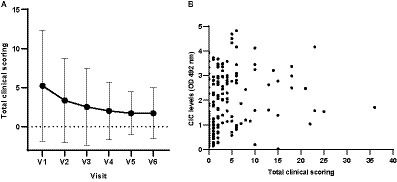
Figure 3. (A) Means and SDs of total clinical scores recorded in each visit. (B) Correlation between total clinical scores and CIC levels (Spearman correlation, r = 0.5135). V1 (Day 0), V2 (Day 30), V3 (Day 90), V4 (Day 180), V5 (Day 270), and V6 (Day 360).
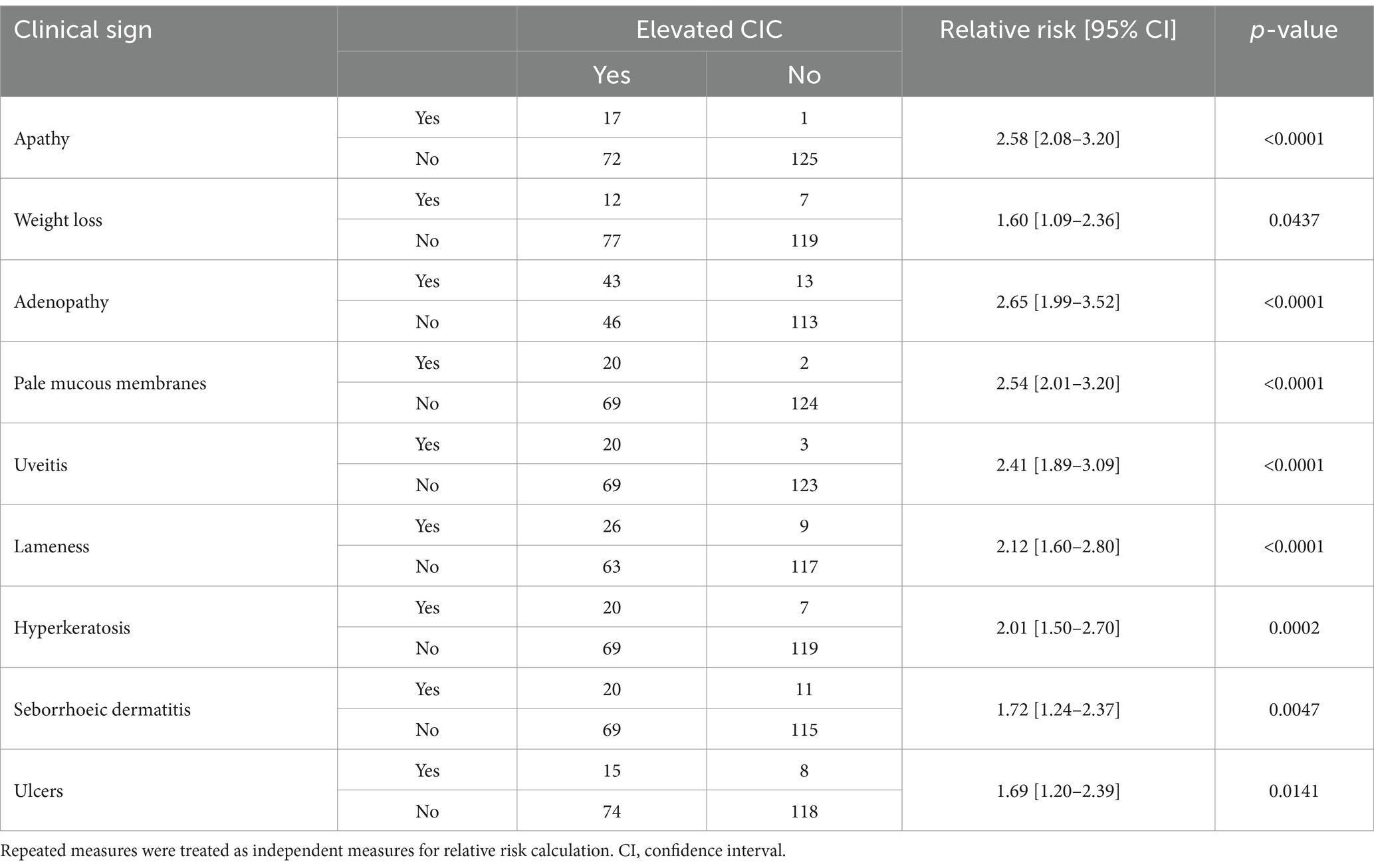
Table 2. Relative risk of clinical signs associated with a significant risk of elevated circulating immune complexes (CIC).
At enrolment, 15 dogs were classified as LeishVet stage I, 20 as stage II, 7 as stage III and 2 as stage IV. Owing to the small number of animals at stage IV, data for stages III and IV were combined for analysis. Dogs at stage I showed significantly lower CIC levels than those classified as having CanL stages II and III (p < 0.0001) (Figure 4). No differences were found between dogs staged as II or III (p = 0.485). Moreover, stage I dogs consistently maintained low CIC levels throughout the study (Figure 4). In stage II patients, CIC levels decreased after treatment and remained stable, whereas in stage III patients, they increased after 90 and 360 days of treatment (Figure 4).
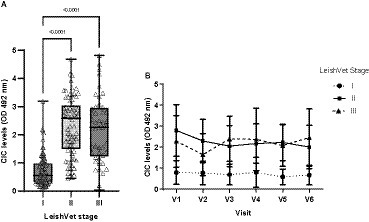
Figure 4. (A) CIC levels recorded in the dogs assigned to different LeishVet stages (non-parametric Kruskal–Wallis test). (B) Changes in mean CIC levels recorded over the 12-month study period in dogs at different LeishVet stages. V1 (Day 0), V2 (Day 30), V3 (Day 90), V4 (Day 180), V5 (Day 270), and V6 (Day 360).
3.3 CIC and laboratory findings
Leukopenia was the most frequent abnormality detected in the CBC, occurring in 27.78% of dogs, followed by thrombocytopenia (22.62%) and non-regenerative anemia (18.23%). However, significant differences in CIC levels were only found for anemic dogs (p < 0.0001) (Figure 5), which not only had higher CIC levels but also experienced a gradual increase in these levels as early as 6 months after treatment cessation (Figure 5). Finally, we observed moderate negative correlation between hematocrit and CIC level (r = −0.547).
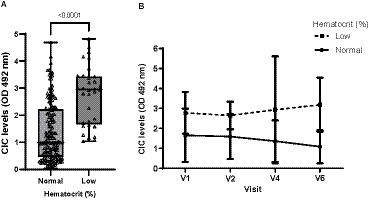
Figure 5. (A) CIC levels recorded in dogs with or without anemia (non-parametric Wilcoxon test). (B) Changes in CIC means produced during the study in dogs with or without anemia. Reference value hematocrit: 37–55%. V1 (Day 0), V2 (Day 30), V3 (Day 90), V4 (Day 180), V5 (Day 270), and V6 (Day 360).
Among the biochemical variables measured, creatinine was found elevated in only 8.57% of dogs, whereas alanine aminotransferase (ALT) was increased in 11.49%. No significant differences were found in CIC levels between dogs with normal or elevated creatinine (p = 0.789, r = −0.327) or ALT (p = 0.434, r = −0.216) levels.
Hyperproteinemia was detected in 37.22% of visits. Dogs displaying hyperproteinemia had significantly higher CIC levels (p < 0.0001) (Figure 6). At visit 3, which was the first follow up visit after the end of treatment, both groups showed reduced CIC levels. However, in dogs with dysproteinemia, these levels subsequently increased, while dogs without dysproteinemia consistently maintained lower CIC levels (Figure 6). Additionally, we found moderate positive correlation between CIC and total protein levels (r = 0.533, p < 0.0001).
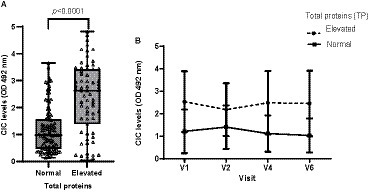
Figure 6. (A) CIC levels recorded in dogs with or without hyperproteinemia (non-parametric Wilcoxon test). (B) Changes in mean CIC levels produced during the study in dogs with or without hyperproteinemia. Normal reference value total proteins: 5,8–7,5 g/dL. Frequencies: Normal: 57.78%; hyperproteinemia: 37.22%. V1 (Day 0), V2 (Day 30), V4 (Day 180), and V6 (Day 360).
Hypoalbuminemia was present in 22.02% of patients. Dogs with hypoalbuminemia displayed significantly more elevated CIC levels (p = 0.02) (Figure 7), and low negative correlation was found between CIC and albumin levels (r = −0.306).
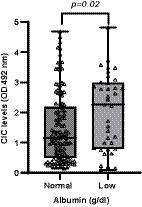
Figure 7. CIC levels recorded in dogs according to albumin level (non-parametric Wilcoxon test). Normal reference value albumin: 2.4–3.9 g/dL.
Among the globulins, gamma globulin was the most affected with hypergammaglobulinemia present in 31.1% of animals, followed by hyperbetaglobulinemia (9.82%). Dogs with these abnormalities showed significantly higher CIC levels (p < 0.0001) (Figure 8), and we found positive correlation between CIC and beta (r = 0.444) and gamma (r = 0.720) globulin levels. Elevated alpha-1 and alpha-2 globulins were noted in 2.48 and 8.18% of dogs, respectively, but no differences in CIC levels were detected between dogs with normal or high blood alpha-1 globulins (p = 0.960). Dogs with elevated alpha-2 globulins had higher CIC levels (p = 0.022). No correlation was found between CIC levels and alpha-1 and alpha-2 globulins (r = 0.021; r = −0.022, respectively).
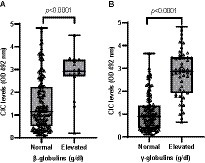
Figure 8. (A) CIC levels recorded in dogs according to the presence of beta-or (B) gamma-hyperglobulinemia (non-parametric Wilcoxon test). Normal reference values beta-globulins: 1.3–2.7 g/dL; gamma-globulins: 0.5–2 g/dL.
Among all variables examined, dysproteinemia was the most common laboratory finding, detected in 41.66% of animals. Dogs with dysproteinemia showed significantly higher CIC levels (p < 0.0001), and high negative correlation was found between CIC level and the A/G ratio (r = −0.618). When we assessed the effect of the level of dysproteinemia on CIC level (Figure 9), we found no differences between dogs with mild (p = 1.0), moderate (p = 0.419) or severe (p = 0.121) dysproteinemia. Throughout the study, CIC levels remained below 1.5 OD in dogs without dysproteinemia, while levels were consistently over 2.5 OD in those with severe dysproteinemia (Figure 9).
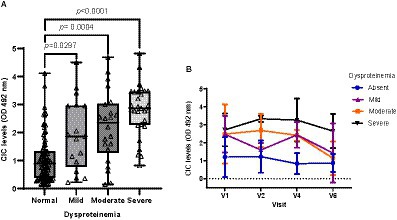
Figure 9. (A) CIC levels in dogs with a normal A/G ratio and different degrees of dysproteinemia (non-parametric Kruskal–Wallis test). (B) Changes in CIC means detected in dogs with a normal A/G ratio and different degrees of dysproteinemia. Normal A/G ratio > 0.8; mild dysproteinemia: 0.7–0.8; moderate dysproteinemia: 0.6–0.5; severe dysproteinemia: A/G < 0.5. V1 (Day 0), V2 (Day 30), V4 (Day 180), and V6 (Day 360).
A large proportion (36.18%) of dogs showed a normal UPC ratio. Borderline proteinuria and proteinuria were recorded in 26.32 and 36.18% of the patients, respectively. Dogs with borderline proteinuria (UPC = 0.2–0.5) had higher CIC levels than dogs without proteinuria (UPC < 0.5) (p = 0.035, r = 0.172). However, no differences in CIC were found between dogs with borderline proteinuria or proteinuria (p = 0.69).
3.4 CIC and IFAT titres
The IFAT titres ranged from negative to 1:6400, with the cut-off set at >1/100. Significant differences in CIC levels were observed among groups showing negative, low, medium, or high antibody titres (p < 0.0001) (Figure 10), and there was strong positive correlation between CIC levels and IFAT titres (r = 0.735; p < 0.0001). Further, throughout the study, CIC levels remained consistently low in clinically healthy infected dogs returning negative IFAT results (Figure 10).
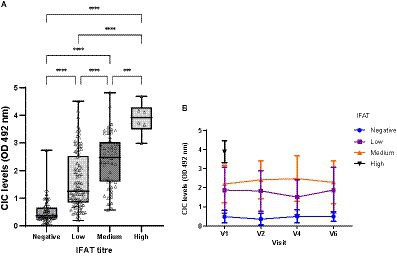
Figure 10. (A) CIC levels recorded in dogs showing different titres of antibodies against L. infantum. IFAT < 200 negative, 200–400 low, 800–1600 medium, >1600 high (non-parametric Kruskal–Wallis test; ***p < 0.0005, ****p < 0.0001). (B) Changes in CIC means produced during the study in dogs showing different antibody titres against L. infantum. High titres are not displayed due to the low number detected. V1 (Day 0), V2 (Day 30), V4 (Day 180), and V6 (Day 360).
3.5 CIC and treatment
As CanL is a chronic disease, at the time of enrolment some dogs were already receiving treatment with allopurinol (n = 15/44), domperidone (n = 1/44) (22–24), nucleotides (n = 5/44) (25–28), prednisone (n = 1/44) or the angiotensin-converting enzyme inhibitor benazepril (n = 2/44). The treatment regimens of the dogs after enrolment in this study are detailed in Table 3.
The CIC levels were significantly higher in dogs treated with meglumine antimoniate (p = 0.0036) or allopurinol (p = 0.015) and in those with proteinuria treated with benazepril (p = 0.0039). Dogs treated with meglumine antimoniate or allopurinol or that received benazepril to control proteinuria were almost two times more likely to have high CIC levels (Table 4). In contrast, no differences were detected according to treatment with nucleotides (p = 0.0519) or prednisone (p = 0.2312).
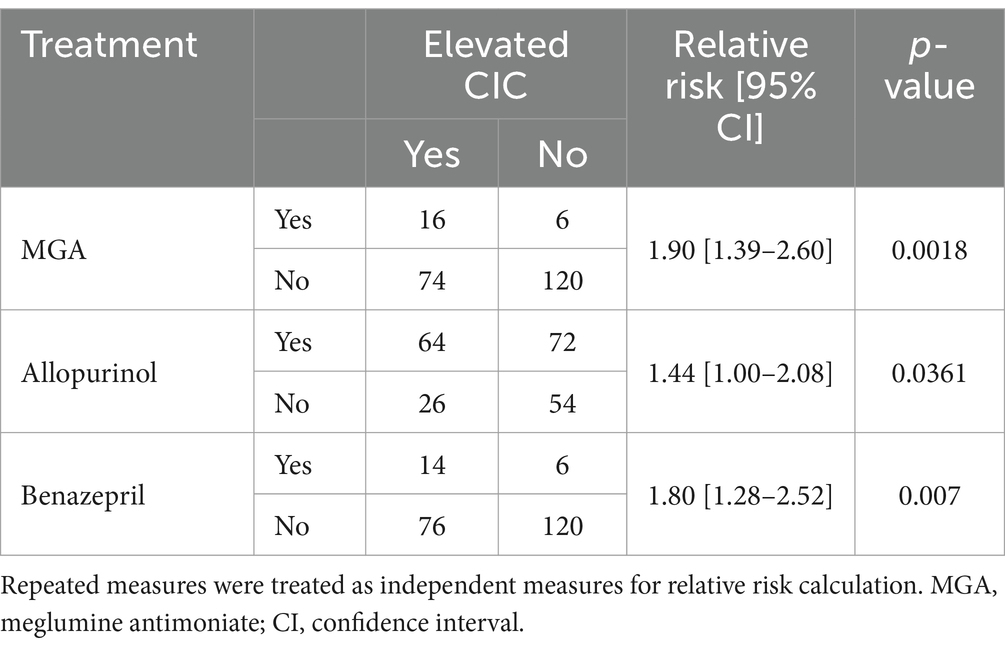
Table 4. Relative risk of treatment regimens associated with a significant risk of elevated circulating immune complexes (CIC).
3.6 CIC and treatment response
To assess the impacts of treatment in sick dogs, we also examined CIC levels over time. As the data were not normally distributed, we employed the non-parametric Friedman test. Nine of the 44 dogs, each missing one visit, were excluded from this part of the analysis. Our results show that mean CIC levels gradually decreased after treatment and remained relatively stable throughout the study period (Figure 11). Nevertheless, significant differences in CIC levels were only detected between the first (D0) and last visit (D360) (Friedman test p = 0.022) (Figure 11).
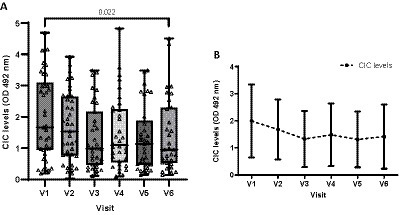
Figure 11. Box chart (A) and (B) line graph displaying changes in mean CIC levels recorded during the study. (A) Non-parametric Friedman test.
Of the 44 dogs initially included in our study, 28 were classified as good responders, 11 as poor responders and 5 could not be classified, as they were lost to follow-up. Good responders showed significantly lower CIC levels than poor responders (p < 0.0001) (Figure 12). Moreover, good responders showed lower CIC levels and they declined throughout the study (Figure 12). In poor responders, while CIC reduction was observed after treatment, levels progressively increased as early as 90 days later (Figure 12).
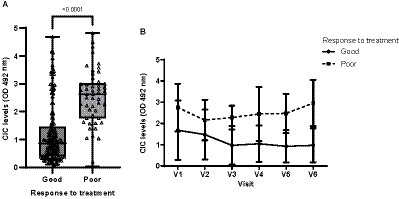
Figure 12. (A) CIC levels recorded in dogs showing a good or poor response to treatment (non-parametric Wilcoxon test). (B) Changes in CIC means produced during the study in dogs showing a good or poor response to treatment. V1 (Day 0), V2 (Day 30), V3 (Day 90), V4 (Day 180), V5 (Day 270), and V6 (Day 360).
3.7 CIC and relapses
Relapses were detected in 23.18% of the animals. At inclusion, 18 of the 44 dogs had had a previous diagnosis of CanL and were referred for treatment due to clinical relapse. Over the 12-month follow-up period, six dogs had one relapse and two had two relapses (Table 5).
Dogs experiencing relapse had significantly higher CIC levels compared to dogs not experiencing relapse (p < 0.0001) (Figure 13); these were elevated consistently in all visits (OD > 2) (Figure 13).
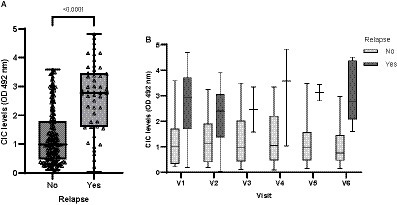
Figure 13. (A) CIC levels recorded in dogs experiencing or not experiencing relapse (non-parametric Wilcoxon test). (B) CIC levels recorded over the study period in dogs experiencing or not experiencing relapse. V1 (Day 0), V2 (Day 30), V3 (Day 90), V4 (Day 180), V5 (Day 270), and V6 (Day 360).
Interestingly, we detected markedly increased CIC levels in V4 (D180) (Figure 14), persisting into V5 (D270) and V6 (D360) and coinciding with the relapses recorded in these dogs (Table 5). In contrast, dogs not relapsing had CIC levels below 2 OD during the entire study period (Figure 14).
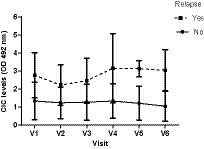
Figure 14. Changes in CIC means recorded over the study in dogs experiencing or not experiencing relapse. V1 (Day 0), V2 (Day 30), V3 (Day 90), V4 (Day 180), V5 (Day 270), and V6 (Day 360).
Importantly, through ROC curve analysis we were able to determine that CIC was a good discriminator of relapse (AUC = 0.808, p < 0.0001; 95% CI: 0.736–0.881) (Figure 15). Our results indicate that the optimal cut-off to correctly identify a relapse was 1.539 OD (sensitivity 82.4%; specificity 70.9%).
4 Discussion
To our knowledge, this is the first study to clinically validate the use of CICs as biomarkers of the progression of canine leishmaniosis. Dogs in advanced stages of this disease show higher CIC levels (11) and a higher risk of multiple organ damage, which can significantly impair quality of life and dramatically reduce survival (6–8, 29). Currently, diagnostic methods for quantifying CIC are lacking. Parody et al. (11) introduced a non-invasive Leishmania-specific method of measuring CIC levels in serum samples from dogs with leishmaniosis. This method was recently laboratory validated following the recommendations of the NIH Biomarkers group (12). The method’s specificity and robustness suggest this new biomarker could be useful not only for a diagnosis of CanL but also for tracking disease progression and potentially assessing treatment efficacy (13). In the present real-world study, we sought to clinically validate the performance of this new biomarker.
In our study, 34 dogs naturally infected with L. infantum at different LeishVet stages were followed for 12 months. Our data show that CIC levels are significantly higher in young adult dogs than senior dogs (p < 0.001) while no differences were detected according to sex or breed. The influence of sex, breed, and age on susceptibility to acquiring the infection has been explored in numerous studies with contrasting results (30–34). Nonetheless, the authors of two epidemiologic studies involving a large number of dogs, which took into account the presence of clinical signs of CanL, described that the age distribution for affected animals was bimodal, with a first peak corresponding to animals of around 3 years of age and a second, less evident, peak representing animals around 8 years old (30, 31). Age also seems to be an important risk factor associated with visceral leishmaniasis (VL) in humans according to two systematic reviews (35, 36) such that children are more susceptible to both infection and illness. Our results point to younger dogs controlling the disease less effectively and thus being more likely to have formed CIC.
Mean clinical scores progressively declined during the study and significant differences were detected in total clinical scores between visits. Moreover, we found positive correlation between clinical score and CIC levels (r = 0.5135, p < 0.0001). These results suggest that a decrease in clinical score will be accompanied by a drop in CIC levels. Nevertheless, some dogs had a low clinical score (≤5) despite having high levels of CIC (≥2 OD). The only clinical signs observed in these dogs were lameness, uveitis, skin ulcers, or polyuria/polydipsia. Hence, measuring CIC levels could be useful in dogs that do not have an overall picture of generalized CanL yet show clinical signs of immune complex deposition. Future studies will bring light to the relationship between clinical scores and CIC levels.
The CIC levels were significantly lower in stage I dogs compared to stages II and III, consistent with the findings of Parody et al. (11), who reported a clear association between CIC levels and disease progression. In our study, we examined trends in CIC levels over 12 months in dogs at different clinical stages of CanL. Our results indicate that dogs in early disease stages have low CIC levels that remain low for long periods. Conversely, in advanced stages, dogs consistently show high levels of immune complexes, and increases may be observed as early as 90 days after initiating specific treatment. These results highlight the importance of close and frequent monitoring of CIC deposition in dogs in advanced stages of CanL, and suggest this new biomarker could be used to detect relapses.
Non-regenerative anemia is one of the most frequent clinical-pathological abnormalities reported in dogs with CanL. Some authors describe correlation between anemia or the severity of clinical signs (21, 37, 38) and parasite load (38–40). Dysproteinemia is also frequently observed in dogs with CanL (19–21). Protein electrophoresis has proven extremely useful for the diagnosis and monitoring of CanL and is routinely performed in clinical practice (41–44). Indeed, several studies have shown that this technique may show abnormalities very early during the course of disease, even before the onset of overt clinical signs (21, 41, 42, 45, 46). In our study, we observed strong correlation between CIC and hematocrit (r = 0.524), total proteins (r = 0.507), beta- (r = 0.426) and gamma globulins (r = 0.673), and the A/G ratio (r = −0.647). Further, our results also show that dogs with non-regenerative anemia and/or dysproteinemia have significantly higher CIC levels. When we examined the kinetics of these immune complexes, we noted that dogs with anemia and/or dysproteinemia showed higher CIC levels throughout the study despite specific treatment. Taken together, our findings point to the high reliability of this new biomarker, as it correlates with other tools used for the diagnosis and clinical management of CanL. Moreover, they confirm that dogs with anemia or dysproteinemia are at a higher risk of CIC deposition in specific organs.
The IFAT is one of the most used quantitative serologic techniques for the detection of anti-Leishmania antibodies and is considered the gold standard test for Leishmania infection. Extensive research has identified correlation between IFAT results and clinical signs (47–51), becoming more evident in severe clinical forms of CanL (51). In human VL, high anti-Leishmania antibodies also correlate with disease progression (4). Studies have also shown that IgG antibodies not only fail to protect against this intracellular parasite but also contribute to disease progression by reversing the inflammatory cytokine profile of immune cells and inducing the production of high IL-10 levels via the receptor FcγRIII (4, 52). Here, significant differences in CIC levels were found among dogs showing negative, low, medium or high antibody titres, and high positive correlation was observed between CIC and IFAT results (r = 0.735). These findings are in line with those described by Parody et al. (11), who also noted high correlation between these two variables (r = 0.754). Thus, dogs with high antibody titres have significantly higher CIC levels and are thus at greater risk of their deposition in target organs.
Mean CIC levels dropped after treatment and remained under 1.5 OD throughout the study, although significant differences were only found between the first and the last visits. Likely reasons for this are: (1) the small number of animals included in the study, and thus reduced statistical power when using the Friedman test with Bonferroni correction; and (2) in dogs showing a poor response to treatment, CIC levels can remain elevated for several months. In this study, sick dogs were treated with antimonials in combination with allopurinol, which is the standard treatment for CanL in Europe (3). Four dogs treated with antimonials, and one treated with allopurinol also received prednisone for 1 month to control clinical signs of CIC deposition. Dogs treated with antimonials or allopurinol, or those that received benazepril to control proteinuria, had significantly higher CIC levels and were up to two times more likely to have elevated CIC levels in serum. This is not surprising as, except for allopurinol, these medications are used in more advanced stages of the disease. Due to the small number of animals that received treatment with prednisone, no conclusions can be made regarding the kinetics of CIC levels in these dogs. Further studies are needed to examine the optimal use of this new biomarker in tailoring and monitoring corticosteroid use in dogs experiencing CIC deposition.
Good responders had significantly lower CIC levels compared to poor responders (p < 0.0001), which progressively decreased over the study course. In poor responders, although a CIC reduction was observed after treatment, levels progressively increased as early as 90 days post-treatment. These results suggest that measuring CIC levels before and after treatment could serve to assess treatment effectiveness.
Dogs experiencing relapse after treatment had significantly higher CIC levels (p < 0.0001); over 2 OD in all visits. During the study, six dogs had one relapse and two had two relapses. Most relapses occurred in visit 6, 1 year after treatment. Nevertheless, three dogs had a relapse as early as at 6 months post-treatment. Studies have shown that the presence of immune complexes contributes to the establishment of chronic infections in murine models and human VL (4, 52). One key mechanism is their ability to stimulate macrophages and other immune cells to produce IL10. This cytokine reduces the expression of inducible nitric oxide synthase (iNOS) and the intracellular production of nitric oxide, which are both vital for eliminating intracellular Leishmania parasites. Moreover, IL-10 downregulates the Th1-associated IFN-γ response, which is essential to activate nitric oxide production within infected cells and eliminate the parasite. Consequently, dogs that relapsed had higher CIC levels, which may have been responsible for their inability to control the infection despite receiving a specific leishmanicidal treatment.
Importantly, our ROC curve analysis revealed that CIC were a good discriminator of relapse (AUC = 0.808) and that the optimal cut-off for accurately identifying relapse was 1.539 OD. This is relevant as the cut-off for positivity adopted in the study by Parody et al. (11) was 0.274 OD, which may be too low to be associated with clinical disease. In fact, CIC levels ranged between 0.8 and 1.0 OD in clinically healthy infected dogs, while sick dogs had levels between 1.9 and 3.1 OD. Further larger studies are needed to confirm our findings and establish definitive cut-offs to distinguish between healthy infected and sick dogs.
It is important to mention that our study did not include a formal sample size calculation due to the lack of prior data concerning expected effect sizes for the novel biomarker under investigation. However, our study yielded statistically significant results, providing valuable insights into the association between CIC clinical signs and clinicopathological parameters related to CanL. Moving forward, sample size calculations in future studies could enhance the robustness of our findings and contribute to a deeper understanding of CIC’s role in the progression of the disease.
5 Conclusion
Our findings indicate elevated serum CIC concentrations in both young adult dogs and/or those in advanced stages of CanL with anemia and/or dysproteinemia. Dogs showing a favorable response to treatment showed declining CIC levels. Conversely, dogs displaying a poor treatment response and recurrent clinical relapses consistently exhibited high CIC levels. In these dogs, vigilant monitoring is essential to enable prompt and targeted treatment and prevent irreversible organ damage due to CIC deposition.
While studies in larger populations of dogs are still needed to confirm our findings, the use of CICs as a complementary biomarker to track disease progression in CanL is promising. Further studies using this new biomarker may provide insight into specific organ tropism and relapse mechanisms in non-responders. Such studies will also improve our understanding of long-lasting cell-mediated immunity in resistant dogs, and aid in the development of Leishmania vaccines.
Data availability statement
The raw data supporting the conclusions of this article will be made available by the authors, without undue reservation.
Ethics statement
The animal studies were approved by Ethical committee Universidad Complutense de Madrid. The studies were conducted in accordance with the local legislation and institutional requirements. Written informed consent was obtained from the owners for the participation of their animals in this study.
Author contributions
JS: Data curation, Formal analysis, Investigation, Methodology, Project administration, Resources, Writing – original draft, Writing – review & editing. NP: Investigation, Validation, Writing – review & editing. AM: Resources, Writing – review & editing. CC-L: Investigation, Validation, Writing – review & editing. JB: Resources, Writing – review & editing. RC: Resources, Writing – review & editing. MD: Resources, Writing – review & editing. JC: Conceptualization, Funding acquisition, Project administration, Supervision, Writing – review & editing. GM: Conceptualization, Funding acquisition, Methodology, Project administration, Resources, Supervision, Writing – review & editing.
Funding
The author(s) declare financial support was received for the research, authorship, and/or publication of this article. This study was partially funded by the LETI Pharma S.L.U.
Acknowledgments
The authors would like to thank all the dogs and their owners who participated in this study.
Conflict of interest
NP, CC-L, and JC were employed by the LETI Pharma S.L.U.
The authors declare that this study received funding from LETI Pharma S.L.U. The funder had the following involvement in the study: study protocol design, CIC quantification, and editing of the final manuscript.
The remaining authors declare that the research was conducted in the absence of any commercial or financial relationships that could be construed as a potential conflict of interest.
Publisher’s note
All claims expressed in this article are solely those of the authors and do not necessarily represent those of their affiliated organizations, or those of the publisher, the editors and the reviewers. Any product that may be evaluated in this article, or claim that may be made by its manufacturer, is not guaranteed or endorsed by the publisher.
References
1. Moreno, J, and Alvar, J. Canine leishmaniasis: epidemiological risk and the experimental model. Trends Parasitol. (2002) 18:399–405. doi: 10.1016/S1471-4922(02)02347-4
2. Miró, G, Montoya, A, Mateo, M, Alonso, A, García, S, García, A, et al. A leishmaniosis surveillance system among stray dogs in the region of Madrid: ten years of serodiagnosis (1996–2006). Parasitol Res. (2007) 101:253–7. doi: 10.1007/s00436-007-0497-8
3. Solano-Gallego, L, Koutinas, A, Miró, G, Cardoso, L, Pennisi, MG, Ferrer, L, et al. Directions for the diagnosis, clinical staging, treatment and prevention of canine leishmaniosis. Vet Parasitol. (2009) 165:1–18. doi: 10.1016/j.vetpar.2009.05.022
4. Miles, SA, Conrad, SM, Alves, RG, Jeronimo, SMB, and Mosser, DM. A role for IgG immune complexes during infection with the intracellular pathogen Leishmania. J Exp Med. (2005) 201:747–54. doi: 10.1084/jem.20041470
5. Toepp, AJ, and Petersen, CA. The balancing act: immunology of leishmaniosis. Res Vet Sci. (2020) 130:19–25. doi: 10.1016/j.rvsc.2020.02.004
6. Geisweid, K, Mueller, R, Sauter-Louis, C, and Hartmann, K. Prognostic analytes in dogs with Leishmania infantum infection living in a non-endemic area. Vet Rec. (2012) 171:399. doi: 10.1136/vr.100637
7. Pereira, MA, Santos, R, Oliveira, R, Costa, L, Prata, A, Gonçalves, V, et al. Prognostic factors and life expectancy in canine leishmaniosis. Vet Sci. (2020) 7:128. doi: 10.3390/vetsci7030128
8. de Jong, MK, Rappoldt, A, Broere, F, and Piek, CJ. Survival time and prognostic factors in canine leishmaniosis in a non-endemic country treated with a two-phase protocol including initial allopurinol monotherapy. Parasit Vectors. (2023) 16:163. doi: 10.1186/s13071-023-05777-2
9. Cortese, L, Pelagalli, A, Piantedosi, D, Mastellone, V, Di Loria, A, Lombardi, P, et al. The effects of prednisone on haemostasis in leishmaniotic dogs treated with meglumine antimoniate and allopurinol. Vet J. (2008) 177:405–10. doi: 10.1016/j.tvjl.2007.05.011
10. Aroch, I, Ofri, R, and Baneth, G. Concurrent epistaxis, retinal bleeding and hypercoagulability in a dog with visceral leishmaniosis. Isr J Vet Med. (2017) 72:35–48. Available at: http://www.ijvm.org.il/sites/default/files/aroch_1.pdf
11. Parody, N, Cacheiro-Llaguno, C, Osuna, C, Renshaw-Calderón, A, Alonso, C, and Carnés, J. Circulating immune complexes levels correlate with the progression of canine leishmaniosis in naturally infected dogs. Vet Parasitol. (2019) 274:108921. doi: 10.1016/j.vetpar.2019.108921
12. Osuna, C, Parody, N, Cacheiro-Llaguno, C, Renshaw-Calderón, A, and Carnés, J. Laboratory validation of an ELISA method to measure circulating immune complexes levels in canine visceral leishmaniasis. Vet Immunol Immunopathol. (2022) 254:110518. doi: 10.1016/j.vetimm.2022.110518
13. Cacheiro-Llaguno, C, Parody, N, Escutia, MR, and Carnés, J. Role of circulating immune complexes in the pathogenesis of canine Leishmaniasis: new players in vaccine development. Microorganisms. (2021) 9:712. doi: 10.3390/microorganisms9040712
14. Miró, G, Oliva, G, Cruz, I, Cañavate, C, Mortarino, M, Vischer, C, et al. Multicentric, controlled clinical study to evaluate effectiveness and safety of miltefosine and allopurinol for canine leishmaniosis. Vet Dermatol. (2009) 20:397–404. doi: 10.1111/j.1365-3164.2009.00824.x
15. Mancianti, F, and Meciani, N. Specific serodiagnosis of canine leishmaniasis by indirect immunofluorescence, indirect hemagglutination, and counterimmunoelectrophoresis. Am J Vet Res. (1988) 49:1,409–11.
16. IRIS . International renal interest society. Treatment recommendations for CKD in dogs. (2023). Available at: http://www.iris-kidney.com/guidelines/recommendations.html (Accessed June 16, 2023).
17. Creevy, KE, Grady, J, Little, SE, Moore, GE, Strickler, BG, Thompson, S, et al. AAHA canine life stage guidelines. J Am Anim Hosp Assoc. (2019) 55:267–90.
18. Solano-Gallego, L, Miró, G, Koutinas, A, Cardoso, L, Pennisi, MG, Ferrer, L, et al. LeishVet guidelines for the practical management of canine leishmaniosis. Parasit Vectors. (2011) 4:86. doi: 10.1186/1756-3305-4-86
19. Meléndez-Lazo, A, Ordeix, L, Planellas, M, Pastor, J, and Solano-Gallego, L. Clinicopathological findings in sick dogs naturally infected with Leishmania infantum: comparison of five different clinical classification systems. Res Vet Sci. (2018) 117:18–27. doi: 10.1016/j.rvsc.2017.10.011
20. Almeida, MAO, EEV, J, MLB, S-A, Alves, LC, MEA, B, and Atta, AM. Clinical and serological aspects of visceral leishmaniasis in northeast Brazilian dogs naturally infected with Leishmania chagasi. Vet Parasitol. (2005) 127:227–32. doi: 10.1016/j.vetpar.2004.10.010
21. Ribeiro, RR, da Silva, SM, de Oliveira Fulgêncio, G, Michalick, MSM, and FJG, F. Relationship between clinical and pathological signs and severity of canine leishmaniasis. Rev Bras Parasitol Vet. (2013) 22:373–8. doi: 10.1590/S1984-29612013000300009
22. Sabaté, D, Llinás, J, Homedes, J, Sust, M, and Ferrer, L. A single-Centre, open-label, controlled, randomized clinical trial to assess the preventive efficacy of a domperidone-based treatment programme against clinical canine leishmaniasis in a high prevalence area. Prev Vet Med. (2014) 115:56–63. doi: 10.1016/j.prevetmed.2014.03.010
23. Wylie, CE, Carbonell-Antoñanzas, M, Aiassa, E, Dhollander, S, Zagmutt, FJ, Brodbelt, DC, et al. A systematic review of the efficacy of prophylactic control measures for naturally-occurring canine leishmaniosis, part I: vaccinations. Prev Vet Med. (2014) 117:7–18. doi: 10.1016/j.prevetmed.2014.06.015
24. Fernandez, M, Tabar, MD, Arcas, A, Mateu, C, Homedes, J, and Roura, X. Comparison of efficacy and safety of preventive measures used against canine leishmaniasis in southern European countries: longitudinal retrospective study in 1647 client-owned dogs (2012-2016). Vet Parasitol. (2018) 263:10–7. doi: 10.1016/j.vetpar.2018.09.014
25. Segarra, S, Miró, G, Montoya, A, Pardo-Marín, L, Boqué, N, Ferrer, L, et al. Randomized, allopurinol-controlled trial of the effects of dietary nucleotides and active hexose correlated compound in the treatment of canine leishmaniosis. Vet Parasitol. (2017) 239:50–6. doi: 10.1016/j.vetpar.2017.04.014
26. Segarra, S, Miró, G, Montoya, A, Pardo-Marín, L, Teichenné, J, Ferrer, L, et al. Prevention of disease progression in Leishmania infantum-infected dogs with dietary nucleotides and active hexose correlated compound. Parasit Vectors. (2018) 11:103. doi: 10.1186/s13071-018-2705-z
27. Dea-Ayuela, MA, Segarra, S, Serrano, DR, and Bolás-Fernández, F. Nucleotides and AHCC enhance Th1 responses in vitro in Leishmania-stimulated/infected murine cells. Molecules. (2020) 25:3918. doi: 10.3390/molecules25173918
28. Segarra, S . Nutritional modulation of the immune response mediated by nucleotides in canine leishmaniosis. Microorganisms. (2021) 9:2601. doi: 10.3390/microorganisms9122601
29. Roura, X, Fondati, A, Lubas, G, Gradoni, L, Maroli, M, Oliva, G, et al. Prognosis and monitoring of leishmaniasis in dogs: a working group report. Vet J. (2013) 198:43–7. doi: 10.1016/j.tvjl.2013.04.001
30. Miranda, S, Roura, X, Picado, A, Ferrer, L, and Ramis, A. Characterization of sex, age, and breed for a population of canine leishmaniosis diseased dogs. Res Vet Sci. (2008) 85:35–8. doi: 10.1016/j.rvsc.2007.09.003
31. Gálvez, R, Miró, G, Descalzo, MA, Nieto, J, Dado, D, Martín, O, et al. Emerging trends in the seroprevalence of canine leishmaniasis in the Madrid region (Central Spain). Vet Parasitol. (2010) 169:327–34. doi: 10.1016/j.vetpar.2009.11.025
32. Silva, SS, de Macedo, LO, de Oliveira, JCP, Alves, LC, de Carvalho, GA, and Ramos, RAN. Canine visceral leishmaniasis: risk factors and spatial analysis in an endemic area of Northeastern Brazil. Rev Bras Parasitol Vet. (2023) 32:e003223. doi: 10.1590/S1984-29612023029
33. Dos Reis, AT, do Carmo Silva, CF, Rocha, TB, DJB, C, da Costa, AP, Costa, FB, et al. Exposure to and infection by Leishmania infantum among domestic dogs in an area of the Cerrado biome, Maranhão, Brazil. Vet Parasitol Reg Stud Reports. (2023) 39:100851. doi: 10.1016/j.vprsr.2023.100851
34. Chiyo, L, Dos Santos, AG, de Souza, AB, Rivas, AV, Valle, SB, da Paixão Sevá, A, et al. Cross-sectional spatial and epidemiological analysis of canine visceral leishmaniasis cases in the triple border region, Brazil, Argentina and Paraguay, between 2015 and 2020. Acta Trop. (2023) 239:106811. doi: 10.1016/j.actatropica.2022.106811
35. da SS, CC, Barbosa, DS, Oliveira, VC, Cardoso, DT, Guimarães, NS, and Carneiro, M. Factors associated with human visceral leishmaniasis cases during urban epidemics in Brazil: a systematic review. Parasitology. (2021) 148:639–47. doi: 10.1017/S0031182021000019
36. Ávila, IR, de Araújo, GR, Barbosa, DS, and Bezerra, JMT. Occurrence of human visceral leishmaniasis in the central-west region of Brazil: a systematic review. Acta Trop. (2023) 237:106707. doi: 10.1016/j.actatropica.2022.106707
37. Amusategui, I, Sainz, A, Rodríguez, F, and Tesouro, MA. Distribution and relationships between clinical and biopathological parameters in canine leishmaniasis. Eur J Epidemiol. (2003) 18:147–56. doi: 10.1023/a:1023090929302
38. Reis, AB, Martins-Filho, OA, Teixeira-Carvalho, A, Carvalho, MG, Mayrink, W, França-Silva, JC, et al. Parasite density and impaired biochemical/hematological status are associated with severe clinical aspects of canine visceral leishmaniasis. Res Vet Sci. (2006) 81:68–75. doi: 10.1016/j.rvsc.2005.09.011
39. da Costa-Val, AP, Cavalcanti, RR, de Figueiredo, GN, Marques Michalick, MS, Alexander, B, Williams, P, et al. Canine visceral leishmaniasis: relationships between clinical status, humoral immune response, haematology and Lutzomyia (Lutzomyia) longipalpis infectivity. Vet J. (2007) 174:636–43. doi: 10.1016/j.tvjl.2006.11.006
40. Torrecilha, RBP, Utsunomiya, YT, Bosco, AM, Almeida, BF, Pereira, PP, Narciso, LG, et al. Correlations between peripheral parasite load and common clinical and laboratory alterations in dogs with visceral leishmaniasis. Prev Vet Med. (2016) 132:83–7. doi: 10.1016/j.prevetmed.2016.08.006
41. Riera, C, Valladares, JE, Gállego, M, Aisa, MJ, Castillejo, S, Fisa, R, et al. Serological and parasitological follow-up in dogs experimentally infected with Leishmania infantum and treated with meglumine antimoniate. Vet Parasitol. (1999) 84:33–47. doi: 10.1016/S0304-4017(99)00084-9
42. Paradies, P, Sasanelli, M, de Caprariis, D, Testini, G, Traversa, D, Lia, RP, et al. Clinical and laboratory monitoring of dogs naturally infected by Leishmania infantum. Vet J. (2010) 186:370–3. doi: 10.1016/j.tvjl.2009.09.011
43. Paltrinieri, S, Gradoni, L, Roura, X, Zatelli, A, and Zini, E. Laboratory tests for diagnosing and monitoring canine leishmaniasis. Vet Clin Pathol. (2016) 45:552–78. doi: 10.1111/vcp.12413
44. Villanueva-Saz, S, Ballesté, C, Casasnovas, AF, Estevez, AY, and Arribas, MTV. Bisalbuminemia in a dog with leishmaniosis after anti-Leishmania therapeutic protocol administration: a rare condition detected in the electrophoretogram. Vet Rec Case Rep. (2020) 8:e001290. doi: 10.1136/vetreccr-2020-001290
45. Torres, M, Bardagí, M, Roura, X, Zanna, G, Ravera, I, and Ferrer, L. Long term follow-up of dogs diagnosed with leishmaniosis (clinical stage II) and treated with meglumine antimoniate and allopurinol. Vet J. (2011) 188:346–51. doi: 10.1016/j.tvjl.2010.05.025
46. Foglia Manzillo, V, Di Muccio, T, Cappiello, S, Scalone, A, Paparcone, R, Fiorentino, E, et al. Prospective study on the incidence and progression of clinical signs in naïve dogs naturally infected by Leishmania infantum. PLoS Negl Trop Dis. (2013) 7:e2225. doi: 10.1371/journal.pntd.0002225
47. Rodríguez, A, Solano-Gallego, L, Ojeda, A, Quintana, J, Riera, C, Gállego, M, et al. Dynamics of Leishmania-specific immunoglobulin isotypes in dogs with clinical leishmaniasis before and after treatment. J Vet Intern Med. (2006) 20:495–8. doi: 10.1892/0891-6640(2006)20[495:doliii]2.0.co;2
48. Teixeira Neto, RG, Giunchetti, RC, Carneiro, CM, de Almeida Vitor, RW, Coura-Vital, W, Quaresma, PF, et al. Relationship of Leishmania-specific IgG levels and IgG avidity with parasite density and clinical signs in canine leishmaniasis. Vet Parasitol. (2010) 169:248–57. doi: 10.1016/j.vetpar.2010.01.023
49. de Freitas, JCC, Lopes-Neto, BE, de Abreu, CRA, Coura-Vital, W, Braga, SL, Reis, AB, et al. Profile of anti-Leishmania antibodies related to clinical picture in canine visceral leishmaniasis. Res Vet Sci. (2012) 93:705–9. doi: 10.1016/j.rvsc.2011.12.009
50. Koutinas, AF, and Koutinas, CK. Pathologic mechanisms underlying the clinical findings in canine leishmaniosis due to Leishmania infantum/chagasi. Vet Pathol. (2014) 51:527–38. doi: 10.1177/0300985814521248
51. Proverbio, D, Spada, E, Bagnagatti de Giorgi, G, Perego, R, and Valena, E. Relationship between Leishmania IFAT titer and clinicopathological manifestations (clinical score) in dogs. Biomed Res Int. (2014) 2014:412808. doi: 10.1155/2014/412808
Keywords: Leishmania infantum, immune complexes deposition, canine leishmaniosis, biomarker, PEG-ELISA
Citation: Sarquis J, Parody N, Montoya A, Cacheiro-Llaguno C, Barrera JP, Checa R, Daza MA, Carnés J and Miró G (2024) Clinical validation of circulating immune complexes for use as a diagnostic marker of canine leishmaniosis. Front. Vet. Sci. 11:1368929. doi: 10.3389/fvets.2024.1368929
Edited by:
Barbara Moroni, Experimental Zooprophylactic Institute for Piedmont, Liguria and Valle d'Aosta (IZSPLVA), ItalyReviewed by:
Vinícius Silva Belo, Universidade Federal de São João del-Rei, BrazilGiulia Morganti, University of Perugia, Italy
Copyright © 2024 Sarquis, Parody, Montoya, Cacheiro-Llaguno, Barrera, Checa, Daza, Carnés and Miró. This is an open-access article distributed under the terms of the Creative Commons Attribution License (CC BY). The use, distribution or reproduction in other forums is permitted, provided the original author(s) and the copyright owner(s) are credited and that the original publication in this journal is cited, in accordance with accepted academic practice. No use, distribution or reproduction is permitted which does not comply with these terms.
*Correspondence: Guadalupe Miró, gmiro@ucm.es