Influence of Postemergence Dicamba/Glyphosate Timing and Inclusion of Acetochlor as a Layered Residual on Weed Control and Soybean Yield
- Department of Agronomy, University of Wisconsin-Madison, Madison, WI, United States
Roundup Ready 2 Xtend® [glyphosate- and dicamba-resistant (DR)] soybean is a novel trait option for postemergence (POST) control of herbicide-resistant broadleaf weeds in soybean. With increased use of labeled dicamba products POST in DR soybean and recommendations to include a soil-residual herbicide POST (e.g., layered residual approach), research on how combinations of these approaches influence weed control, weed seed production, and soybean grain yield is warranted. The objective of this research was to evaluate the effects of (1) flumioxazin applied preemergence (PRE) followed by (fb) dicamba plus glyphosate applied POST at different crop developmental stages and (2) acetochlor POST as a layered residual approach on weed control, weed seed production, and soybean yield to determine the optimal POST timing in DR soybean. A field study was conducted in Wisconsin at three sites in 2018 and four sites in 2019 to evaluate flumioxazin (43.4 g ai ha−1, WDG 51%) PRE fb dicamba (560 g ae ha−1, SL) plus glyphosate (1,101 g ae ha−1, SL) POST in DR soybean at three stages: early-POST (EPOST, V1-V2), mid-POST (MPOST, V3-V4), and late-POST (LPOST, V5-V6/R1) with or without a soil-residual herbicide POST (acetochlor, 1,262 g ai ha−1, ME). Weed community composition was site-specific; difficult-to-control broadleaf species included giant ragweed (Ambrosia trifida L.) and waterhemp [Amaranthus tuberculatus (Moq.) J.D. Sauer]. Dicamba plus glyphosate applied MPOST and LPOST provided greater control, weed biomass reduction, and density reduction of giant ragweed and waterhemp when compared with EPOST treatments. Giant ragweed and waterhemp had not reached 100% cumulative emergence at EPOST, and plants that emerged after EPOST produced seed. There was some benefit to including acetochlor as a layered residual at EPOST as indicated by a residual by POST timing interaction for waterhemp density reduction. Complete waterhemp control was not attained at one site-year. For remaining site-years, dicamba plus glyphosate applied MPOST (V3-V4) provided season-long weed control, reduced weed seed production, and optimized soybean grain yield compared with other POST treatments. Results highlight the importance of timely POST applications and suggest utilization of a POST layered residual needs to be timed appropriately for the window of active weed species emergence.
Introduction
Soybean [Glycine max (L.) Merr] is a major global commodity and a key component of annual cropping systems in the United States, accounting for 30.6 million planted crop ha nationally and 708 thousand planted crop ha in Wisconsin in 2019 (USDA-NASS, 2019). Soybean cultivars with stacked resistance to glyphosate and dicamba [Roundup Ready® 2 Xtend® (RR2X), Bayer Crop Science, St. Louis, MO] were commercially available starting in 2016 (Behrens et al., 2007). Traited cultivars are tolerant to applications of dicamba preemergence (PRE) and postemergence (POST), offering another tool for management of key, difficult-to-control broadleaf species with evolved resistance to glyphosate and other herbicides, without risk of crop injury. Two reduced-volatility dicamba formulations were first labeled for use in glyphosate and dicamba-resistant (DR) soybean in 2017 (Werle et al., 2018; Anonymous, 2020a,b; EPA, 2020): the diglycolamine salt (DGA) of dicamba with an acetic acid-acetate buffer system “VaporGrip® technology” (VG) (MacInnes, 2016; Abraham, 2018) (DGA+VG) and the N-N-Bis-(3-aminopropyl) methylamine salt (BAPMA) of dicamba.
Single pass weed management programs with an effectively timed POST herbicide application once provided season-long weed control in glyphosate-resistant (GR) crops in the Midwest US (Mulugeta and Boerboom, 2000; Knezevic et al., 2003), but two-pass programs [i.e., PRE followed by (fb) POST] generally provided more consistent weed control and higher net returns (Proost et al., 2006). For instance, Fickett et al. (2013) reported that delaying one pass POST applications of glyphosate from V2 until V3–V4 growth stage prolonged early-season weed competition and resulted in a 5% predicted soybean yield loss across 64 site-years in southern Wisconsin. A five-year registration granted in late 2020 allows for POST use of dicamba products in DR soybean up to flowering or the nationwide cut-off date of June 30, whichever occurs first (Anonymous, 2020a,b). Delaying POST control measures can result in weed stage exceeding the label recommended height (ht) of 10-cm, which may decrease herbicide efficacy. Hedges et al. (2018) reported delaying control until 25-cm GR horseweed (Erigeron canadensis L.) with glyphosate plus dicamba applied POST resulted in 14–25% lower control than treatments sprayed at 5- and 15-cm weed ht, when averaged across herbicide rates 28 days after treatment (DAT). Moreover, it is well-established that use of effective PRE herbicides and/or preplant tillage operations can help to alleviate early-season competition for limited nutrients, light, water, and space (Ross and Lembi, 2008). Knezevic et al. (2019) reported applying a soil-residual herbicide PRE delayed the critical period for weed control by several weeks in soybean, providing flexibility for growers to complete POST applications in accordance with their weed pressure, weed stage, and soybean canopy closure.
Utilizing dicamba POST in combination with glyphosate is a common recommendation with the RR2X technology. Underwood et al. (2017) reported increased control of broadleaf weed populations with no known herbicide resistance [i.e., common ragweed (Ambrosia artemisiifolia L.) and common lambsquarters (Chenopodium album L.)] with 900 g ae ha−1 glyphosate plus 300 g ae ha−1 DGA+VG, regardless of POST timing, when compared with glyphosate alone. Vink et al. (2012) reported 900 g ae ha−1 glyphosate plus 300 or 600 g ae ha−1 DGA dicamba provided greater control of (GR) giant ragweed (Ambrosia trifida L.) than glyphosate alone when applied as a single POST (78–100 vs. 40–46%, respectively). Spaunhorst et al. (2014) reported that a PRE herbicide fb DGA dicamba plus glyphosate at V3–V4 soybean growth stage (10-cm weed ht) increased control of GR waterhemp [Amaranthus tuberculatus (Moq.) J.D. Sauer] from glyphosate alone from 50 to 89%. This indicates including dicamba as part of a chemical weed management program has the potential to enhance GR broadleaf weed control.
Another recommended approach in soybean is to utilize a layered residual approach, where in addition to applying a full rate of residual herbicide PRE, a full rate of soil-applied residual herbicide is also included as part of the POST program [~21–30 days after planting (DAP)] to control germinating weed seeds (Norsworthy et al., 2012; Goplen et al., 2017; Hartzler and Anderson, 2019). In soybean, the main options for soil residual herbicides that are labeled for POST use include very long chain fatty acid (VLCA, Group 15/K3 N; i.e., acetochlor, S-metolachlor), protoporphyrinogen oxidase (PPO, Group 14/E; i.e., fomesafen), and acetolactate synthase (ALS, Group 2/B) inhibitor herbicides (i.e., chlorimuron-ethyl, cloransulam-methyl, imazethapyr). Product labels enforce region-specific cut-offs that influence when and how labeled products might fit into a layered residual approach. This approach has become a frequently recommended integrated weed management (IWM) practice (Norsworthy et al., 2012). Steckel et al. (2002) and Sarangi and Jhala (2018) reported that a layered residual approach improved control of Palmer amaranth (Amaranthus palmeri S. Watson) and waterhemp, respectively; however, the utility of this approach in conjunction with the RR2X technology across diverse weed communities has not been fully documented in the literature. This integrative approach is a promising technique to attain season-long control of small-seeded, difficult-to-control broadleaf weeds with extended emergence windows while also minimizing the need for additional herbicides applied POST, thus reducing selection pressure for herbicide resistance evolution (Norsworthy et al., 2012).
With the recent launch of RR2X and increased adoption of the layered residual approach, research on how combinations of these two approaches influence control and seed production of multiple weed species, including difficult-to-control broadleaf weed species such as giant ragweed and waterhemp, is warranted. The objective of this research was to evaluate the effects of (1) a herbicide applied PRE fb dicamba plus glyphosate applied POST at different crop developmental stages and (2) acetochlor POST as part of a layered residual approach on weed control, viable weed seed production, and soybean grain yield to determine the optimal POST timing for weed control in DR soybean in southern Wisconsin. The hypothesis of this research was that a herbicide applied PRE fb dicamba plus glyphosate early-POST (EPOST) in combination with a soil-residual herbicide would provide >90% weed control, near zero weed seed production, and sustain soybean grain yield in respect to other PRE fb POST treatments across distinct weed community compositions in southern Wisconsin.
Materials and Methods
A field study was conducted at seven-site years in Wisconsin in 2018 and 2019. The study was established at the University of Wisconsin-Madison Arlington Agricultural Research Station (ARS) near Arlington, Wisconsin (WI; 2018 and 2019), Lancaster ARS near Lancaster, WI (2018 and 2019), Rock County Farm near Janesville, WI (2018 and 2019), and a grower's field near Brooklyn, WI (2019). Field sites and soil information for site-years are listed in Table 1. All field sites were part of an annual corn—soybean rotation; five of the site-years were following a corn crop, while the Janesville and Brooklyn sites in 2019 were following soybean. All sites had a history of conventional tillage (<30% soil surface residue after planting), which is common practice in Wisconsin (Werle and Oliveira, 2018). Janesville and Lancaster in 2018 and Arlington in 2018 and 2019 were chisel-plowed the preceding fall. In the spring, the seedbed was prepared for planting with a field cultivator at Arlington in 2018 and 2019, a field cultivator with a field finisher at Janesville in 2018 and 2019, vertical tillage at Lancaster in 2018, a chisel-plow fb a cultimulcher at Lancaster in 2019, and a disk fb a field cultivator with a field finisher at Brooklyn in 2019. Dicamba-resistant (DR) soybean (2018: Asgrow 21X8; 2019: Asgrow 21X7, Bayer Crop Science) was planted in 76 cm row spacing at 2.5–3.8 cm depth, depending on site, from mid-May to early June and seeded at 345,940 seeds ha−1 for all site-years, except for Janesville 2018, which was seeded at 296,520 seeds ha−1 (Table 1). Plot size was 3 m wide (4 soybean rows) by 9 m long at all sites.
The study consisted of eight treatments organized in a randomized complete block design with four replications. The study was designed as a 3 × 2 factorial with three levels of POST timing: V1–V2 (EPOST), V3–V4 (mid-POST, MPOST), and V5–V6/R1 (late POST, LPOST), and addition or exclusion of a soil-residual herbicide applied POST. Two additional treatments were included, a non-treated control (NTC) and a PRE herbicide only treatment (PRE-only), for a total of eight treatments. All products selected were included at recommended field rates per label specifications by soil type and soil organic matter content except for the rate of glyphosate, which was 157 g ae ha−1 lower than the maximum rate permitted. Flumioxazin (43.4 g ai ha−1, Valor SX®, Valent, Walnut Creek, CA) was applied PRE in all treatments within 3 d after planting except for the NTC, which received no PRE application. The POST treatments included 560 g ae ha−1 DGA+VG (XtendiMax® with VaporGrip® Technology, Bayer Crop Science) plus potassium salt of glyphosate at 1,101 g ae ha−1 (Roundup PowerMax®, Bayer Crop Science). For treatments with a layered residual approach, 1,262 g ai ha−1 encapsulated acetochlor (Warrant®, Bayer Crop Science) was included with the POST treatment. All POST treatments included a water conditioning agent plus non-ionic surfactant blend at 1% v/v (Class Act® Ridion®, Winfield United, St. Paul, MN) and a drift reduction agent containing polyethylene glycol, choline chloride, and guar gum at 0.5% v/v (IntactTM, Precision Laboratories, LLC, Waukegan, IL). Application dates and soybean growth stages are listed in Table 2. Herbicide treatments were applied using a CO2-pressurized backpack sprayer equipped with a 3.0-m hand-held spray boom with Extended Range (XR) 11002 flat-fan nozzles (TeeJet Technologies, Spraying Systems Co., Wheaton, IL) for the PRE applications and Turbo TeeJet Induction (TTI) 110015 nozzles (TeeJet Technologies) for POST applications on 50.8-cm spacing calibrated to deliver 140 L ha−1 carrier vol at 165 kPa for PRE applications and 275 kPa for POST treatments and a walking speed of 4.8 km h−1.
Data Collection
Meteorological Data
Weather data for all site-years were collected with a Watchdog 1650 Micro Station (Spectrum Technologies, Aurora, IL) positioned adjacent to study area at each site to record the following parameters in 15 min intervals: air temperature at 1 m, relative humidity at 1 m, soil temperature and soil moisture at 2-cm depth. The 30-yr normal monthly temperature and precipitation values were obtained in R statistical software version 4.0.2 (R Core Team, 2020) using daily Daymet weather data for 1 km grids (“daymetr” package 1.4; Thornton et al., 2020; Correndo et al., 2021). Temperature and precipitation for 2018 and 2019 growing seasons and 30 year averages for each site are presented in Table 3. Adequate rainfall for activation occurred within 10 d following PRE flumioxazin application for all site-years (18.6–53.4 mm), with the first rainfall occurring within 0–7 d following application across site-years (data not shown).
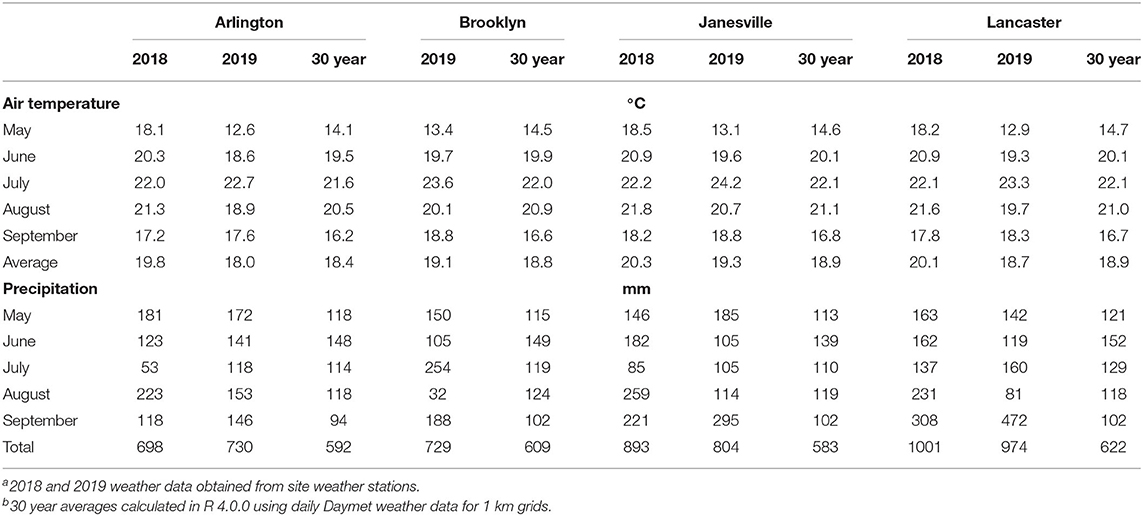
Table 3. Mean monthly air temperature and total precipitation in 2018 and 2019 at Arlington, Janesville, and Lancaster, and 2019 in Brooklyn, WI, and during the past 30 yearsa, b.
Weed Emergence
Weed species richness and density varied across sites and years. Broadleaf species were more abundant than monocot species at all sites. Emergence of weed species were monitored at all site-years from crop establishment through 28 days (d) after the LPOST treatment in 7–10 d increments in two 0.1 m2 permanent quadrats between the center two rows per plot in the PRE-only and NTC treatments. At each data collection point, emerged seedlings were identified, counted, removed, and discarded. Most weed seedlings were <2.5-cm in ht at removal. Caution was taken to minimize soil disturbance while seedlings were identified, enumerated, and removed.
Weed and Crop Developmental Stage at Post Treatments
Shoot weed biomass (g m−2), weed density (plants m−2), and weed ht (cm) were recorded immediately prior to each POST herbicide treatment for each species in the respective plots from three randomly placed 0.1 m2 quadrats between the center two rows (Table 4). Weeds were identified, heights measured, counted, clipped at ground level, and bagged by species for each quadrat. Weed biomass was dried at 52°C for 2 weeks (wk) until constant dry weight. To estimate crop canopy at each POST timing, one to eight images of the center two rows of treated plots were captured at 1.2 m ht with an iPhone 7 (Apple, Inc., Cupertino, CA) camera. The camera was adjusted to square mode in order to produce a repeatable image frame with its lens centered in between the two soybean rows and perpendicular to the ground. Images were edited to remove green area from weeds and then batch analyzed for green area cover using Canopeo (Oklahoma State University, Stillwater, OK) add-on within Matlab (The Math Works, Inc., Natick, MA) (Arsenijevic et al., 2020; Potratz et al., 2020). Default Canopeo settings were used (Red/Green: 0.95, Blue/Green: 0.95, Noise reduction: 100) to maintain continuity across analyses. Averages (± SE) for soybean canopy and shoot biomass, density, and ht for the most abundant weed species at each site-year are presented (Table 4).
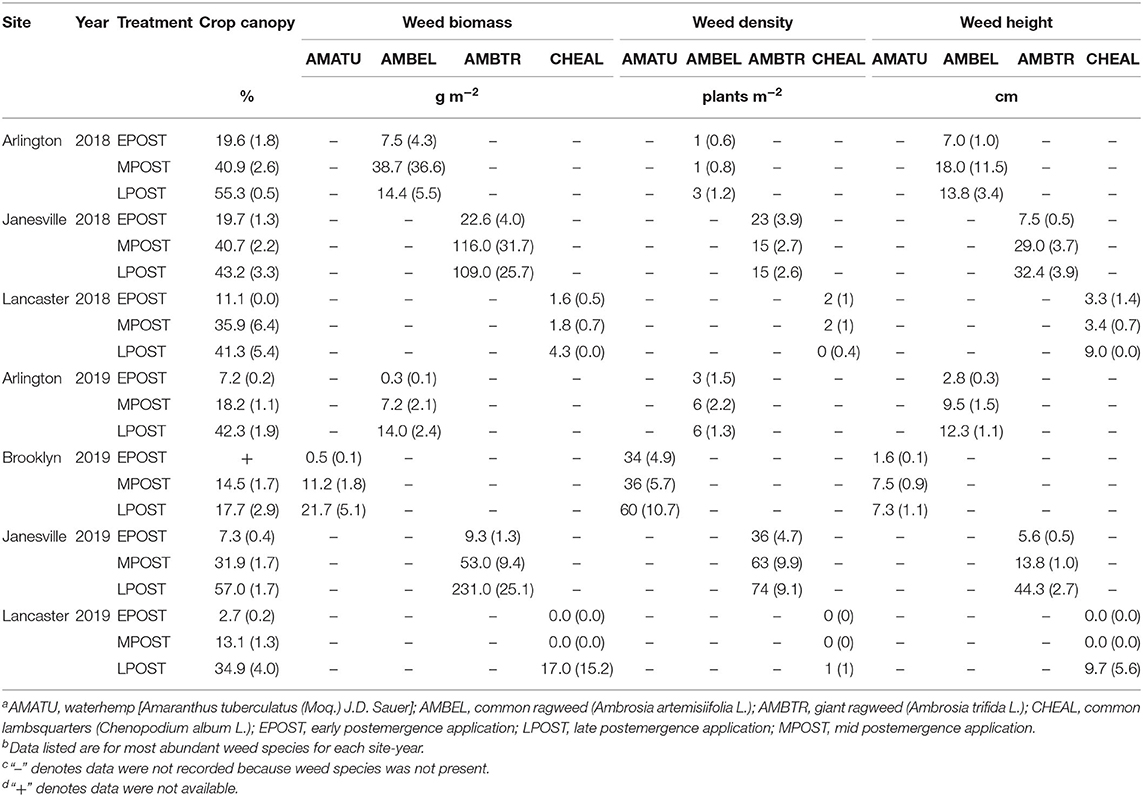
Table 4. Average (± SE) crop canopy, weed biomass, density, and height collected prior to POST herbicide application in 2018 and 2019 at Arlington, Janesville, and Lancaster, and 2019 in Brooklyn, WIa−d.
Weed Control and Density
By species and overall control ratings from 0 to 100% were collected 28 d after the LPOST treatment. Weed density (m−2) was also collected from two randomly placed 0.1 m2 quadrats between the center two rows per plot from all treatments at this time. Weed density (by species) was utilized to calculate percent reduction (y) from the NTC:
where a is the average density (by species) for the NTC treatment and b is the observed density (by species) for an individual experimental plot; calculations were completed within site-years.
Weed Seed Production and Viability
Prior to crop harvest, giant ragweed and waterhemp seed production was estimated at two (Janesville 2018 and 2019) and one (Brooklyn 2019) site-years, respectively. These site-years were selected due to high and consistent pressure of the most abundant weed species (>15 plants m−2) and lack of complete control with POST herbicide treatments. Weed seedheads were collected late August to early October (after weeds reached physiological maturity) from two plants per plot. Care was taken to select seedheads with minimal shattering from representative plants. Seedhead samples were dried for 2 wk in laboratory settings at 21°C air temperature, hand-threshed, and cleaned using mesh sieves and an Oregon Seed Blower (Hoffman Manufacturing, Inc., Corvallis, OR). Seeds per plant (determined as described below) and species density (estimated from two randomly placed 0.1 m2 quadrats per plot) were used to estimate seed production m−2.
Giant ragweed seeds per sample were counted individually. Two subsamples of 15 non-conditioned giant ragweed seeds per sample were subjected to a tetrazolium (Tz) viability test as described by Glettner and Stoltenberg (2015). Estimated seed production m−2 and percent viability were used to estimate viable seed production m−2. Waterhemp seed production was estimated by recording five, 100 seed count weights and the total mass for each sample, dividing the average 100 seed count weight by the total mass of the sample, and multiplying that number by 100 (adapted from Clay et al., 2005). Total seed counts were multiplied by 0.5 to account for assumption of a 50:50 male to female ratio of waterhemp (Heneghan and Johnson, 2017). Care was taken to collect female plants at seed collection.
Soybean Grain Yield
At crop maturity, the center two rows of each plot were mechanically harvested using an Almaco plot combine (Almaco Co., Nevada, IA) equipped with a Seed Spector LRX (Almaco Co.) grain gauge. Soybean yield data were adjusted to 13% moisture and presented in kg ha−1.
Statistical Analyses
Modeling Weed Emergence and Total Emergence
All analyses were completed in R statistical software version 4.0.0 (R Core Team, 2020). Total weed emergence for the NTC and PRE-only monitored quadrats over time following study area preparation and planting were used to calculate relative cumulative emergence of species over time for each treatment. These models do not account for early season weed emergence, as emerged weeds were eliminated prior to planting during spring tillage and seedbed preparation as described above. Thus, cumulative emergence at experiment establishment was assumed to be 0%. The cumulative emergence of the most abundant broadleaf species over day of year (DOY) for each site were subjected to a non-linear regression analysis (“drc” package 3.0–1; Ritz et al., 2015). Cumulative emergence data were fit to a 4-parameter log-logistic model (Ritz et al., 2015):
where y is cumulative emergence, x is DOY, b is slope, e is the inflection point (also represents time to 50% cumulative emergence[T50]), c is the lower limit, and d is the upper limit, which was restricted to 1. Proportions were used for cumulative emergence in the model and then converted to percentage for discussion and data presentation. Estimates were obtained for T10 and T90 (days to 10 and 90% cumulative seedling emergence, respectively) (“drc” package 3.0–1; Ritz et al., 2015). Total weed species emergence within sites for the NTC and PRE-only treatments were subjected to a two-sample t-test (“stats” package 4.0.0; R Core Team, 2020) by site.
ANOVA
Sites were analyzed separately due to differences in weed species composition. Years within a site were included as a random effect and considered as environments sampled at random for all response variables (Carmer et al., 1989; Blouin et al., 2011). All response variables were analyzed as a 3 × 2 factorial; replication and year were nested within sites as random effects. The NTC and PRE treatments were excluded from analyses because the objective of this study was to determine the optimal POST timing and evaluate the influence of a layered soil-residual herbicide applied POST in the context of a two-pass PRE fb POST program. Weed seed production, viable weed seed production, and soybean yield data were fit to a linear mixed model (LMM; “lme4” package 1.1–23; Bates et al., 2015). Model assumptions for LMMs were evaluated using Pearson chi-square test for normality (“nortest” package 1.0–4; Gross and Ligges, 2015) and Levene's test for homogeneity of variance (“car” package 3.0–8; Fox and Weisberg, 2019). A square root transformation was applied to weed seed production and viable weed seed production to satisfactorily meet model assumptions (back-transformed means are presented for ease of interpretation). Weed density reduction from NTC, weed control, and weed seed viability data were fit to a generalized linear mixed model (GLMM) with Template Model Builder with a beta distribution and logit link (“glmmTMB” package 1.0.1; Brooks et al., 2017). ANOVA was performed (LMM: “car” package; GLMM: Anova.glmmTMB function) and means were separated using Fisher's Protected LSD at P ≤ 0.05 (“emmeans” package 1.4.7; Lenth, 2020).
Results and Discussion
The ANOVA results for POST timing and layered residual interaction and main effects are summarized for all response variables presented herein (Table 5). Broadleaf weed emergence for all sites throughout the season is detailed in Table 6. Weed control 28 d after the LPOST treatment for PRE fb POST treatments ranged from 66 to 99% across weed species (Table 7; Figures 1, 2). Density reduction relative to the NTC 28 d after the LPOST treatment ranged from 58 to 99% across species (Table 7; Figures 1, 2).
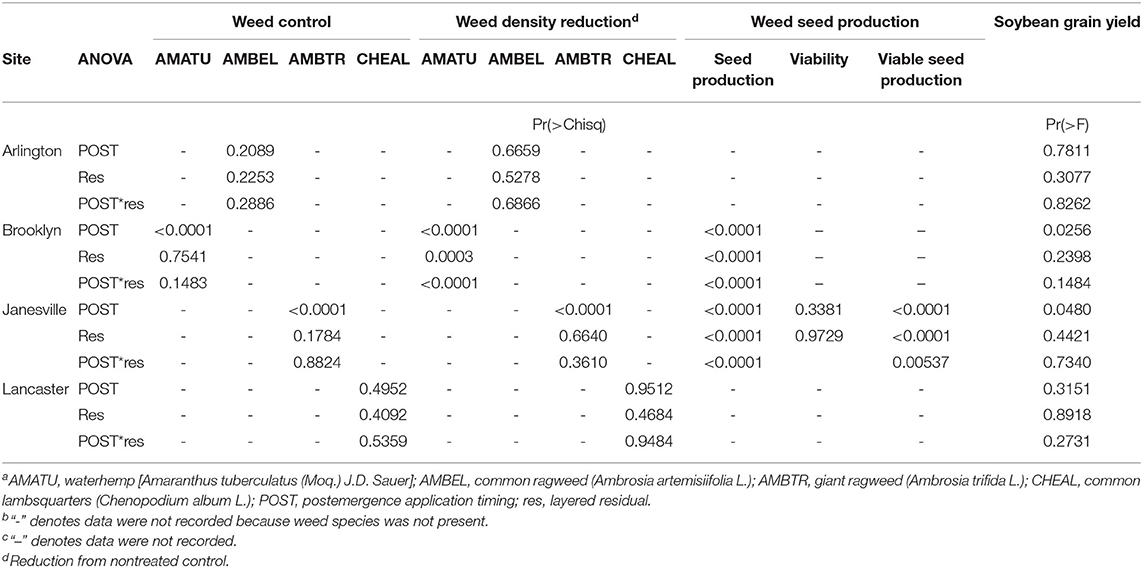
Table 5. Summary of main effects and interactions for weed control and weed density reduction by species, weed seed fecundity and viability, and soybean grain yield at Arlington, Janesville, and Lancaster pooled across two years, and in 2019 at Brooklyn, WIa−c.
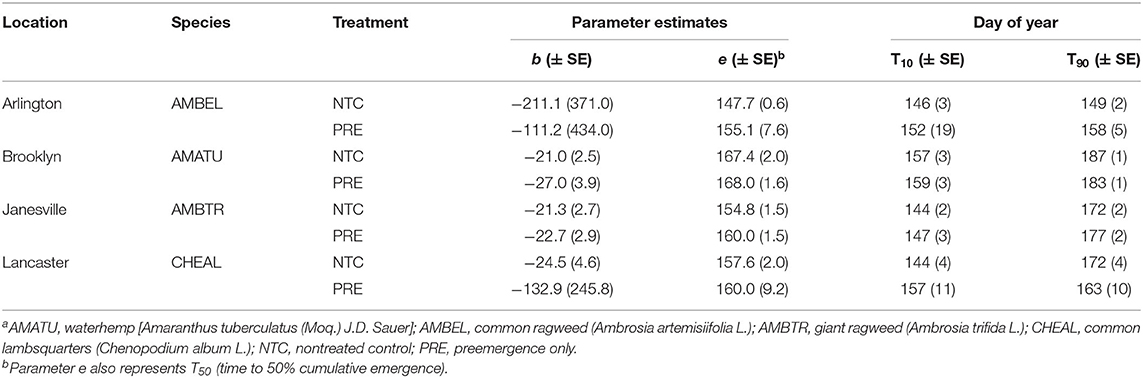
Table 6. Parameter estimates for slope (b) and the inflection point (e); time to 10 and 90% total emergence of most abundant broadleaf weed species (T10 and T90, respectively) for the monitored quadrats in the non-treated control and PRE flumioxazin treatments at Arlington, Janesville, and Lancaster pooled across two years, and in 2019 at Brooklyn, WIa.
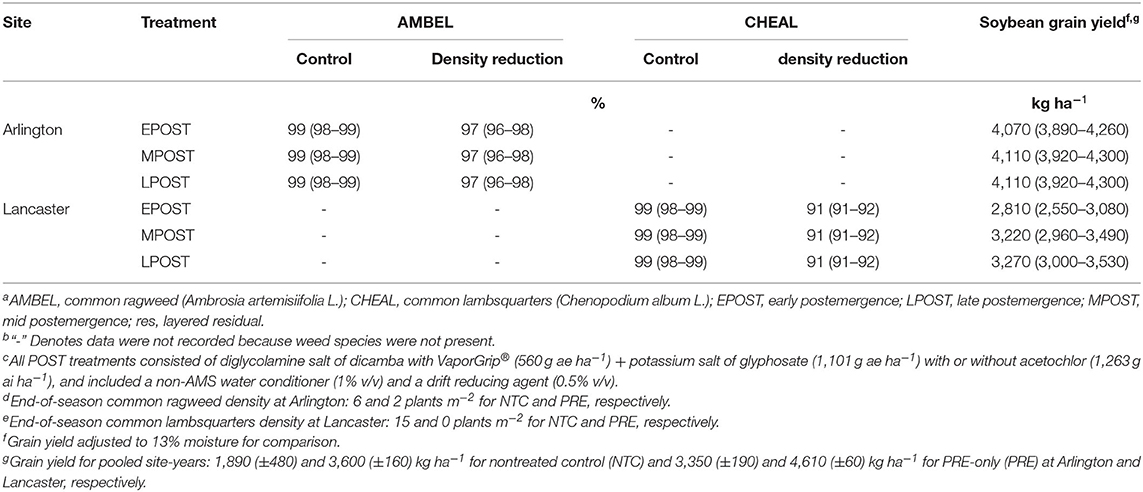
Table 7. Mean weed control and density reduction relative to the non-treated control (NTC) collected 28 d after late POST and soybean grain yield and the lower and upper limits of the 95% confidence interval around the means for experiments conducted at Arlington and Lancaster, WI pooled across two yearsa−e.
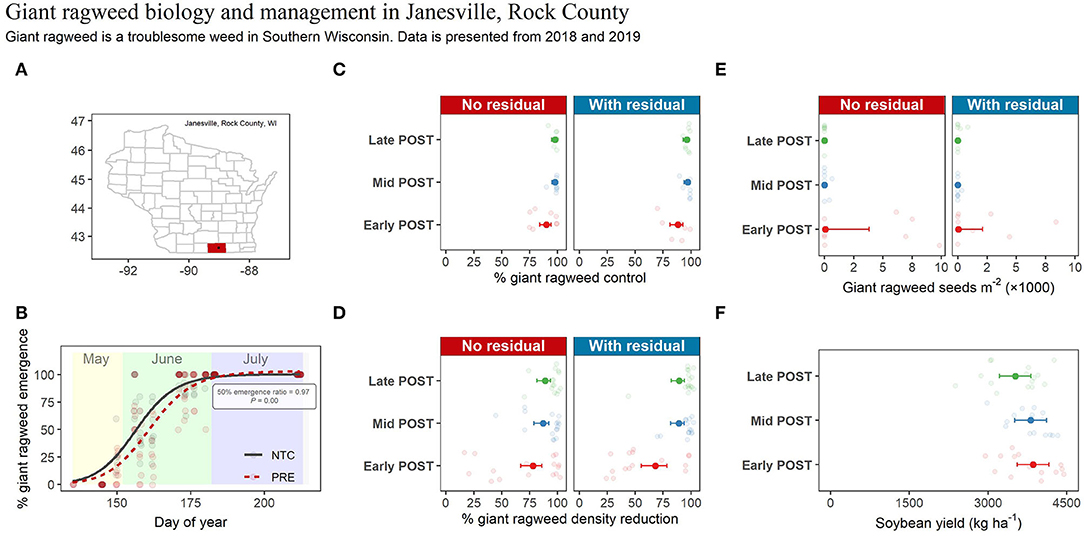
Figure 1. Giant ragweed biology and management at Janesville, WI pooled across 2 years. Experiment location (A), giant ragweed emergence over time for non-treated control (NTC) and preemergence-only (PRE) treatments (B), control (C), density reduction relative to NTC (D), giant ragweed seed production (E), and soybean grain yield (F). (C–F) Only, bars represent the 95% confidence intervals built around means. POST, postemergence.
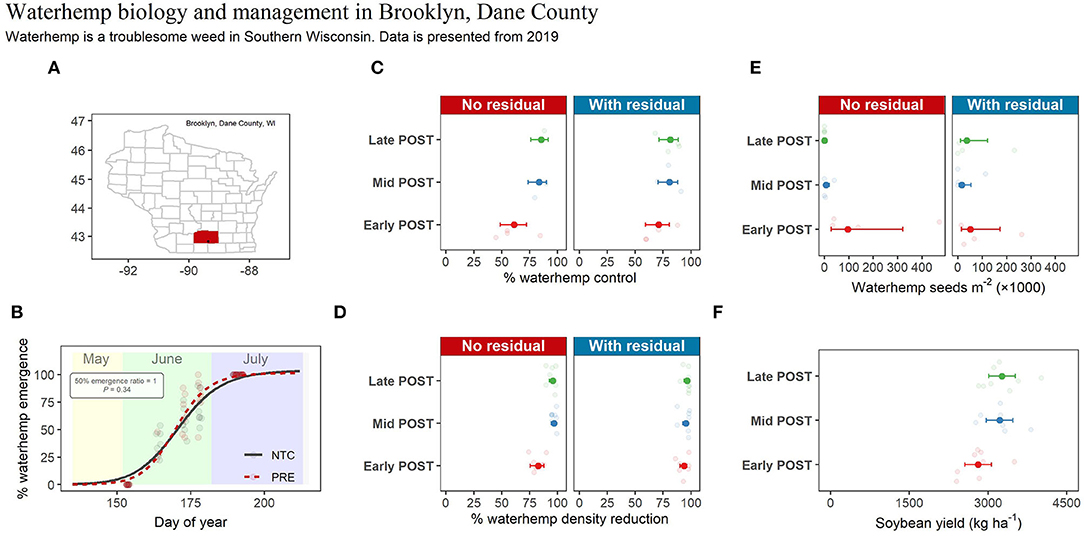
Figure 2. Waterhemp biology and management at Brooklyn, WI in 2019. Experiment location (A), waterhemp emergence over time for non-treated control (NTC) and preemergence-only (PRE) treatments (B), control (C), density reduction relative to NTC (D), waterhemp seed production (E), and soybean grain yield (F). (C–F) Only, bars represent the 95% confidence intervals built around means. POST, postemergence.
Janesville
Giant Ragweed Control and Density Reduction
Flumioxazin applied PRE alone provided 29% giant ragweed control and 30% density reduction 28 d after the LPOST treatment at Janesville, respectively (data not shown). Total giant ragweed emergence did not differ between the PRE-only treatment and the NTC at Janesville (P < 0.05). Giant ragweed is listed as controlled or suppressed at the use rate selected on the flumioxazin product label (e.g., Valor SX; Anonymous, 2016). However, flumioxazin is typically applied in combination with an ALS inhibitor when applied PRE to increase control of giant ragweed (i.e., cloransulam-methyl; Kaur et al., 2014; Spaunhorst et al., 2014; Wuerffel et al., 2015). Control of giant ragweed was influenced by POST timing at Janesville (P < 0.0001; Table 5). Adequate rainfall (12.7–19 mm) for layered residual activation occurred within 10 d after each POST treatment for all applications except for the LPOST treatment at Janesville in 2018 (1.3 mm rainfall within 10 d after treatment; data not shown). At Janesville, >25% of giant ragweed had yet to emerge after the EPOST treatment, yet 100% cumulative emergence was attained by the LPOST treatment (Figure 1B). Giant ragweed control was lower for the EPOST treatment when compared with the MPOST and LPOST treatments (Figure 1C).
Vink et al. (2012) reported PRE fb DGA plus glyphosate POST (V1-V2 soybean growth stage) provided higher than 96% giant ragweed control 4 wk after application. Spaunhorst et al. (2014) reported similar levels of GR giant ragweed control for pre-plant (PP) fb EPOST (10 cm weed ht) and PP fb MPOST (~20 cm weed ht) of DGA dicamba plus glyphosate and attributed the response due to no additional emergence through the remainder of the season. Giant ragweed is one of the earliest emerging summer annuals in the Midwest (Werle et al., 2014); biotypes exhibit either a rapid emergence pattern (50% emergence in less than a wk) or an extended emergence window and require different management practices (Sprague et al., 2004; Schutte et al., 2008, 2012). Giant ragweed biotypes with an extended emergence window can be exceptionally challenging to manage (Wuerffel et al., 2015). The giant ragweed population at the Janesville site had an extended emergence window well into June (Figure 1B).
End-of-season giant ragweed density in Janesville for the NTC and PRE-only treatments was 27 (±5) and 24 (±4) plants m−2, respectively (data not shown). The POST timing (P < 0.0001) influenced giant ragweed density reduction at Janesville, while layered residual had no impact (P = 0.6640; Table 5). Giant ragweed density reduction was lower for the EPOST treatment compared with the MPOST and LPOST treatments (Figure 1D). As described above, most giant ragweed seedlings that emerged following EPOST treatments were controlled by later POST treatments (Figures 1B–D). Applying acetochlor as part of a layered residual approach, regardless of POST timing, did not influence giant ragweed control or density reduction (Table 5). It is well-established that use of a residual herbicide early- or late-spring (i.e., saflufenacil, simazine, chlorimuron-ethyl, tribenuron-methyl) improves control of giant ragweed (Wuerffel et al., 2015). Layering residual in-season has not been frequently evaluated for giant ragweed; however, Spaunhorst et al. (2014) evaluated addition of fomesafen and cloransulam-methyl with EPOST treatments and reported minimal improvement in control over glyphosate alone POST (all >90% control). It is important to note the giant ragweed biotype evaluated by Spaunhorst et al. (2014) had a short monophasic emergence pattern. A layered residual approach for giant ragweed control could have more utility for biotypes exhibiting the prolonged biphasic emergence pattern observed herein. Furthermore, acetochlor is not effective on large-seeded broadleaf weeds (e.g., does not suppress giant ragweed). More research is needed to evaluate other residual herbicides (i.e., cloransulam-methyl) as part of a layered residual approach for control of giant ragweed.
Giant Ragweed Seed Production, Viability, and Viable Seed Production
The average giant ragweed seed production in the NTC and PRE-only was 7,490 (± 2,210) and 3,870 (± 1,110) seeds m−2, at Janesville (data not shown). There was a POST timing by layered residual interaction among PRE fb POST treatments for giant ragweed seed production at Janesville (P < 0.0001; Table 5). The EPOST treatment without layered residual had the greatest amount of total giant ragweed seed production when compared with other POST treatments, fb the EPOST treatment with layered residual (Figure 1E). All MPOST and LPOST treatments resulted in similar amounts of total giant ragweed seed production (<150 seeds m−2), regardless of level of layered residual (Figure 1E). Giant ragweed seed viability was not influenced by POST timing (P = 0.3381; Table 5) or layered residual (P = 0.9729; Table 5). There was a POST timing by layered residual interaction for viable giant ragweed seed production at Janesville (P = 0.00537; Table 5) where treatment means closely followed the trends reported above for total seed production.
Soybean Yield
Soybean grain yield in Janesville for the NTC and PRE-only treatments was 420 (± 200) and 710 (± 270) kg ha−1, respectively (data not shown). All PRE fb POST treatments had average yield higher than 3,000 kg ha−1, indicating a clear increase compared with NTC and PRE-only treatments. Soybean grain yield was influenced by POST timing at Janesville (P = 0.0480; Table 5). Yield was similar for the MPOST and LPOST treatments; yield was lower for the LPOST compared with the EPOST treatment (Figure 1F). Prolonged competition between the weed population and the crop for the delayed POST application treatments likely contributed to the yield reduction observed for LPOST treatments (Figures 1B–D).
Brooklyn
Waterhemp Control and Density Reduction
Flumioxazin applied PRE provided 11% waterhemp control and 86% waterhemp density reduction 28 d after the LPOST treatment (data not shown). Total waterhemp emergence was lower for the PRE-only treatment compared to the NTC at Brooklyn (P < 0.0001). At Brooklyn, 15% of waterhemp had yet to emerge after the EPOST treatment while 100% cumulative emergence was attained by the LPOST treatment (Figure 2B). Control of waterhemp was influenced by POST timing at Brooklyn (P < 0.0001; Table 5). Adequate rainfall for layered residual activation occurred within 10 d after each POST treatment at Brooklyn (data not shown). Waterhemp control was lowest for the EPOST treatment compared to MPOST and LPOST treatments for which waterhemp control did not differ (Figure 2C). The EPOST treatment occurred at <80% cumulative waterhemp emergence, while MPOST and LPOST treatments occurred at higher than 95% cumulative emergence (Figure 2B). None of the PRE fb POST treatments were able to attain complete waterhemp control at Brooklyn. Numerous waterhemp escapes the previous cropping year in soybean and heavy rainfall that occurred after planting, contributing to poor soybean emergence and slow/incomplete canopy closure, are two factors that likely influenced the heavy waterhemp pressure observed (end of season waterhemp density for NTC = 452 plants m−2, data not shown).
End-of-season waterhemp density in Brooklyn for the NTC and PRE-only treatments was 452 (±41) and 62 (±15) plants m−2, respectively (data not shown). There was a POST timing by layered residual interaction for waterhemp density reduction at Brooklyn (P < 0.0001; Table 5). Waterhemp density reduction was lowest for the EPOST treatment without layered residual. The EPOST and MPOST treatments with layered residual and the LPOST treatment without layered residual all showed similar levels of density reduction (Figure 2D). All MPOST and LPOST treatments provided higher than 95% waterhemp density reduction, regardless of level of layered residual. As described above, a flush of waterhemp emerged following EPOST treatment; however, the layered residual did not similarly affect control (Figure 2D). This suggests a layered residual approach may be effective at reducing plant density when sprayed earlier (24 DAP) rather than later (>35 DAP) in the season, which is supported by recommendations for this approach (Norsworthy et al., 2012; Hartzler and Anderson, 2019). Steckel et al. (2002) reported that utilizing either acetochlor, S-metolachlor, or dimethenamid as part of a layered residual PRE fb POST approach in corn improved control of common waterhemp 56 DAP. Similarly, Sarangi and Jhala (2018) observed improved Palmer amaranth control in soybean with a PRE fb POST plus layered residual program, averaged across VLCFA herbicides. Conversely, Spaunhorst et al. (2014) reported PRE flumioxazin plus chlorimuron fb EPOST dicamba plus glyphosate with and without acetochlor as a layered residual provided similar levels of waterhemp control, which corroborates our findings. Product selection likely influences the efficacy of the layered residual approach for distinct weed species compositions. For instance, Hay et al. (2018) reported that encapsulated acetochlor applied PRE provided 2–14% and up to 45% lower control of waterhemp and Palmer amaranth, respectively, compared with other VLCFA herbicides (e.g., S-metolachlor, dimethenamid-P, and pyroxasulfone), pooled across three rates tested.
Waterhemp Seed Production
Waterhemp seed production in NTC and PRE-only treatments was 511,000 (± 134,000) and 314,000 (± 173,000) seeds m−2, respectively (P = 0.404; data not shown). While effective in-season weed control should reduce weed seed production, weed seedbank response to herbicide treatment is infrequently evaluated (Norsworthy et al., 2018). Preventing weeds from reaching maturity and producing viable seed can minimize seed spread and seedbank deposits (Hill et al., 2016). Seed production was lower for all PRE fb POST treatments when compared with NTC treatment (P < 0.05), with exception of the EPOST treatment without layered residual (P = 0.094). Moreover, waterhemp seed production differed among POST timings at Brooklyn (P = 0.006; Table 5). Waterhemp plant density was greater in EPOST treatments compared to MPOST and LPOST treatments; therefore, higher estimated seed production was expected for the EPOST treatments. The highest waterhemp seed production was in the EPOST treatments; the MPOST and LPOST treatments produced similar levels of total waterhemp seed production (Figure 2E). Thus, the treatment producing the highest amount of waterhemp seed was the EPOST without layered residual.
Soybean Yield
Soybean grain yield in Brooklyn for the NTC and PRE-only treatments was 940 (±80) and 1,500 (±110) kg ha−1, respectively (data not shown). Soybean grain yield was influenced by POST timing at Brooklyn (P = 0.026; Table 5). At Brooklyn in 2019, the EPOST treatment was the lowest yielding, while yield for the MPOST and LPOST treatments was similar, pooled across levels of layered residual (Figure 2F). Following the EPOST treatment, a flush of waterhemp emerged (Figures 2B–D); therefore, adequate weed interference was likely present to reduce yield. Although waterhemp density reduction 28 d after LPOST was higher at the EPOST treatment with layered residual there was no difference in soybean yield amongst levels of layered residual at the EPOST treatment.
Arlington and Lancaster
Other Broadleaf Control and Density Reduction
Flumioxazin applied PRE was effective at suppressing establishment of many weed species present across sites (i.e., common lambsquarters, common ragweed) as has been reported by Oliveira et al. (2017). Flumioxazin applied PRE provided >80% control of common lambsquarters (97% control, 96% density reduction) and common ragweed (82% control, 82% density reduction) 28 d after the LPOST treatment at Arlington and Lancaster, respectively (data not shown). Total emergence of common ragweed and common lambsquarters was lower for the PRE-only treatment compared to the NTC at Arlington (P = 0.038) and Lancaster (P = 0.026), respectively (data not shown). At Arlington and Lancaster, nearly 100% cumulative weed emergence had occurred prior to EPOST (Table 6). No interaction nor main effects were significant for the response variables at these two sites (P > 0.05; Table 5). Adequate rainfall for layered residual activation occurred within 10 d after each POST timing, except for the two later POST treatments at Arlington in 2018 (3.6 and 0-mm rainfall, respectively; data not shown). All PRE fb POST treatments resulted in 99% common ragweed control and higher than 93% common ragweed density reduction (Table 7). Results were similar for common lambsquarters. This indicates POST timing of dicamba plus glyphosate and use of a layered residual approach may not be as influential for easier to control species that have not evolved resistance to herbicides or difficult-to-control broadleaf weeds (i.e., waterhemp, giant ragweed) present in lower densities compared with higher densities. While all weeds were controlled regardless of POST timing and weed ht, the recommended practice is to spray before weed ht exceeds the label-recommended 10-cm in order to optimize product performance and reduce selection pressure (Anonymous, 2020a,b).
Other Broadleaf Seed Production
Complete weed control was attained at both sites (Table 7), thus, no common ragweed or common lambsquarters plants produced seed in PRE fb POST treatments.
Soybean Yield
Soybean grain yield for the NTC was 1,890 (±480) and 3,600 (±160) kg ha−1 and 3,350 (±190) and 4,610 (±60) kg ha−1 for the PRE-only treatments at Arlington and Lancaster, respectively (data not shown). Soybean grain yield was not influenced at either site by POST timing (P = 0.7811 at Arlington and 0.3151 at Lancaster) or layered residual (P = 0.3077 at Arlington and 0.8918 at Lancaster; Tables 5, 7).
Summary
Our findings indicate a PRE fb DGA+VG plus glyphosate applied MPOST (V3-V4, 32-43 DAP), not EPOST as we had hypothesized, optimized season-long weed control, reduced seed-producing escapes, and protected yield potential for locations with difficult to control broadleaf weed species in southern Wisconsin. Herbicides applied MPOST compared to LPOST may also serve as a proactive approach toward reducing potential for off-target movement as weather conditions are generally more favorable for dicamba application earlier in the growing season (i.e., lower temperature; Striegel et al., 2020). In further contrast of our hypothesis, the addition of acetochlor POST as a layered residual did not consistently improve weed control or reduce weed seed production, except for Brooklyn 2019. Although this finding is for only one site-year, it corroborates the findings of Spaunhorst et al. (2014) that waterhemp control was not improved with the addition of acetochlor POST. Weed community composition is an important consideration for appropriate herbicide selection; though this is a recommended approach for management of small-seeded annual broadleaf (i.e., Amaranthus spp.) and grass species, addition of a layered residual herbicide POST may not always be necessary, thus reducing program costs and environmental impact. The inability to attain complete control at site-years with predominantly broadleaf weed communities despite use of a two-pass PRE fb POST herbicide program with multiple SOA supports the need for inclusion of integrated weed management practices in addition to chemical control measures in present and future weed management programs. Moreover, dicamba could have provided short residual activity on broadleaf species as has been reported previously (Johnson et al., 2010; Underwood et al., 2017), thus concealing the value of the layered residual approach with acetochlor in this research. More research is needed to understand the benefit of the layered residual approach across residual herbicide chemistries and distinct weed communities.
Data Availability Statement
The raw data supporting the conclusions of this article will be made available by the authors, without undue reservation.
Author Contributions
SS conducted research, analysis, and writing. MO supervised analysis and writing. RD supervised research. DS and SC supervised analysis and writing. RW supervised research, analysis, and writing. All authors contributed to the article and approved the submitted version.
Funding
This study received funding from Bayer Crop Science. The funder was not involved in the study design, collection, analysis, interpretation of data, the writing of this article or the decision to submit it for publication.
Conflict of Interest
The authors declare that the research was conducted in the absence of any commercial or financial relationships that could be construed as a potential conflict of interest.
Publisher's Note
All claims expressed in this article are solely those of the authors and do not necessarily represent those of their affiliated organizations, or those of the publisher, the editors and the reviewers. Any product that may be evaluated in this article, or claim that may be made by its manufacturer, is not guaranteed or endorsed by the publisher.
Acknowledgments
The authors would like to thank the staff at Arlington ARS, Lancaster ARS, Rock County Farm, and O'Brien Farms, Daniel Smith, and the University of Wisconsin-Madison Cropping Systems Weed Science research group for their technical assistance in establishing field studies.
References
Abraham, W.. (2018). The Chemistry Behind Low-Volatility Dicamba. St. Louis, MO: Bayer Crop Science.
Anonymous (2016). Valor SX Herbicide Product Label. EPA Registration No. 59639-99. Walnut Creek, CA: Valent.
Anonymous (2020a). Engenia herbicide product label. EPA Registration No. 7969-472. Research Park, NC: BASF.
Anonymous (2020b). XtendiMax With VaporGrip Technology Herbicide Product Label. EPA Registration No. 264-1210. St. Louis, MO: Bayer CropScience LP.
Arsenijevic, N., de Avellar, M., Butts, L., Arneson, N. J., and Werle, R. (2020). Influence of sulfentrazone and metribuzin applied preemergence on soybean development and yield. Weed Technol. 35, 210–215. doi: 10.1017/wet.2020.99
Bates, D., Maechler, M., Bolker, B., and Walker, S. (2015). Fitting linear mixed-effects models using lme4. J. Stat. Softw. 67, 1–48. doi: 10.18637/jss.v067.i01
Behrens, M., Mutlu, N., Chakraborty, S., Dumitru, R., Jiang, W. Z., LaVallee, B. J., et al. (2007). Dicamba resistance: enlarging and preserving biotechnology-based weed management strategies. Science 316, 1185–1188. doi: 10.1126/science.1141596
Blouin, D. C., Webster, E. P., and Bond, J. A. (2011). On the analysis of combined experiments. Weed Technol. 25, 165–169. doi: 10.1614/WT-D-10-00047.1
Brooks, M. E., Kristensen, K., van Benthem, K. J., Magnusson, A., Berg, C. W., Nielsen, A., et al. (2017). glmmTMB balances speed and flexibility among packages for zero-inflated generalized linear mixed modeling. R. J. 9, 378–400. doi: 10.32614/RJ-2017-066
Carmer, S. G., Nyquist, W. E., and Walker, W. M. (1989). Least significant differences for combined analyses of experiments with two- or three-factor treatment designs. Agron. J. 81, 665–672. doi: 10.2134/agronj1989.00021962008100040021x
Clay, S. A., Kleinjan, J., Clay, D. E., Forcella, F., and Batchelor, W. (2005). Growth and fecundity of several weed species in corn and soybean. Agron. J. 97, 294–302. doi: 10.2134/agronj2005.0294a
Correndo, A. A., Moro Rosso, L. H., and Ciampitti, I. A. (2021). Agrometeorological Data Using R-Software. Harvard Dataverse, V5.
EPA (2020). US Environmental Protection Agency. Registration of Dicamba for Use on Dicamba-Tolerant Crops. Ingredients used Pestic. Prod. Available online at: https://www.epa.gov/ingredients-used-pesticide-products/registration-dicamba-use-dicamba-tolerant-crops (accessed November 9, 2020).
Fickett, N. D., Boerboom, C. M., and Stoltenberg, D. E. (2013). Soybean yield loss potential associated with early-season weed competition across 64 site-years. Weed Sci. 61, 500–507. doi: 10.1614/WS-D-12-00164.1
Fox, J., and Weisberg, S. (2019). An {R} Companion to Applied Regression, 3rd Edn. Thousand Oaks, CA: Sage.
Glettner, C. E., and Stoltenberg, D. E. (2015). Noncompetitive growth and fecundity of Wisconsin giant ragweed resistant to glyphosate. Weed Sci. 63, 273–281. doi: 10.1614/WS-D-14-00040.1
Goplen, J., Behnken, L., Miller, R., and Stahl, L. (2017). “Back to the basics: integrating weed biology into weed management plans,” in Proceedings of the 29th Annual Integrated Crop Management Conference (Ames, IA), 63–67. doi: 10.31274/icm-180809-239
Gross, J., and Ligges, U. (2015). nortest: Tests for Normality. R package version 1.0-4. Available online at: https://CRAN.R-project.org/package=nortest (accessed March 20, 2020).
Hartzler, R. G., and Anderson, M. (2019). Achieving Full-Season Waterhemp Control in Soybean. Integrated Crop Management News. 2514. Ames, IA.
Hay, M. M., Shoup, D. E., and Peterson, D. E. (2018). Palmer amaranth (Amaranthus palmeri) and common waterhemp (Amaranthus rudis) control with very-long-chain fatty acid inhibiting herbicides. Crop. Forage Turfgrass Manag. 4, 1–9. doi: 10.2134/cftm2018.05.0035
Hedges, B. K., Soltani, N., Hooker, D. C., Robinson, D. E., and Sikkema, P. H. (2018). Influence of glyphosate/dicamba application rate and timing on the control of glyphosate-resistant horseweed in glyphosate/dicamba-resistant soybean. Weed Technol. 32, 678–682. doi: 10.1017/wet.2018.72
Heneghan, J. M., and Johnson, W. G. (2017). The growth and development of five waterhemp (Amaranthus tuberculatus) populations in a common garden. Weed Sci. 65, 247–255. doi: 10.1017/wsc.2016.20
Hill, E. C., Renner, K. A., VanGessel, M. J., Bellinder, R. R., and Scott, B. A. (2016). Late-season weed management to stop viable weed seed production. Weed Sci. 64, 112–118. doi: 10.1614/WS-D-15-00096.1
Johnson, B., Moechnig, M., Steckel, L., Matthews, J., Smeda, R., Al-Khatib, K., et al. (2010). Weed control in dicamba-resistant soybeans. Crop Manag. 9, 1–23. doi: 10.1094/CM-2010-0920-01-RS
Kaur, S., Lowell, L. D., Lindquist, J. L., and Jhala, A. J. (2014). Glyphosate-resistant giant ragweed (Ambrosia trifida) control in glufosinate-resistant soybean. Weed Technol. 28, 569–577. doi: 10.1614/WT-D-14-00009.1
Knezevic, S. Z., Evans, S. P., and Mainz, M. (2003). Yield penalty due to delayed weed control in corn and soybean. Online. Crop Manag. 2, 1–7. doi: 10.1094/CM-2003-0219-01-RS
Knezevic, S. Z., Pavlovic, P., Osipitan, O. A., Barnes, E. R., Beiermann, C., Oliveira, M. C., et al. (2019). Critical time for weed removal in glyphosate-resistant soybean as influenced by preemergence herbicides. Weed Technol. 33, 393–399. doi: 10.1017/wet.2019.18
Lenth, R.. (2020). emmeans: Estimated Marginal Means, aka Least-Squares Means. R package version 1.4.7. Available online at: https://CRAN.R-project.org/package=emmeans (accessed March 20, 2020).
MacInnes, A.. (2016). “VaporGrip technology; how it works and its benefits,” in Proceedings of the 71st Annual Meeting of the North Central Weed Science Society (Des Moines, IA: North Central Weed Science Society) 85.
Mulugeta, D., and Boerboom, C. M. (2000). Critical time of weed removal in glyphosate-resistant Glycine max. Weed Sci. 48, 35–42. doi: 10.1614/0043-1745(2000)048[0035:CTOWRI]2.0.CO;2
Norsworthy, J. K., Korres, N. E., and Bagavathiannan, M. V. (2018). Weed seedbank management: revisiting how herbicides are evaluated. Weed Sci. 66, 415–417. doi: 10.1017/wsc.2018.11
Norsworthy, J. K., Ward, S. M., Shaw, D. R., Llewellyn, R. S., Nichols, R. L., Webster, T. M., et al. (2012). Reducing the risks of herbicide resistance: best management practices and recommendations. Weed Sci. 60, 31–62. doi: 10.1614/WS-D-11-00155.1
Oliveira, M. C., Feist, D., Eskelsen, S., Scott, J. E., and Knezevic, S. Z. (2017). Weed control in soybean with preemergence- and postemergence-applied herbicides. Crop. Forage Turfgrass Manag. 3, 1–7. doi: 10.2134/cftm2016.05.0040
Potratz, D. J., Mourtzinis, S., Gaska, J., Lauer, J., Arriaga, F. J., and Conley, S. P. (2020). Strip-till, other management strategies, and their interactive effects on corn grain and soybean seed yield. Agron. J. 112, 72–80. doi: 10.1002/agj2.20067
Proost, R. T., Heider, D. J., and Boerboom, C. M. (2006). “Wisconsin farmers take the 2-pass challenge against weeds,” in Proceedings of the 18th Annual Integrated Crop Management Conference (Ames, IA), 129–133. doi: 10.31274/icm,-180809-853
R Core Team (2020). R: A Language and Environment for Statistical Computing. R Foundation for Statistical Computing, Vienna, Austria. Available online at: https://www.r-project.org/ (accessed June 17, 2020)
Ritz, C., Baty, F., Strebig, J. C., and Gerhard, D. (2015). Dose-response analysis using R. PLoS ONE 10:e0146021. doi: 10.1371/journal.pone.0146021
Ross, M. A., and Lembi, C. A. (2008). Applied Weed Science: Including the Ecology and Management of Invasive Plants. 3rd Edn. Upper Sadle River, NJ: Pearson Education, Inc.
Sarangi, D., and Jhala, A. J. (2018). Palmer amaranth (Amaranthus palmeri) and velvetleaf (Abutilon theophrasti) control in no-tillage conventional (non–genetically engineered) soybean using overlapping residual herbicide programs. Weed Technol. 33, 95–105. doi: 10.1017/wet.2018.78
Schutte, B. J., Regnier, E. E., and Harrison, S. K. (2012). Seed dormancy and adaptive seedling emergence timing in giant ragweed (Ambrosia trifida). Weed Sci. 60, 19–26. doi: 10.1614/WS-D-11-00049.1
Schutte, B. J., Regnier, E. E., Harrison, S. K., Schmoll, J. T., Spokas, K., and Forcella, F. (2008). A hydrothermal seedling emergence model for giant ragweed (Ambrosia trifida). Weed Sci. 56, 555–560. doi: 10.1614/WS-07-161.1
Spaunhorst, D. J., Siefert-Higgins, S., and Bradley, K. W. (2014). Glyphosate-resistant giant ragweed (Ambrosia trifida) and waterhemp (Amaranthus rudis) management in dicamba-resistant soybean (Glycine max). Weed Technol. 28, 131–141. doi: 10.1614/WT-D-13-00091.1
Sprague, C., Wax, L., Hartzler, R., and Harrison, S. (2004). “Variations in emergence patterns of giant ragweed biotypes from Ohio, Illinois, and Iowa,” in Proceedings of the –th Annual Meeting of the Weed Science Society of America: City, State.
Steckel, L. E., Sprague, C. L., and Hager, A. G. (2002). Common waterhemp (Amaranthus rudis) control in corn (Zea mays) with single preemergence and sequential applications of residual herbicides. Weed Technol. 16, 755–761. doi: 10.1614/0890-037X(2002)016[0755:CWARCI]2.0.CO;2
Striegel, S., Oliveira, M. C., Arneson, N., Conley, S. P., Stoltenberg, D. E., and Werle, R. (2020). Spray solution pH and soybean injury as influenced by synthetic auxin formulation and spray additives. Weed Technol. 35, 113–127. doi: 10.1017/wet.2020.89
Thornton, M. M., Shrestha, R., Wei, Y., Thornton, P. E., Kao, S., and Wilson, B. E. (2020). Daymet: Daily Surface Weather Data on a 1-km Grid for North America, Version 4. ORNL DAAC, Oak Ridge, TN, USA.
Underwood, M. G., Soltani, N., Hooker, D. C., Robinson, D. E., Vink, J. P., Swanton, C. J., et al. (2017). Benefit of tank mixing dicamba with glyphosate applied after emergence for weed control in dicamba- and glyphosate-resistant soybean. Can. J. Plant Sci. 97, 891–901. doi: 10.1139/C.J.P.S.-2016-0292
USDA-NASS (2019). Department of Agriculture-National Agricultural Statistics Service. Quick Stats. Available online at: https://quickstats.nass.usda.gov/ (accessed January 23, 2020).
Vink, J. P., Robinson, D. E., Lawton, M. B., Soltani, N., Tardif, F. J., and Sikkema, P. H. (2012). Glyphosate-resistant giant ragweed (Ambrosia trifida) control in dicamba-tolerant soybean. Weed Technol. 26, 422–428. doi: 10.1614/WT-D-11-00184.1
Werle, R., and Oliveira, M. (2018). 2018 Wisconsin Cropping Systems Weed Science Survey - Where Are We At? Wisconsin Crop. Syst. Weed Sci. Available online at: http://www.wiscweeds.info/post/2018-wisconsin-cropping-systems-weed-science-survey/ (accessed April 9, 2020).
Werle, R., Oliveira, M. C., Jhala, A. J., Proctor, C. A., Rees, J., and Klein, R. (2018). Survey of Nebraska farmers' adoption of dicamba-resistant soybean technology and dicamba off-target movement. Weed Technol. 32, 754–761. doi: 10.1017/wet.2018.62
Werle, R., Sandell, L. D., Buhler, D. D., Hartzler, R. G., and Lindquist, J. L. (2014). Predicting emergence of 23 summer annual weed species. Weed Sci. 62, 267–279. doi: 10.1614/WS-D-13-00116.1
Keywords: synthetic auxin, weed management, soil seedbank, growth stage, residual herbicide
Citation: Striegel S, Oliveira MC, DeWerff RP, Stoltenberg DE, Conley SP and Werle R (2021) Influence of Postemergence Dicamba/Glyphosate Timing and Inclusion of Acetochlor as a Layered Residual on Weed Control and Soybean Yield. Front. Agron. 3:788251. doi: 10.3389/fagro.2021.788251
Received: 01 October 2021; Accepted: 24 November 2021;
Published: 17 December 2021.
Edited by:
Ali Ahsan Bajwa, New South Wales Department of Primary Industries, AustraliaReviewed by:
Hossein Ghanizadeh, Massey University, New ZealandAbdul Khaliq, University of Agriculture, Faisalabad, Pakistan
Eshagh Keshtkar, Tarbiat Modares University, Iran
Copyright © 2021 Striegel, Oliveira, DeWerff, Stoltenberg, Conley and Werle. This is an open-access article distributed under the terms of the Creative Commons Attribution License (CC BY). The use, distribution or reproduction in other forums is permitted, provided the original author(s) and the copyright owner(s) are credited and that the original publication in this journal is cited, in accordance with accepted academic practice. No use, distribution or reproduction is permitted which does not comply with these terms.
*Correspondence: Rodrigo Werle, rwerle@wisc.edu