Site Logistics Factors Impacting Resource Use on Construction Sites: A Delphi Study
- Texas A&M University, College Station, TX, United States
Over 48% of the world’s energy is consumed by buildings in their construction and operation alone, which add to over 39% of global carbon emissions. In addition, the building sector generates over 569 million tons of waste every year within just the United States. Reducing the environmental impacts and construction waste generated by buildings has, therefore, become a concerning global issue. Very few studies have addressed how site logistics planning may impact construction resource use on a site and how it may affect a project’s embodied energy. Site logistics plans control the sequencing of labor, materials, and equipment. Inefficient plans may lead to rework, material damage, and waste generation, requiring additional materials to complete the same task, which eventually increases the embodied energy of a project. In this study, we investigated, identified, and ranked site logistics-related factors that may affect the amount of resources used in a construction project. The Delphi Method is applied to determine, verify, and rank these factors to help improve existing methods of site logistics planning from an embodied energy perspective. Results show that the installer’s skill, technology/equipment, prefabrication, planning and forecasting, and material movement are among the top influential site logistics-related factors that may help reduce construction waste. Considering these factors while developing the site logistics plan will help lower the energy and carbon footprint of a construction project.
Introduction
A major portion of the world’s natural resources (48%) is consumed by buildings during their life span, making the construction industry a main target for the global sustainability agenda (Cabeza et al., 2014). Therefore, building professionals are constantly looking for ways to construct energy-efficient buildings, while attempting to lower cost and maintain quality. Commonly used approaches to improve the energy performance of a building involve changing building design features (e.g., material choices, fenestration areas, interior layouts, etc.) or installing energy-efficient systems (Venkatraj et al., 2020). Literature shows that efforts to reduce energy consumption predominantly focus on the design and operation phase of a project, while the energy expenditure that occurs during the construction and demolition phase is seldom considered. More specifically, not much emphasis is given to evaluating environmental impacts caused by improper construction planning (Formoso et al., 1999).
Construction managers are responsible for managing resources such as construction material, labor, and equipment; their proper sequencing is key for construction planning. Ultimately, the success of a project critically depends on construction planning, since it provides a set of guidelines to construct a quality building on time, within budget, and without safety incidents. In most cases, the lack of proper planning and management generates massive amounts of construction waste (Formoso et al., 1999; Ajayi et al., 2017). According to the Environmental Protection Agency (EPA, 2017), nearly 569 million tons of construction and demolition (C&D) waste was created in 2017 in the United States. On a yearly basis, the magnitude of C&D waste generated is greater than twice the amount of municipal solid waste (MSW) generated. MSW, or trash, comprises everyday commodities, articles, or items that consumers usually discard (e.g., food waste, packaging waste, electronic waste, yard trimmings, etc.). Most MSW definitions do not include industrial, medical, or C&D waste (EPA, 2017). Therefore, the efficiency with which construction resources are transported, handled, stored, and used on the construction site may influence a project’s energy consumption, as each resource contains embodied energy (EE). EE represents the sum of direct and indirect energy used in construction materials and processes during the construction of a building (Dixit, 2017). During the process of initial construction, EE is used directly in the processes of onsite construction, transportation, fabrication, and administration, and indirectly through building materials, assemblies, and equipment (Dixit, 2017). The EE used in initial construction is termed initial embodied energy (IEE). On average, over 90% of the IEE can be attributed to building materials (Dixit, 2017). Because construction planning governs how well construction tasks have been planned and managed, it influences this resource-based energy consumption (IEE) on construction sites.
Nowadays, owners are interested not only in the schedule and cost of construction projects but also in the overall environmental performance and life cycle management of a building. To improve energy performance during the construction phase, we need to holistically reduce EE, specifically IEE. In this study, we identify site logistics factors that influence material usage on construction sites, eventually affecting the IEE of construction projects. We applied the Delphi method to determine, verify, and rank these site logistics-related factors to help improve existing methods of site logistics planning from an energy perspective. The results of the study would potentially help understand how site logistics planning could be geared towards reducing resource use to enhance the fields of Life Cycle Assessment (LCA) of buildings and lean construction. The results may also enable developing computational algorithm to optimize a site logistics plan for reduced material damage, rework, and construction waste.
The manuscript is organized in seven main sections. The first section of Background offers the current state of research to understand a need to identify factors that may influence and cause the problem of material damage, rework, and waste on a construction site. This section leads to the Problem Statement and Research Goal section that explains the main goal and research objectives to address this problem. Section 4 of Research Methods provides a step-by-step process from identifying an initial list of site logistics factors through literature review, selecting participants, to completing Delphi iterations. Results of the study are presented in the fifth section and discussed in the sixth section of Discussion. Finally, key conclusions and future research directions are offered in the seventh section.
Background
Site Logistics
Logistics deals with the overall process of planning, coordinating, and executing several tasks related to the purchase, supply, storage, transportation, maintenance, and handling of resources such as materials, labor, and equipment (Regassa, 2015; Magill et al., 2020). Implementing this idea on a construction site requires great skill and a deep knowledge base. In construction, logistics mainly refers to the movement of materials and construction equipment on-site. Additional factors that play an important role in efficiently managing construction resources on-site include material procurement, handling, and storage (Tunji-Olayeni et al., 2017; Misron et al., 2018). A successful site logistics plan needs significant amounts of pre-planning and coordination between various trades and is, therefore, considered a complicated task (Tunji-Olayeni et al., 2017). Inefficient site logistics plans generate large amounts of waste and material losses, subsequently leading to time and cost overruns (Tunji-Olayeni et al., 2017; Misron et al., 2018). Ogundipe et al. (2020) identified 25 factors that hamper the full exploitation of the benefits of effective building materials management (EBMM), most of which relate to improper material handling and inefficient site logistics. Owolabi et al. (2021) also discussed how improper material management through poor construction site logistics planning could produce not just material waste but also time and cost overruns.
Construction Site Layout Planning
Construction site layout planning (CSLP) is a subset of project planning. An optimal CSLP improves project productivity and the level of safety on a construction site. Several practitioners and researchers, therefore, believe that an effective CSLP is of great importance to finish a construction project successfully (Ning et al., 2010; Misron et al., 2018; Magill et al., 2020). As we already know, because construction activities are usually performed in sequential or parallel stages, the late completion of a construction task can affect the start time of the next task. For example, poor weather conditions, moving equipment/labor, improper packaging, using improper equipment to offload material, poor staging conditions, or improper staging placement, may damage construction materials stored on-site (Ying et al., 2014; Misron et al., 2018). As a result, the material may require reordering, thus not being available for the workforce in a timely fashion. This highlights the importance of properly managing material logistics on a construction site (Misron et al., 2018; Owolabi et al., 2021). The field of lean construction directly connects CSLP to material and resource use, thereby linking CSLP to a construction project’s environmental impacts (Ghanem et al., 2018; Francis and Thomas, 2020). Utilizing innovative lean construction techniques such as just in time (JIT) to develop an efficient material logistics strategy minimizes the loss of material, time, and cost (Francis and Thomas, 2020). The JIT system was promoted in the early 1950s by the Toyota Motor Corporation to improve productivity in the manufacturing sector. Cars manufactured using this system were of better quality, highly reliable, and reduced the costs of maintaining inventory (Pheng and Hui, 1999). In fact, unnecessary and excessive use of resources such as material, equipment, and labor due to poor and inefficient site logistics layout and planning is considered a type of waste in lean construction theory (Francis and Thomas, 2020). Studies such as Lota and Trivedi (2020) connected lean construction and resulting environmental benefits to site logistics planning and layout and explored Building Information Modeling (BIM) to visualize and model space and route constraints, safety, and progress monitoring and reporting to reduce different type of waste defined by the lean construction theory. Some studies (e.g., Tetik et al., 2021) explored emerging lean constructs of material kitting to simplify construction site logistics, particularly relating to material handling and generate savings of materials as well as time and cost. Studies (e.g., Misron et al., 2018; Owolabi et al., 2021; Popoola et al., 2018) suggest that using a similar system as JIT to manage material logistics on construction sites would be extremely beneficial. An effective CSLP helps practitioners determine the set of activities that must occur in a certain sequence to avoid disrupting on-site work. This allows the project team to efficiently and safely maneuver people, mobilize equipment, and store/transport material. In addition, the CSLP also enables the project team to critically evaluate current issues and forecast the projects’ progress (Ning et al., 2011). A CSLP, therefore, may impact not just the use and waste of construction resources but IEE as well. Studies such as Whitlock et al. (2021) and Magill et al. (2020) explored how 3D and 4D Building Information Modeling (BIM) can help computationally optimized construction logistics management (CLM) to gain efficiencies of material handling, equipment use, and labor productivity, all of which can help save resources potentially leading to saving embodied impacts. The planning, layout, and management of construction site logistics can significantly affect the onsite use of construction resources (Popoola et al., 2018).
Rework in Construction Projects
Rework refers to re-doing a specific construction task that was previously carried out in an incorrect manner (Mahamid, 2020). The incidence of rework in construction projects is inevitably associated with cost and time overruns (Love et al., 2002; Popoola et al., 2018; Luangcharoenrat et al., 2019). Previous research shows that the overall cost of rework is between 2 and 3% of the contract value (Love et al., 1999). The general causes of rework include lack of skilled labor, variability associated with missing or inconsistent information, non-compliance with specification requirements, lack of supervision, lack of efficient relationships between construction phases, and change to the project scope (Mall, 2019; Lota and Trivedi, 2020; Mahamid, 2020). It is important to note that no studies discuss the concept of rework or have a clear definition from an industrial engineering standpoint. Moreover, limited discourse exists concerning the techniques used to measure rework.
Construction Waste
Construction waste is caused by inefficient use of equipment, manpower, resources, or capital (Fini and Forsythe, 2020; Correia et al., 2021; Luangcharoenrat et al., 2019; Formoso et al., 1999). It is defined by the Building Research Establishment as “the difference between materials ordered and those placed for fixing on building project” (Al-Hajj and Hamani, 2011). This includes material losses and carrying out unnecessary construction tasks/rework, which increases the project cost but adds no real value to the final product (Formoso et al., 1999; Luangcharoenrat et al., 2019). This also adversely influences a construction project’s IEE. Al-Hajj and Hamani (2011) also discussed other wastes in terms of time and values; anything that does not add value to a project can be considered waste in lean construction (Lota and Trivedi, 2020). Over the last few decades, lean strategies frequently have been implemented to reduce or eliminate waste (Nahmens and Ikuma, 2012). Ohno and Bodek (1988) defined seven types of waste that lean construction has adopted: Overproduction, Conveyance, Inventory, Correction, Processing, Motion, and Waiting. Case studies on lean construction report these seven manifestations in the production of buildings. Koskela (2004) observed that the first five types of waste refer to the flow of material, while the remaining relate to human work. Discrete waste, that is, material waste, is classified by type, weight (Gavilan and Bernold, 1994), volume (Alwi et al., 2002; Ekanayake and Ofori, 2004; Luangcharoenrat et al., 2019), and cost (Love et al., 1999; Love and Li, 2000), and it exists at the task level (Fernandez-Solis and Rybkowski, 2015). Task refers to a construction-related activity, such as pouring a concrete foundation or installing plumbing systems. Task-level metrics are used by contractors to measure the output and time taken (labor hours) to complete a task. The National Research Council (National Research Council, 2009) states that task-level metrics are utilized to determine the workforce’s performance on a daily or weekly basis. These evaluations enable contractors to quickly identify and resolve issues on active projects, and ultimately, reduce the amount of waste generated. In a recent study, Luangcharoenrat et al. (2019) conducted a review of literature to identify 28 causes of construction waste under four categories, three of which (materials and procurement; construction methods and planning; and human resources) cover factors relating to site logistics. Magill et al. (2020) examined the role of 4D Building Information Modeling (BIM) in achieving integrated construction supply chain logistics (ICSCL) to avoid clashes of onsite material management, stage out areas, and plan and equipment management for not just time and quality efficiency but also environmental benefits.
Construction Waste Management
The construction industry is plagued by massive amounts of construction waste (CW) generated each year (Udawatta et al., 2015; Park and Tucker, 2017). According to the United Kingdom’s Department for Environment Food and Rural Affairs report (DEFRA, 2013), the construction industry contributes 44% of the waste that ends up in a landfill, whereas commercial and domestic industries generate waste as low as 14 and 13%, respectively. This huge proportion of construction waste has prompted governments around the world to implement waste management strategies that would reduce the generation of CW and prevent the “garbage siege” phenomenon (Tan, 2011). In addition, several legislative and fiscal provisions as well as substantial research efforts are focused on developing policies to help mitigate construction waste (Ajayi et al., 2017). Measures to reduce waste may include logistics management, supply chain management, modern construction methods, and training and incentivizing, most of which are related to site logistics planning (Al-Hajj and Hamani, 2011). Despite these efforts, the amount of waste generated by construction activities continuously increases (Ajayi et al., 2015). Ding et al. (2016) created a dynamic framework to effectively reduce and manage CW during the construction phase of a project. Their study showed that implementing the waste reduction framework reduced waste generated by nearly 27%. Few sources of CW generated on a construction site include material losses due to design modifications or detailing errors, packaging waste, and damaged materials. Waste minimization design (WMD) is a widely adopted strategy to holistically reduce waste (Baldwin et al., 2009). Al-Hajj and Hamani (2011) discussed the concept of advanced Material Logistics Plan to reduce waste on construction sites. Correia et al. (2021) explored barriers that impede the adoption of the Reverse Logistics (RL) process to minimize construction waste and enhance reuse and recycling for moving products back to the vendors or manufacturers. Likewise, Fini and Forsythe (2020) researched construction logistics-related barriers to reusing and recycling waste from office fit-out demolition.
Influence of Site Logistical Factors on Embodied Energy
To understand EE, one must first understand the concept of building life cycle energy (LCE). The total building LCE includes embodied and operating energy (Crowther, 1999; Ding, 2004; Venkatraj et al., 2020; Pradeep Kumar et al., 2022). EE is consumed during the process of construction, whereas operating energy is consumed to maintain thermal comfort within the building (Venkatraj et al., 2020; Pradeep Kumar et al., 2022). To elaborate further, EE is sequestered in all building materials/products as well as processes of procuring/mining raw materials, product manufacturing, construction, building maintenance during use, and final demolition (Dixit et al., 2010; Venkatraj et al., 2020; Pradeep Kumar et al., 2022). Buildings are constructed using different kinds of materials and each of these materials consumes energy during the life cycle phases of production, operation, and demolition. Based on these life cycle phases, EE can be segregated into initial embodied energy (IEE), recurrent embodied energy (REE), and demolition energy (DE) (Venkatraj and Dixit, 2021). IEE is consumed by the material/product manufacturing processes, including the mining of raw materials, production, fabrication, and transporting the final product to the construction site (Dixit et al., 2010; Dixit and Singh, 2018). REE is consumed by processes associated with building maintenance, repair, replacement, and refurbishment during their useful life (Dixit, 2019; Venkatraj and Dixit, 2021). DE is the net energy consumed to deconstruct the building and dispose of the waste (Dixit et al., 2010).
The IEE of a construction project consists of direct and indirect energy components (Dixit et al., 2010; Dixit, 2019). Direct energy is used by processes such as construction, manufacturing, product delivery, and administration, whereas indirect energy is consumed through construction materials/assemblies, or other building systems installed in the building. The management of resources on a construction site affects both the direct and indirect aspects of IEE. For example, proper construction planning can prevent 1) rework that requires additional energy in terms of labor, management, administration, etc (direct energy), and 2) the use of additional material (indirect energy) to complete a specific construction task (Popoola et al., 2018; Luangcharoenrat et al., 2019; Mall, 2019). Therefore, the efficient use of resources would help reduce the IEE of a construction project. In this paper, we study factors relating to site logistics that may influence IEE.
Problem Statement and Research Goals
Our review of the literature revealed that site logistics planning may influence the use of construction resources ultimately affecting IEE. However, there is little research on site logistics factors that may influence the use of construction materials responsible for over 90% of the IEE of a building. Such an investigation is essential to help understand how construction planning can be geared toward reducing construction resource use and the overall LCE of a construction project. The goal of this study is to enable IEE reduction by efficiently managing construction resources on a job site. This goal will be achieved by the following two research objectives:
• Identify factors relating to site logistics planning that may help reduce the amount of construction materials used in a project.
• Determine and analyze the rank of these factors in terms of their influence on onsite material and resource use.
• Discuss the implied relationship of these factors to construction projects’ embodied energy impacts, particularly the initial embodied energy (IEE).
Research Methods
This study was conducted in three stages: 1) literature review; 2) data collection using the Delphi method; and 3) data analysis as described in the subsequent sections.
Literature Review
To identify relevant literature, we first defined the research question and determined the scope of our study (Figure 1). Following this, keywords such as construction waste, waste minimization, material damage, construction rework, embodied energy, site logistics, material movement, Delphi method, etc., were entered into the Google Scholar search engine. In addition, we also used the Texas A&M University library and the ASCE library to collect related information. This search helped us collect peer-reviewed journal and conference papers, government reports, and other technical documents. Inclusion and exclusion criteria were developed by accounting for the research questions. We included studies that focus specifically on construction sites. Furthermore, the articles for the literature review were selected based on text availability, language, article type, and date of publication. As a result, articles focusing on time wastage, cost wastage, and design factors were excluded. Eventually, we shortlisted 13 relevant articles as described and listed in Table 1.
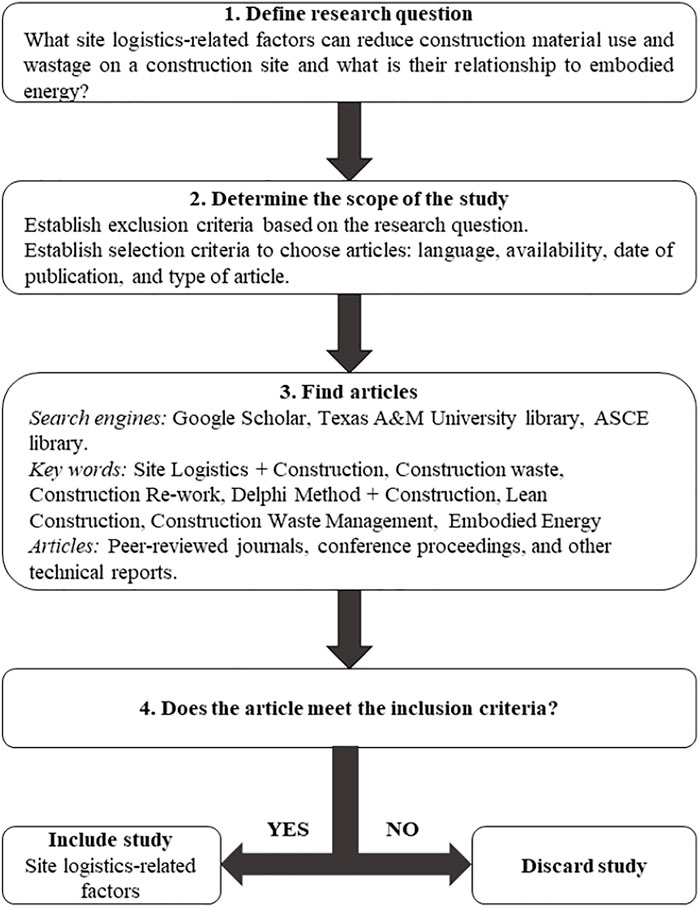
FIGURE 1. Systemic literature review framework (adopted from Hurwitz et al., 2016).
Data Collection Using Delphi Method
Delphi Method
This study applied the Delphi method to identify and rank site logistics planning factors that may influence EE. The Delphi method draws from expert opinions on a topic under study to seek consensus through an iterative process involving 2-3 rounds of discussions/data collection. To successfully achieve a reliable consensus, we engaged a panel of 28 experts through the Delphi technique. The expert panel consisted of construction professionals with varying levels of education, industry experience, and positions. In the context of this paper, the term “expert” indicates a person who is knowledgeable or skillful in the construction domain. These members participated in three rounds of a structured questionnaire survey. Due to limited time availability, the questionnaires were administered to the panelists via either email or telephonic interviews. The responses from the panelists were also collected using the same approach. After each round, the survey facilitator prepared a summary of participant responses collected in the round. The participants’ anonymous opinions were distributed before the next round of the survey to provide participants with the opportunity to refine and finalize their previous responses based on their review of the administered summary. The main intention of this process was to help participants reach consensus regarding the correct response. When using the Delphi technique, this process continues either until the completion of a certain number of rounds or a unanimous decision is made (Hallowell and Gambatese, 2010). Our literature review showed that very few studies in the construction practice domain have utilized the Delphi method. According to Sourani and Sohail (2015), the lack of awareness, proper guidelines, and detailed explanation of how the Delphi method operates impede its application in construction-related research. The following four steps were applied:
Step 1: Selecting Delphi Panelists: Potential participants with at least 5 years of industry experience from different construction companies were contacted through emails and phone calls and asked to participate in the two or more rounds of the Delphi process. The members of the panel were carefully selected based on their qualifications, years of professional experience, and varying backgrounds to ensure minimal bias. Hallowell and Gambatese (2010) developed an expert evaluation system that can be used to determine whether an individual can be qualified as a panel “expert.” According to their system, an individual must score a minimum of 11 points to qualify as an “expert.” We utilized a similar expert evaluation system to select qualified individuals to participate in our study; Table 2 lists selection criteria and points used to select the panel. The characteristics of the 28 Delphi expert panelists are presented in Table 3. Since the focus of the study was on construction sites, we limited the expert panel to industry professionals. While selecting participants, we assumed that panelists may not have a complete understanding of embodied energy. In other words, participants’ opinion was directly linking site logistics factors to material damage, rework, and waste. The linkage of material damage, rework, and waste to embodied energy was implied and not directly expressed by the participants.
Step 2: Determining the number of Delphi rounds: The purpose of administering several Delphi rounds is to reach a consensus amongst the members of the expert panel (Sourani and Sohail, 2015). This iterative approach of utilizing controlled feedback is known to improve the accuracy of the judgment (Hallowell and Gambatese, 2010). Our review of the literature did not provide conclusive guidelines to determine the optimal number of Delphi rounds. Although previous studies show that Delphi expert panelists often reach the desired consensus after either two or three rounds (Chan et al., 2001; Hallowell and Gambatese, 2010; Ameyaw et al., 2016), having three rounds helps collect different viewpoints as well as enough justifications for each response (Hallowell and Gambatese, 2010). Consequently, we conducted three Delphi rounds in this study.
Step 3: Determining the number of Delphi panelists: Existing literature does not offer significant direction for ascertaining the number of Delphi panelists. From the 66 construction-related research articles Ameyaw et al. (2016) reviewed, 41 studies involved 8 to 20 panelists. The main difference between the Delphi approach and other traditional statistical survey methods is that the former relies on responses given by experts in the field, whereas the latter utilizes a representative sample of the entire population. Since the Delphi technique considers expert opinion, it is considered to yield more accurate results in comparison to other statistical methods (Kirun and Varghese, 2015). In this study, the panel size decreased from 28 in Round I to 25 and 21 in Rounds II and III, respectively.
Step 4: Applying the Delphi method: Delphi method was applied in the following three rounds:
Round I: From the literature, we first identified a list of 9 site logistics factors that cause material waste and damage on construction job sites, and eventually influence IEE. We also conducted open-ended interviews with the experts to 1) include a broader perspective on the issue, and 2) improve the site logistics factors list. Eventually, we made no changes to the initial list of factors identified through the literature review. The panelists were asked for agreement on the 9 original choices, and for adding 4 additional factors, including installers’ skill, material movement, company culture, and finished material protection. These factors had the most frequent occurrence and were added to the original list, resulting in a total of 13 factors. This process took 27 days.
Round II: In this round, the panelists were asked to rank the level of importance of each site logistics factor based on their impact on material use and IEE. The questionnaire for Round II was developed based on the findings of the literature review and responses obtained in the first round. The questionnaire included two sections. The first section gathered demographic information such as professional background, gender, job title, and years of experience with the construction industry, whereas the second section collected the experts’ perspectives on the factors influencing site logistics planning. The survey was saved as a Word document and was administered using email and phone conversations. The experts participated voluntarily, and participant information collected during this survey process was kept confidential. Round II of the survey took 20 days to complete.
Round III: This round of the survey allowed the experts to reevaluate their responses from Round II. The questionnaire for this round was based on responses received in the second round. The feedback we received in Round II showed that it was impossible for the experts to differentiate the importance of the 13 factors. According to Miller’s law (1956), the limitations of average human memory allow an individual to compare only 7 ± 2 factors, simultaneously. This could be one reason the experts failed to distinguish the relative importance of the 13 factors. Accordingly, Round III was designed to investigate if the participants could reach consensus on the importance of a smaller number of factors. With this purpose in mind, the seven factors with the lowest mean ranks in Round II were selected for Round III. The participants were asked for agreement on these 7 factors being important site logistics factors. This round lasted for 15 days.
Data Analysis
The data obtained from the three Delphi rounds was analyzed to evaluate differences in the importance of the 13 site logistics planning factors. A Friedman Test was conducted to test the following null and alternative hypotheses:
H0∶ In the experts’ opinions, there is no difference in the importance of the 13 site logistics planning factors.
Ha∶ In the experts’ opinions, there is a difference in the importance of the 13 site logistics planning factors.
For a significance level of α (that is 0.05 in this study), if p-value ≤ α, the null hypothesis is rejected; otherwise, it is not rejected.
Results
Delphi Questionnaire: Round I
The 28 participants were asked to remove unimportant factors from the original list provided in the questionnaire. Participants could also add up to 4 additional factors to the list. Table 4 shows the number and percentage of participants who removed each factor from or added to the original list. Since none of the 9 original factors were removed by at least 20% of the participants, all were retained. The 4 factors added by the highest percentage of participants were installer skill (57%), material movement (43%), company culture (39%), and finished material protection (36%). The fifth highest percentage belonged to defective materials which was added by less than 20% of participants. Accordingly, only the 4 factors with the highest percentages were added to the original list, resulting in a total of 13 factors.
Delphi Questionnaire: Round II
The 25 participants were asked to rank the 13 potential site logistics factors based on their importance. We utilized the Friedman Test to determine any difference in the importance of the 13 potential site logistics factors based on participants’ responses. The calculated results of this test show that χ2 (12) = 18.146, p = 0.111 (Table 5). Since the p-value > 0.05, we supported the null hypothesis (i.e.) that in the experts’ opinions, there were no differences in the importance of the 13 site logistics planning factors. Table 6 provides information regarding the mean rank of each factor.
Furthermore, the entire analysis process was repeated for different subpopulations of the participants to explore potential subpopulation differences in the importance of the 13 site logistics planning factors. In one division, the participants were divided into the following two groups based on their years of experience: 1) seniors with years of experience ≥15 and 2) juniors with years of experience <15. The results of the Friedman test indicated no differences in the importance of the 13 site logistics planning factors from the seniors’ and juniors’ perspectives [χ2 (12) = 9.195, p = 0.686 for seniors and χ2 (12) = 15.712, p = 0.205 for juniors] (Table 5). Table 7 and Table 8 present the mean ranks of each factor given the seniors’ and juniors’ responses, respectively.
In another division, the participants were subdivided into two groups according to educational background: 1) holding a bachelor’s or a master’s degree; and 2) a high school diploma as the highest degree.
The results of the Friedman test indicated no difference in the importance of the 13 site logistics planning factors from the first and second groups’ perspectives [χ2 (12) = 11.458, p = 0.490 for the participants with either a bachelor’s or a master’s degree and χ2 (12) = 8.101, p = 0.777 for the participants with a high school diploma as the highest degree] (Table 5). Table 9 and Table 10 present the mean ranks of each factor given the first and second group’s responses, respectively.
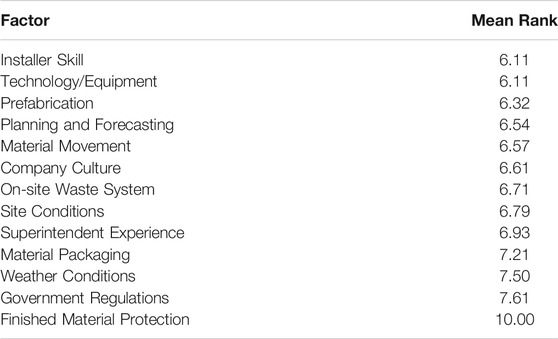
TABLE 9. Mean ranks of the factors: responses of the participants with a bachelor’s or a master’s degree.
Delphi Questionnaire: Round III
For Round III, the 7 factors with the lowest mean ranks (considering all participants’ responses) were selected (Table 6). The participants were asked for agreement on these 7 factors are important site logistics factors. In this round, 21 participants completed the questionnaire, with 81% (17 of 21 participants) agreeing that all 7 factors are important site logistics factors. Two participants believed that “site conditions” must be removed from the list, and two other participants mentioned that “company culture” must be removed from the list; thus, all participants agreed with these 5 items -- installer skill, technology/equipment, prefabrication, planning and forecasting, and material movement -- being important site logistics factors. Moreover, two participants believed that “government regulations” should be added to the list and another participant mentioned that “material packaging” should be added to the list.
Discussion
The results of the literature review helped us initially identify 13 site logistics-related factors that influence the amount of construction material used in a project. These include 1) installer skill, 2) technology/equipment, 3) prefabrication, 4) planning and forecasting, 5) company culture, 6) site condition, 7) material movement, 8) material packaging, 9) superintendent experience, 10) on-site waste system, 11) government regulations, 12) weather conditions, and 13) finished material protection (Table 7). The impacts of these factors can be mainly observed across the three categories of resource use affected by site logistics: 1) material damage, 2) rework, and 3) construction waste. Not all factors influence all three categories. For instance, the installer’s skill may affect all three: material damage, rework, and material waste. Certain installers may carry out a construction task incorrectly due to either the lack of knowledge or skill, which may result in material damage as well as wastage. Moreover, the construction task needs to be re-done accurately, which may involve reordering the required materials. In such cases, the material needed for rework may not be available in a timely fashion, thereby impacting the overall project progress. Similarly, factors such as technology/equipment, prefabrication, planning and forecasting, superintendent experience, and site/weather conditions, have an impact across all three categories of site planning. Other factors such as material movement and material packaging may only impact material damage and construction waste (material damage due to improper storage or handling) categories, whereas other factors such as company culture and on-site waste systems may address construction waste more than the other categories.
Reducing material waste on construction sites is considered a major challenge. As elaborated earlier, waste mainly occurs due to poor logistics and inefficiencies in material procurement. Based on our survey results, we found that installer skill is an important site logistics-related factor affecting the amount of construction material used in a project. As we already know, the competency of the installer affects both productivity and overall quality of the project. Installer inability often extends the end time of a specific task, thereby delaying the start time of the next task. The study conducted by Tunji-Olayeni et al. (2017) further corroborates the findings of our study, listing site logistics-related factors such as technology/equipment and prefabrication as crucial factors.
Several studies have indicated that the EE of a construction project can be reduced by design improvements. However, our study shows that a portion of EE is also impacted by site logistical planning. Handling construction activities efficiently helps lower the amount of material and construction resources used in a project. For instance, an effective CSLP can be extremely beneficial in reducing EE spent on processes such as material manufacturing, labor, transportation of materials, administration, etc. Furthermore, rework and material damage have detrimental implications for the IEE of a project since these require additional material to complete a specific construction task. Therefore, developing an efficient site logistics plan is critical for reducing not just the overall EE of a construction project, but embodied carbon as well. This could help lower the overall energy and environmental footprint of the construction sector.
This paper identified and ranked factors related to site logistics that may impact onsite material damage, rework, and construction waste. The results of this study add new knowledge in three key areas. First, the results explain how site logistics and its planning may impact material consumption and indirectly affect the initial embodied energy (IEE) of a construction project, improving the current understanding of embodied energy analysis. Second, the findings enhance the knowledge base of the field of lean construction by linking a site logistics plan to onsite resource use and waste. This makes the case of efficient and effective project planning more compelling for environmental and business benefits. Third, the identified factors can be integrated into a computation algorithm such as the ant colony algorithm to optimize a site logistics plan for the efficiency of construction operations.
The results of this study may be limited to the state of Texas from which the participants were selected. These may not be generalized to the national and global levels. The relationship of material use and waste to embodied energy is not directly expressed by the participants. It is rather implied by the authors on the basis of the fact that reducing material damage, rework, and waste will lead to saving embodied impacts of construction.
Conclusion
Following a rigorous literature review, this study applied the Delphi method to identify and rank site logistics-related factors that impact the amount of resources used on a construction site. The results indicate that the installer’s skill, technology/equipment, prefabrication, planning and forecasting, and material movement are five important site logistics-related factors that help minimize construction waste. This knowledge is essential to enable construction professionals to develop a more comprehensive site logistics plan that would play a crucial role in a project’s success in terms of business benefits as well as environmental quality. Addressing these factors during the preconstruction phase could potentially help reduce construction waste and the IEE associated with it, not to mention a proportional reduction in carbon emissions. These factors can also be used to develop computational algorithms such as ant colony optimization or multi-objective genetic algorithms to optimize, for instance, the movement and staging of materials, assemblies, equipment, and labor that may generate profound benefits in terms of profit, safety, and a lower energy and carbon footprint. Although this study was limited to the state of Texas, future research could: 1) include a larger and broader population by increasing the number of participants; 2) cover most states of the United States to make results generalizable to the national level; and 3) expand the pool of participants to include research scholars working in the fields of project management, Life Cycle Assessment, lean construction, and industrial ecology.
Data Availability Statement
The datasets presented in this study can be found in online repositories. The names of the repository/repositories and accession number(s) can be found below: http://oaktrust.library.tamu.edu/handle/1969.1/192200.
Author Contributions
JB: conducted the research and wrote the report; VV: helped create the manuscript; FP: conducted data analysis; MD: coordinated the research and writing of the article.
Conflict of Interest
The authors declare that the research was conducted in the absence of any commercial or financial relationships that could be construed as a potential conflict of interest.
Publisher’s Note
All claims expressed in this article are solely those of the authors and do not necessarily represent those of their affiliated organizations, or those of the publisher, the editors, and the reviewers. Any product that may be evaluated in this article, or claim that may be made by its manufacturer, is not guaranteed or endorsed by the publisher.
References
Adebowale, O. J., and Ayodeji, F. J. (2015). Analysis of Construction-Related Factors Affecting the Efficiency Construction Labour. J. Construction Project Manag. Innovation 5 (1), 1115–1130.
Adewuyi, T. O., and Odesola, I. A. (2015). Factors Affecting Material Waste on Construction Sites in Nigeria. J. Eng. Tech. (Jet) 6 (1), 82–99.
Ajayi, S. O., Oyedele, L. O., Akinade, O. O., Bilal, M., Alaka, H. A., Owolabi, H. A., et al. (2017). Attributes of Design for Construction Waste Minimization: A Case Study of Waste-To-Energy Project. Renew. Sust. Energ. Rev. 73, 1333–1341. doi:10.1016/j.rser.2017.01.084
Ajayi, S. O., Oyedele, L. O., Ceranic, B., Gallanagh, M., and Kadiri, K. O. (2015). Life Cycle Environmental Performance of Material Specification: a BIM-Enhanced Comparative Assessment. Int. J. Sust. Building Tech. Urban Dev. 6 (1), 14–24. doi:10.1080/2093761x.2015.1006708
Al-Hajj, A., and Hamani, K. (2011). Material Waste in the UAE Construction Industry: Main Causes and Minimization Practices. Architectural Eng. Des. Manag. 7 (4), 221–235. doi:10.1080/17452007.2011.594576
Alwi, S., Mohamed, S., and Hampson, K. (2002). “Waste in the Indonesian Construction Projects,” in Proceedings of the 1st CIB-W107 International Conference-Creating a Sustainable Construction Industry in Developing Countries (CSIR), 11–13 November, 2002 (Stellenbosch, South Africa: International Council for Research and Innovation in Building and Construction (CIB)), 305–315.
Ameyaw, E. E., Hu, Y., Shan, M., Chan, A. P. C., and Le, Y. (2016). Application of Delphi Method in Construction Engineering and Management Research: a Quantitative Perspective. J. Civil Eng. Manag. 22 (8), 991–1000. doi:10.3846/13923730.2014.945953
Baldwin, A., Poon, C.-S., Shen, L.-Y., Austin, S., and Wong, I. (2009). Designing Out Waste in High-Rise Residential Buildings: Analysis of Precasting Methods and Traditional Construction. Renew. Energ. 34 (9), 2067–2073. doi:10.1016/j.renene.2009.02.008
Cabeza, L. F., Rincón, L., Vilariño, V., Pérez, G., and Castell, A. (2014). Life Cycle Assessment (LCA) and Life Cycle Energy Analysis (LCEA) of Buildings and the Building Sector: A Review. Renew. Sustain. Energ. Rev. 29, 394–416. doi:10.1016/j.rser.2013.08.037
Chan, A. P. C., Yung, E. H. K., Lam, P. T. I., Tam, C. M., and Cheung, S. O. (2001). Application of Delphi Method in Selection of Procurement Systems for Construction Projects. Construction Manag. Econ. 19 (7), 699–718. doi:10.1080/01446190110066128
Correia, J. M. F., de Oliveira Neto, G. C., Leite, R. R., and da Silva, D. (2021). Plan to Overcome Barriers to Reverse Logistics in Construction and Demolition Waste: Survey of the Construction Industry. J. Constr. Eng. Manage. 147 (2), 04020172. doi:10.1061/(asce)co.1943-7862.0001966
Crowther, P. (1999). Design for Disassembly to Recover Embodied Energy. Sustaining the Future: Energy Ecology Architecture PLEA99, September 22–24, 1999 (Brisbane, Australia: PLEA International), 95–100.
Defra, A. (2013). Overview of evidence: A rationale for waste prevention in England. London: Department for Environment, Food and Rural Affairs.
Ding, G. K. C. (2004). The Development of a Multi-Criteria Approach for the Measurement of Sustainable Performance for Built Projects and Facilities. Building Res. Inf. 33 (1), 1.
Ding, Z., Yi, G., Tam, V. W. Y., and Huang, T. (2016). A System Dynamics-Based Environmental Performance Simulation of Construction Waste Reduction Management in China. Waste Manag. 51, 130–141. doi:10.1016/j.wasman.2016.03.001
Dixit, M. K., Fernández-Solís, J. L., Lavy, S., and Culp, C. H. (2010). Identification of Parameters for Embodied Energy Measurement: A Literature Review. Energy and buildings 42 (8), 1238–1247. doi:10.1016/j.enbuild.2010.02.016
Dixit, M. K. (2017). Life Cycle Embodied Energy Analysis of Residential Buildings: A Review of Literature to Investigate Embodied Energy Parameters. Renew. Sust. Energ. Rev. 79, 390–413. doi:10.1016/j.rser.2017.05.051
Dixit, M. K. (2019). Life Cycle Recurrent Embodied Energy Calculation of Buildings: A Review. J. Clean. Prod. 209, 731–754. doi:10.1016/j.jclepro.2018.10.230
Dixit, M. K., and Singh, S. (2018). Embodied Energy Analysis of Higher Education Buildings Using an Input-Output-Based Hybrid Method. Energy and Buildings 161, 41–54. doi:10.1016/j.enbuild.2017.12.022
Ekanayake, L. L., and Ofori, G. (2004). Building Waste Assessment Score: Design-Based Tool. Building Environ. 39 (7), 851–861. doi:10.1016/j.buildenv.2004.01.007
EPA, . (2017). Advancing Sustainable Materials Management. 2017 fact sheet. Washington, DC: United States Environmental Protection Agency.
Fapohunda, J. A., and Chileshe, N. (2014). Essential Factors towards Optimal Utilisation of Construction Resources. J. Eng. Des. Tech. 2, 1. doi:10.1108/jedt-02-2013-0016
Fini, A. A. F., and Forsythe, P. (2020). Barriers to Reusing and Recycling Office Fit-Out: An Exploratory Analysis of Demolition Processes and Product Features. Construction Econ. Building 20 (4), 42–62. doi:10.5130/ajceb.v20i4.7061
Formoso, C. T., Isatto, E. L., and Hirota, E. H. (1999). “Method for Waste Control in the Building Industry,” in Proceedings IGLC (Vol. 7) (IEEE), 325.
Francis, A., and Thomas, A. (2020). Exploring the Relationship between Lean Construction and Environmental Sustainability: A Review of Existing Literature to Decipher Broader Dimensions. J. Clean. Prod. 252, 119913. doi:10.1016/j.jclepro.2019.119913
Gavilan, R. M., and Bernold, L. E. (1994). Source Evaluation of Solid Waste in Building Construction. J. construction Eng. Manag. 120 (3), 536–552. doi:10.1061/(asce)0733-9364(1994)120:3(536)
Ghanem, M., Hamzeh, F., Seppänen, O., and Zankoul, E. (2018). “A New Perspective of Construction Logistics and Production Control: an Exploratory Study,” in Proceedings of the 26th Annual Conference of the International Group for Lean Construction (IGLC) (Chennai, India: IEEE), 16–22. doi:10.24928/2018/0540
Hallowell, M. R., and Gambatese, J. A. (2010). Qualitative Research: Application of the Delphi Method to CEM Research. J. Constr. Eng. Manage. 136 (1), 99–107. doi:10.1061/(asce)co.1943-7862.0000137
Ikau, R., Joseph, C., and Tawie, R. (2016). Factors Influencing Waste Generation in the Construction Industry in Malaysia. Proced. - Soc. Behav. Sci. 234, 11–18. doi:10.1016/j.sbspro.2016.10.213
Hurwitz, D. S., Sanford Bernhardt, K. L., Turochy, R. E., and Young, R. K. (2016). Transportation Engineering Curriculum: Analytic Review of the Literature. J. Prof. Iss. Eng. Ed. Pr. 142 (3), 04016003.
Jaillon, L., Poon, C. S., and Chiang, Y. H. (2009). Quantifying the Waste Reduction Potential of Using Prefabrication in Building Construction in Hong Kong. Waste Manag. 29 (1), 309–320. doi:10.1016/j.wasman.2008.02.015
Khanh, H. D., and Kim, S. Y. (2014). Identifying Causes for Waste Factors in High-Rise Building Projects: A Survey in Vietnam. KSCE J. Civ Eng. 18 (4), 865–874. doi:10.1007/s12205-014-1327-z
Kirun, S. S., and Varghese, S. (2015). A Study on Cost Control Using Delphi Techniques in Construction Projects. Int. J. Sci. Tech. Eng. 2 (5), 44–51.
Koskela, L. (2004). “Making-Do—The Eighth Category of Waste,” in 12th Annual Conference of the International Group for Lean Construction (Helsingor, Denmark: The University of Salford), 3–5.
Lota, P. S., and Trivedi, J. (2020). “Site Layout Planning through BIM Visualisation—A Case Study,” in 27th Annual Conference of the International Group for Lean Construction, IGLC (IEEE).
Love, P. E. D., Holt, G. D., Shen, L. Y., Li, H., and Irani, Z. (2002). Using Systems Dynamics to Better Understand Change and Rework in Construction Project Management Systems. Int. J. project Manag. 20 (6), 425–436. doi:10.1016/s0263-7863(01)00039-4
Love, P. E. D., and Li, H. (2000). Quantifying the Causes and Costs of Rework in Construction. Construction Manag. Econ. 18 (4), 479–490. doi:10.1080/01446190050024897
Love, P. E. D., Mandal, P., and Li, H. (1999). Determining the Causal Structure of Rework Influences in Construction. Construction Manag. Econ. 17 (4), 505–517. doi:10.1080/014461999371420
Luangcharoenrat, C., Intrachooto, S., Peansupap, V., and Sutthinarakorn, W. (2019). Factors Influencing Construction Waste Generation in Building Construction: Thailand's Perspective. Sustainability 11 (13), 3638. doi:10.3390/su11133638
Magill, L. J., Jafarifar, N., Watson, A., and Omotayo, T. (2020). 4D BIM Integrated Construction Supply Chain Logistics to Optimise On-Site Production. Int. J. construction Manag. 1, 1–10. doi:10.1080/15623599.2020.1786623
Mahamid, I. (2020). Impact of Rework on Material Waste in Building Construction Projects. Int. J. Construction Manag. 1, 1–8. doi:10.1080/15623599.2020.1728607
Mall, A. (2019). Reducing Material Waste with the Application of Building Information Modelling. Masters Thesis. Durban, South Africa: Durban University of Technology.
Misron, N. F., Khoiry, M. A., and Hamzah, N. (2018). A Framework of Efficient Material Storage Management on Congested Construction Site. E3s Web Conf. 65, 03005. doi:10.1051/e3sconf/20186503005
Mokhtar, S. N., Mahmood, N. Z., Hassan, C., Masudi, A. F., and Sulaiman, N. M. (2011). “Factors that Contribute to the Generation of Construction Waste at Sites,” in Advanced Materials Research (Bach, Switzerland: Trans Tech Publications Ltd), 4501–4507.
Nagapan, S., Abdul Rahman, I., and Asmi, A. (2011). “A Review of Construction Waste Cause Factors,” in Asian Conference of Real Estate: Sustainable Growth Managing Challenges (ACRE) (Malaysia: At: Thistle Hotel, Johor Bahru).
Nagapan, S., Rahman, I. A., and Asmi, A. (2012). Factors Contributing to Physical and Non-physical Waste Generation in Construction Industry. Int. J. Adv. Appl. Sci. 1 (1), 1–10. doi:10.11591/ijaas.v1i1.476
Nahmens, I., and Ikuma, L. H. (2012). Effects of Lean Construction on Sustainability of Modular Homebuilding. J. Archit. Eng. 18 (2), 155–163. doi:10.1061/(asce)ae.1943-5568.0000054
National Research Council (2009). Advancing the Competitiveness and Efficiency of the US Construction Industry. Washington, DC: National Academies Press.
Ning, X., Lam, K.-C., and Lam, M. C.-K. (2011). A Decision-Making System for Construction Site Layout Planning. Automation in Construction 20 (4), 459–473. doi:10.1016/j.autcon.2010.11.014
Ning, X., Lam, K.-C., and Lam, M. C.-K. (2010). Dynamic Construction Site Layout Planning Using max-min Ant System. Automation in construction 19 (1), 55–65. doi:10.1016/j.autcon.2009.09.002
Ogundipe, K., Owolabi, J., and Ogbebor, C. (2020). “Factors Militating against Effective Building Materials Management (EBMM) in Construction Projects,” in Pro. Of Inter. Str. Eng. & Const. Hol. Over of Stru. Des. & Const (Springer). doi:10.14455/isec.res.2020.7(1).con-19
Ohno, T., and Bodek, N. (1988). Toyota Production System: Beyond Large-Scale Production 1st ed. New York: Productivity Press (Taylor and Francis Group).
Owolabi, O. S. B., Alfred, O. D., and Olufemi, O. D. K. (2021). Appraisal of the Factors that Affect the Material Management Strategies of Construction Works in Ekiti State Nigeria. Int. J. humanit. soc. sci. 26 (9), 21–29.
Park, J., and Tucker, R. (2017). Overcoming Barriers to the Reuse of Construction Waste Material in Australia: a Review of the Literature. Int. J. Construction Manag. 17 (3), 228–237. doi:10.1080/15623599.2016.1192248
Pheng, L. S., and Hui, M. S. (1999). The Application of JIT Philosophy to Construction: a Case Study in Site Layout. Construction Manag. Econ. 17 (5), 657–668. doi:10.1080/014461999371268
Popoola, O. C., Adeleke, J. S., and Ayegbokiki, S. T. (2018). An Investigation into Material Wastage on Building Construction Sites. Technology (IJOSEET) 3 (3), 18–26.
Pradeep Kumar, P., Venkatraj, V., and Dixit, M. K. (2022). Evaluating the Temporal Representativeness of Embodied Energy Data: A Case Study of Higher Education Buildings. Energy and Buildings 254, 111596. doi:10.1016/j.enbuild.2021.111596
Regassa, T. (2015). Study on Importance of Logistics Management in Construction Industry, Case Study. Masters Thesis. Nairobi, Kenya: Jesuit Historical Institute in Africa (JHIA).
Sourani, A., and Sohail, M. (2015). The Delphi Method: Review and Use in Construction Management Research. Int. J. Construction Edu. Res. 11 (1), 54–76. doi:10.1080/15578771.2014.917132
Tam, V. W. Y., Tam, C. M., Chan, J. K. W., and Ng, W. C. Y. (2006). Cutting Construction Wastes by Prefabrication. Int. J. Construction Manag. 6 (1), 15–25. doi:10.1080/15623599.2006.10773079
Tan, X. N. (2011). A Research on Construction Waste Reduction Behaviors. Xi ‘an: Xi ‘an Univ. architecture Technol. 5, 1.
Tetik, M., Peltokorpi, A., Seppänen, O., Leväniemi, M., and Holmström, J. (2021). Kitting Logistics Solution for Improving On-Site Work Performance in Construction Projects. J. Constr. Eng. Manage. 147 (1), 05020020. doi:10.1061/(asce)co.1943-7862.0001921
Tunji-Olayeni, P. F., Afolabi, A. O., Ojelabi, R. A., and Ayim, B. A. (2017). Impact of Logistics Factors on Material Procurement for Construction Projects. Int. J. Civil Eng. Tech. (IJCIET, 8 (12), 1142–1148.
Udawatta, N., Zuo, J., Chiveralls, K., and Zillante, G. (2015). Improving Waste Management in Construction Projects: An Australian Study. Resour. Conservation Recycling 101, 73–83. doi:10.1016/j.resconrec.2015.05.003
Venkatraj, V., and Dixit, M. K. (2021). Life Cycle Embodied Energy Analysis of Higher Education Buildings: A Comparison between Different LCI Methodologies. Renew. Sust. Energ. Rev. 144, 110957. doi:10.1016/j.rser.2021.110957
Venkatraj, V., Dixit, M. K., Yan, W., and Lavy, S. (2020). Evaluating the Impact of Operating Energy Reduction Measures on Embodied Energy. Energy and Buildings 226, 110340. doi:10.1016/j.enbuild.2020.110340
Wahab, A. B., and Lawal, A. F. (2011). An Evaluation of Waste Control Measures in Construction Industry in Nigeria. Afr. J. Environ. Sci. Tech. 5 (3), 246–254.
Wang, J. Y., Kang, X. P., and Tam, V. W. Y. (2008). An Investigation of Construction Wastes: an Empirical Study in Shenzhen. J. Eng. Des. Tech. 2, 1. doi:10.1108/17260530810918252
Whitlock, K., Abanda, F. H., Manjia, M. B., Pettang, C., and Nkeng, G. E. (2021). 4D BIM for Construction Logistics Management. CivilEng 2 (2), 325–348. doi:10.3390/civileng2020018
Keywords: site logistics, material damage, embodied energy, construction waste, delphi method, project planning
Citation: Dixit MK, Venkatraj V, Pariafsai F and Bullen J (2022) Site Logistics Factors Impacting Resource Use on Construction Sites: A Delphi Study. Front. Built Environ. 8:858135. doi: 10.3389/fbuil.2022.858135
Received: 19 January 2022; Accepted: 17 February 2022;
Published: 15 March 2022.
Edited by:
Carlos A. P. Soares, Fluminense Federal University, BrazilReviewed by:
Karoline Figueiredo, Federal University of Rio de Janeiro, BrazilBruno Barzellay Ferreira Da Costa, Federal University of Rio de Janeiro, Brazil
Copyright © 2022 Dixit, Venkatraj, Pariafsai and Bullen. This is an open-access article distributed under the terms of the Creative Commons Attribution License (CC BY). The use, distribution or reproduction in other forums is permitted, provided the original author(s) and the copyright owner(s) are credited and that the original publication in this journal is cited, in accordance with accepted academic practice. No use, distribution or reproduction is permitted which does not comply with these terms.
*Correspondence: Manish Kumar Dixit, mdixit@tamu.edu