Effects of the Herbicide Metsulfuron-Methyl on a Plant Community, Including Seed Germination Success in the F1 Generation
- 1Plant Ecology and Nature Conservation, Wageningen University, Wageningen, Netherlands
- 2Environmental Risk Assessment, Wageningen Environmental Research (Alterra), Wageningen University and Research, Wageningen, Netherlands
A field trial was set up to simulate a field margin environment to analyze sub-lethal effects of the herbicide metsulfuron-methyl on several endpoints of non-target terrestrial plants (NTTPs). Both vegetative and reproductive endpoints were evaluated. The experiment was conducted in an experimentally established field strip with sown species. The treatments consisted of five dosages and a control: 0, 0.0097, 0.0193, 0.058, 0.174, and 0.348 gram active ingredient per hectare (g a.i./ha). The plant cover, number of (flowering) individuals per species and fruit collection were performed and estimated weekly for a period of 4 months. At the end of the growing season, the total dry biomass per species was obtained and the collected fruits were weighed, counted, and sieved to obtain the seeds. The seeds were counted and weighed as well, before they were used in a germination experiment to test the seed emergence of the F1 generation. The herbicide only affected the biomass of Matricaria recutita at the treatment levels tested (0.058 g a.i./ha and higher). Field dosages of 0.174 and 0.348 g a.i./ha differed significantly in the endpoint “plant cover” compared to lower dosages and controls. The F1 generations of Sinapis alba, Centaurea cyanus, and Phacelia tanacetifolia were particularly affected at field dosages of 0.0193 g a.i./ha and higher, showing significantly lower seed germination rates. This would imply that spray drift of metsulfuron-methyl might lead to shifts in species compositions and succession in vegetation in off-crop areas adjacent to arable fields. Conducting germination experiments is necessary to investigate a herbicide's effect on the full life cycle of plants.
Introduction
In recent decades, a decline in the diversity and abundance of wild plants in agro-systems has been reported in both Europe and North America (Geiger et al., 2010; Strandberg et al., 2012; Boutin et al., 2014). This is a worrying trend, as these agro-systems harbor a significant proportion of the overall plant biodiversity (Robinson and Sutherland, 2002). One of the causes of this decline is the increased use of herbicides (Geiger et al., 2010). Applying herbicides is a common method to maximize the yield of crops by suppressing the growth of unwanted wild species competing for the same resources (e.g., space, light, nutrients). However, fractions of the herbicide may end up in adjacent terrestrial sites, where weed control is not intended (Boutin and Rogers, 2000), e.g., due to spray drift. The plants occurring at these sites, called non-target terrestrial plants (NTTPs), need to be protected in view of their important functions in ecosystems, as described in the plant protection goals published by the European Food Safety Authority (EFSA PPR Panel, 2014). To assess the risks of herbicides for NTTPs, two guidelines are available, focusing on tests in laboratory and greenhouse environments (OECD 208, 2006; OECD 227, 2006). However, there is almost no guidance or information available on how to study herbicide impact on NTTPs in the field (OECD 208, 2006; OECD 227, 2006; EFSA PPR Panel, 2014; Schmitz et al., 2015). Spray drift is considered to be the most important source of pollution for NTTPs adjacent to crop fields (EFSA PPR Panel, 2014; Arts et al., 2015).
Sulfonylureas represent one of the largest classes of herbicides, with at least 27 different active ingredients (a.i.) registered around the world (Russell et al., 2002). One of the sulfonylurea compounds is metsulfuron-methyl (C14H15N5O6S), known to control 60 species as a potent inhibitor of plant growth and is especially used for the control of dicotyledon species (Boutin et al., 2004, 2012). Its mode of action involves limiting cell division (Russell et al., 2002).
The risks of spray drift of herbicides to NTTPs is assessed using plant characteristics (or endpoints), sensitive to the mode of action. Endpoints are plant characteristics that are sensitive to the mode of action of the chemical being used (EFSA PPR Panel, 2014). Risk estimates have been based on ED50 (the dosage at which the specific endpoint value decreases by 50%) for the various endpoints selected. In the official guidelines of the Organization for Economic Co-operation and Development (OECD), biomass is used as a vegetative endpoint to assess the risks (OECD 208, 2006; OECD 227, 2006). However, reproductive endpoints such as seed and fruit yield are also important to include in these risk assessments. For example, in the field study by Kjær et al. (2006), exposure of Hawthorn (Crateaegus monogyna) to metsulfuron-methyl resulted in 100% losses of berries at a field application rate of 0.05 g a.i./ha, while the vegetative endpoints (leaves) remained unaffected. Findings of other studies (Boutin et al., 2000; Blackburn and Boutin, 2003; Olszyk et al., 2004; Carpenter and Boutin, 2010; Pfleeger et al., 2012; EFSA PPR Panel, 2014; Schmitz et al., 2014, 2015) also suggest that reproductive endpoints are more sensitive than vegetative endpoints, especially in comparison with biomass as a vegetative endpoint.
Studies of NTTPs and herbicides which only include vegetative endpoints to assess the risk are thus not sufficient to test the overall effect of a herbicide on the whole life cycle of a plant, as the reproductive endpoints may be a crucial factor for the persistence of natural plant populations. Important stages of a natural plant population are the germination of seeds, seedling and juvenile stages, flowering, seed production and the germination rates of these seeds (EFSA PPR Panel, 2014). Completion of the full life cycle of a plant is necessary for it to contribute to a sustainable population, the latter being defined as one of the protection goals by EFSA. The aim of the present study was to investigate whether the sub-lethal dosages of metsulfuron methyl that are currently applied cause effects on plant populations in the field. Several studies (Blackburn and Boutin, 2003; Rokich et al., 2009; EFSA PPR Panel, 2014) investigated the germination of seeds from plants exposed in their mature stage, while none investigated the germination of seeds from plants exposed in their juvenile stage. Studies with juvenile stages could be important as the exposure to most herbicides in real agro-systems might occur when the plants are in their juvenile stage (Arts et al., 2015), however this is not always the case (Strandberg et al., 2012; Boutin et al., 2014).
The present field trial assessed the effects of the herbicide metsulfuron-methyl on seven terrestrial plant species in a field margin freshly sown with a mixture of wild plants. We tried to answer the following research questions: (1) How does the sensitivity of reproductive endpoints differ from that of vegetative endpoints? (2) Is there an effect on the next generation of herbicide-exposed NTTPs? (3) How do species differ in their sensitivity to the herbicide sprayed at environmentally realistic exposure rates?
Materials and Methods
Location
The field trial was carried out at the Sinderhoeve research station in Renkum, The Netherlands. The soil was mainly sandy, well-drained and moderately rich in nutrients. The field margin had a length of 100 m and was 8 m wide. The field margin was larger than the actual plots used for monitoring and sampling (see Supporting Information) in order to prevent intrusion of plants from the surrounding area. The immediately surrounding environment area consisted of a regularly mown unsprayed grassland.
Seed Mixture
A seed mixture containing 11 species was used to establish the vegetation in the 8 × 100 m field margin. The field margin consisted of bare soil at the time of sowing. The mixture of seeds (200 g per species) was evenly spread over the field margin by means of a sower and subsequently harrowed. The commercially available mixture (Table 1) consisted of both annual and perennial species, to simulate a realistic situation. The mixture contained several families which are highly susceptible to the herbicide metsulfuron-methyl like Brassicaceae and Fabaceae (Boutin et al., 2000, 2004). Of the 11 sown species, only six species developed to a mature stage (Table 1). Furthermore, Lupinus perennis and Melilotus officinalis did not produce seeds within the experimental period. In contrast, Glebionis segetum, which was not part of the seed mixture, developed rapidly during the growing season from the existing seed bank. This species was therefore also included as a seventh species in the analysis. The seed mixture was sown on June 17, 2015. The experiment lasted from July 16 to the first week of October 2015.
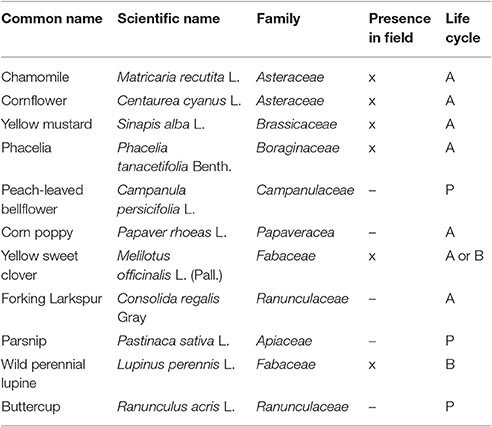
Table 1. List of plant species sown (x, present; –, not present; P, perennial; A, annual; B, biennial).
Experimental Set-Up and Measurements
The set-up used a replicated block design (four blocks with increasing dosages; see Figure 1). Vegetative and reproductive endpoints were assessed in separate parts of the plots. The northern half of each plot in the field margin was used to determine the total aboveground biomass for each species (i.e., the species sown and developed from the seed mixture and G. segetum). The plants were harvested in the first week of October 2015, in two 0.5 m2 sub-plots per plot. The fresh plant material was first sorted by species, after which the fresh biomass was determined in the lab. Subsequently, the plant material was dried in an oven at 70°C for 2 days, and the dry biomass was determined for each species.
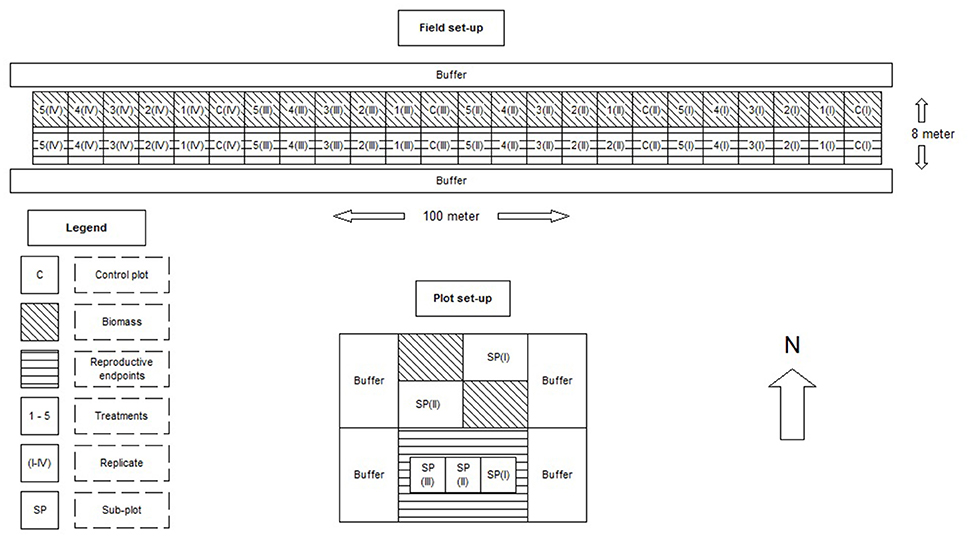
Figure 1. Experimental lay-out of the field margin (above) and the individual plots (below). The treatment levels were: 0, 0.0097, 0.0193, 0.058, 0.174, and 0.348 gram active ingredient per hectare.
The southern half of the margin was used to collect seeds and fruits as reproductive endpoints. In addition, this part of the field margin was also used to estimate the plant cover and to count the number of flowering and non-flowering individuals per species. These measurements were performed weekly from July till first week of October 2015 using an 0.25 m2 plot. Each measurement was based on three sub-plots, which corresponded to three measurements in each plot, using a quadrat. If an individual plant was producing seeds, its fruits were harvested and stored dry in marked paper bags at room temperature. After all fruits had been harvested, the seeds were counted and weighed after physically extracting them from the fruits using sieves and an air extractor. The sieves had a mesh size ranging from 0.5 to 2 mm. After this extraction, all seeds of Sinapis alba and Centaurea cyanus were counted for each subplot, and weighed on a 4-decimal mass balance. For Matricaria recutita, Phacelia tanacetifolia, and G. segetum, a subsample of 50 seeds was taken and counted and weighed for each sub-plot, because of the high abundance of seeds per sample. The total amount of seeds per sub-plot was weighed as well, to determine the total number of seeds per sub-plot by dividing the total seed biomass by the biomass of 50 seeds.
The seeds extracted from the plants were used to test whether the F1 generation was affected in its seed germination success. G. segetum was not included in the experiment, because the fruits were harvested too late in the season to be included in the germination experiment. This was caused by the later development of the seeds in comparison with the other species. Following Baskin and Baskin (2014), seeds were placed in a dry climate room at ~2–6°C for 1 month to break seed dormancy. The germination experiment was conducted in a climate room with an air temperature of 20°C, a light intensity of 350 μE/m2/s at seed level and a light/dark period of 16/8 h (Baskin and Baskin, 2014). The seeds were placed in petri-dishes on a layer of filter paper. Fifty seeds per petri-dish were used for C. cyanus, M. recutita, and P. tanacetifolia, while 30 seeds of S. alba were used per dish, because fewer seeds were available. Treatment and control experiments were carried out in triplicate according to the guidelines by Baskin and Baskin (2014). The petri-dishes were placed in a randomized block design and were rotated after each measurement. The seeds were kept moist by adding tap water if necessary. In the first week, the seed germination was recorded each day, while in the subsequent 3 weeks, measurements were performed every 2 days. The total duration of the germination experiment was 4 weeks.
Chemicals
The treatments consisted of one control and five dosages: 0, 0.0097, 0.0193, 0.058, 0.174, and 0.348 gram active ingredient per hectare (g a.i./ha). We used the herbicide solution “Ally©,” 60% of which consists of the active ingredient metsulfuron-methyl. The dosage intervals (Table S1) were based on a reference dosage, viz. the Hazardous Dosage at which 95% of the plants are potentially protected (HD5), available from greenhouse tests (Boutin et al., 2000). This specific HD5 of 0.058 g a.i./ha and based on 0.01 g a.i./ha is accepted by the US EPA database, based on vegetative endpoints. A factor of 3 was used to determine the other dosages from the reference dosage of 0.058 g a.i./ha (see Supporting Information). The dosages applied were checked by measuring concentrations of metsulfuron-methyl in the spray tank solutions. In the field, a tank sample (volume ~2 mL) was added to 2 mL acetonitrile. Intended concentrations in the spray tank solutions were expected to be at a level in which they could be measured directly. A detailed description of the preparation of the “Ally©” herbicide solution is included in the Supplementary Information (S.I.). The solutions were applied on July 16, 2015, when the juvenile plants generally had 2–4 leaves, which is the intended time for spraying plants according to the guidelines for NTTP testing (OECD 208, 2006; OECD 227, 2006). A field sprayer was used to spray directly to the field margin by means of downward spraying.
Data Analysis
The statistical analysis mainly consisted of comparing mean values of endpoints in multiple groups (>2). The data analysis followed a step-wise approach. ANOVA with a post-hoc Tukey test was performed if the data were normally distributed and if the variances were equal, to test for significant differences between treatments. If data frequencies were not equal between the treatments, the post-hoc Scheffe's ANOVA test was used. If the data was not normally distributed, a Ln-transformation or other transformation (Log10 or ArcSin) was first performed. If the data was then still not normally distributed, a Kruskall Wallis test was applied. If variances were not equal, a Welch ANOVA test was performed. Effective Dosages (EDx values) were calculated in GENstat (18th edition). No Observed Effect Dosages (NOEDs; de Snoo et al., 2005) were calculated at parameter or taxon level using the Williams test (Williams, 1972). The analyses were performed with the Community Analysis computer program (Hommen et al., 1994). Minimum Detectable Differences were calculated in percentages (%MDD). The MDDs were categorized into five classes in accordance with EFSA PPR Panel (2013) and Brock et al. (2015). If the MDD exceeds 100%, no effects can be determined statistically. If the MDD is below 50%, small effects can be determined. In between, there are two further classes (<70%; <90%). All other calculations and statistical tests were performed in SPSS (version 22), R and Microsoft Excel.
Results
Verification of the Dosing Solutions
In the spray tank solutions, concentrations of metsulfuron-methyl were found to be in the range of 87.94–97.12% of intended concentrations (Table S2). These results meet the experimental requirements, i.e., measured concentrations are between 80 and 120% of intended concentrations.
Vegetative and Reproductive Endpoints
The herbicide treatments had very little effect on the biomass of all tested plant species, except for M. recutita. Significant differences between the treatments were found for this species (p < 0.05, Kruskall Wallis test) with an ED50 of 0.06 g a.i./ha (Table S3; Table 2) and a NOED of 0.174 g a.i./ha (MDD: 72.02%). For the other species, intraspecific variability was high among the plots. The abundance of individuals, especially S. alba and L. perennis, was very low (Table S3). A particular plant species might be present in some plots but absent from other plots with the same treatment level.
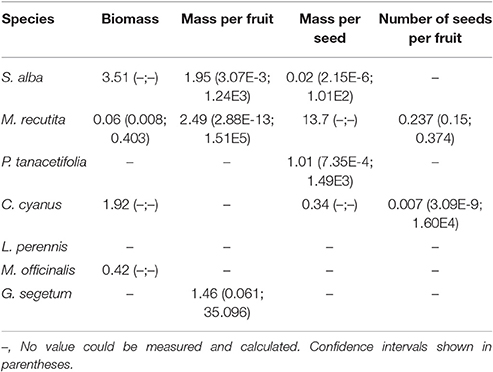
Table 2. Effective Dosages reducing 50% of a specific endpoint (ED50 in g a.i./ha), for several endpoints.
Reproductive endpoints could only be tested for S. alba, M. recutita, P. tanacetifolia, C. cyanus, and G. segetum, because these were the only species from which seeds could be collected. L. perennis and M. officinalis did not produce seeds, as they are perennial plants. Of all the reproductive endpoints we tested (number of seeds and number of fruits, total biomass of seeds and total biomass of fruits, number of seeds per fruit, mass per fruit and mass per seed), the “mass per seed” and “number of seeds per fruit” seemed to be the most sensitive. However, no significant differences were found between the treatments. Table 2 shows the values of three different reproductive endpoints. The control plots had the highest values of these endpoints in comparison with the plots treated with herbicide. S. alba in particular showed a high sensitivity of the “mass per seed” endpoint. C. cyanus and M. reticutata seemed to be mainly affected in their “number of seeds per fruit.”
Plant Cover
Figure 2 shows that, in general, the lowest plant covers were found at the highest field dosages (0.174 and 0.348 g a.i./ha), and that the values at the highest dosage differed significantly from those at the control plots after 4 weeks (p < 0.05, Tukey's ANOVA test). Furthermore, 14 significant differences (p < 0.05, Tukey's ANOVA test) were found between treatment levels of 0, 0.0097, 0.0193 and 0.058 g a.i./ha and the highest dosage (0.348 g a.i./ha). These differences were found in the first 7 weeks after spraying, while after this period only three significant differences (p < 0.05, Tukey's ANOVA tests) were found between the lower dosages and the 0.174 g a.i./ha dosage. Additionally, a NOED of 0.058 g a.i./ha was found in weeks 6 and 7 (MDD: 9.69 and 12.73%, respectively; Table S5). During the first 6 weeks of the monitoring period the highest plant cover was not observed in the controls and the lowest dosage of 0.0097 g a.i./ha, but at the 0.0193 g a.i./ha treatment level. Apparently, this low dosage increased plant abundance. Visual assessments showed that there was a tendency toward an increase in grasses (Poaceae) in plots with field dosages of 0.174 and 0.348 g a.i./ha, at the cost of the species we had sown in the field margin.
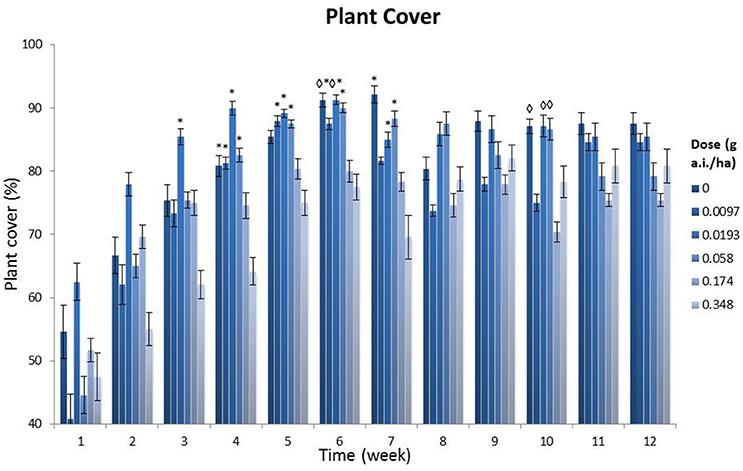
Figure 2. Plant cover per treatment level, showing a time series (weekly monitoring over a total of 12 weeks after spraying). g a.i./ha: gram active ingredient per hectare. The error bars represent the standard error. *Significant difference with the 0.348 g a.i./ha dosage (p < 0.05, one-way Tukey's ANOVA). ♢Significant difference with the 0.174 g a.i./ha dosage (p < 0.05, one-way Tukey's ANOVA).
Germination Experiment
Figure 3 shows the average germination rates per treatment for S. alba (a), M. recutita (b), P. tanacetifolia (c), and C. cyanus (d). Statistical tests (Kruskall Wallis non-parametric test) showed that 3 out of 4 species (p < 0.05 for S. alba, P. tanacetifolia, and C. cyanus) had significant differences in germination percentage at different dosages. The germination of S. alba seeds from plots with dosages of 0.058 g a.i./ha and higher was affected. The seed germination rate of S. alba was quite low (<30% in controls), while the seeds from the lowest dosage plots (0.0097 and 0.0193 g a.i./ha) showed a relatively high germination rate (50 and 24%, respectively %). The germination rates at 0.058 g a.i./ha and higher are affected (Figure 3).
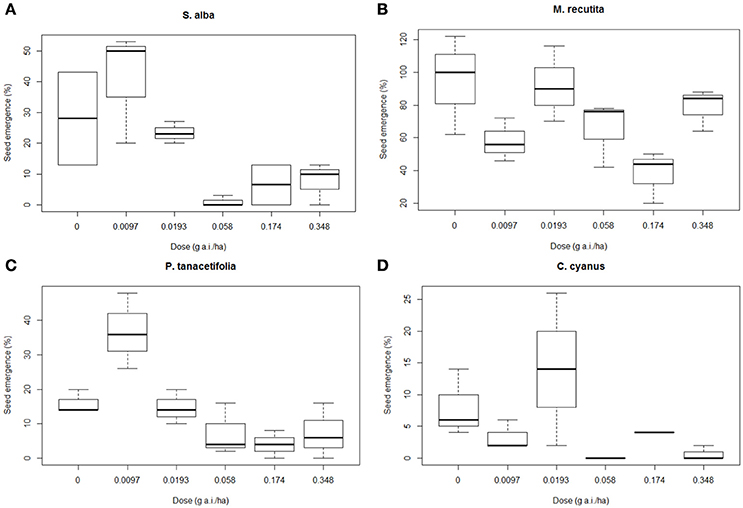
Figure 3. Boxplots presenting the means of germination succes for each species and each treatment level, as a percentage of the total number of seeds per replicate. (A) Sinapis alba, (B) Matricaria recutita, (C) Phacelia tanacetifolia, and (D) Centaurea cyanus. g a.i./ha: gram active ingredient per hectare.
M. recutita showed a high germination rate (100% in controls). The germination rate of the seeds of M. recutita was the highest of all species (71.1% in the controls). Although no direct trend was found between the dosages (Figure 3), we found an NOED of 0.058 g a.i./ha (MDD: 39%; Table S4). For P. tanacetifolia and C. cyanus, there was a reduction in the germination of seeds from plots with dosages of 0.058 and 0.0193 g a.i./ha, respectively (MDD: 78 and 69% respectively) and higher (Table S4). However, the seed germination was very low for both species (14% in controls for P. tanacetifolia and 6% in controls for C. cyanus). Hormesis seemed to occur in S. alba, P. tanacetifolia and C. cyanus, implying that relatively low dosages of metsulfuron-methyl (0.0097 and 0.0193 g a.i./ha) stimulated seed germination.
Discussion
Discussion of Results
The results show that biomass was not affected by the herbicide for any of the species except M. recutita. On the other hand, several other vegetative plant endpoints (width, height etc.) could be potentially affected, as metsulfuron-methyl limits cell division (Russell et al., 2002). This can limit the size of the plant, without the biomass being affected. However, we did not measure these endpoints. We found no significant differences between the treatments regarding the reproductive endpoints. However, the data suggests that the values of “mass per seed” and “number of seeds per fruits” in particular decreased with increasing dosages. The lack of statistical differences can be explained by the low number of observations, which also varied between the plots, reducing the power of the statistical tests. Other studies have demonstrated that reproductive endpoints are indeed very sensitive to metsulfuron-methyl (Boutin et al., 2000; Blackburn and Boutin, 2003; Olszyk et al., 2004; Kjær et al., 2006; Carpenter and Boutin, 2010; Pfleeger et al., 2012; Schmitz et al., 2014, 2015). Our results also showed hormesis occurring in S. alba, P. tanacetifolia and C. cyanus, at low dosages of metsulfuron-methyl and in both vegetative and reproductive endpoints. Hormesis is a phenomenon often observed in plant studies (Duke et al., 2006).
Our results showed that plant cover differed significantly between the highest dosage (0.348 g a.i./ha) on the one hand and the control and other treatment levels on the other hand. Fourteen significant differences were found in the first 7 weeks after the herbicide exposure. Thereafter, only in 1 week were three significant differences found between treatments. In addition, an NOED of 0.058 g a.i./ha was found in weeks 6 and 7, while an NOED of 0.348 g a.i./ha was found in week 8. This difference suggests that plants can recover from spraying, as was also found in other studies (de Snoo et al., 2005; Carpenter and Boutin, 2010; Strandberg et al., 2012; Schmitz et al., 2014). In comparison with lower-level treatments, plots with dosages of 0.174 and 0.348 g a.i./ha featured more grasses (Poaceae), as we found through visual observation. Grasses are not a direct target of the herbicide (Russell et al., 2002) and are more resistant to dosages of 0.174 and 0.348 g a.i./ha. Grasses in these high dosage plots may have contributed to the recovery of the plant cover, instead of the species we sowed.
The statistically significantly lower seed germination at the higher herbicide dosages can thus potentially lead to shifts in species composition and succession of the vegetation (Schmitz et al., 2014), with higher frequencies of tolerant species (Geiger et al., 2010). For example, S. alba could disappear from field margins next to arable fields in the long run, because of reduced seed quality (such as seed biomass and seed germination success) at dosages of 0.0193 g a.i./ha or higher at a normal field application dosage of 0.058 g a.i./ha. In addition, P. tanacetifolia and C. cyanus also showed significantly reduced germination of seeds at higher field dosages (higher than 0.0193 or 0.058 g a.i./ha). In the long term, this could lead to a situation where these susceptible species disappear and tolerant species (such as M. recutita in the present experiment, based on the germination results) gain a greater abundancy. However, the biomass of this species was highly affected by the herbicide. This indicates that the effects of metsulfuron-methyl are complex, because there is no single endpoint which is the most sensitive for all species. Hence, this study shows that the accepted HD5 of 0.058 g a.i./ha is not protective in terms of plant cover, and especially in terms of F1 seed germination success, as the NOED was 0.058 g a.i./ha or lower.
In the present study, the difference between the biomass and reproductive endpoints at various dosages, based on the NOEDs, was minor. However, plant cover and seed germination rates had much lower NOEDs (0.058 g a.i./ha and lower). This indicates that these endpoints were the most sensitive ones in this field study. The vegetative and reproductive endpoints tested in this field trial showed a high variability, which limited clear dose-response results. Apparently, it is not only the herbicide treatment which influenced the effects, but also many co-variables. Several possible factors could be mentioned. First, the test duration was restricted to one growing season. More data based on multiple years would give a better estimate of the mean effect, as the field study by Schmitz et al. (2014) revealed, where the effects of a herbicide became stronger over time. At the time when we sowed the seeds, there was a severe drought lasting several weeks, which could have influenced the germination success of the seeds. Testing across multiple years would average out these (extreme) weather conditions. Second, some individuals were exposed directly, while other individuals of the same species had not germinated yet at the moment of spraying. However, the herbicide is mobile in the soil (Blair and Martin, 1988; Russell et al., 2002), so the herbicide could have been taken up by both foliage and roots and transported via xylem and phloem later on (Blair and Martin, 1988). Third, the present study used a realistic field margin set-up with a seed mixture consisting of multiple species, and additional species developed in the field. This could have resulted in species interactions and competition, leading to different abundances per species and plot. However, we were not able to find any statistically significant interactions between species in terms of plant abundances, population dynamics or succession.
Recommendations
The present paper has focused on the germination experiment and some results regarding vegetative and reproductive endpoints. However, this study also intended to provide information and recommendations for further experiments with NTTPS and herbicides in real field settings. The most important recommendations in this respect are listed in Table 3.
Conclusion
In the introduction, the following questions were raised: (1) How does the sensitivity of reproductive endpoints differ from that of vegetative endpoints? (2) Is there an effect on the next generation of herbicide-exposed NTTPs? (3) How do species differ in their sensitivity to the herbicide? Related to question 1, this paper has shown that reproductive endpoints, especially “mass per seed” and “number of seeds per fruit,” seemed to be more sensitive than vegetative endpoints. Only the biomass of M. recutita was affected at the treatment levels we tested (0.058 g a.i./ha and higher). Field dosages of 0.174 and 0.348 g a.i./ha led to significantly different plant cover values compared to lower dosages and controls. The answer to question 2, i.e., are there any effects on the next generation of herbicide-exposed NTTPs, was shown by studying the seed germination of the F1 generation. Some species were affected at relatively low dosages of the sulfonyl urea herbicide metsulfuron-methyl. The results of the germination experiment to test whether the herbicide had an impact on the next generation showed that three out of four species (S. alba, P. tanacetifolia, and C. cyanus) had significantly lower seed germination rates at herbicide dosages of 0.0193 g a.i./ha and higher. The third question that is related to the differences in sensitivity between species, can be confirmed. The plant species involved reacted differently to the herbicide. S. alba was highly affected in terms of reproductive endpoints and seed germination, while biomass proved more sensitive in M. recutita. Reproductive endpoints and seed germination were more sensitive in P. tanacetifolia and C. cyanus. We recommend that plant reproductive endpoints and germination experiments are included in this type of risk assessment to investigate the total herbicide effect on the full life cycle and population fitness of the plants. Currently, effects on reproduction are not included in standard guidelines.
Author Contributions
JN performed the field research for his master, provided the manuscript, performed the data analyses, and literature research. RW participated in the field work and contributed to writing and critically reviewed the manuscript. IR organized the pesticide dosing of the field margin, performed part of the statistical analyses and critically reviewed the manuscript. GA was supervisor of JN and had the final responsibility for the manuscript, the editing and the submission.
Funding
The research was part of a master of Wageningen University and Research.
Conflict of Interest Statement
The authors declare that the research was conducted in the absence of any commercial or financial relationships that could be construed as a potential conflict of interest.
Acknowledgments
We would like to thank A.M. (Arrienne) Matser for her valuable contribution by preparing the spray solutions. In addition, we appreciate the work of C.H.F.M (Henri) JN, who measured several endpoints and assisted in setting up the germination experiment.
Supplementary Material
The Supplementary Material for this article can be found online at: https://www.frontiersin.org/article/10.3389/fenvs.2017.00010/full#supplementary-material
References
Arts, G. H. P., Dollinger, M., Kohlschmid, E., Maltby, L., Ocho-Acuna, H., and Poulsen, V. (2015). An ecosystem services approach to pesticide risk assessment and risk management of non-target terrestrial plants: recommendations from a SETAC Europe workshop. Environ. Sci. Pollut. Res. Int. 22, 2350–2355. doi: 10.1007/s11356-014-3637-6
Baskin, C. C., and Baskin, J. M. (2014). Seeds: Ecology, Biogeography, and Evolution of Dormancy and Germination. San Diego, CA: Academic Press.
Blackburn, L. G., and Boutin, C. (2003). Subtle effects of herbicide use in the context of genetically modified crops: a case study with Glyphosate (Roundup®). Ecotoxicology 12, 271–285. doi: 10.1023/A:1022515129526
Blair, A. M., and Martin, T. D. (1988). A review of the activity, fate and mode of action of sulfonylurea herbicides. Pest Manage. Sci. 22, 195–219. doi: 10.1002/ps.2780220303
Boutin, C., Aya, K. L., Carpenter, D., Thomas, P. J., and Rowland, O. (2012). Phytotoxicity testing for herbicide regulation: shortcomings in relation to biodiversity and ecosystem services in agrarian systems. Sci. Total Environ. 415, 79–92. doi: 10.1016/j.scitotenv.2011.04.046
Boutin, C., Elmegaard, N., and Kjaer, C. (2004). Toxicity testing of fifteen non-crop plant species with six herbicides in a greenhouse experiment: implications for risk assessment. Ecotoxicology 13, 349–369. doi: 10.1023/B:ECTX.0000033092.82507.f3
Boutin, C., Lee, H. B., Peart, E. T., Batchelor, P. S., and Maguire, R. J. (2000). Effects of the sulfonylurea herbicide metsulfuron methyl on growth and reproduction of five wetland and terrestrial plant species. Environ. Toxicol. Chem. 19, 2532–2541. doi: 10.1002/etc.5620191020
Boutin, C., and Rogers, C. A. (2000). Pattern of sensitivity of plant species to various herbicides—an analysis with two databases. Ecotoxicology 9, 255–272. doi: 10.1023/A:1026518027350
Boutin, C., Strandberg, B., Carpenter, D., Mathiassen, S. K., and Thomas, P. J. (2014). Herbicide impact on non-target plant reproduction: what are the toxicological and ecological implications? Environ. Pollut. 185, 295–306. doi: 10.1016/j.envpol.2013.10.009
Brock, T. C. M., Hammers-Wirtz, M., Hommen, U., Preuss, T. G., Ratte, H. T., Roessink, I., et al. (2015). The Minimum Detectable Difference (MDD) and the interpretation of treatment-related effects of pesticides in experimental ecosystems. Environ. Sci. Pollut. Res. 22, 1160–1174. doi: 10.1007/s11356-014-3398-2
Carpenter, D., and Boutin, C. (2010). Sublethal effects of the herbicide glufosinate ammonium on crops and wild plants: short-term effects compared to vegetative recovery and plant reproduction. Ecotoxicology 19, 1322–1336. doi: 10.1007/s10646-010-0519-7
de Snoo, G. R., Tamis, W. L. M., and van de Brink, P. J. (2005). Non Target Plant Field Study: Effects of Glufosinate-Ammonium on Off Crop Vegetation. CML report 161, Department of Environmental Biology, 114.
Duke, S. O., Cedergreen, N., Velini, E. D., and Belz, R. G. (2006). Hormesis: is it an important factor in herbicide use and allelopathy? Outlooks Pest Manage. 17, 29–33. doi: 10.1564/16feb10
EFSA PPR Panel (EFSA Panel on Plant Protection Products their Residues) (2014). Scientific Opinion addressing the state of the science on risk assessment of plant protection products for non-target terrestrial plants. EFSA J. 12:3800. doi: 10.2903/j.efsa.2014.3800
EFSA PPR Panel (EFSA Panel on Plant Protection Products their Residues) (2013). Guidance on tiered risk assessment for plant protection products for aquatic organisms in edge-of-field surface waters. EFSA J. 11:3290. doi: 10.2903/j.efsa.2013.3290
Geiger, F., Bengtsson, J., Berendse, F., Weisser, W. W., Emmerson, M., Morales, M. B., et al. (2010). Persistent negative effects of pesticides on biodiversity and biological control potential on European farmland. Basic Appl. Ecol. 11, 97–105. doi: 10.1016/j.baae.2009.12.001
Hommen, U., Dülmer, U., Veith, D. (1994). “A computer program to evaluate plankton data from freshwater field tests,” in Freshwater Field Tests for Hazard Assessment of Chemicals, eds I. A, Hill, F. Heimbach, P. Leeuwangh, and P. Matthiesen (Boca Raton, FL: Lewis Publishers), 503–513.
Kjær, C., Strandberg, M., and Erlandsen, M. (2006). Metsulfuron spray drift reduces fruit yield of hawthorn (Crataegus monogyna L.). Sci. Total Environ. 356, 228–234. doi: 10.1016/j.scitotenv.2005.03.019
OECD 208 (2006). GUIDELINES FOR THE TESTING OF CHEMICHALS. Terrestrial Plant Test: Seedling Emergence and Seedling Growth Test. Paris: OECD.
OECD 227 (2006). GUIDELINES FOR THE TESTING OF CHEMICHALS. Terrestrial Plant Test: Vegetative Vigour Test. Paris: OECD.
Olszyk, D. M., Burdick, C. A., Pfleeger, T. G., lee, E. H., and Watrud, L. S. (2004). Assessing the risks to non-target terrestrial plants from herbicides. J. Agric. Meteorol. 60, 221–242. doi: 10.2480/agrmet.60.221
Pfleeger, T., Blakeley-Smith, M., King, G., Lee, E. H., Plocher, M., and Olszyk, D. (2012). The effects of glyphosate and aminopyralid on a multi-species plant field trial. Ecotoxicology 21, 1771–1787. doi: 10.1007/s10646-012-0912-5
Robinson, R. A., and Sutherland, W. J. (2002). Post-war changes in arable farming and biodiversity in Great Britain. J. Appl. Ecol. 39, 157–176. doi: 10.1046/j.1365-2664.2002.00695.x
Rokich, D. P., Harma, J., Turner, S. R., Sadler, R. J., and Tan, B. H. (2009). Fluazifo-p-p-butyl herbicide: implications for germination, emergence and growth of Australian plant species. Biol. Conserv. 142, 850–869. doi: 10.1016/j.biocon.2008.12.013
Russell, M. H., Saladini, J. L., and Lichtner, F. (2002). Sulfonylurea herbicides. Pesticide Outlook 13, 166–173. doi: 10.1039/b206509f
Schmitz, J., P., Stahlschmidt, C. A., Brühl (2015). Protection of Terrestrial Non-Target Plant Species in the Regulation of Environmental Risks of Pesticides. Umweltbundesamt.
Schmitz, J., Schäfer, K., and Brühl, C. A. (2014). Agrochemicals in field margins—field evaluation of plant reproduction effects. Agric. Ecosyst. Environ. 189, 82–91. doi: 10.1016/j.agee.2014.03.007
Strandberg, B., Bruus, M., Kjær, C., Damgaard, C., Andersen, H. V., Bossi, R., et al. (2012). Effects of Herbicides on Non-Target Plants: How Do Effects in Standard Plant Tests Relate to Effects in Natural Habitats? Miljøstyrelsen.
Keywords: non-target terrestrial plants, metsulfuron-methyl, field trial, reproductive endpoints, seed germination, ecological risk assessment
Citation: Nelemans JB, van Wijngaarden RPA, Roessink I and Arts GHP (2017) Effects of the Herbicide Metsulfuron-Methyl on a Plant Community, Including Seed Germination Success in the F1 Generation. Front. Environ. Sci. 5:10. doi: 10.3389/fenvs.2017.00010
Received: 29 November 2016; Accepted: 03 March 2017;
Published: 28 March 2017.
Edited by:
Carsten A. Brühl, University of Koblenz and Landau, GermanyReviewed by:
Céline Boutin, Government of Canada, CanadaIlias Travlos, Agricultural University of Athens, Greece
Copyright © 2017 Nelemans, van Wijngaarden, Roessink and Arts. This is an open-access article distributed under the terms of the Creative Commons Attribution License (CC BY). The use, distribution or reproduction in other forums is permitted, provided the original author(s) or licensor are credited and that the original publication in this journal is cited, in accordance with accepted academic practice. No use, distribution or reproduction is permitted which does not comply with these terms.
*Correspondence: Gertie H. P. Arts, gertie.arts@wur.nl