Biomimetic spray coating for fruit preservation based on UiO-1 67 metal–organic framework nanozyme
- 1School of Energy and Construction Engineering, Harbin University of Commerce, Harbin, Heilongjiang, China
- 2College of Engineering, China Agricultural University, Beijing, China
- 3Management School, Harbin University of Commerce, Harbin, Heilongjiang, China
The application of edible coatings for preparing composite antibacterial spray coatings for fruit preservation by incorporating antibacterial nanoparticles has gained increasing attention. Chitosan (CS) is a natural polysaccharide used as an edible coating to preserve fruit; it has properties such as reducing water loss, enhancing appearance, and improving mechanical properties. By combining it with antibacterial material, it can reduce fruit microorganisms. Cerium (Ce) has excellent antibacterial activity combined with the advantages of safety and low cost. Therefore, this study proposes a biocatalytic spray coating for fruit preservation using a CS composite metal–organic framework (CS@Ce-MOF) with strawberry as a model fruit. CS@Ce-MOFs are superior to Ce-MOFs in the aqueous stability of their chemical structure, inoxidizability, antibacterial duration, and validity. The well-characterized CS@Ce-MOF was verified to simultaneously mimic good oxidase- and apyrase-like activities. CS@Ce-MOF biocatalytic spray coating demonstrated excellent antibacterial properties against two common foodborne pathogens: Escherichia coli and the Gram-positive bacterium Staphylococcus aureus, with high killing rates of up to 94.5%. This is due to the unique structure of the CS@Ce-MOF composite, which presents a large surface area for contact with pathogens and enhances the catalytic activity of the incorporated cerium oxide nanoparticles, leading to efficient sterilization. Furthermore, the scavenging rate of DPPH and ABTS free radicals is more than 80%, indicating that CS@Ce-MOF has excellent antioxidant properties. Moreover, CS@Ce-MOF minimized the weight loss and firmness of strawberries and bananas over 7 days of ambient storage. The use of such a biocatalytic spray coating has enormous potential for preserving the quality and safety of fresh produce, reducing food waste, and promoting sustainable agricultural practices.
1 Introduction
Fruits are an irreplaceable part of the human diet with a rich variety of nutrients; as organisms, they constantly breathe, consuming oxygen and produce carbon dioxide (Pontesegger et al., 2023; Valenzuela, 2023). In this process, many organic components such as organic acids, proteins, and fats are metabolized (Jung et al., 2019). Therefore, over time, the nutritional content, flavor, color, and other characteristics of fruit will diminish (Ebrahimi et al., 2022; Pathare et al., 2023). Because it takes some time for fruit to go through processing, transportation, and retail, effective methods are needed to extend its duration of freshness (Joung et al., 2021). Present methods of preservation are mainly by refrigeration, ozone, chemicals, and coatings. Coating is considered a simple, convenient, green, and safe method, and has attracted attention and popularity in the food industry due to its simplicity, convenience, and safety (Souza et al., 2010; Mantilla et al., 2013; Nor and Ding, 2020; Stuparu-Cretu et al., 2023). A thin layer of edible coating acts as a barrier to protect fruit and vegetables from external causes of spoilage. By reducing water loss through transpiration, the coating helps maintain the firmness and texture of produce. It also helps retain natural color and flavor by protecting against oxidation and reducing exposure to light. Additionally, some types of edible coatings have antimicrobial properties that can help inhibit the growth of microorganisms on the surface of the produce (Jung et al., 2019). The coating is designed to be breathable, thus allowing for gas exchange to occur, which can help prevent the buildup of harmful gases like ethylene. Coatings can consist of natural polymers such as chitosan, starch, cellulose, and essential oils, with or without the addition of bioactive compounds or antimicrobial agents (Bai et al., 2023).
Nanozymes are a subset of nanomaterials with enzyme mimicking properties (Li et al., 2018; Fang et al., 2023; Meng et al., 2023). They can catalyze corresponding substrates to produce reactive oxygen species in the physiological environment. Their advantages are simple preparation, low cost, high catalytic efficiency, easy large-scale production, and high stability, which have broad application prospects in the field of food preservation (Rao et al., 2021; Sheng et al., 2023). Unlike analogous natural enzymes, nanozymes exhibit many unique merits such as ease of synthesis, low cost, a wide range of sources, high tolerance against adverse environments, and multiple activities (Huang et al., 2019). A variety of nanomaterials, such as metal nanoparticles (MNPs), metal oxide or sulfide nanoparticles (MxOy/MxSy NPs), and metal–carbon complex nanomaterials, have been found to have enzyme-like properties (Kalinkevich et al., 2018; Stuparu-Cretu et al., 2023). Metal–organic frameworks (MOFs) are a kind of material with a periodic network structure formed by the self-assembly of a metal center and organic ligand, with abundant adsorption sites and load channels (Garkani Nejad et al., 2023). Previously, MOFs had been mainly used for gas storage, catalysis, and separation. However, with further study, MOFs have been found to have antibacterial properties and can be used as a repository of antibacterial agents (Alavijeh et al., 2018). For example, MOFs are used as repositories of metal ions such as silver, zinc, copper, or nickel. The decomposition of MOF skeletons is used to gradually release metal ions to provide lasting antibacterial activity and achieve antibacterial durability, which are useful for improving the quality and safety of food storage (Park et al., 2016; Zhang et al., 2016; Slavin et al., 2017; Alavijeh et al., 2018; Aryanejad et al., 2019).
Researchers have prepared a series of new nanoenzymes based on MOFs, which show excellent enzyme-like activities and good prospects for application (Natalini et al., 2021; Ni et al., 2021; Zhang et al., 2023). Wang et al. synthesized a Cu-MOF with peroxidase-like activity using Cu2+ and 4,4′-bipyridine using a coordinated induction strategy (Chen et al., 2020). Dalapati et al. synthesized a ceric MOF by a solvothermal method using cerium(IV) ammonium nitrate and 3, 4-dimethyl and 2,3-b thiophen-2, 5-dicarboxylic acid. Ce(III) and Ce(IV) ions were present in the MOFs. It can oxidize 3,3′, 5,5′-tetramethylbenzidine (TMB), 2,2′-diazodi3-ethylbenzothiazolin-6-sulfonic acid (ABTS), o-phenylenediamine (OPD), and other substances, showing good oxidase-like activity (Li et al., 2021). MOFs@NC composite material not only integrates the inherent advantages of a single component but also creates unique properties through the synergy between MOFs and NC (Zhou et al., 2023).
However, most research into MOFs focuses on their antimicrobial properties and less on their antibacterial properties. The powdery form and water instability of MOFs also limit their further actual application (Chai et al., 2022). An effective method to fix MOFs on a solid substrate was proposed by Zhai et al., who hydrothermally coated Ag-MOFs in nitrogen-doped porous carbons (N-PC). Due to the strong interaction between the N atom of N-PC and Ag+ ions, N-PC@Ag-MOFs composite material has high stability, but the preparation conditions of N-PC are harsh, and N-PC may cause food safety issues (Zhang et al., 2022). Therefore, MOF nanozymes as bioinspired spray coatings for fruit preservation still face many challenges.
The crossover between cellulose chemistry and MOF chemistry has provoked a multitude of research interests in more versatile, reliable, biocompatible, and sustainable packaging materials (Vodyanoy, 2021). Huang Guohuan et al. constructed a carrier of composite biologics through the chemical combination of porous nano-MOFs and CMFP (Wang et al., 2016). The results showed that the MOFs@CMFP film containing curcumin has good antioxidant and antibacterial activities and can prolong the shelf life of dragon fruit. This composite material has the potential for use as a super long-lasting food preservative. Moreover, the release of curcumin is controllable, which has a good application prospect in the development of long-lasting and stable food preservation film. MOFs containing iron ions were used to load Cap, and composite packaging film Cap-FeIII-HMOF-5 containing gelatin and CS has been prepared and coated onto fresh apple slices to observe the preservation effect (Zhao et al., 2020). The results showed that there was had been no bacterial decay and oxidation on the apple slices on the fifth day of storage. This demonstrated that composite packaging film can effectively prolong the shelf life of freshly cut apples.
CS is a product of chitosan deacetylated, which is the only basic cationic polysaccharide found so far (Vargas and Gonzalez-Martinez, 2010; Deng et al., 2020). It has broad-spectrum antibacterial activity and good film forming and antioxidant properties. Therefore, CS can be utilized in the food sector as an efficient, non-toxic, biodegradable film preservative and antibacterial agent for fruit and vegetables (Deng et al., 2020; He et al., 2022). For example, CS nanoparticles were introduced into potato starch (PS, film-forming matrix) to prepare a nanocomposite film without incorporating additional antibacterial agents as a potential candidate for food packaging materials (Deng et al., 2022).
This study developed nanozyme-based biomimetic active packaging with natural polymer CS complexing enzyme-like Ce@UiO-67-BPY (abbreviated as CS@Ce-MOF) as a spray-coating colloidal agent. First, UiO-67-BPY crystals were prepared by the solvothermal self-assembly of zirconium salt (ZrCl4) and bipyridine dicarboxylic acid ligand (H2BPYDC). The open bipyridine site in the ligand was coordinated with Ce by post-modification to obtain cerium-doped Ce@UiO-67-BPY material. This was made into a CS@Ce-MOF nanozyme spray coating by complexing it with CS. Spray is a convenient choice in fruit preservation, so a CS@Ce-MOF suspension was sprayed onto strawberries and bananas to evaluate its fruit-preserving effect.
2 Materials and methods
2.1 Materials
Zirconium tetrachloride (ZrCl4), 5,5′-dicarboxylic acid, 2,2' -bipyridine (H2BDC), glacial acetic acid, and N,N-dimethylformamide (DMF) were purchased from Sinopharm Chemical Reagent Co., LTD. (Shanghai, China). Chitosan, absolute ethanol, and acetone were purchased from Maclin Biochemical Technology Co., Ltd (Shanghai, China). All chemicals were of analytical grade and could be used directly without further purification.
2.2 Nanozyme fabrication
Ce-UiO-67 was synthesized by a solvothermal method. First, H2BDC (152 mg) was dissolved in 5 mL of DMF solution in a beaker, then ZrCl4(46.6 mg) and 4,4′-bifenthalic acid (48 mg) were added to the solution in a Teflon-lined reactor, followed by an aqueous solution of cerium(IV) ammonium nitrate (3 mL, 0.5 M) drop by drop. Second, 15 mL DMF and 0.4 mL acetic acid were added to the Teflon-lined reactor and reacted in an oven for 24 h. Finally, it was cleaned twice with DMF and acetone, and white Ce@UiO-67-BPY powder was thus obtained.
The Ce@UiO-67-BPY powder was then dispersed into 80 mL DMF solution of CS (350 mg). The in situ templated growth of MOF was accomplished by heating the mixture under stirring for 40 min at 80 °C. After natural cooling, the final CS@Ce-MOF samples were collected and washed thrice with acetone and water.
2.3 Characterizations
The morphology of CS@Ce-MOF samples was characterized by a scanning electron microscope (SEM, Zeiss) model (S-3400, working voltage 20 KV) manufactured by Hitachi, Japan. Energy-dispersive X-ray diffraction (EDX) analysis was performed by an X-ray spectrometer attached to a scanning electron microscope. The X-ray diffractometer (XRD, Ultima IV, Rigaku Corporation, Akishima, Japan) was selected to collect the diffraction patterns of Ce-MOF and CS@Ce-MOF using Cu Kα radiation (λ = 0.15406 nm, 40 kV, 1.64 mA) in the ranges of 10°–80° of 2-theta angle. A NEXUS 6700 infrared spectrometer (FTIR) was used to characterize the chemical structure of Ce-MOF and CS@Ce-MOF in the range of 400–3,000 cm-1. Thermogravimetric analysis (TGA) of the as-prepared samples was conducted by a thermogravimetric analyzer (Netzsch TG 209 F1 Libra, Germany), and the temperature was increased to 800°C at 10 °C/min in a nitrogen atmosphere.
2.4 Antibacterial experiments
The antimicrobial performances of CS@Ce-MOF were evaluated with Escherichia coli and Staphylococcus aureus (Wu et al., 2018). Luria–Bertani (LB) liquid medium was prepared by mixing distilled water, pancreatic peptone, yeast powder, and sodium chloride, and LB solid medium was prepared by mixing distilled water, pancreatic peptone, yeast powder, sodium chloride, and AGAR powder. The prepared liquid medium and solid culture were cultured at 120 °C and sterilized for 15 min. When the solid medium was cooled to about 50–60 °C, it was poured into a Petri dish and solidified and then incubated overnight (12 h) in a constant temperature stirrer (37 °C, 200 rpm). Finally, the CS@Ce-MOF suspension was added to the media of E. coli and S. aureus, respectively, and 100 μL was taken with a pipetting gun, coated onto an LB plate, and cultured at 37 °C for 24 h.
2.5 Antioxidant experiments
In order to test the antioxidant activity of the sample, DPPH and ABTS free radicals were selected as the elimination targets for the assay. We took 0.04 mg/mL DPPH–ethanol solution 1.5 mL, added an appropriate amount of Ce-MOF and CS@Ce-MOF suspension in 95% ethanol to make up 3 mL. It stood for 30 min, and we measured absorbance at 517 nm.
In Formula (1), R is the clearance rate, A1 the absorbance of DPPH and ABTS solution after sample suspension, A2 the absorbance of the sample suspension, and A0 is the absorbance of DPPH and ABTS solution without adding the sample suspension.
2.6 Fruit storage experiments
The preservation effect of CS@Ce-MOF nanozyme spray coating was studied by a fruit preservation experiment. Strawberry was selected as the model fruit. The CS@Ce-MOF suspension (1 mg/mL) was sprayed three times with an airbrush with 50 μL each time on the surface of the fruit to form a film to ensure uniform coating. The fruit was then put into a pre-sterilized box at 25–30 °C with relative humidity (RH) of 65%–70%. The morphological changes and weight loss of the fruit were recorded on days 1, 3, 5, and 7. The weight loss rate of the fruit was measured gravimetrically, and the average weight loss rate of each group of fruit samples was taken as the detection index. The weight loss rate was calculated by the following formula (Pirozzi et al., 2021):
In Formula (2), m0 is the weight of the fruit sample on day 0 before storage and mt is the weight of the fruit sample on day t after storage.
3 Results and discussion
3.1 Structural characterization analysis
First, the morphologies of the synthesized UiO-67 and Ce@UiO-67 were characterized. SEM was used to demonstrate the effective preparation of UiO-67 and Ce@UiO-67. Figure 1A depicts the morphology of the UiO-67 crystals, which have a cuboidal granular structure with a uniform size of approximately 2 mm and a good regular octahedral arrangement. Figure 1B shows the microtopography of Ce-UiO-67, which also showed a relatively intact regular octahedral configuration, indicating that Ce doping penetrated deep into the skeleton rather than just surface adhesion. However, the overall crystal structure was slightly broken, indicating that the integrity of the crystals was reduced after the addition of cerium ion. Figure 1C shows the EDX energy spectrum of Ce@UiO-67, from which it is apparent that the component elements of Ce@UiO-67 are Zr, Ce, Cl, C, and O. This indicates that the Ce-UiO-67 preparation was successful. To further demonstrate the successful preparation of Ce-MOF and CS@Ce-MOF, XRD patterns of Ce-MOF and CS@Ce-MOF are shown in Figure 1D. For Ce-MOF, the main diffraction peaks are found at 2θ = 5.8°, 15.4°, and 24.2°; for CS@Ce-MOF, the main diffraction peaks are found at 2θ = 5.8°, 10.2°, 15.4°, and 30.2°. The position of the characteristic diffraction peaks of the two samples is basically the same, indicating that the crystal structure is also the same (Travlou et al., 2018; Yang et al., 2020). In addition, the samples presented a broad peak of around 5°, implying that amorphous Ce-MOF was formed on the surface of CS (Thomas et al., 2018), and in the diffractogram of CS@Ce-MOF, there is a peak at 10° that may be the CS. This furthermore revealed that the crystal structure integrities of CS@Ce-MOF were consistent with Ce-MOF, indicating the presence of Ce-MOF in the composites. The FT-IR spectra of Ce-MOF and CS@Ce-MOF are shown in Figure 1E. For Ce-MOF and CS@Ce-MOF samples, the peaks were at 1,028 cm−1, 1,384 cm−1, and 1,450 cm−1. The appearance of the peak at 1,450 cm−1 corresponded to the stretching vibrations of the coordinated Ce-O and Ce-O-C metal nodes, indicating the successful coordination of cerium ions with the H2BDC (Ren et al., 2019). In addition, the thermal properties of CS and CS@Ce-MOF were tested by TGA at temperatures ranging from 30 °C to 800°C. For the thermogravimetric analysis in Figure 1F, the degradation temperature of CS was 220 °C in the thermograms of CS, but the decomposition temperature of CS@Ce-MOF was about 300 °C, indicating an interaction between CS and Ce-MOF. However, a weight loss of over 350°C would indicate the gradual carbonization of CS and framework collapse of Ce-MOF (Sharma et al., 2022). At the same time, it shows that CS@Ce-MOF has thermal stability within 350°C.
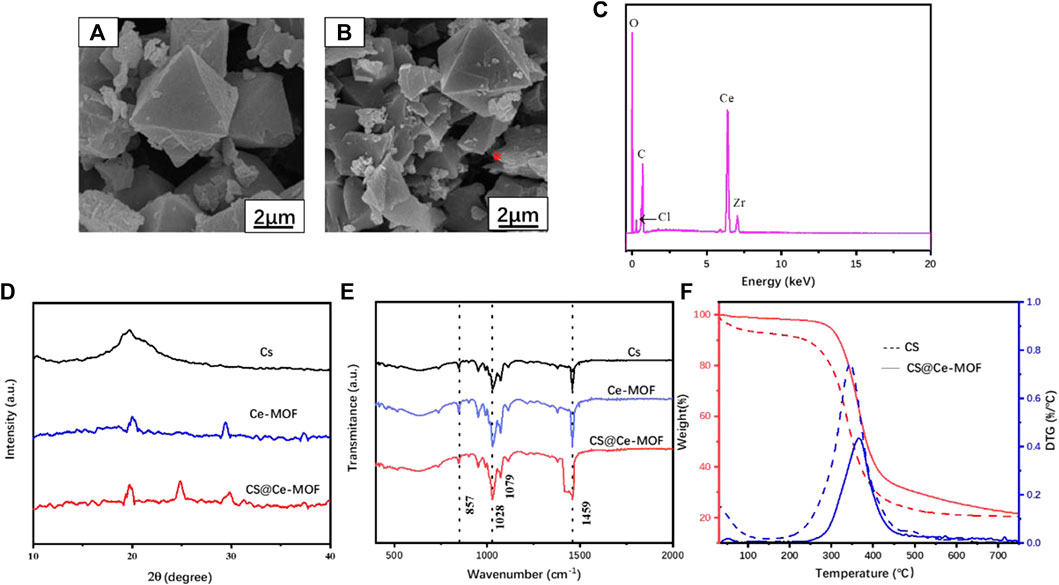
Figure 1. (A, B) SEM images of UiO-67 and Ce@UiO-67; (C) EDX energy spectrum of Ce@UiO-67; (D) XRD patterns of CS, Ce-MOF, and CS@Ce-MOF; (E) FT-IR spectra of Cs, Ce-MOF, and CS@Ce-MOF; (F) thermogravimetric analysis of CS and CS@Ce-MOF.
3.2 Optical properties of CS@Ce-MOF
The optical properties of spray coating components affect the preservation of fruit; thus, we investigated the optical properties of the CS@Ce-MOF spray coating. Figure 2A shows the ultraviolet-visible transmission spectrum in the range of 300–800 nm for the CS and CS@Ce-MOF suspension. Figure 2B shows the ultraviolet-visible adsorption spectrum in the range of 300–800 nm of the CS and CS@Ce-MOF suspension. It can be observed that, in the range of visible light (400–800 nm), the CS suspension has a high transmittance of nearly 80% while the CS@Ce-MOF suspension has a transmittance of about 40%. In the range of ultraviolet light (300–400 nm), CS@Ce-MOF suspension has a lower transmittance of about 40% (Figure 2A). Meanwhile, the CS@Ce-MOF suspension has high adsorption in the range of ultraviolet light (300–400 nm) that is observed from Figure 2B, possibly due to the UV-barrier performance of Ce-MOF. The above results demonstrate that CS@Ce-MOF has good light transmittance, indicating its capability to preserve fruit against discoloration and denaturation.
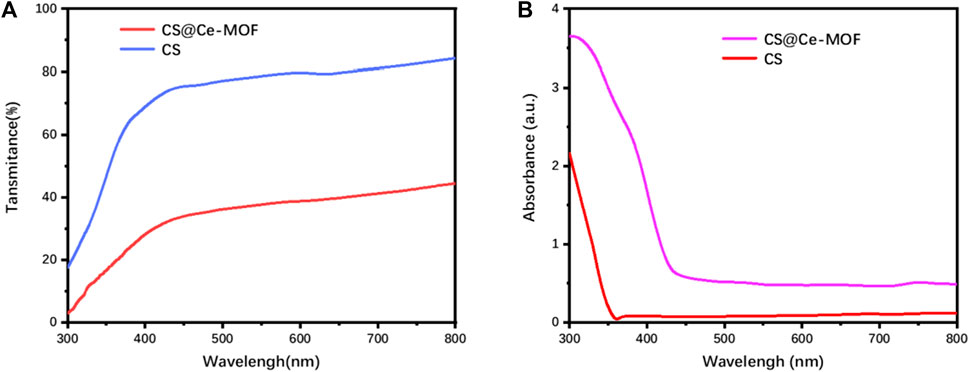
Figure 2. (A) UV–vis transmittance spectra of CS and CS@Ce-MOF suspension; (B) UV–vis adsorption spectra of CS and CS@Ce-MOF suspension.
3.3 XPS analysis of Cs@Ce-MOF
The active spots on the surface of CS@Ce-MOF were characterized by XPS survey. The characteristic peaks attributed to the Ce s2p, O 1s, N 1, and C 1s regions can be seen in Figure 3A. In addition, Figures 3B,C show the high-resolution XPS spectra of Ce and O in Cs@Ce-MOF, with peaks at 88.5 eV and 530.8 eV, indicating the presence of cerium ions. The signal of O 1 s at 530.8 eV could be the Ce-O bond in the composite, revealing the successful preparation of CS@Ce-MOF (An et al., 2014).
3.4 Antibacterial performance of CS@Ce-MOF
In order to explore the antibacterial activity of the CS@Ce-MOF nanozyme, the antibacterial activity of CS@Ce-MOF against E. coli and S. aureus was investigated by the plate counting method (Yang et al., 2020). Figure 4A shows the number of E. coli and S. aureus colonies remaining after Ce-MOF and CS@Ce-MOF treatment. We could observe an obvious reduction of plate colonies when treated with Ce-MOF. More importantly, only a few plate colonies were found in the groups treated by CS@Ce-MOF for all these bacterial samples. In addition, separate quantification results for the plate count assay are displayed in Figures 4B,C for E. coli and S. aureus. It can be observed from Figures 4A,B that CS@Ce-MOF has better bacteriostatic performance than Ce-MOF; in particular, the bacteriostatic rate of CS@Ce-MOF to S. aureus reaches 94.5%. The results indicate that CS@Ce-MOF shows better antibacterial properties with longer lasting antibacterial duration against E. coli and S. aureus.
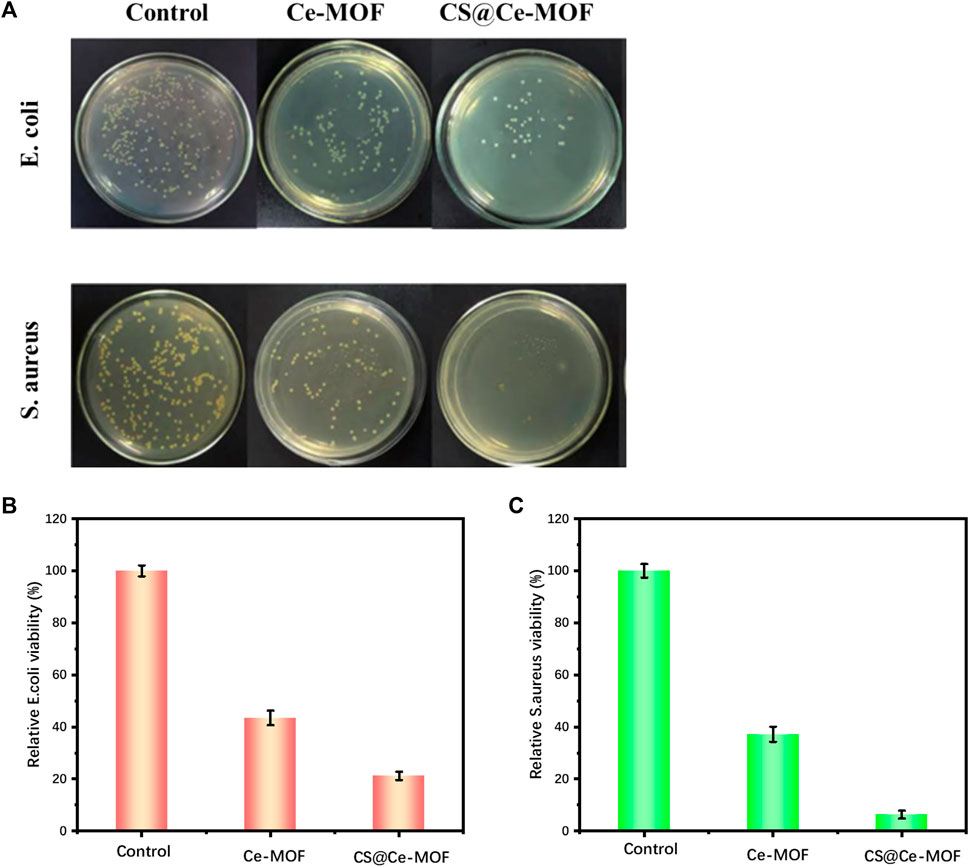
Figure 4. (A) AGAR plates treated with Ce-MOF and CS@Ce-MOF for E. coli and S. aureus; (B) bacterial viability rates of E. coli and (C) S. aureus.
The CS@Ce-MOF nanozyme thus exhibited promise in combating foodborne pathogenic bacteria due to its antibacterial properties. These allow the nanozyme to efficiently degrade harmful biofilms and inhibit bacterial growth, making it an excellent candidate for food industry applications such as disinfection and preservation. Additionally, the broad-spectrum biocidal activity of the CS@Ce-MOF nanozyme makes it useful for targeting multiple types of foodborne pathogens, thus ensuring a safer food supply chain. Such antibacterial performance qualifies CS@Ce-MOF to serve as a spray coating for fruit preservation.
3.5 Analysis of antioxidant activity
The oxidation of vitamins in fruit is one of the main causes of nutrient loss and deterioration (Bobasa et al., 2023). Therefore, fruit preservation coatings require excellent antioxidant properties. We tested the antioxidant properties of the prepared Cs, Ce-MOF, and CS@Ce-MOF with free-radical scavenging DPPH and ABTS (Figure 4). It can be seen from Figure 5A that, with the increase in the extract concentration, the ability of free-radical scavenging is enhanced. When the sample concentration reached 0.5 mg/mL, the DPPH free-radical clearance rate of the CS@Ce-MOF reached more than 80%. In addition, it can be observed from Figure 5B that both Ce-MOF and CS@Ce-MOF have slightly better scavenging ability for ABTS than DPPH. When the sample concentration reached 0.5 mg/mL, the DPPH free-radical clearance rate of the CS@Ce-MOF reached 85%. This result shows that the antioxidant activity of the CS@Ce-MOF suspension is very high and can be widely used in food preservation.
3.6 Fruit preservation study
A fruit preservation experiment was conducted to explore the utilization of CS@Ce-MOF nanozyme for food preservation. We choose strawberry and banana, which are not easy to store, for a fruit storage experiment. Figure 6A shows the comparison of the appearance changes of strawberries under different treatments during storage. It can be observed that untreated strawberries began to show signs of decay after storage for 5 days, and, after storage for 5 days, most of the pulp was clearly rotten. Strawberries sprayed with CS@Ce-MOF nanozyme suspension looked fresher and remained fresh after storage for 5 days and only showed tiny signs of decay after 7 days of storage. As a typical fruit of respiratory menopause, water in strawberry is easily consumed and diffused to the external environment during postharvest storage due to respiration and transpiration, resulting in its reduced water content and weight. Figure 6B shows the changing trend of the weight loss rate of strawberry during storage. It is apparent that the weight-loss rate of strawberry generally rises during the whole storage cycle; the strawberry samples without any preservation treatment have serious water loss, and the weight-loss rate rises faster, reaching 43% after 7 days of storage. The weight-loss rate of strawberries treated by spraying CS@Ce-MOF nanozyme suspension increased slowly, reaching 29% after 7 days of storage, confirming the good effect of the spray in preventing fruit rot. Morphological observation of bananas at different times with various treatments is shown in Figure 6c. During the green-yellow life of banana (0–3 days of storage), CS@Ce-MOF nanozyme spray coating slowed chlorophyll degradation. During the yellow-brown life of bananas (5–7 days of storage), CS@Ce-MOF nanozyme spray coating further reduced the incidence of browning spots on the fruit surfaces compared to uncoated bananas (Deng et al., 2017). After 7 days, the weight of control bananas at room temperature dropped by more than 20% while the sprayed CS@Ce-MOF nanozyme bananas retained about 90% of original weight (Figure 6D). These results suggest that the CS@Ce-MOF nanozyme as a bio-inspired spray coating can protect the freshness of fruit during storage and can significantly delay its decay, with shelf-life extension of up to 4 days.
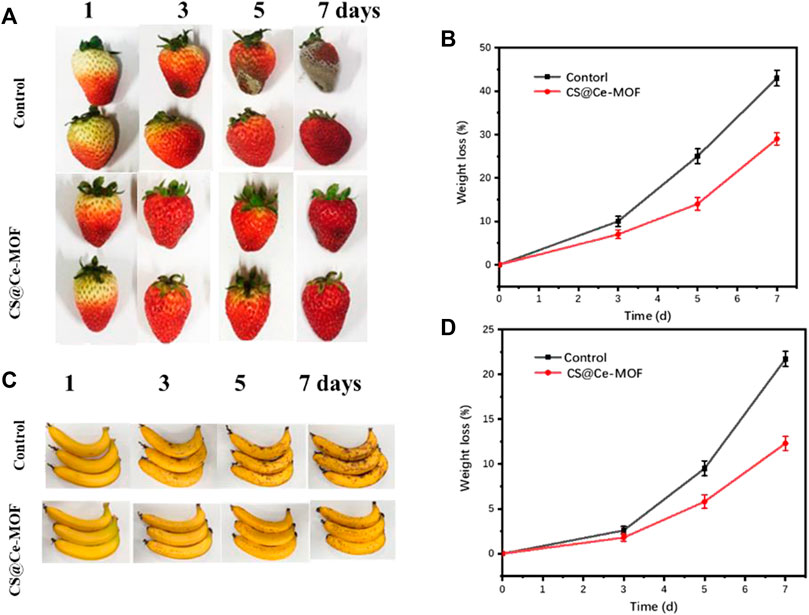
Figure 6. (A) Appearance of strawberries during storage; (B) weight loss rates of strawberries with different treatments; (C) appearance of bananas during storage; (D) weight loss rates of bananas with different treatments.
4 Conclusion
CS@Ce-MOF nanozyme spray coating was successfully prepared for fruit preservation. Combining Ce-MOF with CS creates CS@Ce-MOF. This method is a possible strategy for preparing a biocatalytic coating with good stability and anti-microbial properties for fruit preservation. The two materials before and after modification were then characterized by XRD, FT-IR, SEM, EDX, and other basic methods. It was demonstrated that the modified MOFs not only successfully introduced cerium element but also maintained good morphology and crystallinity. Through experimental research, we found that the CS@Ce-MOF bionanocomposites attained UV-blocking capability, antibacterial activity, and isolation ability against O2 and moisture. In addition, spraying CS@Ce-MOF suspension effectively extended the freshness and enhanced the quality of strawberries and bananas during storage. The findings of this research contribute to the advancement of metal-containing nano-materials and the use of biocompatible nanozymes. Furthermore, this research has potential application in the preservation of fresh food, particularly fruit.
Data availability statement
The original contributions presented in the study are included in the article/Supplementary Material; further inquiries can be directed to the corresponding author.
Author contributions
JL: formal analysis, project administration, writing–original draft, and writing–review and editing. DW: data curation, investigation, writing–original draft, and writing–review and editing. YL: data curation, methodology, supervision, writing–original draft, and writing–review and editing.
Funding
The author(s) declare that financial support was received for the research, authorship, and/or publication of this article. The project was supported by the Natural Science Foundation of Heilongjiang Province: Theoretical and Experimental Study on Heat and Mass Transfer of Physalis pubescens Modified Atmosphere Preservation (Fund Projects Number: LH 2023E027).
Conflict of interest
The authors declare that the research was conducted in the absence of any commercial or financial relationships that could be construed as a potential conflict of interest.
Publisher’s note
All claims expressed in this article are solely those of the authors and do not necessarily represent those of their affiliated organizations or those of the publisher, the editors, and the reviewers. Any product that may be evaluated in this article, or claim that may be made by its manufacturer, is not guaranteed or endorsed by the publisher.
References
Alavijeh, R. K., Beheshti, S., Akhbari, K., and Morsali, A. (2018). Investigation of reasons for metal-organic framework's antibacterial activities. Polyhedron 156, 257–278. doi:10.1016/j.poly.2018.09.028
An, J., Ji, Z., Wang, D., Luo, Q., and Li, X. (2014). Preparation and characterization of uniform-sized chitosan/silver microspheres with antibacterial activities. Mater. Sci. Eng. C 36, 33–41. doi:10.1016/j.msec.2013.11.037
Aryanejad, S., Bagherzade, G., and Moudi, M. (2019). Design and development of novel Co-MOF nanostructures as an excellent catalyst for alcohol oxidation and Henry reaction, with a potential antibacterial activity. Appl. Organomet. Chem. 33 (6), 4820. doi:10.1002/aoc.4820
Bai, Y., Qiu, T., Chen, B., Shen, C., Yu, C., Luo, Z., et al. (2023). Formulation and stabilization of high internal phase emulsions: stabilization by cellulose nanocrystals and gelatinized soluble starch. Carbohydr. Polym. 312, 120693. doi:10.1016/j.carbpol.2023.120693
Bobasa, E. M., Srivarathan, S., Phan, A. D. T., Netzel, M. E., Cozzolino, D., and Sultanbawa, Y. (2023). Influence of blanching on the bioactive compounds of Terminalia ferdinandiana Exell fruit during storage. J. Food Meas. Charact. 17 (1), 244–252. doi:10.1007/s11694-022-01581-1
Chai, Y. D., Pang, Y. L., Lim, S., Chong, W. C., Lai, C. W., and Abdullah, A. Z. (2022). Recent progress on tailoring the biomass-derived cellulose hybrid composite photocatalysts. Polymers 14 (23), 5244. doi:10.3390/polym14235244
Chen, M., Cen, H., Guo, C., Gao, J., Chen, Y., Zhang, Y., et al. (2020). Impact of gonadotropin-releasing hormone agonist pre-treatment on the cumulative live birth rate in infertile women with adenomyosis treated with IVF/ICSI: a retrospective cohort study. J. Mol. Liq. 11, 318. doi:10.3389/fendo.2020.00318
Deng, Z., Jung, J., Simonsen, J., and Zhao, Y. (2017). Cellulose nanomaterials emulsion coatings for controlling physiological activity, modifying surface morphology, and enhancing storability of postharvest bananas (Musa acuminate). Food Chem. 232, 359–368. doi:10.1016/j.foodchem.2017.04.028
Deng, Z., Wu, Z., Tan, X., Deng, F., Chen, Y., Chen, Y., et al. (2022). Preparation, characterization and antibacterial property analysis of cellulose nanocrystals (CNC) and chitosan nanoparticles fine-tuned starch film. Molecules 27 (23), 8542. doi:10.3390/molecules27238542
Deng, Z., Zhu, K., Li, R., Zhou, L., and Zhang, H. (2020). Cellulose nanocrystals incorporated β-chitosan nanoparticles to enhance the stability and in vitro release of β-galactosidase. Food Res. Int. 137, 109380. doi:10.1016/j.foodres.2020.109380
Ebrahimi, A., Zabihzadeh Khajavi, M., Ahmadi, S., Mortazavian, A. M., Abdolshahi, A., Rafiee, S., et al. (2022). Novel strategies to control ethylene in fruit and vegetables for extending their shelf life: a review. Int. J. Environ. Sci. Technol. 19 (5), 4599–4610. doi:10.1007/s13762-021-03485-x
Fang, Y., Wu, W., Qin, Y., Liu, H., Lu, K., Wang, L., et al. (2023). Recent development in antibacterial activity and application of nanozymes in food preservation. Crit. Rev. Food Sci. Nutr. 63, 9330–9348. doi:10.1080/10408398.2022.2065660
Garkani Nejad, F., Beitollahi, H., and Sheikhshoaie, I. (2023). A UiO-66-NH2 MOF/PAMAM dendrimer nanocomposite for electrochemical detection of tramadol in the presence of acetaminophen in pharmaceutical formulations. Biosensors 13 (5), 514. doi:10.3390/bios13050514
He, B., Yu, H., and Zhang, L. (2022). Preparation of potato starch/PVA composite film and its application in pork preservation. China Plast. Ind. 50 (6), 118–124.
Huang, L., Sun, D.-W., Pu, H., and Wei, Q. (2019). Development of nanozymes for food quality and safety detection: principles and recent applications. Compr. Rev. Food Sci. Food Saf. 18 (5), 1496–1513. doi:10.1111/1541-4337.12485
Joung, J., Boonsiriwit, A., and Kim, M. (2021). Application of ethylene scavenging nanocomposite film prepared by loading potassium permanganate-impregnated halloysite nanotubes into low-density polyethylene as active packaging material for fresh produce. LWT-Food Sci. Technol. 145, 111309. doi:10.1016/j.lwt.2021.111309
Jung, J., Deng, Z., and Zhao, Y. (2019). A review of cellulose nanomaterials incorporated fruit coatings with improved barrier property and stability: principles and applications. J. Food Process Eng. 43 (2). doi:10.1111/jfpe.13344
Kalinkevich, O. V., Kalinkevich, A. N., and Danilchenko, S. N. (2018). “Chitosan-based composite materials comprising metal or metal oxide nanoparticles synthesis, characterization and antimicrobial activity,” in Proceedings of the 2018 IEEE 8th International Conference Nanomaterials: Application and Properties (NAP-2018), Zatoka, Ukraine, 09-14 September 2018 (IEEE).
Li, S., Liu, X., Chai, H., and Huang, Y. (2018). Recent advances in the construction and analytical applications of metal-organic frameworks-based nanozymes. TRAC-Trends Anal. Chem. 105, 391–403. doi:10.1016/j.trac.2018.06.001
Li, Z., Liu, G., Fan, C., and Pu, S. (2021). Ratiometric fluorescence for sensitive detection of phosphate species based on mixed lanthanide metal organic framework. Anal. Bioanal. Chem. 413 (12), 3281–3290. doi:10.1007/s00216-021-03264-0
Mantilla, N., Castell-Perez, M. E., Gomes, C., and Moreira, R. (2013). Multilayered antimicrobial edible coating and its effect on quality and shelf-life of fresh-cut pineapple (Ananas comosus). LWT-Food Sci. Technol. 51 (1), 37–43. doi:10.1016/j.lwt.2012.10.010
Meng, F., Zhu, P., and Yang, L. (2023). Nanozymes with atomically dispersed metal centers: structure-activity relationships and biomedical applications. Chem. Eng. J. 452, 1.
Natalini, A., Martinez-Diaz, V., Ferrante, A., and Pardossi, A. (2021). Effect of slicing and storage temperatures on biochemical aspects of membrane integrity in two different genotypes of tomato. J. Sci. Food Agric. 101 (14), 6134–6142. doi:10.1002/jsfa.11479
Ni, X., Yu, J., and Shao, P. (2021). Preservation of Agaricus bisporus freshness with using innovative ethylene manipulating active packaging paper. Food Chem. 345, 128757. doi:10.1016/j.foodchem.2020.128757
Nor, S. M., and Ding, P. (2020). Trends and advances in edible biopolymer coating for tropical fruit: a review. Food Res. Int. 134, 109208. doi:10.1016/j.foodres.2020.109208
Park, T. J., Lee, K. G., and Lee, S. Y. (2016). Advances in microbial biosynthesis of metal nanoparticles. Appl. Microbiol. Biotechnol. 100 (2), 521–534. doi:10.1007/s00253-015-6904-7
Pathare, P. B., Al-Dairi, M., Al-Yahyai, R., and Al-Mahdouri, A. (2023). Physiological response of stored pomegranate fruit affected by simulated impact. Foods 12 (6), 1122. doi:10.3390/foods12061122
Pirozzi, A., Ferrari, G., and Donsi, F. (2021). The use of nanocellulose in edible coatings for the preservation of perishable fruits and vegetables. Coatings 11 (8), 990. doi:10.3390/coatings11080990
Pontesegger, N., RüHMER, T., and Siegmund, B. (2023). Physicochemical attributes, aroma profile, and sensory quality of organic crimson crisp apples after storage. Foods 12 (9), 1876. doi:10.3390/foods12091876
Rao, H., Xue, X., Luo, M., Liu, H., and Xue, Z. (2021). Recent advances in the development of colorimetric analysis and testing based on aggregation-induced nanozymes. Chin. Chem. Lett. 32 (1), 25–32. doi:10.1016/j.cclet.2020.09.017
Ren, X., Yang, C., Zhang, L., Li, S., Shi, S., Wang, R., et al. (2019). Copper metal–organic frameworks loaded on chitosan film for the efficient inhibition of bacteria and local infection therapy. Nanoscale 11 (24), 11830–11838. doi:10.1039/c9nr03612a
Sharma, V. K., Sharma, M., Usmani, Z., Pandey, A., Singh, B. N., Tabatabaei, M., et al. (2022). Tailored enzymes as next-generation food-packaging tools. Trends Biotechnol. 40 (8), 1004–1017. doi:10.1016/j.tibtech.2022.01.009
Sheng, J., Wu, Y., Ding, H., Feng, K., Shen, Y., Zhang, Y., et al. (2023). Multienzyme-like nanozymes: regulation, rational design, and application. Adv. Mater. 2023, e2211210. doi:10.1002/adma.202211210
Slavin, Y. N., Asnis, J., Hafeli, U. O., and Bach, H. (2017). Metal nanoparticles: understanding the mechanisms behind antibacterial activity. J. Nanobiotechnology 15, 65. doi:10.1186/s12951-017-0308-z
Souza, B. W. S., Cerqueira, M. A., Teixeira, J. A., and Vicente, A. A. (2010). The use of electric fields for edible coatings and films development and production: a review. FoodD Eng. Rev. 2 (4), 244–255. doi:10.1007/s12393-010-9029-x
Stuparu-Cretu, M., Braniste, G., Necula, G.-A., Stanciu, S., Stoica, D., and Stoica, M. (2023). Metal oxide nanoparticles in food packaging and their influence on human health. Foods 12 (9), 1882. doi:10.3390/foods12091882
Thomas, B., Raj, M. C., Athira, K. B., H, R. M., Joy, J., Moores, A., et al. (2018). Nanocellulose, a versatile green platform: from biosources to materials and their applications. Chem. Rev. 118 (24), 11575–11625. doi:10.1021/acs.chemrev.7b00627
Travlou, N. A., Algarra, M., Alcoholado, C., Cifuentes-Rueda, M., Labella, A. M., Lázaro-Martínez, J. M., et al. (2018). Carbon quantum dot surface-chemistry-dependent Ag release governs the high antibacterial activity of Ag-Metal–Organic framework composites. ACS Appl. Bio Mater. 1 (3), 693–707. doi:10.1021/acsabm.8b00166
Valenzuela, J. L. (2023). Advances in postharvest preservation and quality of fruits and vegetables. Foods 12 (9), 1830. doi:10.3390/foods12091830
Vargas, M., and Gonzalez-Martinez, C. (2010). Recent patents on food applications of chitosan. Nutr. Agric. 2 (2), 121–128. doi:10.2174/2212798411002020121
Vodyanoy, V. (2021). The role of endogenous metal nanoparticles in biological systems. Biomolecules 11 (11), 1574. doi:10.3390/biom11111574
Wang, H., Lashkari, E., Lim, H., Zheng, C., Emge, T. J., Gong, Q., et al. (2016). The moisture-triggered controlled release of a natural food preservative from a microporous metal-organic framework. Chem. Commun. 52 (10), 2129–2132. doi:10.1039/c5cc09634k
Wu, Z., Huang, X., Li, Y.-C., Xiao, H., and Wang, X. (2018). Novel chitosan films with laponite immobilized Ag nanoparticles for active food packaging. Carbohydr. Polym. 199, 210–218. doi:10.1016/j.carbpol.2018.07.030
Yang, Y., Wu, X., He, C., Huang, J., Yin, S., Zhou, M., et al. (2020). Metal–organic framework/Ag-based hybrid nanoagents for rapid and synergistic bacterial eradication. ACS Appl. Mater. Interfaces 12 (12), 13698–13708. doi:10.1021/acsami.0c01666
Zhang, Y. C., Lin, Z. H., and He, Q. W. (2022). Enhanced aqueous stability and long-acting antibacterial of silver-based MOFs via chitosan-crosslinked for fruit fresh-keeping. Appl. Surf. Sci. 571, 151351. doi:10.1016/j.apsusc.2021.151351
Zhang, B., Luo, Y., Kanyuck, K., Bauchan, G., Mowery, J., and Zavalij, P. (2016). Development of metal-organic framework for gaseous plant hormone encapsulation to manage ripening of climacteric produce. J. Agric. Food Chem. 64 (25), 5164–5170. doi:10.1021/acs.jafc.6b02072
Zhang, Y., Yuan, S., and Chen, X. (2023). Basin scale soil moisture estimation with grid SWAT and LESTKF based on WSN. Food Packag. Shelf Life 24, 35. doi:10.3390/s24010035
Zhao, J., Wei, F., and Xu, W. (2020). Enhanced antibacterial performance of gelatin/chitosan film containing capsaicin loaded MOFs for food packaging. Appl. Surf. Sci. 510, 145418. doi:10.1016/j.apsusc.2020.145418
Keywords: metal–organic framework, nanozyme, bioinspired spray coating, fruit preservation, CS@Ce-MOF
Citation: Li J, Wang D and Liu Y (2024) Biomimetic spray coating for fruit preservation based on UiO-1 67 metal–organic framework nanozyme. Front. Mater. 11:1303449. doi: 10.3389/fmats.2024.1303449
Received: 03 October 2023; Accepted: 05 April 2024;
Published: 12 April 2024.
Edited by:
Hailan Kang, Shenyang University of Chemical Technology, ChinaCopyright © 2024 Li, Wang and Liu. This is an open-access article distributed under the terms of the Creative Commons Attribution License (CC BY). The use, distribution or reproduction in other forums is permitted, provided the original author(s) and the copyright owner(s) are credited and that the original publication in this journal is cited, in accordance with accepted academic practice. No use, distribution or reproduction is permitted which does not comply with these terms.
*Correspondence: Donglin Wang, dlj0175@cau.edu.cn