Ultrastructure of silk threads of the water spider Argyroneta aquatica (Clerck, 1757) (Araneae, Cybeidae) in comparison with that of some mites
- Zoological Institute of the Russian Academy of Science, St. Petersburg, Russia
Silk of the water spider Argyroneta aquatica (Clerck, 1757) was obtained in the laboratory in the form of a capturing web irrespective of the formation of a diving bell and egg cocoon. Silk threads were examined by light microscopy, scanning (SEM), and transmission electron microscopy (TEM) as well as by atomic force (AFM) microscopy. Based on the diameter and internal organization, the silk threads have been divided into five types—a-, b-, c-, d-, and e-types interlaced freely in the thread bundles taken for examinations. Threads of all types are infinitely long, non-branched, round in shape, uniform in width through their course, and rarely found to be ruptured. Threads of the a-type are mostly straight, up to 1,000 nm in diameter, and composed of a fibrillary wall enveloping an electron-dense vacuolated core, which may be occasionally absent. The wall may also become lost, leaving a single core substance surrounded by a slightly wrinkled double membrane. Threads of the b-, c-, and d-types are organized nearly identical, being composed of a fine granular electron-light uniform material enveloped by a fine membrane. The diameter of these threads varies from max. 500 nm in the b-type and 120 nm in the c-type to around 50 nm in the d-type. Threads of these types are more convoluted and more numerous than threads of the a-type. Threads of the last kind, the e-type, occur rarely and are significantly larger with a diameter of around 3.5–4.5 µm. They possess the layered membranous wall surrounding a uniformly dense core. The silk of the water mites Limnochares aquatica (L., 1758) and Limnesia maculata (O.F. Müller, 1776) is composed of threads of only one type that closely corresponded to the a-type threads of A. aquatica. Conversely, the silk of the two-spotted spider mite Tetranychus urticae (C.L. Koch, 1836) consists of threads highly correlated with the d-type threads of A. aquatica. This finding shows that in different phylogenetic lineages of arachnids, the mechanisms of silk production are quite similar.
1 Introduction
All spiders are known to produce silk for the whole life and for realization of different vital functions (Foelix, 1996; Craig, 1997; Craig et al., 2000; Craig, 2003; Krafft and Cookson, 2012). Correspondingly, spiders possess several kinds of abdominal silk glands and up to six types of silk of different morphology, amino acid content, and protein structure (Foelix, 1996; Craig et al., 2000; Blamires et al., 2017). Representatives of other arthropod groups belonging to different evolutionary lineages, such as insects, crustaceans, pseudoscorpions, and mites, which produce silk, reveal only one type of silk glands synthesizing silk for a quite local and specific functional task (e.g., Sutherland et al., 2010; Annamalai and Jayaprakash, 2012; Kronenberger et al., 2012; Kakui and Hiruta, 2014; Büsse et al., 2015; Osborn Popp et al., 2016). Consequently, both glands and silks in arthropods are expected to be non-homologues because they are thought to have developed many times in the course of evolution of different groups (Sehnal and Akai, 1990; Craig et al., 2000; Young and Merritt, 2003; Sutherland et al., 2010; Ashton et al., 2011; Büsse et al., 2015). At the same time, based on the molecular data, it was hypothesized that “the spider orb web evolved only once” in the evolution (Blackledge et al., 2009).
Silks in arthropods and, in particular, in spiders are mostly built of fibrillary proteins (Kovoor and Zylberberg, 1980, 1982; Stubbs et al., 1992; Gosline et al., 1999; Sponner et al., 2005; Yonemura et al., 2006; Sponner et al., 2007; Blackledge et al., 2009; Blackledge, 2013; Arakawa et al., 2022) but, owing to their different origin, show quite different composition and structural properties (Vollrath et al., 1996; Gosline et al., 1999; Craig et al., 2000; Vollrath, 2000; Vollrath and Knight, 2001; Bakker et al., 2006; Schniepp et al., 2023). On the fine microscopic level, it was shown that generally each individual silk fiber might consist of an envelope, variously expressed, and of a core inward substance containing various fibrils differently organized (Kovoor and Zylberberg, 1980; Stubbs et al., 1992; Li et al., 1994; Vollrath et al., 1996; Sponner et al., 2007; Riekel et al., 2019, 2020; Blamires et al., 2023; Perera et al., 2023). Silk threads reveal a heterogeneous protein composition (Stubbs et al., 1992; Wang et al., 2022) and the presence of lipids in the envelope (core-skin structure) (Sponner et al., 2007). The expression of these two components of the spider silk fibers and their composition varies significantly in different spider species (Gould et al., 1999; Knight and Vollrath, 2002; Hajer et al., 2009). There is no doubt that silk in its different forms and composition, providing important functions, significantly increases the vital resources of arthropods (Alberti and Ehrnsberger, 1977; Manson and Gerson, 1996; Hajer et al., 2009; Clotuche et al., 2011; Kronenberger et al., 2012; Kakui and Hiruta, 2014; Hatano and Nagashima, 2015; Blamires et al., 2017).
Silk of the water spider Argyroneta aquatica (Clerck) was investigated previously by SEM methods with respect to diving bell and egg cocoon (Bakker et al., 2006). The authors recognized four types of silk threads in this species–threads of the diving bell, anchor threads, walking threads, and threads of the egg cocoon. Only threads of the first type show a diameter bigger than 1 µm; threads of other types seem to be much delicate and thinner (Bakker et al., 2006). The structure and function of the “attachment discs” in this species, providing the attachment of silk threads to substrates under water, were studied in detail recently (Schaber et al., 2023). The attachment discs, with special regard to piriform silk, was also studied in representatives of four aerial spider families using TEM methods (Wirth et al., 2019).
Surprisingly, the phenomenon of silk production was discovered in water mites (Acariformes, Hydrachnidia) (Shatrov et al., 2014, 2016; Shatrov et al., 2023) as related to the activity of their dermal glands (Shatrov, 2013; Shatrov and Soldatenko, 2016, 2022)—an acquisition and synapomorphy of freshwater mites after their ancestors have come down to fresh waters ca. 235 MYA (Dabert et al., 2016). The silk of Limnochares aquatica (L.) (Limnocharidae) and Limnesia maculata (O.F. Müller) (Limnesiidae) and some other water mite species was examined using both SEM and TEM methods. It was shown that silk in these two species is represented by only one type of threads quite similar in their organization (Shatrov et al., 2014, 2016; Shatrov et al., 2023). These threads are extremely long, unbranched, uniform tubes of around 1 to 2 µm in diameter containing a core substance (residual substance) or without it. In the latter case, silk threads are flattened on the substrate, as observed by SEM, resembling an elastic ribbon. With this character, silk threads of water mites are similar to ribbon-like threads of sicarid spider Loxosceles laeta (Nicolet) (Knight and Vollrath, 2002; Schniepp et al., 2023). In both cases, these ribbon-like threads are able to stick together either by van der Waal’s forces (Knight and Vollrath, 2002) or by the adhesiveness of the thread surface itself which bears fine fibrils of the thread’s wall rising vertically (Shatrov et al., 2023). This feature seems to give a possibility to web function as an elastic net (Knight and Vollrath, 2002).
In terrestrial mites, the production of silk is known in representatives of several families (Tetranychidae, Eriophyidae, Camerobiidae, Cunaxidae, and Bdellidae) (Wallace and Mahon, 1972; Bolland, 1983; Gerson, 1985; Alberti and Coons, 1999; Shin et al., 2006). Silk in these mites provides many important vital functions, such as in prey capturing, communication, migration, nest buildings, and others (Manson and Gerson, 1996; Clotuche et al., 2011; Kanazawa et al., 2011; Le Goff et al., 2011; Fernandez et al., 2012; Yano, 2012). Apparently, the same role is played by silk in other arthropods. Unfortunately, among many sophisticated methods applied for the study of the silk structure and composition in spiders and other arthropods, TEM methods were used only sporadically (Kovoor and Zylberberg, 1980; Stubbs et al., 1992; Li et al., 1994; Vollrath et al., 1996; Sponner et al., 2007; Wirth et al., 2019; Perera et al., 2023).
The main purpose of this study is to examine the ultrastructural organization of silk in the water spider Argyroneta aquatica (Clerck, 1757) (Araneae, Cybeidae) in comparison with that of some acariform mites, both water mites Limnochares aquatica (L., 1758) (Limnocharidae) and Limnesia maculata (O.F. Müller, 1776) (Limnesiidae), studied previously (Shatrov et al., 2016, Shatrov et al., 2023) and terrestrial aerial tetranychid two-spotted spider mite Tetranychus urticae (C.L. Koch, 1836) (Tetranychidae) studied previously only by SEM (Gerson, 1985).
2 Materials and methods
2.1 Animals and laboratory observations
The main task of the laboratory maintenance of different arachnids in our experiments was to collect pure web samples. For this reason, we have eliminated from the experiments, whenever possible, all interfering unnecessary factors (excess food, any substrate, plant leaves, etc.).
2.1.1 Water spider Argyroneta aquatica (Clerck, 1757)
One specimen (adult female spider, around 10 mm long) (Figure 1A) was captured on June 22, 2023 in the river of Panika, Volgograd province, 50°48′50.0″ N, 42°27′15.9″ E. The spider was identified with the Identification Key (Tsalolokhin, 1997). The spider was kept in a 100-mL plastic vial (Figure 1B) in natural waters, which were regularly added and changed, for the period of expedition until August 2023. During this time, the spider was not artificially fed. Furthermore, in the laboratory, the natural water was changed to pure bottled water. In the laboratory, observations were proceeded at room temperature until the spider died on October 10, 2023. During this period, the spider was fed with Daphnia magna (Straus), purchased commercially (Figure 1A), and Asellus aquaticus (L.), captured in the river of Teplaya, Leningrad province, Gatchina district, and further cultured in the laboratory. The food was inserted into the vial at no more than two times a week, and the volume of food did not exceed the size of the spider itself. The vial cap was not tightly sealed for inflow of oxygen and air exchange. The spider periodically rose into the air space between the water surface and the cap, where it spent several hours at a time.
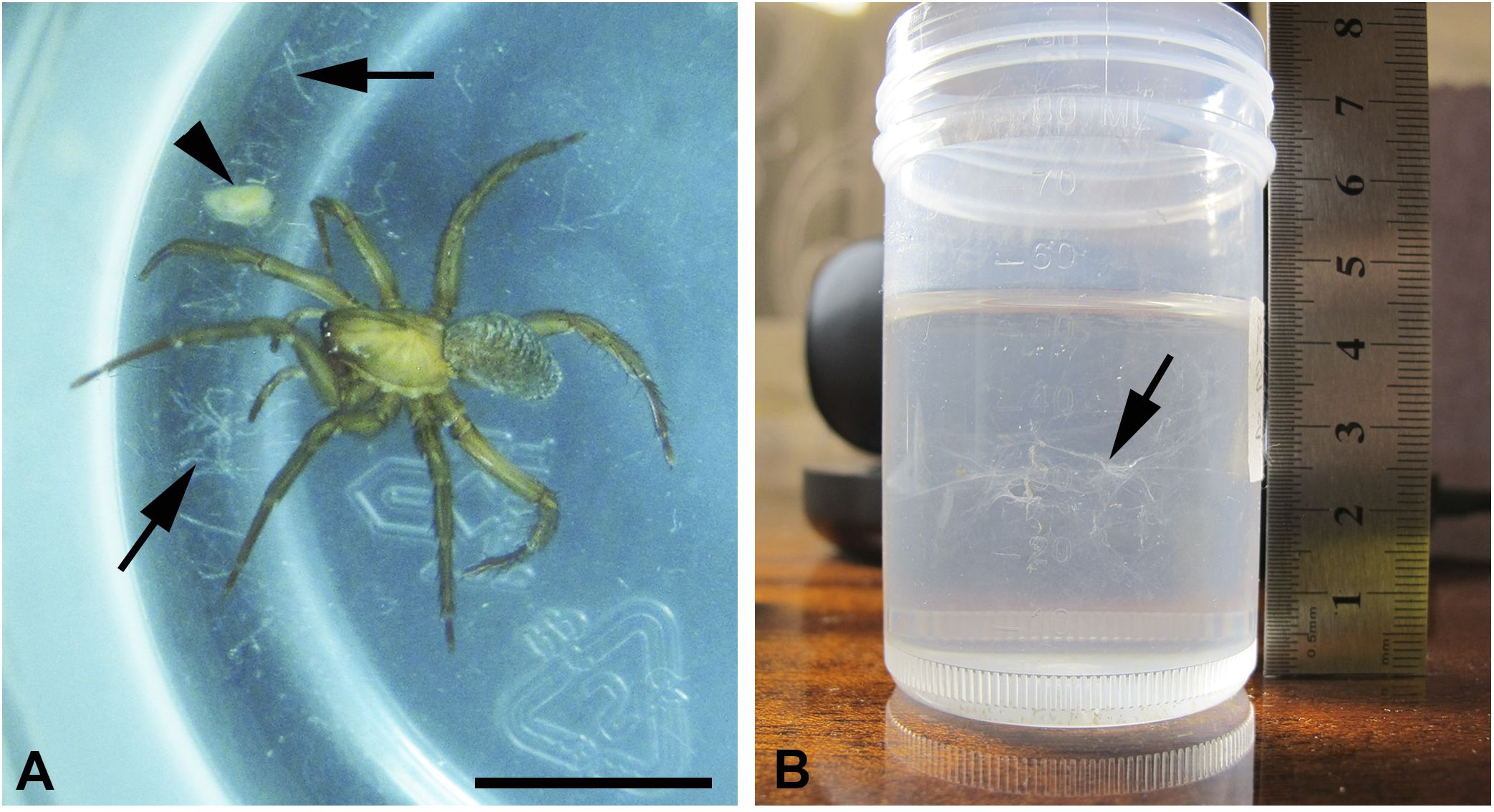
Figure 1 (A, B) Laboratory maintenance of the water spider Argyroneta aquatica. (A) The spider in a cultural vial with the web it produced (arrows) and a young Daphnia captured by the web (arrowhead). Macrophotography. Scale bar, approximately 10 mm. (B) A cultural vial with the web (arrow). The spider was transferred to another container. Macrophotography.
Due to the restricted volume of the container and the absence of any straws and wooden sticks inside, the spider did not construct a diving bell and an egg cocoon. Nevertheless, it periodically produced a web in the form of a network that occupied the whole width of the vial. Before feeding, i.e., during July–August, the spider has built an enormous strongly ramified network several times. Afterward, the spider produced much smaller and delicate webs (Figures 1A, B), in one case after consuming a big Daphnia, which was captured by the spider itself without the help of a web as in other cases with big preys. Conversely, in the cases of small Daphnia, the spider caught them with the help of the web (Figure 1A). Initially, the spider attached the web to the walls of the vial by movements of the end of the opisthosoma. This network served to capture smaller preys, e.g., small Daphnia that may hang on the web for some time. Nevertheless, the web did not stick to instruments (tweezers, needles, etc.), and it was very difficult to extract the web from the water. Being artificially disconnected from the wall of the vial, the web immediately became cloggy and did not form a tail within the water. Conversely, on air, the web, probably due to the tension of the water film, adhered to needles, and it was very difficult to spread it over a microscope slide. After drying on air, the web became fragile.
The living spider and silk in the container were photographed and video-registered with a Canon G11 digital camera alone or combined with MBS-9 dissecting microscope (LOMO-Microsystems).
2.1.2 Water mites Limnochares aquatica (L., 1758) and Limnesia maculata (O.F. Müller, 1776)
Initially, adults of these mite species were collected in nature and kept in the laboratory for years [see, for details, Shatrov et al. (2016); Shatrov et al. (2023)]. The mites produced silk in water, and this silk is comparable with that of the water spider. Here we present data on the silk structure of these species for comparison purposes only.
2.1.3 Terrestrial two-spotted spider mite Tetranychus urticae (C.L. Koch, 1836)
Several tens of adult mites were taken from the mass culture maintained in All-Russian Institute of Plant Protection (St-Petersburg, Pushkin, Russia). The mites were reared on bean plant leaves in a Petri dish and produced silk in mass. The silk threads are very sticky, adhere to instruments during manipulations, and tend to stick together. Usually, the silk of these mite species is contaminated with excrement pellets and molt cuticles.
2.2 Light microscopy
In the case of spider and water mites, the silk was carefully extracted from the containers with a dissecting needle or a fine pipette. Then, the silk was mounted and spread on microscope slides in a drop of water and covered with a cover slip tightly applied to the slide and sealed with nail polish. The silk samples were then examined and photographed (i) with a Leica DM LS-2 light microscope (Leica Microsystems GmbH Wetzlar, Germany) combined with a MC-6.3 digital camera (LLC LOMO-Microsystems), (ii) under a differential–interferential contrast with a NEXCOPE NE910 light microscope (Nanjing Jiangnan Novel Optics Co., Ltd., China) coupled with a MC-8.3 digital camera (LLC LOMO-Microsystems, St.-Petersburg, Russia, https://lomo-microsystems.ru), and (iii) using both a phase-contrast method and a plane-polarized emission by the corresponding devices coupled with a MIKMED-6 light microscope (LLC LOMO-Microsystems) combined with an MS-6.3 digital camera (LLC LOMO-Microsystems),.
2.3 Atomic force microscopy
For the atomic force microscope examination, a bundle of the spider’s threads removed from the vial was spread over a cover slip, dried on air, and placed into atomic force microscopy (AFM). Images were taken using an NTEGRA Aura atomic force microscope (NT-MDT, Zelenograd, Moscow, Russia) in semi-contact mode with NSG10 cantilever, tip curvature radius of 10 nm, and force constant of 3.1–37.6 N/m.
2.4 Scanning electron microscopy
For the SEM examination, thread bundles of spider and water mites were extracted from the containers, spread over a cover slip, and dried on air. These cover slips were then placed on a microscope stub, covered with a platinum layer in an Eiko IB-5 ion coater, and examined with SEM Quanta-250 (FEI Company) at 15–20 kV. In the case of T. urticae, silk threads were strained on air between two glass capillaries, dried on air, covered with a platinum layer, and examined with the same scanning electron microscopy (SEM).
2.5 Transmission electron microscopy (TEM)
For TEM observations, silk samples of both the water and terrestrial arachnids were fixed in 2.5% glutaraldehyde in 0.1 M cacodylate buffer (pH 7.2–7.4) for several days. The samples were then washed in 0.2 M cacodylate buffer for 1 h, postfixed in 2% osmium tetroxide in 0.1 M cacodylate buffer for 5 h, dehydrated in graded ethanol and acetone series, and finally embedded in an araldite mixture. After fixation, the silk became not black but slightly brown. Before hardening of the epoxy resin, the silk bundles were spread within blocks for better orientation of the threads. Ultra-thin sections from these blocks were made on a Leica UC-7 ultramicrotome (Leica Microsystems GmbH Wetzlar, Germany) using a diamond knife (Ultra 45°, Diatome Ltd., Switzerland). The sections were then mounted on copper grids with an oval hole provided with a formvar support and, after staining with uranyl-acetate and lead citrate (Reynolds, 1963), were examined and photographed with a TEM Morgagni 268-D (FEI Company) at 80 kV (digital visualization). Control semi-thin sections were made using the same ultramicrotome with glass knives and examined under a Leica DM LS-2 light microscope.
All light optical, SEM, and TEM preparations obtained in the present study are deposited at the Zoological Institute of the Russian Academy of Sciences (St. Petersburg, Russia).
3 Results
3.1 Water spider Argyroneta aquatica
3.1.1 Light microscope observations of the silk threads
The spider produced its web as, apparently, a capturing network (cobweb) irrespective of the construction of a diving bell (Bakker et al., 2006).
Threads in the silk bundles on the microscope slides are chaotically interlaced within the tight clot (Figure 2A) artificially spread upon the substrate during preparation for better exposition of different threads. Initially, the threads in such associations may be roughly ranged into three types by their thickness (diameter)—a-, b-, and c-type (Table 1; Figures 2B–D). Additionally, threads of much smaller diameter, around 50 nm, d-type, may be also recognized in light preparations (Figures 2C, D). Threads of another, fifth, e-type, of a much larger diameter at around 3 to 4 µm (see below), if present, may be rarely identified with certainty (see Figure 2D). Threads of all types are very long, non-branched, uniform in width through their course, and rarely found to be ruptured or broken (Figures 2B, D). The proportion of thread types in the threads’ associations is barely to be estimated precisely, but threads of a-, b-, and e-type with a larger diameter appear to be present in a smaller number, whereas threads of c- and d-types with the smallest diameter seem to be more numerous.
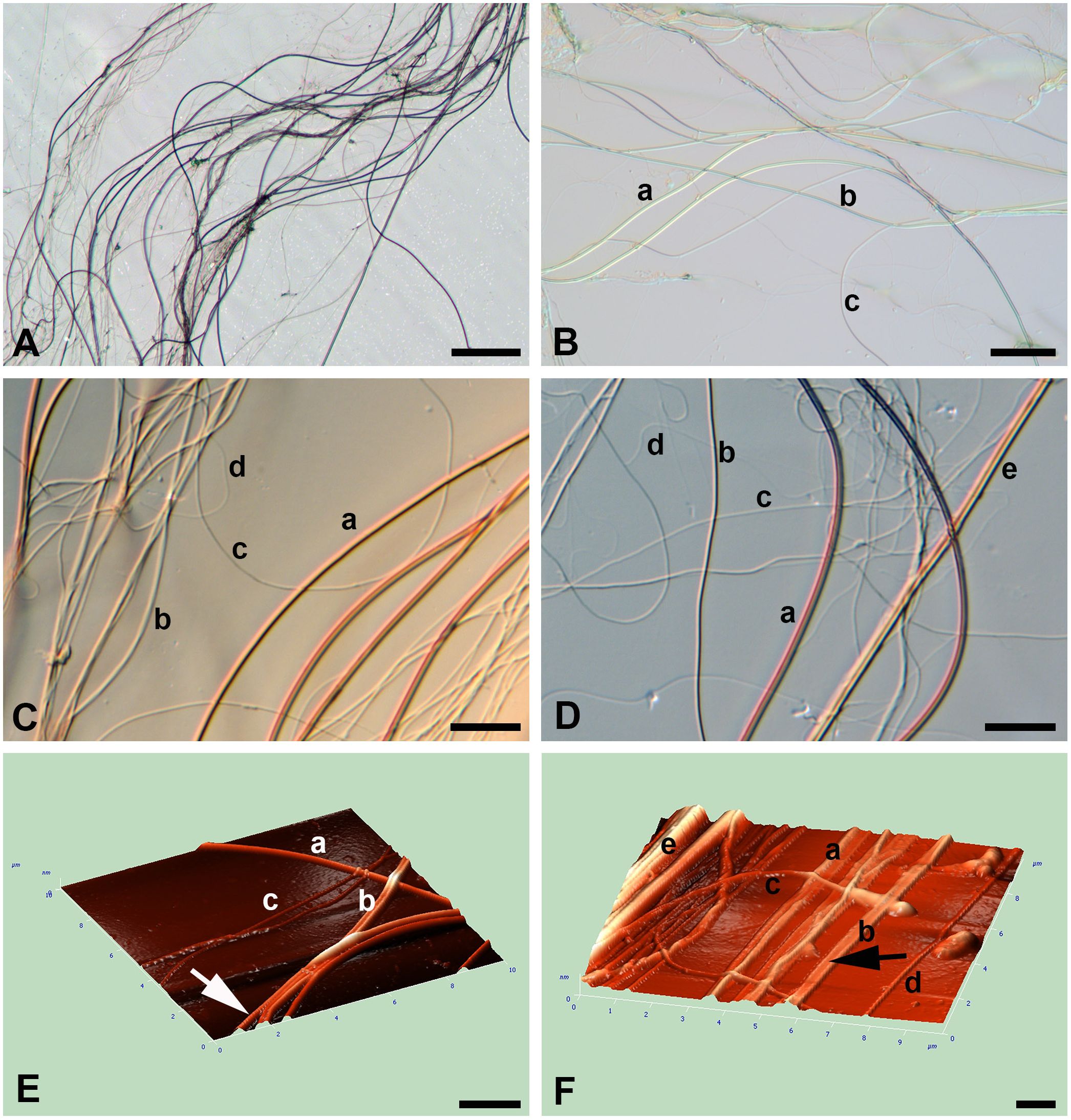
Figure 2 (A–F) Light (A–D) microscopy and AFM (E, F) of silk of the water spider Argyroneta aquatica. (A) A thread’s bundle composed of different threads. The thinner threads are badly visible. Scale bar, 50 µm. (B) An association of different thread types. Scale bar, 20 µm. (C) Part of the thread association showing different thread types and their characteristic arrangement. Differential–interferential contrast. Scale bar, 10 µm. (D) Thread association composed of different thread types. Differential–interferential contrast. Scale bar, 10 µm. (E) An angular projection showing different thread types. Note a slightly wrinkled surface in b-thread (arrow). Scale bar, 2 µm. (F) An angular projection showing the bundle of interlacing threads. Note the presumptive b-thread flattened on the substrate (arrow). Scale bar, 1 µm. a, b, c, d, e—types of threads.
Threads of a-, b-, and, supposedly, e-types are mostly straight or regularly curved to form folds and loops, whereas threads of c- and d-types are usually curved and rather sinuous and rarely look straight (Figures 2C, D). The latter threads, although they are more numerous, are hardly distinguishable in preparations due to their smallest diameter and weakest manifestation on the slides. Importantly, the threads of all types quite rarely show local depressions of their thickness and are always uniform in their width.
3.1.2 AFM observations of the silk threads
In AFM, the volume characteristic of threads and their dimensions are most pronounced (Figures 2E, F). Threads of different types are freely interlaced and tend to stick together into bundles. Threads of all types are devoid of local depressions throughout their course. Threads of a- and, supposedly, e-type are mostly round and straight, whereas threads of b-type may be flattened on the glass substrate (Figures 2E, F). AFM do not show any recognizable relief of the threads’ surface despite, sometimes, fine transverse striations or small wrinkles (Figure 2E).
3.1.3 SEM observations of the silk threads
The SEM examination (Figures 3A–F) reveals the same appearance of silk threads as observed in light microscopy and AFM but with a larger magnification and better resolution that give the opportunity to measure the threads’ sizes more accurately (Table 1).
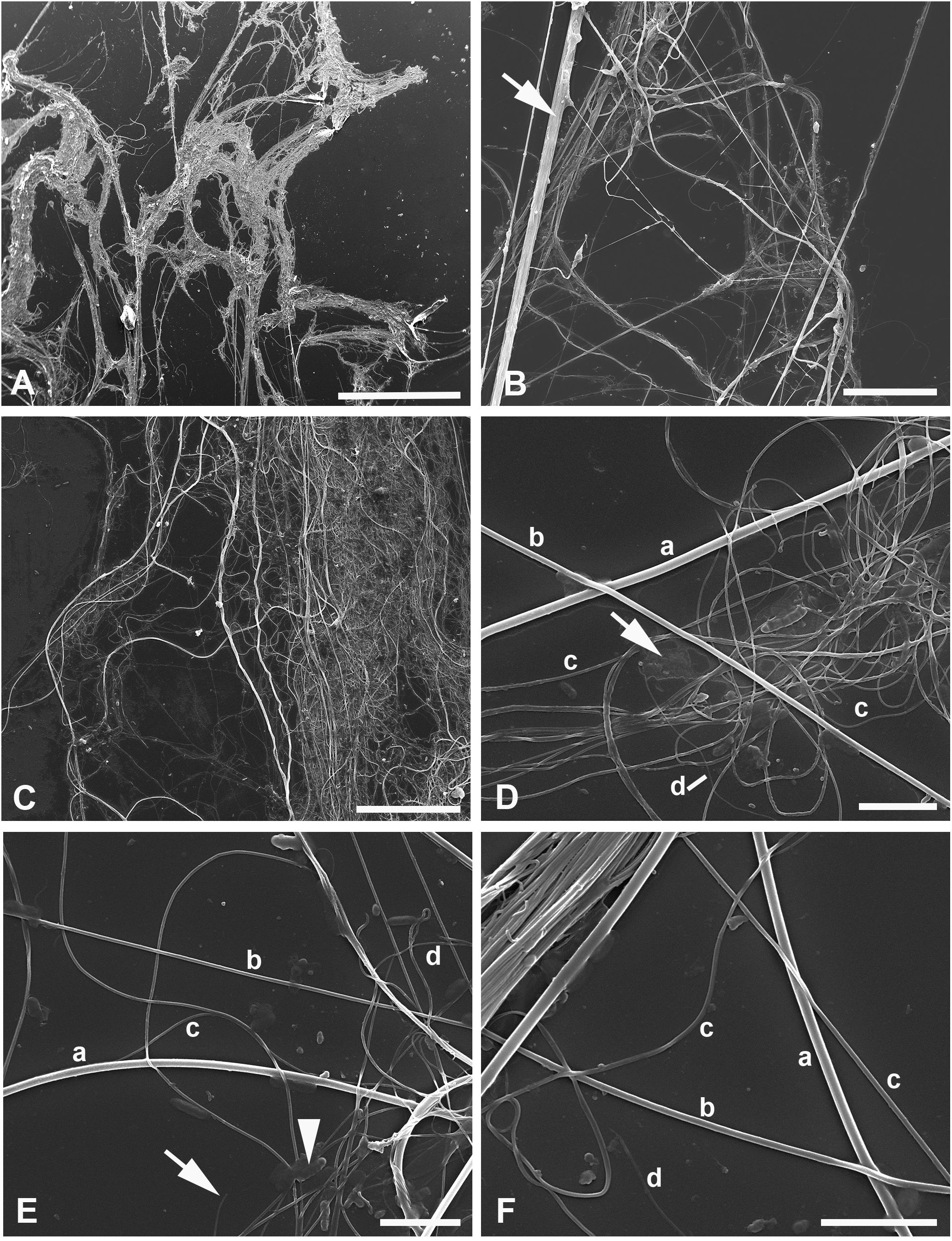
Figure 3 (A–F) SEM of silk of the water spider Argyroneta aquatica after ethanol fixation (A, B) and simple drying after water (C–F). (A) Silk association with tightly interlaced threads. Note the virtual absence of an additional homogeneous substance. Scale bar, 1 mm. (B) Different threads stretched upon the substrate. Note a thick band (arrow) composed of different threads tightly stuck together. Scale bar, 50 µm. (C) An association of threads with an additional homogeneous substance that can be seen on the right-hand side. Scale bar, 50 µm. (D) Part of the thread clot with highly interlaced c- and d-type threads. Note a film of unknown organic substance (arrow). Scale bar, 5 µm. (E) A part of thread association with the clearly exposed different thread types. Note the d-type thread torn off (arrow) and a bacteria-like microorganism (arrowhead). Scale bar, 5 µm. (F) Threads of different types near a band of the tightly stuck threads. Scale bar, 5 µm. a, b, c, d—types of threads.
As shown in the table, the diameter of threads of different types varies significantly and may overlap each other. The threads with the smaller diameter than that of c-type, i.e., substantially less than 100 nm (around 50 nm), may belong to the presumptive fourth type, d-type, which may be rarely distinguished in SEM preparations (Figures 3D–F). Conversely, threads with a much larger diameter, the presumptive e-type, were not clearly identified in SEM.
In SEM preparations, it is more obvious that silk threads of different types tend to stick together so that it may be difficult to distinguish separate threads in the compound threads’ bundles (Figures 3A–C). The latter may contain different thread types, e.g., thin threads of c-type may gather round threads of b- or a-type, and in these cases, the former threads may be straight going tightly along the thicker and initially straight threads (Figures 3B, F). Quite rarely, some threads, supposedly of a-type, may show some local depressions through their course (see below). Generally, however, threads of all types show a regular diameter. In contrast with AFM observations, no striations were identified on the threads’ surface.
The film of a certain organic substance [see Bakker et al. (2006)] frequently accompanies the thread associations and is better presented in the samples prepared from water (Figures 3C, D). Most likely, this substance represents the adhesive piriform cement, or glue, secreted together with the piriform silk (Schaber et al., 2023) (see below). Conversely, in the ethanol-preserved material, this additional secretion is less pronounced (Figure 3A). Single bacteria-like microorganisms may be seen scattered in a small number throughout the glass substrate (Figure 3E). Their presence within the threads (see Shatrov et al., 2023) can scarcely be expected owing to their sizes being larger than the diameter of most threads.
3.1.4 TEM observations of the silk threads
TEM (Figures 4, 5) gives the most important information on the threads’ organization. Nevertheless, the greatest difficulty is to associate threads seen in SEM and TEM into one certain type. In sections of the threads’ bundles, threads of different types are mixed freely (Figures 4A, 5A) but may be rarely found in tight associations and are scattered separately, especially threads of the presumptive a-type.
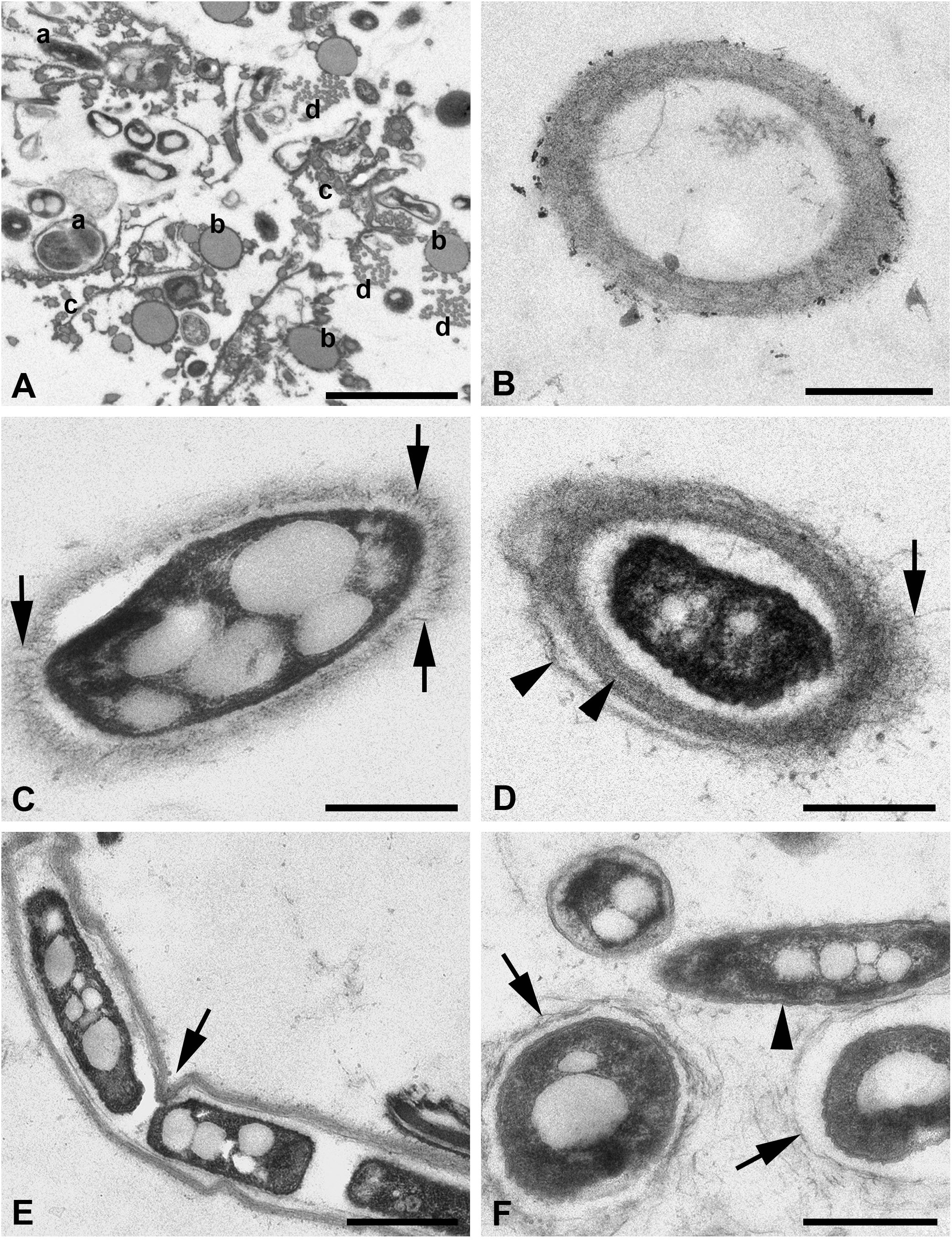
Figure 4 (A–F) TEM of silk threads of the water spider Argyroneta aquatica. (A) Thread bundle with different thread types in transverse section. Note the variants of the a-type threads (a). Scale bar, 2 µm. (B) Empty silk thread of the a-type with a fine fibrillary wall. Scale bar, 0.5 µm. (C) Thread of the a-type in obliquely transverse section with an electron-dense vacuolated core. Note the fibers rising vertically from the thread wall (arrows). Scale bar, 0.5 µm. (D) Thread of the a-type with a core and the layered fine fibrillary wall. Note the fibers rising from the external layer of the wall (arrow) and two layers of the thread wall (arrowheads). Scale bar, 0.5 µm. (E) Thread of the a-type with a dense vacuolated core and a local depression (arrow) of the thread wall. Scale bar, 1 µm. (F) Variations of the a-type threads with the thin walls built of the coarse fibers (arrows) and the thread without wall surrounded by a double membrane-like envelope (arrowhead). Scale bar, 0.5 µm. a, b, c, d—types of threads.
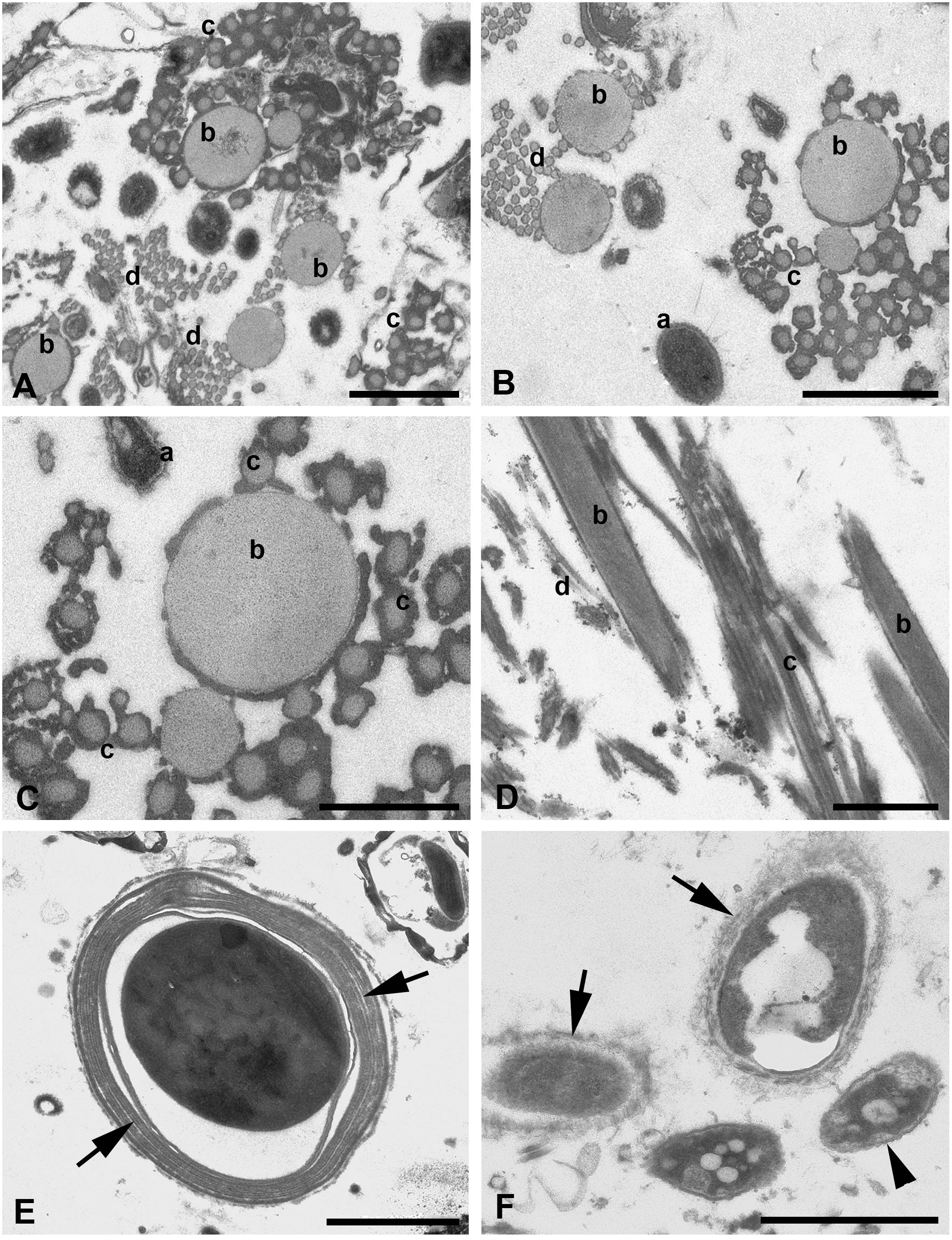
Figure 5 (A–F) TEM of silk threads of the water spider Argyroneta aquatica. (A) Thread bundle mostly composed of b-, c-, and d-type threads. Scale bar, 1 µm. (B) Threads of b-, c-, and d-types in tight associations in transverse section. Note a variation of the a-type thread manifestation (a). Scale bar, 1 µm. (C) Threads of b- and c-types in a higher magnification. Scale bar, 0.5 µm. (D) Threads of b-, c-, and d-types in oblique longitudinal section. Scale bar, 1 µm. (E) Thread of the presumptive e-type in transverse section. Note a compound thread wall composed of several layers (arrows). Scale bar, 2 µm. (F) Variations of the a-type threads with the dense core substance surrounded by variously expressed fine fibrillary walls (arrows) and by a double membrane-like wrinkled envelope (arrowhead). Scale bar, 1 µm. a, b, c, d—types of threads.
The latter are represented in small number and show the largest diameter, larger than that found in SEM, which varies from 400 to 1,000 nm (Figures 4B–F). This difference may be explained by the contraction of the threads’ diameter in SEM after drying of the threads’ samples or, on the contrary, by the enlargement of the threads’ diameter in water solutions during fixation for TEM. These threads have a fibrillary electron-light wall (Figures 4B, C) with up to three nearly identical layers separated by narrow clear spaces (Figure 4D). The external surface of the wall may show fine fibers much loosely arranged and frequently rising vertically from the wall to the surrounding space (Figure 4C). Alternatively, the wall may show coarser and loosely packed fibers (Figures 4F, 5F). The wall may also become very thin (Figure 4F), but generally, its width is restricted within 80–300 nm. The walls may be slightly wavy or may show small depressions, most likely depending on the character of the internal core (Figure 4E). The internal space of these threads may be electron-lucent without any additional substances (Figure 4B) or, on the contrary, may contain an electron-dense, frequently vacuolated and granulated material (core) (Figures 4C–E). No fibers are recognized within this core. The latter may be in contact with the thread’s wall but more often is separated from the wall by a certain clear space. The core substance usually shows a single or even double frequently wrinkled membrane-like envelope (Figure 4F). Generally, this thread type demonstrates a great variation of the above-mentioned characters.
The threads, which may be closely related to the b-type threads in SEM, are completely different from threads of the a-type. In TEM, these threads are also thicker than in SEM with a diameter that varied within 200–500 nm. These threads show a quite simple organization (Figures 4A, 5A–D) and are represented by regularly round solid tubes with a uniform matrix of low electron density containing tightly packed, fine, longitudinal fibrils that are hardly recognizable (Figures 5C, D). They are covered with a fine membrane-like envelope and frequently accumulate small dense particles of unknown origin on the surface (Figure 5C). These threads tend to cluster in groups of two to three threads (Figures 5B, D).
Threads supposedly of the c-type tend to group into large associations frequently near threads of the b-type and have a uniform diameter of around 100 nm (Figures 5A–D), which is closely related to their diameter found in SEM. These threads reveal the same organization as threads of the b-type and accumulate dense substances on their surface (Figure 5C). Nevertheless, transitional forms between threads of b- and c-types by their diameter may be found rather rarely.
Threads of the presumptive fourth type, d-type, may be more obviously recognized in TEM (Figures 5A, B, D). These threads are grouped into large associations and are uniform in their diameter of around 50 nm. They show the same characteristic as threads of the c-type but may be rarely found.
Quite rarely, much larger single threads of the presumptive e-type may be recognized within the threads’ bundles. These threads show the envelope consisting of two to four concentric membranous layers and a variously expressed but mostly uniformly dense core placed in some distance from the envelope (Figure 5E). The diameter of these threads may reach 3.5–4.5 µm. The external surface of these threads does not bear loosely packed vertical fibers as the threads of the a-type.
In bundles composed of different thread types fixed for TEM, some other non-qualified elements and additional substances may be present, which do not directly correspond with any of the above-mentioned thread types (see Figures 4A, 5A).
For example, one of these elements is a particular “thread type” highly resembling the “wrinkled” internal core of the a-type threads but totally devoid of the external wall (Figures 4F, 5F). The diameter of these threads varies within 200–500 nm (Figures 4F, 5F). Most likely, these threads are a particular form of the a-type threads, which have completely lost their fibrillary walls for unknown reasons. Moreover, some transitional forms may be apparently observed (Figure 3F).
Other substances, which may be found in the thread bundles, are rather variously expressed by their organization, represented in small number, and cannot be characterized as silk threads but only as some additional material of the spider secretory activity.
3.2 Water mites Limnochares aquatica and Limnesia maculata
Silk threads of these mites were examined previously (Shatrov et al., 2016; Shatrov et al., 2023), and here we only reproduce some important data for comparison and figures that were not published earlier.
The silk threads of these mites are represented by only one type. These threads are identically organized in these two species and highly resemble the a-type threads of A. aquatica (Figures 6A, B). They are composed of the fibrillary wall and dense internal substance with great variation of their expressions (Figure 6B). However, the silk threads of water mites are larger in diameter (1–3 µm) than those of A. aquatica, and the empty threads usually collapse against the substrate in SEM (Figure 6A). In TEM, all threads are uniformly round in transverse sections (Figure 6B). The surface of the threads’ wall show fibers rising vertically, as in A. aquatica, but in contrast with the latter species, it may also reveal fine longitudinal striation in SEM and AFM. Despite the relatively high variation in the diameter of threads, there is no reason to suspect the presence of several types of silk threads in water mites (Shatrov et al., 2016; Shatrov et al., 2023).
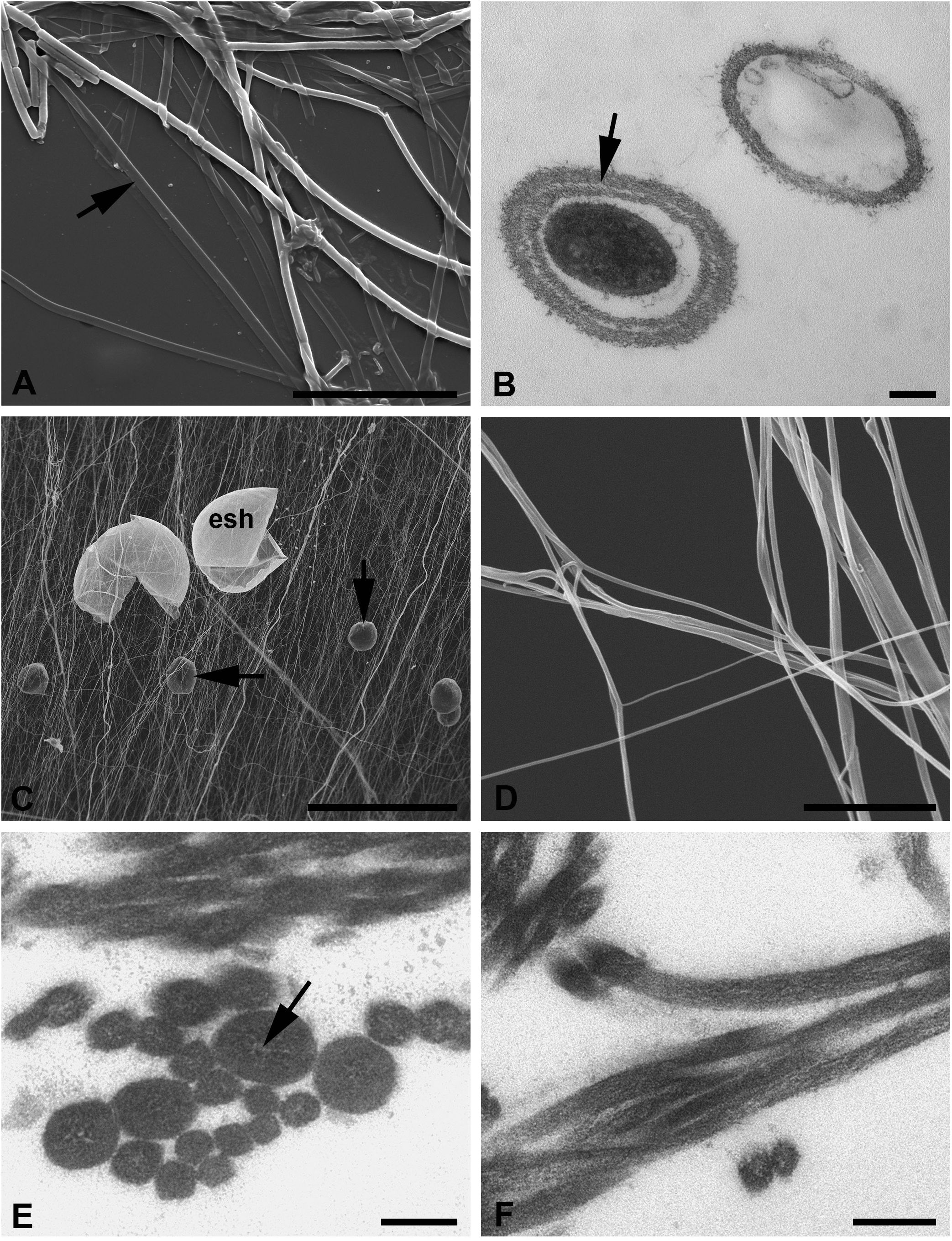
Figure 6 (A–F) Silk of the water mite Limnesia maculata (A, B) and terrestrial mite Tetranychus urticae (C–F). (A) SEM of the interlaced silk threads spread on the substrate (cover slip). Note the flattened threads apparently without a core substance (arrow). Scale bar, 30 µm. (B) TEM of threads with and without core substance and the layered wall in one of them (arrow). Scale bar, 0.2 µm. (C) SEM of the large area of silk with empty eggshells and fecal pellets (arrows). Scale bar, 200 µm. (D) SEM of both single and stuck threads in higher magnification. Scale bar, 3 µm. (E) TEM of the transverse section of threads showing their uniform contents but various thickness. Note the hardly identified clear lacunas in the central region of the thickest threads. Scale bar, 0.1 µm. (F) TEM of the obliquely longitudinal sections of wavy threads. Scale bar, 0.2 µm. esh, eggshell.
3.3 Two-spotted spider mite Tetranychus urticae
Fine uniform threads freely interlaced on air and frequently sticking together into bundles represent the silk of this mite species (Figures 6C, D). In the mite colony, such web occupies a huge area on the leaves and among them, where threads are stretched in different directions and highly contaminated with eggshells and fecal pellets (Figure 6C).
Threads of the web of T. urticae highly correspond to the d-type threads of A. aquatica. They are uniformly round and very thin, around 50–100 nm in diameter, and it is only rarely that single threads may reach 120–150 nm in width (Figures 6E, F). Nevertheless, all threads are identically organized and may be considered as a single thread type. The threads contain fine tightly packed longitudinal or obliquely arranged fibers enclosed within a matrix of moderate to high electron density. A fine nearly imperceptible membrane (Figures 6E, F) envelops the threads. The surrounding membrane is devoid of any external additional fibrillary layers. Sometimes, in the thicker threads, the internal material may leave a rather narrow clear space in the middle region (Figure 6E).
4 Discussion
The water spider A. aquatica has a Palearctic distribution and the only species from the order Araneae living underwater (Masumoto et al., 1998; Bakker et al., 2006). The spiders maintain an air layer around the abdomen and thorax by hydrophobic setae (Schaber et al., 2023). This air volume is used for the construction of the diving bell and spinning of dragline and piriform silk with the help of the attachment discs (Bakker et al., 2006; Schaber et al., 2023). The spiders construct the egg cocoon (egg sac) inside the diving bell from silk, which was investigated previously by SEM methods (Bakker et al., 2006). The authors divided this silk into four categories, and the diving bell, in turn, was found to consist of three different types of threads. Threads of these types correspond well in size to threads found in our study, in particular, to types a, b, c, and d used apparently as a capturing net. Probably, threads of the same origin in the spiders may be used for different purposes—building of the egg cocoon and formation of the capturing net. In the recent work, functioning of the attachment discs in A. aquatica was considered in tight association with the secretion of both dragline and piriform silks and the piriform adhesive cement (Schaber et al., 2023). In light of these data, it is clearly seen that the a- and, probably, e-types in our study closely correspond to dragline silk; the b-, c-, and d-types are directly associated with different types of piriform silk threads, whereas the amorphous substance is nothing more than the piriform adhesive cement (Schaber et al., 2023). Nearly the same mechanism of attachment of silk threads to various substrates, with the help of the glue-coated piriform nano-silk fibers due to the action of disk-like structures, was also shown for several species of terrestrial spiders (Wirth et al., 2019).
Despite the fact that, in nature, A. aquatica “spends its entire life cycle underwater” (Schaber et al., 2023), it rests for some time within the diving bell (Masumoto et al., 1998). Our observations show that the spider spends some time within the restricted air capacity above the water, the probable analogue of the diving bell. This resting outside the water most likely is just because of the fact that the container was maintained without any additional structures where the spider could fix its diving bell to. Importantly, that the spider produced web only periodically as well as constructed diving bells (Masumoto et al., 1998) as an alternate activity with periods of long rest.
Although it seems nothing is known about the formation and structure of a capturing net in this spider, it is apparent, however, that the spider constructs the web from the same threads used to build the egg cocoon. Nevertheless, the assortment of threads used for the formation of the egg sac (Bakker et al., 2006) appears to be wider than that used to produce the capturing net. In this regard, it is nearly possible to assume that (i) the spider uses different kinds of threads for every case and (ii) the formation of a kind of a capturing net in our observations is only the imitation of producing of the type of the egg cocoon in the absence of any favoring materials like wooden sticks, etc. Although we tried to exclude any hampering factors, like plants, substrate particles, etc., from our work with silk, in samples of silk associations examined in TEM, many additional particles of unknown origin were observed. However, the amorphous substance sometimes indicated in SEM most likely is a piriform adhesive glue secreted together with the piriform silk.
Morphologically, silk threads of the water spider A. aquatica are infinitely long, uniformly wide, and unbranched. However, threads of the a-type show a wide spectrum of their diameter and internal organization. In contrast with the “walking threads” (Bakker et al., 2006), which “are branched off into several fibers as soon as the substrate is reached” (p. 140), we did not observe branching of any threads even if they were detached from the walls of the container. In contrast to a- and e-type threads revealing a variously expressed internal structure, threads of the other types show a quite simple organization, probably possessing fine longitudinal fibers inside. Importantly, the a-type threads or their portions, which are free from the internal core substance, were not seen flattened on the substrate in SEM in contrast with the similar threads of the water mites with a significantly larger diameter (Shatrov et al., 2014; Shatrov et al., 2016; Shatrov et al., 2023).
The comparison with the studied silk threads of terrestrial spiders (e.g. Stubbs et al., 1992; Li et al., 1994; Vollrath et al., 1996; Gosline et al., 1999; Gould et al., 1999; Sponner et al., 2007; Hajer et al., 2009) does not reveal an exact morphological similarity between the studied silk threads of water and terrestrial spiders. The core substance in terrestrial spiders may be built of “pleated fibril-like structures, which are arranged in two concentric cylinders” in a coaxial manner as in Nephila clavipes (L.) (Li et al., 1994; Vollrath et al., 1996) or of a bundle of parallel nanofibrils as in Theridiosoma gemmosum (C.L. Koch) (Hajer et al., 2009; see also Blamires et al., 2017). In the latter case, the egg cocoon is constructed from several thread types of quite different diameter values from 600–800 nm up to 4–6 µm. Alternatively, in the cobweb scaffolding threads of Latrodectus hesperus (Chamberlin et Ivie) (black widow), thicker fibers (300 nm) oriented parallel to the thread axis and thinner ones (10–100 nm) oriented across the thread axis were identified in the highly ordered regions of the silk threads (Gould et al., 1999). In Argiope aurantia Lucas, a core substance of threads of the egg case consists of numerous electron-light fibrils immersed into the electron-dense matrix (Stubbs et al., 1992).
The envelope morphology of the spider silk threads disclosed previously in a number of works (e.g. Stubbs et al., 1992; Sponner et al., 2007) attracted lesser attention than that of the core substance and is completely different by its organization to that revealed in A. aquatica—for example, the wall of the thread fibers may be nearly imperceptible, like in Argiope (Stubbs et al., 1992), or may consist of a number of thin layers of differently expressed spidroins, as occurs in Nephila clavipes dragline silk (Sponner et al., 2007). The adaptations to different ecological conditions and feeding diets probably greatly influence on the spider silk and its morphology and properties.
The recent studies of the spider silk ultrastructure based on different methodological approaches revealed a quite complex nanostructure of the spider silk fibers (e.g. Schniepp et al., 2023; Blamires et al., 2017; Riekel et al., 2019; Wirth et al., 2019; Riekel et al., 2020; Wang et al., 2022; Blamires et al., 2023; Perera et al., 2023). In particular, it was shown that the spiders’ dragline fiber consists of a protein core of tightly packed nanofibrils within a protein matrix (Blamires et al., 2023). The proposed dragline silk threads in the present study show a quite different organization in comparison with the above-mentioned ones. Undoubtedly, nanofibers of the external fibrillary wall may provide both adhesive properties and extension of fibers, whereas the internal core may contain greatly coiled protein fibers, which may stretch and thus reveal the high tensile strength of these threads (Foelix, 1996; Blamires et al., 2017; Blamires et al., 2022). At the same time, it is hardly possible to suspect that exfoliation of these threads could give multiple fine fibers as it is shown in some spiders (Perera et al., 2023) or even a more complicated internal structure (Riekel et al., 2019, 2020). In any case, it may be disclosed by methods that are more sophisticated like AFM tomography, X-ray diffraction, gas chromatography, and others (e.g. Schniepp et al., 2023; Riekel et al., 2019, 2020)—for instance, the b-, c-, and d-type threads of A. aquatica are supposedly composed of longitudinally oriented nanofibers like in Trichonephila clavipes (L.) (Perera et al., 2023), although in the latter species these threads could be of a much greater diameter (up to 4 µm). Importantly, the surface of the ribbon-like silk threads in Loxosceles spider reveals fine papillae supposedly contributing to their adhesive properties (Schniepp et al., 2023) that was disclosed by AFM tomography and SEM. Conversely, in A. aquatica, the surface of different silk threads may show only fine striation that may be provoked by some contraction during the sample preparation.
Silk of the water-inhabiting caddisflies (Trichoptera) investigated thoroughly by different approaches (Engster, 1976; Yonemura et al., 2006; Ashton et al., 2011; Hatano and Nagashima, 2015) differs substantially from that of the water spider A. aquatica despite inhabiting similar aquatic environments. First, “trichopteran larval silk is extruded from a pair of silk glands that fold back and forth several times along the ventral side of the alimentary canal and terminate at the spinneret on the ventral side of the mouthparts and alimentary orifice” (Ashton et al., 2011). To form the final thread, the initial silk fibers are fused into a paired elliptical fiber embedded in a layer of glue proteins with adhesive properties (Yonemura et al., 2006). Although these silk fibers are principally proteinaceous, the peripheral layer also shows the presence of both neutral and acidic polysaccharides (Engster, 1976). These composite silk threads of caddisflies are used for two purposes—constructing both retreats and cocoons for larvae and capturing nets to filter food particles (Hatano and Nagashima, 2015). The composite silk of the water spider differs by morphological criteria from that of Trichoptera but quite similar to the latter by their functions.
Another large water-inhabiting arachnid group, water mites, reveals a partial similarity in the silk threads’ organization. The a-type threads of A. aquatica and silk threads of water mites (Shatrov et al., 2014, 2016; Shatrov et al., 2023) are nearly identical by their morphology but are slightly different in size. Importantly, the fibrillary walls in both cases may point to the high adhesive properties of the threads that help to form, owing to their sticking together, a rather strong capturing net. The core substance, characteristic of this type of silk, until fibers are found in it, may play a stabilizing and solidifying role in the thread composition. The latter may indicate a high tensile strength of this kind of threads, which is highly characteristic for the spider silk (Foelix, 1996; Blamires et al., 2017).
Threads of other types, except for the e-type with the simplest organization, in the light of available data, are hard to be directly compared with any known morphology of silk threads in spiders. Nevertheless, due to the possible presence of fine fibers inside, these threads are also capable of stretching. Therefore, owing to their abundance, they possibly play an important role in net construction. The fifth, the largest, and the fewest e-type thread of A. aquatica silk is rarely observed and probably play a general constructive role in the thread association closely resembling thick dragline threads. These threads are comparable with the Nephila dragline silk, where the micro-fibril walls are crossing under certain angles and may move along themselves as well (Vollrath et al., 1996). This type of threads, in contrast to others, is obviously capable of stretching.
Silk of the terrestrial tetranychid, the so-called spider mite T. urticae, demonstrates great simplicity in the organization of its threads (e.g. Gerson, 1985; Shin et al., 2006). This type of silk is highly comparable with the b- and c-types threads of A. aquatica by the dimensional characteristics and the possible fine-fibrillary contents. Despite such simplest morphology, these mites produce this silk in great abundance and use it in quite different ways (Clotuche et al., 2011). Apparently, contrary to the assumption that some insects “produce only one type of silk, and, in general, they use it for one purpose during a limited period in their lives” (Craig et al., 2000), spider mites use this kind of silk, uniform and simply organized, as wide as possible for the realization of different living-related purposes.
5 Conclusion
The water spider A. aquatica produces a compound silk consisting of several types of threads, which are comparable on one side with those of water mites and on the other side with those of aerial tetranychid mites. This finding shows that, in different evolutionary lineages of arachnids, a small number of possible variations limit the spectrum of available ways to produce silk proteins packed in threads of various morphology. On the other hand, the same water environment, in the cases of water mites and spiders, may provoke a similarity of the silk threads in both formation and morphology. At the same time, water spider produces silk resembling that of aerial mite that point to a wide range of its adaptations to different environmental conditions. However, the sources of these silks are different—if in water spider and water mites the glands producing silk are abdominal (Shatrov et al., 2019; Shatrov et al., 2023), in tetranychid mites these glands are prosomal (e.g., Gerson, 1985), i.e., of a quite different origin.
Data availability statement
The original contributions presented in the study are included in the article/supplementary material. Further inquiries can be directed to the corresponding author.
Ethics statement
The manuscript presents research on animals that do not require ethical approval for their study.
Author contributions
AS: Conceptualization, Data curation, Investigation, Methodology, Project administration, Software, Supervision, Writing – original draft, Writing – review & editing. ES: Data curation, Investigation, Methodology, Software, Visualization, Writing – original draft.
Funding
The author(s) declare that no financial support was received for the research, authorship, and/or publication of this article.
Acknowledgments
This study is undertaken and performed under the State Government Research Programs #122031100263-1 and #122031100275-4. The TEM and SEM procedures were realized using the Core Facilities Centre ‘Taxon’ at the Zoological Institute of the Russian Academy of Sciences, St. Petersburg, Russia, https://www.ckp-rf.ru/ckp/3038/. The AFM methods were performed in the Centre for Microscopy and Microanalysis (St. Petersburg State University, St. Petersburg, Russia). We thank KA Benken, an engineer of this center for the assistance with the AFM.
Conflict of interest
The authors declare that the research was conducted in the absence of any commercial or financial relationships that could be construed as a potential conflict of interest.
Publisher’s note
All claims expressed in this article are solely those of the authors and do not necessarily represent those of their affiliated organizations, or those of the publisher, the editors and the reviewers. Any product that may be evaluated in this article, or claim that may be made by its manufacturer, is not guaranteed or endorsed by the publisher.
References
Alberti G., Coons L. B. (1999). “Acari: mites,” in Microscopic Anatomy of Invertebrates, vol. 8C . Eds. Harrison F. W., Foelix R. F. (Wiley-Liss, New York), 515–1217.
Alberti G., Ehrnsberger R. (1977). Rasterelektronenmikroskopische Untersuchungen zum spinnvermögen der Bdelliden und Cunaxiden (Acari, Prostigmata). Acarologia 19, 55–61.
Annamalai M., Jayaprakash K. (2012). Structural studies on silk protein fibre from pseudoscorpion. Int. J. Life Sci. Pharma Res. 2, 49–54.
Arakawa K., Kono N., Malay A. D., Tateishi A., Ifuku N., Masunaga H. (2022). 1000 spider silkomes: Linking sequences to silk physical properties. Sci. Adv. 8, eabo6043. doi: 10.1126/sciadv.abo6043
Ashton N. N., Taggart D. S., Stewart R. J. (2011). Silk tape nanostructure and silk gland anatomy of Trichoptera. Biopolymers 97, 432–445. doi: 10.1002/bip.21720
Bakker d., Beatens K., Nimmen van E., Gellynck K., Mertens J., Langenhove van L., et al. (2006). Description of the structure of different silk threads produced by the water spider Argyroneta aquatica (Clerck 1757) (Araneae: Cybaeidae). Belg. J. Zool. 136, 137–143.
Blackledge T. A. (2013). “Spider silk: molecular structure and function in webs,” in Spider Ecophysiology. Ed. Nentwig W. (Springer-Verlag, Berlin, Heidelberg), 267–280.
Blackledge T. A., Scharffb N., Coddingtonc. J. A., Szütsb T., Wenzeld J. W., Hayashie C. Y., et al. (2009). Reconstructing web evolution and spider diversification in the molecular era. PNAS 106, 5229–5234. doi: 10.1073/pnas.0901377106
Blamires S. J., Blackledge T. A., Tso I.-M. (2017). Physicochemical property variation in spider silk: ecology, evolution and synthetic production. Annu. Rev. Entomol. 62, 443–460. doi: 10.1146/annurev-ento-031616-035615
Blamires S. J., Nobbs M., Wolff J. O., Heu C. (2022). Nutritionally induced nanoscale variations in spider silk structural and mechanical properties. J. Mech. Behav. Biomed. Mater. 125, 104873. doi: 10.1016/j.jmbbm.2021.104873
Blamires S. J., Rawal A., Edwards A. D., Yarger J. L., Oberst S., Allardyce B. J., et al. (2023). Methods for silk property analyses across structural hierarchies and scales. Molecules 28, 2120. doi: 10.3390/molecules28052120
Bolland H. R. (1983). A description of Neophyllobius aesculi n. sp. and its developmental stages (Acari: Camerobiidae). Entomol. Ber. 43, 42–47.
Büsse S., Hörnschemeye T., Hohu K., McMillan D., Edgerly J. S. (2015). The spinning apparatus of webspinners – functional-morphology, morphometrics and spinning behavior. Sci. Rep. 5, 9986. doi: 10.1038/srep09986
Clotuche G., Mailleux A.-C., Astudillo F. A., Deneubourg J.-L., Detrain C., Hance T. (2011). The formation of collective silk balls in the spider mite Tetranychus urticae Koch. PloS One 6, e18854. doi: 10.1371/journal.pone.0018854
Craig C. L. (1997). Evolution of arthropod silks. Ann. Rev. Entomol. 42, 231–267. doi: 10.1146/annurev.ento.42.1.231
Craig C. L. (2003). Spiderwebs and silk: Tracing evolution from molecules to genes to phenotypes (Oxford: Oxford Univ. Press Inc).
Craig C. L., Riekel C., Herberstein M. E., Weber R. S., Kaplan D., Pierce N. E. (2000). Evidence for diet effects on the composition of silk proteins produced by spiders. Mol. Biol. Evol. 17, 1904–1913. doi: 10.1093/oxfordjournals.molbev.a026292
Dabert M., Proctor H., Dabert J. (2016). Higher-level molecular phylogeny of the water mites (Acariformes: Prostigmata: Parasitengonina: Hydrachnidiae). Mol. Phylog. Evol. 101, 75–90. doi: 10.1016/j.ympev.2016.05.004
Engster M. S. (1976). Studies on silk secretion in the Trichoptera (F. L imnephilidae). I. Histology, histochemistry, and ultrastructure of the silk glands. J. Morph. 150, 183–212. doi: 10.1002/jmor.1051500109
Fernandez A. A., Hance T., Clotuche G., Mailleux A.-C., Deneubourg J. L. (2012). Testing for collective choices in the two-spotted spider mite. Exp. Appl. Acarol. 58, 11–22. doi: 10.1007/s10493-012-9558-5
Gerson U. (1985). “Webbing,” in Spider mites. Their biology, natural enemies and control, vol. 1A . Eds. Helle W., Sabelis M. W. (Elsevier, Amsterdam), 223–232.
Gosline J. M., Guerette P. A., Ortlepp C. S., Savage K. N. (1999). The mechanical design of spider silks: from fibroin sequence to mechanical function. J. Exp. Biol. 202, 3295–3303. doi: 10.1242/jeb.202.23.3295
Gould S. A., Tran K. T., Spagna J. C., Moore A. M., Shulman J. B. (1999). Short and long range order of the morphology of silk from Latrodectus hesperus (Black Widow) as characterized by atomic force microscopy. Int. J. Biol. Macromol. 24, 151–157. doi: 10.1016/S0141-8130(99)00003-3
Hajer J., Malý J., Hrubá L., Reháková D. (2009). Egg sac silk of Theridiosoma gemmosum (Araneae: Theridiosomatidae). J. Morph. 270, 1269–1283. doi: 10.1002/jmor.10757
Hatano T., Nagashima T. (2015). The secretion process of liquid silk with nanopillar structures from Stenopsyche marmorata (Trichoptera: Stenopsychidae). Sci. Rep. 5, 9237. doi: 10.1038/srep09237
Kakui K., Hiruta C. (2014). Diverse pereopodal secretory systems implicated in thread production in an apseudomorph tanaidacean crustacean. J. Morph. 275, 1041–1052. doi: 10.1002/jmor.v275.9
Kanazawa M., Sahara K., Saito Y. (2011). Silk threads function as an A’dhesive cleaner’ for nest space in a social spider mite. Proc. Biol. Sci. 278, 1653–1660. doi: 10.1098/rspb.2010.1761
Knight D. P., Vollrath F. (2002). Spinning an elastic ribbon of spider silk. Phyl. Trans. R. Soc Lond. B 357, 219–227. doi: 10.1098/rstb.2001.1026
Kovoor J., Zylberberg L. (1980). Fine structural aspects of silk secretion in a spider (Araneus diadematus). I. Elaboration in the pyriform glands. Tissue Cell 12, 547–556. doi: 10.1016/0040-8166(80)90044-0
Kovoor J., Zylberberg L. (1982). Fine structural aspects of silk secretion in a spider. II. Conduction in the pyriform glands. Tissue Cell 14, 519–530. doi: 10.1016/0040-8166(82)90044-1
Krafft B., Cookson L. J. (2012). The role of silk in the behaviour and sociality of spiders. Psyche. 2012, 529564. doi: 10.1155/2012/529564
Kronenberger K., Moore P. G., Halcrow K., Vollrath F. (2012). Spinning a marine silk for the purpose of tube-building. J. Crustac. Biol. 32, 191–202. doi: 10.1163/193724011X615532
Le Goff G. J., Hance T., Detrain C., Deneubourg J.-L., Clotuche G., Mailleux A.-C. (2011). Impact of starvation on the silk attractiveness in a weaving mite, Tetranychus urticae (Acari: Tetranychidae). J. Ethol. 30, 125–132. doi: 10.1007/s10164-011-0305-x
Li S. F. Y., McGhie A. J., Tang S. L. (1994). New internal structure of spider dragline silk revealed by atomic force microscopy. Biophys. J. 66, 1209–1212. doi: 10.1016/S0006-3495(94)80903-8
Manson D. C. M., Gerson U. (1996). “Web spinning, wax secretion and liquid secretion by eriophyoid mites,” in Eriophyoid mites – their biology, natural enemies and control. Eds. Lindquist E. E., Sabelis M. W., Bruin J. (Elsevier, BV), 251–258.
Masumoto T., Masumoto T., Yoshida M., Nishikawa Y. (1998). Time budget of activity in the water spider Argyroneta aquatica (Araneae: Argyronetidae) under rearing condition. Acta Arachnologica 47, 125–131. doi: 10.2476/asjaa.47.125
Osborn Popp T. M., Addison J. B., Jordan J. S., Damle V. G., Rykaczewski K., Chang S. L. Y., et al. (2016). Surface and wetting properties of embiopteran (webspinner) nanofiber silk. Langmuir 32, 4681–4687. doi: 10.1021/acs.langmuir.6b00762
Perera D., Li L., Walsh C., Silliman J., Xiong Y., Wang Q., et al. (2023). Natural spider silk nanofibrils produced by assembling molecules or disassembling fibers. Acta Biomaterialia 168, 323–332. doi: 10.1016/j.actbio.2023.06.044
Reynolds E. S. (1963). The use of lead citrate at high pH an electron-opaque stain in electron microscopy. J. Cell Biol. 17, 208–212. doi: 10.1083/jcb.17.1.208
Riekel C., Burghammer M., Rosenthal M. (2019). Nanoscale X-ray diffraction of silk Fibers. Front. Mater 6. doi: 10.3389/fmats.2019.00315
Riekel C., Burghammer M., Rosenthal M. (2020). Mesoscale structures in amorphous silks from a spider’s orb−web. Sci. Rep. 10, 18205. doi: 10.1038/s41598-020-74638-0
Schaber C. F., Grawe I., Gorb S. N. (2023). Attachment discs of the diving bell spider Argyroneta aquatica. Commun. Biol. 6, 1232. doi: 10.1038/s42003-023-05575-7
Schniepp H. C., Koebley S. R., Vollrath F. (2023). Brown recluse spider’s nanometer scale ribbons of stiff extensible silk. Adv. Mater. 25, 7028–7032. doi: 10.1002/adma.201302740
Sehnal F., Akai H. (1990). Insect silk glands: their type, development and function, and effects of environmental factors and morphogenetic hormones on them. Int. J. Insect Morphol. Embryol. 19, 79–132. doi: 10.1016/0020-7322(90)90022-H
Shatrov A. B. (2013). Anatomy and ultrastructure of dermal glands in an adult water mite, Teutonia cometes (Koch 1837) (Acariformes: hydrachnidia: teutoniidae). Arthr. Str. Dev. 42, 115–125. doi: 10.1016/j.asd.2012.10.006
Shatrov A. B., Soldatenko E. V. (2016). Dermal glands in freshwater mites Limnesia undulata (O.F. Müller 1776) and L. fulgida (C.L. Koch 1836) (Acariformes, Limnesiidae). Arthr. Str. Dev. 45, 341–355. doi: 10.1016/j.asd.2016.05.003
Shatrov A. B., Soldatenko E. V. (2022). Organization of dermal glands and characteristic of secretion in the freshwater mite, Limnesia maculata (O.F. Muller 1776) (Acariformes, Limnesiidae). J. Morph. 283, 346–362. doi: 10.1002/jmor.21447
Shatrov A. B., Soldatenko E. V., Benken K. A., Petrov A. A. (2023). The structural analysis of secretion in the freshwater mite Limnesia maculata (Acariformes, Limnesiidae) supports the idea of a new form of arthropod silk. Exp. Appl. Acarol. 90, 277–300. doi: 10.1007/s10493-023-00826-y
Shatrov A. B., Soldatenko E. V., Gavrilova O. V. (2014). Observation on silk production and morphology of silk in water mites (Acariformes: Hydrachnidia). Acarina 22, 133–148.
Shatrov A. B., Soldatenko E. V., Gavrilova O. V. (2016). Morphology of tube-like threads related to Limnochares aquatica (L. 1758) (Acariformes: Hydrachnidia: Limnocharidae) in the laboratory. J. Nat. Hist. 50, 2199–2214. doi: 10.1080/00222933.2016.1193643
Shatrov A. B., Soldatenko E. V., Stolbov V. A., Smirnov P. A., Petukhova O. A. (2019). Ultrastructure and functional morphology of dermal glands in the freshwater mite Limnochares aquatica (L. 1758) (Acariformes, Limnocharidae). Arthr. Str. Dev. 49, 85–102. doi: 10.1016/j.asd.2018.11.010
Shin H.-K., Yoo S.-H., Lee W.-K., Park J.-W., Lee I.-Y. (2006). Microstructure of faecal pellets and silk of the two spotted spider mite, Tetranychus urticae (Tetranychidae: Acarina). Korean J. Soil Zool. 11, 7–12.
Sponner A., Schlott B., Vollrath F., Unger E., Grosse F., Weisshart K. (2005). Characterization of the protein components of Nephila clavipes dragline silk. Biochemistry 44, 4727–4736. doi: 10.1021/bi047671k
Sponner A., Vater W., Weisshart K. (2007). Composition and hierarchical organisation of a spider silk. PloS One 2, e998. doi: 10.1371/journal.pone.0000998
Stubbs D. G., Tillinghast E. K., Townley M. A. (1992). Fibrous composite structure in a spider silk. Naturwissenschaften 79, 231–234. doi: 10.1007/BF01227136
Sutherland T. D., Young J. H., Weisman S., Hayashi C. Y., Merritt D. J. (2010). Insect silk: one name, many materials. Ann. Rev. Entomol 55, 171–188. doi: 10.1146/annurev-ento-112408-085401
Tsalolokhin S. J. (1997). Key to freshwater invertebrates of Russia and adjacent lands. T. 3. Arachnida, Lower Insects (St.-Petersburg: Zoological Institute of the Russian academy of Sciences).
Vollrath F. (2000). Strength and structure of spiders’ silks. Rev. Mol. Biotech. 74, 67–83. doi: 10.1016/S1389-0352(00)00006-4
Vollrath F., Holtet T., Thøgersen H. C., Frische S. (1996). Structural organization of spider silk. Proc. R. Soc London B 263, 147–151.
Vollrath F., Knight D. P. (2001). Liquid crystalline spinning of spider silk. Nature 410, 541–548. doi: 10.1038/35069000
Wallace M. M. H., Mahon J. A. (1972). The taxonomy and biology of Australian Bdellidae (Acari). I. Subfamilies Bdellinae, Spinibdellinae and Cytinae. Acarologia 14, 544–580.
Wang Q., McArdle P., Wang S. L., Wilmington R. L., Xing Z., Greenwood A., et al. (2022). Protein secondary structure in spider silk nanofibrils. Nat. Commun. 13, 4329. doi: 10.1038/s41467-022-31883-3
Wirth M., Wolff J. O., Appel E., Gorb S. N. (2019). Ultrastructure of spider thread anchorages. J. Morph. 280, 534–543. doi: 10.1002/jmor.20962
Yano S. (2012). Cooperative web sharing against predators promotes group living in spider mites. Behav. Ecol. Soc. doi: 10.1007/s00265-012-1332-5
Yonemura N., Sehnal F., Mita K., Tamura T. (2006). Protein composition of silk filaments spun under water by caddisfly larvae. Boimacromolecules 7, 3370–3378. doi: 10.1021/bm060663u
Keywords: silk threads, web, ultrastructure, water spider, water mites, tetranychid mites
Citation: Shatrov AB and Soldatenko EV (2024) Ultrastructure of silk threads of the water spider Argyroneta aquatica (Clerck, 1757) (Araneae, Cybeidae) in comparison with that of some mites. Front. Arachn. Sci. 3:1384553. doi: 10.3389/frchs.2024.1384553
Received: 09 February 2024; Accepted: 05 April 2024;
Published: 26 April 2024.
Edited by:
André Walter, Aarhus University, DenmarkReviewed by:
Sean J. Blamires, University of New South Wales, AustraliaClemens F. Schaber, University of Kiel, Germany
Copyright © 2024 Shatrov and Soldatenko. This is an open-access article distributed under the terms of the Creative Commons Attribution License (CC BY). The use, distribution or reproduction in other forums is permitted, provided the original author(s) and the copyright owner(s) are credited and that the original publication in this journal is cited, in accordance with accepted academic practice. No use, distribution or reproduction is permitted which does not comply with these terms.
*Correspondence: Andrey B. Shatrov, Andrey.Shatrov.1954@mail.ru
†ORCID: Andrey B. Shatrov, orcid.org/0000-0001-9710-2078
Elena V. Soldatenko, orcid.org/0000-0002-0602-0913