Abstract
The latest science shows that, to stop global warming, we must reach “net zero” emissions by 2050. This means that any carbon dioxide (CO2) humans are still releasing into the atmosphere in 2050 would need to be balanced out by taking CO2 from the atmosphere and locking it away. The process of removing CO2 from the atmosphere is called carbon dioxide removal (CDR). This article will explain why CDR is needed and will introduce some methods of removing CO2, including the strengths and weaknesses of those methods. There are many actions we can take. Many bring lots of other benefits, while some have drawbacks too. But overall, Carbon Dioxide Removal has a big role to play in successfully tackling climate change.
What is Carbon Dioxide Removal?
It is well known that carbon dioxide (CO2) released into the atmosphere by human activities is causing our climate to change, read more in this Frontiers for Young Minds article, and that something needs to be done about it. The science is clear and shows that, to stop global warming, we must reach “net zero” emissions by 2050 [e.g. [1, 2]]. In other words, we must take action to make sure we are not putting any more CO2 into the air. In this article, we will explain how carbon dioxide removal (CDR) can be one of those helpful actions. Imagine a bathtub that is filling up with water. If we do not turn the water off, the bathtub will soon be full. This is exactly the stage we are at with CO2 emissions in Earth’s atmosphere (Figure 1). If we continue to put the same amount of emissions into the atmosphere as we have been, there are less than 6 years left before the bathtub flows over, which means we are emitting more CO2 than we should if we wish to keep global warming below 1.5°C. We have set 1.5°C as the goal because, if global warming exceeds that number, the risk of dangerous impacts from climate change is increased and our societies and the Earth may even experience irreversible harm. Luckily, CDR can be used to “mop up” some of the overflow by taking some of the CO2 out of the atmosphere and locking it away.
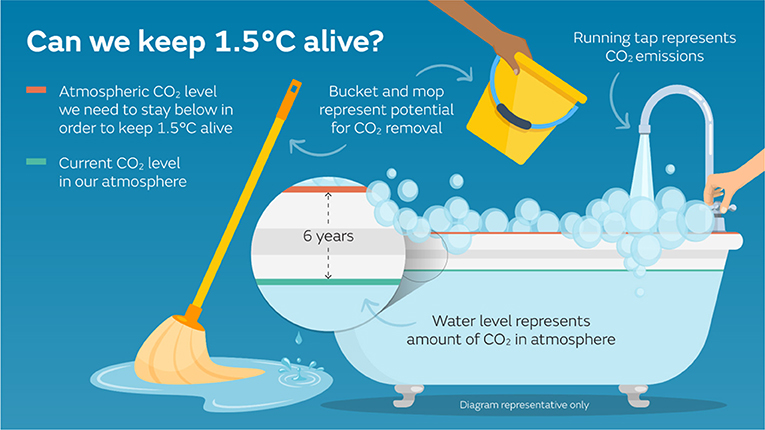
- Figure 1- Can we keep 1.5°C alive? The water level in the bathtub represents the amount of CO2 in Earth’s atmosphere. The tap represents ongoing CO2 emissions caused by human activities. The tub will overflow if too much CO2 is emitted, which scientists predict will happen within 6 years if CO2 emissions are not reduced. The overflow can be “mopped up” by CDR. We can also capture CO2 as it is being released (represented by the bucket) to keep CO2 in the atmosphere from increasing.
CDR can help in two ways. First, it can help us to deal with CO2 emissions that are difficult to completely eliminate. In our bathtub example, that means that the water is still trickling out into the bathtub, so we must take a bucket and remove some of the water, so that the water level does not rise any further. Second, if we have already emitted more CO2 than we are allowed and therefore cause global temperatures to rise more than 1.5°C, we can use CDR to try to reverse this extra warming. In terms of our bathtub story, that means if the bathtub really flows over, we can clean the water off the floor.
However, we want to avoid the bathtub overflowing if we can. Turning off the water is better than cleaning up the mess afterwards, and there is a limit to how much we can mop up if water continues to overflow! This is why we must prioritize reducing CO2 emissions and not become too dependent on CDR. We know that some effects of climate change (like the loss of certain species) will be irreversible, even if we manage to bring global warming back on track. So, avoiding the CO2 mess rather than cleaning it up is our main goal. There are several methods to remove CO2 from the atmosphere (Figure 2) and we will summarize a few of them in the sections that follow. All of these techniques have strengths and weaknesses [3], as summarized in Figure 3.
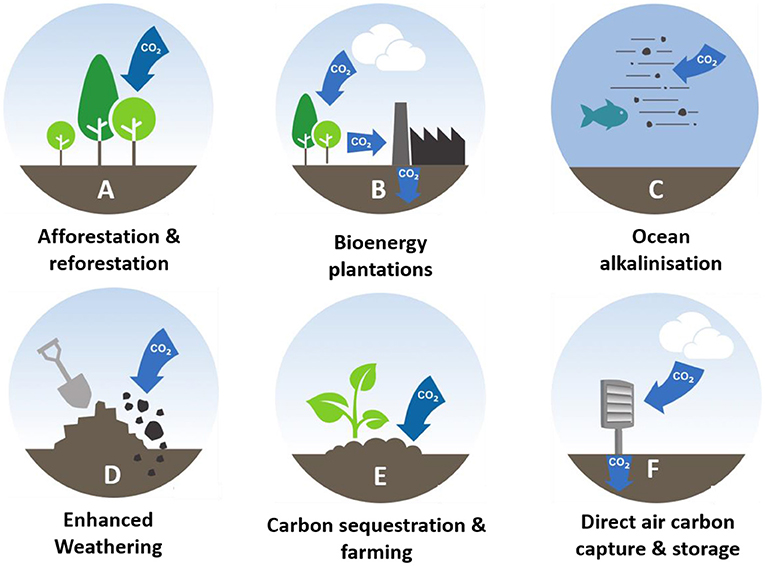
- Figure 2 - Methods of CDR include: (A) planting trees to remove CO2, (B) capturing CO2 in crops that are then burned for energy, (C) adding crushed minerals to the oceans to absorb CO2, (D) crushing minerals like those found in rocks to speed up weathering, (E) adding biochar to soils, and (F) removing CO2 from the air with filters and storing it underground.
- All of these have some advantages. They also have disadvantages. Which means that none of them are the perfect solution, and we will likely need to use a combination of methods.
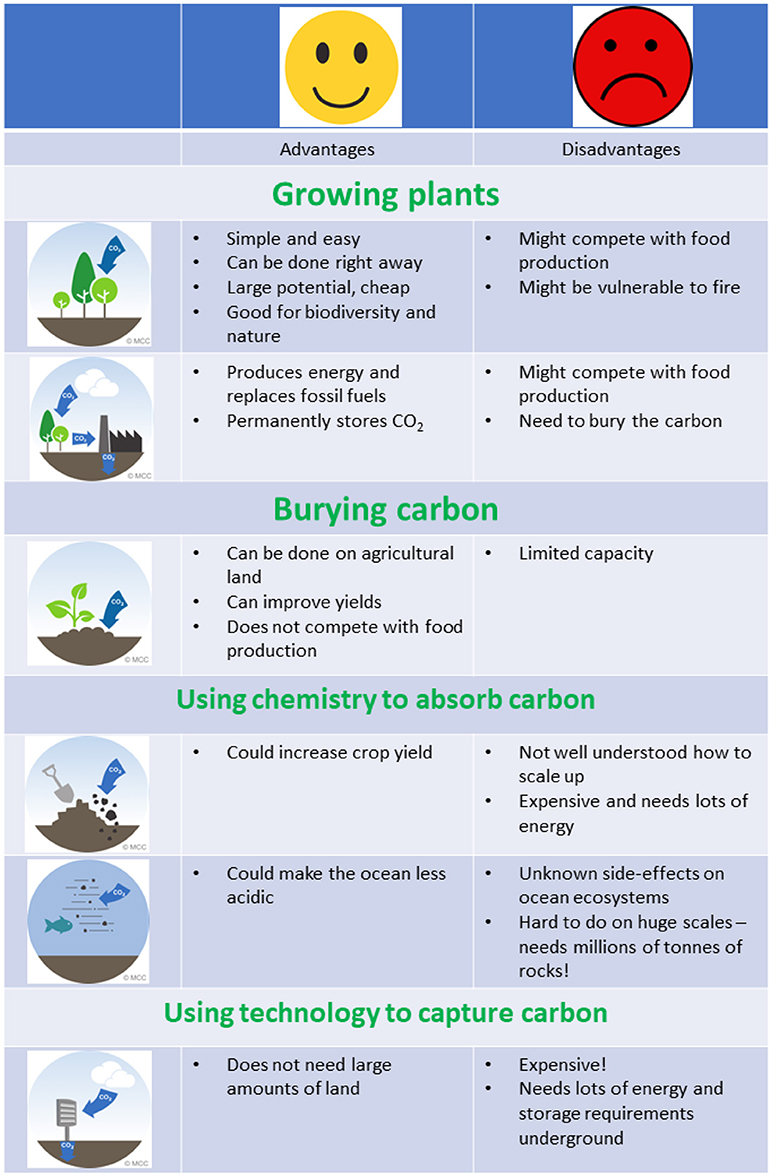
- Figure 3 - All methods of CDR shown in Figure 2 have advantages and disadvantages, as listed in this table.
Growing Plants To Absorb CO2
The most straightforward way to remove CO2 from the atmosphere is afforestation. This means planting additional trees, which can remove CO2 from the air by photosynthesis. Afforestation has a couple of advantages. Planting trees does not require a lot of materials, so it can be done right away. It is also much cheaper than other methods of CDR. Afforestation can also have many other benefits. For example, regrowing forests can expand and connect habitats for wildlife and thus help biodiversity. More trees can also help improve the local climate by lowering temperatures and cleaning the air.
In addition to planting trees, we can also use land to grow crops that can be burned to produce energy in place of fossil fuels. This is known as bioenergy with carbon capture and storage (BECCS). In BECCS, CO2 is captured in plants and then used to produce energy. The trick is not to let the CO2 escape when we burn the crops to create energy, but rather to capture the CO2 and store it underground. The advantages of BECCS are that energy is produced and CO2 is stored permanently. However, the systems and structures needed to transport and store CO2 have not yet been fully developed. BECCS would greatly benefit from politicians creating policies to support this method, so that it becomes established in society.
There are also downsides to BECCS. If it is done incorrectly, then biodiversity, water flow, and other important benefits that forests provide for people can be disturbed. Forests are already suffering from climate change, and the CO2 held in those forests can quickly be released again if forests burn in wildfires or die because of pests like bark beetles. Also, if BECCS is done on a large scale, it would take up a lot of land, which is also needed to feed Earth’s growing population and as habitat for wildlife.
Burying Carbon in the Land
Further land-based techniques for CDR include storing carbon in the soil. This can be done by growing special plants called cover crops on farm fields, or by plowing the fields less, for example. These techniques are good for farmers because they can actually improve the amount of food that can be grown. Also, these methods do not cost much or require additional land, which is an advantage compared to afforestation and BECCS.
Heating up biomass in an oxygen-free environment (a process known as pyrolysis) produces a substance called biochar—a type of charcoal that stores a lot of CO2 and can then be mixed into soils or used in products like building materials. If biochar is created in a large facility, the gases and heat generated in the process can also be used to produce energy. Applying biochar to soils can have many additional benefits, like improving soil quality and filtering harmful substances out of rainwater. The Indigenous people of South America improved their soils using biochar as far back as 4,000 years ago!
Using Chemistry
Earth’s minerals, like those found in rocks, usually break down very slowly—over thousands of years—by a process called weathering. Weathering absorbs CO2. It is possible to enhance the natural process of weathering by grinding the minerals. Grinding makes the surface area much larger, allowing more CO2 to be taken out of the atmosphere in a much shorter time. If ground-up minerals are spread on agricultural fields, crop yields can be increased. However, more experiments are needed to better understand how this works at a large scale and what the side effects are. Also, the grinding needs a lot of energy, and generating that energy must not release CO2.
Other techniques use the ocean to absorb CO2. Alkalinisation involves putting crushed minerals that can bind CO2 (much like what was described for enhanced weathering) into the ocean. This would also make oceans less acidic, which protects marine life from other bad effects of dissolved CO2. But with this technique too, more work is needed to better understand its effects on ecosystems and whether any unintended damage might be caused.
Using Technology
One CDR technology that does not need much land is direct air carbon capture and storage (DACCS). In DACCS, CO2-containing air is sucked into a collector, where it flows through a filter. CO2 sticks to the filter and, when the filter is full, the collector closes and is heated up to 100°C, so the CO2 molecules are released from the filter and can be captured and stored. Similar to BECCS, using DACCS on a large scale will require development of systems and structures to transport and store CO2. Also, DACCS needs a lot of energy, which is currently not yet CO2-free and makes DACCS a relatively expensive technology compared to the others. However, there is a lot of activity around DACCS, with large sums of money promised for its development, and several companies are interested in working on this technology.
What About Sustainability?
As we have explained, all the methods to clean up CO2 have their own disadvantages. Some need lots of land, which might be used for growing food, some need lots of energy, others cannot be used widely before we fully understand their side effects. In the end, none of these techniques will save us on its own—we will most likely use a combination of techniques to remove CO2, depending on location, timing, and amount of CO2 that must be removed. The most important message, however, is that there will be far less CO2 to remove if we reduce the amount we are emitting as quickly and completely as possible. To go back to our bathtub example once more, it is far better to prevent the tub from overflowing than to clean up the mess later on. Just because we have the technology to clean up after ourselves does not mean it is OK to increase the mess we have already created! So, in addition to continuing to develop CDR, people should keep working to decrease the amount of CO2 released into the atmosphere.
Glossary
Carbon Dioxide Removal (CDR): ↑ Human actions that remove CO2 from the atmosphere and lock it away.
Emissions: ↑ Greenhouse gases, such as CO2, that are released into the atmosphere by human activities and harm the planet.
Afforestation: ↑ Planting trees on land that previously did not have forests on it, or on land that had forests that were cut down.
Bioenergy With Carbon Capture and Storage (BECCS): ↑ Bioenergy is energy made by burning plants rather than fossil fuels. Carbon capture and storage means catching the CO2 before it goes into the atmosphere and burying it underground.
Pyrolysis: ↑ This means heating up wood or other material, but without oxygen so that it cannot catch fire and burn. This leaves a special type of charcoal which lasts a long time and can store carbon if we bury it.
Biochar: ↑ A kind of charcoal obtained by burning trees and plants in the absence of oxygen.
Weathering: ↑ A natural process that happens over thousands of years as minerals are broken down and can absorb CO2. Speeding up weathering might help to reduce CO2 in the air.
Alkalinisation: ↑ A chemical process that involves adding minerals to the ocean so that ocean water can absorb more CO2.
Direct Air Carbon Capture and Storage (DACCS): ↑ Filtering CO2 directly out of the air and storing it underground.
Conflict of Interest
The authors declare that the research was conducted in the absence of any commercial or financial relationships that could be construed as a potential conflict of interest.
Acknowledgments
We want to thank George Burningham at the Met Office for the design of Figure 1 and William Lamb at MCC for help with Figure 2. IS acknowledges the financial support by the project CDRSynTra, funded by the German Federal Ministry of Education and Research (BMBF), grant number: 01LS2101A. SF acknowledges funding from the European Union’s Horizon Europe research and innovation programme under grant agreement No 101081521 (UPTAKE).
References
[1] ↑ IPCC. 2021. “Summary for policymakers”, in Climate Change 2021: The Physical Science Basis. Contribution of Working Group I to the Sixth Assessment Report of the Intergovernmental Panel on Climate Change, eds V. Masson-Delmotte, P. Zhai, A. Pirani, S. L. Connors, C. Péan, S. Berger, et al. (Cambridge: Cambridge University Press).
[2] ↑ IPCC. 2022. “Summary for policymakers”, in Climate Change 2021: Mitigation of Climate Change. Contribution of Working Group I to the Sixth Assessment Report of the Intergovernmental Panel on Climate Change, eds P. R. Shukla, J. Skea, R. Slade, A. Al Khourdajie, R. van Diemen, D. McCollum, et al. (Cambridge: Cambridge University Press).
[3] ↑ Smith, P., Davis, S., Creutzig, F., Fuss, S., Minx, J., Gabrielle, B., et al. 2016. Biophysical and economic limits to negative CO2 emissions. Nat. Clim. Change 6, 42–50. doi: 10.1038/nclimate2870