- Center for Development and Behavioral Neuroscience, Psychology and Biology Departments, Binghamton University – State University of New York, Binghamton, NY, USA
Parental investment can be used as a forecast for the environmental conditions in which offspring will develop to adulthood. In the rat, maternal behavior is transmitted to the next generation through epigenetic modifications such as methylation and histone acetylation, resulting in variations in estrogen receptor alpha expression. Natural variations in maternal care also influence the sexual strategy adult females will adopt later in life. Lower levels of maternal care are associated with early onset of puberty as well as increased motivation to mate and greater receptivity toward males during mating. Lower levels of maternal care are also correlated with greater activity of the hypothalamus–pituitary–gonadal axis, responsible for the expression of these behaviors. Contrary to the transition of maternal care, sexual behavior cannot simply be explained by maternal attention, since adoption studies changed the sexual phenotypes of offspring born to low caring mothers but not those from high caring dams. Indeed, mothers showing higher levels of licking/grooming have embryos that are exposed to high testosterone levels during development, and adoption studies suggest that this androgen exposure may protect their offspring from lower levels of maternal care. We propose that in the rat, maternal care and the in utero environment interact to influence the reproductive strategy female offspring display in adulthood and that this favors the species by allowing it to thrive under different environmental conditions.
In humans, parental styles have been shown to be transmitted across generations. The way one is brought up is likely to be the way one will raise one’s own children. Thus, child abuse is more likely to occur in households where parents were abused as children (Widom and Maxfield, 1996; Bifulco et al., 2002). Parenting style is often dependent on environmental factors. Low socio-economic status creates stress and increases parental anxiety, which directly influences parent–child interaction (McLoyd, 1990; McLoyd and Wilson, 1990). Indeed, more anxious mothers have been shown to be less responsive to their infants (Fleming and Corter, 1988; Fleming et al., 1999; Seymour, 2005; Tu et al., 2005). Unfortunately, most people raise their children under the same socio-economic constraints they experienced growing up, resulting in a continued cycle from generation to generation.
While the field of child psychology has provided compelling research findings regarding parent–child interactions in humans, these findings are unfortunately only correlational and do not offer direct evidence of the effects of parenting on the development of children. Thus, the mechanisms involved in parent–child interaction on child development are still unknown. Research using animal models is an important tool which can provide the means to understand the mechanisms involved in this system. As in humans, infant-directed behaviors are transmitted from mother to daughter in both non-human primates (Suomi, 1978) and in the rat (Champagne et al., 2003a). We also know that environmental factors influence parental behaviors in these species, suggesting that the inter-generational transmission of behaviors is under the same constraints as those found within the human population. Furthermore, as in humans, parental (particularly, maternal) care has been shown to have an important influence on primate (McCormack et al., 2006) and rodent offspring development (Champagne et al., 2003a). This review will discuss the effects of maternal care on the offspring’s development and behaviors and will suggest mechanisms involved in this system.
Epigenetics and Reproductive Strategy
In most animal species, an individual may survive and succeed in a variety of environmental conditions. For a species to produce individuals with the capacity to adapt to a wide variety of milieu, plasticity is required, as reproductive strategies need to be adjusted to the prevailing environmental conditions. Parental investment is one of the environmental conditions that can directly influence the development of the offspring and, later in life, the offspring’s reproductive strategy. Plasticity in specific reproductive traits among same-sex members of a species are derived from variations in the quality of the prevailing environment during development which are mediated by alterations in parent–offspring interactions. As in humans, research has determined that, for most species studied, the variations in parental care that define the reproductive phenotype of the offspring are influenced by the quality of the environment (i.e., nutrient availability, predation, infection, population density, etc., Hinde, 1986; Rhen and Crews, 2002; Coall and Chisholm, 2003).
Researchers have used various paradigms to affect early life environment and observe its effects on the development of pups. Among these paradigms, prenatal stress (DeFries et al., 1967; Chapillon et al., 2002; Clinton et al., 2008), differential rearing conditions (Masur and Struffaldi, 1974; Gonzales et al., 2001), and postnatal handling (Levine, 1967) have proven particularly interesting tools. For the last 10 years, observation of natural variation in maternal care in the rat and its effects on the offspring has also been shown to be an important tool in the study of the consequences of early environment (Liu et al., 1997, 2000; Champagne et al., 2003b, 2006; Cameron et al., 2008a,b). Thus, the epigenetic field is now equipped with various animal models to study the effects of parental care on the development, physiology, and behavior of offspring.
Epigenetic
It is difficult to discern between environmental and genetic influences on development, bringing us back to the old debate between nature and nurture. Interestingly, we now know that environmental factors influence the way genes are transcribed without modifying the genome. Such “epigenetic” effects have an important role to play in shaping individual variations of behaviors, and have been the object of extensive research.
Conrad Waddington (1905–1975) was the first to use the term “epigenetics” to describe phenomena that could not be explained by genetic principles, defining it as “ the branch of biology which studies the causal interactions between genes and their products, which bring the phenotype into being” (Waddington, 1942). To be epigenetic, an event must alter chromatin structure without changing the underlying DNA sequence of the gene. Generally, DNA is tightly packaged into nucleosomes, where it is bound around histone proteins (Figure 1). The electrostatic bond is maintained by the difference in charge between DNA and histone, a configuration which prohibits transcription factors from binding to DNA, thus regulating gene expression. On histones, acetyl groups allow for loosening of the bond and the transcription of genes into proteins (Figure 1). DNA methylation, covalent histone modification, non-covalent mechanisms such as chromatin remodeling, and non-coding RNA can all interfere with gene transcription and define epigenetic identity.
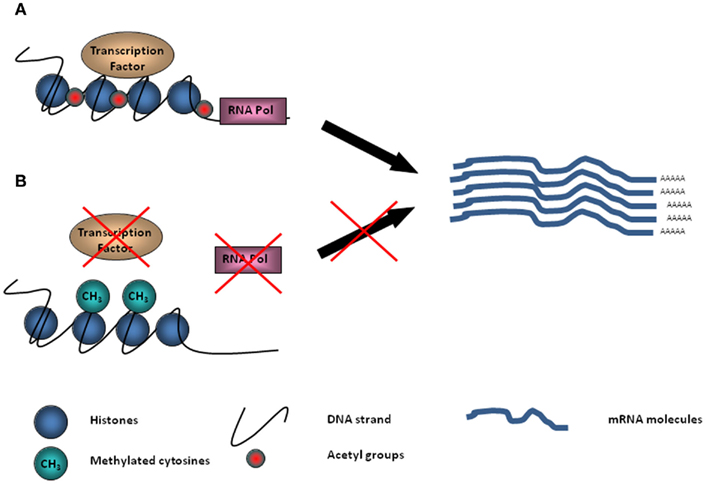
Figure 1. Schematic diagram demonstrating DNA tightly packaged into nucleosomes and bound around histone proteins. (A) On histones, acetyl groups provide the opportunity for transcriptional machinery to bind to DNA and transcription of genes into proteins to take place. (B) Methyl groups (–CH3) located on the DNA can inhibit transcription factors and RNA Pol II binding silencing gene transcription.
Maternal Care
In our laboratory, we use Long-Evans rats to study the effect of natural variations of maternal care on the offspring. As in humans, there are natural variations in both the quality and amount of maternal care that lactating rats provide to their offspring. Dams vary in the amount of licking/grooming (LG) they display toward their pups during the first week post-partum (Champagne et al., 2003a). The frequency of this behavior has been shown to be normally distributed, with dams displaying LG on average 10.6% of observed time, but can vary from 4 to 15% (Champagne et al., 2003a). Lactating females that display this behavior at a frequency 1 SD above the mean are characterized as High LG mothers and females showing an LG frequency 1 SD below the mean are characterized as Low LG mothers (Champagne et al., 2003a; Cameron et al., 2008a). The greatest variation between the two groups of mothers is most apparent during the first 6 days postpartum and it is not associated with litter size, sex ratio of the litter or weaning weight of the pups (Champagne et al., 2003a). It is important to note that High and Low LG dams are the two ends of a continuous distribution and are selected to allow the comparison of the two extremes of this behavior. Licking and grooming are of importance since rat pups are born at an early stage of their development, and these behaviors contribute to their thermoregulation and to the stimulation needed for urination and defecation (Moore and Power, 1992). The amount of LG reduces as pups grow older and gain physiological maturity (Champagne et al., 2003a).
As in humans, rat parental behavior is transmitted across generations. Female offspring of High LG mothers are likely to become High LG mothers themselves in adulthood, and Low LG female offspring will also adopt their mother’s caring behavior with their own pups (Champagne et al., 2003a). Cross-fostering studies are an effective means of investigating the genetic and environmental contributions of the inheritance of maternal behaviors. In these experiments, a few pups (two to four) are usually cross-fostered less than 12 h after birth to an age-matched litter and the maternal behaviors of both the adoptive dam and the adult female offspring are observed. The results show that female offspring of Low LG mothers raised by High LG dams become High LG mothers and High LG offspring raised by Low LG mothers become Low LG mothers themselves (Champagne et al., 2006). In contrast to the simply correlational data gained from humans, these experimental manipulations demonstrate that environmental factors such as the level of maternal care received are more important than the genome in influencing maternal care displayed by adult female offspring.
Differences in maternal care are also associated with estrogen receptor alpha (ERα) expression in the female offspring brain (Champagne et al., 2006). Estrogen plays an important role in the regulation of both the hypothalamic–pituitary–gonadal axis (HPG) and sexual behavior. In the brain of both sexes, estrogen receptors are located in abundance in the hypothalamus, as well as in the brainstem and the hippocampus (Shughrue et al., 1997; Österlund and Hurd, 2001). ERα activity in the brain is region-specific and, interestingly, maternal care appears to regulate ERα gene expression in opposite manners depending upon brain region. High LG mothers and their female offspring express more ERα mRNA than Low LG females in the medial preoptic area (MPOA), an area important for the display of maternal behavior (Champagne et al., 2006). In the anteroventral periventricular nucleus (AVPv) an area involved in the regulation of gonadal function, Low LG female offspring express more ERα mRNA than High LG offspring (Cameron et al., 2008a). These findings suggest that maternal care programming of reproduction is mediated by tissue-specific differences in ERα expression. There is evidence that epigenetic mechanisms are involved in these effects.
Maternal Programming
Estrogen receptor alpha is a ligand-activated transcription factor that regulates genes (Bjornstrom and Sjoberg, 2005). The maternal effect on ERα expression in the MPOA is associated with differential cytosine methylation of the ERα 1b promoter (Champagne et al., 2006). The exon 1b region contains a signal transducer and activator of transcription (Stat)5b response elements which are necessary for transcriptional regulation of constitutive expression of the rat ERα gene in the brain (Schibler and Sierra, 1987). DNA methylation is associated with repression of gene expression. Increases in the cytosine methylation of the ERα 1b promoter can limit binding to the Stat5b consensus sequence by attracting repressor complexes containing histone deacetylases that block the acetylation of histone tails. This favors a chromatin configuration that excludes transcription factor binding (Bird, 2002; Frasor and Gibori, 2003; Figure 1). ERα is a nuclear hormone receptor that when bound to estrogen moves to the nucleus and acts as a transcription factor that regulates gene expression. Champagne et al. (2006) have found increased methylation across the ERα 1b promoter in the MPOA of female offspring of Low LG mothers compared with High LG mothers. Using a chromatin-precipitation assay to examine Stat5b binding in the MPOA and in the ventral medial hypothalamus (VMH), an area important for the display of sexual behavior in the female, we and others have found that female offspring of High LG mothers show more binding to the exon 1b promoter in the MPOA and less in the VMH compared to Low LG female offspring (Cameron et al., 2008c). The binding maps onto the behavior of High females, which display more maternal behavior and, as we will discuss below, are also less motivated to mate than Low LG female offspring. While a direct effect of methylation on the ERα expression remains to be demonstrated, these findings reveal a tissue-specific maternal programming of gene expression.
A cross-fostering study showed that maternal care is responsible for the differences seen in ERα expression in the MPOA (Champagne et al., 2006). Biological offspring of High LG mothers cross-fostered to Low LG mothers show ERα mRNA expression that resembles the expression of Low LG offspring. This result is important, as the rearing-mother effect provides evidence for a direct relation between maternal care and ERα expression which surpasses correlational findings.
Thus far, we have discussed how early environmental conditions are crucial in determining the maternal behavior that adult female offspring display, but changes in the quality of the environment of adult animals may also result in changes in maternal care level. Champagne and Meaney (2006) have shown that 7 days of intermittent stress during the last week of gestation resulted in a reduction in the amount of maternal care displayed by pre-characterized High LG mothers. Interestingly, in this study the behavior of Low LG mothers was not affected by the stressor, suggesting that it represents the lower end of the maternal care spectrum and that lowering the amount of care any further may jeopardize reproduction in this species. Furthermore, although the dams were not re-exposed to the stressor after the second litter (the first litter was used to characterize the dam), the effect persisted even for subsequent litters. The authors also showed that female offspring from the post-stress second and third litters of stressed High LG mothers displayed maternal care and anxiety behaviors similar to Low LG female offspring. This study demonstrated that, as in humans, parental care in the rat is very sensitive to environmental factors and may result in lasting effects for generations to come.
The quality of the parent–child interaction in humans has been shown to influence the mental health and behavior of children. Parental neglect and abuse have been shown to be associated with increased anxiety and stress in children (Heim et al., 2000). It can also lead to increases in risky behaviors such as drug and alcohol abuse in adolescence and adulthood (De Bellis, 2002). Several studies have shown that parental monitoring of teenagers protects them against early onset of sexual activity (Meschke and Silbereisen, 1997) and risky sexual behaviors such as intercourse and multiple partners (Huebner and Howell, 2003). The quality of maternal care in particular has a direct effect on the behavior of the child. In a recent study, Belsky et al. (2010) found that greater maternal harshness is associated with earlier onset of puberty which in turn leads to greater sexual risk taking in 15-year-old girls. These studies reflect the importance of the parental influence in children’s behavior and physiology.
Effects of Maternal Care on Offspring
Natural variations in maternal care have been associated with effects on the development of neural systems such as the hypothalamic–pituitary–adrenal axis, which mediates stress reactivity. Indeed, Low LG offspring have been shown to be more responsive to stressors, and the effects are associated with differences in gene expression, receptor sensitivity, hormone level, and behavior compared to High LG offspring (Liu et al., 1997; Caldji et al., 1998). Low LG offspring also show a deficit in learning compared to High LG offspring (Bredy et al., 2003); here again, the key mechanism of the maternal effect is the variation in gene expression. Hippocampal glucocorticoid receptor (GR) gene expression is modulated by maternal care through two processes: the methylation of the NGFI-A consensus sequence which activates the GR gene and through the acetylation of histones H3–H9 (Fish et al., 2004). Low levels of maternal care are associated with increased methylation of the NGFI-A consensus sequence on the exon 17 promoter region and lower levels of acetylation of histone H3–H9, and both processes decrease the expression of GRs (Weaver et al., 2004a,b). As mentioned earlier, methylation is responsible for silencing genes, while acetylation of histone tends to increase gene expression. The result is a reduction in GR expression in the hippocampus in Low LG offspring, suggesting that more glucocorticoid hormones are needed to activate this brain area. Indeed, offspring of Low LG mothers show a greater hormonal response to stress (Liu et al., 1997). The contribution of maternal care in this system has been demonstrated using cross-fostering studies. When Low LG offspring were reared by High LG mothers, their behavior in a novel environment and their response to stressors were similar to biological offspring of High LG mothers (Francis et al., 1999a,b, 2002a). Interestingly, a week of daily brain (lateral ventricle) infusion of L-methionine, a precursor to S-adenosyl-methionine that serves as the donor groups for DNA methylation, also reversed the phenotype of adult High LG male offspring (Weaver et al., 2005). Increasing methylation decreased hippocampus GR expression and increased stress reactivity in High LG male offspring. This experimental manipulation demonstrates that although DNA methylation seems to be stable throughout life, availability of methyl donors may alter DNA methylation in adulthood and potentially reverse the adult phenotype.
Even very subtle variations in maternal care can influence gene expression and synaptic plasticity. A recent study by van Hasselt et al. (2011) showed that in a litter, there is always a small variation in the level of care a mother provides to individual pups. The inter-litter variation is rarely more than 1%, and males may receive 0.2% more licking than females. Thirty years ago, Celia Moore was the first to investigate sex difference in LG received (see review Moore and Power, 1992). She described how male rats receive more licking (particularly in the anogenital area) than females. This sex difference is testosterone (T)-dependent and can be reversed by injecting a female with T on the day of birth (Moore and Power, 1992). Differences in chemical content of urine between the sexes seem to be responsible for the mother preferring licking males over females (Brouette-Lahlou et al., 1999). Nevertheless, these small differences in maternal care modulate hippocampal development and functioning later in life. Indeed, LG scores of individual pups correlated with both periadolescent and adult hippocampal GR mRNA expression, and with the capacity to induce synaptic potentiation in the dentate gyrus (van Hasselt et al., 2011). This study demonstrated that even small variations in maternal care have a major impact on adult hippocampal function, and may be responsible for individual differences in stress reactivity and in learning and memory.
Many other neural systems are also affected by maternal care, with Low LG offspring showing a reduction in adrenoreceptor expression (Caldji et al., 1998), in oxytocin expression (Francis et al., 2002b), in oxytocin plasma levels in response to socio-sexual interactions (Moscovice, Borrow, and Cameron, in preparation), and in central receptor binding and in CBZ and NMDA receptor expression (Bredy et al., 2003). Greater maternal investment seems to result in higher quality offspring with increased learning capacity and less responsiveness to stressors.
Natural variations in maternal care also influence offspring reproductive strategy through modulation of the HPG axis. Differential expression of ERα in the brain has been associated with maternal care in females for both mothers and offspring. As mentioned above, High LG female offspring show an increase in the ERα receptor in the MPOA, a region important for the control of maternal care (Champagne et al., 2006). Interestingly, in the VMH and the AVPv, which are involved in the control of the HPG axis and sexual behavior in the female, Low LG female offspring show more ERα expression compared to High LG females (Cameron et al., 2008a). This differential expression of ERα correlates very well with the reproductive strategies displayed by the two rat phenotypes; High LG mothers raise female offspring that demonstrate greater levels of maternal care but are less receptive to males during mating and Low LG mothers raise female offspring that seem to have a more “efficient” reproductive strategy, since they are more receptive to males and more reproductively successful under laboratory conditions but give less maternal care to their offspring. This dichotomy is obviously artificial, since the two phenotypes are at the ends of this species’ female behavioral spectrum; nevertheless, they are showing very different behaviors.
Female Sexual Behavior
Female rat sexual behavior can be divided into two components, proceptivity and receptivity. During the proceptive phase of sexual behavior, females display a series of paracopulatory behaviors to attract males (McClintock and Adler, 1978). This pattern consists of an approach toward, orientation to, and rapid run-away from the male that can also be described as “hopping and darting,” and which often results in a mount, intromission, or ejaculation (Erskine, 1989). This pattern of behaviors is not stereotypical and is more likely to be observed in a large enclosure than if the female is in close proximity to the male, which is the case in small cages. To allow for the observation of these behaviors, researchers use large cages that allow a female to escape the male. Erskine (1985) was the first to use a pacing chamber which was made of multiple compartments separated by walls with holes too small for the male but large enough to allow the female to escape the male while he was confined in a section of the chamber (Erskine, 1985). In this chamber, the female paces mating by escaping at will and returning to the male when she wants to solicit mating. In this context, the scoring of the inter-intromission-interval (III) allows researchers to investigate female motivation to mate. In addition to proceptivity, the receptivity of rodent females to the male can be assessed by scoring the expression of lordosis behavior (Hardy and DeBold, 1972). A female rat must arch her back and move her tail to the side in order to allow the male to achieve penile intromission. This behavior is estrogen-dependent and under the control of the VMH. A decrease in ERα in this brain area has been shown to decrease the expression of this behavior (Walf et al., 2007). Our research has been able to demonstrate that expression of most of the female sexual behaviors mentioned above is influenced by natural variation in maternal care.
Maternal Care Effect on the HPG Axis
The maternal effect on female sexual behavior in the rat is extremely robust (Cameron et al., 2008a). There is a negative correlation between the percentage LG of the mother and the lordosis rating of the female offspring (Kendall’s tau-b = −0.53; p < 0.001) such that Low LG female offspring are more receptive to males during mating in a pacing chamber than High LG female offspring (Figure 2).
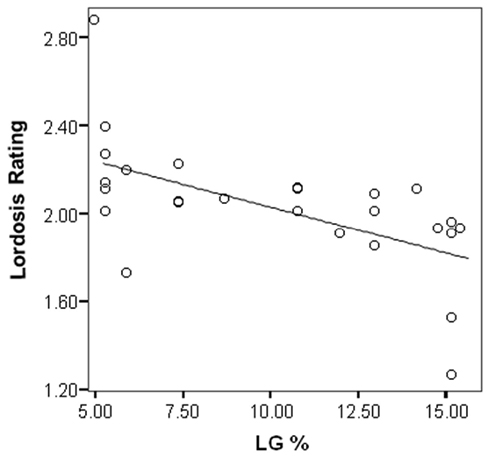
Figure 2. Lordosis rating of female offspring during mating with a male in a pacing chamber is negatively correlated with amount of licking/grooming (LG) received during the first 6 days of life (Cameron et al., 2008a). R-Square = 0.35; p < 0.001.
As previously mentioned, the display of lordosis behavior is a marker of receptivity in rodents. The behavior is scored on a scale from 0 (no arching of the back) to 3 (maximum arching), with a 2 or a 3 generally necessary for the male to achieve an intromission or an ejaculation. Not surprisingly, High LG female offspring have been shown to receive more mounts without intromission than Low LG female offspring and fewer ejaculations when tested in a pacing chamber (Cameron et al., 2008a,b). Under these normal laboratory conditions, the pregnancy rate of High LG female offspring was also significantly lower than that of Low LG female offspring (Cameron et al., 2008a). Interestingly, Lehman and Erskine (Erskine et al., 2004; Lehmann and Erskine, 2004) have shown that a greater lordosis score favors the establishment of pregnancy in the Long Evans rat. These data suggest that High levels of maternal care produce female offspring that are less receptive to males and less likely to be reproductively successful.
Most studies investigating mating behavior in the rat have used a pair of animals (one male and one female). Interestingly, in a semi-natural environment with multiple males and females (hence availability of the females is greater) McClintock and Anisko (1982) showed that the speed at which males copulate is faster than females. In this species, stimulation of the vagina or the cervix by a glass rod is sufficient to initiate the onset of hormonal changes related to pregnancy (or pseudopregnancy). Edmonds et al. (1972) have found that to achieve a progestational state (an increase in progesterone associated with pregnancy or pseudopregnancy) 10 intromissions were more efficient than only 2 intromissions. However, they also showed that five intromissions received at an III of 4–5 min were more efficient than if received faster, demonstrating that the interval between intromissions is critical for the establishment of pregnancy. In addition to being less receptive to males during mating, we have also demonstrated that in a pacing chamber, High LG female offspring return to the male compartment at a slower pace than Low LG female offspring. The difference in III between High and Low female offspring suggests an individual variation in the best interval to achieve pregnancy in the rat species. For Low LG female offspring, seven intromissions received at a 4- to 5-min interval induced pseudopregnancy significantly more often than in High LG female offspring (Cameron et al., 2011). This demonstrates an individual variation in the sensitivity to the III that may be influenced by early environmental conditions.
The studies we have described above were all performed under normal laboratory conditions: testing took place in a dark room under dim red light (rats are nocturnal) and the females were usually tested for 45 min or until they received 15 intromissions/ejaculations. These environmental conditions and testing criteria have favored the reproductive success of females raised by Low LG mothers. High LG females usually take longer to mate and often need the entire 45 min in the pacing chamber. In contrast, Low LG females often don’t need 45 min to achieve 15 intromissions/ejaculations (Cameron et al., 2008b). Investigating the behavior of rats in a more naturalistic environment could help us understand why there is so much variability in female rat sexual behavior. We have recently performed a group mating experiment which suggests that high levels of maternal care may also produce female offspring that can be reproductively successful in a more competitive environment (Prior, K., Meaney, M. J., and Cameron, N. M., unpublished results). In this study, groups of two Low and two High LG female offspring, synchronized to reach proestrus at the same time, were placed into a large pacing chamber (90 cm × 150 cm) for 36 h with two males. Sexual behaviors were recorded and scored both for the first 15 intromissions/ejaculations and for the entire 36 h. Two different mating strategies appeared in this group mating condition: The female offspring of Low LG mothers spent more time mating, while High LG offspring spent less time but were more efficient at soliciting the males. Low LG offspring showed longer III than High LG female offspring and required more time to receive an intromission after entering the male compartment during the first 15 intromissions/ejaculations.
The difference in timing of mating between High LG and Low LG female offspring in the group mating study is also very interesting. The female and male rats in this study were allowed to mate to satiety, providing High LG female offspring the opportunity to “catch up” to the Low LG female offspring and mate at the ideal rate for inducing the progestational state required for pregnancy. In a pacing chamber, where an individual female mated with only one male, we reported that High female offspring showed an III of 174.97 + 29.73 s, compared to 106.56 + 16.39 s for Low LG female offspring (Cameron et al., 2008a). In the group mating study the III of the females during the first 15 VCS was double the previously reported interval in the High LG female offspring (315.87 + 25.88 s) and almost six times greater than in a pacing chamber for the Low LG female offspring (609.72 + 177.00 s; Prior, Meaney, and Cameron, in preparation) despite the fact that they spent more time in the male compartment. Furthermore, High LG female offspring spending less time mating with the males was not surprising, as we previously reported that they may be more likely to reach estrous termination earlier (Cameron et al., 2011). Indeed, we found that the VMH, thought to be involved in estrous termination (Georgescu et al., 2009), was activated more by 15 intromissions/ejaculations in the High LG female offspring than in Low LG daughters (Cameron et al., 2011). Activation of the VMH is steroid-dependent and the difference in behavior between the two groups of females may be driven by the difference in plasma progesterone levels, which is lower in High LG female offspring during proestrus compared to Low LG daughters, or might also be caused by the difference in ERα expression in this brain area (Cameron et al., 2008a). We can speculate that the difference in timing of mating seen in this experiment may be related to both the onset and the termination of sexual behavior, which are both dependent on the hormonal state of the animal. Experiments involving hormonal manipulation using the same testing conditions would be needed to verify this hypothesis.
In the group mating study, Low LG female offspring received more ejaculations than High LG female offspring during the first 15 intromissions/ejaculations, which may be due to the difference in lordosis ratio between the two groups of females (Prior, Meaney, and Cameron, in preparation). Interestingly, this difference in the number of ejaculations disappeared as mating continued. During both the short and long periods observed, High LG female offspring’s approaches to males resulted more often in an intromission, suggesting that males were more receptive to High LG than Low LG female offspring. Most importantly, contrary to previous studies using pacing chambers where an individual female mated with only one male, no difference in pregnancy rate was seen between the two groups, demonstrating that both mating strategies are reproductively successful under competitive conditions (Prior, Meaney, and Cameron, in preparation). Nevertheless, to achieve the same success it took less time mating for High LG females, since they were favored by the males when they entered the male chamber. This study was the first to suggest that male rats may choose their partner according to the level of maternal care they have received, or perhaps according to the maternal care that they will provide to their young.
Mechanisms Involved in the Programming by Maternal Care
Gonadotropin-releasing hormone (GnRH) plays an important role in the HPG axis. In the rat, GnRH neurons develop from the embryonic olfactory placode and migrate caudally to the septum/preoptic area by day 17 of pregnancy (Gao et al., 1996). In adults, GnRH neurons are located in the median preoptic nucleus, the AVPv, the MPOA, the lateral preoptic nucleus, and the diagonal band nucleus (Wray and Hoffman, 1986). The release of GnRH in the median eminence is mediated by estrogen and reaches the anterior pituitary gonadotrope cells, where it stimulates the release of luteinizing hormone (LH) and follicular stimulating hormone (Cooper et al., 1974; Ojeda and McCann, 1978), thereby influencing the timing of puberty, ovulation, and other physiological processes as well as sexual behavior.
The effect of natural variations in maternal care on the sexual behavior of female offspring is likely to result from differential modulation of the GnRH neuron population. Indeed, we have described a difference in the number of GnRH cells detected using immunohistochemistry techniques between female offspring of Low LG and High LG mothers. Ovariectomized steroid-primed female offspring of Low LG mothers show increased amounts of GnRH neurons compared to the offspring of High LG mothers (Cameron et al., 2008a). LH and progesterone release during proestrus have also been observed to be greater in females that received lower levels of maternal care (Cameron et al., 2008a). Although we found no effect of maternal care on estrogen release in proestrus females, sensitivity to estrogen was greater in Low LG compared to High LG female offspring. Indeed, we found that lower levels of maternal care are associated with increased LH release in response to estrogen in ovariectomized females (Cameron et al., 2008a). The difference in estrogen sensitivity is probably linked to the difference in ER-α expression seen in the AVPv; however, additional studies using siRNA or antisense oligonucleotide, which can modulate the expression of the receptor, are needed to verify this hypothesis. Both the variations in ER-α expression and in GnRH neuron populations could be responsible for the differences in LH and progesterone in proestrus females as well as the difference in sexual behaviors. Interestingly, the estrogen modulation of GnRH release is indirect, as GnRH neurons lack estrogen receptors (Herbison and Theodosis, 1992). Fortunately, an intermediary system sensitive to estrogen and capable of modulating GnRH has recently been identified.
Kisspeptin neuron populations are mainly located in two brain areas: the rostral periventricular area of the third ventricle (RP3V), which includes the AVPv and the preoptic ventricular nucleus (Yeo and Herbison, 2011), and the arcuate nucleus (Clarkson et al., 2009). These neurons express ER-α and project to GnRH neurons, where they activate the kisspeptin receptor (GPR-54, also known as KISS-1R; Clarkson et al., 2009). Research has demonstrated that the regulation of GnRH neurons by kisspeptin is critical for normal pubertal onset and normal estrous cycling in the female rodent (Clarkson et al., 2009). The RP3V kisspeptin neuron population is known to be involved in a positive estrogen feedback circuit (estrogen → kisspeptin → GnRH neurons → gonadotrope cells → estrogen) that is essential for the full elaboration of puberty onset and the estrous cycle (Clarkson et al., 2009). Since it is such an important contribution to the HPG axis, research will be needed to verify the effect of maternal care on kisspeptin neuron populations.
An effect of maternal care on kisspeptin neurons is suspected, considering that we have already described a variation in the onset of puberty in this animal model. Indeed, Low LG offspring show vaginal opening, which is a marker of onset of puberty in rodents, approximately 5 days earlier than High LG offspring (Cameron et al., 2008a). This difference in the onset of puberty is important since the estrous cycle in the rat is approximately 4–5 days. This earlier onset may allow Low LG female offspring to give birth to their first litter earlier than High LG females. Under harsh environmental conditions, an earlier onset of puberty and pregnancy may be important assets.
Our understanding of the mechanisms involved in maternal programming of the reproductive system of female offspring although improved by the uses of experimental manipulation in rats, is still limited. The parallel that can be made between humans and rats, particularly in individual variations in parental and in sexual behavior, suggests that much more can be discovered using this animal model. Research focusing on the HPG axis and reproductive function are particularly important to understand the mechanisms of maternal programming. Most of the data is still correlational and must be followed by experimental manipulation aimed to understand this system. For instance, differences in maternal care levels are associated with differences in GnRH expression in the brain of female rat offspring. GnRH has recently been identified as a candidate for epigenetic modifications that could lead to variation in pubertal onset in non-humans primates (Terasawa et al., 2010). It is possible that GnRH is under the same epigenetic influence as ERα and could be another mechanism of maternal programming in this species. Another example concerns the most recent player in this system; although the importance of kisspeptin as a modulator of the HPG axis is gaining recognition, we still do not know if it plays a role in maternal programming of reproductive function. Studies using kisspeptin infusions in peripubertal animals could shed light on its effects on the maternal programming of puberty onset. It should also be noted that most of the findings described here were discovered using only High and Low LG offspring and little is known about where the average (Mid) female Long-Evans rats would fit. We have observed Mid LG females’ sexual behavior and found that they lie between High LG and Low LG female offspring (Cameron et al., 2008a). We suspect that this would be the case for most variables, but research should try to determine if it is really the case.
Although we have described an important number of individual variations in the HPG axis of the female that are associated with natural variation in maternal care (Figure 3), most of these results are only correlational. We have used cross-fostering studies in an effort to try to verify the effect of maternal care versus genetic and in utero environment contributions on the reproductive system. Interestingly, contrary to the effects on the female offspring’s maternal care or the HPA axis described above, we have found that cross-fostering was able to only partially reverse sexual behavior (Cameron et al., 2008a). Indeed, Low LG female offspring raised by High LG mothers showed a lordosis rating equivalent to High LG offspring raised by High LG mothers. In contrast, High LG female offspring raised by Low LG mothers displayed a lordosis rating resembling the one displayed by their siblings raised by High LG mothers. This effect could be due to high levels of maternal care programming the endocrine system and somehow masculinizing the Low LG female offspring. Interestingly, when embryos were collected at day 22 of pregnancy from pre-characterized High (n = 5) and Low (n = 7) pregnant LG animals, we showed that in utero High LG female offspring are exposed to greater T levels than Low LG female offspring (Cameron et al., 2008a). We measured the anogenital distance (AGD) and used the anogenital index (AGD/weight: AGI) to assess differences between groups of fetuses and we observed an expected significant difference between sex (Mann–Whitney U = 686.5, p < 0.001). Males (1.02 + 0.01) had a greater AGI compared to female (0.77 + 0.02) offspring. An in utero environment effect (Mann–Whitney U = 207.0, p < 0.001) was also seen between the females, such that the offspring of High LG mother had a greater AGI than female offspring of Low LG mothers. These results demonstrate that High LG female offspring are masculinized during development via T exposure. The HPG axis is influenced by maternal care but also by in utero androgen exposure. In males, T production by the fetuses’ testes masculinize the brain and later sexual behavior (Phoenix et al., 1959). More studies are needed to identify the source of T in female fetus plasma, which could be synthesized by male siblings or produced by the mother, the placenta, or the female fetuses’ own adrenal glands. Wherever it comes from, this prenatal androgen exposure masculinizes the females and may protect the High LG female offspring from the effects of lower levels of maternal care, including an improvement in lordosis rating. To verify this hypothesis, studies such as embryo transplant or injection of pharmaceutical agents to control for the in utero environment will be needed. More studies are also needed to evaluate the effect of the maternal in utero environment on the epigenome. This is a clear example of the complexity of the research on epigenetic effects on behavior. The mechanisms controlling maternal programming of sexual behavior are not as clear as the one controlling ERα expression and maternal care. Thus, it is probable that gene–environment interactions are involved in this system.
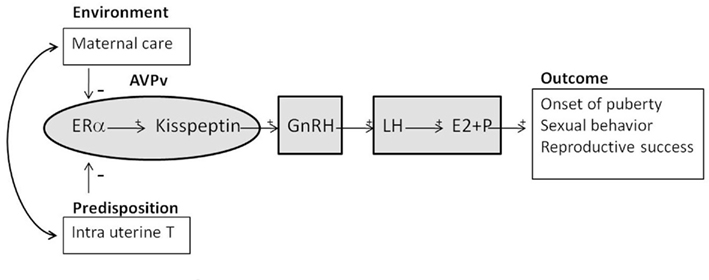
Figure 3. Schematic diagram showing the effects of early environment such as maternal care and predisposition such as masculinization caused by in utero T exposure on the hypothalamic–pituitary–gonadal (HPG) axis. Both can inhibit estrogen receptor α (ERα) and kisspeptin in the anteroventral-periventricular (AVPv) nucleus, which in turn modulate gonadotropin-releasing hormone (GnRH). GnRH is secreted from the hypothalamus and released into the median eminence; it then reaches the anterior pituitary where it stimulates the release of luteinizing hormone (LH). LH stimulates the release of the steroid hormones estrogen (E2) and progesterone (P) from the ovary. LH, E2 and P stimulate onset of puberty and improve sexual behavior and reproductive success.
Reproductive Strategies
So far, we have described variations in the phenotypes of female rats that are influenced by their mothers (Table 1). We have also described variations in the reproductive strategies used by female rats which are modulated by maternal care: female offspring that receive high levels of maternal care show a delayed pubertal onset, their HPG axis is dampened, and they are less receptive and motivated to mate, resulting in fewer pregnancies. As mothers, they display high levels of maternal care and they raise offspring that learn better and are less responsive to stress. The phenotype of female offspring raised under lower levels of maternal care is the opposite and is probably favoring the quantity and not the quality of offspring. So what are the advantages to rats raised with low levels of maternal care? What are the evolutionary benefits that maintain this wide variation in reproductive function and behavior?
Conclusion
Many parallels can be drawn between the effects of parental care in humans and maternal care in rats with regards to the reproductive system. In both cases the early environment provided by the parents influences the development of the progeny. The variation in behaviors that results from differences in parental care provides the species with variation in phenotypes. Differences in these phenotypes are adaptive, since both humans and rats may survive and thrive in various environments. Some of these environments may require a more rapid onset of reproduction because of a higher risk of mortality and may favor a phenotype that produces a greater number of offspring over offspring of a better quality. Unfortunately, parental influences on progeny are, to date, not entirely understood. However, as researchers gather more and more information about this system, it is becoming clear that, as in the rat, human’s parental programming of reproductive system is likely to involve gene–environment interactions.
Conflict of Interest Statement
The author declares that the research was conducted in the absence of any commercial or financial relationships that could be construed as a potential conflict of interest.
References
Belsky, J., Steinberg, L., Houts, R., Halpern-Felsher, B., and Network, N. E. C. C. R. (2010). The development of reproductive strategy in females: early maternal harshness –> earlier menarche –> increased sexual risk takingThe development of reproductive strategy in females: early maternal harshness –> earlier menarche –> increased sexual risk taking. Dev. Psychol. 46, 120–128.
Bifulco, A., Moran, P. M., Ball, C., Jacobs, C., Baines, R., Bunn, A., and Cavagin, J. (2002). Childhood adversity, parental vulnerability and disorder: examining inter-generational transmission of risk. J. Child Psychol. Psychiatry 43, 1075–1086.
Bjornstrom, L., and Sjoberg, M. (2005). Mechanisms of estrogen receptor signaling: convergence of genomic and nongenomic actions on target genes. Mol. Endocrinol. 19, 833–842.
Bredy, T. W., Humpartzoomian, R. A., Cain, D. P., and Meaney, M. J. (2003). Partial reversal of the effect of maternal care on cognitive function through environmental enrichment. Neuroscience 118, 571–576.
Brouette-Lahlou, I., Godinot, F., and Vernet-Maury, E. (1999). The mother rat’s vomeronasal organ is involved in detection of dodecyl propionate, the pup’s preputial gland pheromone. Physiol. Behav. 66, 427–436.
Caldji, C., Tannenbaum, B., Sharma, S., Francis, D., Plotsky, P. M., and Meaney, M. J. (1998). Maternal care during infancy regulates the development of neural systems mediating the expression of fearfulness in the rat. Proc. Natl. Acad. Sci. U.S.A. 95, 5335–5340.
Cameron, N. M., Del Corpo, A., Diorio, J., McAllister, K., Sharma, S., and Meaney, M. J. (2008a). Maternal programming of sexual behavior and hypothalamic-pituitary-gonadal function in the female rat. PLoS ONE 3, e2210. doi:10.1371/journal.pone.0002210
Cameron, N. M., Fish, E. W., and Meaney, M. J. (2008b). Maternal influences on the sexual behavior and reproductive success of the female rat. Horm. Behav. 54, 178–184.
Cameron, N. M., Shahrokh, D., Del Corpo, A., Dhir, S. K., Szyf, M., Champagne, F. A., and Meaney, M. J. (2008c). Epigenetic programming of phenotypic variations in reproductive strategies in the rat through maternal care. J. Neuroendocrinol. 20, 795–801.
Cameron, N. M., Soehngen, E., and Meaney, M. J. (2011). Variation in maternal care influences ventromedial hypothalamus activation in the rat. J. Neuroendocrinol. 23, 393–400.
Champagne, F. A., Francis, D. D., Mar, A., and Meaney, M. J. (2003a). Variation in maternal care in the rat as a mediating influence for the effects of environment on development. Physiol. Behav. 79, 359–371.
Champagne, F. A., Weaver, I. C. G., Diorio, J., Sharma, S., and Meaney, M. J. (2003b). Natural variations in maternal care are associated with estrogen receptor α expression and estrogen sensitivity in the medial preoptic area. Endocrinology 144, 4720–4724.
Champagne, F. A., and Meaney, M. J. (2006). Stress During gestation alters postpartum maternal care and the development of the offspring in a rodent model. Biol. Psychiatry 59, 1227–1235.
Champagne, F. A., Weaver, I. C. G., Diorio, J., Dymov, S., Szyf, M., and Meaney, M. J. (2006). Maternal care associated with methylation of the estrogen receptor-alpha1b promoter and estrogen receptor-alpha expression in the medial preoptic area of female offspring. Endocrinology 147, 2909–2915.
Chapillon, P., Patin, V., Roy, V., Vicent, A., and Caston, J. (2002). Prenatal stress does not alter innate novelty-seeking behavioral traits, but differentially affects individual differences in neuroendocrine stress responsivity. Dev. Psychobiol. 33, 162–177.
Clarkson, J., D’Anglemont de Tassigny, X., Colledge, W. H., Caraty, A., and Herbison, A. E. (2009). Distribution of kisspeptin neurones in the adult female mouse brain. J. Neuroendocrinol. 21, 673–682.
Clinton, S., Miller, S., Watson, S. J., and Akil, H. (2008). Prenatal stress does not alter innate novelty-seeking behavioral traits, but differentially affects individual differences in neuroendocrine stress responsivity. Psychoneuroendocrinology 33, 162–177.
Coall, D. A., and Chisholm, J. S. (2003). Evolutionary perspectives on pregnancy: maternal age at menarche and infant birth weight. Soc. Sci. Med. 57, 1771–1781.
Cooper, K. J., Fawcett, C. P., and McCann, S. M. (1974). Inhibitory and facilitatory effects of estradiol 17beta on pituitary responsiveness to a luteinizing hormone-follicle stimulating hormone releasing factor (LH-RF–FSH RF) preparation in the ovariectomized rat. Proc. Soc. Exp. Biol. Med. 145, 1422–1426.
De Bellis, M. D. (2002). Developmental traumatology: a contributory mechanism for alcohol and substance use disorders. Psychoneuroendocrinology 27, 155–170.
DeFries, J. C., Weir, M. W., and Hegmann, J. P. (1967). Differential effects of prenatal maternal stress on offspring behavior in mice as a function of genotype and stress. J. Comp. Physiol. Psychol. 63, 332–334.
Edmonds, S., Zoloth, S. R., and Adler, N. T. (1972). Storage of copulatory stimulation in the female rat. Physiol. Behav. 8, 161–164.
Erskine, M. (1985). Effects of paced coital stimulation on estrus duration in intact cycling rats and ovariectomized and ovariectomized-adrenalectomized hormone-primed rats. Behav. Neurosci. 99, 151–161.
Erskine, M. S. (1989). Solicitation behavior in the estrous female rat: a review. Horm. Behav. 23, 473–502.
Erskine, M. S., Lehmann, M. L., Cameron, N. M., and Polston, E. K. (2004). Co-regulation of female sexual behavior and pregnancy induction: an exploratory synthesis. Behav. Brain Res. 153, 295–315.
Fish, E., Shahrokh, D., Bagot, R., Caldji, C., Bredy, T., Szyf, M., and Meaney, M. (2004). Epigenetic programming of stress responses through variations in maternal care. Ann. N. Y. Acad. Sci. 1036, 167–180.
Fleming, A. S., and Corter, C. (1988). Factors influencing maternal responsiveness in humans: usefulness of an animal model. Psychoneuroendocrinology 13, 189–212.
Fleming, A. S., O’Day, D. H., and Kraemer, G. W. (1999). Neurobiology of mother-infant interactions: experience and central nervous system plasticity across development and generations. Neurosci. Biobehav. Rev. 23, 673–685.
Francis, D., Diorio, J., Lui, D., and Meaney, M. J. (1999a). Nongenomic transmission across generations of maternal behavior and stress responses in the rat. Science 286, 1155–1158.
Francis, D. D., Caldji, C., Champagne, F., Plotsky, P. M., and Meaney, M. J. (1999b). The role of corticotropin-releasing factor–norepinephrine systems in mediating the effects of early experience on the development of behavioral and endocrine responses to stress. Biol. Psychiatry 46, 1153–1166.
Francis, D. D., Diorio, J., Plotsky, P. M., and Meaney, M. J. (2002a). Environmental enrichment reverses the effects of maternal separation on stress reactivity. J. Neurosci. 22, 7840–7843.
Francis, D. D., Young, L. J., Meaney, M. J., and Insel, T. R. (2002b). Naturally occurring differences in maternal care are associated with the expression of oxytocin and vasopressin (V1a) receptors: gender differences. J. Neuroendocrinol. 14, 349–353.
Frasor, J., and Gibori, G. (2003). Prolactin regulation of estrogen receptor expression. Trends Endocrinol. Metab. 14, 118–123.
Gao, C., Abou-Nasr, R., and Norgren, R. B. Jr. (1996). Subpopulations of migrating neurons express different levels of LHRH in quail and chick embryos. Brain Res. Dev. Brain Res. 91, 237–244.
Georgescu, M., Sabongui, C., Del Corpo, A., Marsan, L., and Pfaus, J. G. (2009). Vaginocervical stimulation induces Fos in glutamate neurons in the ventromedial hypothalamus: attenuation by estrogen and progesterone. Horm. Behav. 56, 450–456.
Gonzales, A., Lovic, V., Ward, G. R., Wainwright, P. E., and Fleming, A. S. (2001). Intergenerational effects of complete maternal deprivation and replacement stimulation on maternal behavior and emotionality in female rats. Dev. Psychol. 38, 11–32.
Hardy, D. F., and DeBold, J. F. (1972). Effects of coital stimulation upon behavior of the female rat. J. Comp. Physiol. Psychol. 78, 449–458.
Heim, C., Newport, D. J., Heit, S., Graham, Y. P., Wilcox, M., Bonsall, R., Miller, A. H., and Nemeroff, C. B. (2000). Pituitary-adrenal and autonomic responses to stress in women after sexual and physical abuse in childhood. JAMA 284, 592–597.
Herbison, A. E., and Theodosis, D. T. (1992). Localization of oestrogen receptors in preoptic neurons containing neurotensin but not tyrosine hydroxylase, cholecystokinin or luteinizing hormone-releasing hormone in the male and female rat. Neuroscience 50, 283–298.
Hinde, R. A. (1986). “Some implications of evolutionary theory and comparative data for the study of human prosocial and aggressive behaviour,” in Development of Anti-Social and Prosocial Behaviour, eds D. Olweus J. Block, and M. Radke-Yarrow (Orlando: Academic Press), 13–32.
Huebner, A. J., and Howell, L. W. (2003). Examining the relationship between adolescent sexual risk-taking and perceptions of monitoring, communication, and parenting styles. J. Adolesc. Health 33, 71–78.
Lehmann, M. L., and Erskine, M. S. (2004). Induction of pseudopregnancy using artificial VCS: importance of lordosis intensity and prestimulus estrous cycle length. Horm. Behav. 45, 75–83.
Levine, S. (1967). Maternal and environmental influences on the adrenocortical response to stress in weanling rats. Science 156, 258–260.
Liu, D., Diorio, J., Day, J. C., Francis, D. D., and Meaney, M. J. (2000). Maternal care, hippocampal synaptogenesis and cognitive development in rats. Nat. Neurosci. 3, 799–806.
Liu, D., Diorio, J., Tannenbaum, B., Caldji, C., Francis, D., Freedman, A., Sharma, S., Pearson, D., Plotsky, P., and Meaney, M. (1997). Maternal care, hippocampal glucocorticoid receptors, and hypothalamic-pituitary-adrenal responses to stress. Science 277, 1620–1621.
Masur, J., and Struffaldi, G. (1974). Division of labor between rats: influence of differential social rearing conditions. Behav. Biol. 12, 233–241.
McClintock, M., and Adler, N. (1978). The role of the female during copulation in wild and domestic Norway rats (Rattus norvegicus). Behaviour 67, 67–96.
McClintock, M., and Anisko, J. (1982). Group mating among Norway rats: sex differences in the pattern and neuroendocrine consequences of copulation. Anim. Behav. 30, 398–409.
McCormack, K., Sanchez, M., Bardi, M., and Maestripieri, D. (2006). Maternal care patterns and behavioral development of rhesus macaque abused infants in the first 6 months of life. Dev. Psychol. 48, 537–550.
McLoyd, V., and Wilson, L. (1990). Maternal behavior, social support, and economic conditions as predictors of distress in children. New Dir. Child Dev. 46, 49–69.
McLoyd, V. C. (1990). The impact of economic hardship on black families and children: psychological distress, parenting, and socioemotional development. Child Dev. 61, 311–346.
Meschke, L. L., and Silbereisen, R. K. (1997). The influence of puberty, family processes, and leisure activities on the timing of first sexual experience. J. Adolesc. 20, 403–418.
Moore, C. L., and Power, K. L. (1992). Variation in maternal care and individual differences in play, exploration, and grooming of juvenile Norway rat offspring. Dev. Psychol. 25, 165–182.
Ojeda, S. R., and McCann, S. M. (1978). Control of LH and FSH release by LHRH: influence of putative neurotransmitters. Clin. Obstet. Gynaecol. 5, 283–303.
Österlund, M. K., and Hurd, Y. L. (2001). Estrogen receptors in the human forebrain and the relation to neuropsychiatric disorders. Prog. Neurobiol. 64, 251–267.
Phoenix, C. H., Goy, R. W., Gerall, A. A., and Young, W. C. (1959). Organization action of prenatally administered testosterone propionate on the tissues madiating mating behavior in the female guinea pig. Endocrinology 65, 369–382.
Rhen, T., and Crews, D. (2002). Variation in reproductive behaviour within a sex: neural systems and endocrine activation. J. Neuroendocrinol. 14, 517–531.
Schibler, U., and Sierra, F. (1987). Alternative promoters in developmental gene expression. Annu. Rev. Genet. 21, 237–257.
Seymour, L. (2005). Developmental determinants of sensitivity and resistance to stress. Psychoneuroendocrinology 30, 939–946.
Shughrue, P. J., Lane, M. V., and Merchenthaler, I. (1997). Comparative distribution of estrogen receptor-alpha and -beta mRNA in the rat central nervous system. J. Comp. Neurol. 388, 507–525.
Suomi, S. (1978). Maternal behavior by socially incompetent monkeys: neglect and abuse of offspring. J. Pediatr. Psychol. 3, 28–34.
Terasawa, E., Kurian, J. R., Guerriero, K. A., Kenealy, B. P., Hutz, E. D., and Keen, K. L. (2010). Recent discoveries on the control of gonadotrophin-releasing hormone neurones in nonhuman primates. J. Neuroendocrinol. 22, 630–638.
Tu, M. T., Lupien, S. J., and Walker, C.-D. (2005). Measuring stress responses in postpartum mothers: perspectives from studies in human and animal populations. Stress 8, 19–34.
van Hasselt, F. N., Cornelisse, S., Yuan Zhang, T., Meaney, M. J., Velzing, E. H., Krugers, H. J., and Joëls, M. (2011). Adult hippocampal glucocorticoid receptor expression and dentate synaptic plasticity correlate with maternal care received by individuals early in life. Hippocampus. doi:10.1002/hipo.20892. [Epub ahead of print].
Walf, A. A., Ciriza, I., Garcia-Segura, L. M., and Frye, C. A. (2007). Antisense oligodeoxynucleotides for estrogen receptor-[beta] and [alpha] attenuate estradiol’s modulation of affective and sexual behavior, respectively. Neuropsychopharmacology 33, 431–440.
Weaver, I. C., Diorio, J., Seckl, J. R., Szyf, M., and Meaney, M. J. (2004a). Early environmental regulation of hippocampal glucocorticoid receptor gene expression: characterization of intracellular mediators and potential genomic target sites. Ann. N. Y. Acad. Sci. 1024, 182–212.
Weaver, I. C. G., Cervoni, N., Champagne, F. A., D’Alessio, A. C., Sharma, S., Seckl, J. R., Dymov, S., Szyf, M., and Meaney, M. J. (2004b). Epigenetic programming by maternal behavior. Nat. Neurosci. 7, 847–854.
Weaver, I. C. G., Champagne, F. A., Brown, S. E., Dymov, S., Sharma, S., Meaney, M. J., and Szyf, M. (2005). Reversal of maternal programming of stress responses in adult offspring through methyl supplementation: altering epigenetic marking later in life. J. Neurosci. 25, 11045–11054.
Widom, C. S., and Maxfield, M. G. (1996). A prospective examination of risk for violence among abused and neglected childrena. Ann. N. Y. Acad. Sci. 794, 224–237.
Wray, S., and Hoffman, G. E. (1986). A developmental study of the quantitative distribution of LHRH neurons within the central nervous system of postnatal male and female rats. J. Comp. Neurol. 252, 522–531.
Keywords: maternal care, sexual behavior, reproductive strategies, estrogen receptor alpha, GnRH, masculinization
Citation: Cameron NM (2011) Maternal programming of reproductive function and behavior in the female rat. Front. Evol. Neurosci. 3:10. doi: 10.3389/fnevo.2011.00010
Received: 06 September 2011; Paper pending published: 29 September 2011;
Accepted: 02 December 2011; Published online: 22 December 2011.
Edited by:
Melanie L. Shoup-Knox, University of Pittsburgh, USAReviewed by:
Jeffrey Bedwell, University of Central Florida, USAPaul M. Nealen, Indiana University of Pennsylvania, USA
Copyright: © 2011 Cameron. This is an open-access article distributed under the terms of the Creative Commons Attribution Non Commercial License, which permits non-commercial use, distribution, and reproduction in other forums, provided the original authors and source are credited.
*Correspondence: Nicole M. Cameron, Psychology and Biology Departments, Binghamton University – State University of New York, PO Box 6000, Binghamton, NY 13902-6000, USA. e-mail:bmNhbWVyb25AYmluZ2hhbXRvbi5lZHU=